- 1Department of Food Technology and Nutrition, School of Agriculture, Lovely Professional University, Phagwara, Punjab, India
- 2Department of Horticulture, Faculty of Agriculture, Ataturk University, Erzurum, Türkiye
- 3HGF Agro, ATA Teknokent, Erzurum, Türkiye
- 4Seed Science and Technology, ICAR-Indian Agricultural Research Institute, New Delhi, India
- 5Department of Food Analysis and Chemistry, Faculty of Technology, Tomas Bata University in Zlín, Zlín, Czechia
Grape leaves, scientifically known as Vitis vinifera, the primary by-product obtained after the processing of grapes, are gathered in enormous amounts and disposed of as agricultural waste. For more sustainable agriculture and better food systems, it is crucial to investigate these byproducts’ nutritional values. The primary bioactive compounds present in grape leaves are quercetin, resveratrol, caffeic acid, kaempferol, and gallic acid, which favour pharmacological effects on human health such as antioxidant, anti-inflammatory, anti-obesity, anti-diabetic, and hepatoprotective. Furthermore, grape leaves extract has been used as a functional ingredient for creating both food and non-food products. The aim of the current review is to review the nutritional and phytochemical composition of various varieties of grape leaves, their health-promoting characteristics and their applications. The study also highlights the various extraction techniques including conventional and non-conventional methods for extracting the various bioactive compounds present in grape leaves. Grape leaves bioactives can be extracted using environmentally safe and sustainable processes, which are in line with the rising demand for eco-friendly and healthful products worldwide. These methods are perfectly suited to the changing needs of both customers and industries since they lessen environmental effect, enhance product quality, and offer financial advantages.
1 Introduction
Grapes are one of the world’s most valued horticultural crops. As per the information given by the Food and Agriculture Organization of the United Nations (FAO) statistical database, grape production in 2019 was 77 million tons (Li et al., 2022). The production of grapes often known as viticulture is one of the biggest agricultural product. Around 10,000 varieties of grapes can be found worldwide (Ins et al., 2021). China produces the most grapes in the world (Li et al., 2022). Moreover, other countries which focus on the production part of fresh grape leaves are India, Mexico, Iran, Egypt, Brazil and Turkey (Ins et al., 2021). Grape leaves, a vegetative portion of grapes are still neglected by-product of the sector where bioactive components of grapevine are investigated at the grape level (Maia et al., 2019).
Grape (Vitis vinifera L.) is one of the most significant perennial crops worldwide (Gülcü et al., 2020). Vine leaves have long been consumed both fresh and canned. Vine leaves that have been brined are a good source of organic acids, sugars, and phenolic compounds. The primary output of the vine industry is wine and alcoholic beverages. However, they do not stand alone in the market. Vitis vinifera employed in the grape business provides fresh grapes, raisins, juices, jellies, molasses, jam, and dishes prepared with vine leaves. Turkish, Balkan and Middle Eastern cuisines have long been known for their framed dishes made with freshly harvested, stuffed grape leaves (dolma) (Banjanin et al., 2021). There are currently over 2000 distinct cultivars of Vitis vinifera grown all over the world and utilised for grape production. About 50% of the grapes grown world-wide are used to make wine, which is a highly strategic industry for the economics of many different nations. The remaining 50% are either dried or used to make grape juice or must (Maia et al., 2019). According to the Food and Agriculture Organization (FAO), approximately 78 million of grapes are produced globally (Madadian et al., 2022).
When grape vines are produced, grape leaves are discarded, which decreases the economic growth of farmers. However, if grape leaves were used for their therapeutic properties, farmers might see a rise in their financial situation as well as environmental benefits for sustainable viticulture (dos Santos Lacerda et al., 2014). In Southwestern Europe, where grape leaves are a major environmental issue since they are released as waste after winemaking. In order to address this issue, bioactive components from Vitis vinifera leaves are extracted and employed (Lantzouraki et al., 2020). Vitamins, anthocyanins, flavonoids, polyphenols, and stillbenoids are some of the secondary bioactive metabolites found in grapes that are used to make supplements and cosmetics (Maia et al., 2019). Numerous researches on the V. vinifera L. plant materials demonstrate their value as a top source of minerals, organic acids (such as malic, oxalic, fumaric, ascorbic, citric, and tartaric acid), polyphenols, enzymes, and other nutrients essential for human nutrition (Pantelić et al., 2017).
Furthermore, oxidative stress caused by both intrinsic factors (e.g., DNA damage) and extrinsic factors such as lifestyle, cigarette smoking, pollution, and radiation, leads to the production of Reactive Oxygen Species (ROS), which in turn results in aging and the development of neurodegenerative disorders, including Parkinson’s Disease and Alzheimer’s Disease. Notably, the polyphenols found in Vitis vinifera leaves have the ability to protect against oxidative stress. Activity of endogenous antioxidants are enhanced by polyphenols; other mechanism of polyphenols is by preventing the production of Reactive Oxygen Species (ROS) and can delay or block oxidative stress. Several polyphenols from Vitis Vinifera leaves were purified and isolated, this offers the food and pharmaceuticals industries new and profitable prospects (Dresch et al., 2018; Uddin et al., 2020). Generation of free radicals and oxidation are prevented by grape leaf extract which possesses antioxidant activity due to high polyphenolic contents in it. According to the several studies, Vitis vinifera leaf extract can reduce the release of IL-8 which is induced in the TNF-α pathway which is a cytokine which trigger inflammation process (Vinceković et al., 2019; Jang et al., 2021). Grapes leaves and grapes are considered a vey rich source of phenolic compounds and have a huge potential for the development of new foods and beverage (Vilela and Pinto, 2019).
2 Morphology
The leaf of Vitis vinifera is a typical mesomorphic leaf with a dorsoventrally flattened lamina, where it is simple to differentiate between the colouration of the upper surface (green-dark) and lower surface (clear green). Despite some differences in the morphological and anatomical characteristics of Vitis vinifera cultivars, this species’ leaves are typically big, alternating, petiolate, and palmately lobed, giving them the appearance of being in the shape of a hand (Cosme et al., 2017). Grapevine leaf tissue includes cuticle, upper andmesophyll, and lower epidermis, which contains spongy and palisade parenchyma with embedded xylem and phloem. The tissue between the two epidermal layers of a leaf is known as the mesophyll. The mesophyll is composed of four to six layers of spongy parenchyma, also known as spongy mesophyll, and a single layer of elongated cells known as palisade parenchyma (Keller, 2020). The ability of the epidermis to protect the leaf from uncontrolled water loss, environmental degradation, and microbial attack is formed by the cuticle, which is a separate layer formed by the incrustation of the outer wall with cutin (Cosme et al., 2017).
Mesophyll, the primary component of a leaf, is a plant’s photosynthetic system. Although it does not have phenolic chemicals, the tissue is primarily chlorophyll rich. The cuticle, a very thin but significant layer rich in phenolics (particularly hydroxycinnamic acids and flavonoids), protects the top and lower epidermis, which in turn protects the mesophyll (Štambuk et al., 2022).
There are many factors which determined the form of grape leaves other than serration and lobing. According to the Galet formula and Procrustean method, landmarks of the leaf are plotted in orange dot while pseudo-landmarks are in magenta lines. The regions of the leaf are indicated as’p’- proximal, “d”-distal and, “m” midvein. Midvein as L1, distal/superior as L2, proximal/inferior as L3 and petiolar veins as L4 are provided in the Galet formula and Procrustean methods. According to Galet formula and Procrustean methods, landmarks and pseudo-landmarks are provided which combine and generate vectors, which are styled as black arrows and have their names specified. Each arrow’s base and tip represent the start and finish of a vector along the blade (Figure 1). The direction of the vein vector starting at a similar branch site within the leaf and ending at the tip is indicated by an arrow starting from the vein tip. Gallet’s nomenclature is on the left side of the sheet. L1, L2, L3 and L4 refer to the middle, distal veins (Chitwood, 2021).
3 Phytochemical composition of grape leaves of different varieties
The vine-wine industry produces a lot of agricultural waste. The wastes have almost no value and may need to be disposed of at a higher expense. These phenolic compound-containing wastes all serve as a source for high-value goods (Simonetti et al., 2020). Grapevine leaves have been found to contain 132 phenolic chemicals in total (Goufo et al., 2020). The phenolic content of Michele Palier grape leaves, which was 47.76 ± 1.26 g/L, was the highest of all the grape leaf kinds. The amount of non-flavonoids in Maraština was highest at 27.7 ± 0.9 g/L. Narince had the greatest concentration of total flavonoids at 575.25 ± 0.01 g/L. The highest amount of total flavanols, 2.786 ± 0.03 g/L, was found in Merlot. The highest FRAP content found in Merlot was 183.5 ± 2.2 mM. The greatest TEAC ABTS level was found in Yapıncak, which was 524.42 ± 27.51 μmol/g. Narince had the highest antioxidant percentage, which was 88.46% ± 0.03% (Table 1) (Katalinic et al., 2013; Al Juhaimi et al., 2019; Gülcü et al., 2020).
It was discovered that total flavonoids (TFC) and phenolic acids were the highest concentration in grape leaves, which were detected in the extract of V. viniferaleaf extract (16.75 mg/g total flavonoid content and 6.39 mg/g caffeic acid derivatives). Compared to some grape varieties from Turkey, Croatia, or India, V. vinifera leaves from Romania had a greater total flavonoid concentration (Moldovan et al., 2020). Thus, the secondary metabolites of V. vinifera are a great source of natural antioxidants that can be used to improve human health.
4 Color property of grape leaves
One of the most crucial characteristics for fresh grape leaves is colour (Güler and Candemir, 2014). Based on a global standard suggested by the Commission Internationale d'Eclairage (CIE) and utilised in colour measurements, this colour measurement method was created in 1931. The system was updated in 1976 and given the name CIE L, a, b (Doğan and Cüneyt, 2020). L* stands for lightness in the CIE Lab colour space, which ranges from 0 (black) to 100 (white); a* for redness, which ranges from green (-a*) to red (+a*); and b* for yellowness, which ranges from blue (-b*) to yellow (+b*) (Flamminii et al., 2020). In a 2014 study by Güler and Candemir, the colour characteristics of grape leaves from several grape cultivars were evaluated. By using a Minolta Colorimeter, the samples’ L*, a*, and b* values were determined, and a/b values were computed. Samples’ L* values ranged from 37.92 to 45.0, while their a* and b* values were −8.26 to −3.86 and7.34 to 15.03, respectively (Güler and Candemir, 2014). In some grape cultivars, leaves may have rough surfaces (fluffy, wavy) and different thicknesses. Such differences may result in darker colors on higher or swelling sections (Doğan and Cüneyt, 2020). Fresh green grape leaves were dried by microwave, airand combination of microwave-airto study the effects of thermal treatment on color parameter of grape leaves. In all the drying experiments, the colour criteria that was closest to that of fresh leaves occurred at 500 W-75°C, whereas the color criteria that was furthest from that of fresh leaves occurred at 100°C (Alibas, 2014).
5 Extraction techniques for the preparation of grape leaf extract
Extraction can be thought of as an intermediate stage between analytical processes and future product production (Picot-Allain et al., 2021). Every extraction method’s primary goal is to optimise the recovery of the desired components from a sample matrix while maintaining the integrity of the relevant molecules and minimising the co-extraction of additional contaminants or undesired chemicals (Garcia-Vaquero et al., 2018). Many biological actions, including as antioxidant, antimicrobial, antispasmodic, anti-inflammatory, anti-allergic, hepatoprotective, and anti-carcinogenic capabilities, can be attributed to the diverse collection of secondary metabolites that are found in plants known as polyphenols (Jovanović et al., 2017). The right extraction technique needs to be taken into account in the first step. The chemical makeup of the component, particle size of the sample, and the presence of interference-causing compounds all have an impact on the choice of extraction method that should be used (BrglezMojzer et al., 2016). The extraction techniques can be categorized into two–conventional and non–conventional extraction techniques as shown in Figure 2.
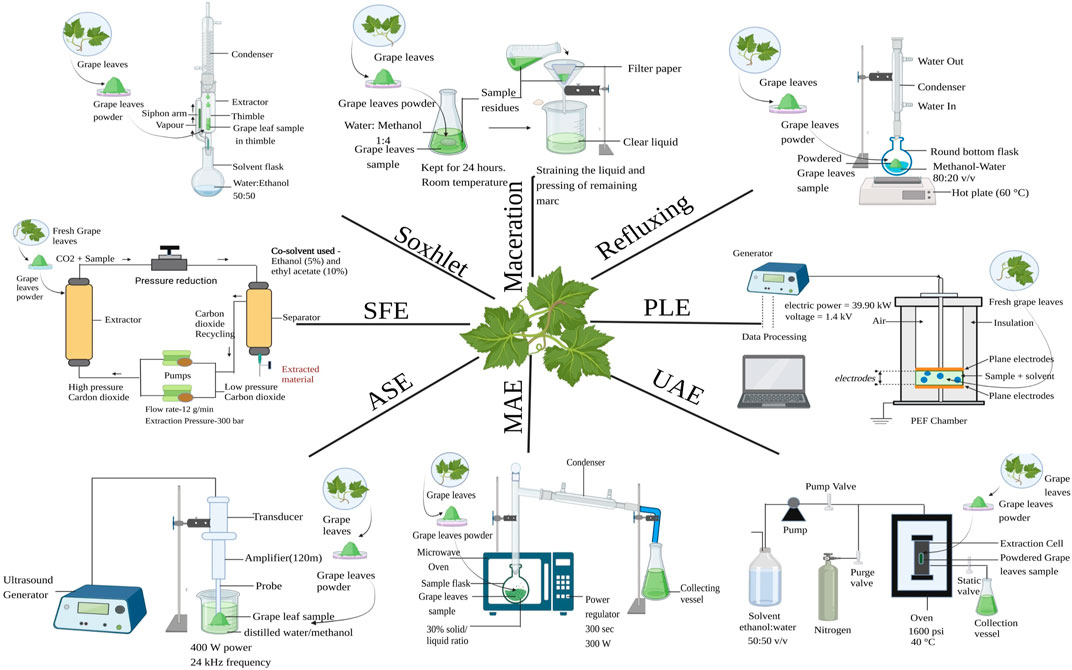
FIGURE 2. Extraction procedure of grape leaves, MAE = Microwave-assisted extraction, UAE = Ultrasound-assisted extraction, ASE = Accelerated solvent extraction, SFE = Supercritical fluid extraction and PLE = Pulsed Electric Field.
