- 1Chemistry Department, Faculty of Science, Cairo University, Giza, Egypt
- 2Department of Environmental Biotechnology, Genetic Engineering and Biotechnology Research Institute, University of Sadat City, Sadat, Egypt
- 3Department of Chemistry, College of Science, University of Jeddah, Jeddah, Saudi Arabia
- 4Chemistry Department, Faculty of Science (Girls), Al-Azhar University, Nasr, Cairo, Egypt
- 5Department of Molecular Diagnostics and Therapeutics, Genetic Engineering and Biotechnology Research Institute, University of Sadat City, Sadat, Egypt
- 6Chemistry Department, Faculty of Science, New Valley University, El-Kharga, Egypt
The main objective of this study is to synthesize and characterize of a new three complexes of Pd (II), Cu (II), and Cu (I) metal ions with novel ligand ((Z)-2-(phenylamino)-N'-(thiophen-2-ylmethylene)acetohydrazide) H2LB. The structural composition of new compounds was assessed using several analytical techniques including FT-IR, 1H-NMR, electronic spectra, powder X-ray diffraction, and thermal behavior analysis. The Gaussian09 program employed the Density Functional Theory (DFT) approach to optimize the geometry of all synthesized compounds, therefore obtaining the most favorable structures and crucial parameters. An investigation was conducted to examine the impact of γ-irradiation on ligands and complexes. Before and after γ-irradiation, the antimicrobial efficiency was investigated for the activity of ligands and their chelates. The Cu(I) complex demonstrated enhanced antibacterial activity after irradiation, as well as other standard medications such as ampicillin and gentamicin. Similarly, the Cu(I) complex exhibited superior activity against antifungal species relative to the standard drug Nystatin. The docking investigation utilized the target location of the topoisomerase enzyme (2xct) chain A.
1 Introduction
Hydrazone derivatives are a large class of chemicals used in various medicinal applications (Abdalla et al., 2021) because of their broad range of pharmacokinetic features (Popiołek et al., 2018; Aneja et al., 2019; El-saied et al., 2020; Katariya et al., 2020), particularly in drug detection programmers. The hydrazone and carbaldehyde derivatives, as well as their complexes, have been reported to reveal a wide range of biological features (Sepay and Dey, 2014; Parveen et al., 2018; Naveen et al., 2020; Zülfikaroğlu et al., 2020), such as anticancer (Mandewale et al., 2017; Manohar et al., 2018; Yousefi et al., 2019; Babahan et al., 2020), antibacterial (Ekennia et al., 2018; Özbek et al., 2019; Khan et al., 2020), antimicrobial (Cao et al., 2018; Philip et al., 2018; Santiago et al., 2020), antifungal (Rocha et al., 2019; Elsayed et al., 2020), antimalarial (Maurya et al., 2017), antiviral (Sreepriya et al., 2020), antimycobacterial (Mandewale et al., 2017; Mandewale et al., 2018), antileishmanial (Coimbra et al., 2019), antiplatelet (Margariti et al., 2020), anti-analgesic antitubercular, anticonvulsant (Dehestani et al., 2018), anti-uropathogenic (Alodeani et al., 2015), antiproliferative (Bergamini et al., 2019), antiarthritic (Shabbir et al., 2014), and antioxidant properties (Vanucci-Bacqué et al., 2016; Anastassova et al., 2018; Al-Hazmi et al., 2020); potent immunomodulatory agents (Meira et al., 2018); and potent antiangiogenic agents in atherosclerosis (Vanucci-Bacqué et al., 2016). They are also essential in Alzheimer’s disease treatment (Haghighijoo et al., 2017; Parlar et al., 2019).
Garoufis et al. evaluated several scholarly studies on the antibacterial, antiviral, and antifungal properties of Pd II) chelates with diverse ligands (Other ligands include nitrogen and sulfur donor sites, Schiff base ligands, and other medications) (Guerra et al., 2005; Garoufis et al., 2009). Other studies have recently been published in the literature that revealed diverse palladium complex activity intensities on distinct bacteria and fungus species (Aghatabay et al., 2007; Biyala et al., 2008; Vieira et al., 2009).
Copper is the primary component of copper doorknobs and touch surfaces in hospitals and healthcare facilities that prevent bacteria and diseases from growing and spreading (“contact killing") (Tian et al., 2012). Many copper complexes with potential and diverse biological action have been discovered in the literature, including antibacterial (Beeton et al., 2014; Lobana et al., 2014), anticancer (Qiu et al., 2015; Stefani et al., 2015), anticonvulsant (Lemoine et al., 2002), antifungal (Soroceanu et al., 2016), anti-inflammatory (Hoonur et al., 2010), antimalarial (Hubin et al., 2014), anti-neurodegenerative (Quintanova et al., 2015), antiobesity (Perontsis et al., 2016), antioxidant (Tolia et al., 2013), anti-rheumatic (Sherif and Hosny, 2014), antithyroid (Urquiza et al., 2015), antitubercular (Netalkar et al., 2014), and antiviral activity (Dorotíková et al., 2015).
Radiation is widely used in biomaterials science to alter surface properties, clean surfaces, and improve bulk properties. In addition to biochips and situ photopolymerizable bioglues, radiation is used to develop biochips (Aly and Elembaby, 2020b). Gamma radiation, great-energy electrons, and ultraviolet radiation are the most common energy sources used to irradiate biomaterials (Balashova et al., 2019; Aly et al., 2022a; Elganzory et al., 2022). The manuscript aimed to prepare, characterize, and investigate the impact of gamma irradiation on Pd(II), Cu(II), and Cu(I) metal complexes and also an antimicrobial study of these compounds.
2 Experimental method
2.1 Synthesis of hydrazone ligand
First, 20 mL of ultra-grade ethanol was added to a round flask along with 0.01 mol of 2-(p-tolylamino)acetohydrazide and 0.01 mol of 5-hydroxy-4-oxo-4H-pyran-3-carbaldehyde. The resultant liquid was mixed for approximately 6 h at room temperature (Abdalla et al., 2021). The resulting precipitate was separated after washing and drying into a filter paper. The washing solutions were ethyl alc and diethyl ether (Aly et al., 2021).
C15H15N3O4 (H2LB): Yellow, Molecular Weight: 301.3, Yield = 94%; Analytical Calculated: H, 5.02; C, 59.80; N, 13.95. Exp. (%):H, 4.98; C, 59.76; N, 13.92. The FTIR bands (cm-1) at 3,392, 3,202, 1,677, 1,639, and 1,604 related to (OH/H2O), (N-H), (C=O)side, and (C=N) respectively. Electronic transitions: λmax: 341, 399. 1HNMR: δ (ppm): 2.10, 3.91, 5.84–6.63, 7.11, 11.10, and 15.90 were related to (s, 3H, CH3), (s, 2H, NCH2), (s, 1H, NH), (s, 1H, NCH), (m, 4H, p-sub. Ph-H), (s, 2H, pyran-H), (bs, 1H, NHC = O) and (bs, 1H, OH) respectively. 13CNMR: δ (ppm): 19.2, 45.3, 148.2,(116.7, 128.7, 163.1, 169.1, 170.5), (112.4, 116.4, 128.8, 148.1) and 181.5 corresponded to (CH3), (NCH2), (C=N), (pyran-C), (Ph-C) and (C=O) respectively. Supplementary Figure S2.
2.2 Synthesis of metal complexes
According to a standard approach (Scheme 1), the metal salt was added to the ligand with a stoichiometric quantity (1 mmol; = 0.177 g Pd II); 0.099 g Cu(I); 0.223 g Cu(II) to 1 mmol = 0.301 of ligand). The solvent used was EtOH (20 mL). The reaction mixture was refluxed for 6 hours while stirring at 60°C. The colorful reaction product that resulted from the reaction was filtered out of the reaction mixture, extensively cleaned with ethyl alc to eliminate the unreacted starting residues, and vacuum-dried (Frisch et al., 2009a). TLC was used to verify the complexes' purity.
2.3 Analytical and physical measurements
All of the starting materials used in this investigation (which were of analytical quality and were not purified before use) were provided by Sigma-Aldrich and Fluka. Detailed information on the tools and procedures used for structure confirmation and application are provided in the Supplementary Material.
2.4 Quantum chemical calculation QCC
GaussView 5.0.8 (Wallingford, CT 2009) (Frisch et al., 2009a) was used to prepare the input files of compounds. Gaussian 09 rev. A.02 was used for all computations (Wallingford, CT, 2009) (Frisch et al., 2009b). The DFT/B3LYP technique was used. The standard basis sets were 6311G and LANL2DZ concerning the synthesized H2L and its metal complexes, respectively (Ammar et al., 2014; Maurya et al., 2015; Ding et al., 2018; Abdalrazaq et al., 2022; Aly et al., 2022b; Mahani and Mohammadi, 2022; Ugurlu and Harmankaya, 2022). The topoisomerase IIa was simulated using MOE 2009 (Molecular Operating Environment) software. The topoisomerase II DNA gyrase protein crystal structure (PDB ID: 2XCT) was downloaded from the protein data bank. The docking steps involved constructing the investigated compounds using Chembio3D-ultra software followed by their optimization. The selected protein was downloaded from Protein Data Bank PDB. Finally, the binding affinity of each compound to the protein was evaluated.
