- 1Department of Pharmaceutical Chemistry, Faculty of Pharmacy, The Islamia University of Bahawalpur, Bahawalpur, Pakistan
- 2Department of Clinical Pharmacy, College of Pharmacy, King Khalid University, Abha, Saudi Arabia
- 3Department of Pharmacognosy, College of Pharmacy, Prince Sattam bin Abdul Aziz, Al-Khar, Saudi Arabia
- 4Department of Pharmacognosy, Faculty of Pharmacy, Mansoura University, Mansoura, Egypt
- 5Department of Pharmaceutics, College of Pharmacy, King Khalid University, Abha, Saudi Arabia
Typha domingensis, a medicinal plant with significant traditional importance for curing various human diseases, has potentially bioactive compounds but was less explored previously. Therefore, this study aims to investigate the therapeutic potential of T. domingensis by evaluating the phytochemical profile through high-performance liquid chromatography (HPLC) techniques and its biological activities (in vitro and in vivo) from the methanolic extract derived from the entire plant (TDME). The secondary metabolite profile of TDME regulated by reverse phase ultra-high-performance liquid chromatography–mass spectrometry (RP-UHPLC–MS) revealed some bioactive compounds by -ve and +ve modes of ionization. The HPLC quantification study showed the precise quantity of polyphenols (p-coumaric acid, 207.47; gallic acid, 96.25; and kaempferol, 95.78 μg/g extract). The enzyme inhibition assays revealed the IC50 of TDME as 44.75 ± 0.51, 52.71 ± 0.01, and 67.19 ± 0.68 µgmL-1, which were significant compared to their respective standards (indomethacin, 18.03 ± 0.12; quercetin, 4.11 ± 0.01; and thiourea, 8.97 ± 0.11) for lipoxygenase, α-glucosidase, and urease, respectively. Safety was assessed by in vitro hemolysis (4.25% ± 0.16% compared to triton × 100, 93.51% ± 0.36%), which was further confirmed (up to 10 g/kg) by an in vivo model of rats. TDME demonstrated significant (p < 0.05) potential in analgesic activity by hot plate and tail immersion tests and anti-inflammatory activity by the carrageenan-induced hind paw edema model. Pain latency decreased significantly, and the anti-inflammatory effect increased in a dose-dependent way. Additionally, in silico molecular docking revealed that 1,3,4,5-tetracaffeoylquinic acid and formononetin 7-O-glucoside-6″-O-malonate possibly contribute to enzyme inhibitory activities due to their higher binding affinities compared to standard inhibitors. An in silico absorption, distribution, metabolism, excretion, and toxicological study also predicted the pharmacokinetics and safety of the chosen compounds identified from TDME. To sum up, it was shown that TDME contains bioactive chemicals and has strong biological activities. The current investigations on T. domingensis could be extended to explore its potential applications in nutraceutical industries and encourage the isolation of novel molecules with anti-inflammatory and analgesic effects.
1 Introduction
Natural products and their pharmaceutical compounds are used to treat a wide range of human illnesses. Despite advances in medical science over the last few decades, many major diseases remain difficult to cure (Singh, 2010). Medicinal plants have a wide range of bioactive qualities and have been used for therapeutic purposes since ancient times, and they continue to play an important role in modern medical study and practice (Sofowora et al., 2013). Cancer, bacterial infections, and immunological abnormalities are examples of these ailments, and over 25% of all prescribed medications in the world come from plants (Uddin et al., 2011). Chronic inflammatory diseases continue to be a major global health concern and are linked to many health conditions (Khan N. U. et al., 2022). The body’s natural and reversible defense mechanism against a variety of harmful substances, including toxins, microbial attacks, physical agents, and immunological responses, is inflammation. Inflammation that lasts too long or becomes uncontrolled can lead to serious illnesses (Chen et al., 2018; Khan K. et al., 2022).
The naturally occurring bioactive compounds including various phenolics and flavonoids occurring in medicinal plants were found to be promising inhibitors of several enzymes involved in human pathologies (Shahzad et al., 2022). Pain is a sensual modality that, under many circumstances, is the solitary indication used to diagnose a variety of disorders. Throughout history, numerous therapeutic methods have been used for pain relief, with the widespread use of medicinal plants standing out prominently (Uritu et al., 2018). Furthermore, in recent times, there has been a notable increase in the exploration of wild medicinal plants for their potential antidiabetic effects, and it was found that highly effective natural antidiabetic agents that were reported with phytochemicals exhibit several possible modes of action (Ahmed et al., 2022b). Urease inhibitors are also gaining importance due to their potential applications in gastric and urinary tract infections (UTIs). Gastric ulcers, urolithiasis, and UTIs are frequent ailments of this era that involve urease enzymes in their pathology (Nile et al., 2017).
Typha domingensis, commonly known as cattail, is a herbaceous, perennial wetland plant found all around the world (Beare and Zedler, 1987). There are 30 species of Typha in the family Typhaceae (Pandey and Verma, 2018). Turkish folk medicine uses female Typha species topically to stop bleeding (Akkol et al., 2011). Additionally, these were also used for healing wounds and burns. The pollen is astringent, diuretic, desiccant, hemostatic, and vulnerable. The leaves are diuretics. Typha domingensis also has nutritional value. Species of Typha such as Typha elephantine (Roxb), Typha angustifolia (Watt), and Typha latifolia (Edgew) are recognized as reservoirs of antimycobacterial agents (Rao et al., 2016). All parts of the Typha plant, predominantly the rhizomes, are edible for consumption. The presence of starch grains in grinding stones suggests that they were utilized as a food source in Europe a considerable time ago (Zeng et al., 2020). Because of their higher carbohydrate content than potatoes, rootstocks and rhizomes are consumed as a nutritious food source during the spring season. Furthermore, they have protein levels equivalent to maize and rice (Morton, 1975; Zeng et al., 2020). The root can likewise be dried, ground into a powder, and afterward added to cereal flours or utilized as a thickener in different dishes. This flour, which is high in protein, is used to make bread, cakes, biscuits, etc. (Aljazy et al., 2021).