5.1 Conventional techniques
In order to retrieve phenolic chemicals from plant matrix, extraction is a crucial technique (Al et al., 2018a). Maceration, percolation, digestion, decoction, serial exhaustive extraction, infusion and Soxhlet extraction are the conventional techniques used for the extraction ofphenolic compounds (Alara et al., 2021). The majority of these methods rely on the extracting ability of various solventsas well as the use of heat or mixing (Azmir et al., 2013). Traditional extraction techniques are notoptimally consistent since they typically involve using larger amount of extraction solvents and labour-intensive manual processes that are mostly dependent on the researcher (Alara et al., 2021). Liquid-liquid and solid-liquid extraction arethe most popular extraction techniques, in spite of a number of drawbacks. Simplicity, effectiveness, and broad applicability of the conventional methods have led to wide spread acceptance for a long time (BrglezMojzer et al., 2016). The required polyphenol molecules are captured by a variety of solvents, including water, ether, benzene, chloroform, hexane, ethanol, acetonitrile and methanol, when pre-treated plant material obtained by washing, drying, etc., are subjected to it (Suwal and Marciniak, 2018). For extraction of polyphenol from grape leaves following conventional techniques can be used.
5.1.1 Soxhlet extraction
Among the earliest procedures known to be employed for the extraction of polyphenol compounds is Soxhlet extraction, also known as solid-liquid extraction (Suwal and Marciniak, 2018). The solvent is transferred into a distillation flask, and the sample is placed in a thimble-wrapped Soxhlet extractor. In order to make the evaporated solvent useable again, condenser devices are utilised in this extraction process (Tambun et al., 2021). The vapours begin to condense as soon as the heated solvent (ultrapure water:ethanol, 50:50) contacts the condenser and thereafter, the solvent was gathered in the collection vessel. This exhausted extraction procedure was carried out continually for about 6 h until the solvent in the siphon tube turned clear without any extraction material dissolving in it. Then filtration of extract was done and rotary evaporator was used to evaporate the liquid (Labanca et al., 2020). In comparison to more advanced extraction methods like supercritical fluid extraction, this process is not viewed as being environmentally friendly and may worsen the pollution problem (Azwanida, 2015).
5.1.2 Classic solvent extraction
Solvent or liquid–liquid extraction is a two-phase solute distribution wherein two phases of immiscible liquid are placed in proximity with other and the solute is dispersed between them (El-Nadi, 2017). This technique is simple and easy to use. 250 mL of ethanol/water in the ratio 80/20 v/v at 60 °C for 60 min was used as solvent for extraction of 5 g of homogenized grape leaves powder. Whatman No. 1 filter paper was used filter the extract to remove any remaining residue after cooling at ambient temperature for 30 min and 3 × 10 mL of solvent was used to wash the leftover residue (Katalinić et al., 2009).
5.1.3 Reflux extraction
Reflux extraction is a method of solid-liquid extraction in which the solvent is evaporated and condensed for one to 3 hours at a fixed temperature of 60°C–80 °C (Kumar et al., 2023). Compared to percolation or maceration, reflux extraction is more effective as it requires less solvent and extraction time (Zhang et al., 2018). 100 mL of methanol-water solvent in ratio 80–20 v/v was used to extract polyphenols from 40 g of crushed leaves with 0.1 mL/mL of concentrated HCl to prevent its oxidation. A 30-min reflux extraction procedure was carried out at 60 °C. The extracts were then filtered followed by centrifugation at 3,000 rpm for time span of 20 min at 25 °C and later kept in dark at 4 °C (Selka et al., 2019).
5.1.4 Maceration
Maceration is another technique of solid liquid extraction. The inert container is filled with the substance to be extracted, and the solvent is then entirely poured over it (Naviglio et al., 2019). Maceration is a traditional extraction technique that is the simplest and cheapest because it only needs a container for the extraction to take place but it is time consuming. Methanol, ethyl acetate, ethanol and distilled water are typical solvents used in maceration (Tambun et al., 2021). 1,000 mL of water-methanol mixture in the ratio 1:4 was used as solvent for extraction of 150 g of powdered leaves at room temperature by maceration in sealed container for period of 24 h with interval shaking (Borah et al., 2018).
5.2 Non-conventional techniques
While there is unquestionably a clear need to develop affordable, safe, efficient, and environmentally innovative extraction methods, it is important that these techniques not only enable clean label status but also ensure increased yields with little impact on the quality of the finished product (Tiwari, 2015). Keeping in consideration the flaws offered by conventional methods, new and advanced green technologies have been developed for better extraction of polyphenolic compounds such as microwave-assisted extraction, ultrasound assisted extraction, pulsed electric field and enzyme-assisted extractions. With the development of new technology, extraction techniques have improved, and are cleaner resulting in products that are less expensive and that do not contain organic solvents (Panja, 2018). Such methods are sometimes referred to as “cold extraction techniques” because temperature used while extracting a molecule is relatively minimal and has little impact on the stability of the isolated compounds (Tiwari, 2015).
5.2.1 Microwave-assisted extraction
The fundamental principle of the MAE technique is the following conversion of microwave energy into thermal energy. Microwaves warm the liquid within the cells of solid samples throughout the MAE. This occurrence raises the pressure inside the cells, which causes the cell wall to degrade. As a result, the sample becomes more porous, allowing the extraction solvent to penetrate the matrix and enhancing extraction efficiency more effectively (Tomaz et al., 2019). The MAE devices can be divided into two categories based on the microwave energy they apply to the sample: Multimode and single-mode system. Multimode systems disperse microwave radiation throughout a space to treat samples uniformly, and single-mode or focused systems apply microwave energy only specific to the sample for a more effective extraction. Focused systems are often employed with open vessels running at atmospheric pressure, whereas multimode systems are applied to closed containers, allowing the simultaneous application of high pressure throughout the extraction process (Gomez et al., 2020). Dipole rotation and ionic conduction—which involves reversing dipoles and dispersing charged ions—are the two ways by which energy is transferred during the microwave heating process. These two systems frequently operate together in various applications. Ions move electrophoretically when an electromagnetic field is applied, and when a solution resists this flow of ions, there is friction that causes the solution to heat up. This process is known as ionic conduction. Dipole rotation entails moving the dipoles around in response to the applied field (Deo et al., 2015).
Several researchers have described MAE as a quick extraction approach that has various benefits over traditional extraction techniques, including high extraction rates, quick extraction periods, and low solvent use (Mustapa et al., 2015). When MAE is used, the extraction time is significantly shortened since microwaves heat the solution directly, whereas with traditional extraction methods, the vessel must first be heated for a certain amount of time before heat is delivered to the solution. Solvent and matrix types, solvent volume, microwave power, exposure time, sample size, moisture content, and temperature are the primary factors affecting MAE performance (Llompar et al., 2019). The rapid rise in temperature of the extraction mixture, which could end the extraction process soon when the solvent boils, is one of the challenges faced by MAE. The extraction yield is decreased when the extraction is stopped too soon because the desired compounds are not properly dispersed into the solvent from the material. In contrast to continuous microwave heating, cooling the extraction mixture with intermittent microwave operation utilising 30 s “on” and 30 s “off” could greatly lengthen the extraction time (Chuyen et al., 2018).
For maximum antioxidant component extraction from grapevine leaves, the ideal microwave conditions were 30% solid/liquid for 300 s at 300 W of microwave power. After being microwaved, samples were centrifuged at 9,000 rpm for time span of 15 min at temperature of 25 °C and filtered using filter paper and supernatants were collected and stored in storage at −18 °C (Hayta and İşçimen, 2021). The primary benefits of MAE over Soxhlet extraction relate to the sharp decrease in solvent consumption (5 V 100 mL) and extraction duration (40S V 6h) (Chaturvedi, 2018).
5.2.2 Ultrasound-assisted extraction (UAE)
Various molecules and biomaterials such as proteins, peptides, fine chemicals, polysaccharides, and bioactive components are extracted from a complex matrix of a plant. Ecological-friendly extraction techniques are required in order to reduce both environmental and health risks which are associated with the use of toxic solvents which have safety risks. Green extraction techniques provide safe, effective-way of extraction of molecules, reduce the use of toxic chemicals/solvents and are affordable which are undoubtedly offers higher extraction yield with improve quality of extract (Tiwari, 2015). The ease of use and affordable equipment make the ultrasound-assisted extraction (UAE) method especially appealing. It is based on the utilisation of ultrasound energy (sound waves that have frequency higher than 20 kHz) to speed up the extraction of compounds from solid samples using a solvent that is chosen based on the characteristics of the solutes that need to be extracted (Carrera et al., 2012).
The water-circulating double-layered mantle enabled cooling and heating systems is used to regulate the temperature during extraction (Both et al., 2014). It is also one among the green technology. Two mechanisms, mechanical mixing effect and ultrasonic cavitation, control the rise in extraction efficiency as well as the decrease in extraction time (Oroian et al., 2020). The primary driving force behind ultrasound-assisted extraction (UAE), which can create bubbles due to temperature and pressure variations, is acoustic cavitation force. It has a ability to cause a series of compressions and rarefaction in the molecules of current solvent (Medina-Torres et al., 2017). Cell walls and solvent diffusion are broken down with application of high-frequency waves, which perturb the solute-solvent combinations. In order to get a higher efficiency, factors like swelling rate, disruption and particle size post-treatment must be taken into account. Breakdown of particle-particle bond occurs as a result of applied high intensity. When the bonds are broken down, this causes solvent to enter the compounds so deeply and called it as cavitation (Sridhar et al., 2021). Erosion occurs as a result of implosion of cavitations bubbles on the surface of the plant tissues which may potentially be responsible for this degradation. Extraction yield is enhanced by the erosion as area that has been eroded, makes it easier for the solvent to contact it. The release of bioactive components present in the cell membrane of plant is caused by the development of pores during the process of cavitation. This phenomenon is known as sonopration (Kumar et al., 2021). Overall, a number of mechanisms, including fragmentation, erosion, sonocapillary effect, sonoporation, local shear stress, and destruction-detexturization of plant structures, have been found as contributing to ultrasound-assisted extraction (Chemat et al., 2017).
In ultrasound assisted extraction, the sample was weighed at 10% (w/w) solid/liquid ratio into 250 mL beaker then 50 mL of distilled water/methanol was added. Titanium probe (H22D, 22 mm) with 400W power, 24 kHz frequency, and 120 m amplitude was employed with the ultrasound equipment. To prevent the sample from overheating during the ultrasound treatment, a 1000 mL beaker was employed. 100% of the amplitude of ultrasound was used for 10 min. Supernatants were collected, freeze-dried, and kept at 4 °C after being centrifuged at 9,000 rpm for 10 min at 20°C (Hayta and Işçimen, 2018). Despite the widespread use of UAE, the technique has two issues which includes uneven ultrasound energy distribution and a power decrease over time (Ajila et al., 2011).
5.2.3 Accelerated solvent extraction
Accelerated solvent extraction is also known as Pressurized liquid extraction (PLE), it is regarded as advanced extraction technique due to its advantage over conventional extraction techniques. In order to maintain solvent in the liquid state, temperature of the solvent is increased and this allows enhancement of mass transfer rate by decreasing solvent surface tension and viscosity, increases diffusivity. Increased pressure maintains the solvent below its boiling point. The solubility of analytes increases, this allows solvent to penetrate easily into food matrix being extracted (Alvarez-Rivera et al., 2020; Osorio-Tobón, 2020). The benefits of using PLE are higher extraction yield, saves energy since a liquid’s sensible heat is lower than its vaporisation heat. Major solvents employed are water and alcohols in this extraction technique. These solvents are non-toxic and good to the environment. These solvents are inexpensive as a sizable port ion is liquid water in this technique. Equipments used in the extraction process such as extractor and the related setup are simple (Panja, 2018).
The extraction solvent is moved through an extraction cell holding the sample in an accelerated solvent extraction process. The sample cell is heated by direct interaction with the oven. Direct contact between the hot solvent and the sample is used for both static and dynamic extraction. When extraction is finished, compressed nitrogen is used to transport all the solvent from the cell to the vial for analysis. Away from the sample matrix, the filtered extract is gathered and prepared for examination. A broad variety of applications, extractions for sample sizes ranging from 1 to 100 g in minutes, a dramatic reduction in solvent consumption, and handling of both acidic and alkaline matrices are all clear advantages of ASE (Mottaleb and Sarker, 2012). Other advantages include simple automation, quicker sample analysis, higher repeatability, low risk of solvent exposure, and preservation of samples in an oxygen- and light-free environment. Furthermore, by optimising the temperature, pressure, extraction duration, and number of extractions, accelerated extraction systems give the operator better control over the amount of chemicals extracted from the plant (Gomes et al., 2017). Additionally, it has a broad range of applications, from tissues from plants, animals, and environmental toxins, having the capacity to handle both basic and acidic sample (Ahmad et al., 2019). The assays were conducted at 1,600 psi for three rounds of 5 min each. Before being recovered with ethanol and water in ratio 50:50 v/v, the dried leaves were packed into the cell and filled while it was heated to 40°C. It was collected and filtered to extract the mixture (Labanca et al., 2020).
