- Department of Chemistry, Yasouj University, Yasouj, Iran
The yolk-shell structured silica nanocomposites have been considered by many researchers due to their specific physical and chemical properties. These materials have been widely used in adsorption and catalysis processes. Especially, the void space of yolk−shell nanostructures can provide a unique environment for storage, compartmentation, and confinement in host−guest interactions. In this paper, for the first time, the preparation, characterization, and catalytic application of a novel amine-containing magnetic methylene-based periodic mesoporous organosilica with yolk-shell structure (YS-MPMO/pr-NH2) are developed. The magnetic periodic mesoporous organosilica nanocomposite was synthesized through surfactant-directed co-condensation of bis(triethoxysilyl)methane (BTEM) and tetraethoxysilane around Fe3O4 nanoparticles. After Soxhlet extraction, the surface of YS-MPMO nanocomposite was modified with 3-aminopropyl trimethoxysilane to deliver YS-MPMO-pr-NH2 nanocatalyst. This catalyst was characterized by using EDX, FT-IR, VSM, TGA, XRD, nitrogen-sorption, and SEM analyses. The catalytic activity of YS-MPMO/pr-NH2 was studied in the Knoevenagel reaction giving the corresponding products in a high yield and selectivity. The YS-MPMO/pr-NH2 nanocatalyst was recovered and reused at least four times without a significant decrease in efficiency and activity. A leaching test was performed to study the nature of the catalyst during reaction conditions Also, the catalytic performance of our designed nanocomposite was compared with some of the previous catalysts used in the Knoevenagel reaction.
1 Introduction
In recent years, silica-based nanocomposites have received much attention between researchers in various fields of chemistry. These materials have been extensively employed in chemical processes due to the good properties of silica such as high chemical and thermal stability, high colloidal stability, biocompatibility and easy surface modification (Maleki et al., 2015; Purbia and Paria, 2015; Sun et al., 2015; Cheng et al., 2017; Wang et al., 2019; Gopalan Sibi et al., 2020). Among these, yolk-shell (YS) structured silica nanocomposites have been considered and studied by many researchers (Nagaraju et al., 2017; Bai et al., 2018; Du et al., 2018). These nanocomposites have many applications in the areas of drug delivery, catalysis, charge transfer and storage in batteries, solar cells and supercapacitors, adsorbents for gases and pollutants, gene therapy, etc (Nagaraju et al., 2017; Xie et al., 2017; Bai et al., 2018; Du et al., 2018). For example, recently, the YS-structured nanocomposites have been used as catalyst in the synthesis of pyranopyrazoles (Neysi and Elhamifar, 2023), the Chan-Lum coupling reaction (Shaker and Elhamifar, 2021), and the reduction of nitrobenzenes (Wang et al., 2018).
Among the various types of yolk-shell structured silica nanocomposite, those that are composed of Fe3O4 core and PMO shell have been highly regarded by researchers due to their unique magnetic response, high adsorption capacity, high surface area, and high hydrophobicity (Haffer et al., 2010; Croissant et al., 2014; Lu et al., 2016; Wei et al., 2016; Abaeezadeh et al., 2019; Yu L. et al., 2019; Liu et al., 2019; Kargar et al., 2020). These nanocomposites have been used in various fields such as biomedicine, battery development, fuel cell technology, sensor technology, gene therapy, and nanocatalysis (Li H. et al., 2018; Li J. et al., 2018; Lin et al., 2018; Wang et al., 2018; Li X.-P. et al., 2019; Yu K. et al., 2019; Zhang et al., 2019). Some of recently reported nanocomposites with Fe3O4 core and PMO shells are Fe3O4@SiO2@PMO (Mirbagheri et al., 2021), Fe3O4-YS-PMO (Wei et al., 2016), YS-Fe3O4@Au@PMO (Liu et al., 2019), Fe3O4@mSiO2 (Li Y. et al., 2019), Fe3O4@PMO-NH2 (Rosso et al., 2020) and Fe3O4@MePMO-IL/Pd (Shaker and Elhamifar, 2020).
