- 1Functional Ecology and Environment Engineering Laboratory, Faculty of Science and Technology, Sidi Mohamed Ben Abdellah University, Fez, Morocco
- 2Medicinal Aromatic and Poisonous Plants Research Centre, College of Pharmacy, King Saud University, Riyadh, Saudi Arabia
- 3Department of Horticulture, Faculty of Horticulture, Ataturk University, Erzurum, Türkiye
- 4HGF Agro, Ata Teknokent, Erzurum, Türkiye
For many years, chitosan has been widely regarded as a promising eco-friendly polymer thanks to its renewability, biocompatibility, biodegradability, non-toxicity, and ease of modification, giving it enormous potential for future development. As a cationic polysaccharide, chitosan exhibits specific physicochemical, biological, and mechanical properties that depend on factors such as its molecular weight and degree of deacetylation. Recently, there has been renewed interest surrounding chitosan derivatives and chitosan-based nanocomposites. This heightened attention is driven by the pursuit of enhancing efficiency and expanding the spectrum of chitosan applications. Chitosan’s adaptability and unique properties make it a game-changer, promising significant contributions to industries ranging from healthcare to environmental remediation. This review presents an up-to-date overview of chitosan production sources and extraction methods, focusing on chitosan’s physicochemical properties, including molecular weight, degree of deacetylation and solubility, as well as its antibacterial, antifungal and antioxidant activities. In addition, we highlight the advantages of chitosan derivatives and biopolymer modification methods, with recent advances in the preparation of chitosan-based nanocomposites. Finally, the versatile applications of chitosan, whether in its native state, derived or incorporated into nanocomposites in various fields, such as the food industry, agriculture, the cosmetics industry, the pharmaceutical industry, medicine, and wastewater treatment, were discussed.
1 Introduction
Today, the range of applications for biopolymers has broadened with the demand for innovative and environmentally friendly materials offering enhanced properties. Chitin, the parent compound of chitosan and the second most abundant biopolymer in nature after cellulose, is a linear polysaccharide composed of poly-β-[1,4]-N-acetyl-D-glucosamine units (Kou et al., 2022). This green polymer can be obtained from a variety of sources especially crustacean exoskeletons and its extraction requires several steps including demineralization, deproteinization, and decolorization (Jiménez-Gómez and Cecilia, 2020). The structure of completely acetylated chitin limits its applications due to its high thermochemical stability and its high insolubility in aqueous solutions and most organic solvents (Khajavian et al., 2022). However, chitosan is defined as the N-deacetylated form of chitin obtained by a chemical or enzymatic deacetylation process, and is also found naturally in the cell walls of certain fungi. It is a linear copolymer composed of repeated hydrophilic units (D-glucosamine units) and residual hydrophobic units (N-acetyl-D-glucosamine units) (Yarnpakdee et al., 2022). Molecular weight (Mw) and degree of deacetylation (DD) are the most characteristic features that influence the structure-function relationships of chitosan and its derivatives (Joseph et al., 2021). The solubility of chitosan is mainly linked to the amino groups along the polymer chain, which are fully protonated under acidic conditions, making chitosan highly soluble. This solubility generally increases with increasing degree of deacetylation and decreasing molecular weight (Mukhtar et al., 2021). Due to its cationic nature, chitosan has good biological activities such as bacteriostatic, antioxidant, antitumor, anti-inflammatory and antifungal activity (Aranaz et al., 2021; Ke et al., 2021). This natural bioactive polymer exhibits high biocompatibility, excellent biodegradability, exceptional functionality, cost-effectiveness, and non-toxicity, making it useful and powerful in various engineering applications. Figure 1 shows a schematic illustration from the origin of chitosan to its potential uses.
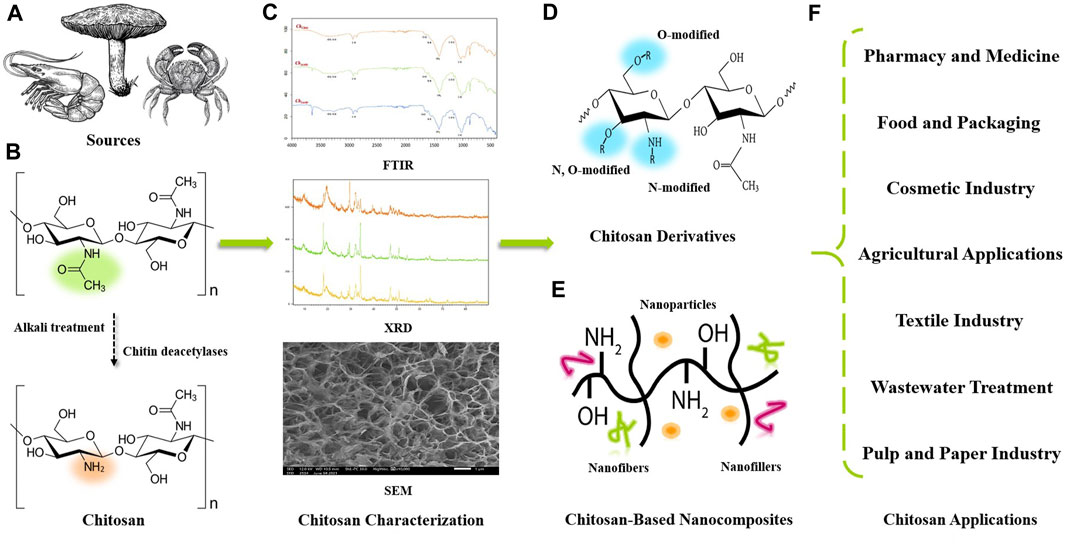
FIGURE 1. Schematic illustration from chitosan origin to potential applications in different emerging fields. (A) Various chitosan sources. (B) Conversion from chitin to chitosan by chemical or enzymatic deacetylation process. (C) Chitosan characterization by FTIR, XRD, and SEM. (D) N; O; or N, O-modified chitosan derivatives form. (E) Chitosan-based nanocomposites preparation. (F) Various chitosan engineering applications.
The presence of functional groups, amino (-NH2) and hydroxyl (-OH), in chitosan is a key attribute for its versatile chemical modification to improve its physicochemical, mechanical, and biological properties while maintaining its unique characteristics (Negm et al., 2020). Moreover, researchers have actively pursued the development of various chitosan derivatives to introduce innovative functions or properties to the material. The rapid strides in nanotechnology have notably intensified the focus on chitosan, positioning it as a highly sought-after matrix for nanocomposites. This heightened attention has not only bolstered the material’s efficiency but has also broadened its application spectrum across diverse fields. The synergy of chitosan and nanotechnology holds immense promise, unlocking new possibilities for advancements in materials science and technology (Yu et al., 2021). Chitosan, whether in its native state, derived or incorporated into nanocomposites, is an indispensable element in food packaging and preservation. This indispensability is attributed to its remarkable film-forming ability and outstanding antimicrobial properties. Chitosan’s inherent versatility enables it not only to improve the structural integrity of packaging materials, but also to act as an effective barrier to microbial activity, making a significant contribution to extending the shelf life of various food products (Hameed et al., 2022; Oladzadabbasabadi et al., 2022). Consequently, the application of chitosan in various forms underlines its crucial role in ensuring the safety and longevity of packaged foods. Chitosan and its derivatives, as well as chitosan-based nanocomposites, have attracted considerable scientific attention in the agricultural sector, mainly due to their notable impact on stimulating plant growth. This increased interest testifies to the versatility of chitosan’s applications in promoting agricultural sustainability and productivity (M. Zhang et al., 2022). The unique characteristics of chitosan, whether in its original form or in the form of modified derivatives and nanocomposites, have promoted its popularity for a variety of applications in different biomedical fields. These include its essential role as a diagnostic and therapeutic tool, as well as its incorporation into cosmeceuticals and cosmetics (Gao and Wu, 2022; Gupta et al., 2022). In the world of biopolymers, chitosan, in derived or nanocombined form, stands out for its relevance in a variety of absorption-related applications. These include its effectiveness in removing heavy metal ions and anionic dyes (Haripriyan et al., 2022). In summary, the versatility of chitosan allows it to be tailored to specific goals across a wide range of applications, making it a valuable material in many industries. This article aims to present an updated overview of chitosan production sources and extraction methods. The physicochemical and functional properties of the biopolymer were also emphasized. In addition, the review highlights methods for chitosan modification and the preparation of chitosan-based nanocomposites. This paper also gathers updated knowledge on the versatile applications of chitosan and its derivatives in diverse fields, such as the food industry, agriculture, the cosmetic industry, the pharmaceutical industry, medicine, and wastewater treatment.
2 Sources of chitosan production
Chitin is a polysaccharide naturally found in the exoskeletons of crustaceans, insects and mollusks, and in the cell walls of certain algae and fungi (Santos et al., 2020; Pellis et al., 2022). In addition, chitin is associated with other constituents such as minerals, proteins, lipids and pigments, whatever the initial extraction source. In general, the chitin content varies on average from 20% to 30% in crustacean exoskeletons (Kou et al., 2021), from 5% to 25% in insect cuticles (Zainol Abidin et al., 2020), and from 2% to 44% in fungi cell walls (Abo Elsoud and El Kady, 2019). The α-chitin, β-chitin and γ-chitin are the three allomorphic crystalline forms of chitin that differ in the unit size, number of chains, and degree of hydration. The α-microfibril is the most stable crystalline form of chitin and is found mainly in the exoskeleton of arthropods (Pellis et al., 2022). Shell waste from the seafood industry is a valuable source of raw materials and can be utilized to generate high value-added co-products and reduce environmental impacts, thus ensuring the sustainable development of the seafood industry. These economic and environmental advantages favor the utilization of crustacean sources, in particular crab and shrimp shells, for chitin and chitosan extraction, due to their availability as waste products and ease of extraction. Shrimp shells contain on average 30%–40% chitin, while crab shells contain 15%–30% (Pakizeh et al., 2021; Terkula Iber et al., 2022). Previous studies have shown that the source of the polymer and the extraction process have a direct impact on the physicochemical and biological properties of chitosan.
3 Chitosan extraction methods
3.1 Chemical extraction
Chitin is found mainly in association with minerals, proteins, glucans, pigments, and lipids. The abundance of these components varies according to the source and species of chitin. This variation requires a special extraction process that involves demineralization, deproteinization, and discoloration steps (Figure 2). The extraction of chitosan from crustacean sources is widely discussed in the literature. Demineralization of shellfish is a critical step due to the presence of minerals. This process occurs via the decomposition of calcium carbonate into calcium chloride, resulting in the release of carbon dioxide through acid treatment (Santos et al., 2020). According to the literature, sulfuric, hydrochloric, formic, acetic, oxalic, and nitric acids are generally used for their effectiveness in removing inorganic salts (Srinivasan et al., 2018). Efforts have been made to replace these mineral acids with more environmentally friendly organic acids. El-araby et al. (2022a) developed an ecological and economical demineralization step for chitosan extraction from shrimp shell waste using citric, acetic, and lactic acids. The results showed that during the demineralization phase, mineral acids can be replaced by organic acids for environmentally friendly extraction. The elimination of associated proteins is an important step in the chitin purification process. The deproteinization step consists in destroying the chemical bonds linking proteins and chitin by means of an alkaline treatment (Yadav et al., 2019). This step is usually done by strong bases, mostly with NaOH, at high temperatures and during a long incubation time (Pellis et al., 2022). Discoloration or bleaching is an optional step in the extraction process to remove pigments naturally present in chitin sources. This step requires the use of organic or inorganic solvents such as sodium hypochlorite, hydrogen peroxide, and acetone (El Knidri et al., 2018). Deacetylation is a final step in the extraction of chitosan and consists of the elimination of acetyl functional groups (-COCH3) from the chitin linear chain, with the release of amino groups (-NH2). The deacetylation process is generally carried out by heat treatment with a concentrated alkali, such as sodium hydroxide or potassium hydroxide (Kou et al., 2021). The proportion of acetylated versus deacetylated glucosamine units is important in determining the balance between hydrophilic and hydrophobic residues. Based on this, chitin with at least 75% deacetylation degree is called chitosan (Confederat et al., 2021).
3.2 Biological extraction
Microbial fermentation and enzymatic processing have been developed to overcome these problems and produce chitosan from crustacean by-products in a more environmentally friendly and cost-effective manner. The enzymatic extraction can be substituted during the deproteinization and the deacetylation steps to replace alkalis and high reaction temperatures with enzymes (Marzieh et al., 2019). Nevertheless, this method shares the similar demineralization process as chemical methods (Figure 2). Various proteases have been used for the enzymatic deproteinization of chitin, and these enzymes are generally extracted from fish viscera or microbes (Kou et al., 2021). In addition, various deacetylases derived from various biological sources such as bacteria, fungi, and certain insects have been employed for the enzymatic deacetylation step of chitin to obtain chitosan (Pellis et al., 2022). Extraction by microbial fermentation can be used in deproteinization, demineralization, and deacetylation steps, using proteases and lactic acid-producing bacteria (Doan et al., 2019). These fermentation processes for chitin extraction exploit the ability of bacteria to produce proteases such as Pseudomonas aeruginosa, Pseudomonas maltophilia, Bacillus subtilis, and Serratia marcescens (Navarrete-Bolaños et al., 2020).
3.3 Advantages and disadvantages of the two extraction methods
The chemical method produces chitosan with a high degree of deacetylation, medium to low molecular weight, and stronger biological properties. This method also involves a short treatment time and can therefore be easily applied on an industrial scale. This multi-step process, including the deacetylation step, implies the usage of strong bases and acids, which causes negative effects on the environment, diminishes the economic sustainability of the extraction process and often leads to a decrease in the quality of the resulting product. Furthermore, biological methods have significant limitations. The most important is the high cost of the enzymes involved in the enzymatic processing, which usually requires one or more different enzymes for deproteinization and deacetylation. Microbial fermentation indeed has the advantage of reducing the high cost of enzymes, but it generally requires specific microbial strains. Thus, unlike chemical extraction, biological extraction requires a long processing time (several days), which limits its use on an industrial scale. Despite the progress made to develop efficient and eco-friendly chitosan extraction methods, chemical extraction is the preferred method to date, due to the availability of chemicals and the possibility of industrial scale-up.
4 Physicochemical properties
4.1 Deacetylation degree
Chitosan is composed of three reactive functional groups, an amino group, primary hydroxyl group, and secondary hydroxyl group in each glycosidic unit. However, the -NH2 group is responsible for the cationic nature and physicochemical properties of chitosan thanks to its intramolecular and intermolecular hydrogen bonds (Bakshi et al., 2020). The physicochemical properties of chitosan depend on many factors, mainly the deacetylation degree (DD), the molecular weight (Mw), the solubility, and the degree of crystallinity. The distinctive feature of chitosan consists on its high DD and its low proportion of crystalline zones (Azmana et al., 2021; M. Zhang et al., 2022). The deacetylation degree of chitosan, the ratio of D-glucosamine (deacetylated unit) and N-acetyl-D-glucosamine (acetylated unit) structural units, increases proportionally with increasing alkali treatment time. As it is known, chitosan is a deacetylated form of chitin, with at least 75% deacetylation degree (Confederat et al., 2021). A high degree of deacetylation reflects a higher concentration of free -NH2 groups in the polymer chain and allows the molecule to exhibit enhanced biological activities and higher water solubility due to protonation of the amino functional group (Abd El-Hack et al., 2020). The degree of deacetylation of chitosan is one of the critical parameters influencing its physicochemical, mechanical, and biological properties and, therefore, its application spectrum. Table 1 summarizes sources, extraction methods, and physicochemical properties (yield, DD, solubility, and Mw) of chitosan obtained in various studies.