5.2.4 Supercritical fluid extraction
A green extraction method known as supercritical fluid extraction (SFE) has been used extensively to recover high-value components from a variety of materials, at both levels, in laboratories and industries (Chaves et al., 2020). The principle behind supercritical extraction is the transformation of the gas used in supercritical fluid caused by changes in temperature and pressure. A supercritical fluid (SCF) is a fluid whose critical temperature (Tc) and critical pressure (Pc) values are exceeded. Due to its low critical temperature and nonexplosive nature, CO2 is the most widely used SCF. It is also safe, affordable, and has significant benefits for pharmaceutical applications (Fomo et al., 2020). Surface tension is absent at supercritical conditions, or above the critical point, where there is no liquid-gas phase barrier. Fluid contains the characteristics of both a gas and a liquid at the same time and behaves like a single phase. At this point, fluid diffuses like a gas into the solid matrix and dissolves the active components. This is the foundational idea behind supercritical fluid extraction (Panja, 2018). A basic SFE system is made up of the following components: a mobile phase tank for the solvent (typically CO2), a pump for moving and pressurizing the solvent, a cosolvent vessel, a heater for the solvent or supercritical mixture, a pressure vessel for the extraction, a controller to keep the system’s pressure at a high level, and a collection vessel to collect the extract (Chaves et al., 2020).
For extraction of grape leaves Supercritical fluid extractions (SFE) were carried out in a cylindrical 0.5 L lab scale extractor from Applied Separations Inc. (United States) with internal dimensions of 7.3 cm and a height of 12 cm. The supercritical CO2 (SC-CO2) flow rate and extraction pressure were both kept constant at 12 g/min and 300 bar, respectively. Ethanol (E) and ethyl acetate (EA), at concentrations of 5 and 10 weight % relative to the constant SC-CO2 flow rate, were the cosolvents added to the ground particles. The overall yield of the SFE tests utilising crushed (less than 10 mm) and ground (less than 1 mm) biomass ranged from 1.86 to 7.52 wt%, with the best outcomes coming from the extraction of ground particles using SC-CO2 with 10 wt% ethyl acetate at temperature of 80 C (de Melo et al., 2020). Since SFE uses supercritical solvents, which have unique physicochemical properties including density, diffusivity, viscosity, and dielectric constant, it has a number of operational advantages over traditional extraction techniques. Supercritical fluids having a low viscosity and a reasonably high diffusivity, has increased transport capabilities than liquids, the ability to diffuse efficiently through solid surfaces, and the ability to provide faster extraction rates. Other benefits includes the use of solvents that are generally recognised as safe (GRAS), the extraction process’s higher efficiency in terms of increasing yields and cutting down on extraction times, and the potential for direct coupling with analytical chromatographic methods like gas chromatography (GC) or supercritical fluid chromatography (SFC) (Da Silva et al., 2016). Since CO2 exhibits polarity similar to pentane in the supercritical area, it can be used to extract lipophilic compounds. Because CO2 lacks the polarity necessary to remove polar compounds, this is its primary drawback (Yousefi et al., 2019).
5.2.5 Pulsed electric field extraction
Another non-thermal food processing technology with a lot of potential is pulsed electric field (PEF). In the case of PEF treatment, application of the critical electrical potential to cell membranes results in rapid mass transfer, tissue breakdown, and augmentation of tissue permeability (Roselló-Soto et al., 2015).
The electroporation phenomenon of cell membranes, which occurs when a potential difference develops across a membrane, is the foundation of the PEF-assisted approach. The process of molecular orientation known as electroporation occurs when an electric field is present, molecules gravitate towards the membrane by aligning themselves with it. The membrane begins to tear and develop pores as a result of electrocompression. A temporary (reversible) or permanent (irreversible) reduction of membrane permeability can occur from this. For plant cells that range in size from 40 to 200 μm, a critical electric field strength of 1–2 kV/cm determines how much permeability is lost and how much pore development occurs. A high voltage generator, an impulse generator, a Schmitt trigger circuit, a high voltage switch, an oscilloscope, and two plate electrodes (1.4301) make up the setup for pulsed electric field assisted extraction (PEF). A 20 mL mixed glass beaker included plate electrodes with a separation distance d of 0.42 cm and a surface A of 6 cm2. The PEF aided extraction procedure was carried out using fresh red vine leaves at a voltage of U = 1.4 kV, a conductivity of σi = 1.425 mS/cm, and an electric power of Pel = 39.90 kW (Bachtler and Bart, 2018). The PEF continuous extraction can produce larger yields in less time while using less organic solvent and reducing pollutants. Additionally, the entire procedure can be completed at or just a little above ambient temperature. Thus, by avoiding the thermal stimulator, the bioactive compounds won't be damaged. But when the electric field strength is strong enough, there will be substantial concentrations of hydroxyl radicals, which will cause bioactive components to degrade during the PEF extraction (Xi et al., 2021). PEF’s use in the food sector is growing in popularity; it is now regarded as a cutting-edge method for food processing that is suitable for the pre-treatment of edible liquid and semi-solid products. To prevent the dielectric breakdown, it is only applicable to materials with low electrical conductivity and no air bubbles (G et al., 2019).
The microwave-assisted extraction approach has shown the greatest results when compared to other polyphenol extraction techniques. The benefit of microwave extraction is that it may be performed with high efficiency while using the least amount of solvent and least amount of time. Additionally, MAE is superior to conventional extraction techniques in terms of the ease of maintaining extraction containers, improvement of analytical capacities (such as improvement of recovery and reproducibility), and the capacity to simultaneously extract numerous samples (Rajbhar et al., 2015). Table 2 covers different extraction methods for extracting various bioactive components present in grape leaf with bioactive properties. Detail of it is mentioned below:
Gallic acid, 3,4-dihydroxybenzoic acid (+)-catechin, 1,2-dihydroxybenzene, rutin-trihydrate, quercetin, apigenin-7-glucoside, and caffeic acid are the most commonly found bioactive components obtained from grape leaves during the maceration process. It was discovered that the extraction yield of grape leaves was 19.25w/w%. Due to the presence of bioactive components like catechins and their metabolites, which are decomposed into (+)-catechin or (+)-epicatechin and gallic acid which have great bioactivity in reducing inflammation in the body, a variety of bioactive properties have been discovered. These properties may be essential in suppressing neurodegenerative illnesses. In addition to this, by blocking hepatitis C virus (HCV) replication and the production of inflammatory proteins COX-2 and Nuclear factor kappa B (NF-κB) (+)-catechin prevented cirrhosis brought on by a chronic HCV infection, thus helpful in prevention of liver damage (Borai et al., 2017; Borah et al., 2018; Banjanin et al., 2021; Kim and Heo, 2022). The highest extraction yield was observed in Soxhlet (SOX) extraction of about 30.45%. SOX extract of grape leaf tissue allowed the identification of the bioactive components such as flavonoid, gallic and caftaric acid, quercetin-3-O-glucoside and quercetin, quercetin-3-O-galactoside, quercetin-3-O-glucuronide and quercetin-3-O-glycoside (Brad et al., 2017; Labanca et al., 2020). One of bioactive components such as gallic acid shows anti-inflammatory property. In order to inhibit the release of inflammatory factors (TNF-α, IL-1β/6), chemokines (CCL-2, ICAM-1, TIMP-1) and other inflammatory mediators (COX-2, nitric oxide), gallic acid attenuates the activation of MAPK and NF-κB/AP-1 signaling pathways (Bai et al., 2021). Detail of Nuclear factor kappa B (NF-κB) and inflammation process is described below (see Section 6.2).
Microwave-assisted extraction (MAE) was used to identify and quantify the bioactive components found in grape leaves, including gallic and caftaric acids, phytosterols, quercetin-3-glucuronide, quercetin-3-glucoside, and kaempferol-3-rutinoside (Djemaa-Landri et al., 2020). Microwave-assisted extraction (MAE) of grape leaves was performed with a power of 300 W (Hayta and Işçimen, 2021). It was discovered that MAE increases yield, shortens extraction times, and uses less solvent (Reis et al., 2015; Hayta and Işçimen, 2021). Numerous studies have revealed that quercetin is associated with melanogenesis and offers pharmacological effects such as anti-inflammatory, anti-atherosclerotic, antioxidant, anticancer, and skin protecting qualities. Quercetin 3-O-β-D-glucuronide (Q-3-G) has been shown to exhibit anti-inflammatory, anticancer, and antioxidant properties in lipopolysaccharide-stimulated macrophages. This has been demonstrated by an increase in Nrf2, one of the key regulators of oxidative stress in cells (Ha et al., 2021). Ultrasound was applied 100% amplitude in extraction of grape leaves through Ultrasound-Assisted Extraction (UAE) (Hayta and Işçimen, 2018). The extraction yield was found to be 13.81%. Resveratrol, Caftaric acid (+)-Catechin, Benzoic acid, Rutin, Quercetin-3-O-galactoside, Quercetin -3-O-glucuronide, Quercetin-3-O-glycoside, Kaempferol-3-O-glucoside were identified in grape leaves by the use of UAE. However, gallic and caftaric acid, quercetin-3-O-glucoside and quercetin were not present in UAE (Labanca et al., 2020). Numerous biological activities of resveratrol include anti-aging, glucose metabolism, anti-tumor, anticancer, antitumor, antidiabetic, antioxidant, and anti-obesity activities (Paczkowska-Walendowska et al., 2023). These bioactive components possess various bioactivity, one of it has been demonstrated that quercetin-3-O-galactoside, also known as hyperoside, may have therapeutic and preventive benefits for liver disorders. By inhibiting CYP2E1, HPS regulated the glutathione-related metabolites and enzymes, preventing oxidative stress-induced liver damage brought on by Acetaminophen (APAP) (Hu et al., 2020).
The extraction yield was found to be 6.44% with the use of Accelerated solvent extraction (ASE) in grape leaves. Caftaric acid (+)-Catechin, Benzoic acid, Rutin and its derivatives, Quercetin-3-O-galactoside, Quercetin -3-O-glucuronide, Quercetin-3-O-glycoside, Kaempferol-3-O-glucoside were found in grape leaves (Labanca et al., 2020). By inhibiting NF-kB activity and prostaglandin synthesis, catechins lower inflammation. By stabilizing the levels of glutathione, nitric oxide, and glutathione peroxidase, quercetin protected the liver from oxidative damage (Arika et al., 2019). The activity of acetylcholinesterase (AChE) is likewise decreased by troxerutin which is derivative of rutin. Acetylcholine (ACh) is a neurotransmitter that is more abundant in the synaptic cleft and facilitates better neurotransmission when AChE activity is reduced (Babri et al., 2014). Although Supercritical fluid extraction is mostly useful for lipid extraction, but it can also be used to extract some polyphenols, including catechins and caffeic acid (Lefebvre et al., 2021). The bioactive activities of these compounds were found to be antioxidant potential, anti-inflammatory (Arika et al., 2019; Chao et al., 2019). Using undried red vine leaves, the Pulsed electric field (PEF) assisted extraction process was executed with an electric power of 39.90 kW and extracted flavonoids a subgroup of ployphenols (Bachtler and Bart, 2018). Numerous studies have demonstrated the potential of pulsed electric field (PEF) with higher energy/intensity or pulses for longer processing times as a non-thermal treatment for increasing yield (Shiekh et al., 2021). The anticholinesterase, pro-cholinergic, anti-inflammatory, antiapoptotic, and antioxidative properties of Vitis leaf polyphenols may contribute to their memory-improving properties and may have a function in the clinical treatment of neurological illnesses (Borai et al., 2017).
6 Bioactive properties of grape leaves
A wide range of benefits from grape leaves and its bioactive components, such as antioxidant, antidiabetic, antibacterial, anti-inflammatory, antiviral, anti-obesity, and hepatoprotective, and gastroprotective activities (Figure 3) could be used in healthcare as products. Bioactive properties of Vitis vinifera leaf extract is due to bioactive components present in it which include six hydroxybenzoic acids, namely, 2,5-dihydroxybenzoic acid, protocatechuic acid, p-hydroxybenzoic acid, vanillic acid, gallic acid and syringic acid and six hydroxycinnamic acids, namely, p-coumaric acid, o-coumaric acid, cinnamic acid, ferulic acid, caffeic acid, and sinapic acid (Meng et al., 2021). Table 3 covers experimental studies to represent the bioactive properties of grape leaf.
6.1 Antibacterial property
Various medicinal plants contain an unsaturated phytosterol known as stigmasterol or stigmasterin (Mohan et al., 2022). Stigmasterol has been considered as bactericidal property in grape leaf extract. Both gram-positive and gram-negative bacteria are strongly inhibited by Stigmasterol, which means that Stigmasterol is potential as an antimicrobial agent (Khumaidah et al., 2019). Bacterial components are degraded by stigmasterol by means of two ways-surface interaction of stigmasterol with bacteria and forming pores in the bacterial cell wall. Other reasons of showing antimicrobial effect of grape leaves are due to inactivation of microbial adhesions by tannins present in grape leaves, capacity of it to improve the morphology of microorganisms (Sharma et al., 2021).
6.2 Anti-inflammatory property
Anti-inflammatory effect of grape leaves is due to components present in it, which include kaempferol, resveratrol, quinic acid and quercetin (Ins et al., 2021). During process of inflammation, reactive oxygen species (ROS) and nitrogen species are involved which causes cellular damage, moreover release of free radicals causes oxidative stress. There are certain transcription factors such as Tumor necrosis factor (TNF-α) and interleukins (ILs) which are released and resulting in inflammation, these mediators are further directly and indirectly induced by ROS (Reactive oxygen species) and RNS (reactive Nitrogen species). More free radicals are produced and this continued during the whole inflammatory process (Direito et al., 2021). Anti-inflammatory activity of Vitis vinifera has been showed by acting on NF- κB pathway and decrease of IL-8 (Interleukin- 8) in Tumor necrosis factor alpha (TNF- α), which is cytokine. Fibroblast-like synoviocytes (FLS) cells and macrophages produce Interleukin (IL) such as IL-6, causes release of chemokines by stimulating endothelial cells and also activates lymphocytes like B and T cells. Vascular endothelial growth factor (VEGF) production, which is responsible for pannus formation, is actively facilitated by IL-6. Macrophages produce IL-1β in the inflamed synovium (Ali et al., 2023). Various pathways are activated by these inflammatory cytokines to induce inflammation such as NF-κB(Nuclear factor kappa B). During inflammatory process, IL- 8 is a chemokine released by keratinocytes (Sangiovanni et al., 2019).