2.5 Assay for antimicrobials
The antibacterial activity of the generated compounds was evaluated using the agar well diffusion method as reported in detail (Abdalla et al., 2020; Al-Farhan et al., 2021) and specifics of the antimicrobial screening process are provided in the Supplementary Material.
2.6 Irradiation studies
Solid ligand and chelate complex samples were submitted to irradiation tests at a dosage of 60 kGy (Aly and Elembaby, 2020a) Supplementary Section S3.
3 Result and discussion
3.1 Characterization
The chelates' analytical results confirmed the predicted chemical formulae and demonstrated the formation of 1:2 of Pd(II) and 1:1 of Cu(II) and Cu(I) (M:L) (Table 1). The molar conductance values were measured in 10−3 M DMF for the Cu (II), Cu(I), and Pd(II) complexes to give 25, 30, and 65 Ω-1cm2mol-1 respectively. This result revealed that the Cu(II) and Cu(I) chelates were non-electrolytic (Aly and Fathalla, 2020); on the other hand, the Pd(II) complex was electrolytic.
3.2 1H- NMR interpretation of the non-irradiated and radiated ligands
(H2L) 1H-NMR spectra in DMSO-d6 (defining each ligand before and after irradiation) were confirmed. After radiation exposure, the signals stayed in the same spot or slightly moved in the ligand’s 1H-NMR spectrum (Supplementary Figure S1). Even yet, the bands' strength after irradiation was greater than before. The 1H-NMR spectrum of the NH proton had a singlet signal at 5.94 ppm and a wide single peak at 11.1 ppm that identified the NHC = O proton in the hydrazone linkage. The spectral signature of aromatic ring protons also included a multiple signal between 6.70 and 6.75 ppm. The spectrum also exhibited singlet signals at 7.11 ppm, which were pyrene proton signals, and a wide single peak at 15.90 ppm, a hydroxyl group C-OH proton signal.
3.3 FT-IR spectra
Table 2 and Supplementary Figures S3-S6 clarify the IR spectra of the irradiated (A) and non-irradiated (B) states of H2L and its metal chelates. The IR spectra of H2LB and H2LA have revealed bands at 3,392, 3,391; 1,677, 1,675; 1,639, 1,635; and 1,604, 1,600 cm-1 which corresponded to the Hydroxyl group, ν(carbonyl) side, ring, and ν(C=N) for the non-irradiated and irradiated states respectively. Upon complexation, these bands were shifted to a higher or lower value where the band related to ν(OH) was shifted to higher values as in the Pd(II), Cu(II), and Cu(I) chelates exhibited at 3,435, 3,434; 3,440, 3,490 and 3,490, 3,487 cm-1, respectively. The ν (C=N) bands were changed at higher frequency after irradiation and were displayed at 1,545, 1,547; 1,550, 1,548; and 1,550 cm-1, respectively. The bands exhibited at 1,687, 1,685; 1,696, 1,698; and 1,695, 1,693 cm-1 related to ν(OH) and ν(C=O)side respectively were changed to a higher frequency, while the bands of ν(C=O)ring and ν(C=N) were changed to a lower frequency. New signals were seen in the region at 603, 600; 537, 536; and 537, 579 cm-1 related to ν (M-Oxygen) and ν(M-Nitrogen) were seen in the region at 539, 545; 485, 479; and 508, 513 cm-1 respectively (Elganzory et al., 2022). The infrared spectra for irradiated H2L and chelates revealed changes in the size and intensity of the bands with the action of irradiation in all compounds (Abdalla et al., 2021). Supplementary Figure S7 shows the theoretical FT-IR spectra powder pattern of ligand and its complexes.
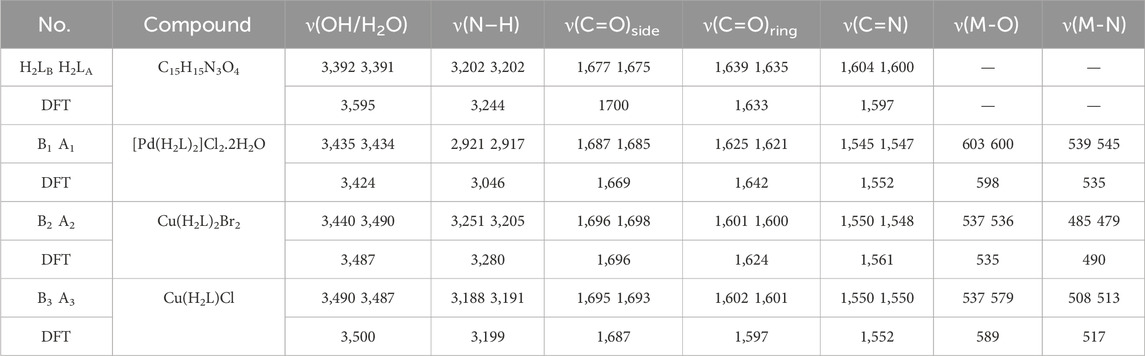
TABLE 2. FT- IR spectral bands all compounds before (B) and after (A) irradiation in 4,000–400 cm-1, A = After irradiation and B = Before irradiation.
3.4 Mass spectra
Pd(H2L)2]Cl2.2H2O, Cu(H2L)2Br2, and Cu(H2L)Cl showed molecular ion peaks at m/z 815.11 (21%), 825.42 (12%), and 399.62 (32%), These findings were supported by the proposed molecular formulae that have been provided (calc. 815.95, 825.96, and 400.30 amu), respectively (Supplementary Figures S8-S10).
The mass fragmentation patterns of complexes are shown in Scheme S1-S3, where the mass spectra’s multi-peaks pattern resulted in a sequence of peaks corresponding to the distinct fragments.
3.5 Electronic spectra
Table 3 and Supplementary Figures S11, S12 show all compounds' UV–Vis spectra before and after irradiation at room temperature in a 10−3 DMF solution in the 200–800 nm range. In the UV range, the absorption spectra of H2LB and H2LA showed two absorption bands (Liu et al., 2013); the first bands were seen at λmax = 341, 335 nm related to π–π* transition, and the second transitions were seen at λmax = 399, 394 nm assigned to n–π* transition. The Pd(II) chelates (B1 and A1) explored bands at 332; 326, 393; 389 and 511; 502 nm, assigned to 1A1g → 1B1g transition representing a square planar geometry (El-Boraey and El-Gammal, 2015; Shankarwar et al., 2015). The electronic spectra of Cu II) complexes (B2 and A2) revealed bands at 352, 346; 399, 394; and 571, 563 nm, pointing to n–π* and d-d transition representing octahedral geometry. Moreover, Cu(I) chelates(B3 and A3) before and after irradiation revealed four bands at 343, 331; 399, 397; 487, 469; and 615, 595 nm, respectively, pointing to n–π* and d-d transition representing tetrahedral geometry. The difference between the electronic transitions of the H2L and all chelates was noted after irradiation as the λmax position and the absorbance value (Abdalla et al., 2021).
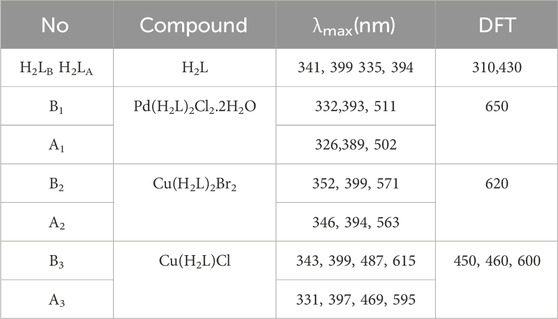
TABLE 3. Electronic spectra data of all compounds before (H2LB, B1- B3) and after (H2LA, A1- A3) irradiation.
3.6 X-ray diffraction
PXRD was used when the single crystals of the produced chelates failed to grow. Before and after irradiation, powder diffraction patterns of ligands (H2LB, H2LA) and Cu(II) complexes (B2) and (A2) were recorded across 2θ = 5°–80° (Table 4; Figure 1, Supplementary Figure S13). The greatest intensity peak was also identified, along with its location, half-maximum width, and d spacing. The reflection in the H2L diffractogram peaks was shown at 2θ = 15.822° and 15.801, which corresponded to d values of 0.559119 and 0.558271 nm, respectively. Coordination compounds have been generated because the powder XRD patterns of the ligands (H2LB, H2LA) and the complexes (B2 and A2) were completely different. The patterns exhibited well-characterized crystalline peaks, indicating that the ligand was in crystallized form in the (B2 and A2) and (H2LB, H2LA) complexes. The average particle sizes of (H2LB, H2LA) and (B2 and A2) chelates were calculated using the Scherer equation (Muniz et al., 2016; Abdalla et al., 2020; Abdel Rahman et al., 2022; Abdel-Rahman et al., 2022) and were 37.48; 31.99 and 51.64; 40.25 nm, respectively. The crystal size variation might be due to the Nano range caused by the irradiation.