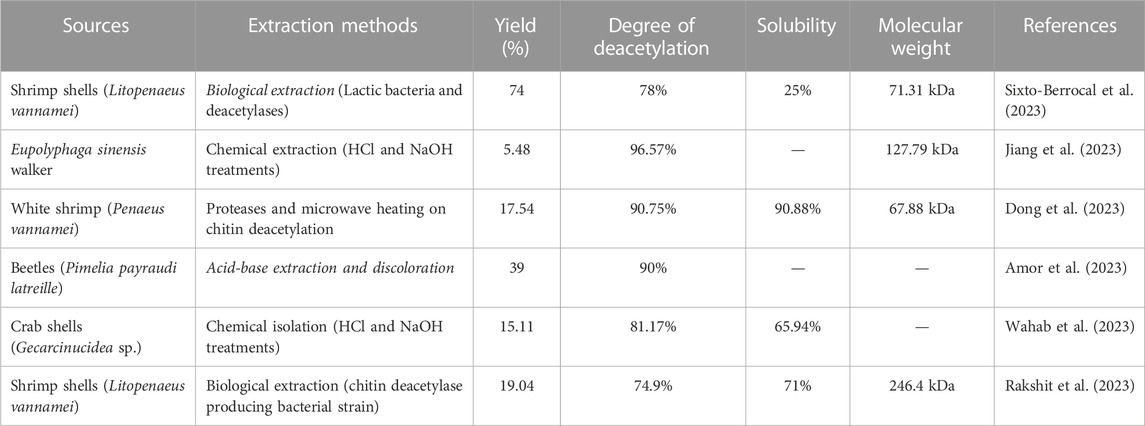
TABLE 1. Sources, extraction methods, and physicochemical properties of chitosan obtained in various studies.
4.2 Molecular weight
Besides the degree of deacetylation, the molecular weight (Mw) is another key factor that significantly affects the physicochemical properties of chitosan and, therefore, its biofunctionality and biological activities (Kou et al., 2021). Polymer viscosity is a parameter of considerable practical interest, as highly viscous solutions are difficult to handle, and it decreases, as the Mw of chitosan is reduced (Aranaz et al., 2021). Several studies have revealed that chitosan with a lower Mw and a higher DD generally exhibits higher bioactivities. In addition, previous studies have described different molecular weight threshold values for differentiating between high and low molecular weight chitosans. High Mw chitosans ranged from 190 kDa to 375 kDa, while low Mw chitosans ranged from 20 kDa to 190 kDa (Azmana et al., 2021). In general, the difference in molecular weight is related to the extraction process and the initial source of chitosan (Bakshi et al., 2020). Chitosan oligosaccharides (COS), degraded polymer products of polymer obtained by physical, enzymatic, or chemical hydrolysis, have gained attention in recent years owing to their lower Mw, higher DD, lower polymerization degree, low viscosity and greater water solubility. These physicochemical properties have significant beneficial effects and strong potential for biomedical, pharmacological, and industrial applications (Benchamas et al., 2021). Molecular weight has a major influence on the rheological properties of chitosan, which has a direct impact on the formulation of chitosan-based nanocomposites.
4.3 Solubility
The higher the DD of chitosan, the greater the degree of protonation of the -NH2 groups in the linear molecular chain, which facilitates its dissolution in acidic aqueous media, since its pKa value is around 6.5. This solubility is also influenced by the degree of deacetylation of chitosan. However, the higher the Mw of chitosan, the greater the number of intramolecular and intermolecular hydrogen bonds formed in its molecular chain, making it intertwined and therefore difficult to dissolve (Wang et al., 2020a; Aranaz et al., 2021). In addition, the polymer solubility depends on the position of acetyl groups along the linear chain, deacetylation procedure, and ionic strength. Various studies have shown that chitosan has great solubility when the degree of deacetylation exceeds 85% (M. Zhang et al., 2022). The low solubility of chitin in water and most organic solvents has limited its uses and applications. In contrast, the presence of -OH and -NH2 functional active groups in chitosan allows the formulation of a variety of derivatives that enhance its solubility, and therefore, increase the spectrum of applications in various fields. Carboxymethylation is an alternative method to improve the solubility of the biopolymer in aqueous solution (Jiménez-Gómez and Cecilia, 2020). Similarly, the quaternization of chitosan increased its solubility compared to unmodified chitosan (Wei et al., 2019). Chemical modification can both improve the physicochemical characteristics and biological properties of chitosan while retaining its unique properties and extend the range of chitosan derivatives applications.
5 Functional properties
5.1 Antibacterial activity
Chitosan is known as one of the most effective antibacterial substances because of its potential antibacterial properties. This biopolymer has been shown in previous studies to have antimicrobial activity against a wide range of Gram-positive bacteria (Savin et al., 2020; Valdez et al., 2022) and Gram-negative bacteria (Sudatta et al., 2020; Xing et al., 2021). The antibacterial properties of chitosan are influenced by numerous factors such as the molecular weight (Mw) of chitosan, the degree of deacetylation (DD), the polymer concentration, the initial source of chitosan, the pH value, and the storage temperature of the chitosan solution. Table 2 summarizes studies on chitosan’s antibacterial activity with respect to its DD and Mw. Likewise, it was found that the type of microorganism and the cell growth phase have a direct effect on the antibacterial potential of chitosan (Li and Zhuang, 2020; Aranaz et al., 2021). According to the literature, the higher the degree of deacetylation of chitosan, the stronger its antibacterial activity. Additionally, studies have shown that the antibacterial properties of chitosan solutions are inversely proportional to their molecular weight. This activity could increase with increasing molecular weight in an acidic medium and decrease in a neutral medium. This shows that the antimicrobial activity could be affected by the pH of its dissolution solution (Benhabiles et al., 2012; Chang et al., 2015). The main mechanism of action of chitosan’s antimicrobial activity is its interaction with the cell wall, cell membrane, and cytoplasmic constituents of bacteria (Abd El-Hack et al., 2020). The type of microorganism is known to have a significant influence on the antibacterial capacity of chitosan and its derivatives. The interaction of positively charged chitosan with the negatively charged microbial membrane of Gram-negative bacteria via electrostatic interactions could increase wall permeability by replacing divalent cations (Ca2+, Mg2+) from their binding sites and reducing the interaction between lipopolysaccharide molecules, thereby causing membrane rupture and cell lysis, leading to bacterial death. For Gram-positive bacteria, the absence of the outer membrane leads to a direct diffusion of chitosan into the bacterial cell wall (Duan et al., 2019; Wang et al., 2020a). This may explain the hypothesis that chitosan is more powerful against Gram-positive bacteria than Gram-negative bacteria.
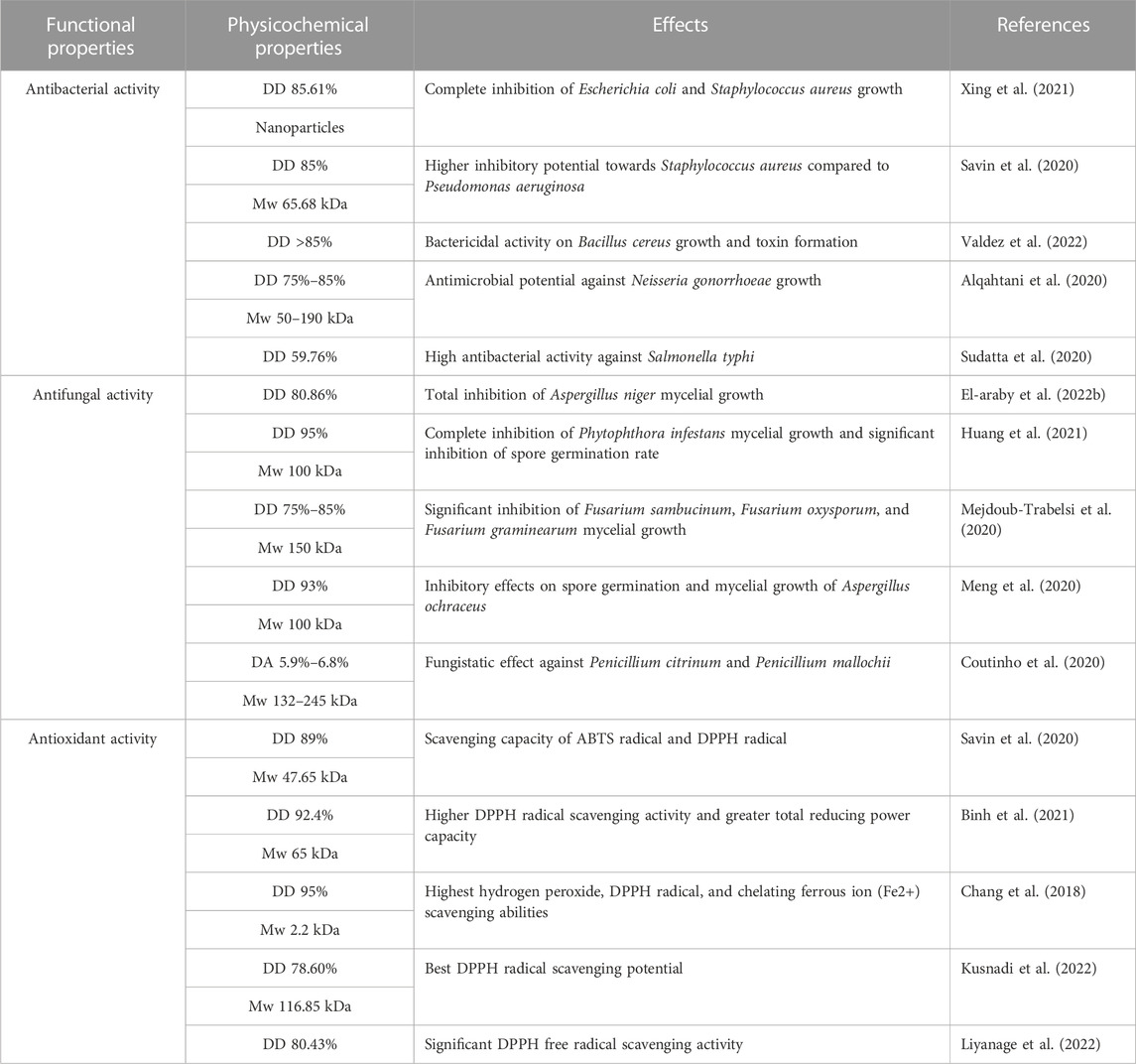
TABLE 2. Studies on chitosan’s antibacterial, antifungal, and antioxidant activity with respect to its DD and Mw.
5.2 Antifungal activity
Various studies have reported that chitosan exhibits exceptional antifungal properties (Shih et al., 2019; Ashrafi et al., 2020) against a broad spectrum of molds and yeasts (Bernabé et al., 2020; Mejdoub-Trabelsi et al., 2020). The degree of deacetylation and the molecular weight are two parameters that play a critical role in controlling the antifungal properties of chitosan. Table 2 summarizes studies on chitosan’s antifungal activity with respect to its DD and Mw. In addition to its antifungal activity, low-molecular-weight chitosan is capable of penetrating the cell wall and surface, inhibiting DNA/RNA and protein synthesis (Kravanja et al., 2019). Similarly, the minimum inhibitory concentrations of chitosan against fungi were strongly correlated with the pH of the solvent and the type of fungus targeted (Garcia et al., 2018; Lopez-Moya et al., 2019). The antifungal activity of chitosan also depends on the concentration of the biopolymer. Studies have reported that concentrations of chitosan between 1% and 5% have been revealed to provide optimal antifungal activity Confederat et al., 2021). Chitosan is reported in the literature to have strong antifungal activity against chitosan-sensitive fungi, and the hypothesis predicts that positively charged chitosan can interact with negatively charged phospholipids in the fungal cell membrane, provoking membrane damage and entry into the cytoplasm (Verlee et al., 2017; Azmana et al., 2021). Chitosan-resistant fungi cell membrane constitutes a barrier to the polymer, which remains on the outer surface. In general, chitosan antifungal potential is considered to be fungistatic rather than fungicidal, and highly effective in inhibiting spore germination, radial growth, and germ tube elongation (Qin et al., 2020). The modification of chitosan is easily achievable thanks to the presence of -OH and -NH2 groups, which enhances the polymer’s antimicrobial properties and therefore its antimicrobial range.
5.3 Antioxidant activity
Chitosan and its derivatives have been shown to be powerful and potential antioxidants (Binh et al., 2021; Kusnadi et al., 2022). This antioxidant action appears to be highly correlated with the characteristics of the chitosan studied, and is closely related to the degree of deacetylation and molecular weight. Table 2 summarizes studies on chitosan’s antioxidant activity with respect to its DD and Mw. Chitosans with a lower molecular weight or a higher degree of deacetylation would have better antioxidant activity (Wang et al., 2020b). The NH2 and OH functional groups of this polymer are responsible for free radical scavenging and metal chelation (Chang et al., 2018; Aranaz et al., 2021). Chitosan antioxidant activity has been established by its strong hydrogen-donating capacity. Chitosan’s antioxidant action consists in protecting the target organism from oxidative stress-induced damage by interrupting the oxidation chain reaction (Muthu et al., 2021). Chitosan’s stability and reactivity are enhanced by chelation with another, more powerful antioxidant (Kadam et al., 2018; H. Zhang et al., 2018). For example, phenolic acids are perfect antioxidants, but they degrade rapidly in the body. Binding a slow-digesting compound such as chitosan inhibits its premature degradation by reducing its hydrophilicity (Carocho et al., 2018). It helps to stabilize phenolic compounds while maintaining biological chitosan properties. Chitosan chemical modification is possible due to its unique structure on which other active groups can be introduced to improve its solubility and bioactivity and generate new active functions (Qing et al., 2019; Sun et al., 2019).
6 Chitosan modification: Chitosan derivatives
Chitosan is a versatile polymer that opens the possibility of various chemical modifications to produce a wide range of chitosan derivatives thanks to its hydroxyl and amino functional active groups. These modifications represent an effective way of improving the physicochemical characteristics of chitosan, while retaining its unique biological properties, leading to its increasingly widespread application in many fields (Jiménez-Gómez and Cecilia, 2020). The chemical structure of chitosan is characterized by NH2, primary OH, and secondary OH groups in positions C2, C6, and C3, respectively (Aranaz et al., 2021). The C3-OH group has a high resistance to the space site and is, therefore, relatively difficult to modify. In general, the order of reactivity of chitosan functional groups is as follows: C2-NH2> C6-OH> C3-OH (M. Zhang et al., 2022). Chemical modification of chitosan can occur at one of the active sites or equally at both sites to form N-, O-, or N, O-modified chitosan derivatives, and this can be achieved by a variety of chemical reactions (Figure 3). Chen et al. (2022) discuss the latest advances in chitosan chemical modification methods and review the uses of chitosan and its derivatives in several fields. The choice of modification depends on the desired properties and the intended use of the modified chitosan.
These modifications can be tailored to suit the properties required for specific applications in fields such as biomedicine, agriculture, food technology, wastewater treatment, and more. Here are some common chemical modifications of chitosan, for example, the alkylation reaction occurs by introducing an alkyl group onto C2-NH2 (N-alkylation) or onto C6-OH (O-alkylation) of the chitosan. The addition of an alkyl group weakens intermolecular hydrogen bonds, improving the solubility of the resulting molecule (Azmana et al., 2021). Phosphorylated chitosan is another derivative that can be obtained by reacting the polymer with phosphorus pentoxide in the presence of methane sulfonic acid at low temperature. The phosphorylated form of chitosan is recognized for its high water solubility, ability to chelate metals, and bactericidal properties (Negm et al., 2020). Quaternization of chitosan is a chemical modification achieved by incorporating quaternary ammonium groups or small-molecule quaternary ammonium salts into the C6-OH or C2-NH2 group. Quaternized chitosan exhibits greater solubility and antimicrobial properties than normal chitosan, favoring its use in biomedical fields (Wei et al., 2019). Thiolated chitosan is a derivative produced by the formation of a covalent bond between the thiol group (-SH) and the C2-NH2 group of chitosan, giving it high solubility in water compared with unmodified chitosan (Liu et al., 2021). In addition to the chemical processes described above to boost chitosan functional properties, cross-linking is a chemical process that can take place between molecules or inside the molecule and provides the polymer derivative greater stability through covalent bonding with the cross-linker (Saheed et al., 2021).