Nuclear factor kappa B (NF- κB) regulate important physiological processes such as inflammation, immunity, cell growth and death of cell and targets the defense mechanism of the body by regulating expressions of certain genes in the process including cytokines and pro-inflammatory cytokines (Zinatizadeh et al., 2021), represents plays an important role in a variety of conditions of skin-inflammation including psoriasis which refers to an inflammatory dermatosis, indicated by high levels of NF- κB (Goldminz et al., 2013). Vitis vinifera has capability to inhibit the NF- κB (Nuclear factor kappa B) pathway through the impairment of translocation from the cytoplasm into the nucleus in human gastric epithelial cells (Vinceković et al., 2019). The highest dose of Leaf extract causes reduction in levels of interleukins such as IL-6 by 60% and IL-8 by 40%, whereas IL-1β concentration was reduced to the basal level by the extract (Moldovan et al., 2020).
6.3 Antioxidant activity
Antioxidant plays an important role in delaying, inhibiting or preventing oxidation of oxidizable materials by scavenging free radicals and thus oxidative stress is diminished. Powerful antioxidants have been considered in vitro to be more vigorous than Vitamin C and E, carotenoids, some of derivatives of hydroxycinnamic acid such as caffeic and chlorogenic acid and flavonoids such as quercetin and its derivatives, which possesses antioxidant activity in vine leaves. Vine leaves extract inhibited peroxidation of linoleic acid due to strong activity possess by compounds present in leaves extract (Bajomo et al., 2022). Caffeic acid is found in many of plant-based foods sources which involves in biosynthetic pathway as intermediate, moreover phenolic acids and precursor molecule of lignin are also produced by caffeic acid. Due to resonance stabilization of structure of caffeic acid, it have a high antioxidant potential than other compounds such as hydroxycinnamates such as ferulic acid (Chao et al., 2019). One of bioactive component of vine extract such as Quercetin is responsible for the increase of the levels of enzymatic and non-enzymatic antioxidants, thus reducing oxidative stress and improving the antioxidant defense mechanism (Babri et al., 2014). Quercetin has ability to scavenge some of radicals, namely, superoxide radicals, hydroxyl radicals, and lipid peroxyl radicals through its antioxidant activities. Quercetin supplements protects the liver from damage caused by oxidative stress by normalizing the levels of nitric oxide, glutathione and glutathione peroxidase as shown by previous studies in mice (Babri et al., 2014).
6.4 Neuroprotective activity
In elders, Alzheimer disease is the most common cause of decline in memory and neurocognitive function, which involves formation of amyloid-b (Ab) plaques, neurofibrillary tangles (NFTs), cholinergic dysfunction, and oxidative pathogenic factors (Babri et al., 2014).
In humans and animals, a decline in motor and cognitive could be more susceptible to the effects caused by oxidative stress and inflammation (Zhang et al., 2013). Vitis vinifera leaves contains a wide range of polyphenols such as anthocyanins, flavonoids and also organic acids, namely, malic acid, oxalic acid and tartaric acid is found. Polyphenols present in vine leaves interacts with various pathways including neuronal and glial signalling. In addition to, vine leaves also decrease ROS production, neuronal damage caused by neurotoxins and neuroinflammation, resulting in improvement of neurological health (Borai et al., 2017).
6.5 Anti-obesity property
The antiobesity effect of many phenolic acids has been reported. Vanillic acid reduces obesity by the activation of the AMPKpathway (AMP-activated protein kinase) (Mohan et al., 2022), AMPK (AMP-activated protein kinase) acts as energy homeostasis, as an enzyme it is involved in the energy metabolism of in liver, muscle and fat tissue, inhibition of AMPK is one of the most important cause of accumulation of liver fat. The enzyme ACC, i.e., Acetyl-CoA carboxylase was phosphorylated by the activation of AMPK. Malonyl-CoA synthesis was enabled by the ACC enzyme, which potentially inhibits fatty acid oxidation in mitochondria (Jung et al., 2018).
Mature, lipid-containing adipocytes are converted from preadipocytes in a process of proliferation is called adipogenesis (Jakab et al., 2021). Adipocyte differentiation was accelerated by reactive oxygen species (ROS) production, whereas differentiation was diminished in the presence of antioxidants. The hydroxybenzoic structure of syringic acid, containing methoxyl groups in positions 3 and 5 and a hydroxyl group in 4, was made a potent antioxidant (John and Arockiasamy, 2020). The ability of syringic acid to inhibit adipogenesis and promote lipolysis in adipocytes, possibly through a reactive oxygen species (ROS)-mediated mechanism, had demonstrated its anti-obesity effect (Meng et al., 2021).
6.6 Anti-viral effect
V.vinifera leaf extract showed a strong antiviral activity against two important human pathogens, i.e., HSV-1 and severe acute respiratory syndrome coronavirus 2 (SARS-CoV-2) (Zannella et al., 2021). The host angiotensin-converting enzyme 2 (ACE2) was utilized by SARS-CoV-2, like SARS-CoV-1, for binding and entry into host cells (Zhao et al., 2020).
V.vinifera leaf extract inhibit the attachment of both of the viruses, namely, HSV-1 and SARS- CoV-2 into the host cell. V. vinifera hinders some of interacting site inside the viral glycoprotein deputed to the fusion, i.e., HSV-1 glycoprotein b and SARS-CoV-2 spike protein, process of formation of infection, binding of viral envelope with cell membrane are prevented. Much of the viral gene expression was blocked by the extract and the replication of HSV-1 was inhibited, almost having at a concentration of 10 μg/mL. The literature demonstrating the leaf extract had antiviral action and results were also supported. Leaf extract could be used as a prophylactic treatment for herpetic infections (Zannella et al., 2021).
6.7 Anti-diabetic activity
The anti-diabetic activity of V. vinifera leaves was suggested to be contributed to by condensed tannins and flavonoids (Negam et al., 2020). The degree of inhibition of alpha amylase enzyme was investigated by estimating antidiabetic activity of leaf extracts of Vitis vinifera (Dar et al., 2022).
The inhibition mechanism of alpha amylase is still unclear. However, starch and nitrous acid which are derived from the oral cavity can be interacted with flavonoids present in food in the stomach, this interaction is occurred before transportation to the intestine. Two mechanisms of action are mainly seen, (a) flavonoids showing mechanism of action by inhibiting activity of α-amylase activity, and (b) flavonoids interacts with starch through hydrophobic interactions and resulting in the formation of starch-flavonoids complex which leads to suppression of starch digestion. The inhibition of tannins is correlated to the number of hydroxyl groups in the B cycles of alpha-amylase. Hydrogen bonds are formed between the OH group (hydroxyl group) of these compounds and the residues of the active site of the alpha-amylase, causing the alpha-amylase to be inhibited. Diabetes risk can be reduced by flavonoids-rich food sources through the modulation of glucose uptake and insulin secretion (Mohammed et al., 2020).
It had been shown by a report that various potential diabetes inhibitors from plants which can inhibit α-amylase and α-glucosidase belong to flavonoid group (Al et al., 2018b).
6.8 Gastroprotective effect
The phenolic compounds of hydroxymethanolic extracts of two varieties of grape leaves, i.e., "Nefza-I″ and “Marsaoui” can protect the damage of gastric mucosa which is caused by the ethanol alcohol by scavenging free radicals as per shown in the report. The anti-ulcerogenic effect is attributed to decrease blood flow and enhanced oxidative stress, changes in the mucosal barrier caused by severe choroid plexus lesions, and the well-known ruptured blood vessels that result from ethanol consumption. Gastric acid secretion was inhibited, stimulation of mucus and bicarbonates were seen and gastroprotective mechanism of action represent by increases blood flow on gastric mucosal. Pre-treatment of rats with grape leaves extract at the levels of 100 mg/kg and 200 mg/kg were given, it has been found that this showed higher effects than the pre-treatment of rats with omeprazole. In this sense, the lesion formation was prevented and hydroxymethanolic extract of grape leaves extract promoted better health of gastric mucosa as a gastroprotective effect even more than the drugs used for gastric ulcers (Saadaoui et al., 2020).
6.9 Hepatoprotective effect
Ascorbate and glutathione or Glutathione peroxidase scavenge reactive oxygen species directly or indirectly by the principal antioxidant, particularly reduced glutathione (GSH), known as glutathione (Sahoo et al., 2017). During metabolism and immune response of the body, levels of ROS increase, therefore in order to maintain optimum levels of glutathione, upstream regulators, such as nuclear factor E2-related factor 2 (Nrf 2) are essential to increase or decrease synthesis and recycling of the molecules (Teskey et al., 2018).
The activity of Glutathione reductase (GR) is increased in rats treated with ethanol alcohol, moreover, recycling of reduced glutathione is increased which further adds up to the increase of activity, while radical scavenging activity is decreased by the decrease in Glutathione peroxidase (GPx). Grape leaf extract could normalise the oxidative stress that occurs in the liver by above mentioned markers. Activation of nuclear erythroid-related factor 2 (Nrf2) by secondary metabolites present in the extract (e.g., apigenin derivative, epicatechin, quercetin derivatives, caffeic acid, rosmarinic acid derivative, and isorhamnetin derivatives) may be involved. partly attributed to the antioxidant effects observed for hepatoprotective effect (Amen et al., 2020).
7 Food and non-food applications of grape leaves
Grape leaf has potential application in developing various value-added products. In food applications, grape leaf extract is formulated in bakery products; beverages and it provide health benefits. In non-food applications, grape leaf has been used and act as preservative, it has been used in cosmetics and played a role in anti-hyperpigmatation. Few researchers have shown grape leaves utilization as traditional and processed foods, other researchers have shown the use of grape leaves in non-food applications (Table 4) (Dogan et al., 2015; Hefnawy et al., 2016; Cosme et al., 2017; Lin et al., 2017; Jridi et al., 2019; Capone et al., 2021; Kaur et al., 2023).
7.1 Food applications of grape leaves
After the grapes are processed, grape leaves are collected as a significant waste in the agriculture industry (Zannella et al., 2021). In traditional medicine, grapevine leaves are already used to treat bleeding, inflammation, diarrhoea, and diabetes-related hepatic problems because they are relatively common plant material and contain a variety of phenolic chemicals and antioxidants (Maia et al., 2019).
7.1.1 Bakery products
Walid and Rania (2023) state that fats are necessary for dough production since they give the desired flavour. Not only can nutritious values vary as a result of lipid oxidation that occurs in biscuits, but it also produces organoleptic alterations. Consumers find biscuits to be undesirable due to a bad taste, a lack of desired texture, and an unpleasant odour. According to Walid and Rania (2023) and Mildner-Szkudlarz et al. (2022) lipid oxidation in biscuits is caused by polyunsaturated fatty acids included in the shortening used to prepare the dough. Lipids that are preserved in their natural state give optimal texture and dimensional properties while extending the shelf life of biscuits (Gebreselassie and Clifford, 2016).
A study conducted by Hefnawy and El-Shourbagy (Ferhi et al., 2019) carried out an experiment in order to determine the antioxidant activity of some plant extracts, such as Grape leaf extract (GLE), and to determine the stability of lipids included in cookies. Biscuits were prepared enriched with grape leaf extract at 1% (w/w) level. To make biscuits, sugar and fat were first whipped, then extracts, dough water containing sodium and ammonium bicarbonate, sodium chloride, and wheat flour were added. The dough was sheeted, baked, cooled and stored. The biscuits with GLE were the least moist. The ash content in biscuits is another crucial factor. According to the current investigation, the ash concentration of biscuits containing GLE was comparable to that of the control. Moisture and ash content of the biscuits was 3.99% ± 0.09% and 1.28 ± 0.01 respectively while that of the control sample was 4.18 ± 0.08 and 1.26 ± 0.01. When GLE was added, the amount of force needed to break the biscuits decreased. All sample’s peroxide values (PV) increased after 15 days of storage, but the GLE-enriched sample’s PV spiked suddenly from 0.034 to 0.374, indicating that it was ineffective at preventing the oxidation of lipids. Regarding color, crumb colour, texture, and mouth feel, the biscuits made using GLE received favourable reviews.
Greek cuisine has long utilised grape leaves in form of the traditional dish “Sarma”. Sarma, which translates to “wrapped” in Turkish, refers to leaves that are either raw or more frequently quickly blanched, or held in salt brine, and then rolled around a stuffing of rice, bulgur, or minced meat, along with other potential vegetables and seasoning plants particularly onion, before being gently cooked by stewing or boiling in a pot and typically eaten warm with meat or cold without the addition of meat (Marabini et al., 2020). It is one of the most well-liked savouries from the south-eastern European and Middle Eastern cuisines. It is prepared as a side dish or as a main component for a meat supper. The leaves do, in fact, carry a hint of the flavour and aroma of grape (Cosme et al., 2017).
Jridi et al. (2019) utilised Vine leaves powder (VLP) in pistachio calissons. A local pastry shop made pistachio calissons using the usual ingredients: pistachio paste and meringue. The three formulations that were developed included the control formulation referred as F0, F1 enhanced with 3% VLP, and F2 which was enhanced with 5% VLP. The findings of the texture analysis of calissons indicated hardness dramatically increased for F2 from 7.3 ± 1.2 N to 9.5 ± 0.2 N. The final product’s elasticity and cohesiveness were not significantly affected by VLP enrichment. The antiradical activity of the calissons increased because of VLP enrichment. Several samples’ peroxide values grew over the course of storage. The pistachio calisson’s oxidation, however, was significantly reduced by the addition of VLP from 2.6 ± 0.1meq/kg to 1.5 ± 0.1meq/kg,. One factor influencing its appeal was the color of the pistachio calisson. The 3% enriched pistachio calisson retained its characteristically greenish color due to the chlorophyll-rich grape leaves powder. For formulations F1 and F2, the odour and taste ratings were also favourable. It was permitted to add 3% PFV to pistachio calisson.
