- Department of Chemistry, Hankuk University of Foreign Studies, Yongin, Republic of Korea
The use of organocatalysts and a pot economy has strengthened recent organic syntheses. Synthetic methodologies may be applicable in laboratory preparation or in the industrial production of valuable organic compounds. In most cases, synthetic challenges are overcome by highly efficient and environmentally benign organocatalysts in a pot-economical manner. This is exemplified by the recent synthesis of tetrahydropyridine-containing (−)-quinine.
In the last several decades, great progress has been made in organic synthesis. However, we are still quite far away from the ideal goal. In particular, synthetic reactions should be environmentally benign and should remove costly purification procedures derived from the step-by-step procedures used in a myriad of protecting and deprotecting protocols. In recent years, great success was achieved in overcoming these drawbacks by using several techniques, including fluorous catalysis (De Wolf et al., 1999; Mika and Horvath, 2018), solid-supported catalysis (Nafiu et al., 2023), biocatalysis (Pyser et al., 2021), and organocatalysis with green chemistry metrics (Martínez J. et al., 2022). Various trials with solvent-free and aqueous reactions (Ruiz-Lopez et al., 2020) or ionic liquids (Earle and Seddon, 2000) also succeeded in organic synthesis, with certain limitations. In addition, multicomponent reactions (Boukis et al., 2018; Cimarelli, 2019) were also applied based on pot-economical synthesis (Grondal C. et al., 2010). In recent years, great success has been achieved using a combination of organocatalysis and pot-economical synthesis. The one-pot synthesis of a target molecule in the same reaction vessel is widely considered to be an efficient approach in synthetic organic chemistry.
Although catalytic reactions have been known for a long time (List, 2010; Vogel et al., 2016), including various metallic compounds and a few organic molecules, no systematic investigation has been performed (Hajos and Parrish, 1974; Bernhard and Wolfgang, 1978). The concept and the term “organocatalyst” were established in earnest by the novel laurates B. List (List, 2007) and D. MacMillan (MacMillan, 2008) in 2007. They carried out various reactions, such as the addition of electron-deficient olefins like aldol and even Diels-Alder reactions (Chen et al., 2022). The advantages of organocatalysts include their lack of sensitivity to moisture and oxygen, making them easy to handle. In addition, most of them are readily available at low cost, with relatively low toxicity compared with metal catalysts (Albrecht et al., 2022). Diverse organocatalysts were developed as chiral catalysts to warrant the streamlined synthesis of optically active and/or pure products needed in many areas, including pharmaceutical industries (Hughes, 2018; Han, et al., 2021).
In terms of the great synthetic challenges of any organic compounds requiring many steps, all the necessary reactions should proceed in a highly efficient manner, including a pot-economy. A minimum reaction vessel required for the reaction to complete is an economical approach, such as in a pot economy. (Grondal C. et al., 2010). Reactions utilizing step-by-step protocols under different conditions require costly work-up and purification procedures. For example, the following flowchart shows three different reactions needed to get D as a product from three different reactions I, II, and III, yielding B and C as synthetic intermediate molecules. However, when we want to get D from the starting material A through several reactions, without isolating B and C, we are able to save laborious costly work-up and purification.
The best way to perform the different reactions in an efficient and easy way is to carry out all necessary reactions I to III in one pot under the same condition with the same reagents in the same solvent. For example, the synthesis of substituted 2,6-disubstituted piperidine from 3-alkynyl-2-(N-α-methylbenzylaziridine) was successfully achieved in the same way as shown in Scheme 1 by using a one-pot reaction under catalytic hydrogenation, without isolating any synthetic intermediate (Yadav et al., 2016). Under this condition, four different reactions, entailing the hydrogenation of alkyne, aziridine ring opening, reductive cyclization, and deprotection of the α-methylbenzyl group at the nitrogen ring occurred, without changing anything else throughout the whole reaction sequences.