On the other hand, the Knoevenagel reaction (Gordel-Wojcik et al., 2022) is one of the most famous carbon-carbon coupling process to synthesize α,β-unsaturated compounds. In recent years, the synthesis of the Knoevenagel products in the presence of heterogeneous and homogeneous catalysts has been investigated under different conditions. Due to difficulty in the separation of homogeneous catalysts, the use of magnetic heterogeneous catalysts is a good option to improve the efficiency of the catalytic processes. Some of recently reported studies in this matter are Fe3O4@SiO2@propyl@DBU (Zhang et al., 2021), L-proline-Cu/TCT@NH2@Fe3O4 (Kalantari et al., 2022), MgFe2O4(Ghomi and Akbarzadeh, 2018) and Fe3O4–cysteamine hydrochloride (Maleki et al., 2017).
In view of the above, in this research, a novel magnetic yolk-shell structured PMO supported propylamine (YS-MPMO/pr-NH2) is prepared, characterized and its catalytic application is developed in the Knoevenagel reaction under green conditions.
2 Experimental section
2.1 Synthesis of Fe3O4 nanoparticles
Fe3O4 NPs were firstly prepared according to our previous procedure (Neysi et al., 2020). According to this method, FeCl2.4H2O (1.5 g) and FeCl3.6H2O (3 g) were dissolved in 160 mL of deionized water. Then, aqueous ammonia (40 mL, 28% wt) was slowly added and the obtained mixture was stirred at room temperature (RT) for 60 min under argon atmosphere. The resulting product was collected using an external magnet and it was washed completely with distilled water and EtOH. This product was dried at 70°C for 12 h under vacuum and called Fe3O4 nanoparticles.
2.2 Preparation of yolk-shell structured magnetic PMO (YS-MPMO)
To prepare of YS-MPMO, firstly, Fe3O4 NPs (1 g) were completely dispersed in H2O (20 mL). Then, this mixture was added to a reaction vessel containing H2O (36 mL), EtOH (16 mL), cetyltrimethylammonium bromide (CTAB, 0.72 g), pluronic P123 (1.7 g) and ammonia (0.9 mL, 25% wt). The obtained combination was stirred at 35°C–40°C for 30 min. Next, 1,2-bis(triethoxysilyl)methane (BTEM, 2.1 g) and tetraethoxysilane (TEOS, 0.7 g) were added while stirring under the same conditions for 1 h. After that, the resulting mixture was heated at 100°C for 17 h under static conditions. Finally, the product was magnetically separated, washed with EtOH and H2O and dried. The surfactants were removed using a Soxhlet apparatus to give the YS-MPMO product.
2.3 Synthesis of YS-MPMO/pr-NH2
For this, firstly, the YS-MPMO nanocomposite (1 g) was dispersed in toluene (25 mL) at RT. Then, APTMS (3-aminopropyltrimethoxysilane, 98%, 1 mmol) was added and the resulting mixture was stirred at 100°C for 24 h. In the following, the product was magnetically separated, washed with EtOH and H2O, dried at 60°C for 12 h and called YS-MPMO/pr-NH2 nanocomposite. According to the CHN and EDX analyses the loading of amine groups on the designed nanocomposite surface was found to be 0.5 mmol/g.
2.4 Procedure for Knoevenagel reaction
For this, aldehyde (1 mmol), malononitrile (1 mmol) and YS-MPMO/pr-NH2 catalyst (2.25 mol%) were added in a reaction vessel while sonicating under solvent-free conditions at RT. In the end of reaction, monitored by TLC, EtOH (5 mL) was added and catalyst was magnetically removed. Then, the EtOH solvent was evaporated and impure products were recrystallized in EtOH and n-hexane solvents to give pure Knoevenagel products.
2.5 IR, 1H and 13C-NMR data of Knoevenagel products
2.5.1 2-(2-Chlorobenzylidene)malononitrile
IR (KBr, cm−1): 3035(=C–H, stretching vibration, sp2), 2223 (C≡N), 1480–1612 (C=C, Ar stretching sp2). 1H-NMR (400 MHz, CDCl3): δ (ppm), 7.58–7.63 (m, 1H), 7.66–7.75 (m, 1H), 8.06 (d, 1H, J = 6.0 Hz), 8.58 (d, 1H, J = 4.0), 8.70 (s, 1H). 13C-NMR (100 MHz, CDCl3): δ (ppm), 63.1, 87.14, 112.8, 113.9, 130.3, 130.8, 134.7, 135.3, 159.5.