The goal of this study is to investigate T. domingensis, as a medicinal plant, with a variety of useful features, including toxicology and central analgesic activity, as well as anti-inflammatory and enzymatic inhibition activities. This might open the door to the discovery of bioactive chemical substances without the negative side effects usually connected with synthetic drugs.
2 Materials and methods
2.1 Collection, identification, and preparation of the methanolic extract of T. domingensis
The mature plant as a whole was inspected and authorized by the herbarium of the Department of Botany, which is part of the Faculty of Life Science at the Islamia University of Bahawalpur in Pakistan. In March 2019, a specimen was then assigned the reference number 412 and cataloged in the herbarium.
After being collected, the plant was carefully cleaned before being spread out on a fresh sheet of paper to dry naturally for 20 days. The plant pieces were mechanically crushed into a powdery state after drying. Approximately 3 kg of the powdered substance was submerged in 80% methanol for 14 days while being stirred on occasion. To assure further purification, the subsequent solutions were first filtered through a muslin cloth and then through Whatman 1 filter paper having a pore size of 11 µm. By applying reduced pressure to a rotary evaporator (Heidolph Laborota 4,000, Schwabach, Germany), the filtrate that resulted was concentrated into a partially solid mass. A lyophilizer was then used to completely dry the concentrated solution. The semisolid mass was subsequently consolidated in the open air, producing 270 g of the finished product. The labeled T. domingensis specimens were properly preserved in an airtight container and set aside for future examination according to the previously reported procedures (Nazir et al., 2021).
2.2 Phytochemical analysis of TDME
2.2.1 Reverse phase ultra-high-performance liquid chromatography–mass spectrometry analysis of TDME
The Agilent 1,290 Infinity UHPLC system, which was coupled to the Agilent 6,520 Accurate-Mass Q-TOF mass spectrometer, was used in conjunction with the Agilent Eclipse XDB-C18 column, which had dimensions of 2.1 × 150 mm and a particle size of 3.5 m. Throughout the analysis, the column temperature was held constant at 25 °C, while the auto-sampler temperature was set and held at 4 °C [18]. Mobile phase A, which flowed at a rate of 0.5 mL/min and contained a 0.1% formic acid solution in water, was chosen for the analysis. This was chosen in preference to another mobile phase (mobile phase B), which was a 0.1% solution of formic acid in acetonitrile. The analysis was performed for 25 min after infusing 1.0 µL of plant extracts that had been diluted in methanol of high-performance liquid chromatography (HPLC) grade. A post-run time of 5 minutes was noticed after the run. Nitrogen gas was used to create nebulization at a start-up flow rate of 25 L per hour. This was followed by increasing the flow rate to 600 L per hour to facilitate drying at 350 °C. The fragmentation voltage was kept at 125 V, while the capillary voltage was set at 3500 V (Saleem et al., 2019). Similar to previous findings, RP-UHPLC–MS analyses were carried out to evaluate the polyphenolic composition of TDME. Agilent MassHunter Qualitative Analysis B.05.00 software was used to evaluate the data using the Metabolomics-2017–00004. m method. Utilizing particular criteria, such as a match tolerance of 5 ppm, the compounds were found using the exploration database METLIN_AM_PCDL-Ne 170502 cdb. Both negative ion modes like H- and positive ion modes like H+, Na+, and NH4+ were employed (Locatelli et al., 2017).
2.2.2 Polyphenolic profile by HPLC (quantitative analysis)
A Shimadzu HPLC system from Japan was used to quantify the sample extract (Al-Qahtani et al., 2023). To determine the phenolic profile, a dried sample weighing 0.1 g was combined with 1 mL of methanol. The sample was filtered through a 0.45 μm syringe filter and centrifuged at 6,000 g for 15 min. A 10 µL volume was then injected into the HPLC apparatus after that. The Shimadzu Shim-pack CLC-ODS C-18 column (with dimensions of 5 cm × 4.5 mm and a particle size of 5 m) was used to analyze the samples. The standards used in this analysis for the quantification of polyphenols are chlorogenic acid, p-coumaric acid, gallic acid, ƿ-hyydroxy benzoic acid, caffeic acid, vanillic acid, kaempferol, sinapic acid, ferulic acid, salicylic acid, coumarin, quercetin, benzoic acid, and rutin. The calculation of the k-factor was used. The limit of detection and limit of quantification of these compounds were in the range of 0.15–0.46 and 0.42–2.47 μg/mL, respectively. Glacial acetic acid (1% in water), which was solution A, and methanol B were combined in the mobile phase at the volumetric ratios of 90:10, 84:16, 72:28, 65:35, 50:50, and finally, to 90:10 (acetic acid:methanol) at time intervals of 0–5, 6–20, 21–35, 36–45, and 46–60 min, and the final concentration in the mobile phase was run for 61–75 min to restore the original conditions, before the new sample was injected. The flow rate was maintained at 0.8 mL/min, and the injection volume was 10 µL. An RS Diode UV–VIS matrix detector was used to detect the signals of chromatogram and operated in a wavelength range of 270–370 nm. The temperature of the column was maintained at 25 °C, and identification of peaks was performed by the comparison of peaks obtained by running the standard compounds under similar conditions. The similar procedure was also reported by Basit et al. (2023). The experiment was performed in triplicates, and the obtained chromatogram is shown in Figure 1.