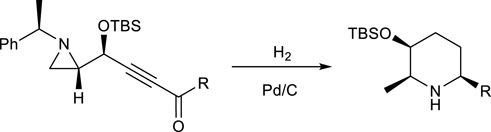
SCHEME 1. Synthesis of piperidine from multi-reactions of the starting material 3-alkynyl-2-(N-α-methylbenzyl)aziridine.
This is quite an exceptional case. In most cases, a preparative amount of product is accessible from reactions with different reagents under very specific conditions. Obtaining a decent amount of the final product in an efficient and handy protocol is possible with a one-pot synthesis. One-pot synthesis involves successive chemical reactions in just one reactor, from which sufficient purity is secured further in the sequence, without any purification. To carry out this synthesis properly, a decent reaction yield should be provided, with different reagents for the reaction to proceed to the specific position and functional group, even under different solvents. The reagents used for the previous reactions should not harm the subsequent reactions. For example, reactions II and III should be carried out by selecting proper reagents after killing the reactivity from the previous reactions to avoid harming the next reaction, i.e., reaction II from I and reaction III from both I and II. For this purpose, the synthetic intermediates in most cases are not stable for the next reaction, or they are in equilibrium for the next reaction. Selecting solvents is also a crucial factor for the specific reaction. In most cases, changing the solvents should be simple after one reaction, owing to the relatively low boiling points for them to be removed via simple evaporation.
For an efficient and environmentally benign synthesis, a pot-economical method is better for use in conjunction with organocatalysts. For example, the Michael reactions of nitroalkenes shown in Scheme 2 were investigated intensively, taking advantage of their superior reactivity toward the nucleophile and its practicability. Proper selection of organocatalysts derived from proline makes this transformation asymmetric, with high yields, and these are used in many cases. Though various organocatalysts were developed and utilized to make these reactions possible, diarylprolinol silyl ethers (Cat) are the most widely used as simple and very efficient catalysts. They carry out the reactions, including Michael reactions of nitroalkanes between 2 and 3, in a highly efficient manner to yield products 4 with high optically purities (Y. Hayashi et al., 2005; Jensen et al., 2012; Reyes-Rodríguez et al., 2019) (Scheme 2). Once the key bond has been made, the others are followed by stereoselective reactions, with carbocyclic or heterocyclic ring transformations. The newly constructed bonds formed from organocatalysts are green, with specific examples indicated by the green-colored bonds in Scheme 2. In this manner, there are many reports showing that the organocatalytic reactions were very efficient ways of making the bonds needed in biologically important molecules, including pharmaceuticals and natural products such as acyclic and cyclic molecules. Some representative examples of organocatalyst proline derivatives, including diarylprolinol silyl ethers (Cat) are gabapentin (Gotoh et al., 2007), sacubitril (Hughes, 2018), prostaglandin PGF2α (Coulthard et al., 2012; Scheffler and Mahrwald, 2013), oseltamivir (Ishikawa et al., 2009; Ishikawa et al., 2010; Hayashi and Ogasawara, 2016), (+)-(α)-lycorane (Meng et al., 2014), (+)-microminutinin (Huang et al., 2017), and pyrrolysine (Han et al., 2013).