7.1.2 Beverage
In a study by Capone et al. (2021), grapevine leaves were used in the production of Shiraz wine, and the effects of the leaves on the flavor and volatile composition of the wine were examined. Grapevine leaves were used as MOG (material other than grapes) in this investigation. Shiraz fruit was first used to make wine before 500g of grapevine leaves (or around 1% w/v) were added to each batch along with other components such grape rachis or peduncles. Wine yeast was added to each juice and must in a subsequent step, and fermentation was carried out at a temperature of 15°C–19 °C. Wines were filtered after fermentation was finished and kept at 15 °C for analysis. C6 alcohols at higher amounts, including hexanol and (Z)-3-hexen-1-ol, were studied in wine that had grapevine leaves added. According to a different study, grape leaves had higher levels of C6 alcohols than grapes because they had more enzymatic activity. Carotenoid concentration in wine made with the inclusion of grapevine leaves was enhanced eight times higher in comparison to grape pulp, and C13 norisoprenoids, such as 1,1,6-trimethyl-1,2-dihydronapthalene (TDN), which is responsible for flavour, were more abundant in grape leaves. A number of other substances, including 2-methylpropanol, β-damascenone, 2- and 3-methylbutanol, were present in considerable concentrations. According to the sensory study performed by a panel of nine assessors trained by AWRI, wine created with grapevine leaves had positive fragrance characteristics like “vanilla,""floral,""confection,""sweet,” and “overall fruit".
7.2 Non-food/applications of grape leaves
Grape leaf extract has been formulated in various non-food products (discussed below) in order to represent its benefits. In non-food applications, grape leaf has been used in order to preserve the food by increasing shelf life of product, played an essential role in cosmetics.
7.2.1 Cosmetics
In the cosmetics market of Asia, a wide variety of substances are uses for the production of products related to skin care in order to treat hyperpigmentation which occur as a result of enzyme named tyrosinase which causes skin pigmentation which catalyse the reaction in which L-tyrosine is oxidized to DOPA, then to dopachrome (Lin et al., 2017). At the end of the whole reaction process, melanin is produced, responsible for pigmentation of skin. According to Lin et al. (2017), in order to inhibit the activity of tyrosine, red vine leaf extract (RVLE) was used as an inhibitor against tyrosine activity. 3.84 mg/mVL was determined as the IC50 for the RVLE solution. Tyrosine’s activity was proven to be lowered by RVLE, which has been demonstrated to be safe for usage in cosmetics.
In a study done by Lee et al. (2022) on mice, Vitis vinifera leaf and shoot extracts were given to the animals as supplements. V. vinifera Jingzaojing dried leaves and stems (100 g) were treated with 1 L of 3% acetic acid-30% ethanol for 3 h at 90 C. The extracted solution was centrifuged for 10 minutes at 13,000 rpm, and the supernatant was filtered, lyophilized, and powdered. Comparing the mice in the JLSE-supplemented group to the mice in the control group, it was clear that the JLSE-supplemented group had considerably longer maximal running times, greater running distances, and longer run times until exhaustion. Another study found that red grape leaf extract boosts the expression of certain enzymes involved in fatty acid oxidation and mitochondrial biogenesis.
Grapes are one of the top 20 raw fruits in terms of consumption, according to the Code for Federal Regulations (21CFR101, subpart C), and they are governed as foods by the Food and Drug Administration (FDA). The following are evaluated as being safe in this report: 24 Ingredients produced from Vitis vinifera (grape) for use in cosmetic formulations includes: Vitis vinifera (grape) leaf extract, Vitis vinifera (grape) leaf oil, Vitis vinifera (grape) leaf/skin/seed extract, Vitis vinifera (grape) leaf water, Vitis vinifera (grape) leaf wax (Fiume et al., 2014). Vitis vinifera derived ingredients are used in the formulations of cosmetics products (Table 5) (Devi and Singh, 2017).
7.2.2 Red grape leaf extract (RGLE) to enhance endurance capacity
According to study by Minegishi et al. (2011), the Mediterranean region has traditionally used red grape leaf extract (RGLE) as a natural medicine. According to a study, mice’s endurance was noticeably improved by red grape leaf extract (RGLE). In the Mediterranean region, venous insufficiency is treated with red grape leaves Multiple polyphenolic substances, including stilbenes, anthocyanins, flavanols, flavonols, flavons, and ellagitannins, are prevalent in red grape leaf extract (RGLE). The study was conducted on endurance capacity in mice. Three experimental groups of male BALB/c mice were created; the control group, the 0.2% (w/w), and the 0.5% RGLE group. All three groups had comparable swimming times and body weights. During the 10-week trial period, swimming times were assessed once a week to assess endurance capacity. Blood analysis of mice was taken. At week 10, the 0.5% RGLE group’s endurance capacity was much longer than the control groups, indicating that red grape leaf extract (RGLE) improved endurance capacity in a dose-related way while simultaneously stimulating lipid catabolism in the muscle. In the muscle, RGLE increased the expression of genes involved in fatty acid oxidation and boosted the activity of fatty acid oxidising enzymes by 27% with the 0.5% RGLE group. After exercise, plasma lactate levels in the 0.5% RGLE group were considerably lower than those in the control group. When compared to control mice, the endurance capacities of mice fed 0.2% and 0.5% RGLE were higher by 11.4% and 34.3%, respectively.
7.2.3 Tablets
Azhdari et al. (2020) state that CVI is a condition caused by inadequate venous return from the lower limbs and is linked to an increase in venous hypertension. The flavonoids in red vine leaf extract shown impacts on venular endothelium and healing of endothelial cell lesions, as well as effects on inflammatory indicators and reaction process of inflammation in the pathogenesis of CVI. This is the mechanism underlying the effect of red vine leaf extract in CVI. Nearly all studies where red vine leaf extract reduced oxidative stress and edema showed improvements in symptoms associated to CVI. In an experiment, Belcaro (2015) compared the effects of two distinct plant extracts on chronic venous insufficiency (CVI). Red grape leaf extract (GLE) is the active component in Antistax (tablet 360 mg), a registered trademark of Boheringer-Ingelheim, Germany. CVI with moderate or severe clinical symptoms was quantified with antistax. 200 subjects were pre-selected, of which 183 were included (with 17 being dropped due to certain personal issues). Out of 166 patients, 55 subjects took the GLE/Antistax medication and finished the 8-week study. Results indicated that issues with leg fatigue and leg aches that are related to a working lifestyle have decreased. Even after prolonged standing or sitting, the walls of the individuals’ leg veins were maintained, ensuring a healthy circulation. As a result, it aids in keeping a regular venous flow and reducing edema symptoms. Two capsules of Antistax were advised to be taken daily for at least 6 weeks.
Another study conducted by Belcaro et al. (2017) compared products used to control symptoms of CVI. Antistax (360 mg of red grape leaf extract) was used as an active ingredient with dosage of 700–1000 mg per day. Results for edema were seen well with a decrease of 86 mL in volume. It was regarded safe-self treatments in several countries. Oxidative stress was also reduced with Antistax of about 28%. It was found that Reactive oxygen species (ROS) levels in the blood was significantly decreased and findings suggest that patient might benefit from Antistax regarding complications associated with T2DM such as wound healing.
7.2.4 Preservatives
Grape leaf and stem extracts were micro-capsulated and used as food preservatives. Epicatechin, quercetin, and rutin were all found in higher concentrations in the leaf extract than in the other. Previous research has shown that phenolic acids have a variety of biological effects, including the ability to kill bacteria. Phenolic acids present in grape leaf showed antifungal properties, including apigenin, luteolin, catechin, chlorogenic acid, and epicatechin. Due to their high phenolic component content, grape byproducts (leaves and stem) have strong antioxidant properties. Grape by-product (including leaves) extracts microencapsulated could increase food viability and the bioactive ingredients could shield it from degradation, used in fruit-based food models such juice and ready-to-eat fruit demonstrated the capacity to lower ochratoxins levels and increase shelf life. Grape byproduct extracts could be used as an innovative method of food bio-preservation (Badr et al., 2021).
Altunkaya (2014) conducted a study to determine how grape leaf extract (GLE) affected the inhibition of lettuce polyphenol oxidase (PPO) and the loss of phenolic components in freshly cut lettuce during storage. The addition of GLE that had been heated at 100C for 15 min had a stronger inhibiting effect on the lettuce PPO activity than the freshly extracted GLE did. Heat-treated GLE was discovered to noncompetitively inhibit lettuce PPO. In lettuce that was being kept track of for storage, the addition of GLE stopped lightness loss. According to the findings of the current investigation, grape leaf extract (GLE) significantly inhibited PPO and stopped the enzymatic browning of freshly cut lettuce. As a result of its widespread occurrence, low cost, and non-toxicity, GLE has the potential to be used as a natural inhibitor of PPO. However, additional feasibility studies are required to maximise effectiveness of grape leaf extract as an anti-browning agent in its use in the food industry.
8 Toxicity and recommendations
The extent to which a material can endanger people or animals is known as its toxicity. Acute and sub-acute toxicity are the two different forms of toxicity (Štambuk et al., 2022). Acute toxicology experiments identify the LD50, or median lethal dose, as dosage level at which half of the animals’ death take place. The greatest concentration at which a tested substance does not exhibit any negative effects, also known as the NOEL (No Effect Level), is established in sub-acute toxicity tests (Abbas Momtazi-Boroje et al., 2017).
In a study, ten male Wistar albino rats, each weighing between 180 and 200 g were examined for acute toxicity. Five rats per group were used to separate the animals into control and test groups. Orally administered to the test group of rats was a powder made from grape leaves at a dosage of 5,000 mg/kg body weight. The same volume of distilled water was given to control rats. After extract administration, animals were closely monitored for the following 24 h and 14 d. The rats were monitored for toxicity, morphological changes, and death. The investigated extract’s median lethal dose (LD50) exceeded 5,000 mg/kg body weight, as evidenced by the absence of any obvious toxic effects, aberrant behaviours, or mortality following a single oral dosage of 5,000 mg/kg body weight of V. vinifera leaf extract. All animals were alive for 14 days. Based on this the effective dose of (500 mg/kg) was chosen (Sayed et al., 2016).
9 Future perspectives and conclusion
The importance of leaves as a medicinal property in the food industry is highlighted by the growing interest in using various types of leaves that were considered waste. This review focuses on the phytochemical composition of the various grape leaf varieties that are grown throughout the world and their potential for bioactivity in the food business. The significant effects of grape leaves as an antioxidant, anti-inflammatory, anti-obesity, anti-viral, and hepatoprotective agent are demonstrated by pharmacological research. Each bioactive component acts on the body differently and through different pathways to ensure its functionality. Due to the high incidence of non-communicable diseases including cancer, cirrhosis, arthritis, etc., dietary habits and consumption of people have evolved to emphasise eating a balanced diet and preventing these diseases. Even though leaves have nutritional benefits, the current food market prioritises producing products at low costs that are affordable to the majority of the population. This is made possible by using inexpensive food materials like grape leaves, which are typically discarded and considered waste. Consumer demand for low-cost items rises as a result of the use of agricultural waste, which ultimately boosts economic growth and the food market. Grape leaves have a range of health advantages, including antioxidant, anti-diabetic, anti-bacterial, anti-obesity, anti-inflammatory, antiviral, hepatoprotective, and gastroprotective effects. Grape leaves are advantageous for use in the management of healthcare because they are nutrient-rich and include bioactive substances such as flavonoids, phenolic acids, antioxidants, and phenols. In addition to being used as a meal and in their raw state in many countries as traditionally, grape leaves have been utilised in baking. In the future, a variety of food items made from grape leaves could be developed that is useful in addressing problems related to nutrition. For the best utilisation of the bioactive components present in grape leaves, the use of grape-leaf-based value-added food products should be supported.
Author contributions
JS: Writing–original draft. PR: Conceptualization, Supervision, Writing–review and editing. RK: Investigation, Writing–original draft. Harmandeep Kaur: Data curation, Formal Analysis, Writing–review and editing. RG: Validation, Writing–review and editing. SK: Supervision, Writing–review and editing. SE: Formal Analysis, Visualization, Writing–review and editing. RC: Validation, Writing–review and editing. SS: Formal analysis, Supervision, Writing–review and editing. JM: Funding acquisition, Formal analysis, Supervision, Writing–review and editing.
Funding
The author(s) declare no financial support was received for the research, authorship, and/or publication of this article.
Acknowledgments
The authors thank Lovely professional university for providing secondary data for writing the manuscript.
Conflict of interest
The authors declare that the research was conducted in the absence of any commercial or financial relationships that could be construed as a potential conflict of interest.
Publisher’s note
All claims expressed in this article are solely those of the authors and do not necessarily represent those of their affiliated organizations, or those of the publisher, the editors and the reviewers. Any product that may be evaluated in this article, or claim that may be made by its manufacturer, is not guaranteed or endorsed by the publisher.