3.7 Thermal studies
The thermal TGA of all compounds before (H2LB, B1-B3) and after (H2LA, A1-A3) irradiation were investigated with a heating range from (20°C–800°C). Table 5 and Supplementary Figure S14.
3.7.1 Pd(II) chelates under the two irradiated and unirradiated cases
The TGA curves of chelates (B1 and A1) (Supplementary Figure S14) showed three steps for losing weight (Calc./Exp.%: 13.35/13.27 and 13.35/13.32), assigned to the release of two hydrated water molecules and two hydrogen chloride molecules within the heat at 45–225°C. The second step showed the appearance of the decomposition within the heat from 225 to 386°C with losing weight (Calc/Exp.%: 71.64/71.59 and 71.64/71.61) and corresponded to C30H28N6O7 leaving PdO as a residue (Aly and El-Boraey, 2019).
3.7.2 Cu(II) chelates under the two irradiated and unirradiated cases
The TGA curves of the chelates (B2 and A2) were four steps apart (Supplementary Figure S14), where weight loss was shown in the temperature ranges of 30–210 and 30–191°C (Calc./Found percent: 19.59/19.53 and 9.79/9.76) which agreed to the loss of two and one molecules of hydrogen bromide. The decomposition of (B2 and A2) was within the heating range 210–305 and 191°C–461°C with weight loss (Calc./Exp.%: 23.03/23.13 and Calc./Found%: 34.88/34.91), which corresponded to the dissociation of C10H12N3O and C10H14N3O2Br. The third step in the range 305–591 and 461°C–610 °C indicated the removal of C20H16N3O6 and C20H14N3O6 with weight loss (Calc./Found%: 47.74/47.71 and 47.50/47.53). The final step over 591°C and 610 °C indicated the removal of CuO and Cu as the final remainder from unirradiated (B2) and irradiated Cu(II) complexes(A2), respectively (El-Boraey and Mansour, 2018).
3.7.3 Cu(I) chelates under the two irradiated and unirradiated cases
The TGA curves of chelates (B3 and A3) (Supplementary Figure S14) showed similar four steps within the heat range 100–19°C, which estimated the loss of hydrogen chloride molecule with mass loss (Calc./Found%: 9.12/9.09). The second step showed the mass loss (Calc./Found%: 38.25/38.21) in a temperature range of 198–495°C, corresponding to the losses of C6H5N2O3. The decomposition stage of (B3 and A3) through temperature range 495–571°C providing mass loss (Calc./Exp.%: 36.76/36.83) indicated the removal of C9H9NO, leaving copper metal as final residue. Finally, the thermal stability of the irradiated complexes using gamma rays was more thermally stable than the unirradiated complexes (Abdalla et al., 2021).
3.8 Molecular structure
When doing quantum chemistry research, the LUMO (p acceptor) and HOMO (p donor) molecular structures of H2LB are important considerations. Frontier molecular orbitals (FMOs) are the name given to these molecular orbitals. In HOMO and LUMO (Figure 2), the molecular structures of H2LB and its complexes were shown. The energies (EHOMO, ELUMO) of H2LB and its complexes are tabulated in Table 6. Both the EHOMO and ELUMO had negative values, indicating the stability. (ΔE = ELUMO - EHOMO) was the energy band gap that related to the charge transfer interface within the LUMO-HOMO of the molecule as specified in Table 6. The equations for dipole moment D), hardness η), softness σ), chemical potential μ), and electronegativity χ) have been obtained and the corresponding results are shown in Table 6.
Where I = the ionization potential of the molecule.
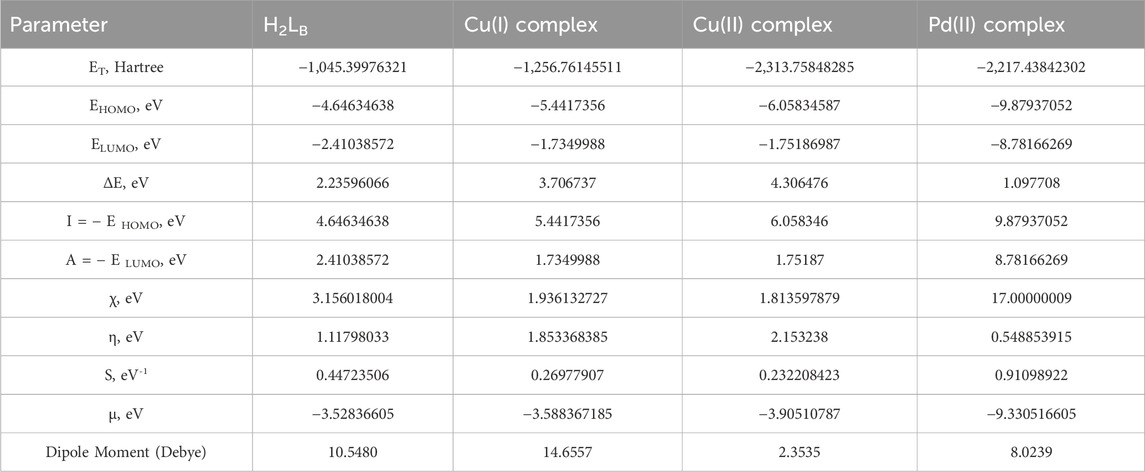
TABLE 6. Ground state criteria of ligand and Cu(I), Cu(II), and Pd(II) complexes using B3LYP/6-311G and B3LYP/LANL2DZ, respectively.
A = electron affinity of the molecule.
Hardness and softness, frequently used as chemical reactivity and stability indicators, were crucial parameters.
The molecule had a narrow gap between the HOMO and LUMO orbitals and became more reactive and softer, as smaller hardness ratings suggested higher reactivity. The order of softness was Pd > Ligand > Cu(I) > Cu(II). Except for the Pd(II) complex, every complex had a greater energy gap than the ligand. Therefore, the stability of most complexes under investigation was higher than the free ligand. The greater reactivity of the Pd II) complex may be explained by the higher polarizability of the second-row transition elements (Palladium) in comparison to the first-row elements (Copper).
The atom ordering of H2LB was given in the molecular structure, and complexes were also presented (Figure 3). The following observations were based on the calculated bond lengths and orientations directed at the compounds (Table 7).
In the complexes some bond lengths were increased [(C3-C4), (N7-C8), (C14-O16), (N6-N7) and (C4-O5)] and others were decreased [(C8-C9) and (C9-C14)] to adjust the coordination via the N7,O5, and O16-atoms in both Cu(I) and Pd(II) complexes with the emergence of new O16-M, (N7-M), and (O5-M) bonds. In the case of the Cu (II) complex, the coordination was carried out through N7 and O5 atoms comp, leaving the coordination sphere via bromide bonding. As seen in Table 7, coordination modified the H2LB bond angles, and when the metal center was changed, significant changes in the angles surrounding the metal also occurred. The significant swing in angle values due to bonding during complex formation was either increased or decreased. N7, O5, and O16 atoms in the ligand calculated natural charges of −0.197, −0.583, and −0.589 respectively as seen in Supplementary Table S1. Charge transfer from L(ligand)→M(metal) could be supported by decreased calculated charges on metal ions after coordination (Hassan and Gomha, 2019). The charges changed from Cu (I), Cu (II), and Pd (II) to 0.503, 0.0255, and 0.488 respectively. The theoretical results of FTIR showed good agreement with the experimental results as seen in Table 2 and Supplementary Figures S3-S6. The theoretical infrared spectra of the ligand L showed noteworthy absorption regions at 344, 1700, 1,633, and 1,597 cm-1 corresponding to v N–H), (C=O)side, (C=O)ring, and (C=N) vibrations. However, when these vibrations were complexed with metal ions, their positions shifted from their initial values. Also, the electronic transitions of ligands and complexes in the presence of solvent effect were calculated and the transition values are tabulated in Table 3.
3.9 Antimicrobial study
The antibacterial properties of the H2L and its chelates were tested against different Gram-positive and negative strains of bacteria both before and after exposure to gamma irradiation. Additionally, it had antifungal effects against Candida albicans and Aspergillus Nigar. The conventional antimicrobial agents used for antibacterial and antifungal research purposes included ampicillin, gentamicin, and nystatin.