As research continues, the physical modification of chitosan is gaining importance to develop a new biomaterial with unique and distinctive physical characteristics to meet the needs of various applications. Physical modifications typically involve changes in the structure, morphology, or processing conditions of chitosan without introducing chemical alterations. This type of modification can be carried out by a variety of processes such as mechanical grinding, ultrasonic treatment, ionizing radiation, particle size reduction, blending, hydrogel formation, freeze-drying (Lyophilization), physical cross-linking, and heat treatment (Wang et al., 2020b). The biopolymer enzymatic modification is also possible for an ecological and less energy-consuming modification. The process involves the use of enzymes to introduce specific changes to the chitosan structure, often in a controlled and mild manner. Enzymes can selectively modify functional groups on chitosan, providing a route to tailor its biological properties for various applications (Bakshi et al., 2020). These enzymatic approaches offer a sustainable and biocompatible way of adapting chitosan to specific applications in fields such as biomedicine, food technology, and materials science, but they are still under development.
7 Chitosan-based nanocomposites
The presence of functional groups allows chitosan to be easily accessible to other biomaterials or active compounds, including biopolymers and metal ions, to form chitosan-based bionanocomposites. The availability and functionality make chitosan a promising candidate in the advanced field of nanotechnology. Chitosan-based nanocomposites are materials that combine chitosan with nanoscale additives such as nanoparticles, nanofillers and nanofibers (Figure 4) under controlled conditions to create a new material with enhanced properties (Azmana et al., 2021). These nanocomposites often exhibit improved mechanical strength, thermal stability, barrier properties and, other functionalities, making them suitable for a wide range of applications (Yu et al., 2021). Chitosan-based nanocomposites are synthesized by a variety of production processes, including solution casting, in situ synthesis, electrospinning, freeze-drying, layer-by-layer assembly, emulsion techniques, and the sol-gel method (Azmana et al., 2021). Each method offers unique advantages and allows for the tailoring of chitosan-based nanocomposites for various purposes. In fact, ongoing research is aimed at continuously improving production methods for chitosan-based nanocomposites, in order to increase their efficiency, properties, and applicability.
Chitosan-based nanocomposites offer a versatile platform for tailoring materials with enhanced properties for specific applications, ranging from medicine to packaging and beyond. The choice of nanoscale additive depends on the desired properties and the intended use of the nanocomposite. For example, chitosan-based nanocomposites obtained with cerium IV - zirconium IV oxide nanoparticles have shown remarkable improvements in morphological, structural, thermal, and mechanical properties. These nanocomposites, thanks to their developed properties, can be adapted to numerous applications such as the food packaging industry (Ahmed et al., 2021). Rodrigues et al. (2020) have developed chitosan-octadecylammonium and zinc oxide nanoparticles with improved mechanical, thermal, and antimicrobial properties, making them suitable for promising applications in active food packaging. In addition, chitosan-based nanocomposites are widely used as biological materials for wound and burn treatment, tissue engineering, and drug delivery systems (S. Ahmad et al., 2021). These nanocomposites, because of their small size and surface-to-volume ratio, are able to cross various biological barriers and deliver drugs to a specific site, making them promising to increase therapeutic efficacy in oral drug delivery (Khan and Alamry, 2021). Furthermore, chitosan derivatives via the cross-linking of polyethylene glycol diglycidyl ether using a microwave-assisted green method and its nanoparticles could potentially be used for biomedical applications (Ali et al., 2023). Nanocomposite materials can be also used in agricultural applications for controlled release of fertilizers and pesticides. Chitosan-based nanocomposites may also enhance plant growth and protect against pathogens (Yu et al., 2021). In addition, previous studies have revealed that chitosan-based nanocomposites are used for water purification and wastewater treatment. They can efficiently adsorb heavy metals, dyes, and other pollutants due to the high surface area and adsorption capacity of nanomaterials (Ahmad N. et al., 2020). The versatility of chitosan-based nanocomposites makes them suitable for a wide range of applications, and ongoing research continues to explore new possibilities and optimize their performance in different industries.
8 Application of chitosan, chitosan derivatives, and chitosan-based nanocomposites
Chitosan and its derivatives stand out as transformative materials with remarkable attributes, making them game-changing materials in a myriad of industries, including the food sector, agriculture, cosmetics, pharmaceuticals, medicine, and wastewater treatment (Figure 5). The availability, cost-effectiveness, non-toxicity, and versatile characteristics of chitosan and its derivatives make them indispensable materials in advancing sustainable practices and innovations across various fields. The broad spectrum of applications highlights the versatility and utility of chitosan, its derivatives, and nanocomposites in addressing challenges in a variety of sectors.
8.1 Food industry
8.1.1 Food additives
Chitosan and its derivatives have a wide range of uses as food additives, thanks to their bioactive and cationic nature. In addition, chitosan is specifically biocompatible and biodegradable substance, which allows it to be broadly applied in the food industry (Morin-Crini et al., 2019b). Several studies have examined the safety and efficacy of chitosan and its suitability as a natural food additive. It has recently been certified as Generally Recognized As Safe (GRAS) by the United States Food and Drug Administration (USFDA) (Hu et al., 2019). Chitosan is widely used for its hypocholesterolemic property. Miyazawa et al. (2018) investigated the effects of chitosan from mushrooms on dietary obesity by providing mice with a chitosan-administered high-fat diet. The chitosan groups showed marked suppression of body weight gain. In addition, a clear reduction in low-density lipoprotein cholesterol and an increase in high-density lipoprotein cholesterol were observed, suggesting that the administered mushroom chitosan improved blood lipid metabolism. The mushroom chitosan showed an anti-obesity effect by inhibiting the digestion and absorption of lipid molecules. The use of chitosan in winemaking is widespread (Castro Marín et al., 2021). The potential use of biocompatible chitosan as a healthy additive or as an alternative to sulfur dioxide (a chemical additive) to prevent the oxidative degradation of white wine has been proven. It was found that the use of chitosan prevents the formation of the 1-hydroxyethyl radical. In the same report, a direct (dose-dependent) OH radical scavenging effect was revealed up to 98% at 2 g/L of chitosan (Castro Marín et al., 2019). Likewise, chitosan can be utilized in mimetic food as a fat substitute. A study by Rios et al. (2018) explored the capacity of a chitosan derivative as a fat alternative in cake formulations. The addition of 2 g of succinyl chitosan to 100 g of wheat flour increased the emulsifying capacity of the flour. The succinyl chitosan revealed a great potential for use as a partial fat substitute (up to 50%) in cake recipes. This chitosan derivative also reduced the rate of cake hardening during storage. The polymer has been tested for its ability to prolong the conservation period of catfish fillets and protect them from storage conditions (4°C). Vacuum tumbling with a chitosan solution stabilized the quality and color of refrigerated fillets by preventing the growth of aerobic bacteria (Bonilla et al., 2018). Carboxymethyl chitosan, an ampholic derivative of chitosan, is widely used as an effective food additive. Zhu et al. (2022) found that carboxymethyl chitosan improved storage stability and textural properties of the dough by limiting water migration and delaying protein deterioration during freezer storage. Today’s consumer is more attracted to natural food additives than synthetic ones. Chitosan is a natural non-toxic polymer. If mixed with food products, it will be safe for human’s body and will preserve the freshness and prolong the shelf life of perishable food products by preventing microbial spoilage and chemical deterioration (Hameed et al., 2022).
8.1.2 Food packaging
Chitosan is an eco-friendly material for active food packaging owing to its natural availability, non-toxicity, renewable nature, inherent biodegradablity, and ease of modification. This biopolymer has excellent film-forming and antimicrobial properties that allow it to be considered as a suitable alternative source of food packaging materials to replace non-biodegradable and non-renewable polymers (Morin-Crini et al., 2019a; Y.-L. Zhang et al., 2021). Chitosan, as an active food packaging, can be applied, alone or in combination, in the form of a comestible thin film or an edible coating. Coating is a thin layer developed to cover a food product, delivered in liquid form by dipping the product in a chitosan solution or by spraying the solution onto the product (Cazón and Vázquez, 2019). The application of a chitosan film or coating shows promise for extending the storage period of highly perishable fruit and vegetables during post-harvest processing (Hameed et al., 2022; Oladzadabbasabadi et al., 2022). Chitosan’s capacity to scavenge free radicals as a natural antioxidant can be seen as an advantage for its potential use as a food packaging material (Wang et al., 2020b). According to the literature, chitosan is widely applied as a promising food packaging material to control the microbial growth of food, prevent oxidative deterioration of products, maintain the quality and nutritional characteristics during conservation, and also extend the shelf life of food products. Table 3 represents different applications of chitosan derivatives and chitosan-based nanocomposites as active food packaging materials. Chitosan, as an active and promising packaging for food preservation, can be applied in its native, modified or combined form. The main advantage of chitosan is that it can undergo a wide range of chemical and physical modifications to improve its functionality (Inanli et al., 2020). Chitosan nanocomposites have been shown to improve the physical properties of the biopolymer, making it useful in various applications such as the food packaging industry.
A study by El-araby et al. (2022b) evaluated post-harvest treatment of strawberries (Fragaria x ananasa) with chitosan solution as a preservative coating. The results showed that the coating reduced post-harvest losses and prolonged the storage period by 7–8 days by minimizing microbial spoilage load, maintaining anthocyanin pigments, and retaining fruit firmness during storage. Batista Silva et al. (2018) found that chitosan coating improved the shelf life and stabilized the overall quality of guava (Psidium guajava L.) fruits after harvest by enhancing antioxidant processes and retarding ripening while stored at room temperature. Chitosan coating has been shown to reduce fresh weight loss, preserve fruit skin firmness and color, and delay chlorophyll degradation without significant effect on titratable acidity. Chitosan films prepared by adding peanut skin and pink pepper residue extracts were studied as active packaging for chicken products. Treatment with active films guarantees the oxidative stability and quality of chicken products, thanks to antioxidant and antimicrobial performances (Serrano-León et al., 2018). A study by Gedarawatte et al. (2021) developed spray coatings of chitosan and gelatin to prolong the shelf life of vacuum-packaged beef. The edible chitosan coating significantly reduced lipid oxidation and prevented lactic acid bacteria growth when compared to uncoated and gelatin-coated beef samples. This study shows that chitosan coating in spray form is easily adaptable to industrial environments as an antimicrobial and antioxidant application prior to the meat vacuum packaging process. Y.-L. Zhang et al. (2021) evaluated the effect of an edible coating of carboxymethyl chitosan and gelatin on a variety of sweet cherry cultivars during post-harvest processing. The coating maintained the nutritional quality and properties of the sweet cherry varieties. The developed preservation method reduced weight loss and maintained initial peel color, stem freshness, firmness of the fruit, ascorbic acid content, titratable acidity, total phenolic content, and total anthocyanin concentration.
8.2 Agriculture
The world’s growing population, shrinking arable land, and the development of plant diseases are major challenges facing the agricultural sector, resulting in considerable economic losses. However, the continued use of fertilizers, pesticides, fungicides, and large amounts of chemical nutrients has an impact on the sustainable development of the agricultural economy and public health security. Hence the importance of developing modern agriculture that takes these challenges into account and that must be adapted to climate change. Chitosan and its derivatives have been largely proposed as environmentally friendly alternative sources to the application of agrochemicals. The bioactivities of chitosan such as antifungal activity, crop yield improvement, induction of plant defense system, and promotion of plant growth play a crucial role in its application in agriculture (Morin-Crini et al., 2019b). Chitosan, as a biostimulant, has the ability to act inside and outside the plants and apply physical or physicochemical effects on them. It is broadly recognized for its physiological effects on nutritional efficiency, and on the response to abiotic stress (Bhupenchandra et al., 2020). Several studies have indicated that chitosans induce the activities of genes responsible for various events in plant life processes, such as systemic acquired resistance, photosynthesis, plant defense system, hormone metabolism, and alteration of protein metabolism, resulting in increased storage protein content (Landi et al., 2017; Xoca-Orozco et al., 2017). The advantageous functions of chitosan are mainly related to the tolerance of plants to abiotic stress factors such as salinity, high temperatures and drought, as well as to the enhancement of their photosynthetic performance (Shahrajabian et al., 2021). Chitosan’s hydrophilic nature reduces transpiration rate and promotes water absorption. Rahman et al. (2018) revealed that foliar application of chitosan to strawberries improved plant growth and fruit yield. A significant increase in fruit weight and higher levels of anthocyanins, carotenoids, flavonoids, and phenolic compounds were observed when plants were sprayed with a chitosan solution compared to the untreated control. Another study reported that the biomass and number of flowers of mycorrhizal tomato plants were improved by foliar spraying with chitosan (El Amerany et al., 2020).
Saharan et al. (2016) report that Cu-chitosan nanoparticles, through α-amylase and protease activity, improve the growth of corn seedlings by exploiting reserved feed, mainly starch and protein. A study by Jogaiah et al. (2020) revealed that the application of chitosan to cucumber seeds had a positive impact on disease protection and improved plant development. The results show that chitosan increased phytohormone regulation and synthesized defense enzymes, inducing resistance to mildew disease, and enhancing cucumber plant development. In addition, chitosan-based biodegradable and biocompatible nanomaterials are employed in soil conditioners, seed coatings, and foliar treatments to promote plant growth and protect plants against fungi, bacteria and viruses, offering a novel and promising material for durable crop protection (Shahrajabian et al., 2021). Vanti et al. (2020) synthesized copper nanoparticles coupled with chitosan and evaluated their benefits on chili, cowpea, and tomato crops. The prepared nanoparticles had beneficial effects as fungicide and growth promoter and explored their possible application as a safe alternative to conventional pesticides to avoid hazardous effects on the environment. Apart from the direct effects of chitosan, chitosan nanoparticles have synergistic effects with plant-friendly metals such as Zn, Cu, Ag and Ni and can enhance their fungicidal and disinfectant properties. Table 4 represents other uses of chitosan derivatives and chitosan-based nanocomposites in agriculture. A study by Choudhary et al. (2019) showed that Zn-chitosan nanoparticles presented strong antifungal and growth-promoting activities in corn seedlings. Zn-chitosan nanoparticles have been shown to combat disease by boosting plant immunity through increased antioxidant and defense enzymes, regulation of reactive oxygen species and increased lignin uptake.