2.3 In vitro biological investigation
2.3.1 Enzyme inhibition assays
2.3.1.1 Lipoxygenase inhibition activity
The anti-lipoxygenase action was evaluated using the same modification test techniques as previously published (Singsai et al., 2020). Lipoxygenase from soybean and linoleic acid (substrate) were used in their native forms as supplied by Sigma-Aldrich, and the extract’s analytic solution was prepared in methanol (1 mg/mL) and then diluted in different aliquots (0.05–1 mg/mL). Following the procedure indicated by Singsai et al., the reaction solution was created, and then, 1.0 mL of substrate was added (linoleic acid at a concentration of 0.6 mM) following the incubation process. At 234 nm, the absorbance was measured after thorough mixing. As a reference inhibitor of lipoxygenase, indomethacin at the concentration of 1 mg/mL in methanol (with dilutions as mentioned for the extract) was used to quantify the percentage of inhibition. The percentage of inhibition was calculated by comparing it to the inhibitory action of indomethacin, a well-known standard lipoxygenase inhibitor. Then, the percentage inhibition was determined using the following expression:
2.3.1.2 α-Glucosidase inhibition activity
The standard procedure was already established (Nisar et al., 2022) for the α-glucosidase inhibition activity, with a few minor adjustments. The extracts and the standard solutions were prepared in the concentration of 1 mg/mL along with different dilutions of 0.05–1 mg/mL. An α-glucosidase enzyme solution of 1U/mL in 50 mM phosphate buffer (pH 6.8), 0.5 mM para-nitrophenyl-β-D-glucopyranoside (pNPG) solution, and standard solution or positive control (acarbose) with different concentrations was prepared. The α-glucosidase enzyme (10 μL) was added to 96-well plates for the α-glucosidase inhibition experiment. The wells were formerly filled with 20 µL of the extracts or a reference solution and 50 µL of phosphate buffer at a pH of 6.8. After 15 min of incubation at 37 °C to stop the enzymatic activity, the mixture was tested for absorbance at a wavelength of 405 nm. The same response mixture received 20 µL of a 0.5 mM solution of para-nitrophenyl-D-glucopyranoside (pNPG). The mixture was allowed to sit at room temperature for 30 min. Using the provided formula, the final absorbance at 405 nm was measured to estimate the level of inhibition of the α-glucosidase enzyme. Methanol (20 μL) was added as the blank in place of the sample/standard.
2.3.1.3 Urease inhibition activity
The urease inhibition assay for TDME was appropriate with minor changes to an earlier described procedure (Nisar et al., 2022). The extract and standard (thiourea) solutions were prepared at a concentration of 1 mg/mL, and different dilutions were also prepared (0.05–1 mg/mL). In 1M phosphate buffer with a pH of 7.0, the urease enzyme was produced as a solution at a concentration of 0.025%. The produced enzyme solution was then poured (20 µL) into 96-well plates. Methanol (20 μL) was added as the blank in place of the sample/standard. The reaction mixture was then given a 60 µL addition of a 2.25% urea solution after the preceding procedures. The resulting mixture was then incubated for 15 min at a temperature of 37 °C. The mixture’s absorbance was then assessed at λ:630 nm. The reaction mixture was then given a 60 µL boost from the phenol reagent before receiving a 100 µL boost from the sodium hypochlorite solution. The resulting mixture was then incubated at a temperature of 37 °C for further 15 min. The reaction mixture’s absorbance was subsequently measured at a wavelength of 630 nm. The technique for the negative control was also performed as described previously, except that 20 µL of methanol was used in place of the extracts or standards. The following formula determines the inhibition of the urease enzyme. IC50 values were used to represent the results.
2.3.4 Hemolytic activity
The method outlined earlier was utilized to measure the hemolytic impact of the TDME. A total of 10 mL of human blood was obtained from volunteer participants and placed in a sterile EDTA tube with a screw top. The tube was then put in a centrifuge and spun for 5 min at an acceleration of 850×g. After removing the top layer, the erythrocytes were carefully washed with 10 mL of sterile, cooled, isotonic PBS (pH 7.4). The previously cleaned erythrocytes were then reconstituted in 20 mL of sterile, cold PBS. The extracts were then added to the erythrocyte solution at a concentration of 1,000 μg/mL, and the mixture was then incubated at 37 °C for 60 min. The absorbance of hemoglobin in the supernatant at 540 nm was measured to estimate the rate of hemolysis. The proportion of hemolysis was computed using the stated formula. Triton X-100 (0.1%) was used as the +ve control, and PBS was used as the -ve control (Tabassum et al., 2022).