2.5.2 2-(4-Nitrobenzylidene)malononitrile
IR (KBr, cm−1): 3105 (C-H, stretching vibration, sp2), 2204 (C≡N), 1514, 1358 (NO2, stretching vibration), 1411–1609 (C=C, Ar stretching sp2).1H-NMR (400 MHz, CDCl3): δ (ppm), 7.04 (d, 2H, J = 8.4 Hz), 6.6 (d, 2H, J = 8.4 Hz), 5.23 (s, 1H). 13C-NMR (100 MHz, CDCl3): δ (ppm), 85.39, 113.08, 114.13, 125.32, 128.40, 136.33, 148.49, 159.74.
3 Result and discussion
Firstly, core-shell structured magnetic periodic mesoporous organosilica (MPMO) was synthesized via hydrolysis and co-condensation of BTEM and TEOS around Fe3O4 NPs in the presence of CTAB and pluronic P123 surfactants. After Soxhlet extraction of surfactants, the YS-MPMO was produced. This material was then modified with 3-aminopropyltrimethoxysilane (APTMS) to give YS-MPMO/pr-NH2 nanocomposite (Figure 1).
Figure 2 shows the FT-IR spectra of Fe3O4, YS-MPMO and YS-MPMO/pr-NH2 nanoparticles. For all materials, the characteristic peaks of Fe−O and O-H bonds are, respectively, appeared at 588 and 3,400 cm−1 (Figures 2A–C). In the FT-IR spectra of YS-MPMO and YS-MPMO/pr-NH2, the peaks at 940 and 1,090 cm−1 are, respectively, assigned to symmetric and asymmetric vibrations of the Si-O-Si bonds proving the successful formation of silica layer around the Fe3O4 NPs. Also, for YS-MPMO and YS-MPMO/pr-NH2 nanocomposits, the C-H signals of aliphatic moieties are appeared at 2,880–2,911 cm−1 (Figures 2B,C).
The SEM analysis of YS-MPMO/pr-NH2 demonstrated a morphology with spherical particles and an average size of about 45 nm (Figure 3). These type nanoparticles are very important in the fields of catalysis and adsorption processes.
The EDX analysis of YS-MPMO/pr-NH2 nanocomposite successfully confirmed the presence of Fe, O, C, N and Si elements in its framework (Figure 4).
Also, the EDX mapping analysis revealed the well distribution of aforementioned elements in the framework of the YS-MPMO/pr-NH2 nanocomposite (Figure 5). These are in good agreement with the FT-IR results confirming well immobilization/incorporation of methylene and propylamine moieties on/in the material framework.
The magnetic properties of YS-MPMO/pr-NH2 nanocomposite were evaluated by using VSM analysis. The result of this study showed that YS-MPMO/pr-NH2 nanocomposite has a superparamagnetic behavior. Also, the amount of magnetic saturation of this nanocomposite was about 43 emu/g (Figure 6).
In the wide angle PXRD pattern of YS-MPMO/pr-NH2, the presence of 6 peaks at 2θ: 30.3, 36, 43.5, 54.5, 57.5 and 63°, corresponding to the crystalline structure of Fe3O4 NPs, affirms the high stability of these nanoparticles during the preparation of the YS-MPMO/pr-NH2 nanocomposite (Figure 7).
The TGA curve of YS-MPMO/pr-NH2 nanocomposite showed three weight losses. The first one (about 2%) in the range of 25°C–130°C is assigned to removal of water and organic solvents. The second one (about 2%) at 150°C–280°C is due to the elimination of remained CTAB and pluronic P123 surfactants. The third one at 300°C–700°C (about 11%) is corresponded to the removal of grafted propylamine moieties on the shell surface and also incorporated methylene groups in the shell framework (Figure 8) (Neysi and Elhamifar, 2023).
The N2 adsorption-desorption analysis of the YS-MPMO/pr-NH2 showed a type IV isotherm with a H2 hysteresis loop, corresponding to ordered mesostructured PMO shell (Figure 9). According to this analysis, the BET surface area and pore volume of the nanocomposite were found to be 470.67 m2/g and 0.973 cm3/g, respectively.