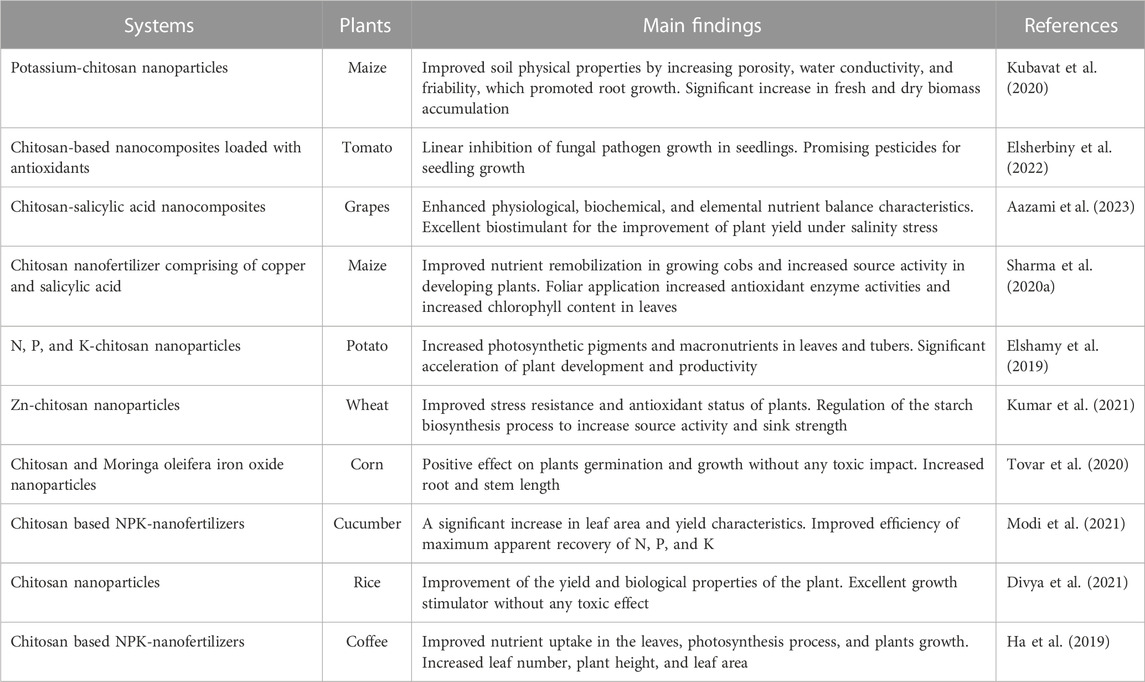
TABLE 4. Applications of chitosan derivatives and chitosan-based nanocomposites in agricultural sector.
8.3 Cosmetic industry
These days, bio ingredients are attracting a lot of interest in the cosmetics sector to overcome the undesirable effects of synthetic active ingredients, such as skin irritation, itching, photoallergy, and phototoxicity. Researchers are therefore increasingly interested in chitosan and its derivatives for the preparation of cosmetic products, due to its natural abundance, ease of extraction and excellent cosmetic qualities. Chitosan is the only natural cationic polymer whose bacteriostatic, fungistatic, moisturizing, and film-forming properties favor its use in cosmetic formulations (Jiménez-Gómez and Cecilia, 2020; Aranaz et al., 2021) and make it attractive in skin, nail, hair, and oral care applications (Morin-Crini et al., 2019a; Azmana et al., 2021). Chitosan is used in hair treatment products including shampoos, hairsprays and dyes to promote and boost the hair’s softness and mechanical strength, to eliminate oils and sebum due to its hydrophobic nature, and to maintain humidity and styling by reducing static electricity in the hair (Abd El-Hack et al., 2020). Thanks to its film-forming qualities, chitosan has the capacity to interact with hair keratin, creating a transparent elastic film on hair fibers, increasing smoothness and force and preventing damage (Wang et al., 2020b). One of the essential benefits of chitosan in the cosmetic field is its use in moisturizing agents to keep the skin well hydrated and nourished. It softens the epidermis and prevents damage caused by external environmental conditions and cleansers. High-molecular-weight chitosan has film-forming characteristics that can help reduce skin water loss, increase elasticity and improve skin smoothness (Casadidio et al., 2019; Gupta et al., 2022).
Petrick et al. (2020) synthetized a chitosan/TiO2 nanocomposite as a multifunctional sunscreen for moderate UV protection with an ability to inhibit the activity of bacteria living on the skin surface up to 99.7% in 2 h. The addition of chitosan to lip care products makes lips softer and protects them from drying out. It is also used as an active ingredient to promote long-term adhesion of lipstick colors (Bakshi et al., 2020). Due to their cationic nature, chitosan and its derivatives are suitable for inclusion in cleansing products such as cleansing milks, face toners, peels, soaps and shower products, by exploiting the ionic attraction between their cationic charge and the anionic nature of the skin surface (Abd El-Hack et al., 2020). Chitosan and its derivatives are widely applied in the treatment of oral problems, through the creation of dental gels, toothpastes, and mouthwashes (Gupta et al., 2022). A toothpaste developed with biosurfactants and chitosan had a positive effect on the inhibition of biofilm formed by Streptococcus mutans compared to the commercial toothpaste tested. The addition of chitosan and its derivatives has also been shown to improve the inhibition of dental biofilm (Resende et al., 2019). The deodorizing effect and antimicrobial characteristics of chitosan enable it to be used as an active ingredient in deodorizers, as it inhibits the activity of enzyme-producing bacteria (Bakshi et al., 2020). Table 5 represents other applications of chitosan derivatives and chitosan-based nanocomposites in the cosmetic industry. The cosmetics sector has developed very rapidly in recent times, and products based on chitosan alone or combined with other components are already available on the market.
8.4 Pharmaceutical and medical applications
8.4.1 Wound dressing and healing
Chitosan has the ability to improve the wound-healing process and prevent bacterial infections due to its hemostatic, antimicrobial, anti-inflammatory, film-forming, and analgesic properties, making it useful as an active material for dressings (Abd El-Hack et al., 2020; M. Zhang et al., 2022). It contributes effectively to cell growth due to its positive surface charge, which has led to thrombosis and blood coagulation (Wang et al., 2020a). Chitosan-based bionanocomposites are utilized as wound healing constituents by enhancing the epithelialization process and collagen deposition on the dermal layer of the skin (Azmana et al., 2021). Chitosan-loaded cyclodextrin hydrogels decreased blood leakage and the duration of hemostasis compared with commercial hydrogels. The results show that artificial hydrogels hold promise as a physiologically safe means of mitigating blood loss in tissue injury situations (Leonhardt et al., 2019). The chitosan microneedle patch has proved to be very useful for wound healing, promoting inhibition of inflammation, collagen synthesis, and tissue renewal during wound healing (Chi et al., 2020). The chitosan nanosilver-based dressing had a greater and quicker healing effect than intradermal injection of mesenchymal stem cells. In the same report, the group receiving the developed dressing showed a significant increase in epidermal thickness, collagen density and nuclear antigen immunoreactivity of proliferating cells (Ghannam et al., 2018). Sulfated chitosan-type I collagen hydrogel accelerated the healing of chronic diabetic wounds by enhancing the functions of macrophages into fibroblasts, resulting in enhanced collagen and extracellular matrix formation in the wound tissue (Shen et al., 2020). Polyvinyl chitosan nanofibers were associated with carboxymethyl chitosan nanoparticles encapsulated with an antibacterial peptide. These nano-system revealed antibacterial properties and stimulated the healing of mouse tissue (Zou et al., 2020). Micro-channeled alkylated chitosan sponges have shown promising clinical translation potential to treat non-compressible lethal hemorrhages and facilitate wound healing. These hemostatic chitosan sponges demonstrated water and blood absorption capacity, and provided higher coagulant and hemostatic potencies in liver perforation wound models of lethally normal and heparinized rats and pigs, compared with treatments used in the clinic (Du et al., 2021). Chitosan functionalized via coupling of chitosan -NH2 groups with 2,4,6-trimethoxybenzaldehyde could be a promising candidate for wound dressing products and cutaneous cancer treatment (Tamer et al., 2023).
8.4.2 Tissue engineering
Chitosan-based bioactive materials have emerged as promising candidates for tissue engineering applications, as they degrade progressively as new tissue is formed, avoiding inflammatory reactions and toxic degradation (Bakshi et al., 2020; Islam et al., 2020). Chitosan-grafted polymethyl methacrylate and modified hydroxyapatite have been successfully developed and applied for bone tissue engineering. The biocomposite exhibited good mechanical strength and long-term stability after soaking, and could be utilized as a scaffold for bone cell growth and drug delivery during bone repair (Tithito et al., 2019). Composite scaffolds of gelatin, chitosan, polyvinyl alcohol, and nano-hydroxyapatite showed positive effects on osteogenic differentiation and were capable of mimicking the structure and function of natural bone. The composite scaffolds effectively promoted cell proliferation and adhesion, making them a promising biomimetic scaffold for bone tissue engineering (Ma et al., 2021). The synthetized cellulose nanofiber-filled chitosan hydrogels have been effective in repairing and regenerating the annulus fibrosus tissue of the intervertebral disc. Nanocomposite material is suitable for use as an implant in annulus fibrosus tissue defects to repair intervertebral disc biomechanics and provide retaining plates against disc nucleus protrusion, while supporting intervertebral disc regeneration and approximating the functionality of a healthy disc (Doench et al., 2019). Pore scaffolds formulated with chitosan, gelatin, and silk proteins exhibited good chondrocyte cell viability and promoted rapid cartilage tissue regeneration in defective rabbit knee articular cartilage (Haghighi and Shamloo, 2021). Chitosan-vitamin C-lactic acid membranes have been used in skin tissue engineering. The prepared porous chitosan composite membranes provided an optimal environment for the attachment, growth, and spreading of skin cells (NIH-3T3 fibroblasts) compared to non-porous membranes (Madni et al., 2019). Composite scaffolds containing silk fibroin, carboxymethyl chitosan, strontium-substituted hydroxyapatite and cellulose nanocrystals proved effective in enhancing osteoblast adhesion and proliferation. The experimental results suggested the applicability of the synthesized scaffolds for bone repair (X. Zhang et al., 2019).
8.4.3 Drug delivery
Biocompatibility, biodegradability, long-term stability, mucoadhesive capacity, non-toxicity, cationic nature, and the presence of amino and hydroxyl groups are the most critical characteristics that make chitosan an active and promising polysaccharide for various drug delivery (Barman et al., 2020; Hameed et al., 2022). Advances in drug delivery systems are attracting growing interest from researchers, and point to the need to develop innovative and improved materials using chitosan-based nanocomposites to enhance drug delivery efficiency. In general, microspheres, tablets, gels, microcapsules, and films with sustained release can be prepared by combining the polymer with drugs by dissolution, coating or adsorption (Wang et al., 2020b). Furthermore, the introduction of new entities into the polymer linear chain enhances other characteristics of chitosan, boosting its suitability for pharmaceutical purposes. For example, O-carboxymethyl and N-trimethyl chitosan are the most promising chitosan derivatives and have excellent potential for drug delivery system (Abd El-Hack et al., 2020). A drug delivery system is a technical device that combines medicine, engineering, and pharmacy to deliver the precise dose of drug to the right place at the correct time, increasing the drug’s bioavailability and therapeutic efficacy, and minimizing adverse effects (Ewart et al., 2019; Wang et al., 2020a). Table 6 represents chitosan-based nanocomposites in different drug delivery systems. Chitosan-based devices are used for the delivery of proteins and peptides, growth promoters, anti-inflammatory agents, antibiotics, and vaccines, also in gene therapy and bioimaging applications (Bakshi et al., 2020).
8.5 Wastewater treatment
The daily discharge of industrial wastewater into landfills results in significant water and environmental contamination. Physico-chemical treatments can be used to eliminate heavy metals and toxic compounds from wastewater. However, these methods are harmful to the environment due to the use of synthetic chemicals. The presence of active functional groups (-NH2 and -OH) has allowed chitosan and its derivatives to gain more popularity than other polysaccharides as an effective adsorbent for water purification. Chitosan-based bionanocomposites have been reported to be very useful as safe and environmentally friendly alternatives for the chelation of heavy metals and dyes in industrial wastewater. Cationization of -NH2 groups leads to absorption of anionic dyes through electrostatic attraction in acidic conditions (Thomas et al., 2019; Azmana et al., 2021). Microfluidically generated chitosan microspheres significantly eliminated Cu and other toxic heavy metal ions from the polluted water. The excellent adsorption capacity, biodegradable properties, and low extraction cost make chitosan microspheres a promising material in industrial wastewater treatment applications (B. Wang et al., 2019). Sessarego et al. (2019) synthesized a phosphonium cross-linked chitosan to eliminate Cr (VI) from wastewater by adsorption. The research indicated that phosphonium functionality is provided to the chitosan by tetrakis (hydroxymethyl) phosphate through an easy-on-easy process of synthesis, leading to improved absorption at pH 6. The N-N-N-triethylammonium chitosan and carboxymethyl chitosan were used to remove Cu (II), Ni (II), and Cr (VI) by size-enhanced ultrafiltration. The optimization study was conducted to maximize the removal of heavy metal ions from aqueous solutions and the binding ability of chitosan derivatives. Chitosan and its nanocomposites are powerful biosorbents owing to the intrinsic characteristics of their amino (-NH2) and hydroxyl (OH) functional groups, which make them suitable for dye removal. Synthesized ZnO-chitosan nanocomposites were effective in removing methylene blue from simulated wastewater. The excellent adsorption characteristics of chitosan nanocomposites have been highlighted by the successful adsorption of methylene blue up to six cycles (Zango et al., 2022). The in-situ precipitation process successfully generated ZnO/chitosan nanocomposites. The application of these nanocomposites in wastewater treatment has shown their effectiveness as a powerful adsorbent for the removal of Congo red from aqueous solutions (Nguyen et al., 2020). Table 7 represents other applications of chitosan derivatives and chitosan-based nanocomposites in wastewater treatment. In addition to the good reputation of chitosan nanocomposites in the removal of heavy metals and dyes, studies regarding antimicrobial properties and degradation efficiencies of organic and emerging contaminants are also conducted in the industry of wastewater treatment and management (Alburquenque et al., 2010; Aizat and Aziz, 2019).
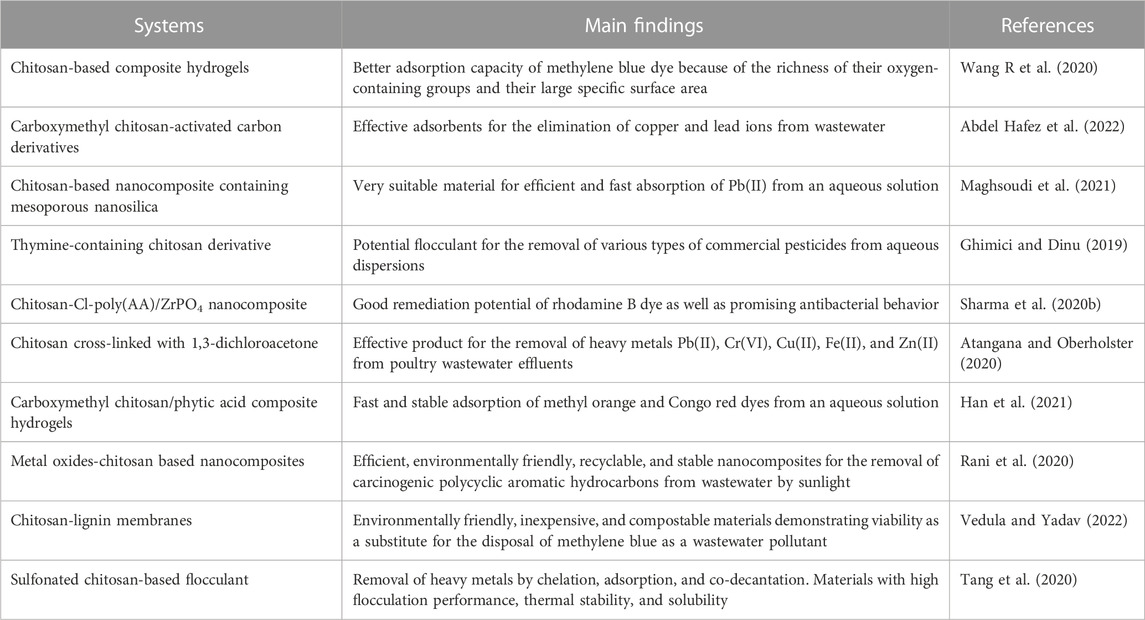
TABLE 7. Applications of chitosan derivatives and chitosan-based nanocomposites in wastewater treatment.