2.3.5 In vitro anti-inflammatory activity
The technique of stabilizing the membrane of the human red blood cell was exploited in this study. Healthy human volunteers’ blood samples were combined with an equal amount of Alsever’s solution (5 mL blood sample and 5 mL Alsever’s solution). Alsever’s solution consists of 2% dextrose, 0.8% sodium citrate, 0.05% citric acid, and 0.42% sodium chloride. The mixture of blood sample and Alsever’s solution was formerly centrifuged at room temperature for 15 min at a speed of 3,000 rpm. These RBCs were washed with saline solution to eliminate impurities and to prepare a 10% suspension of RBCs. At the time of the experiment, different preparations were made: a control using distilled water and a standard solution of diclofenac sodium (at a conc. of 500 μg/mL and various concentrations of TDME at 500, 1,000, and 3,000 μg/mL). Distilled water, 1 mL of phosphate buffer, 2 mL of hyposaline (0.36%), and last, 0.5 mL of the red blood cell (RBC) suspension were added to each of these preparations in that order. The study followed the guidelines set in the Helsinki Declaration and received approval from the institutional ethical committee known as PREC. The assay combination was centrifuged for 20 min at a speed of 3,000 rpm following a 30-min incubation at 37 °C. At 560 nm, the supernatant solution’s hemoglobin content was calculated by spectrophotometry (Chirumamilla et al., 2022).
2.4 In vivo biological evaluation
2.4.1 Chemicals
All of the materials utilized in the study were highly pure and appropriate for scientific investigation. Sigma-Aldrich (United States) provided carrageenan, Novartis Pharma Ltd. provided diclofenac sodium, and Indus Pharma provided pentazocine (Javed et al., 2020).
2.4.2 Drugs
TDME (at dosages of 30, 100, and 300 mg/kg), pentazocine (at a dose of 10 mg/kg), diclofenac sodium, and indomethacin (at a dose of 15 mg/kg) were produced and dissolved in distilled water. These solutions were given intraperitoneally at a dosage of 5 mL/kg, acting as reference substances for assessing their anti-inflammatory and analgesic effects (Javed et al., 2020).
2.4.3 Experimental animals
The Wistar albino rats were housed and cared for in the animal facility of the Pharmacology & Physiology research laboratory in Pakistan (the Islamia University of Bahawalpur’s Faculty of Pharmacy and Alternative Medicine). The rats were both male and female, weighing between 140 and 225 g. In this investigation, polycarbonate cages with a maximum animal occupancy of six were used, measuring 47 × 34 × 18 cm3. Standard environmental parameters were upheld throughout the trial, including a temperature of 25°C ± 2 °C, humidity levels of 50%–55%, and a consistent 12-h cycle of light and darkness. Throughout the trial, the animals received standard animal feed and had full access to water. The experimental circumstances were kept up for a week before the trial began to reduce stress on the animals. Under the reference number PAEC22/74, the institution’s Pharmacy Research Ethics Committee (PREC) authorized the study’s protocols and procedures (Basit et al., 2022).
2.4.4 Acute toxicity assay
An acute toxicity experiment was used to evaluate the safety of the TDME, with a few minor adjustments made based on the previously published research (Lalitha et al., 2012). Five groups of Wistar albino rats, each with five rats, were constructed, having both males and females in each group. The rats were given plenty of food and drink before the trial began, and they also got used to the laboratory environment. The rats that had been fasting for 12 h were given TDME at increasing doses (0.3, 1, 3, and 10 g/kg). The intraperitoneal (i.p.) method was used to administer the extracts. The study also included a control group that received the normal saline solution (10 mL/kg) for comparison. For the first 12 h, the rat was monitored every 1 h and then daily for the next 14 days. The mortality rate was measured after 48 h, and various physical and behavioral signs were identified and closely tracked in the animals. Tremors, convulsions, salivation, perspiration, lacrimation (tear production), the writhing reflex, somatomotor activity, and any notable behavioral abnormalities were among the symptoms.
2.4.5 In vivo anti-inflammatory activity
A modified version of a previously established approach was used to examine the anti-inflammatory properties of TDME. For this assessment, the carrageenan-induced paw edema model was used (Basit et al., 2022). Thirty rats were taken. Six rats were included in each of the five groups from which the animals were separated. As a baseline measurement, the right hind paw of each rat was estimated with a screw gauge. The test drug, TDME, was given to separate groups of rats intraperitoneally (i.p.) at dosages of 30, 100, and 300 mg/kg. Distilled water was used as the administration method, and a dose of 5 mL/kg intraperitoneally was applied. Another group received a normal medication, indomethacin, at a dose of 15 mg/kg i. p. After giving the rats these drugs, 0.1 mL of a 1% carrageenan solution was injected into the plantar tissue of their right hind feet to cause edema. Before 1, 2, 3, and 4 h after the injection of carrageenan, the width of the paws was measured. The difference in paw thickness was calculated by deducting the first measurement (“0 h”) from the measurements collected at the following hourly intervals to calculate the growth in paw thickness (Vázquez et al., 1996).
2.4.6 Analgesic activity
The study used six groups of Wistar albino rats weighing between 150 and 200g and consisted of both males and females. Each group had six animals. The control group of rats received 5 mL/kg of distilled water intraperitoneally (i.p.). The control group obtained intraperitoneal (i.p.) injections of 15 mg/kg diclofenac sodium and 10 mg/kg pentazocine. The other groups received TDME intraperitoneally (i.p.) at dosages of 30, 100, and 300 mg/kg (Javed et al., 2020).
2.4.6.1 Hot plate test
The process mentioned previously was carried out with minor adjustments (Sajid-Ur-Rehman et al., 2021). The hot plate test was carried out at a persistent temperature of 52 °C. By examining the rats’ responses to thermal discomfort, such as paw licking or jumping, the latency period, or reaction time, was assessed in seconds. The reaction time was tested before administration (0 min), as well as 30, 60, 90, and 120 min after administration. A maximum duration limit of 25 s was set to prevent any damage to the paws.