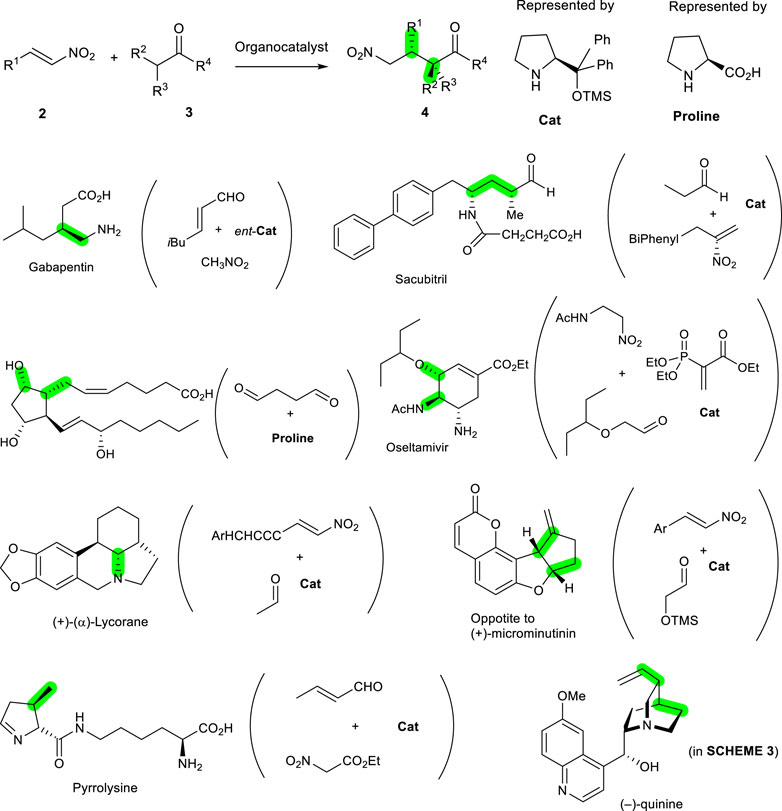
SCHEME 2. General synthetic scheme of bond formation and stereochemistry shown in green in adduct 4 based on organocatalytic nitro-Michael reactions from 2 and 3. The catalyst used was proline or its diarylprolinol silyl ethers (Cat) derivatives. The specific cases of initial organocatalytic reactions with starting materials and catalysts are shown in parenthesis on the right side of the examples, with the construction of green-colored bonds.
Recently, one outstanding publication that is worth looking into in detail deals with the two important aspects, pot-economical and organocatalytic synthesis, and was carried out by Professor Y. Hayashi’s lab. They performed a very impressive synthesis of a (−)-quinine (1) natural product consisting of core azaheterocycle tetrahydropyridine (Terunuma and Hayashi, 2022). The synthesis, with a piperidine ring needed for the preparation of unnatural enantiomer, was previously published with an organocatalyst via (3 + 3) cyclization and Strecker-type cyanation (Shiomi, et al., 2019). However, a strategically different method was used to obtain azaheterocycle tetrahydropyridine as a core ring of the natural enantiomer (−)-quinine. The addition of aldehyde 5 to 2-phenylnitroalkene (6) for an aza-Henry reaction was initiated in the presence of 20 mol% of diphenyl prolinol trimethylsilyl ether as a catalyst (Scheme 3). Moreover, their synthesis was in conjunction with the pot-economical manner, leading to several reactions in the same vessel without any extra procedures or work-up (Hayashi, 2016). The imine needed to make a tetrahydropiperidine ring from the initial adduct between 5 and 6 would enhance the synthesis of 9 in the same vessel for a reaction completed in one pot.