The efficacy of the antibacterial properties of all produced compounds is demonstrated in Supplementary Table S2 and Figure 4 and Figure 5. The findings suggested that complexes exhibited more activity compared to ligands. Furthermore, following irradiation, complexes demonstrated enhanced efficacy as antibacterial and antifungal agents, surpassing their pre-irradiation performance (Aly and Elembaby, 2020a; Abdalla et al., 2021). The increased activity of complexes may be elucidated by Overtone’s notion (Anjaneyulu and Rao, 1986), as evidenced in our prior studies (Khalf-Alla et al., 2019; Hassan and Khalf-Alla, 2020; Hassan and Mohamed, 2020). The reduction in polarity of the metal ion occurred as a result of chelation, due to the interaction between its partial positive charge and the donation sites of the coordinated ligand. Additionally, chelation promoted the dispersion of π-electrons throughout the whole chelate ring, hence enhancing the lipophilic nature of the chemical under investigation. The compound’s lipophilic nature facilitated its penetration through the lipid layer of the cell membrane, resulting in a more potent and destructive effect on the cells. Moreover, the Cu(I) complex exhibited enhanced antibacterial activity after irradiation (A3), surpassing the antibacterial activity of the Cu(I) complex before irradiation (B3) against bacterial species compared to other substances, including standard drugs like ampicillin and gentamicin. Similarly, when nystatin was used as a standard drug, the Cu(I) complex also displayed superior antifungal activity.
3.10 Evaluation of docking studies
A nitrogen-containing heterocyclic molecule is an essential scaffold with antibacterial potential. Therefore, our research aimed to synthesize and investigate new nitrogen-containing heterocyclic compounds experimentally in vitro against different bacterial strains and theoretically using MOE 2008 software (Hassan and Mohamed, 2020). A molecular docking study was performed with the target site of topoisomerase enzyme(2xct) chain a from the protein data bank. The topoisomerase enzyme is a crucial enzyme that plays a vital role in the process of DNA replication (Redgrave et al., 2014). The gyrase enzyme facilitates the relaxing of super-coiled DNA during DNA replication by breaking and rejoining both strands of the DNA chain, allowing for unwinding and replication (Champoux, 2001).
There was a good correlation between the in vitro antimicrobial inhibition assay and the scoring energy values, as shown in Table 8 and Supplementary Table S3. The Palladium (II) and Copper (1) complexes revealed effective results against most microbes. The explored binding affinity was the best-posed interaction with low root mean square deviation values (RMSD). Figure 6 displays the different binding interaction types of compounds with 2xct protein. The Copper(I) complex showed sidechain acceptor and sidechain donor interaction types with Asp-508 and His1081 amino acid residues, respectively. The reactivity sequence relative to the scoring energy values was Cu(I) > Pd(II) > Cu(II) > L, which showed good fitting with the zone inhibition values experimentally where the Cu(I) complex inhibited bacterial growth in both Gram-positive and Gram-negative bacteria, with zone inhibition values that were greater than those of the Pd(II), Cu(II), and ligand compounds. The binding affinity of our compounds consistently demonstrated superior or equivalent values in multiple prior studies targeting the same protein type (Pisano et al., 2019; El-Etrawy and Sherbiny, 2021). Paraphrased, (Several compounds were examined before against the same 2xct protein and observed high affinity to inhibit the examined protein with scoring energies − 9.41 relative to Cu(II) chelate) (Aly et al., 2023). Our new Cu(I) chelate observed a similar affinity to the 2xct protein in the present paper. Also, our compounds achieved a 2xct protein inhibition effect in the docking scoring range (−3.63 to −8.51) observed for the quinolone moiety-based compounds (Patel and Patel, 2014).
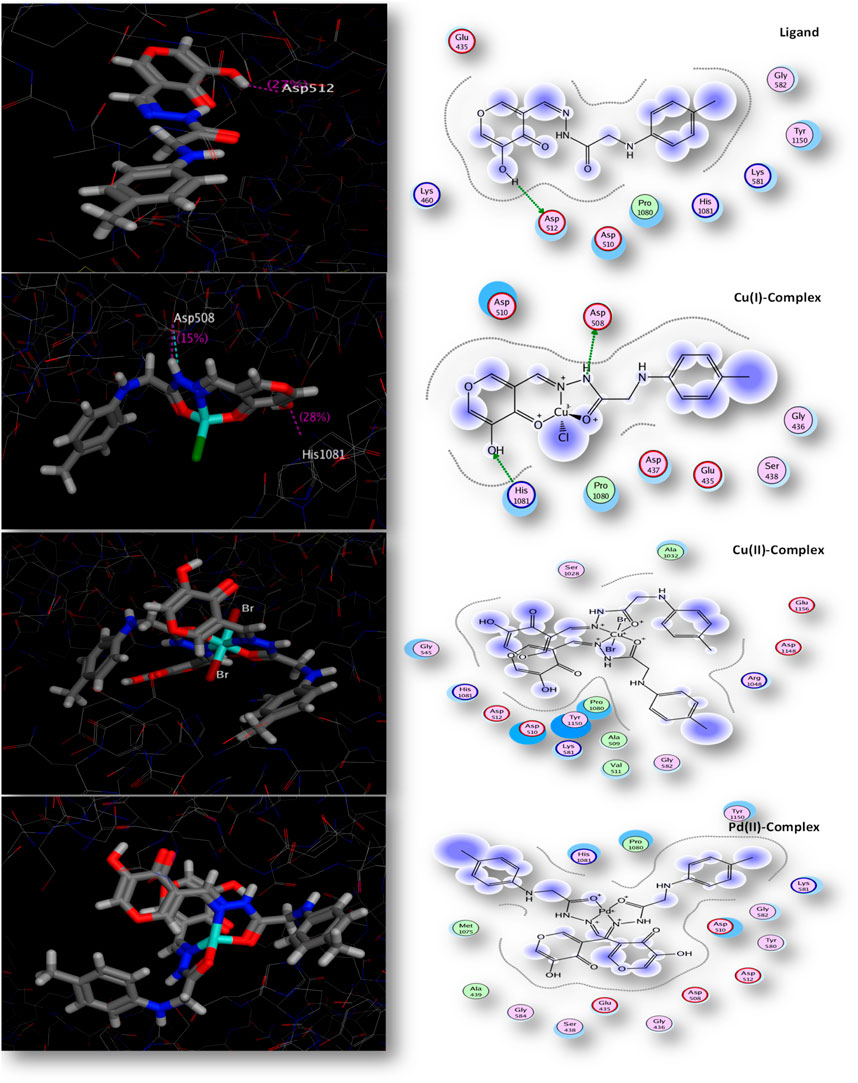
FIGURE 6. 2D and 3D interaction ability of all compounds with topoisomerase IIa enzyme (code: 2xct).
4 Conclusion
Based on a novel (H2LB) ligand (Z)-2-(phenylamino)-N'-(thiophen-2-ylmethylene) acetohydrazide), three new chelates, Pd(II), Cu(II), and Cu(I), have been isolated. Based on the outcomes of various characterization approaches, the hypothesized structures of the (H2LB) and its chelates verified the production of 1:2 Pd(II) and Cu(II) and 1:1 (M:L) Cu(I) chelates. The molar conductance values of the chelates demonstrated their non-electrolytic character except for the ionic Pd(II) Complex. The effectiveness of the antibacterial and antifungal treatments was compared to the industry-standard medications ampicillin, gentamicin, and nystatin. Paraphrased, (Zone inhibition values revealed that the Cu(I) complex after irradiation (A3) acquired better antibacterial activity followed by the Cu(I) complex before irradiation (B3) relative to bacterial species than others, when ampicillin and gentamicin as reference drugs, as well as the antifungal species when nystatin was used as a standard drug). The antimicrobial activity of these complexes followed the order: Cu(I) complex > Pd(II) complex > Cu(II) complex > Ligand. The geometries of the Pd(II) complex were square planar, according to the DFT calculations performed on the synthesized compounds. Cu(II) was octahedral, with hex coordinates chosen around the metal ions, while Cu(I) complex had a tetrahedral geometry. The synthesized ligand and the range of antibacterial activity of all the metal complexes against bacterial species were satisfactory. The docking stimulation revealed all of the chemicals' binding models.
Data availability statement
The original contributions presented in the study are included in the article/Supplementary Material, further inquiries can be directed to the corresponding authors.
Author contributions
SH: Data curation, Formal Analysis, Investigation, Methodology, Software, Writing–original draft. SA: Conceptualization, Data curation, Methodology, Supervision, Visualization, Writing–review and editing. AA-S: Data curation, Formal Analysis, Investigation, Resources, Validation, Writing–review and editing. SA: Data curation, Formal Analysis, Resources, Writing–original draft. MS: Conceptualization, Data curation, Formal Analysis, Investigation, Resources, Writing–review and editing. GN: Data curation, Formal Analysis, Investigation, Resources, Writing–review and editing. EA: Conceptualization, Data curation, Formal Analysis, Investigation, Methodology, Validation, Visualization, Writing–original draft, Writing–review and editing.
Funding
The author(s) declare that no financial support was received for the research, authorship, and/or publication of this article.
Conflict of interest
The authors declare that the research was conducted in the absence of any commercial or financial relationships that could be construed as a potential conflict of interest.