2.4.6.2 Tail immersion test
A water heater was used as the instrument for the tail-flick test. The thermostat was adjusted to keep the water bath at a constant temperature of 53°C ± 2 °C. The bottom section of each rat’s tail was measured and plunged in warmed water, which remained at 53°C ± 2 °C. The rats reacted by pulling their tails out of the hot water. The initial latency was measured first, followed by the administration of the proper dosage to each group. The reaction time was then assessed at 30 min, 60 min, 90 min, and 120 min following intravenous injection. To avoid tissue injury, the maximum allowable duration for tail flick measurement was determined at 25 s (Sewell and Spencer, 1976).
2.5 In silico prediction studies
2.5.1 Molecular docking
Computer-aided drug design and the advancement in molecular biology both benefit from the use of molecular docking. For molecular recovery, a technique for producing compounds as PDB files and a targeted search database in a valid Protein Data Bank (PDB) format is required. For this reason, many technologies such as the AutoDock Vina program, MGL Tools, Discovery Studio, PyRx, and Babel can be used. The PDB was used to find the receptor molecule used for the investigation. The responsibility for additional receptor preparation fell to Discovery Studio (Discovery Studio 2021 client). All the compounds screened by LC–MS were downloaded from PubChem as SDF files (structured data format). To make ligand compounds, the Babel was employed. Then, Vina, which was integrated into PyRx, was given the receptors and ligands. Specific target proteins were selected for the in vitro or in vivo assays involving them. α-Glucosidase (1obb) and urease (3la4) were used to find the relationship of compounds with in vitro inhibition properties of TDME. Lipoxygenase (6ncf) and cyclooxygenase (6y3c.COX1 and 6bl4. COX2) proteins were selected to evaluate the effect of compounds on in vitro and in vivo anti-inflammatory, analgesic, and antipyretic activities of TDME. The incorporation of tiny protein molecules into a target requires a planned sample of the ligand’s ability to precisely conform to a certain target pattern to produce an ideal complex form. By using the program evaluation function, this may be done. Vina was applied for docking, and Discovery Studio was used to display the findings of interactions from the docking (Meng et al., 2011).
2.5.2 In silico absorption, distribution, metabolism, excretion, and toxicological characterization of compounds
On 28 June 2023, the absorption, distribution, metabolism, and excretion (ADME) features of bioactive compounds derived from reverse phase ultra-high-performance liquid chromatography–quadrupole time-of-flight mass spectrometry (RP-UHPLC–QTOF-MS) were evaluated by using SwissADME (https://www.swissadme.ch/) online tools, and these substances also underwent docking testing against several enzymes (Khan et al., 2023). Protox-II (https://tox-new.charite.de/) was used to evaluate the toxicity by following the reported procedure (Abdullahi et al., 2021).
2.6 Statistical analysis
The data detailed were given as the mean standard error of the mean (SEM) after three repetitions of each measurement. Following a one-way ANOVA, the LSD post hoc test was used for statistical analysis. The significance level of p ≤ 0.05 was used. Prism GraphPad-7 software was used to analyze the experimental data.
3 Results and discussion
3.1 Analysis and identification of secondary metabolites by RP-UHPLC–QTOF-MS
Furthermore, the methanolic extract of TDME was examined by using reverse phase ultra-high-performance liquid chromatography–mass spectrometry (RP-UHPLC–MS) to acquire comprehensive profiles of several secondary metabolites. To visualize the identified chemicals, standard total ion chromatograms with mass spectrometric peaks for both samples were prepared. This is the first time, according to our research, that this plant has been documented in such detail. Secondary metabolite profiles of TDME were regulated using RP-UHPLC–MS in both -ve and +ve modes. The -ve mode of ionization is shown in Table 1 and Figure 2, as well as Supplementary Figure S1 and Supplementary Figure S2, while the +ve mode is shown in Table 2 and Figure 3, as well as Supplementary Figure S3 and Supplementary Figure S4.
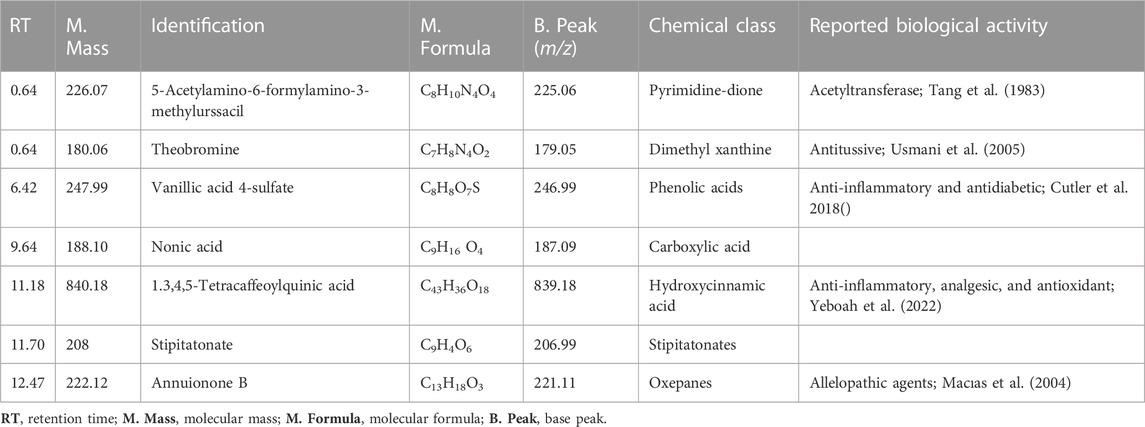
TABLE 1. The secondary metabolites from TDME were tentatively identified using UHPLC-Q–TOF-MS analysis in the -ve ionization mode.