After characterization of the YS-MPMO/pr-NH2 nanocomposite, its catalytic activity was examined in the Knoevenagel condensation under ultrasonic conditions. To optimize the reaction conditions, the condensation between malononitrile and benzaldehyde was selected as a model reaction. Examination of the amount of catalyst in this reaction showed that the best yield is obtained in the presence of 2.25 mol% of YS-MPMO/pr-NH2 (Table 1, entries 1–4). Next, the catalytic activity of YS-MPMO/pr-NH2 was investigated in different solvents of H2O, EtOH and toluene and also solvent-free media. This study showed that the best result is obtained under solvent-free conditions. (Table 1, entry 3 vs. entries 5–7). The H-bonding between protic EtOH and water solvents and malononitrile is a parameter which prevents and restricts the activity of this nucleophile in these solvents. Finally, the catalytic activity of amine-free Fe3O4 and YS-MPMO materials were studied, in which only a little yield of the desired product was obtained confirming that the designed Knoevenagel reaction is catalyzed by supported propylamine groups (Table 1, entry 3 vs. entries 8, 9). Accordingly, the use of 2.25 mol% of catalyst, RT and solvent-free media were selected as optimal conditions (Table 1, entry 3).
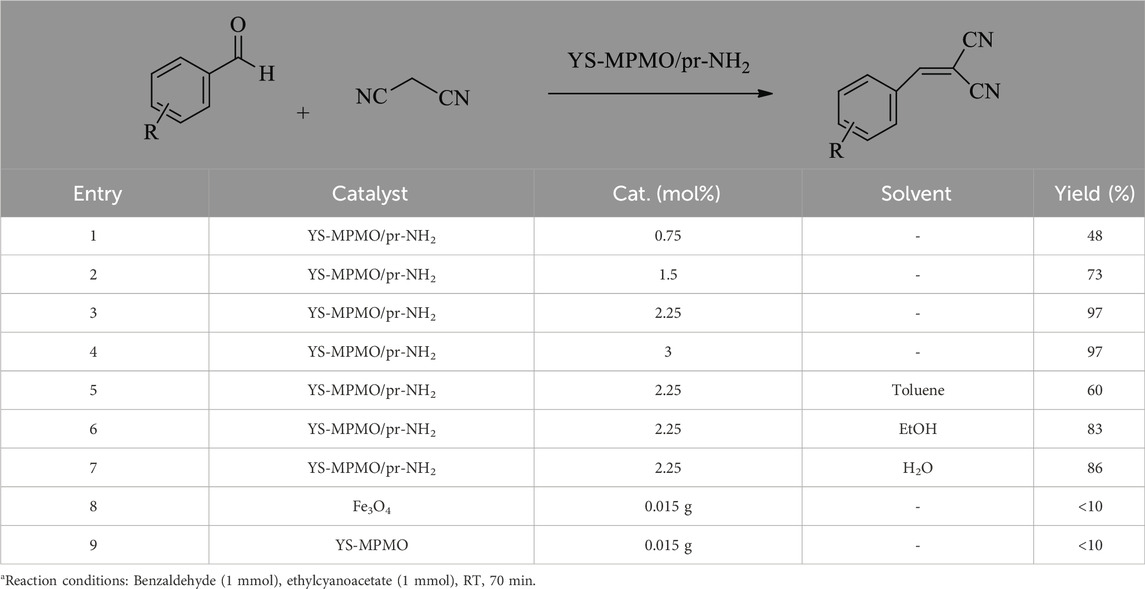
TABLE 1. The effect of solvent and catalyst loading in the Knoevenagel reaction of malononitrile with benzaldehydea.
In the following, the catalytic activity of YS-MPMO/pr-NH2 nanocatalyst was investigated in the condensation of various aldehydes with malononitrile under the optimal conditions. The study demonstrated that all aldehydes, bearing both electron withdrawing and electron donating substituents in various positions, give the corresponding Knoevenagel products in high yield and selectivity (Table 2). This confirms the high efficiency of the designed catalyst for the preparation of a wide range of important Knoevenagel products.
In the following, the recoverability and reusability of the YS-MPMO/pr-NH2 nanocatalyst were investigated in the condensation of malononitrile with benzaldehyde under optimal condition. After completion of the reaction, the catalyst was magnetically removed and reused in the next run under the same conditions as the first run. Based on this study, it was found that YS-MPMO/pr-NH2 can be recycled and reused for four runs without a significant decrease in its performance (Figure 10).
Next, a leaching test was performed to study the nature of catalyst under applied conditions. For this, the YS-MPMO/pr-NH2 nanocatalyst was added to a flask containing benzaldehyde and malononitrile at RT. After the reaction progressed about 50%, the catalyst was separated using an external magnet and the reaction of residue was monitored for 60 min under optimal conditions. The result demonstrated no further progress of the reaction, confirming no leaching and also heterogeneous nature of active catalytic species under applied conditions.