Publisher’s note
All claims expressed in this article are solely those of the authors and do not necessarily represent those of their affiliated organizations, or those of the publisher, the editors and the reviewers. Any product that may be evaluated in this article, or claim that may be made by its manufacturer, is not guaranteed or endorsed by the publisher.
Supplementary material
The Supplementary Material for this article can be found online at: https://www.frontiersin.org/articles/10.3389/fchem.2024.1357330/full#supplementary-material
References
Abdalla, E. M., Abdel Rahman, L. H., Abdelhamid, A. A., Shehata, M. R., Alothman, A. A., and Nafady, A. (2020). Synthesis, characterization, theoretical studies, and antimicrobial/antitumor potencies of salen and salen/imidazole complexes of Co (II), Ni (II), Cu (II), Cd (II), Al (III) and La (III). Appl. Organomet. Chem. 34, e5912. doi:10.1002/aoc.5912
Abdalla, E. M., Hassan, S. S., Elganzory, H. H., Aly, S. A., and Alshater, H. (2021). Molecular docking, DFT calculations, effect of high energetic ionizing radiation, and biological evaluation of some novel metal (II) heteroleptic complexes bearing the thiosemicarbazone ligand. Molecules 26, 5851. doi:10.3390/molecules26195851
Abdalrazaq, E., Jbarah, A. a.Q., Al-Noor, T. H., Shinain, G. T., and Jawad, M. M. (2022). Synthesis, DFT calculations, DNA interaction, and antimicrobial studies of some mixed ligand complexes of oxalic acid and Schiff base trimethoprim with various metal ions. Indonesian J. Chem. 22, 1348–1364. doi:10.22146/ijc.74020
Abdel Rahman, L. H., Al-Zaqri, N., Abdelghani, A. A., and Abdalla, E. M. (2022). Physicochemical, in vitro therapeutic activity, DNA-binding, and in silico molecular docking studies of samarium (III) complexes bearing N, O-chelated Schiff base ligands. J. Coord. Chem. 75, 994–1018. doi:10.1080/00958972.2022.2095267
Abdel-Rahman, L. H., Basha, M. T., Al-Farhan, B. S., Shehata, M. R., and Abdalla, E. M. (2022). Synthesis, characterization, potential antimicrobial, antioxidant, anticancer, DNA binding, and molecular docking activities and DFT on novel Co (II), Ni (II), VO (II), Cr (III), and La (III) Schiff base complexes. Appl. Organomet. Chem. 36, e6484. doi:10.1002/aoc.6484
Aghatabay, N. M., Somer, M., Senel, M., Dulger, B., and Gucin, F. (2007). Raman, FT-IR, NMR spectroscopic data and antimicrobial activity of bis[μ2-(benzimidazol-2-yl)-2-ethanethiolato-N,S,S-chloro-palladium(II)] dimer, [(μ2-CH2CH2NHNCC6H4)PdCl]2·C2H5OH complex. Eur. J. Med. Chem. 42, 1069–1075. doi:10.1016/j.ejmech.2007.01.011
Al-Farhan, B. S., Basha, M. T., Abdel Rahman, L. H., El-Saghier, A. M., El-Ezz, A., Marzouk, A. A., et al. (2021). Synthesis, dft calculations, antiproliferative, bactericidal activity and molecular docking of novel mixed-ligand salen/8-hydroxyquinoline metal complexes. Molecules 26, 4725. doi:10.3390/molecules26164725
Al-Hazmi, G. A., Abou-Melha, K. S., El-Metwaly, N. M., Althagafi, I., Shaaban, F., Elghalban, M. G., et al. (2020). Spectroscopic and theoretical studies on Cr (III), Mn (II) and Cu (II) complexes of hydrazone derived from picolinic hydrazide and O-vanillin and evaluation of biological potency. Appl. Organomet. Chem. 34, e5408. doi:10.1002/aoc.5408
Anjaneyulu, Y., and Rao, R. P. (1986). Preparation, characterization and antimicrobial activity studies on some ternary complexes of Cu (II) with acetylacetone and various salicylic acids. Synth. React. Inorg. Met.-Org. Chem. 16 (2), 257–272.
Alodeani, E. A., Arshad, M., and Izhari, M. A. (2015). Anti-uropathogenic activity, drug likeness, physicochemical and molecular docking assessment of (E-)-N′-(substituted-benzylidene)-2-(quinolin-8-yloxy) acetohydrazide. Asian Pac. J. Trop. Biomed. 5, 676–683. doi:10.1016/j.apjtb.2015.04.010
Aly, S., and El-Boraey, H. A. (2019). Effect of gamma irradiation on spectral, XRD, SEM, DNA binding, molecular modling and antibacterial property of some (Z) N-(furan-2-yl) methylene)-2-(phenylamino) acetohydrazide metal (II) complexes. J. Mol. Struct. 1185, 323–332. doi:10.1016/j.molstruc.2019.02.069
Aly, S., and Elembaby, D. (2020a). Synthesis, spectroscopic characterization and Study the effect of gamma irradiation on VO2+, Mn2+, Zn2+, Ru3+, Pd2+, Ag+ and Hg2+ complexes and antibacterial activities. Arab. J. Chem. 13, 4425–4447. doi:10.1016/j.arabjc.2019.08.007
Aly, S., and Elembaby, D. (2020b). Synthesis, spectroscopic characterization and study the effect of gamma irradiation on VO2+, Mn2+, Zn2+, Ru3+, Pd2+, Ag+ and Hg2+ complexes and antibacterial activities. Arabian J. Chem. 13, 4425–4447. doi:10.1016/j.arabjc.2019.08.007
Aly, S., and Fathalla, S. (2020). Preparation, characterization of some transition metal complexes of hydrazone derivatives and their antibacterial and antioxidant activities. Arabian J. Chem. 13, 3735–3750. doi:10.1016/j.arabjc.2019.12.003
Aly, S. A., Elganzory, H. H., Mahross, M. H., and Abdalla, E. M. (2021). Quantum chemical studies and effect of gamma irradiation on the spectral, thermal, X-ray diffraction and DNA interaction with Pd (II), Cu (I), and Cd (II) of hydrazone derivatives. Appl. Organomet. Chem. 35, e6153. doi:10.1002/aoc.6153
Aly, S. A., Hassan, S. S., El-Boraey, H. A., Eldourghamy, A., Abdalla, E. M., Alminderej, F. M., et al. (2023). Synthesis, biological activity, and the effect of ionization radiation on the spectral, XRD, and TGA analysis of Cu (I), Cu (II), Zn (II), and Cd (II) complexes. Arabian J. Sci. Eng. 49, 361–379. doi:10.1007/s13369-023-07988-2
Aly, S. A., Hassan, S. S., Eldourghamy, A. S., Badr, E. E., El-Salamoney, M. A., Hassan, M. A., et al. (2022a). Synthesis, characterization, XRD, SEM, DNA binding and effect of γ-irradiation of some new Ni (II) and Co (II) complexes with thiosemicarbazone ligand. in vitro antimicrobial and antioxidant activities. Appl. Organomet. Chem. 36, e6727. doi:10.1002/aoc.6727
Aly, S. A., Hassan, S. S., Eldourghamy, A. S., Badr, E. E., El-Salamoney, M. A., Hassan, M. A., et al. (2022b). Synthesis, characterization, XRD, SEM, DNA binding, and effect of γ-irradiation of some new Ni (II) and Co (II) complexes with thiosemicarbazone ligand: in vitro antimicrobial and antioxidant activities. Appl. Organomet. Chem. 36, e6727. doi:10.1002/aoc.6727
Ammar, R. A., Alaghaz, A.-N. M., and Elhenawy, A. A. (2014). DFT, characterization and investigation of vibrational spectroscopy of 4-(4-hydroxy)-3-(2-pyrazine-2-carbonyl) hydrazonomethylphenyl-diazen-yl-benzenesulfonamide and its copper (II) complex. J. Mol. Struct. 1067, 94–103. doi:10.1016/j.molstruc.2014.02.051
Anastassova, N. O., Yancheva, D. Y., Mavrova, A. T., Kondeva-Burdina, M. S., Tzankova, V. I., Hristova-Avakumova, N. G., et al. (2018). Design, synthesis, antioxidant properties and mechanism of action of new N, N′-disubstituted benzimidazole-2-thione hydrazone derivatives. J. Mol. Struct. 1165, 162–176. doi:10.1016/j.molstruc.2018.03.119
Aneja, B., Khan, N. S., Khan, P., Queen, A., Hussain, A., Rehman, M. T., et al. (2019). Design and development of Isatin-triazole hydrazones as potential inhibitors of microtubule affinity-regulating kinase 4 for the therapeutic management of cell proliferation and metastasis. Eur. J. Med. Chem. 163, 840–852. doi:10.1016/j.ejmech.2018.12.026
Babahan, I., Özmen, A., Aksel, M., Bilgin, M. D., Gumusada, R., Gunay, M. E., et al. (2020). A novel bidentate ligand containing oxime, hydrazone and indole moieties and its BF2+ bridged transition metal complexes and their efficiency against prostate and breast cancer cells. Appl. Organomet. Chem. 34, e5632. doi:10.1002/aoc.5632