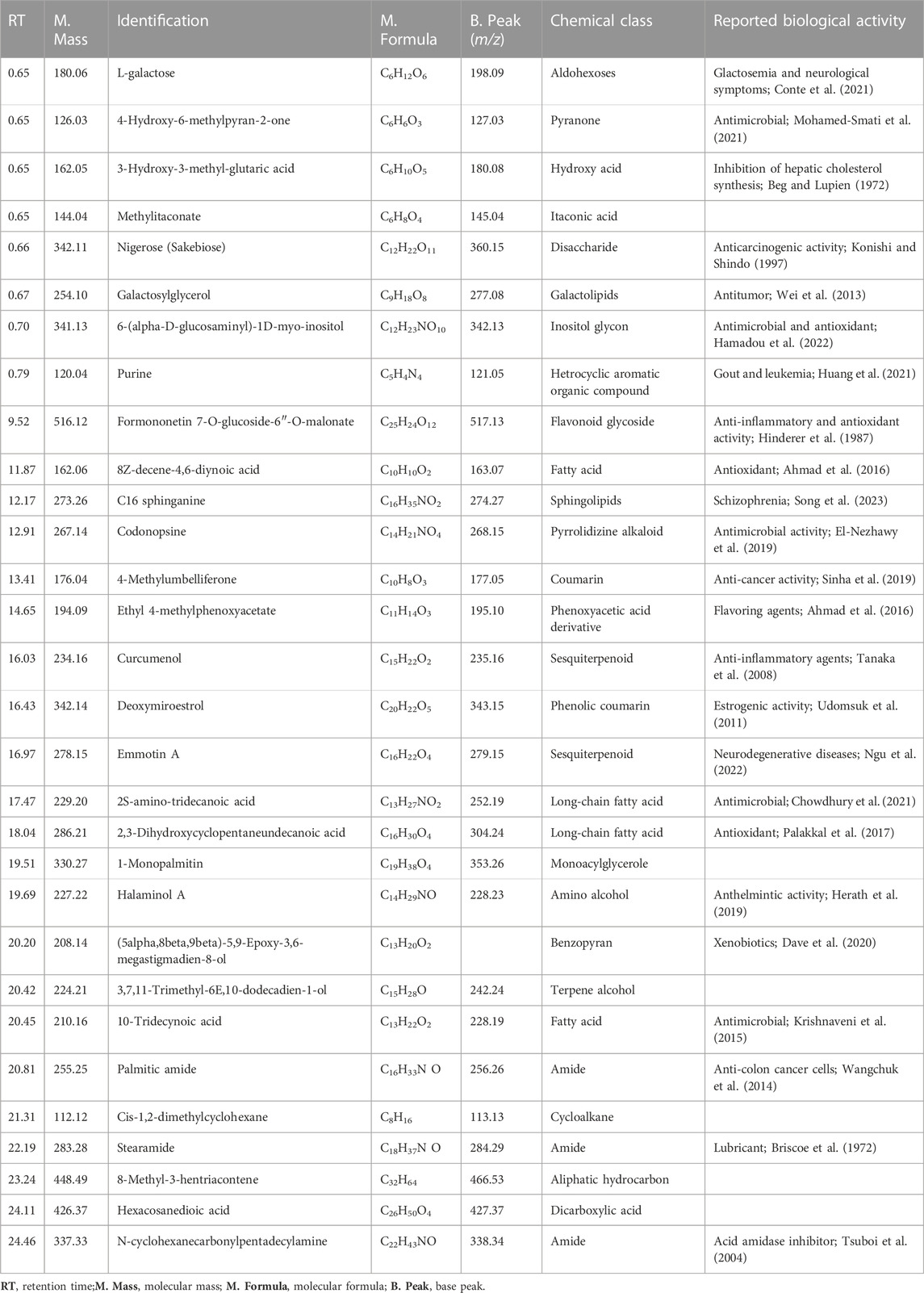
TABLE 2. The secondary metabolites from TDME were tentatively identified using UHPLC-Q–TOF-MS analysis in the +ve ionization mode.
Several compounds were revealed in different classes, e.g., pyrimidine-dione, dimethyl xanthine, phenolic acids, carboxylic acid, hydroxycinnamic acid, stipitatonates, oxepanes, aldohexoses, pyranone, hydroxy acid, itaconic acid, disaccharide, galactolipids, inositol glycon, fatty acid, pyrrolidizine alkaloid, coumarin, phenoxyaceticacid derivative, sesquiterpenoid, monoacylglycerole, benzopyran, and dicarboxylic acid. Previous studies on various parts and extracts also indicated the presence of several compounds belonging to the classes of terpenoids, fatty acids, esters, and steroids by GCMS analysis; moreover, the presence of polyphenols was also observed by spectroscopic methods (Dilshad et al., 2022; Khalid et al., 2022). The occurrence of phenolic compounds was further established in another study by Akhter et al. (2021). This evidence signifies our results, showing the existence of various bioactive phytochemicals from fatty acids, esters, steroids, alcohols, and polyphenolic compounds.
These classes of chemical compounds showed a lot of biological and medicinal activities, e.g., mildly diuretic, respiratory stimulant, mutagenic agents, anti-inflammatory, analgesic, antioxidant, anticancer activity, vitamin D3, inhibition of hepatic cholesterol synthesis, antitumor, antimicrobial, gout, leukemia, anti-obesity, type 2 diabetes, estrogenic activity, P-GP-inhibitor, anthelmintic activity, anti-colon cancer cells, lubricant, and acid amidase inhibitor which are described in Table 1 and Table 2.