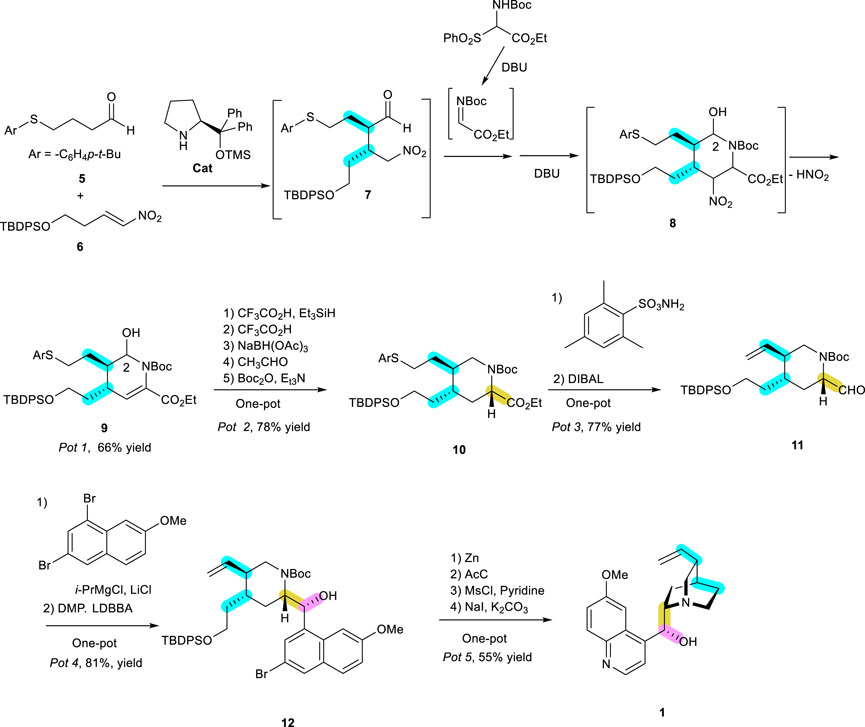
SCHEME 3. (−)-Quinine (1) synthesis reported by Professor Y. Hayashi’s lab (Terunuma and Hayashi, 2022) using an organocatalyst in a pot-economical manner as a key step in constructing the initial important bonds, highlighted in blue, followed by stereoselective reduction and aryl Grignard addition, as shown by the yellow and red bonds.
The initial acyclic adduct 7, as a Niro-Michael product, was reacted with imine generated from the corresponding sulfonyl precursor DBU (1,8-diazabicyclo [5.4.0]undec-7-ene, 3.0 equiv) in a single pot manner. This synthetic intermediate was converted, with the elimination of HNO2 in the presence of DBU as a base, to yield the cyclic product 9 in high yield (66%) and high e. e. (98%), with 1:1 at C2 d.e. The hydroxy substituent at C2 is eventually eliminated. All the reactions starting from 5, 6, and the iminocarboxylate to 9 proceed sequentially in the one-pot protocol. To succeed in this reaction sequence, all synthetic intermediates were kinetically and thermodynamically favorable to afford the next product without any hindrance from the previous reagents, as shown in Scheme 3. The best way to perform this synthetic strategy is for all necessary reactions to succeed in a single operation without changing the reagents and solvents. However, this is very rare, with the assumption that the same reagents under the same solvent can carry out different reactions in a certain direction. Except for rare cases, almost all the reactions require different reagents under different solvents. Therefore, the reaction should be well designed to perform the steps in a pot-economical manner. As a diastereomeric mixture, the initial crude cyclic compound 9 from Pot 1 proceeded further for dehydroxylative reduction by CF3CO2H and Et3SiH. Then, the reduction of enamine to amine was followed by the reducing agent NaBH(OAc)3. All the excessive reductive reagents were destroyed by the reaction with additional acetaldehyde. This second one-pot reaction (Pot 2) afforded the azaheterocycle 10 as an almost single stereoisomer, with the correct stereochemistry. In Pot 3, it is relatively straightforward to obtain olefin 11 with the release of aryl thiol according to the Matsuo protocol (Matsuo et al., 2006) with 2,4,6-trimethylphenylsulfonylamide. The substituent carboxy ester was then reduced by DIBAL under low temperatures to give rise to aldehyde 11. Then, 2-bromo-6-methoxyquinoline was added to this aldehyde, generating a diastereomeric mixture of hydroxy carbon without much stereoselectivity between re- or si face addition. However, this problem was overcome by oxidation of the alcohol adduct to ketone by DMP (Dess-Martin Periodinane), followed by the stereoselective reduction by LDBBA (lithium diisobutyl-tert-butoxyaluminum hydride), leading to the right isomer 12 needed for target 1 with more than 80% yield (Pot 4). The next steps in Pot 5 are the removal of bromine in the aryl ring and deprotecting the TBDPS and Boc groups from the free hydroxy group by treatment with Zn and AcCl. This primary hydroxy group was mesylated for the final cyclization with nitrogen to give quinuclidine under mild conditions using NaI and K2CO3. These four reactions are performed in a one-pot sequence with 77% yield. All these reactions are highly stereospecific, as seen in the synthesis of the expected (−)-quinine (1) in a pot economical manner. This report brings up two important and challenging synthetic methodologies in organic chemistry, i.e., the use of environmentally benign organocatalysts and pot economy.