Finally, a comparison study was performed between the present catalyst and a number of former catalysts applied in the Knoevenagel reaction (Table 3). This showed that YS-MPMO/pr-NH2 is better than others in parameters of recovery times, reaction temperature and stability.
The mechanism of the Knoevenagel reaction is shown in Figure 11. As seen, firstly, one of the active hydrogens of malononitrile methylene is taken by YS-MPMO/pr-NH2 nanocatalyst to deliver anion I. Then, this anion, as a nucleophile, reacts with carbonyl carbon of aldehyde to give anion II. Next, this anion takes a proton from protonated catalyst to deliver intermediate III. Finally, the desired product is formed after elimination of a water molecule.
4 Conclusion
A novel amine-containing magnetic periodic mesoporous organosilica with yolk-shell structure (YS-MPMO/pr-NH2) was successfully synthesized and characterized. The TGA, EDX and FT-IR analyses showed the successful immobilization/incorporation of propylamine and methylene groups into/onto material framework. The SEM image confirmed that the YS-MPMO/pr-NH2 has a spherical morphology. Also, the superparamagnetic behavior of the YS-MPMO/pr-NH2 nanocomposite was proved by VSM analysis. The nitrogen-sorption analysis showed the presence of a shell with high surface area for the designed nanocamposite. The PXRD analysis demonstrated high stability of Fe3O4 NPs during the catalyst preparation. Examination of the catalytic activity of YS-MPMO/pr-NH2 in the Knoevenagel reaction showed that this catalyst has an excellent performance in this process. The leaching test confirmed the heterogeneous nature of active catalytic sites under applied conditions. The catalyst was also recovered and reused several times with maintaining its efficiency.
Data availability statement
The original contributions presented in the study are included in the article/supplementary material, further inquiries can be directed to the corresponding author.
Author contributions
MN: Investigation, Software, Writing–original draft. DE: Data curation, Funding acquisition, Methodology, Project administration, Writing–review and editing.
Funding
The author(s) declare that no financial support was received for the research, authorship, and/or publication of this article.
Conflict of interest
The authors declare that the research was conducted in the absence of any commercial or financial relationships that could be construed as a potential conflict of interest.
Publisher’s note
All claims expressed in this article are solely those of the authors and do not necessarily represent those of their affiliated organizations, or those of the publisher, the editors and the reviewers. Any product that may be evaluated in this article, or claim that may be made by its manufacturer, is not guaranteed or endorsed by the publisher.
References
Abaeezadeh, S., Elhamifar, D., Norouzi, M., and Shaker, M. (2019). Magnetic nanoporous MCM-41 supported ionic liquid/palladium complex: an efficient nanocatalyst with high recoverability. Appl. Organomet. Chem. 33, e4862. doi:10.1002/aoc.4862
Bai, J., Xue, J., Wang, R., Zhang, Z., and Qiu, S. (2018). Synthesis of novel Au@ Void@ Nb 2 O 5 core–shell nanocomposites with enhanced photocatalytic activity. Dalton Trans. 47, 3400–3407. doi:10.1039/c7dt04582d
Cheng, L., Liu, Y., Zou, B., Yu, Y., Ruan, W., and Wang, Y. (2017). Template-etching route to construct uniform rattle-type Fe3O4@ SiO2 hollow microspheres as drug carrier. Mater. Sci. Eng. C 75, 829–835. doi:10.1016/j.msec.2017.02.105