Balashova, T. V., Obolensky, S. V., Trufanov, A. N., Ivin, M. N., Ilichev, V. A., Kukinov, A. A., et al. (2019). Impact of n, γ-irradiation on organic complexes of rare earth metals. Sci. Rep. 9, 13314–13316. doi:10.1038/s41598-019-49962-9
Beeton, M. L., Aldrich-Wright, J. R., and Bolhuis, A. (2014). The antimicrobial and antibiofilm activities of copper (II) complexes. J. Inorg. Biochem. 140, 167–172. doi:10.1016/j.jinorgbio.2014.07.012
Bergamini, F. R., Nunes, J. H., De Carvalho, M. A., Ribeiro, M. A., De Paiva, P. P., Banzato, T. P., et al. (2019). Polynuclear copper (II) complexes with nalidixic acid hydrazones: antiproliferative activity and selectivity assessment over a panel of tumor cells. Inorganica Chim. acta 484, 491–502. doi:10.1016/j.ica.2018.09.084
Biyala, M. K., Sharma, K., Swami, M., Fahmi, N., and Singh, R. V. (2008). Spectral and biocidal studies of palladium (II) and platinum (II) complexes with monobasic bidentate Schiff bases. Transit. Metal. Chem. 33, 377–381. doi:10.1007/s11243-008-9053-3
Cao, W., Liu, Y., Zhang, T., and Jia, J. (2018). Synthesis, characterization, theoretical and antimicrobial studies of tridentate hydrazone metal complexes of Zn (II), Cd (II), Cu (II) and Co (III). Polyhedron 147, 62–68. doi:10.1016/j.poly.2018.03.012
Champoux, J. J. (2001). DNA topoisomerases: structure, function, and mechanism. Annu. Rev. Biochem. 70, 369–413. doi:10.1146/annurev.biochem.70.1.369
Coimbra, E. S., De Souza, M. V. N., Terror, M. S., Pinheiro, A. C., and Da Trindade Granato, J. (2019). Synthesis, biological activity, and mechanism of action of new 2-pyrimidinyl hydrazone and N-acylhydrazone derivatives, a potent and new classes of antileishmanial agents. Eur. J. Med. Chem. 184, 111742. doi:10.1016/j.ejmech.2019.111742
Dehestani, L., Ahangar, N., Hashemi, S. M., Irannejad, H., Masihi, P. H., Shakiba, A., et al. (2018). Design, synthesis, in vivo and in silico evaluation of phenacyl triazole hydrazones as new anticonvulsant agents. Bioorg. Chem. 78, 119–129. doi:10.1016/j.bioorg.2018.03.001
Ding, C.-C., Wu, S.-Y., Xu, Y.-Q., Wu, L.-N., and Zhang, L.-J. (2018). DFT studies for three Cu (II) coordination polymers: geometrical and electronic structures, g factors and UV–visible spectra. Chem. Phys. 508, 20–25. doi:10.1016/j.chemphys.2018.04.016
Dorotíková, S., Kožíšková, J., Malček, M., Jomová, K., Herich, P., Plevová, K., et al. (2015). Copper (II) complexes with new fluoroquinolones: synthesis, structure, spectroscopic and theoretical study, DNA damage, cytotoxicity and antiviral activity. J. Inorg. Biochem. 150, 160–173. doi:10.1016/j.jinorgbio.2015.06.017
Ekennia, A. C., Osowole, A. A., Onwudiwe, D. C., Babahan, I., Ibeji, C. U., Okafor, S. N., et al. (2018). Synthesis, characterization, molecular docking, biological activity and density functional theory studies of novel 1, 4-naphthoquinone derivatives and Pd (II), Ni (II) and Co (II) complexes. Appl. Organomet. Chem. 32, e4310. doi:10.1002/aoc.4310
El-Boraey, H. A., and El-Gammal, O. A. (2015). New 15-membered tetraaza (N4) macrocyclic ligand and its transition metal complexes: spectral, magnetic, thermal and anticancer activity. Spectrochimica Acta Part A Mol. Biomol. Spectrosc. 138, 553–562. doi:10.1016/j.saa.2014.11.015
El-Boraey, H. A., and Mansour, A. I. (2018). Synthesis, spectral and gamma ray irradiation studies on metal complexes of N, N′-naphthalene-1, 8-diylbis (2-aminobenzamide). Inorg. Nano-Metal Chem. 48, 8–15. doi:10.1080/24701556.2017.1357588
El-Etrawy, A.-a.S., and Sherbiny, F. F. (2021). Design, synthesis, biological assessment and molecular docking studies of some new 2-Thioxo-2, 3-dihydropyrimidin-4 (1H)-ones as potential anticancer and antibacterial agents. J. Mol. Struct. 1225, 129014. doi:10.1016/j.molstruc.2020.129014
Elganzory, H. H., Hassan, S. S., Aly, S. A., and Abdalla, E. M. (2022). Synthesis, characterization, PXRD studies, theoretical calculation, and antitumor potency studies of a novel N, O-multidentate chelating ligand and its Zr (IV), V (IV), Ru (III), and Cd (II) complexes. Bioinorg. Chem. Appl. 2022, 1–20. doi:10.1155/2022/2006451
El-Saied, F. A., Shakdofa, M. M., Al-Hakimi, A. N., and Shakdofa, A. M. (2020). Transition metal complexes derived from N′-(4-fluorobenzylidene)-2-(quinolin-2-yloxy) acetohydrazide: synthesis, structural characterization, and biocidal evaluation. Appl. Organomet. Chem. 34, e5898. doi:10.1002/aoc.5898
Elsayed, S. A., El-Gharabawy, H. M., Butler, I. S., and Atlam, F. M. (2020). Novel metal complexes of 3-acetylcoumarin-2-hydrazinobenzothiazole Schiff base: design, structural characterizations, DNA binding, DFT calculations, molecular docking and biological studies. Appl. Organomet. Chem. 34, e5643. doi:10.1002/aoc.5643
Frisch, A., Hratchian, H. P., Dennington Ii, R., Keith, T., Millam, J., Nielsen, B., et al. (2009a). GaussView version 5.0. 8. Wallingford, CT: Gaussian. Inc.
Frisch, M., Trucks, G., Schlegel, H., Scuseria, G., Robb, M., Cheeseman, J., et al. (2009b). Gaussian 09, revision A. 02. Wallingford, CT: Gaussian, Inc.
Garoufis, A., Hadjikakou, S., and Hadjiliadis, N. (2009). Palladium coordination compounds as anti-viral, anti-fungal, anti-microbial and anti-tumor agents. Coord. Chem. Rev. 253, 1384–1397. doi:10.1016/j.ccr.2008.09.011
Guerra, W., De Andrade Azevedo, E., De Souza Monteiro, A. R., Bucciarelli-Rodriguez, M., Chartone-Souza, E., Nascimento, A. M. A., et al. (2005). Synthesis, characterization, and antibacterial activity of three palladium (II) complexes of tetracyclines. J. Inorg. Biochem. 99, 2348–2354. doi:10.1016/j.jinorgbio.2005.09.001
Haghighijoo, Z., Firuzi, O., Hemmateenejad, B., Emami, S., Edraki, N., and Miri, R. (2017). Synthesis and biological evaluation of quinazolinone-based hydrazones with potential use in Alzheimer’s disease. Bioorg. Chem. 74, 126–133. doi:10.1016/j.bioorg.2017.07.014
Hassan, S. S., and Gomha, S. M. (2019). Novel functionalized thiosemicarbazone ligands and their Pd (II) complexes: synthesis, characterization, antibacterial and cytotoxic activities. Chem. Pap. 73, 331–344. doi:10.1007/s11696-018-0592-6
Hassan, S. S., and Khalf-Alla, P. A. (2020). Anti-hepatocellular carcinoma, antioxidant, antiinflammation and antimicrobial investigation of some novel first and second transition metal complexes. Appl. Organomet. Chem. 34 (4), e5432.
Hassan, S. S., and Mohamed, E. F. (2020). Antimicrobial, antioxidant and antitumor activities of Nano-Structure Eu (III) and La (III) complexes with nitrogen donor tridentate ligands. Appl. Organomet. Chem. 34, e5258. doi:10.1002/aoc.5258
Hoonur, R. S., Patil, B. R., Badiger, D. S., Vadavi, R. S., Gudasi, K. B., Dandawate, P. R., et al. (2010). Transition metal complexes of 3-aryl-2-substituted 1, 2-dihydroquinazolin-4 (3H)-one derivatives: new class of analgesic and anti-inflammatory agents. Eur. J. Med. Chem. 45, 2277–2282. doi:10.1016/j.ejmech.2010.01.072
Hubin, T. J., Amoyaw, P.N.-A., Roewe, K. D., Simpson, N. C., Maples, R. D., Freeman, T. N. C., et al. (2014). Synthesis and antimalarial activity of metal complexes of cross-bridged tetraazamacrocyclic ligands. Bioorg. Med. Chem. 22, 3239–3244. doi:10.1016/j.bmc.2014.05.003
Katariya, K. D., Shah, S. R., and Reddy, D. (2020). Anticancer, antimicrobial activities of quinoline based hydrazone analogues: synthesis, characterization and molecular docking. Bioorg. Chem. 94, 103406. doi:10.1016/j.bioorg.2019.103406
Khalf-Alla, P. A., Hassan, S. S., and Shoukry, M. M. (2019). Complex formation equilibria, DFT, docking, antioxidant and antimicrobial studies of iron (III) complexes involving Schiff bases derived from glucosamine or ethanolamine. Inorganica Chim. Acta 492, 192–197.