9 Conclusion
Chitosan represents an interesting and valuable way to valorize marine by-products. This polymer is an environmentally friendly material with several promising biological activities and specific physicochemical properties that make it the most polyfunctional and versatile of all other biopolymers. The presence of active functional groups is particularly advantageous to ensure the modification of chitosan and consequently broaden its application spectrum. Chemical and physical modification of chitosan is widely discussed to produce a variety of chitosan derivatives with high solubility and enhanced properties. However, the enzymatic route is still under development, which suggests a very bright future for the sustainable modification of chitosan. With advances in nanotechnology, chitosan-based nanocomposites are the subject of intensive research with a bright future, thanks to their unique and exceptional properties that make them more effective in many sectors. However, further research into chitosan chemistry will open up broad prospects for chitosan derivatives and chitosan in nanoparticle form and therefore offer valuable options for promising chitosan applications. The evolution of chitosan and its derivatives should be strategically oriented towards precision, responsiveness and sustainability, encompassing a broad spectrum of applications. This intentional approach positions chitosan as a key player in meeting contemporary challenges across all industries, promising a future where its diverse applications will be characterized by both efficiency and environmental responsibility.
Author contributions
AE-A: Conceptualization, Formal Analysis, Funding acquisition, Investigation, Methodology, Project administration, Resources, Supervision, Validation, Visualization, Writing–original draft, Writing–review and editing. WJ: Conceptualization, Data curation, Methodology, Project administration, Resources, Software, Validation, Visualization, Writing–review and editing. RU: Funding acquisition, Investigation, Project administration, Resources, Software, Validation, Visualization, Writing–review and editing. SE: Funding acquisition, Investigation, Project administration, Resources, Software, Validation, Visualization, Writing–review and editing. FE: Conceptualization, Funding acquisition, Methodology, Resources, Supervision, Validation, Writing–review and editing.
Funding
The author(s) declare that no financial support was received for the research, authorship, and/or publication of this article. This research received no external funding.
Acknowledgments
Authors wish to thank Research Center College of Pharmacy and Deanship of Scientific Research King Saud University Riyadh Saudi Arabia.
Conflict of interest
The authors declare that the research was conducted in the absence of any commercial or financial relationships that could be construed as a potential conflict of interest.
Publisher’s note
All claims expressed in this article are solely those of the authors and do not necessarily represent those of their affiliated organizations, or those of the publisher, the editors and the reviewers. Any product that may be evaluated in this article, or claim that may be made by its manufacturer, is not guaranteed or endorsed by the publisher.
References
Aazami, M. A., Maleki, M., Rasouli, F., and Gohari, G. (2023). Protective effects of chitosan based salicylic acid nanocomposite (CS-SA NCs) in grape (Vitis vinifera cv. ‘Sultana’) under salinity stress. Sci. Rep. 13 (1), 883. doi:10.1038/s41598-023-27618-z
Abd El-Hack, M. E., El-Saadony, M. T., Shafi, M. E., Zabermawi, N. M., Arif, M., Batiha, G. E., et al. (2020). Antimicrobial and antioxidant properties of chitosan and its derivatives and their applications: a review. Int. J. Biol. Macromol. 164, 2726–2744. doi:10.1016/j.ijbiomac.2020.08.153
Abdel Hafez, O. M., Mohamed, R. R., Abou Kana, M. T. H., Mohamed, E. A., and Negm, N. A. (2022). Treatment of industrial wastewater containing copper and lead ions using new carboxymethyl chitosan-activated carbon derivatives. Egypt. J. Chem. 65 (2), 0–132. doi:10.21608/ejchem.2021.82163.4050
Abdel-Naeem, H. H. S., Zayed, N. E. R., and Mansour, H. A. (2021). Effect of chitosan and lauric arginate edible coating on bacteriological quality, deterioration criteria, and sensory attributes of frozen stored chicken meat. LWT 150, 111928. doi:10.1016/j.lwt.2021.111928
Abo Elsoud, M. M., and El Kady, E. M. (2019). Current trends in fungal biosynthesis of chitin and chitosan. Bull. Natl. Res. Centre 43 (1), 59. doi:10.1186/s42269-019-0105-y
Ahmad, N., Sultana, S., Khan, M. Z., and Sabir, S. (2020a). “Chitosan based nanocomposites as efficient adsorbents for water treatment,” in Modern age waste water problems. Editors M. Oves, M. O. Ansari, M. Zain Khan, M. Shahadat, and I. M. I. Ismail (Cham: Springer International Publishing), 69–83. doi:10.1007/978-3-030-08283-3_4
Ahmad, S., Abbasi, A., Manzoor, K., Mangla, D., Aggarwal, S., and Ikram, S. (2021). “9—chitosan-based bionanocomposites in drug delivery,” in Bionanocomposites in tissue engineering and regenerative medicine. Editors S. Ahmed,, and A. Annu (Sawston: Woodhead Publishing), 187–203. doi:10.1016/B978-0-12-821280-6.00024-6
Ahmad, Z., Khan, M. I., Siddique, M. I., Sarwar, H. S., Shahnaz, G., Hussain, S. Z., et al. (2020b). Fabrication and characterization of thiolated chitosan microneedle patch for transdermal delivery of tacrolimus. AAPS PharmSciTech 21 (2), 68. doi:10.1208/s12249-019-1611-9
Ahmed, E. M., Saber, D., ElAziz, K. A., Alghtani, A. H., Felemban, B. F., Ali, H. T., et al. (2021). Chitosan-based nanocomposites: preparation and characterization for food packing industry. Mater. Res. Express 8 (2), 025017. doi:10.1088/2053-1591/abe791
Aizat, M. A., and Aziz, F. (2019). Chitosan nanocomposite application in wastewater treatments. Nanotechnol. Water Wastewater Treat., 243–265. doi:10.1016/B978-0-12-813902-8.00012-5
Alburquenque, C., Bucarey, S. A., Neira-Carrillo, A., Urzúa, B., Hermosilla, G., and Tapia, C. V. (2010). Antifungal activity of low molecular weight chitosan against clinical isolates of Candida spp. Med. Mycol. 48 (8), 1018–1023. doi:10.3109/13693786.2010.486412
Ali, M., Mohamed, M. I., Taher, A. T., Mahmoud, S. H., Mostafa, A., Sherbiny, F. F., et al. (2023). New potential anti-SARS-CoV-2 and anti-cancer therapies of chitosan derivatives and its nanoparticles: preparation and characterization. Arab. J. Chem. 16, 104676. doi:10.1016/j.arabjc.2023.104676
Alqahtani, F., Aleanizy, F., El Tahir, E., Alhabib, H., Alsaif, R., Shazly, G., et al. (2020). Antibacterial activity of chitosan nanoparticles against pathogenic N. Gonorrhoea. Int. J. Nanomedicine 15, 7877–7887. doi:10.2147/IJN.S272736
Amor, I. B., Hemmami, H., Laouini, S. E., Temam, H. B., Zaoui, H., and Barhoum, A. (2023). Biosynthesis MgO and ZnO nanoparticles using chitosan extracted from Pimelia payraudi latreille for antibacterial applications. World J. Microbiol. Biotechnol. 39, 19. doi:10.1007/s11274-022-03464-5
Aranaz, I., Alcántara, A. R., Civera, M. C., Arias, C., Elorza, B., Heras Caballero, A., et al. (2021). Chitosan: an overview of its properties and applications. Polymers 13 (19), 3256. doi:10.3390/polym13193256
Ashrafi, M., Bayat, M., Mortazavi, P., Hashemi, S. J., and Meimandipour, A. (2020). Antimicrobial effect of chitosan–silver–copper nanocomposite on Candida albicans. J. Nanostructure Chem. 10 (1), 87–95. doi:10.1007/s40097-020-00331-3
Atangana, E., and Oberholster, P. J. (2020). Modified biopolymer (Chitin–Chitosan derivatives) for the removal of heavy metals in poultry wastewater. J. Polym. Environ. 28 (2), 388–398. doi:10.1007/s10924-019-01616-7
Azmana, M., Mahmood, S., Hilles, A. R., Rahman, A., Arifin, M. A. B., and Ahmed, S. (2021). A review on chitosan and chitosan-based bionanocomposites: promising material for combatting global issues and its applications. Int. J. Biol. Macromol. 185, 832–848. doi:10.1016/j.ijbiomac.2021.07.023
Bakshi, P. S., Selvakumar, D., Kadirvelu, K., and Kumar, N. S. (2020). Chitosan as an environment friendly biomaterial – a review on recent modifications and applications. Int. J. Biol. Macromol. 150, 1072–1083. doi:10.1016/j.ijbiomac.2019.10.113
Bao, Z., Yu, A., Shi, H., Hu, Y., Jin, B., Lin, D., et al. (2021). Glycol chitosan/oxidized hyaluronic acid hydrogel film for topical ocular delivery of dexamethasone and levofloxacin. Int. J. Biol. Macromol. 167, 659–666. doi:10.1016/j.ijbiomac.2020.11.214
Barman, M., Mahmood, S., Augustine, R., Hasan, A., Thomas, S., and Ghosal, K. (2020). Natural halloysite nanotubes/chitosan based bio-nanocomposite for delivering norfloxacin, an anti-microbial agent in sustained release manner. Int. J. Biol. Macromol. 162, 1849–1861. doi:10.1016/j.ijbiomac.2020.08.060
Batista Silva, W., Cosme Silva, G. M., Santana, D. B., Salvador, A. R., Medeiros, D. B., Belghith, I., et al. (2018). Chitosan delays ripening and ROS production in guava (Psidium guajava L.) fruit. Food Chem. 242, 232–238. doi:10.1016/j.foodchem.2017.09.052
Benchamas, G., Huang, G., Huang, S., and Huang, H. (2021). Preparation and biological activities of chitosan oligosaccharides. Trends Food Sci. Technol. 107, 38–44. doi:10.1016/j.tifs.2020.11.027
Benhabiles, M. S., Salah, R., Lounici, H., Drouiche, N., Goosen, M. F. A., and Mameri, N. (2012). Antibacterial activity of chitin, chitosan and its oligomers prepared from shrimp shell waste. Food Hydrocoll. 29 (1), 48–56. doi:10.1016/j.foodhyd.2012.02.013
Bernabé, P., Becherán, L., Cabrera-Barjas, G., Nesic, A., Alburquenque, C., Tapia, C. V., et al. (2020). Chilean crab (Aegla cholchol) as a new source of chitin and chitosan with antifungal properties against Candida spp. Int. J. Biol. Macromol. 149, 962–975. doi:10.1016/j.ijbiomac.2020.01.126
Bhupenchandra, I., Soibam, H., Basumatary, A., Dutta, S., Singh, L., Kalita, P., et al. (2020). Biostimulants: potential and prospects in agriculture. Int. Res. J. Pure Appl. Chem., 20–35. doi:10.9734/irjpac/2020/v21i1430244
Bikiaris, N. D., Michailidou, G., Lazaridou, M., Christodoulou, E., Gounari, E., Ofrydopoulou, A., et al. (2020). Innovative skin product emulsions with enhanced antioxidant, antimicrobial and UV protection properties containing nanoparticles of pure and modified chitosan with encapsulated fresh pomegranate juice. Polymers 12 (7), 1542. doi:10.3390/polym12071542
Binh, N. T. T., Bao, H. N. D., Prinyawiwatkul, W., and Trung, T. S. (2021). Antioxidative and antimicrobial effects of low molecular weight shrimp chitosan and its derivatives on seasoned-dried Pangasius fillets. Int. J. Food Sci. Technol. 56 (10), 5119–5129. doi:10.1111/ijfs.15280
Bonilla, F., Chouljenko, A., Reyes, V., Bechtel, P. J., King, J. M., and Sathivel, S. (2018). Impact of chitosan application technique on refrigerated catfish fillet quality. LWT 90, 277–282. doi:10.1016/j.lwt.2017.12.010
Carocho, M., Morales, P., and Ferreira, I. C. F. R. (2018). Antioxidants: reviewing the chemistry, food applications, legislation and role as preservatives. Trends Food Sci. Technol. 71, 107–120. doi:10.1016/j.tifs.2017.11.008
Casadidio, C., Peregrina, D. V., Gigliobianco, M. R., Deng, S., Censi, R., and Di Martino, P. (2019). Chitin and chitosans: characteristics, eco-friendly processes, and applications in cosmetic science. Mar. Drugs 17 (6), 369. 6. doi:10.3390/md17060369
Castro Marín, A., Colangelo, D., Lambri, M., Riponi, C., and Chinnici, F. (2021). Relevance and perspectives of the use of chitosan in winemaking: a review. Crit. Rev. Food Sci. Nutr. 61 (20), 3450–3464. doi:10.1080/10408398.2020.1798871
Castro Marín, A., Culcasi, M., Cassien, M., Stocker, P., Thétiot-Laurent, S., Robillard, B., et al. (2019). Chitosan as an antioxidant alternative to sulphites in oenology: EPR investigation of inhibitory mechanisms. Food Chem. 285, 67–76. doi:10.1016/j.foodchem.2019.01.155
Cazón, P., and Vázquez, M. (2019). “Applications of chitosan as food packaging materials,” in Sustainable agriculture reviews 36: chitin and chitosan: applications in food, agriculture, pharmacy, medicine and wastewater treatment. Editors G. Crini,, and E. Lichtfouse (Cham: Springer International Publishing), 81–123. doi:10.1007/978-3-030-16581-9_3
Chaiwong, N., Leelapornpisid, P., Jantanasakulwong, K., Rachtanapun, P., Seesuriyachan, P., Sakdatorn, V., et al. (2020). Antioxidant and moisturizing properties of carboxymethyl chitosan with different molecular weights. Polymers 12 (7), 1445. doi:10.3390/polym12071445
Chaiwong, N., Phimolsiripol, Y., Leelapornpisid, P., Ruksiriwanich, W., Jantanasakulwong, K., Rachtanapun, P., et al. (2022). Synergistics of carboxymethyl chitosan and mangosteen extract as enhancing moisturizing, antioxidant, antibacterial, and deodorizing properties in emulsion cream. Polymers 14 (1), 178. doi:10.3390/polym14010178
Chang, S.-H., Lin, H.-T. V., Wu, G.-J., and Tsai, G. J. (2015). PH Effects on solubility, zeta potential, and correlation between antibacterial activity and molecular weight of chitosan. Carbohydr. Polym. 134, 74–81. doi:10.1016/j.carbpol.2015.07.072
Chang, S.-H., Wu, C.-H., and Tsai, G.-J. (2018). Effects of chitosan molecular weight on its antioxidant and antimutagenic properties. Carbohydr. Polym. 181, 1026–1032. doi:10.1016/j.carbpol.2017.11.047