Croissant, J., Cattoen, X., Man, M. W. C., Gallud, A., Raehm, L., Trens, P., et al. (2014). Biodegradable ethylene-bis (Propyl) disulfide-based periodic mesoporous organosilica nanorods and nanospheres for efficient in-vitro drug delivery. Adv. Mater. 26, 6174–6180. doi:10.1002/adma.201401931
Du, Y., Huang, Z., Wu, S., Xiong, K., Zhang, X., Zheng, B., et al. (2018). Preparation of versatile yolk-shell nanoparticles with a precious metal yolk and a microporous polymer shell for high-performance catalysts and antibacterial agents. Polymer 137, 195–200. doi:10.1016/j.polymer.2017.12.069
Ghomi, J. S., and Akbarzadeh, Z. (2018). Ultrasonic accelerated Knoevenagel condensation by magnetically recoverable MgFe2O4 nanocatalyst: a rapid and green synthesis of coumarins under solvent-free conditions. Ultrason. sonochemistry 40, 78–83. doi:10.1016/j.ultsonch.2017.06.022
Ghosh, M. K., Jain, K., Khan, S., Das, K., and Ghoral, T. K. (2020). New dual-functional and reusable bimetallic Y2ZnO4 nanocatalyst for organic transformation under microwave/green conditions. ACS omega 5, 4973–4981. doi:10.1021/acsomega.9b03875
Gopalan Sibi, M., Verma, D., and Kim, J. (2020). Magnetic core–shell nanocatalysts: promising versatile catalysts for organic and photocatalytic reactions. Catal. Rev. 62, 163–311. doi:10.1080/01614940.2019.1659555
Gordel-Wojcik, M., Nyk, M., Bajorek, A., Zych, E., Samoc, M., and Jedrzejewska, B. (2022). Synthesis and optical properties of linear and branched styrylpyridinium dyes in different environments. J. Mol. Liq. 356, 119007. doi:10.1016/j.molliq.2022.119007
Haffer, S., Tiemann, M., and Froba, M. (2010). Periodic mesoporous organosilica (PMO) materials with uniform spherical core-shell structure. Chemistry–A Eur. J. 16, 10447–10452. doi:10.1002/chem.201000643
Heravi, M. M., Tehrani, M. H., Bakhtiari, K., and Oskooie, H. A. (2006). A practical Knoevenagel condensation catalysed by imidazole. J. Chem. Res. 2006, 561–562. doi:10.3184/030823406778521329
Kalantari, F., Rezayati, S., Ramazani, A., Aghahosseini, H., Ślepokura, K., and Lis, T. (2022). Proline-cu complex based 1, 3, 5-triazine coated on Fe3O4 magnetic nanoparticles: a nanocatalyst for the knoevenagel condensation of aldehyde with malononitrile. ACS Appl. Nano Mater. 5, 1783–1797. doi:10.1021/acsanm.1c03169
Kargar, S., Elhamifar, D., and Zarnegaryan, A. (2020). Core–shell structured Fe3O4@ SiO2-supported IL/[Mo6O19]: a novel and magnetically recoverable nanocatalyst for the preparation of biologically active dihydropyrimidinones. J. Phys. Chem. Solids 146, 109601. doi:10.1016/j.jpcs.2020.109601
Karimkhah, F., Elhamifar, D., and Shaker, M. (2021). Ag2CO3 containing magnetic nanocomposite as a powerful and recoverable catalyst for Knoevenagel condensation. Sci. Rep. 11, 18736. doi:10.1038/s41598-021-98287-z
Kolahdoozan, M., Kalbasi, R. J., Shahzeidi, Z. S., and Zamani, F. (2013). Knoevenagel condensation of aldehydes with ethyl cyanoacetate in water catalyzed by P4VP/Al2O3-SiO2. J. Chem. 2013, 1–8. doi:10.1155/2013/496837
Li, H., Bao, S., Li, Y., Huang, Y., Chen, J., Zhao, H., et al. (2018a). Optimizing the electromagnetic wave absorption performances of designed Co3Fe7@ C yolk–shell structures. ACS Appl. Mater. interfaces 10, 28839–28849. doi:10.1021/acsami.8b08040
Li, J., Yan, D., Hou, S., Lu, T., Yao, Y., Chua, D. H., et al. (2018b). Metal-organic frameworks derived yolk-shell ZnO/NiO microspheres as high-performance anode materials for lithium-ion batteries. Chem. Eng. J. 335, 579–589. doi:10.1016/j.cej.2017.10.183