This perspective focuses on the use of organocatalysts in a pot-economical manner for the recent synthesis of tetrahydropyridine-containing (−)-quinine. These synthetic methodologies may be applicable in laboratory preparation or in the industrial production of valuable organic compounds (Bernardi, et al., 2021). Most synthetic challenges are highly efficient and environmentally benign with organocatalysts in a pot-economical manner.
Data availability statement
The datasets presented in this article are not readily available because there are not any restrictions for readers to request. Requests to access the datasets should be directed to hjha@hufs.ac.kr.
Author contributions
H-JH: Conceptualization, Funding acquisition, Investigation, Project administration, Resources, Supervision, Validation, Visualization, Writing–original draft, Writing–review and editing.
Funding
The author(s) declare financial support was received for the research, authorship, and/or publication of this article. This work was supported by the National Research Foundation of Korea (SRC NRF 2021R1A5A6002803, Centre for New Directions in Organic Synthesis) and the HUFS fund 2024.
Conflict of interest
The author declares that the research was conducted in the absence of any commercial or financial relationships that could be construed as a potential conflict of interest.
Publisher’s note
All claims expressed in this article are solely those of the authors and do not necessarily represent those of their affiliated organizations, or those of the publisher, the editors and the reviewers. Any product that may be evaluated in this article, or claim that may be made by its manufacturer, is not guaranteed or endorsed by the publisher.
References
Albrecht, L., Albrecht, A., and Dell'Amico, L. (2022). Asymmetric organocatalysis: new Strategies, catalysts, and opportunities. Wiley VCH. ISBN-13, 978-3527349074.
Bernardi, L., Carlone, A., and Fini, F. (2021). Industrial relevance of asymmetric organocatalysis in the preparation of chiral amine derivatives. in The book “Methodologies in amine synthesis: challenges and Applications (A. Ricci, and L. Bernardi) Wiley VCH, Weinheim.
Bernhard, N., and Wolfgang, S. (1978). Simple method for the esterification of carboxylic acids. Angew. Chem. Int. Ed. 17, 522–524. doi:10.1002/anie.197805221
Boukis, A. C., Reiter, K., Frölich, M., Hofheinz, D., and Meier, M. A. (2018). Multicomponent reactions provide key molecules for secret communication. Nat. Commun. 9, 1–10. doi:10.1038/s41467-018-03784-x
Chen, Z., Yang, Q.-Q., Du, W., and Chen, Y. C. (2022). Asymmetric organocatalysis involving double activation. Tetrahedron Chem. 2, 100017. doi:10.1016/j.tchem.2022.100017
Coulthard, G., Erb, W., and Aggarwal, V. K. (2012). Stereocontrolled organocatalytic synthesis of prostaglandin PGF2a in seven steps. Nature 489, 278. doi:10.1038/nature11411
De Wolf, E., van Koten, G., and Deelman, B.-J. (1999). Fluorous phase separation techniques in catalysis. Chem. Soc. Rev., 37–41. doi:10.1039/A805644G
Earle, M. J., and Seddon, K. R. (2000). Ionic liquids. Green solvents for the future. Pure Appl. Chem. 72, 1391–1398. doi:10.1351/pac200072071391
Gotoh, H., Ishikawa, H., and Hayashi, Y. (2007). Diphenylprolinol silyl ether as catalyst of an asymmetric, catalytic, and direct Michael reaction of nitroalkanes with α,β-unsaturated Aldehydes. Org. Lett. 9, 5307. doi:10.1021/ol702545z
Grondal, C., Jeanty, M., and Enders, D. (2010). Organocatalytic cascade reactions as a new tool in total synthesis. Nat. Chem. 2, 167–178. doi:10.1038/nchem.539