Chen, G., Svirskis, D., Lu, W., Ying, M., Li, H., Liu, M., et al. (2021). N-trimethyl chitosan coated nano-complexes enhance the oral bioavailability and chemotherapeutic effects of gemcitabine. Carbohydr. Polym. 273, 118592. doi:10.1016/j.carbpol.2021.118592
Chen, Q., Qi, Y., Jiang, Y., Quan, W., Luo, H., Wu, K., et al. (2022). Progress in research of chitosan chemical modification technologies and their applications. Mar. Drugs 20, 536. doi:10.3390/md20080536
Chi, J., Zhang, X., Chen, C., Shao, C., Zhao, Y., and Wang, Y. (2020). Antibacterial and angiogenic chitosan microneedle array patch for promoting wound healing. Bioact. Mater. 5 (2), 253–259. doi:10.1016/j.bioactmat.2020.02.004
Choudhary, R. C., Kumaraswamy, R. V., Kumari, S., Sharma, S. S., Pal, A., Raliya, R., et al. (2019). Zinc encapsulated chitosan nanoparticle to promote maize crop yield. Int. J. Biol. Macromol. 127, 126–135. doi:10.1016/j.ijbiomac.2018.12.274
Çoban, M. Z. (2021). Effectiveness of chitosan/propolis extract emulsion coating on refrigerated storage quality of crayfish meat (Astacus leptodactylus). CyTA - J. Food 19 (1), 212–219. doi:10.1080/19476337.2021.1882580
Confederat, L. G., Tuchilus, C. G., Dragan, M., Sha’at, M., and Dragostin, O. M. (2021). Preparation and antimicrobial activity of chitosan and its derivatives: a concise review. Molecules 26 (12), 3694. doi:10.3390/molecules26123694
Coutinho, T. C., Ferreira, M. C., Rosa, L. H., de Oliveira, A. M., and Oliveira Júnior, E. N. de. (2020). Penicillium citrinum and Penicillium mallochii: new phytopathogens of orange fruit and their control using chitosan. Carbohydr. Polym. 234, 115918. doi:10.1016/j.carbpol.2020.115918
Deshkar, S., Sikchi, S., Thakre, A., and Kale, R. (2021). Poloxamer modified chitosan nanoparticles for vaginal delivery of acyclovir. Pharm. Nanotechnol. 9 (2), 141–156. doi:10.2174/2211738508666210108121541
Divya, K., Thampi, M., Vijayan, S., Shabanamol, S., and Jisha, M. S. (2021). Chitosan nanoparticles as a rice growth promoter: evaluation of biological activity. Archives Microbiol. 204 (1), 95. doi:10.1007/s00203-021-02669-w
Doan, C. T., Tran, T. N., Nguyen, V. B., Vo, T. P. K., Nguyen, A. D., and Wang, S.-L. (2019). Chitin extraction from shrimp waste by liquid fermentation using an alkaline protease-producing strain, Brevibacillus parabrevis. Int. J. Biol. Macromol. 131, 706–715. doi:10.1016/j.ijbiomac.2019.03.117
Doench, I., Ahn Tran, T., David, L., Montembault, A., Viguier, E., Gorzelanny, C., et al. (2019). Cellulose nanofiber-reinforced chitosan hydrogel composites for intervertebral disc tissue repair. Biomimetics 4 (1), 19. doi:10.3390/biomimetics4010019
Dong, Q., Qiu, W., Feng, Y., Jin, Y., Deng, S., Tao, N., et al. (2023). Proteases and microwave treatment on the quality of chitin and chitosan produced from white shrimp (Penaeus vannamei). eFood 4, e73. doi:10.1002/efd2.73
Du, X., Wu, L., Yan, H., Jiang, Z., Li, S., Li, W., et al. (2021). Microchannelled alkylated chitosan sponge to treat noncompressible hemorrhages and facilitate wound healing. Nat. Commun. 12 (1), 4733. doi:10.1038/s41467-021-24972-2
Duan, C., Meng, X., Meng, J., Khan, Md. I. H., Dai, L., Khan, A., et al. (2019). Chitosan as A Preservative for fruits and vegetables: a review on chemistry and antimicrobial properties. J. Bioresour. Bioprod. 4 (1), 11–21. doi:10.21967/jbb.v4i1.189
Duran, A., and Kahve, H. I. (2020). The effect of chitosan coating and vacuum packaging on the microbiological and chemical properties of beef. Meat Sci. 162, 107961. doi:10.1016/j.meatsci.2019.107961
El Amerany, F., Meddich, A., Wahbi, S., Porzel, A., Taourirte, M., Rhazi, M., et al. (2020). Foliar application of chitosan increases tomato growth and influences mycorrhization and expression of endochitinase-encoding genes. Int. J. Mol. Sci. 21 (2), 535. doi:10.3390/ijms21020535
El-araby, A., El Ghadraoui, L., and Errachidi, F. (2022a). Physicochemical properties and functional characteristics of ecologically extracted shrimp chitosans with different organic acids during demineralization step. Molecules 27 (23), 8285. doi:10.3390/molecules27238285
El-araby, A., El Ghadraoui, L., and Errachidi, F. (2022b). Usage of biological chitosan against the contamination of post-harvest treatment of strawberries by Aspergillus Niger. Front. Sustain. Food Syst. 6, 881434. doi:10.3389/fsufs.2022.881434
Elbagoury, M. M., Turoop, L., Runo, S., Sila, D. N., and Madivoli, E. S. (2022). Postharvest treatments of banana (Musa acuminata cv. ‘Grand Nain’, AAA) during cold and ripening temperatures with chitosan and chitosan nanoparticles to alleviate chilling injury and maintain antioxidant activity. Hortic. Environ. Biotechnol. 63 (5), 677–699. doi:10.1007/s13580-022-00436-4
El Knidri, H., Belaabed, R., Addaou, A., Laajeb, A., and Lahsini, A. (2018). Extraction, chemical modification and characterization of chitin and chitosan. Int. J. Biol. Macromol. 120, 1181–1189. doi:10.1016/j.ijbiomac.2018.08.139
Elshamy, M. T., Elk., S. M., Husseiny, S. M., and Farroh, K. Y. (2019). Application of nano-chitosan NPK fertilizer on growth and productivity of potato plant. J. Sci. Res. Sci. 36 (1), 424–441. doi:10.21608/jsrs.2019.58522
Elsherbiny, A. S., Galal, A., Ghoneem, K. M., and Salahuddin, N. A. (2022). Novel chitosan-based nanocomposites as ecofriendly pesticide carriers: synthesis, root rot inhibition and growth management of tomato plants. Carbohydr. Polym. 282, 119111. doi:10.1016/j.carbpol.2022.119111
Ewart, D., Peterson, E. J., and Steer, C. J. (2019). A new era of genetic engineering for autoimmune and inflammatory diseases. Seminars Arthritis Rheumatism 49 (1), e1–e7. doi:10.1016/j.semarthrit.2019.05.004
Gao, Y., and Wu, Y. (2022). Recent advances of chitosan-based nanoparticles for biomedical and biotechnological applications. Int. J. Biol. Macromol. 203, 379–388. doi:10.1016/j.ijbiomac.2022.01.162
Garcia, L. G. S., Guedes, G. M. de M., da Silva, M. L. Q., Castelo-Branco, D. S. C. M., Sidrim, J. J. C., Cordeiro, R. de A., et al. (2018). Effect of the molecular weight of chitosan on its antifungal activity against Candida spp. in planktonic cells and biofilm. Carbohydr. Polym. 195, 662–669. doi:10.1016/j.carbpol.2018.04.091
Garg, K., and Tirgar, P. (2022). Local delivery of metronidazole-loaded N-trimethyl quaternary ammonium chitosan nanoparticles for periodontitis treatment. Curr. Drug Ther. 17 (4), 233–265. doi:10.2174/1574885517666220331145439
Gaspar, A. L., Gaspar, A. B., Contini, L. R. F., Silva, M. F., Chagas, E. G. L., Bahú, J. O., et al. (2022). Lemongrass (Cymbopogon citratus)-incorporated chitosan bioactive films for potential skincare applications. Int. J. Pharm. 628, 122301. doi:10.1016/j.ijpharm.2022.122301
Gedarawatte, S. T. G., Ravensdale, J. T., Johns, M. L., Azizi, A., Al-Salami, H., Dykes, G. A., et al. (2021). Effectiveness of gelatine and chitosan spray coating for extending shelf life of vacuum-packaged beef. Int. J. Food Sci. Technol. 56 (8), 4026–4037. doi:10.1111/ijfs.15025
Ghannam, S. F., Korayem, H. E., Farghaly, L. M., and Hosny, S. (2018). The effect of chitosan nanosilver dressing versus mesenchymal stem cells on wound healing. J. Afr. Assoc. Physiological Sci. 6 (1), 1. doi:10.4314/jaaps.v6i1
Ghimici, L., and Dinu, I. A. (2019). Removal of some commercial pesticides from aqueous dispersions using as flocculant a thymine-containing chitosan derivative. Sep. Purif. Technol. 209, 698–706. doi:10.1016/j.seppur.2018.09.013
Gholizadeh, H., Cheng, S., Pozzoli, M., Messerotti, E., Traini, D., Young, P., et al. (2019). Smart thermosensitive chitosan hydrogel for nasal delivery of ibuprofen to treat neurological disorders. Expert Opin. Drug Deliv. 16 (4), 453–466. doi:10.1080/17425247.2019.1597051
Gupta, S., Sharma, S., Kumar Nadda, A., Saad Bala Husain, M., and Gupta, A. (2022). Biopolymers from waste biomass and its applications in the cosmetic industry: a review. Mater. Today Proc. 68, 873–879. doi:10.1016/j.matpr.2022.06.422
Ha, N. M. C., Nguyen, T. H., Wang, S.-L., and Nguyen, A. D. (2019). Preparation of NPK nanofertilizer based on chitosan nanoparticles and its effect on biophysical characteristics and growth of coffee in green house. Res. Chem. Intermed. 45 (1), 51–63. doi:10.1007/s11164-018-3630-7
Haghighi, P., and Shamloo, A. (2021). Fabrication of a novel 3D scaffold for cartilage tissue repair: in-vitro and in-vivo study. Mater. Sci. Eng. C 128, 112285. doi:10.1016/j.msec.2021.112285
Hameed, A. Z., Raj, S. A., Kandasamy, J., Baghdadi, M. A., and Shahzad, M. A. (2022). Chitosan: a sustainable material for multifarious applications. Polymers 14 (12), 2335. Art. doi:10.3390/polym14122335
Han, D., Zhao, H., Gao, L., Qin, Z., Ma, J., Han, Y., et al. (2021). Preparation of carboxymethyl chitosan/phytic acid composite hydrogels for rapid dye adsorption in wastewater treatment. Colloids Surfaces A Physicochem. Eng. Aspects 628, 127355. doi:10.1016/j.colsurfa.2021.127355
Haripriyan, U., Gopinath, K. P., and Arun, J. (2022). Chitosan based nano adsorbents and its types for heavy metal removal: a mini review. Mater. Lett. 312, 131670. doi:10.1016/j.matlet.2022.131670
Hartson, M., Coyle, C., and Amin, S. (2022). Methylcellulose-chitosan smart gels for hairstyling. Cosmetics 9 (4), 69. doi:10.3390/cosmetics9040069
Hu, Z. Y., Balay, D., Hu, Y., McMullen, L. M., and Gänzle, M. G. (2019). Effect of chitosan, and bacteriocin – producing Carnobacterium maltaromaticum on survival of Escherichia coli and Salmonella Typhimurium on beef. Int. J. Food Microbiol. 290, 68–75. doi:10.1016/j.ijfoodmicro.2018.10.003
Huang, X., You, Z., Luo, Y., Yang, C., Ren, J., Liu, Y., et al. (2021). Antifungal activity of chitosan against Phytophthora infestans, the pathogen of potato late blight. Int. J. Biol. Macromol. 166, 1365–1376. doi:10.1016/j.ijbiomac.2020.11.016
Inanli, A. G., Tümerkan, E. T. A., Abed, N. E., Regenstein, J. M., and Özogul, F. (2020). The impact of chitosan on seafood quality and human health: a review. Trends Food Sci. Technol. 97, 404–416. doi:10.1016/j.tifs.2020.01.029
Islam, Md. M., Shahruzzaman, Md., Biswas, S., Nurus Sakib, Md., and Rashid, T. U. (2020). Chitosan based bioactive materials in tissue engineering applications-A review. Bioact. Mater. 5 (1), 164–183. doi:10.1016/j.bioactmat.2020.01.012
Jiang, F., Li, X., Duan, Y., Li, Q., Qu, Y., Zhong, G., et al. (2023). Extraction and characterization of chitosan from eupolyphaga sinensis walker and its application in the preparation of electrospinning nanofiber membranes. Colloids Surf. B Biointerfaces 222, 113030. doi:10.1016/j.colsurfb.2022.113030
Jiménez-Gómez, C. P., and Cecilia, J. A. (2020). Chitosan: a natural biopolymer with a wide and varied range of applications. Molecules 25 (17), 3981. doi:10.3390/molecules25173981
Jin, H., Zhao, Z., Lan, Q., Zhou, H., Mai, Z., Wang, Y., et al. (2021). Nasal delivery of hesperidin/chitosan nanoparticles suppresses cytokine storm syndrome in a mouse model of acute lung injury. Front. Pharmacol. 11, 592238. doi:10.3389/fphar.2020.592238
Jogaiah, S., Satapute, P., De Britto, S., Konappa, N., and Udayashankar, A. C. (2020). Exogenous priming of chitosan induces upregulation of phytohormones and resistance against cucumber powdery mildew disease is correlated with localized biosynthesis of defense enzymes. Int. J. Biol. Macromol. 162, 1825–1838. doi:10.1016/j.ijbiomac.2020.08.124
Joseph, S. M., Krishnamoorthy, S., Paranthaman, R., Moses, J. A., and Anandharamakrishnan, C. (2021). A review on source-specific chemistry, functionality, and applications of chitin and chitosan. Carbohydr. Polym. Technol. Appl. 2, 100036. doi:10.1016/j.carpta.2021.100036
Kadam, D., Shah, N., Palamthodi, S., and Lele, S. S. (2018). An investigation on the effect of polyphenolic extracts of Nigella sativa seedcake on physicochemical properties of chitosan-based films. Carbohydr. Polym. 192, 347–355. doi:10.1016/j.carbpol.2018.03.052
Ke, C.-L., Deng, F.-S., Chuang, C.-Y., and Lin, C.-H. (2021). Antimicrobial actions and applications of chitosan. Polymers 13 (6), 904. doi:10.3390/polym13060904
Khajavian, M., Vatanpour, V., Castro-Muñoz, R., and Boczkaj, G. (2022). Chitin and derivative chitosan-based structures — preparation strategies aided by deep eutectic solvents: a review. Carbohydr. Polym. 275, 118702. doi:10.1016/j.carbpol.2021.118702
Khalaji, S., Golshan Ebrahimi, N., and Hosseinkhani, H. (2021). Enhancement of biocompatibility of PVA/HTCC blend polymer with collagen for skin care application. Int. J. Polym. Mater. Polym. Biomaterials 70 (7), 459–468. doi:10.1080/00914037.2020.1725761
Khan, A., and Alamry, K. A. (2021). Recent advances of emerging green chitosan-based biomaterials with potential biomedical applications: a review. Carbohydr. Res. 506, 108368. doi:10.1016/j.carres.2021.108368
Kou, S., Peters, L., and Mucalo, M. (2022). Chitosan: a review of molecular structure, bioactivities and interactions with the human body and micro-organisms. Carbohydr. Polym. 282, 119132. doi:10.1016/j.carbpol.2022.119132
Kou, S., Peters, L. M., and Mucalo, M. R. (2021). Chitosan: a review of sources and preparation methods. Int. J. Biol. Macromol. 169, 85–94. doi:10.1016/j.ijbiomac.2020.12.005