Supplementary material
The Supplementary Material for this article can be found online at: https://www.frontiersin.org/articles/10.3389/fchem.2023.1290619/full#supplementary-material
References
Abbas Momtazi-Borojeni, A., Esmaeili, S. A., Abdollahi, E., and Sahebkar, A. (2017). A review on the pharmacology and toxicology of steviol glycosides extracted from Stevia rebaudiana. Curr. Pharm. Des. 23, 1616–1622. doi:10.2174/1381612822666161021142835
Ahmad, R., Ahmad, N., and Shehzad, A. (2019). Solvent and temperature effects of accelerated solvent extraction (ASE) with Ultra-high pressure liquid chromatography (UHPLC-PDA) technique for determination of Piperine and its ICP-MS analysis. Ind. Crops Prod. 136, 37–49. doi:10.1016/j.indcrop.2019.04.016
Ajila, C. M., Brar, S. K., Verma, M., Tyagi, R. D., Godbout, S., and Valéro, J. R. (2011). Extraction and analysis of polyphenols: recent trends. Crit. Rev. Biotechnol. 31, 227–249. doi:10.3109/07388551.2010.513677
Alara, O. R., Abdurahman, N. H., and Ukaegbu, C. I. (2018a). Soxhlet extraction of phenolic compounds from Vernonia cinerea leaves and its antioxidant activity. J. Appl. Res. Med. Aromat. Plants 11, 12–17. doi:10.1016/j.jarmap.2018.07.003
Alara, O. R., Abdurahman, N. H., and Ukaegbu, C. I. (2021). Extraction of phenolic compounds: a review. Curr. Res. Food Sci. 4, 200–214. doi:10.1016/j.crfs.2021.03.011
Alara, O. R., Abdurahman, N. H., Ukaegbu, C. I., and Azhari, N. H. (2018b). Vernonia cinerea leaves as the source of phenolic compounds, antioxidants, and anti-diabetic activity using microwave-assisted extraction technique. Ind. Crops Prod. 122, 533–544. doi:10.1016/j.indcrop.2018.06.034
Ali, M., Benfante, V., Stefano, A., Yezzi, A., Di Raimondo, D., Tuttolomondo, A., et al. (2023). Anti-arthritic and anti-cancer activities of polyphenols: a review of the most recent in vitro assays. Life 13, 361. doi:10.3390/life13020361
Alibas, I. (2014). Microwave, air and combined microwave-air drying of grape leaves (Vitis vinifera L.) and the determination of some quality parameters. Int. J. Food Eng. 10, 69–88. doi:10.1515/ijfe-2012-0037
Al Juhaimi, F., Uslu, N., Özcan, M. M., Gülcü, M., Mohamed Ahmed, I. A., Alqah, H. A., et al. (2019). Effect of fermentation on antioxidant activity and phenolic compounds of the leaves of five grape varieties. J. Process Preserv 43, e13979. doi:10.1111/jfpp.13979
Altunkaya, A. (2014). Effect of grape leaf extract on phenolic profile and browning of fresh-cut lettuce (L actuca sativa). J. Food Process Preserv 38, 527–534. doi:10.1111/j.1745-4549.2012.00803.x
Alvarez-Rivera, G., Bueno, M., Ballesteros-Vivas, D., Mendiola, J. A., and Ibanez, E. (2020). “Pressurized liquid extraction,” in Liquid-phase extraction (Netherlands: Elsevier), 375–398. doi:10.1016/B978-0-12-816911-7.00013-X
Amen, Y., Sherif, A. E., Shawky, N. M., Abdelrahman, R. S., Wink, M., and Sobeh, M. (2020). Grape-leaf extract attenuates alcohol-induced liver injury via interference with NF-κBsignaling pathway. Biomolecules 10, 558. doi:10.3390/biom10040558
Aouey, B., Samet, A. M., Fetoui, H., Simmonds, M. S., and Bouaziz, M. (2016). Anti-oxidant, anti-inflammatory, analgesic and antipyretic activities of grapevine leaf extract (Vitis vinifera) in mice and identification of its active constituents by LC–MS/MS analyses. Biomed. Pharmacother. 84, 1088–1098. doi:10.1016/j.biopha.2016.10.033
Arika, W., Kibiti, C. M., Njagi, J. M., and Ngugi, M. P. (2019). In vitro antioxidant properties of dichloromethanolic leaf extract of Gnidia glauca (Fresen) as a promising antiobesity drug. J. Evid-Based Integr. Med. 24, 2515690X1988325. doi:10.1177/2515690X19883258
Azhdari, M., Zilaee, M., Karandish, M., Hosseini, S. A., Mansoori, A., Zendehdel, M., et al. (2020). Red vine leaf extract (AS 195) can improve some signs and symptoms of chronic venous insufficiency, a systematic review. Phytother. Res. 34, 2577–2585. doi:10.1002/ptr.6705
Azmir, J., Zaidul, I. S., Rahman, M. M., Sharif, K. M., Mohamed, A., Sahena, F., et al. (2013). Techniques for extraction of bioactive compounds from plant materials: a review. J. Food Eng. 117, 426–436. doi:10.1016/j.jfoodeng.2013.01.014
Azwanida, N. N. (2015). A review on the extraction methods use in medicinal plants, principle, strength and limitation. Int. J. Med. Aromat. 4, 2167–0412. doi:10.4172/2167-0412.1000196
Babri, S., Mohaddes, G., Feizi, I., Mohammadnia, A., Niapour, A., Alihemmati, A., et al. (2014). Effect of troxerutin on synaptic plasticity of hippocampal dentate gyrus neurons in a β-amyloid model of Alzheimer׳ s disease: an electrophysiological study. Eur. J. Pharmacol. 732, 19–25. doi:10.1016/j.ejphar.2014.03.018
Bachtler, S., and Bart, H. J. (2018). Polyphenols from red vine leaves using alternative processing techniques. Processes 6, 262. doi:10.3390/pr6120262
Badr, A. N., Gromadzka, K., Shehata, M. G., Stuper-Szablewska, K., Drzewiecka, K., Abdel-Razek, A. G., et al. (2021). Encapsulated Bioactive Ingredients of grape by-products applicate in fresh-cut fruit and juices diminished the ochratoxins. J. Food Process Preserv 45, e15112. doi:10.1111/jfpp.15112
Bai, J., Zhang, Y., Tang, C., Hou, Y., Ai, X., Chen, X., et al. (2021). Gallic acid: pharmacological activities and molecular mechanisms involved in inflammation-related diseases. Biomed. Pharmacother. 133, 110985. doi:10.1016/j.biopha.2020.110985
Bajomo, E. M., Aing, M. S., Ford, L. S., and Niemeyer, E. D. (2022). Chemotyping of commercially available basil (Ocimumbasilicum L.) varieties: cultivar and morphotype influence phenolic acid composition and antioxidant properties. NFS J. 26, 1–9. doi:10.1016/j.nfs.2022.01.001
Banjanin, T., Uslu, N., Vasic, Z. R., and Özcan, M. M. (2021). Effect of grape varieties on bioactive properties, phenolic composition, and mineral contents of different grape-vine leaves. J. Food Process Preserv 45, e15159. doi:10.1111/jfpp.15159
Belcaro, G. (2015). A clinical comparison of Pycnogenol, Antistax, and stocking in chronic venous insufficiency. Int. J. Angiol. 24, 268–274. doi:10.1055/s-0035-1556060
Belcaro, G., Dugall, M., Luzzi, R., Corsi, M., Ledda, A., Ricci, A., et al. (2017). Management of varicose veins and chronic venous insufficiency in a comparative registry with nine venoactive products in comparison with stockings. Int. J. Angiol. 26, 170–178. doi:10.1055/s-0036-1597756
Borah, R., Kumari, D., Gogoi, A., Biswas, S., Goswami, R., Shim, J., et al. (2018). Efficacy and field applicability of Burmese grape leaf extract (BGLE) for cadmium removal: an implication of metal removal from natural water. Ecotoxicol. Environ. Saf. 147, 585–593. doi:10.1016/j.ecoenv.2017.09.002
Borai, I. H., Ezz, M. K., Rizk, M. Z., Aly, H. F., El-Sherbiny, M., Matloub, A. A., et al. (2017). Therapeutic impact of grape leaves polyphenols on certain biochemical and neurological markers in AlCl3-induced Alzheimer’s disease. Biomed. Pharmacother. 93, 837–851. doi:10.1016/j.biopha.2017.07.038
Both, S., Chemat, F., and Strube, J. (2014). Extraction of polyphenols from black tea–conventional and ultrasound assisted extraction. UltrasonSonochem 21, 1030–1034. doi:10.1016/j.ultsonch.2013.11.005
Brad, K., Zhang, Y., Yang, X., and Wang, T. (2017). “Study on the optimum extraction conditions and antibacterial activities in vitro of total flavonoids from grape leaves,” in 2017 6th international conference on energy and environmental protection (ICEEP 2017) (Netherlands: Atlantis Press), 495–504. doi:10.2991/iceep-17.2017.88
BrglezMojzer, E., KnezHrnčič, M., Škerget, M., Ž, K., and Bren, U. (2016). Polyphenols: extraction methods, antioxidative action, bioavailability and anticarcinogenic effects. Molecules 21, 901. doi:10.3390/molecules21070901
Capone, D. L., Barker, A., Pearson, W., and Francis, I. L. (2021). Influence of inclusion of grapevine leaves, rachis and peduncles during fermentation on the flavour and volatile composition of Vitis vinifera cv. Shiraz wine. Aust. J. Grape Wine Res. 27, 348–359. doi:10.1111/ajgw.12489
Carrera, C., Ruiz-Rodríguez, A., Palma, M., and Barroso, C. G. (2012). Ultrasound assisted extraction of phenolic compounds from grapes. Anal. Chim. acta 732, 100–104. doi:10.1016/j.aca.2011.11.032
Chao, W. W., Chen, S. J., Peng, H. C., Liao, J. W., and Chou, S. T. (2019). Antioxidant activity of graptopetalumparaguayense E. Walther leaf extract counteracts oxidative stress induced by ethanol and carbon tetrachloride Co-induced hepatotoxicity in rats. Antioxidants 8, 251. doi:10.3390/antiox8080251
Chaturvedi, A. K. (2018). Extraction of neutraceuticals from plants by microwave assisted extraction. Syst. Rev. Pharm. 9, 31–35. doi:10.5530/srp.2018.1.6
Chaves, J. O., De Souza, M. C., Da Silva, L. C., Lachos-Perez, D., Torres-Mayanga, P. C., Machado, A. P., et al. (2020). Extraction of flavonoids from natural sources using modern techniques. Front. Chem. 8, 507887. doi:10.3389/fchem.2020.507887
Chemat, F., Rombaut, N., Sicaire, A. G., Meullemiestre, A., Fabiano-Tixier, A. S., and Abert-Vian, M. (2017). Ultrasound assisted extraction of food and natural products. Mechanisms, techniques, combinations, protocols and applications. A review. A Rev. Ultrason. 34, 540–560. doi:10.1016/j.ultsonch.2016.06.035
Chitwood, D. H. (2021). The shapes of wine and table grape leaves: an ampelometric study inspired by the methods of Pierre Galet. Plants People Planet 3, 155–170. doi:10.1002/ppp3.10157
Chuyen, H. V., Nguyen, M. H., Roach, P. D., Golding, J. B., and Parks, S. E. (2018). Microwave-assisted extraction and ultrasound-assisted extraction for recovering carotenoids from Gac peel and their effects on antioxidant capacity of the extracts. Food Sci. Nutr. 6, 189–196. doi:10.1002/fsn3.546
Cosme, F., Pinto, T., and Vilela, A. (2017). Oenology in the kitchen: the sensory experience offered by culinary dishes cooked with alcoholic drinks, grapes and grape leaves. Beverages 3, 42. doi:10.3390/beverages3030042
Dar, L. A., Mir, S. A., and Shafi, S. (2022). Antioxidant, anti-inflammatory and anti-diabetic potential of Vitis vinifera linn leaf extracts. World J. Pharm. Res. 11, 1858–1876. doi:10.20959/wjpr20222-23054
Da Silva, R. P., Rocha-Santos, T. A., and Duarte, A. C. (2016). Supercritical fluid extraction of bioactive compounds. Trends Anal. Chem. 76, 40–51. doi:10.1016/j.trac.2015.11.013
de Melo, M. M., Carius, B., Simões, M. M., Portugal, I., Saraiva, J., and Silva, C. M. (2020). Supercritical CO2 extraction of V. vinifera leaves: influence of cosolvents and particle size on removal kinetics and selectivity to target compounds. J. Supercrit. Fluids 165, 104959. doi:10.1016/j.supflu.2020.104959
Deo, S., Janghel, A., Raut, P., Bhosle, D., Verma, C., Kumar, S. S., et al. (2015). Emerging Microwave Assisted Extraction (MAE) techniques as an innovative green technologies for the effective extraction of the active phytopharmaceuticals. Res. J. Pharm. Technol. 8, 655–666. doi:10.5958/0974-360X.2015.00104.3
Devi, S., and Singh, R. (2017). Evaluation of antioxidant and anti-hypercholesterolemic potential of Vitis vinifera leaves. Food Sci. Hum. Wellness. 6, 131–136. doi:10.1016/j.fshw.2017.07.002
Direito, R., Rocha, J., Sepodes, B., and Eduardo-Figueira, M. (2021). Phenolic compounds impact on rheumatoid arthritis, inflammatory bowel disease and microbiota modulation. Pharmaceutics 13, 145. doi:10.3390/pharmaceutics13020145
Djemaa-Landri, K., Hamri-Zeghichi, S., Valls, J., Cluzet, S., Tristan, R., Boulahbal, N., et al. (2020). Phenolic content and antioxidant activities of Vitis vinifera L. leaf extracts obtained by conventional solvent and microwave-assisted extractions. J. Food Meas. Charact. 14, 3551–3564. doi:10.1007/s11694-020-00596-w
Doğan, A., and Cüneyt, U. Y. (2020). Üzüm yaprak rengini belirlemede farklı bir yaklaşım. J. Agric. Fac. Gaziosmanpaşa Univ. (JAFAG) 37, 44–52. doi:10.13002/jafag4657
Dogan, Y., Nedelcheva, A., Łuczaj, Ł., Drăgulescu, C., Stefkov, G., Maglajlić, A., et al. (2015). Of the importance of a leaf: the ethnobotany of sarma in Turkey and the Balkans. J. Ethnobiol. Ethnomedicine 11, 26–35. doi:10.1186/s13002-015-0002-x
dos Santos Lacerda, D., Santos, C. F., Oliveira, A. S., Zimmermann, R., Schneider, R., Agostini, F., et al. (2014). Antioxidant and hepatoprotective effects of an organic grapevine leaf (Vitis labrusca L.) extract in diabetic rats. RSC Adv. 4, 52611–52619. doi:10.1039/c4ra08396b
Dresch, R. R., Dresch, M. T., Biegelmeyer, R., Argenta, D. F., da Rocha, R. F., Teixeira, H. F., et al. (2018). Potential use of secondary products of the agri-food industry for topical formulations and comparative analysis of antioxidant activity of grape leaf polyphenols. Nat. Prod. Res. 32, 486–492. doi:10.1080/14786419.2017.1315573
Duangjan, C., Rangsinth, P., Zhang, S., Gu, X., Wink, M., and Tencomnao, T. (2021). Vitis vinifera leaf extract protects against glutamate-induced oxidative toxicity in HT22 hippocampal neuronal cells and increases stress resistance properties in Caenorhabditis elegans. Front. Nutr. 8, 634100. doi:10.3389/fnut.2021.634100
El-Nadi, Y. A. (2017). Solvent extraction and its applications on ore processing and recovery of metals: classical approach. Sep. Purif. Rev. 46, 195–215. doi:10.1080/15422119.2016.1240085