Hajos, Z. G., and Parrish, D. R. (1974). Asymmetric synthesis of bicyclic intermediates of natural product chemistry. J. Org. Chem. 39, 1615–1621. doi:10.1021/jo00925a003
Han, B., He, X.-H., Liu, Y.-Q., He, G., Peng, C., and Li, J.-L. (2021). Asymmetric organocatalysis: an enabling technology for medicinal chemistry. Chem. Soc. Rev. 50, 1522–1586. doi:10.1039/D0CS00196A
Han, M.-Y., Wang, H.-Z., An, W.-K., Jia, J.-Y., Ma, B.-C., Zhang, Y., et al. (2013). A Concise synthesis of L-pyrrolysine. Chem. Eur. J. 19, 8078. doi:10.1002/chem.201300403
Hayashi, Y. (2016). Pot-economy and one-pot synthesis. Chem. Sci. 7, 866–880. doi:10.1039/C5SC02913A
Hayashi, Y., Gotoh, H., Hayashi, T., and Shoji, M. (2005). Diphenylprolinol silyl ethers as efficient organocatalysts for the asymmetric Michael reaction of aldehydes and nitroalkenes. Angew. Chem. Int. Ed. 49, 4212–4315. PMID 20175175. doi:10.1002/anie.200906900
Hayashi, Y., and Ogasawara, S. (2016). Time economical total synthesis of (−)-oseltamivir. Org. Lett. 18, 3426–3429. doi:10.1021/acs.orglett.6b01595
Huang, W.-L., Raja, A., Hong, B. C., and Lee, G. H. (2017). Organocatalytic enantioselective Michael−acetalization reduction Nef reaction for a one-pot entry to the functionalized aflatoxin system. total synthesis of (-)-dihydroaflatoxin D2 and (-) and (+)-microminutinin. Org. Lett. 19, 3494. doi:10.1021/acs.orglett.7b01473
Hughes, D. L. (2018). Asymmetric organocatalysis in drug development—highlights of recent patent lterature. Org. Process. Res. Dep. 22, 574–584. doi:10.1039/D0CS00196A
Ishikawa, H., Suzuki, T., and Hayashi, Y. (2009). High-yielding synthesis of the anti-influenza neuramidase inhibitor (−)-oseltamivir by three “one-pot” operations. Angew. Chem. Int. Ed. 48, 1304–1307. doi:10.1002/anie.200804883
Ishikawa, H., Suzuki, T., Orita, H., Uchimaru, T., and Hayashi, Y. (2010). High-yielding synthesis of the anti-influenza neuraminidase inhibitor (−)-oseltamivir by two “one-pot” sequences. Chem. Eur. J. 16, 12616–12626. doi:10.1002/chem.201001108
Jensen, K. L., Dickmeiss, G., Jiang, H., Albrecht, L., and Jørgensen, K. A. (2012). The diarylprolinol silyl ether system: a general organocatalyst. Acc. Chem. Res. 45, 248–264. doi:10.1021/ar200149w
List, B. (2007). Introduction: organocatalysis. Chem. Rev. 107 (12), 5413–5415. doi:10.1021/cr078412e
List, B. (2010). Emil Knoevenagel and the roots of aminocatalysis. Angew. Chem. Int. Ed. 49, 1730–1734. doi:10.1002/anie.200906900
MacMillan, D. W. C. (2008). The advent and development of organocatalysis. Nature 455, 304–308. doi:10.1038/nature07367
Martínez, J., Cortés, J. F., and Miranda, R. (2022). Green chemistry, metrics, A Review. Processes 10, 1274. doi:10.3390/pr10071274
Matsuo, J., Kozai, T., and Ishibashi, H. (2006). Mild preparation of alkenes from phenyl sulfides: one-pot elimination of phenylthio group via sulfilimine at ambient temperature. Org. Lett. 8, 6095–6098. doi:10.1021/ol062620w
Meng, X.-L., Liu, T., Sun, Z.-W., Wang, J.-C., Peng, F.-Z., and Shao, Z.-H. (2014). Asymmetric catalytic conjugate addition of acetaldehyde to nitrodienynes/nitroenynes: applications to the syntheses of (+)-α-lycorane and chiral β-alkynyl acid. Org. Lett. 16, 3044–3047. doi:10.1021/ol501158b
Mika, L. T., and Horváth, I. T. (2018). Fluorous Catalysis in the book green techniques for organic synthesis and medicinal chemistry, (W. Zhang, and B. W. Cue) John Wiley and Sons. Hoboken, USA.