3.2 Polyphenolics profile by HPLC (quantitative analysis)
We evaluated the material toward 14 regularly used reference standards during our investigation. As a consequence, three major compounds were quantified in the sample: p-coumaric acid, 207.47 μg/g; gallic acid, 96.25 μg/g; and kaempferol, 95.78 μg/g (Table 3). A previous study on the roots and leaves of T. domingensis was found compatible with our results in which the presence of p-coumarates and ferulates was reported (He et al., 2015). It was further substantiated by a study previously performed on an aqueous extract of flowers from this plant showing the presence of phenols, hydroxycinnamic acids, flavonoids, and pro-anthocyanidins (Chai et al., 2014).
Natural substances called polyphenols are widely present in the foods and plants we frequently eat (Shams ul Hassan et al., 2022). They are essential for several biological processes that take place in the seeds, leaves, roots, and other plant tissues. These include controlling enzyme activity, regulating protein synthesis, promoting photosynthesis, and maintaining the cytoskeleton’s structural integrity (Zhang et al., 2019). The powerful analytical method known as HPLC is utilized to identify and measure the chemical components in samples. It enables the exact and accurate measurement and identification of various chemicals. HPLC can also be used to separate and gather particular quantities of individual compounds by placing a fraction collector behind the detector’s flow cell, allowing their purification and collection (Javed et al., 2020).
Among the discovered polyphenols from TDME, p-coumaric acid belongs to the hydroxycinnamic acid group and is classified as a phenolic acid with anti-inflammatory, antidiabetic, antioxidant, anti-platelet, anti-ulcer, and anti-cancer characteristics (Ilavenil et al., 2016). Gallic acid is frequently found in antioxidant tea formulations and Ayurvedic plants. It has several health advantages, including antioxidant qualities, anti-inflammatory properties, and possibly anti-cancer potential (Aqeel et al., 2023). Gallic acid, a trihydroxy benzoic acid found in plant metabolites all over the world, is effective in the treatment of gastrointestinal, cognitive, metabolic, and cardiovascular problems. It can defend biological cells, tissues, and organs from damage brought on by oxidative stress because of its outstanding antioxidant and free radical scavenging activities (Kahkeshani et al., 2019).
A polyphenol antioxidant called kaempferol belongs to the flavonoids class and is found in medicinal plants, some fruits, and vegetables. Studies have repeatedly shown that eating foods high in kaempferol can lower the risk of getting chronic illnesses. The body’s antioxidant defenses are strengthened by kaempferol because it fights free radicals. Additionally, it influences crucial processes like metastasis, angiogenesis, inflammation, and apoptosis (Chen and Chen, 2013).
3.3 In vitro biological investigation
3.3.1 Enzyme inhibition activities (lipoxygenase, α-glucosidase, and urease)
The results of the current study showed good results of enzyme inhibitions (Table 4). The lipoxygenase (44.75 ± 0.51 µgmL-1), α-glucosidase (52.71 ± 0.01 µgmL-1), and urease (67.19 ± 0.68 µgmL-1) inhibition showed significant results of TDME, which were comparable to the standards used (indomethacin, 18.03 ± 0.12 µgmL-1; quercetin, 4.11 ± 0.01 µgmL-1; thiourea, 8.97 ± 0.11 µgmL-1). Our results were also in agreement with evidence by Sen et al. on lipoxygenase inhibition by the methanolic extract of another Typha species (Typha elephantina). Lipoxygenases (LOX) are enzymes that play an important part in the manufacture of leukotrienes, which are bioactive lipids. Their strength resides in their involvement in several physiological processes such as inflammation (Abbas et al., 2022), immune response control, and cell signaling, making them promising therapeutic targets in disorders such as asthma and other inflammatory ailments. Furthermore, the ability of lipoxygenases to catalyze lipid oxidation events increases their importance in lipid metabolism and overall cellular homeostasis (Sajid-ur-Rehman et al., 2023).
According to the previous study, T. domingensis is an excellent source of glucosidase inhibitors, iron chelators, and natural antioxidants. Oxidative stress is also a central consideration during the management of diabetes, and many bioactive phytochemicals including polyphenols are acknowledged as potent antioxidants (Ahmed et al., 2022b). The plant’s fruit extract is more effective as an anti-glucosidase and antioxidant than the male and female flower extract. In contrast, the female floral extract has the greatest ability to chelate iron among the three extracts. The phenolic content of the extract can be credited with both antioxidant and anti-glucosidase properties. Our research offers a molecular explanation for TDME’s capacity to treat diabetes and promote wound healing (Chai et al., 2014). This antioxidant potential is primarily due to the inhibition of pro-inflammatory mediator release, free radical neutralization, ROS, and RNS, which reduces lipid peroxidation and activates the cyclooxygenase pathway (Khatri et al., 2019). Additionally, they influence blood glucose levels by several methods, including limiting intestinal glucose absorption, promoting cell insulin production, and improving glucose uptake in insulin-sensitive tissue (Vinayagam et al., 2016).
Anti-urease drugs are now focusing on their potent anti-ulcer properties. Urease enzyme activity has been identified as a key pathogenic determinant in the etiology of several serious disorders that are harmful to human health, animals, and agriculture [67]. Sesquiterpene hydrocarbons or alcohols, or their synergistic action, may have very strong antibacterial urease activity. The primary defense against Helicobacter pylori may be due to urease inhibition, which prevents the bacteria from adhering to the gastric mucosa. The process of urease inhibition was discovered to be non-competitive, with both the substrate and inhibitor being incompetently attached to the enzyme.