Ferhi, S., Santaniello, S., Zerizer, S., Cruciani, S., Fadda, A., Sanna, D., et al. (2019). Total phenols from grape leaves counteract cell proliferation and modulate apoptosis-related gene expression in MCF-7 and HepG2 human cancer cell lines. Molecules 24, 612. doi:10.3390/molecules24030612
Fiume, M. M., Bergfeld, W. F., Belsito, D. V., Hill, R. A., Klaassen, C. D., Liebler, D. C., et al. (2014). Safety assessment of Vitis vinifera (Grape)-derived ingredients as used in cosmetics. Int. J. Toxicol. 33, 48S–83S. doi:10.1177/1091581814545247
Flamminii, F., Di Mattia, C. D., Sacchetti, G., Neri, L., Mastrocola, D., and Pittia, P. (2020). Physical and sensory properties of mayonnaise enriched with encapsulated olive leaf phenolic extracts. Foods 9, 997. doi:10.3390/foods9080997
Fomo, G., Madzimbamuto, T. N., and Ojumu, T. V. (2020). Applications of nonconventional green extraction technologies in process industries: challenges, limitations and perspectives. Sustainability 12, 5244. doi:10.3390/su12135244
Garcia-Vaquero, M., Rajauria, G., Tiwari, B., Sweeney, T., and O’Doherty, J. (2018). Extraction and yield optimisation of fucose, glucans and associated antioxidant activities from Laminaria digitata by applying response surface methodology to high intensity ultrasound-assisted extraction. Mar. Drugs 16, 257. doi:10.3390/md16080257
Gebreselassie, E., and Clifford, H. (2016). “Oxidative stability and shelf life of crackers, cookies, and biscuits,” in Oxidative stability and shelf life of foods containing oils and fats (Urbana, IL: AOCS Press), 461–478. doi:10.1016/B978-1-63067-056-6.00012-4
Goldminz, A. M., Au, S. C., Kim, N., Gottlieb, A. B., and Lizzul, P. F. (2013). NF-κB: an essential transcription factor in psoriasis. J. Dermatol Sci. 69, 89–94. doi:10.1016/j.jdermsci.2012.11.002
Gomes, S. V., Portugal, L. A., dos Anjos, J. P., de Jesus, O. N., de Oliveira, E. J., David, J. P., et al. (2017). Accelerated solvent extraction of phenolic compounds exploiting a Box-Behnken design and quantification of five flavonoids by HPLC-DAD in Passiflora species. Microchem J. 132, 28–35. doi:10.1016/j.microc.2016.12.021
Gómez, B., Munekata, P. E., Gavahian, M., Barba, F. J., Martí-Quijal, F. J., Bolumar, T., et al. (2019). Application of pulsed electric fields in meat and fish processing industries: an overview. Food Res. Int. 123, 95–105. doi:10.1016/j.foodres.2019.04.047
Gomez, L., Tiwari, B., and Garcia-Vaquero, M. (2020). “Emerging extraction techniques: microwave-assisted extraction,” in Sustainable seaweed technologies (Elsevier), 207–224. doi:10.1016/B978-0-12-817943-7.00008-1
Goufo, P., Singh, R. K., and Cortez, I. (2020). A reference list of phenolic compounds (including stilbenes) in grapevine (Vitis vinifera L.) roots, woods, canes, stems, and leaves. Antioxidants 9, 398. doi:10.3390/antiox9050398
Gülcü, M., Ghafoor, K., Al-Juhaimi, F., Özcan, M. M., Uslu, N., Babiker, E. E., et al. (2020). Effect of grape (Vitis vinifera L.) varieties and harvest periods on bioactive compounds, antioxidant activity, phenolic composition, mineral contents, and fatty acid compositions of Vitis leave and oils. J. Food Process Preserv 44, e14890. doi:10.1111/jfpp.14890
Güler, A., and Candemir, A. (2014). Total phenolic and flavonoid contents, phenolic compositions and color properties of fresh grape leaves. TürkTarımDoğaBilimDerg 1 (1), 778–782.
Ha, A. T., Rahmawati, L., You, L., Hossain, M. A., Kim, J. H., and Cho, J. Y. (2021). Anti-inflammatory, antioxidant, moisturizing, and antimelanogenesis effects of quercetin 3-O-β-D-glucuronide in human keratinocytes and melanoma cells via activation of NF-κB and AP-1 pathways. Int. J. Mol. Sci. 23, 433. doi:10.3390/ijms23010433
Hayta, M., and Işçimen, E. M. (2018). Antidiabetic, antihypertensive and antioxidant properties of grapevine leaf extracts obtained by ultrasound, microwave assisted, and classical solvent extraction. Erwerbs-Obstbau. 60. doi:10.1007/s10341-018-0406-6
Hayta, M., and Işçimen, E. M. (2021). Antioxidant properties of grapevine leaves obtained by optimized microwave assisted extraction. ÇukurovaTarımGıdaBilimleriDergisi 36, 1–2. doi:10.36846/CJAFS.2021.30
Hefnawy, H. T., El-Shourbagy, G. A., and Ramadan, M. F. (2016). Phenolic extracts of carrot, grape leaf and turmeric powder: antioxidant potential and application in biscuits. J. Food Meas. Charact. 10, 576–583. doi:10.1007/s11694-016-9339-7
Hu, C., Chen, Y., Cao, Y., Jia, Y., and Zhang, J. (2020). Metabolomics analysis reveals the protective effect of quercetin-3-O-galactoside (Hyperoside) on liver injury in mice induced by acetaminophen. J. Food Biochem. 44, e13420. doi:10.1111/jfbc.13420
Insanu, M., Karimah, H., Pramastya, H., and Fidrianny, I. (2021). Phytochemical compounds and pharmacological activities of Vitis vinifera L.: an updated review. Biointerface Res. Appl. Chem. 11, 13829–13849. doi:10.33263/BRIAC115.1382913849
Jakab, J., Miškić, B., Mikšić, Š., Juranić, B., Ćosić, V., Schwarz, D., et al. (2021). Adipogenesis as a potential anti-obesity target: a review of pharmacological treatment and natural products. Diabetes MetabSynd Ob. 14, 67–83. doi:10.2147/dmso.s281186
Jang, D. I., Lee, A. H., Shin, H. Y., Song, H. R., Park, J. H., Kang, T. B., et al. (2021). The role of tumor necrosis factor alpha (TNF-α) in autoimmune disease and current TNF-α inhibitors in therapeutics. Int. J. Mol. Sci. 22, 2719. doi:10.3390/ijms22052719
John, C. M., and Arockiasamy, S. (2020). Syringic acid (4-hydroxy-3, 5-dimethoxybenzoic acid) inhibits adipogenesis and promotes lipolysis in 3T3-L1 adipocytes. Nat. Prod. Res. 34, 3432–3436. doi:10.1080/14786419.2019.1573820
Jovanović, A., Petrović, P., Đorđević, V., Zdunić, G., Šavikin, K., and Bugarski, B. (2017). Polyphenols extraction from plant sources. Lekovitesirovine 17, 45–49. doi:10.5937/leksir1737045J
Jridi, M., Abdelhedi, O., Kchaou, H., Msaddak, L., Nasri, M., Zouari, N., et al. (2019). Vine (Vitis vinifera L.) leaves as a functional ingredient in pistachio calisson formulations. Food Biosci. 31, 100436. doi:10.1016/j.fbio.2019.100436
Jung, Y., Park, J., Kim, H. L., Sim, J. E., Youn, D. H., Kang, J., et al. (2018). Vanillic acid attenuates obesity via activation of the AMPK pathway and thermogenic factors in vivo and in vitro. FASEB J. 32, 1388–1402. doi:10.1096/fj.201700231RR
Katalinić, V., Generalić, I., Skroza, D., Ljubenkov, I., Teskera, A., Konta, I., et al. (2009). Insight in the phenolic composition and antioxidative properties of Vitis vinifera leaves extracts. Croat. J. Food Sci. Technol. 1, 7–15.
Katalinic, V., Mozina, S. S., Generalic, I., Skroza, D., Ljubenkov, I., and Klancnik, A. (2013). Phenolic profile, antioxidant capacity, and antimicrobial activity of leaf extracts from six Vitis vinifera L. varieties. Int. J. Food Prop. 16, 45–60. doi:10.1080/10942912.2010.526274
Kaur, P., Singh, J., Kaur, M., Rasane, P., Kaur, S., Kaur, J., et al. (2023). Corn silk as an agricultural waste: a comprehensive review on its nutritional composition and bioactive potential. Waste Biomass Valorization 14, 1413–1432. doi:10.1007/s12649-022-02016-0
Khumaidah, L., Purnomo, A. S., and Fatmawati, S. (2019). Antimicrobial activity of Sonneratia ovata backer. HAYATI J. Biosci. 26, 152. doi:10.4308/hjb.26.4.152
Kim, J. M., and Heo, H. J. (2022). The roles of catechins in regulation of systemic inflammation. Food Sci. Biotechnol. 31, 957–970. doi:10.1007/s10068-022-01069-0
Kumar, A., Nirmal, P., Kumar, M., Jose, A., Tomer, V., Oz, E., et al. (2023). Major phytochemicals: recent advances in health benefits and extraction method. Molecules 28, 887. doi:10.3390/molecules28020887
Kumar, K., Srivastav, S., and Sharanagat, V. S. (2021). Ultrasound assisted extraction (UAE) of bioactive compounds from fruit and vegetable processing by-products: a review. UltrasonSonochem 70, 105325. doi:10.1016/j.ultsonch.2020.105325
Labanca, F., Faraone, I., Nolè, M. R., Hornedo-Ortega, R., Russo, D., García-Parrilla, M. C., et al. (2020). New Insights into the exploitation of Vitis vinifera L. cv. Aglianico leaf extracts for nutraceutical purposes. Antioxidants 9, 708. doi:10.3390/antiox9080708
Lantzouraki, D. Z., Tsiaka, T., Soteriou, N., Asimomiti, G., Spanidi, E., Natskoulis, P., et al. (2020). Antioxidant profiles of Vitis vinifera L. and Salvia triloba L. leaves using high-energy extraction methodologies. J. AOAC Int. 103, 413–421. doi:10.5740/jaoacint.19-0261
Lee, Y. G., Woo, H., Choi, C., Ryoo, G. H., Chung, Y. J., Lee, J. H., et al. (2022). Supplementation with Vitis vinifera jingzaojing leaf and shoot extract improves exercise endurance in mice. Nutrients 14, 4033. doi:10.3390/nu14194033
Lefebvre, T., Destandau, E., and Lesellier, E. (2021). Selective extraction of bioactive compounds from plants using recent extraction techniques: a review. J. Chromatogr. A 1635, 461770. doi:10.1016/j.chroma.2020.461770
Li, H., Wei, Y., Zhang, H., Chen, H., and Meng, J. (2022). Fine-grained classification of grape leaves via a pyramid residual convolution neural network. Int. J. Agri Biol. Eng. 15, 197–203. doi:10.25165/j.ijabe.20221502.6894
Lin, Y. S., Chen, H. J., Huang, J. P., Lee, P. C., Tsai, C. R., Hsu, T. F., et al. (2017). Kinetics of tyrosinase inhibitory activity using Vitis vinifera leaf extracts. Biomed. Res. Int. 2017, 1–5. doi:10.1155/2017/5232680
Llompart, M., Celeiro, M., and Dagnac, T. (2019). Microwave-assisted extraction of pharmaceuticals, personal care products and industrial contaminants in the environment. Trends Anal. Chem. 116, 136–150. doi:10.1016/j.trac.2019.04.029
Madadian, E., Rahimi, J., Mohebbi, M., and Simakov, D. S. (2022). Grape pomace as an energy source for the food industry: a thermochemical and kinetic analysis. Food Bioprod. Process 132, 177–187. doi:10.1016/j.fbp.2022.01.006
Maia, M., Ferreira, A. E., Laureano, G., Marques, A. P., Torres, V. M., Silva, A. B., et al. (2019). Vitis vinifera “Pinot noir”leaves as a source of bioactive nutraceutical compounds. Food Funct. 10, 3822–3827. doi:10.1039/C8FO02328J
Marabini, L., Melzi, G., Lolli, F., Dell'Agli, M., Piazza, S., Sangiovanni, E., et al. (2020). Effects of Vitis vinifera L. leaves extract on UV radiation damage in human keratinocytes (HaCaT). J. Photochem. Photobiol. B, Biol. 204, 111810. doi:10.1016/j.jphotobiol.2020.111810
Medina-Torres, N., Ayora-Talavera, T., Espinosa-Andrews, H., Sánchez-Contreras, A., and Pacheco, N. (2017). Ultrasound assisted extraction for the recovery of phenolic compounds from vegetable sources. Agron 7, 47. doi:10.3390/agronomy7030047
Meng, L., Jiao, Y., Zhou, X., Liang, C., Yan, K., Zhao, Y., et al. (2021). Leaf extract from Vitis vinifera L. reduces high fat diet-induced obesity in mice. Food Funct. 12, 6452–6463. doi:10.1039/D1FO00460C
Micheli, L., Mattoli, L., Maidecchi, A., Pacini, A., Ghelardini, C., and Di Cesare Mannelli, L. (2018). Effect of Vitis vinifera hydroalcoholic extract against oxaliplatin neurotoxicity: in vitro and in vivo evidence. Sci. Rep. 8, 14364. doi:10.1038/s41598-018-32691-w
Mildner-Szkudlarz, S., Siger, A., Przygoński, K., Radziejewska-Kubzdela, E., and Zawirska-Wojtasiak, R. (2022). Nɛ-(carboxymethyl) lysine, available lysine, and volatile compound profile of biscuits enriched with grape by-product during storage. Plant Foods Hum. Nutr. 77, 190–197. doi:10.1007/s11130-022-00960-9
Minegishi, Y., Haramizu, S., Hase, T., and Murase, T. (2011). Red grape leaf extract improves endurance capacity by facilitating fatty acid utilization in skeletal muscle in mice. Eur. J. Appl. Psychol. 111, 1983–1989. doi:10.1007/s00421-011-1826-2
Mohammed, C., Mohamed, A., Ahmed, A., and Mariod, A. (2020). Antioxidant and anti-diabetic activity of pomegranate (Punica granatum L.) leaves extracts. Foods Raw Mater 8, 329–336. doi:10.21603/2308-4057-2020-2-329-336
Mohan, P. K., Krishna, T. A., Thirumurugan, A., Kumar, T. S., and Kumari, B. R. (2022). Chemical profiling and in vitro antiurolithiatic activity of pleurolobus gangeticus (L.) J. St.-Hil. ex H. Ohashi and K. Ohashi along with its antioxidant and antibacterial properties. ApplBiochemBiotechnol 194, 5037–5059. doi:10.1007/s12010-022-04017-0
Moldovan, M. L., Carpa, R., Fizeșan, I., Vlase, L., Bogdan, C., Iurian, S. M., et al. (2020). Phytochemical profile and biological activities of tendrils and leaves extracts from a variety of Vitis vinifera L. L. Antioxidants 9, 373. doi:10.3390/antiox9050373
Mottaleb, M. A., and Sarker, S. D. (2012). “Accelerated solvent extraction for natural products isolation,” in Nat prod isolation (Berlin, Germany: Springer), 75–87. doi:10.1007/978-1-61779-624-1_4
Mustapa, A. N., Martin, A., Gallego, J. R., Mato, R. B., and Cocero, M. J. (2015). Microwave-assisted extraction of polyphenols from Clinacanthus nutans Lindau medicinal plant: energy perspective and kinetics modeling. Chem. Eng. Process Process Intensif. 97, 66–74. doi:10.1016/j.cep.2015.08.013
Naviglio, D., Scarano, P., Ciaravolo, M., and Gallo, M. (2019). Rapid Solid-Liquid Dynamic Extraction (RSLDE): a powerful and greener alternative to the latest solid-liquid extraction techniques. Foods 8, 245. doi:10.3390/foods8070245
Negam, D. R., Mustafa, R. A., and Elsawy, N. A. (2020). The biological effect of grape leaves on liver disease rats induced by carbon tetrachloride (CCL4). World J. Med. Educ. Res. 24.