Khan, S. A., Rizwan, K., Shahid, S., Noamaan, M. A., Rasheed, T., and Amjad, H. (2020). Synthesis, DFT, computational exploration of chemical reactivity, molecular docking studies of novel formazan metal complexes and their biological applications. Appl. Organomet. Chem. 34, e5444. doi:10.1002/aoc.5444
Lemoine, P., Viossat, B., Morgant, G., Greenaway, F. T., Tomas, A., Dung, N.-H., et al. (2002). Synthesis, crystal structure, EPR properties, and anti-convulsant activities of binuclear and mononuclear 1, 10-phenanthroline and salicylate ternary copper (II) complexes. J. Inorg. Biochem. 89, 18–28. doi:10.1016/s0162-0134(01)00324-5
Liu, Y.-T., Lian, G.-D., Yin, D.-W., and Su, B.-J. (2013). Synthesis, characterization and biological activity of ferrocene-based Schiff base ligands and their metal (II) complexes. Spectrochimica Acta Part A Mol. Biomol. Spectrosc. 100, 131–137. doi:10.1016/j.saa.2012.03.049
Lobana, T. S., Indoria, S., Jassal, A. K., Kaur, H., Arora, D. S., and Jasinski, J. P. (2014). Synthesis, structures, spectroscopy and antimicrobial properties of complexes of copper (II) with salicylaldehyde N-substituted thiosemicarbazones and 2, 2′-bipyridine or 1, 10-phenanthroline. Eur. J. Med. Chem. 76, 145–154. doi:10.1016/j.ejmech.2014.02.009
Mahani, N. M., and Mohammadi, S. Z. (2022). Studies of quantum mechanics/molecular docking on zanubrutinib as potential repurposed against Covid-19. Bull. Chem. Soc. Ethiop. 36, 479–485. doi:10.4314/bcse.v36i2.19
Mandewale, M. C., Patil, U. C., Shedge, S. V., Dappadwad, U. R., and Yamgar, R. S. (2017). A review on quinoline hydrazone derivatives as a new class of potent antitubercular and anticancer agents. Beni-Suef Univ. J. basic Appl. Sci. 6, 354–361. doi:10.1016/j.bjbas.2017.07.005
Mandewale, M. C., Thorat, B., Nivid, Y., Jadhav, R., Nagarsekar, A., and Yamgar, R. (2018). Synthesis, structural studies and antituberculosis evaluation of new hydrazone derivatives of quinoline and their Zn (II) complexes. J. Saudi Chem. Soc. 22, 218–228. doi:10.1016/j.jscs.2016.04.003
Manohar, C. S., Manikandan, A., Sridhar, P., Sivakumar, A., Kumar, B. S., and Reddy, S. R. (2018). Drug repurposing of novel quinoline acetohydrazide derivatives as potent COX-2 inhibitors and anti-cancer agents. J. Mol. Struct. 1154, 437–444. doi:10.1016/j.molstruc.2017.10.075
Margariti, A., Papakonstantinou, V. D., Stamatakis, G. M., Demopoulos, C. A., Schnakenburg, G., Andreopoulou, A. K., et al. (2020). Substituted pyridine-quinoline ligands as building blocks for neutral rhodium (III) complexes. Synthesis, structural characterization studies and anti-platelet activity towards the Platelet-Activating Factor (PAF). Polyhedron 178, 114336. doi:10.1016/j.poly.2019.114336
Maurya, R., Malik, B., Mir, J., Vishwakarma, P., Rajak, D., and Jain, N. (2015). Nickel (II) complexes of ONS donor Schiff base ligands: synthesis, combined DFT-experimental characterization, redox, thermal, and in vitro biological investigation. J. Coord. Chem. 68, 2902–2922. doi:10.1080/00958972.2015.1064526
Maurya, S. S., Khan, S. I., Bahuguna, A., Kumar, D., and Rawat, D. S. (2017). Synthesis, antimalarial activity, heme binding and docking studies of N-substituted 4-aminoquinoline-pyrimidine molecular hybrids. Eur. J. Med. Chem. 129, 175–185. doi:10.1016/j.ejmech.2017.02.024
Meira, C. S., Dos Santos Filho, J. M., Sousa, C. C., Anjos, P. S., Cerqueira, J. V., Neto, H. a.D., et al. (2018). Structural design, synthesis and substituent effect of hydrazone-N-acylhydrazones reveal potent immunomodulatory agents. Bioorg. Med. Chem. 26, 1971–1985. doi:10.1016/j.bmc.2018.02.047
Muniz, F. T. L., Miranda, M. a.R., Morilla Dos Santos, C., and Sasaki, J. M. (2016). The Scherrer equation and the dynamical theory of X-ray diffraction. Acta Crystallogr. Sect. A Found. Adv. 72, 385–390. doi:10.1107/s205327331600365x
Naveen, P., Vijaya Pandiyan, B., Anu, D., Dallemer, F., Kolandaivel, P., and Prabhakaran, R. (2020). A pseudo trinuclear nickel–sodium complex containing tris (8-methyl-2-oxo-quinolidineamino ethylamine): synthesis, spectral characterization, X-ray crystallography and in vitro biological evaluations. Appl. Organomet. Chem. 34, e5605. doi:10.1002/aoc.5605
Netalkar, P. P., Netalkar, S. P., Budagumpi, S., and Revankar, V. K. (2014). Synthesis, crystal structures and characterization of late first row transition metal complexes derived from benzothiazole core: anti-tuberculosis activity and special emphasis on DNA binding and cleavage property. Eur. J. Med. Chem. 79, 47–56. doi:10.1016/j.ejmech.2014.03.083
Özbek, N., Özdemir, Ü. Ö., Altun, A. F., and Şahin, E. (2019). Sulfonamide-derived hydrazone compounds and their Pd (II) complexes: synthesis, spectroscopic characterization, X-ray structure determination, in vitro antibacterial activity and computational studies. J. Mol. Struct. 1196, 707–719. doi:10.1016/j.molstruc.2019.07.016
Parlar, S., Sayar, G., Tarikogullari, A. H., Karadagli, S. S., Alptuzun, V., Erciyas, E., et al. (2019). Synthesis, bioactivity and molecular modeling studies on potential anti-Alzheimer piperidinehydrazide-hydrazones. Bioorg. Chem. 87, 888–900. doi:10.1016/j.bioorg.2018.11.051
Parveen, S., Govindarajan, S., Puschmann, H., and Revathi, R. (2018). Synthesis, crystal structure and biological studies of new hydrazone ligand, 2- (Methoxycarbonyl-hydrazono)-pentanedioic acid and its silver (I) complex. Inorganica Chim. Acta 477, 66–74. doi:10.1016/j.ica.2018.02.022
Patel, M. M., and Patel, L. J. (2014). Design, synthesis, molecular docking, and antibacterial evaluation of some novel flouroquinolone derivatives as potent antibacterial agent. Sci. World J. 2014, 1–10. doi:10.1155/2014/897187
Perontsis, S., Hatzidimitriou, A. G., Begou, O.-A., Papadopoulos, A. N., and Psomas, G. (2016). Characterization and biological properties of copper (II)-ketoprofen complexes. J. Inorg. Biochem. 162, 22–30. doi:10.1016/j.jinorgbio.2016.06.001
Philip, J. E., Antony, S. A., Eeettinilkunnathil, S. J., Kurup, M. P., and Velayudhan, M. P. (2018). Design, synthesis, antimicrobial and antioxidant activity of 3-formyl chromone hydrazone and their metal (II) complexes. Inorganica Chim. Acta 469, 87–97. doi:10.1016/j.ica.2017.09.006
Pisano, M. B., Kumar, A., Medda, R., Gatto, G., Pal, R., Fais, A., et al. (2019). Antibacterial activity and molecular docking studies of a selected series of hydroxy-3-arylcoumarins. Molecules 24, 2815. doi:10.3390/molecules24152815
Popiołek, Ł., Piątkowska-Chmiel, I., Gawrońska-Grzywacz, M., Biernasiuk, A., Izdebska, M., Herbet, M., et al. (2018). New hydrazide-hydrazones and 1, 3-thiazolidin-4-ones with 3-hydroxy-2-naphthoic moiety: synthesis, in vitro and in vivo studies. Biomed. Pharmacother. 103, 1337–1347. doi:10.1016/j.biopha.2018.04.163