Kravanja, G., Primožič, M., Knez, Ž., and Leitgeb, M. (2019). Chitosan-based (Nano)Materials for novel biomedical applications. Molecules 24 (10), 1960. doi:10.3390/molecules24101960
Kubavat, D., Trivedi, K., Vaghela, P., Prasad, K., Vijay Anand, G. K., Trivedi, H., et al. (2020). Characterization of a chitosan-based sustained release nanofertilizer formulation used as a soil conditioner while simultaneously improving biomass production of Zea mays L. Land Degrad. Dev. 31 (17), 2734–2746. doi:10.1002/ldr.3629
Kumar, A., Prajapati, D., Devi, K. A., Pal, A., Choudhary, U., Dashora, A., et al. (2021). Slow-release Zn application through Zn-chitosan nanoparticles in wheat to intensify source activity and sink strength. Plant Physiology Biochem. 168, 272–281. doi:10.1016/j.plaphy.2021.10.013
Kusnadi, K., Purgiyanti, P., Kumoro, A. C., and Legowo, A. M. (2022). The antioxidant and antibacterial activities of chitosan extract from white shrimp shell (Penaeus indicus) in the waters north of Brebes, Indonesia. Biodiversitas J. Biol. Divers. 23 (3). doi:10.13057/biodiv/d230310
Landi, L., De Miccolis Angelini, R. M., Pollastro, S., Feliziani, E., Faretra, F., and Romanazzi, G. (2017). Global transcriptome Analysis and identification of differentially expressed genes in strawberry after preharvest application of benzothiadiazole and chitosan. Front. Plant Sci. 8, 235. doi:10.3389/fpls.2017.00235
Leonhardt, E. E., Kang, N., Hamad, M. A., Wooley, K. L., and Elsabahy, M. (2019). Absorbable hemostatic hydrogels comprising composites of sacrificial templates and honeycomb-like nanofibrous mats of chitosan. Nat. Commun. 10 (1), 2307. doi:10.1038/s41467-019-10290-1
Li, F., Han, Q., Wang, W., and Wu, S. (2022). Carboxymethyl chitosan-based coatings loaded with glutathione extend the shelf-life of harvested enoki mushrooms (Flammulina velutipes). LWT 166, 113807. doi:10.1016/j.lwt.2022.113807
Li, J., and Zhuang, S. (2020). Antibacterial activity of chitosan and its derivatives and their interaction mechanism with bacteria: current state and perspectives. Eur. Polym. J. 138, 109984. doi:10.1016/j.eurpolymj.2020.109984
Li, S., Lv, H., Chen, Y., Song, H., Zhang, Y., Wang, S., et al. (2022). N-trimethyl chitosan coated targeting nanoparticles improve the oral bioavailability and antioxidant activity of vitexin. Carbohydr. Polym. 286, 119273. doi:10.1016/j.carbpol.2022.119273
Liping, L., Kexin, L., Huipu, D., Jia, L., and Jie, Z. (2020). Study on preparation of a chitosan/vitamin C complex and its properties in cosmetics. Nat. Product. Commun. 15 (10), 1934578X2094687. doi:10.1177/1934578X20946876
Liu, X., Li, X., Zhang, R., Wang, L., and Feng, Q. (2021). A novel dual microsphere based on water-soluble thiolated chitosan/mesoporous calcium carbonate for controlled dual drug delivery. Mater. Lett. 285, 129142. doi:10.1016/j.matlet.2020.129142
Liyanage, C. S., Gonapinuwala, S. T., Fernando, C. A. N., and De Croos, M. D. S. T. (2022). Physico-chemical properties of chitosan extracted from Whiteleg shrimp (Litopenaeus vannamei) processing shell waste in Sri Lanka. Sri Lanka J. Aquatic Sci. 27 (2), 107–122. doi:10.4038/sljas.v27i2.7600
Lopez-Moya, F., Suarez-Fernandez, M., and Lopez-Llorca, L. V. (2019). Molecular mechanisms of chitosan interactions with fungi and plants. Int. J. Mol. Sci. 20 (2), 332. doi:10.3390/ijms20020332
Ma, P., Wu, W., Wei, Y., Ren, L., Lin, S., and Wu, J. (2021). Biomimetic gelatin/chitosan/polyvinyl alcohol/nano-hydroxyapatite scaffolds for bone tissue engineering. Mater. Des. 207, 109865. doi:10.1016/j.matdes.2021.109865
Madni, A., Khan, R., Ikram, M., Naz, S. S., Khan, T., and Wahid, F. (2019). Fabrication and characterization of chitosan–vitamin C–lactic acid composite membrane for potential skin tissue engineering. Int. J. Polym. Sci. 2019, 1–8. doi:10.1155/2019/4362395
Maghsoudi, M., Abbasian, M., and Farhadi, K. (2021). Design and synthesis of novel chitosan–based nanocomposite containing mesoporous nanosilica MCM-41: effective absorbent for the elimination of Pb (II) from aqueous solution. J. Elastomers Plastics 53 (5), 469–488. doi:10.1177/0095244320951895
Marzieh, M.-N., Zahra, F., Tahereh, E., and Sara, K.-N. (2019). Comparison of the physicochemical and structural characteristics of enzymatic produced chitin and commercial chitin. Int. J. Biol. Macromol. 139, 270–276. doi:10.1016/j.ijbiomac.2019.07.217
Mejdoub-Trabelsi, B., Touihri, S., Ammar, N., Riahi, A., and Daami-Remadi, M. (2020). Effect of chitosan for the control of potato diseases caused by Fusarium species. J. Phytopathology 168 (1), 18–27. doi:10.1111/jph.12847
Meng, D., Garba, B., Ren, Y., Yao, M., Xia, X., Li, M., et al. (2020). Antifungal activity of chitosan against Aspergillus ochraceus and its possible mechanisms of action. Int. J. Biol. Macromol. 158, 1063–1070. doi:10.1016/j.ijbiomac.2020.04.213
Miyazawa, N., Yoshimoto, H., Kurihara, S., Hamaya, T., and Eguchi, F. (2018). Improvement of diet-induced obesity by ingestion of mushroom chitosan prepared from Flammulina velutipes. J. Oleo Sci. 67 (2), 245–254. doi:10.5650/jos.ess17159
Modi, S., Kumar, S., and Dubey, P. K. (2021). Dynamics of chitosan based NPK-nanofertilizers in greenhouse cucumber production system. J. Environ. Biol. 42 (1), 162–168. doi:10.22438/jeb/42/1/MRN-1251
Mondéjar-López, M., López-Jiménez, A. J., Martínez, J. C. G., Ahrazem, O., Gómez-Gómez, L., and Niza, E. (2022). Thymoquinone-loaded chitosan nanoparticles as natural preservative agent in cosmetic products. Int. J. Mol. Sci. 23 (2), 898. doi:10.3390/ijms23020898
Montaño-Sánchez, E., Torres-Martínez, B. M., Vargas-Sánchez, R. D., Huerta-Leidenz, N., Sánchez-Escalante, A., Beriain, M. J., et al. (2020). Effects of chitosan coating with green tea aqueous extract on lipid oxidation and microbial growth in pork chops during chilled storage. Foods 9 (6), 766. doi:10.3390/foods9060766
Morin-Crini, N., Lichtfouse, E., Torri, G., and Crini, G. (2019a). “Fundamentals and applications of chitosan,” in Sustainable agriculture reviews 35. Editors G. Crini,, and E. Lichtfouse (Cham: Springer International Publishing), 35, 49–123. doi:10.1007/978-3-030-16538-3_2
Morin-Crini, N., Lichtfouse, E., Torri, G., and Crini, G. (2019b). Applications of chitosan in food, pharmaceuticals, medicine, cosmetics, agriculture, textiles, pulp and paper, biotechnology, and environmental chemistry. Environ. Chem. Lett. 17 (4), 1667–1692. doi:10.1007/s10311-019-00904-x
Mukhtar, M., Fényes, E., Bartos, C., Zeeshan, M., and Ambrus, R. (2021). Chitosan biopolymer, its derivatives and potential applications in nano-therapeutics: a comprehensive review. Eur. Polym. J. 160, 110767. doi:10.1016/j.eurpolymj.2021.110767
Muthu, M., Gopal, J., Chun, S., Devadoss, A. J. P., Hasan, N., and Sivanesan, I. (2021). Crustacean waste-derived chitosan: antioxidant properties and future perspective. Antioxidants 10 (2), 228. doi:10.3390/antiox10020228
Navarrete-Bolaños, J. L., González-Torres, I., Vargas-Bermúdez, V. H., and Jiménez-Islas, H. (2020). A biotechnological insight to recycle waste: analyzing the spontaneous fermentation of shrimp waste to design the hydrolysis process of chitin into n-acetylglucosamine. Rev. Mex. Ing. Quím. 19 (1), 263–274. Art. 1. doi:10.24275/rmiq/Bio544
Negm, N. A., Hefni, H. H. H., Abd-Elaal, A. A. A., Badr, E. A., and Abou Kana, M. T. H. (2020). Advancement on modification of chitosan biopolymer and its potential applications. Int. J. Biol. Macromol. 152, 681–702. doi:10.1016/j.ijbiomac.2020.02.196
Nguyen, N. T., Nguyen, N. T., and Nguyen, V. A. (2020). In situ synthesis and characterization of ZnO/chitosan nanocomposite as an adsorbent for removal of Congo red from aqueous solution. Adv. Polym. Technol. 2020, 1–8. doi:10.1155/2020/3892694
Oladzadabbasabadi, N., Mohammadi Nafchi, A., Ariffin, F., Wijekoon, M. M. J. O., Al-Hassan, A. A., Dheyab, M. A., et al. (2022). Recent advances in extraction, modification, and application of chitosan in packaging industry. Carbohydr. Polym. 277, 118876. doi:10.1016/j.carbpol.2021.118876
Pakizeh, M., Moradi, A., and Ghassemi, T. (2021). Chemical extraction and modification of chitin and chitosan from shrimp shells. Eur. Polym. J. 159, 110709. doi:10.1016/j.eurpolymj.2021.110709
Pan, Q., Zhou, C., Yang, Z., He, Z., Wang, C., Liu, Y., et al. (2022). Preparation and characterization of chitosan derivatives modified with quaternary ammonium salt and quaternary phosphate salt and its effect on tropical fruit preservation. Food Chem. 387, 132878. doi:10.1016/j.foodchem.2022.132878
Pellis, A., Guebitz, G. M., and Nyanhongo, G. S. (2022). Chitosan: sources, processing and modification techniques. Gels 8 (7), 393. Art. doi:10.3390/gels8070393
Peralta-Ruiz, Y., Tovar, C. D. G., Sinning-Mangonez, A., Coronell, E. A., Marino, M. F., and Chaves-Lopez, C. (2020). Reduction of postharvest quality loss and microbiological decay of tomato “chonto” (Solanum lycopersicum L.) using chitosan-E essential oil-based edible coatings under low-temperature storage. Polymers 12 (8), 1822. Art. doi:10.3390/polym12081822
Petrick, J., Ibadurrohman, M., and Slamet, C. (2020). Synthesis of chitosan/TiO2 nanocomposite for antibacterial sunscreen application. AIP Conf. Proc. 2255 (1), 060020. doi:10.1063/5.0014126
Philippart, C., Hendrickx, B., Rharbaoui, S., Natalizio, A., Boisnic, S., Micheels, P., et al. (2021). Safety and efficacy of a carboxymethyl chitosan dermal injection device for the treatment of skin defects: a first-in-man, pilot, comparative, split-body study. Eur. J. Dermatology 31 (4), 549–558. doi:10.1684/ejd.2021.4091
Qin, Y., Li, P., and Guo, Z. (2020). Cationic chitosan derivatives as potential antifungals: a review of structural optimization and applications. Carbohydr. Polym. 236, 116002. doi:10.1016/j.carbpol.2020.116002
Qing, L., Lijie, W., Jingjing, Z., Guodong, G., and Zhanyong, G. (2019). Significantly enhanced antioxidant activity of chitosan through chemical modification with coumarins. Polym. Chem. 10 (12), 1480–1488. doi:10.1039/C8PY01790E
Rahman, M., Mukta, J. A., Sabir, A. A., Gupta, D. R., Mohi-Ud-Din, M., Hasanuzzaman, M., et al. (2018). Chitosan biopolymer promotes yield and stimulates accumulation of antioxidants in strawberry fruit. PLOS ONE 13 (9), e0203769. doi:10.1371/journal.pone.0203769
Rakshit, S., Pal, K., Mondal, S., Jana, A., Mondal, K. C., and Halder, S. K. (2023). Extraction of chitosan from biologically-derived chitin by bacterial chitin deacetylase: process optimization and product quality assessment. Int. J. Biol. Macromol. 244, 125389. doi:10.1016/j.ijbiomac.2023.125389
Rani, M., Rachna, C., and Shanker, U. (2020). Metal oxide-chitosan based nanocomposites for efficient degradation of carcinogenic PAHs. J. Environ. Chem. Eng. 8 (3), 103810. doi:10.1016/j.jece.2020.103810
Resende, A. H. M., Farias, J. M., Silva, D. D. B., Rufino, R. D., Luna, J. M., Stamford, T. C. M., et al. (2019). Application of biosurfactants and chitosan in toothpaste formulation. Colloids Surfaces B Biointerfaces 181, 77–84. doi:10.1016/j.colsurfb.2019.05.032
Ressutte, J. B., Saranti, T. F. S., Moura, M. R., Pozza, M. S. S., Scapim, M. R. S., Stafussa, A. P., et al. (2022). Citric acid incorporated in a chitosan film as an active packaging material to improve the quality and duration of matured cheese shelf life. J. Dairy Res. 89 (2), 201–207. doi:10.1017/S0022029922000383
Rios, R. V., Garzón, R., Lannes, S. C. S., and Rosell, C. M. (2018). Use of succinyl chitosan as fat replacer on cake formulations. LWT 96, 260–265. doi:10.1016/j.lwt.2018.05.041
Rodrigues, C., de Mello, J. M. M., Dalcanton, F., Macuvele, D. L. P., Padoin, N., Fiori, M. A., et al. (2020). Mechanical, thermal and antimicrobial properties of chitosan-based-nanocomposite with potential applications for food packaging. J. Polym. Environ. 28 (4), 1216–1236. doi:10.1007/s10924-020-01678-y
Saharan, V., Kumaraswamy, R. V., Choudhary, R. C., Kumari, S., Pal, A., Raliya, R., et al. (2016). Cu-chitosan nanoparticle mediated sustainable approach to enhance seedling growth in maize by mobilizing reserved food. J. Agric. Food Chem. 64 (31), 6148–6155. doi:10.1021/acs.jafc.6b02239
Saheed, I. O., Oh, W. D., and Suah, F. B. M. (2021). Chitosan modifications for adsorption of pollutants – a review. J. Hazard. Mater. 408, 124889. doi:10.1016/j.jhazmat.2020.124889
Santos, V. P., Marques, N. S. S., Maia, P. C. S. V., Lima, M. A. B. de, Franco, L. de O., and Campos-Takaki, G. M. de. (2020). Seafood waste as attractive source of chitin and chitosan production and their applications. Int. J. Mol. Sci. 21 (12), 4290. doi:10.3390/ijms21124290
Savin, S., Craciunescu, O., Oancea, A., Ilie, D., Ciucan, T., Antohi, L. S., et al. (2020). Antioxidant, cytotoxic and antimicrobial activity of chitosan preparations extracted from ganoderma lucidum mushroom. Chem. Biodivers. 17 (7), e2000175. doi:10.1002/cbdv.202000175
Serrano-León, J. S., Bergamaschi, K. B., Yoshida, C. M. P., Saldaña, E., Selani, M. M., Rios-Mera, J. D., et al. (2018). Chitosan active films containing agro-industrial residue extracts for shelf life extension of chicken restructured product. Food Res. Int. 108, 93–100. doi:10.1016/j.foodres.2018.03.031