Oroian, M., Ursachi, F., and Dranca, F. (2020). Ultrasound-assisted extraction of polyphenols from crude pollen. Antioxidants 9, 322. doi:10.3390/antiox9040322
Osorio-Tobón, J. F. (2020). Recent advances and comparisons of conventional and alternative extraction techniques of phenolic compounds. J. Food Sci. Technol. 57, 4299–4315. doi:10.1007/s13197-020-04433-2
Paczkowska-Walendowska, M., Miklaszewski, A., Michniak-Kohn, B., and Cielecka-Piontek, J. (2023). The antioxidant potential of resveratrol from red vine leaves delivered in an electrospun nanofiber system. Antioxidants 12, 1777. doi:10.3390/antiox12091777
Panja, P. (2018). Green extraction methods of food polyphenols from vegetable materials. CurrOpin Food Sci. 23, 173–182. doi:10.1016/j.cofs.2017.11.012
Pantelić, M. M., Zagorac, D. Č., Ćirić, I. Ž., Pergal, M. V., Relić, D. J., Todić, S. R., et al. (2017). Phenolic profiles, antioxidant activity and minerals in leaves of different grapevine varieties grown in Serbia. J. Food Compost Anal. 62, 76–83. doi:10.1016/j.jfca.2017.05.002
Pari, L., and Suresh, A. (2008). Effect of grape (Vitis vinifera L.) leaf extract on alcohol induced oxidative stress in rats. Food Chem. Toxicol. 46, 1627–1634. doi:10.1016/j.fct.2008.01.003
Picot-Allain, C., Mahomoodally, M. F., Ak, G., and Zengin, G. (2021). Conventional versus green extraction techniques—a comparative perspective. CurrOpin Food Sci. 40, 144–156. doi:10.1016/j.cofs.2021.02.009
Rajbhar, K. A., Dawda, H., and Mukundan, U. S. (2015). Polyphenols: methods of extraction. Sci. Revs ChemCommun 5, 1–6.
Reis, L. C., Carneiro, L. M., Branco, C. R., and Branco, A. (2015). Comparison of conventional microwave and focused microwave-assisted extraction to enhance the efficiency of the extraction of antioxidant flavonols from jocote pomace (Spondias purpurea L). Plant Foods Hum. Nutr. 70, 160–169. doi:10.1007/s11130-015-0473-x
Roselló-Soto, E., Barba, F. J., Parniakov, O., Galanakis, C. M., Lebovka, N., Grimi, N., et al. (2015). High voltage electrical discharges, pulsed electric field, and ultrasound assisted extraction of protein and phenolic compounds from olive kernel. Food Bioproc Tech. 8, 885–894. doi:10.1007/s11947-014-1456-x
Saadaoui, N., Weslati, A., Barkaoui, T., Khemiri, I., Gadacha, W., Souli, A., et al. (2020). Gastroprotective effect of leaf extract of two varieties grapevine (Vitis vinifera L.) native wild and cultivar grown in North of Tunisia against the oxidative stress induced by ethanol in rats. Biomarkers 25, 48–61. doi:10.1080/1354750X.2019.1691266
Sahoo, S., Awasthi, J. P., Sunkar, R., and Panda, S. K. (2017). Determining glutathione levels in plants. Plant Stress Toler. Methods Protoc. 1631, 273–277. doi:10.1007/978-1-4939-7136-7_16
Sangiovanni, E., Di Lorenzo, C., Piazza, S., Manzoni, Y., Brunelli, C., Fumagalli, M., et al. (2019). Vitis vinifera L. leaf extract inhibits in vitro mediators of inflammation and oxidative stress involved in inflammatory-based skin diseases. Antioxidants 8, 134. doi:10.3390/antiox8050134
Sayed, D. A., Fahmy, S. R., Soliman, A. M., and Hussein, N. S. (2016). Antiulcerogenic efficacy of ethanolic extract of vitis vinifera leaves in rats. Int. J. Pharm. Pharm. Sci. 8, 163–172. doi:10.22159/ijpps.2016v8i9.12846
Selka, M. A., Achouri, M. Y., Chenafa, A., Toumi, H., Othman, S. B., and Senia, E. (2019). The study of polyphenolic compounds profile and antioxidant activity of Viti Vinifera L. leaves from western regions of Algeria. Int. JPharmacogn 6, 20–29. doi:10.13040/IJPSR.0975-8232.IJP.6(1).20-29
Sharma, G. N., Singh, S., Singh, P., and Yadav, S. K. (2021). Antioxidant and antibacterial activity of vitis vinifera. Int. JToxicolPharmacol Res. 11, 112–118.
Shiekh, K. A., Olatunde, O. O., Zhang, B., Huda, N., and Benjakul, S. (2021). Pulsed electric field assisted process for extraction of bioactive compounds from custard apple (Annona squamosa) leaves. Food Chem. 359, 129976. doi:10.1016/j.foodchem.2021.129976
Simonetti, G., Brasili, E., and Pasqua, G. (2020). Antifungal activity of phenolic and polyphenolic compounds from different matrices of Vitis vinifera L. against human pathogens. Molecules 25, 3748. doi:10.3390/molecules25163748
Sridhar, A., Ponnuchamy, M., Kumar, P. S., Kapoor, A., Vo, D. V., and Prabhakar, S. (2021). Techniques and modeling of polyphenol extraction from food: a review. Environ. Chem. Lett. 19, 3409–3443. doi:10.1007/s10311-021-01217-8
Štambuk, P., Anić, M., Huzanić, N., Preiner, D., Karoglan, M., Kontić, J. K., et al. (2022). A simple method for the determination of polyphenolic compounds from grapevine leaves. Separations 9, 24. doi:10.3390/separations9020024
Suwal, S., and Marciniak, A. (2018). Technologies for the extraction, separation and purification of polyphenols–A review. Nepal J. Biotechnol. 6, 74–91. doi:10.3126/njb.v6i1.22341
Tambun, R., Alexander, V., and Ginting, Y. (2021). Performance comparison of maceration method, soxhletation method, and microwave-assisted extraction in extracting active compounds from soursop leaves (Annona muricata): a review. InIOP Conf. Ser. Mater. Sci. Eng. 1122, 012095. doi:10.1088/1757-899X/1122/1/012095
Teskey, G., Abrahem, R., Cao, R., Gyurjian, K., Islamoglu, H., Lucero, M., et al. (2018). Glutathione as a marker for human disease. Adv. Clin. Chem. 87, 141–159. doi:10.1016/bs.acc.2018.07.004
Tiwari, B. K. (2015). Ultrasound: a clean, green extraction technology. Trends Anal. Chem. 71, 100–109. doi:10.1016/j.trac.2015.04.013
Tomaz, I., Huzanić, N., Preiner, D., Stupić, D., Andabaka, Ž., Maletić, E., et al. (2019). “Extraction methods of polyphenol from grapes: extractions of grape polyphenols,” in Polyphenols in plants (United States: Academic Press), 151–167. doi:10.1016/B978-0-12-813768-0.00010-4
Uddin, M. S., Al Mamun, A., Kabir, M. T., Ahmad, J., Jeandet, P., Sarwar, M. S., et al. (2020). Neuroprotective role of polyphenols against oxidative stress-mediated neurodegeneration. Eur. J. Pharmacol. 886, 173412. doi:10.1016/j.ejphar.2020.173412
Vilela, A., and Pinto, T. (2019). Grape infusions: the flavor of grapes and health-promoting compounds in your tea cup. Beverages 5, 48. doi:10.3390/beverages5030048
Vinceković, M., Maslov Bandić, L., Jurić, S., Jalšenjak, N., Čaić, A., Živičnjak, I., et al. (2019). The enhancement of bioactive potential in Vitis vinifera leaves by application of microspheres loaded with biological and chemical agents. J. Plant Nutr. 42, 543–558. doi:10.1080/01904167.2019.1568467
Walid, S., and Rania, I. M. (2023). Effect of some packing materials on oxidative stability of fats in date biscuits. Food Technol. Res. J. 1, 15–25. doi:10.21608/ftrj.2023.288515
Xi, J., Li, Z., and Fan, Y. (2021). Recent advances in continuous extraction of bioactive ingredients from food-processing wastes by pulsed electric fields. Crit. Rev. Food Sci. Nutr. 61, 1738–1750. doi:10.1080/10408398.2020.1765308
Yousefi, M., Rahimi-Nasrabadi, M., Pourmortazavi, S. M., Wysokowski, M., Jesionowski, T., Ehrlich, H., et al. (2019). Supercritical fluid extraction of essential oils. Trends Anal. Chem. 118, 182–193. doi:10.1016/j.trac.2019.05.038
Zannella, C., Giugliano, R., Chianese, A., Buonocore, C., Vitale, G. A., Sanna, G., et al. (2021). Antiviral activity of Vitis vinifera leaf extract against SARS-CoV-2 and HSV-1. Viruses 13, 1263. doi:10.3390/v13071263
Zhang, J., Zhen, Y. F., Song, L. G., Kong, W. N., Shao, T. M., Li, X., et al. (2013). Salidroside attenuates beta amyloid-induced cognitive deficits via modulating oxidative stress and inflammatory mediators in rat hippocampus. Behav. Brain Res. 244, 70–81. doi:10.1016/j.bbr.2013.01.037
Zhang, Q. W., Lin, L. G., and Ye, W. C. (2018). Techniques for extraction and isolation of natural products: a comprehensive review. Chin. Med. 13, 20–26. doi:10.1186/s13020-018-0177-x
Zhao, P., Praissman, J. L., Grant, O. C., Cai, Y., Xiao, T., Rosenbalm, K. E., et al. (2020). Virus-receptor interactions of glycosylated SARS-CoV-2 spike and human ACE2 receptor. Cell Host Microbe 28, 586–601.e6. doi:10.1016/j.chom.2020.08.004
Keywords: grape leaves, antioxidant, extraction, polyphenols, health, application
Citation: Singh J, Rasane P, Kaur R, Kaur H, Garg R, Kaur S, Ercisli S, Choudhary R, Skrovankova S and Mlcek J (2023) Valorization of grape (Vitis vinifera) leaves for bioactive compounds: novel green extraction technologies and food-pharma applications. Front. Chem. 11:1290619. doi: 10.3389/fchem.2023.1290619
Received: 07 September 2023; Accepted: 27 November 2023;
Published: 12 December 2023.
Edited by:
Kandi Sridhar, Institut Agro Rennes-Angers, FranceReviewed by:
Chandra Mohan Chandrasekar, University of Milan, ItalyFranklin Chamorro, University of Vigo, Spain
Copyright © 2023 Singh, Rasane, Kaur, Kaur, Garg, Kaur, Ercisli, Choudhary, Skrovankova and Mlcek. This is an open-access article distributed under the terms of the Creative Commons Attribution License (CC BY). The use, distribution or reproduction in other forums is permitted, provided the original author(s) and the copyright owner(s) are credited and that the original publication in this journal is cited, in accordance with accepted academic practice. No use, distribution or reproduction is permitted which does not comply with these terms.
*Correspondence: Jyoti Singh, anlvdGlzaW5naDkzNzdAZ21haWwuY29t