Li, X.-P., Deng, Z., Li, Y., Zhang, H.-B., Zhao, S., Zhang, Y., et al. (2019a). Controllable synthesis of hollow microspheres with Fe@ Carbon dual-shells for broad bandwidth microwave absorption. Carbon 147, 172–181. doi:10.1016/j.carbon.2019.02.073
Li, Y., Tang, Y., Chen, S., Liu, Y., Wang, S., Tian, Y., et al. (2019b). Sequential therapy for pancreatic cancer by losartan-and gemcitabine-loaded magnetic mesoporous spheres. RSC Adv. 9, 19690–19698. doi:10.1039/c9ra02180a
Lin, L. S., Song, J., Yang, H. H., and Chen, X. (2018). Yolk–Shell nanostructures: design, synthesis, and biomedical applications. Adv. Mater. 30, 1704639. doi:10.1002/adma.201704639
Liu, J., You, W., Yu, J., Liu, X., Zhang, X., Guo, J., et al. (2019). Electron holography of yolk–shell Fe3O4@ mSiO2 microspheres for use in microwave absorption. ACS Appl. Nano Mater. 2, 910–916. doi:10.1021/acsanm.8b02150
Lu, N., Tian, Y., Tian, W., Huang, P., Liu, Y., Tang, Y., et al. (2016). Smart cancer cell targeting imaging and drug delivery system by systematically engineering periodic mesoporous organosilica nanoparticles. ACS Appl. Mater. interfaces 8, 2985–2993. doi:10.1021/acsami.5b09585
Maleki, A., Alrezvani, Z., and Maleki, S. (2015). Design, preparation and characterization of urea-functionalized Fe3O4/SiO2 magnetic nanocatalyst and application for the one-pot multicomponent synthesis of substituted imidazole derivatives. Catal. Commun. 69, 29–33. doi:10.1016/j.catcom.2015.05.014
Maleki, R., Kolvari, E., Salehi, M., and Koukabi, N. (2017). Fe3O4–cysteamine hydrochloride magnetic nanoparticles: new, efficient and recoverable nanocatalyst for Knoevenagel condensation reaction. Appl. Organomet. Chem. 31, e3795. doi:10.1002/aoc.3795
Mirbagheri, R., Elhamifar, D., and Hajati, S. (2021). Ru-containing magnetic yolk–shell structured nanocomposite: a powerful, recoverable and highly durable nanocatalyst. RSC Adv. 11, 10243–10252. doi:10.1039/d0ra10304g
Nagaraju, G., Sekhar, S. C., Raju, G. S. R., Bharat, L. K., and Yu, J. S. (2017). Designed construction of yolk–shell structured trimanganese tetraoxide nanospheres via polar solvent-assisted etching and biomass-derived activated porous carbon materials for high-performance asymmetric supercapacitors. J. Mater. Chem. A 5, 15808–15821. doi:10.1039/c7ta04314g
Neysi, M., and Elhamifar, D. (2023). Yolk-shell structured magnetic mesoporous organosilica supported ionic liquid/Cu complex: an efficient nanocatalyst for the green synthesis of pyranopyrazoles. Front. Chem. 11, 1235415. doi:10.3389/fchem.2023.1235415
Neysi, M., Elhamifar, D., and Norouzi, M. (2020). Ionic liquid functionalized magnetic organosilica nanocomposite: a powerful and efficient support for manganese catalyst. Mater. Chem. Phys. 243, 122589. doi:10.1016/j.matchemphys.2019.122589
Purbia, R., and Paria, S. (2015). Yolk/shell nanoparticles: classifications, synthesis, properties, and applications. Nanoscale 7, 19789–19873. doi:10.1039/c5nr04729c
Rosso, C., Filippini, G., and Prato, M. (2020). Carbon dots as nano-organocatalysts for synthetic applications. ACS Catal. 10, 8090–8105. doi:10.1021/acscatal.0c01989
Safapoor, S., Dekamin, M. G., Akbari, A., and Naimi-Jamal, M. R. (2021). Synthesis of (E)-2-(1H-tetrazole-5-yl)-3-phenylacrylenenitrile derivatives under green conditions catalyzed by new thermally stable magnetic periodic mesoporous ognosilica embedded with ZnO nanoparticles.