Nafiu, S. A., Ajeebi, A. M., Alghamdi, H. S., Aziz, M. A., and Shaikh, M. N. (2023). Solid-supported catalysts for organic functional group transformations. Asian J. Org. Chem. 12, e202300051. doi:10.1002/ajoc.202300051
Pyser, J. B., Chakrabarty, S., Romero, E. O., and Narayan, A. R. H. (2021). State-of-the-art biocatalysis. ACS Cent. Sci. 7, 1105–1116. doi:10.1021/acscentsci.1c00273
Reyes-Rodríguez, G. J., Rezayee, N. M., Vidal-Albalat, A., and Jørgensen, K. A. (2019). Prevalence of diarylprolinol silyl ethers as catalysts in total synthesis and patents. Chem. Rev. 119, 4221–4260. doi:10.1021/acs.chemrev.8b00583
Ruiz-Lopez, M. F., Francisco, J. S., Martins-Costa, M. T. C., and Anglada, J. M. (2020). Molecular reactions at aqueous interfaces. Nat. Rev. Chem. 4, 459–475. doi:10.1038/s41570-020-0203-2
Scheffler, U., and Mahrwald, R. (2013). Recent advances in organocatalytic methods for asymmetric C-C bond formation. Chem. Eur. J. 19, 14346–14396. doi:10.1002/chem.201301996
Shiomi, S., Misaka, R., Kaneko, M., and Ishikawa, H. (2019). Enantioselective total synthesis of the unnatural enantiomer of quinine. Chem. Sci. 10, 9433. doi:10.1039/c9sc03879e
Terunuma, T., and Hayashi, Y. (2022). Organocatalyst-mediated five-pot synthesis of (–)-quinine. Nat. Commun. 13, 7503. doi:10.1038/s41467-022-34916-z
Vogel, P., Lam, Y.-H., Simon, A., and Houk, K. N. (2016). Organocatalysis: fundamentals and comparisons to metal and enzyme catalysis. Catalysts 6, 128. doi:10.3390/catal6090128
Keywords: organocatalyst, pot-economy, tetrahydropyridine, (−)-quinine, environmentally benign
Citation: Ha H-J (2023) Recent advances in synthesizing and utilizing nitrogen-containing heterocycles. Front. Chem. 11:1279418. doi: 10.3389/fchem.2023.1279418
Received: 18 August 2023; Accepted: 13 October 2023;
Published: 30 October 2023.
Edited by:
Florenci Vicent González, University of Jaume I, SpainReviewed by:
Praveen Reddy Adiyala, Indian Institute of Chemical Technology (CSIR), IndiaCopyright © 2023 Ha. This is an open-access article distributed under the terms of the Creative Commons Attribution License (CC BY). The use, distribution or reproduction in other forums is permitted, provided the original author(s) and the copyright owner(s) are credited and that the original publication in this journal is cited, in accordance with accepted academic practice. No use, distribution or reproduction is permitted which does not comply with these terms.
*Correspondence: Hyun-Joon Ha, hjha@hufs.ac.kr