Qiu, L., Lv, G., Guo, L., Chen, L., Luo, S., Zou, M., et al. (2015). Synthesis, crystal structure and antitumor effect of a novel copper (II) complex bearing zoledronic acid derivative. Eur. J. Med. Chem. 89, 42–50. doi:10.1016/j.ejmech.2014.10.028
Quintanova, C., Keri, R. S., Chaves, S., and Santos, M. A. (2015). Copper (II) complexation of tacrine hybrids with potential anti-neurodegenerative roles. J. Inorg. Biochem. 151, 58–66. doi:10.1016/j.jinorgbio.2015.06.008
Redgrave, L. S., Sutton, S. B., Webber, M. A., and Piddock, L. J. (2014). Fluoroquinolone resistance: mechanisms, impact on bacteria, and role in evolutionary success. Trends Microbiol. 22, 438–445. doi:10.1016/j.tim.2014.04.007
Rocha, C. S., Bomfim Filho, L. F., De Souza, A. E., Diniz, R., Denadai, Â. M., Beraldo, H., et al. (2019). Structural studies and investigation on the antifungal activity of silver (I) complexes with 5-nitrofuran-derived hydrazones. Polyhedron 170, 723–730. doi:10.1016/j.poly.2019.06.033
Santiago, P. H., Tiago, F. S., Castro, M. S., Souza, P. E., Martins, J. B., and Gatto, C. C. (2020). DFT analysis, spectroscopic study and biological activity of a newly synthesized benzoylhydrazone binuclear Cu (II) complex. J. Inorg. Biochem. 204, 110949. doi:10.1016/j.jinorgbio.2019.110949
Sepay, N., and Dey, S. P. (2014). Synthesis and chemical reactivity of 4-oxo-4H-1-benzopyran-3-carboxaldehyde. J. Heterocycl. Chem. 51, E1–E24. doi:10.1002/jhet.2001
Shabbir, A., Shahzad, M., Ali, A., and Zia-Ur-Rehman, M. (2014). Anti-arthritic activity of N′-[(2, 4-dihydroxyphenyl) methylidene]-2-(3, 4-dimethyl-5, 5-dioxidopyrazolo [4, 3-c] [1, 2] benzothiazin-1 (4H)-yl) acetohydrazide. Eur. J. Pharmacol. 738, 263–272. doi:10.1016/j.ejphar.2014.05.045
Shankarwar, S. G., Nagolkar, B. B., Shelke, V. A., and Chondhekar, T. K. (2015). Synthesis, spectral, thermal and antimicrobial studies of transition metal complexes of 14-membered tetraaza [N4] macrocyclic ligand. Spectrochimica Acta Part A Mol. Biomol. Spectrosc. 145, 188–193. doi:10.1016/j.saa.2015.02.006
Sherif, Y. E., and Hosny, N. M. (2014). Anti-rheumatic potential of ethyl 2-(2-cyano-3-mercapto-3-(phenylamino) acrylamido)-4, 5, 6, 7-tetrahydrobenzo [b] thiophene-3-carboxylate and its Co (II), Cu (II) and Zn (II) complexes. Eur. J. Med. Chem. 83, 338–343. doi:10.1016/j.ejmech.2014.06.038
Soroceanu, A., Vacareanu, L., Vornicu, N., Cazacu, M., Rudic, V., and Croitori, T. (2016). Assessment of some application potentials for copper complexes of the ligands containing siloxane moiety: antimicrobial, antifungal, antioxidant and redox activity. Inorganica Chim. Acta 442, 119–123. doi:10.1016/j.ica.2015.12.006
Sreepriya, R., Kumar, S. S., Sadasivan, V., Biju, S., and Meena, S. S. (2020). Synthesis, characterization and biological studies of Mn (II), Fe (III) and Co (II) complexes of (Z)-1, 5-dimethyl-4- (2- (2-oxopropylidene) hydrazinyl)-2-phenyl-1H-pyrazol-3 (2H)-one. J. Mol. Struct. 1201, 127110. doi:10.1016/j.molstruc.2019.127110
Stefani, C., Al-Eisawi, Z., Jansson, P. J., Kalinowski, D. S., and Richardson, D. R. (2015). Identification of differential anti-neoplastic activity of copper bis (thiosemicarbazones) that is mediated by intracellular reactive oxygen species generation and lysosomal membrane permeabilization. J. Inorg. Biochem. 152, 20–37. doi:10.1016/j.jinorgbio.2015.08.010
Tian, W.-X., Yu, S., Ibrahim, M., Almonaofy, A. W., He, L., Hui, Q., et al. (2012). Copper as an antimicrobial agent against opportunistic pathogenic and multidrug resistant Enterobacter bacteria. J. Microbiol. 50, 586–593. doi:10.1007/s12275-012-2067-8
Tolia, C., Papadopoulos, A. N., Raptopoulou, C. P., Psycharis, V., Garino, C., Salassa, L., et al. (2013). Copper (II) interacting with the non-steroidal antiinflammatory drug flufenamic acid: structure, antioxidant activity and binding to DNA and albumins. J. Inorg. Biochem. 123, 53–65. doi:10.1016/j.jinorgbio.2013.02.009
Ugurlu, G., and Harmankaya, A. (2022). Investigation on molecular structure and electronic properties of zinc (II) complex with 2-acetylpyridinenicotinichydrazone ligand. Eurasia Proc. Sci. Technol. Eng. Math. 20, 58–65. doi:10.55549/epstem.1222639
Urquiza, N. M., Islas, M. S., Ariza, S. T., Jori, N., Medina, J. J. M., Lavecchia, M. J., et al. (2015). Anti-thyroid and antifungal activities, BSA interaction and acid phosphatase inhibition of methimazole copper (II) complexes. Chemico-biological Interact. 229, 64–72. doi:10.1016/j.cbi.2014.12.036
Vanucci-Bacqué, C., Camare, C., Carayon, C., Bernis, C., Baltas, M., Nègre-Salvayre, A., et al. (2016). Synthesis and evaluation of antioxidant phenolic diaryl hydrazones as potent antiangiogenic agents in atherosclerosis. Bioorg. Med. Chem. 24, 3571–3578. doi:10.1016/j.bmc.2016.05.067
Vieira, L. M. M., De Almeida, M. V., Lourenço, M. C. S., Bezerra, F. a.F., and Fontes, A. P. S. (2009). Synthesis and antitubercular activity of palladium and platinum complexes with fluoroquinolones. Eur. J. Med. Chem. 44, 4107–4111. doi:10.1016/j.ejmech.2009.05.001
Yousefi, M., Sedaghat, T., Simpson, J., and Shafiei, M. (2019). Bis-aroylhydrazone based on 2, 2′-bis substituted diphenylamine for synthesis of new binuclear organotin (IV) complexes: spectroscopic characterization, crystal structures, in vitro DNA-binding, plasmid DNA cleavage, PCR and cytotoxicity against MCF7 cell line. Appl. Organomet. Chem. 33, e5137. doi:10.1002/aoc.5137
Zülfikaroğlu, A., Ataol, Ç. Y., Çelikoğlu, E., Çelikoğlu, U., and İdil, Ö. (2020). New Cu (II), Co (III) and Ni (II) metal complexes based on ONO donor tridentate hydrazone: synthesis, structural characterization, and investigation of some biological properties. J. Mol. Struct. 1199, 127012. doi:10.1016/j.molstruc.2019.127012
Keywords: complexes, γ-irradiation, antimicrobial, thermal, DFT, molecular docking
Citation: Hassan SS, Aly SA, Al-Sulami AI, Albohy SAH, Salem MF, Nasr GM and Abdalla EM (2024) Synthesis, characterization, PXRD studies, and theoretical calculation of the effect of gamma irradiation and antimicrobial studies on novel Pd(II), Cu(II), and Cu(I) complexes. Front. Chem. 12:1357330. doi: 10.3389/fchem.2024.1357330
Received: 17 December 2023; Accepted: 22 January 2024;
Published: 12 February 2024.
Edited by:
Hakan Arslan, Mersin University, TürkiyeCopyright © 2024 Hassan, Aly, Al-Sulami, Albohy, Salem, Nasr and Abdalla. This is an open-access article distributed under the terms of the Creative Commons Attribution License (CC BY). The use, distribution or reproduction in other forums is permitted, provided the original author(s) and the copyright owner(s) are credited and that the original publication in this journal is cited, in accordance with accepted academic practice. No use, distribution or reproduction is permitted which does not comply with these terms.
*Correspondence: Samar A. Aly, samar.mostafa@gebri.usc.edu.eg; Ahlam I. Al-Sulami, aialsulami@uj.edu.sa; Ehab M. Abdalla, Ehababdalla99@sci.nvu.edu.eg