Şen, B., Akdere, E. H., Şavk, A., Gultekin, E., Parali, Ö., Goksu, H., et al. (2018). A novel thiocarbamide functionalized graphene oxide supported bimetallic monodisperse Rh-Pt nanoparticles (RhPt/TC@ GO NPs) for Knoevenagel condensation of aryl aldehydes together with malononitrile. Appl. Catal. B Environ. 225, 148–153. doi:10.1016/j.apcatb.2017.11.067
Shaker, M., and Elhamifar, D. (2020). Magnetic methylene-based mesoporous organosilica composite-supported IL/Pd: a powerful and highly recoverable catalyst for oxidative coupling of phenols and naphthols. Mater. Today Chem. 18, 100377. doi:10.1016/j.mtchem.2020.100377
Shaker, M., and Elhamifar, D. (2021). Cu-containing magnetic yolk-shell structured ionic liquid-based organosilica nanocomposite: a powerful catalyst with improved activity. Compos. Commun. 24, 100608. doi:10.1016/j.coco.2020.100608
Sun, Q., Zhang, X.-Q., Wang, Y., and Lu, A.-H. (2015). Recent progress on core-shell nanocatalysts. Chin. J. Catal. 36, 683–691. doi:10.1016/s1872-2067(14)60298-9
Wang, G.-H., Chen, K., Engelhardt, J., Tuysuz, H., Bongard, H.-J., Schmidt, W., et al. (2018). Scalable one-pot synthesis of yolk–shell carbon nanospheres with yolk-supported Pd nanoparticles for size-selective catalysis. Chem. Mater. 30, 2483–2487. doi:10.1021/acs.chemmater.8b00456
Wang, Y., Yang, C., Chen, A., Pu, W., and Gong, J. (2019). Influence of yolk-shell Au@ TiO2 structure induced photocatalytic activity towards gaseous pollutant degradation under visible light. Appl. Catal. B Environ. 251, 57–65. doi:10.1016/j.apcatb.2019.03.056
Wei, Y., Li, X., Elzatahry, A. A., Zhang, R., Wang, W., Tang, X., et al. (2016). A versatile in situ etching-growth strategy for synthesis of yolk–shell structured periodic mesoporous organosilica nanocomposites. RSC Adv. 6, 51470–51479. doi:10.1039/c6ra08541e
Xie, Q., Lin, L., Ma, Y., Zeng, D., Yang, J., Huang, J., et al. (2017). Synthesis of ZnO-Cu-C yolk-shell hybrid microspheres with enhanced electrochemical properties for lithium ion battery anodes. Electrochimica Acta 226, 79–88. doi:10.1016/j.electacta.2016.12.187
Yu, K., Ling, M., Liang, J., and Liang, C. (2019a). Formation of TiO2 hollow spheres through nanoscale Kirkendall effect and their lithium storage and photocatalytic properties. Chem. Phys. 517, 222–227. doi:10.1016/j.chemphys.2018.10.022
Yu, L., Pan, P., Zhang, Y., Zhang, Y., Wan, L., Cheng, X., et al. (2019b). Nonsacrificial self-template synthesis of colloidal magnetic yolk–shell mesoporous organosilicas for efficient oil/water interface catalysis. Small 15, 1805465. doi:10.1002/smll.201805465
Zhang, L., Liu, L., Liu, M., Yu, Y., Hu, Z., Liu, B., et al. (2019). Controllable synthesis of N-doped hollow, yolk-shell and solid carbon spheres via template-free method. J. Alloys Compd. 778, 294–301. doi:10.1016/j.jallcom.2018.11.169
Zhang, Q., Ma, X.-M., Wei, H.-X., Zhao, X., and Luo, J. (2017). Covalently anchored tertiary amine functionalized ionic liquid on silica coated nano-Fe 3 O 4 as a novel, efficient and magnetically recoverable catalyst for the unsymmetrical Hantzsch reaction and Knoevenagel condensation. RSC Adv. 7, 53861–53870. doi:10.1039/c7ra10692k
Keywords: nanocatalyst, magnetic nanoparticles, mesoporous organosilica, yolk-shell structured nanocomposite, Knoevenagel reaction
Citation: Neysi M and Elhamifar D (2024) Amine-containing yolk-shell structured magnetic organosilica nanocomposite as a highly efficient catalyst for the Knoevenagel reaction. Front. Chem. 12:1336855. doi: 10.3389/fchem.2024.1336855
Received: 11 November 2023; Accepted: 23 January 2024;
Published: 06 February 2024.
Edited by:
Behrooz Maleki, University of Mazandaran, IranReviewed by:
Chinnarajesh Ummadisetti, Amplified Science, United StatesTahir Farooq, Government College University, Faisalabad, Pakistan
Copyright © 2024 Neysi and Elhamifar. This is an open-access article distributed under the terms of the Creative Commons Attribution License (CC BY). The use, distribution or reproduction in other forums is permitted, provided the original author(s) and the copyright owner(s) are credited and that the original publication in this journal is cited, in accordance with accepted academic practice. No use, distribution or reproduction is permitted which does not comply with these terms.
*Correspondence: Dawood Elhamifar, ZGF3b29kLmVsaGFtaWZhckBnbWFpbC5jb20=