Sessarego, S., Rodrigues, S. C. G., Xiao, Y., Lu, Q., and Hill, J. M. (2019). Phosphonium-enhanced chitosan for Cr(VI) adsorption in wastewater treatment. Carbohydr. Polym. 211, 249–256. doi:10.1016/j.carbpol.2019.02.003
Shahrajabian, M. H., Chaski, C., Polyzos, N., Tzortzakis, N., and Petropoulos, S. A. (2021). Sustainable agriculture systems in vegetable production using chitin and chitosan as plant biostimulants. Biomolecules 11 (6), 819. doi:10.3390/biom11060819
Sharma, G., Kumar, A., Devi, K. A., Prajapati, D., Bhagat, D., Pal, A., et al. (2020a). Chitosan nanofertilizer to foster source activity in maize. Int. J. Biol. Macromol. 145, 226–234. doi:10.1016/j.ijbiomac.2019.12.155
Sharma, G., Naushad, Mu., Kumar, A., Kumar, A., Ahamad, T., and Stadler, F. J. (2020b). Facile fabrication of chitosan-cl-poly(AA)/ZrPO4 nanocomposite for remediation of rhodamine B and antimicrobial activity. J. King Saud Univ. - Sci. 32 (2), 1359–1365. doi:10.1016/j.jksus.2019.11.028
Shen, T., Dai, K., Yu, Y., Wang, J., and Liu, C. (2020). Sulfated chitosan rescues dysfunctional macrophages and accelerates wound healing in diabetic mice. Acta Biomater. 117, 192–203. doi:10.1016/j.actbio.2020.09.035
Shih, P.-Y., Liao, Y.-T., Tseng, Y.-K., Deng, F.-S., and Lin, C.-H. (2019). A potential antifungal effect of chitosan against Candida albicans is mediated via the inhibition of SAGA complex component expression and the subsequent alteration of cell surface integrity. Front. Microbiol. 10, 602. doi:10.3389/fmicb.2019.00602
Shinde, U. A., Joshi, P. N., Jain, D. D., and Singh, K. (2019). Preparation and evaluation of N-trimethyl chitosan nanoparticles of flurbiprofen for ocular delivery. Curr. Eye Res. 44 (5), 575–582. doi:10.1080/02713683.2019.1567793
Sixto-Berrocal, A. M., Vázquez-Aldana, M., Miranda-Castro, S. P., Martínez-Trujillo, M. A., and Cruz-Díaz, M. R. (2023). Chitin/chitosan extraction from shrimp shell waste by a completely biotechnological process. Int. J. Biol. Macromol. 230, 123204. doi:10.1016/j.ijbiomac.2023.123204
Srinivasan, H., Kanayairam, V., and Ravichandran, R. (2018). Chitin and chitosan preparation from shrimp shells Penaeus monodon and its human ovarian cancer cell line, PA-1. Int. J. Biol. Macromol. 107, 662–667. doi:10.1016/j.ijbiomac.2017.09.035
Sudatta, B. P., Sugumar, V., Varma, R., and Nigariga, P. (2020). Extraction, characterization and antimicrobial activity of chitosan from pen shell, Pinna bicolor. Int. J. Biol. Macromol. 163, 423–430. doi:10.1016/j.ijbiomac.2020.06.291
Sun, X., Zhang, J., Chen, Y., Mi, Y., Tan, W., Li, Q., et al. (2019). Synthesis, characterization, and the antioxidant activity of carboxymethyl chitosan derivatives containing thiourea salts. Polymers 11 (11), 1810. doi:10.3390/polym11111810
Tamer, T. M., Zhou, H., Hassan, M. A., Abu-Serie, M. M., Shityakov, S., Elbayomi, S. M., et al. (2023). Synthesis and physicochemical properties of an aromatic chitosan derivative: in vitro antibacterial, antioxidant, and anticancer evaluations, and in silico studies. Int. J. Biol. Macromol. 240, 124339. doi:10.1016/j.ijbiomac.2023.124339
Tang, X., Huang, T., Zhang, S., Wang, W., and Zheng, H. (2020). The role of sulfonated chitosan-based flocculant in the treatment of hematite wastewater containing heavy metals. Colloids Surfaces A Physicochem. Eng. Aspects 585, 124070. doi:10.1016/j.colsurfa.2019.124070
Terkula Iber, B., Azman Kasan, N., Torsabo, D., and Wese Omuwa, J. (2022). A review of various sources of chitin and chitosan in nature. J. Renew. Mater. 10 (4), 1097–1123. doi:10.32604/jrm.2022.018142
Theerawattanawit, C., Phaiyarin, P., Wanichwecharungruang, S., Noppakun, N., Asawanonda, P., and Kumtornrut, C. (2022). The efficacy and safety of chitosan on facial skin sebum. Skin Pharmacol. Physiology 35 (1), 23–30. doi:10.1159/000517965
Thomas, M. S., Koshy, R. R., Mary, S. K., Thomas, S., and Pothan, L. A. (2019). Starch, chitin and chitosan based composites and nanocomposites. Cham: Springer International Publishing. doi:10.1007/978-3-030-03158-9
Tithito, T., Suntornsaratoon, P., Charoenphandhu, N., Thongbunchoo, J., Krishnamra, N., Tang, I. M., et al. (2019). Fabrication of biocomposite scaffolds made with modified hydroxyapatite inclusion of chitosan-grafted-poly(methyl methacrylate) for bone tissue engineering. Biomed. Mater. 14 (2), 025013. doi:10.1088/1748-605X/ab025f
Tovar, G. I., Briceño, S., Suarez, J., Flores, S., and González, G. (2020). Biogenic synthesis of iron oxide nanoparticles using Moringa oleifera and chitosan and its evaluation on corn germination. Environ. Nanotechnol. Monit. Manag. 14, 100350. doi:10.1016/j.enmm.2020.100350
Tuğcu-Demiröz, F., Saar, S., Kara, A. A., Yıldız, A., Tunçel, E., and Acartürk, F. (2021). Development and characterization of chitosan nanoparticles loaded nanofiber hybrid system for vaginal controlled release of benzydamine. Eur. J. Pharm. Sci. 161, 105801. doi:10.1016/j.ejps.2021.105801
Valdez, M. I., Garcia, J., Ubeda-Manzanaro, M., Martinez, A., and Rodrigo, D. (2022). Insect chitosan as a natural antimicrobial against vegetative cells of Bacillus cereus in a cooked rice matrix. Food Microbiol. 107, 104077. doi:10.1016/j.fm.2022.104077
Vanti, G. L., Masaphy, S., Kurjogi, M., Chakrasali, S., and Nargund, V. B. (2020). Synthesis and application of chitosan-copper nanoparticles on damping off causing plant pathogenic fungi. Int. J. Biol. Macromol. 156, 1387–1395. doi:10.1016/j.ijbiomac.2019.11.179
Vedula, S. S., and Yadav, G. D. (2022). Wastewater treatment containing methylene blue dye as pollutant using adsorption by chitosan lignin membrane: development of membrane, characterization and kinetics of adsorption. J. Indian Chem. Soc. 99 (1), 100263. doi:10.1016/j.jics.2021.100263
Verlee, A., Mincke, S., and Stevens, C. V. (2017). Recent developments in antibacterial and antifungal chitosan and its derivatives. Carbohydr. Polym. 164, 268–283. doi:10.1016/j.carbpol.2017.02.001
Vieira, T. M., Moldão-Martins, M., and Alves, V. D. (2021). Composite coatings of chitosan and alginate emulsions with olive oil to enhance postharvest quality and shelf life of fresh figs (Ficus carica L. Cv. ‘pingo de mel’). Foods 10 (4), 718. doi:10.3390/foods10040718
Wahab, F., Iber, B. T., Chik, C. E. N., Abdullah, S. R. S., Alianto, C. E., Aslamyah, S., et al. (2023). Chitin and chitosan extraction: a comparison of three crab species from fresh, brackish and marine water environments. Bioresour. Technol. Rep. 23, 101517. doi:10.1016/j.biteb.2023.101517
Wang, B., Bai, Z., Jiang, H., Prinsen, P., Luque, R., Zhao, S., et al. (2019). Selective heavy metal removal and water purification by microfluidically-generated chitosan microspheres: characteristics, modeling and application. J. Hazard. Mater. 364, 192–205. doi:10.1016/j.jhazmat.2018.10.024
Wang, J., Chin, D., Poon, C., Mancino, V., Pham, J., Li, H., et al. (2021). Oral delivery of metformin by chitosan nanoparticles for polycystic kidney disease. J. Control. Release 329, 1198–1209. doi:10.1016/j.jconrel.2020.10.047
Wang, R., Zhang, X., Zhu, J., Bai, J., Gao, L., Liu, S., et al. (2020). Facile preparation of self-assembled chitosan-based composite hydrogels with enhanced adsorption performances. Colloids Surfaces A Physicochem. Eng. Aspects 598, 124860. doi:10.1016/j.colsurfa.2020.124860
Wang, W., Meng, Q., Li, Q., Liu, J., Zhou, M., Jin, Z., et al. (2020a). Chitosan derivatives and their application in biomedicine. Int. J. Mol. Sci. 21 (2), 487. doi:10.3390/ijms21020487
Wang, W., Xue, C., and Mao, X. (2020b). Chitosan: structural modification, biological activity and application. Int. J. Biol. Macromol. 164, 4532–4546. doi:10.1016/j.ijbiomac.2020.09.042
Wei, L., Tan, W., Wang, G., Li, Q., Dong, F., and Guo, Z. (2019). The antioxidant and antifungal activity of chitosan derivatives bearing Schiff bases and quaternary ammonium salts. Carbohydr. Polym. 226, 115256. doi:10.1016/j.carbpol.2019.115256
Xie, X., Kurashina, Y., Matsui, M., Nomoto, T., Itoh, M., Okano, H. J., et al. (2022). Transdermal delivery of bFGF with sonophoresis facilitated by chitosan nanocarriers. J. Drug Deliv. Sci. Technol. 75, 103675. doi:10.1016/j.jddst.2022.103675
Xing, Y., Wang, X., Guo, X., Yang, P., Yu, J., Shui, Y., et al. (2021). Comparison of antimicrobial activity of chitosan nanoparticles against bacteria and fungi. Coatings 11 (7), 769. doi:10.3390/coatings11070769
Xoca-Orozco, L.-Á., Cuellar-Torres, E. A., González-Morales, S., Gutiérrez-Martínez, P., López-García, U., Herrera-Estrella, L., et al. (2017). Transcriptomic Analysis of avocado hass (persea americana mill) in the interaction system fruit-chitosan-colletotrichum. Front. Plant Sci. 8, 956. doi:10.3389/fpls.2017.00956
Yadav, M., Goswami, P., Paritosh, K., Kumar, M., Pareek, N., and Vivekanand, V. (2019). Seafood waste: a source for preparation of commercially employable chitin/chitosan materials. Bioresour. Bioprocess. 6 (1), 8. doi:10.1186/s40643-019-0243-y
Yarnpakdee, S., Kaewprachu, P., Jaisan, C., Senphan, T., Nagarajan, M., and Wangtueai, S. (2022). Extraction and physico–chemical characterization of chitosan from Mantis shrimp (oratosquilla nepa) shell and the development of bio-composite film with agarose. Polymers 14 (19), 3983. doi:10.3390/polym14193983
Yu, J., Wang, D., Geetha, N., Khawar, K. M., Jogaiah, S., and Mujtaba, M. (2021). Current trends and challenges in the synthesis and applications of chitosan-based nanocomposites for plants: a review. Carbohydr. Polym. 261, 117904. doi:10.1016/j.carbpol.2021.117904
Zafar, A., Afzal, M., Quazi, A. M., Yasir, M., Kazmi, I., Al-Abaasi, F. A., et al. (2021). Chitosan-ethyl cellulose microspheres of domperidone for nasal delivery: preparation, in-vitro characterization, in-vivo study for pharmacokinetic evaluation and bioavailability enhancement. J. Drug Deliv. Sci. Technol. 63, 102471. doi:10.1016/j.jddst.2021.102471
Zainol Abidin, N. A., Kormin, F., Zainol Abidin, N. A., Mohamed Anuar, N. A. F., and Abu Bakar, M. F. (2020). The potential of insects as alternative sources of chitin: an overview on the chemical method of extraction from various sources. Int. J. Mol. Sci. 21 (14), 4978. doi:10.3390/ijms21144978
Zango, Z. U., Dennis, J. O., Aljameel, A. I., Usman, F., Ali, M. K. M., Abdulkadir, B. A., et al. (2022). Effective removal of methylene blue from simulated wastewater using ZnO-chitosan nanocomposites: optimization, kinetics, and isotherm studies. Molecules 27 (15), 4746. Art. 15. doi:10.3390/molecules27154746
Zhang, H., He, P., Kang, H., and Li, X. (2018). Antioxidant and antimicrobial effects of edible coating based on chitosan and bamboo vinegar in ready to cook pork chops. LWT 93, 470–476. doi:10.1016/j.lwt.2018.04.005
Zhang, M., Zhang, F., Li, C., An, H., Wan, T., and Zhang, P. (2022). Application of chitosan and its derivative polymers in clinical medicine and agriculture. Polymers 14 (5), 958. doi:10.3390/polym14050958
Zhang, X., Chen, Y., Han, J., Mo, J., Dong, P., Zhuo, Y., et al. (2019). Biocompatiable silk fibroin/carboxymethyl chitosan/strontium substituted hydroxyapatite/cellulose nanocrystal composite scaffolds for bone tissue engineering. Int. J. Biol. Macromol. 136, 1247–1257. doi:10.1016/j.ijbiomac.2019.06.172
Zhang, Y.-L., Cui, Q.-L., Wang, Y., Shi, F., Fan, H., Zhang, Y.-Q., et al. (2021). Effect of edible carboxymethyl chitosan-gelatin based coating on the quality and nutritional properties of different sweet cherry cultivars during postharvest storage. Coatings 11 (4), 396. doi:10.3390/coatings11040396
Zhao, R., Guan, W., Zheng, P., Tian, F., Zhang, Z., Sun, Z., et al. (2022). Development of edible composite film based on chitosan nanoparticles and their application in packaging of fresh red sea bream fillets. Food control. 132, 108545. doi:10.1016/j.foodcont.2021.108545
Zhu, X., Yuan, P., Zhang, T., Wang, Z., Cai, D., Chen, X., et al. (2022). Effect of carboxymethyl chitosan on the storage stability of frozen dough: state of water, protein structures and quality attributes. Food Res. Int. 151, 110863. doi:10.1016/j.foodres.2021.110863
Keywords: chitosan and its derivatives, chitosan-based nanocomposites, engineering applications, functional properties, physicochemical properties
Citation: El-Araby A, Janati W, Ullah R, Ercisli S and Errachidi F (2024) Chitosan, chitosan derivatives, and chitosan-based nanocomposites: eco-friendly materials for advanced applications (a review). Front. Chem. 11:1327426. doi: 10.3389/fchem.2023.1327426
Received: 24 October 2023; Accepted: 13 December 2023;
Published: 04 January 2024.
Edited by:
Shahid Ul Islam, Jamia Millia Islamia, IndiaReviewed by:
Iraj Karimi Sani, Urmia University, IranGuangyang Liu, Chinese Academy of Agricultural Sciences, China
Copyright © 2024 El-Araby, Janati, Ullah, Ercisli and Errachidi. This is an open-access article distributed under the terms of the Creative Commons Attribution License (CC BY). The use, distribution or reproduction in other forums is permitted, provided the original author(s) and the copyright owner(s) are credited and that the original publication in this journal is cited, in accordance with accepted academic practice. No use, distribution or reproduction is permitted which does not comply with these terms.
*Correspondence: Abir El-Araby, abir.elaraby@usmba.ac.ma