3.3.2 Hemolytic activity to investigate the in vitro safety of TDME
Data on the hemolytic activity of the TDME are shown in Table 5. TDME has a hemolytic percentage value of 4.25 0.16%, and the cytotoxic positive control showed significantly high hemolysis (93.51% ± 0.36%). Previously, many methanolic extracts from plants were tested by the hemolytic activity to establish their possible safety, and our results fall in a similar range (Tabassum et al., 2023). The ability of some chemicals, such as toxins or enzymes, to cause the lysis or destruction of RBCs is referred to as hemolytic activity. This characteristic is useful in a variety of research and diagnostic applications, such as determining pathogen pathogenicity, researching the impact of certain substances on blood cells, and identifying specific medical problems associated with aberrant hemolysis. It can, however, be a negative component, creating health problems when detected in certain pathogenic agents or poisons. According to the reported methods, the hemolysis activity of less than 30% is considered safe and non-toxic for humans (Tabassum et al., 2022).
3.3.3 In vitro anti-inflammatory activity
Water-induced lysis of RBCs was significantly inhibited by TDME (Table 5). The results of percentage hemolysis inhibition showed a value of 39.16% ± 1.33%, compared to the standard inhibitor of inflammation (diclofenac sodium) in this study, which showed an inhibition value of 55.91% ± 1.78% at the dose of 1,000 μg/mL. It was reported that the denaturation of protein cells and tissue injury are reflected in good correlation with inflammatory diseases [28]. This finding demonstrates the anti-inflammatory action of TDME. Several compounds identified in the current study (Table 1; Table 2; Table 3) may be responsible for this activity.
3.4 In vivo biological investigation
3.4.1 Acute toxicity assay
The TDME was shown to be non-toxic up to a dosage of 10 g/kg in acute toxicity experiments on rates to assess the potential negative effects of increasing doses of TDME. No poisoning symptoms were noticed, and no deaths were reported over the 48-h observation period.
3.4.2 Anti-inflammatory activity (carrageenan-induced paw edema)
All dose levels of TDME showed anti-inflammatory effects (Figure 4 and Table 6). Notably, after carrageenan administration, significant anti-inflammatory effects from TDME were observed during the third and fourth hours. The 300 mg/kg dose had the strongest effect during the fourth hour of the doses examined. Carrageenan is a potent inflammatory agent used to induce edema. Various inflammatory mediators are involved in the development of carrageenan paw edema, and that has been extensively used to assess the anti-edema effects of natural products [69]. In the current study, various phytochemicals, especially polyphenols (Table 1; Table 2; Table 3), may be contributing to the reduction of inflammation. Moreover, several phytochemicals identified by HPLC–MS also have reported anti-inflammatory effects (Table 1; Table 2), which also shows a probability of their participation in declining inflammation.
3.4.3 Analgesic activity
3.4.3.1 Hot plate test
The hot plate test, like the tail-flick test, assesses the pain response in animals. Both the hot plate and tail immersion methods are commonly used to assess centrally acting analgesics [70]. Pentazocine and diclofenac sodium, standard drugs, substantially reduced pain latency at 30, 60, 90, and 120 min, whereas TDME at 30 mg/kg had no significant effect on pain reduction at any of the observation intervals (Figure 5 and Table 7). When compared to the control group, TDME at 100 and 300 mg/kg doses significantly reduced pain latency at all monitoring intervals (30, 60, 90, and 120 min) as compared to the control group.
3.4.3.2 Tail immersion test
An additional measure used to assess a substance’s analgesic potential is the tail immersion test. All three doses of TDME (30, 100, and 300 mg/kg) significantly decreased pain latency when compared to the control group (Figure 6 and Table 8). The tail immersion assay is a thermally based test used to assess a substance’s analgesic capabilities. This particular test has the advantage of not being sedation-affected, unlike other assays like the hot plate test, and is known for evaluating pain-related responses that are predominantly driven by spinal mechanisms. A unique sensitivity to partial agonists and narcotic agonists was seen in the tail immersion test performed on rats. In light of this, it is advised to use this test to assess the antinociceptive efficacy of partial agonists in small laboratory animals (Javed et al., 2020).
3.5 In silico prediction studies
3.5.1 Molecular docking
The crystal structures of α-glucosidase (PDB: 3WY1), urease (PDB: 1EJX), 5-lipoxygenase LOX (PDB: 6NCF), and cyclooxygenases (COX1, PDB: 6y3c and COX2, PDB: 1CX2) were obtained from https://www.rcsb.org/. The ligands were chosen from RP-UHPLC–MS, and their structures were downloaded from PubChem.
α-Glucosidase inhibitors are used for the treatment of type 2 diabetes to slow down the digestion and absorption of carbohydrates, helping to regulate blood sugar levels (Kashtoh and Baek, 2022). The ligands from RP-UHPLC–MS showed very good binding affinity against α-glucosidase. 1,3,4,5-Tetracaffeoylquinic acid (−9.2), deoxymiroestrol (−8.1), formononetin 7-O-glucoside-6″-O-malonate (−8.0), stipitatonate (−6.9), N-cyclohexanecarbonylpentadecylamine (−6.9), 4-methylumbelliferone (−6.8), nigerose (sakebiose) (−6.7), vanillic acid 4-sulfate (−6.5), emmotin A (−6.6), vanillic acid 4-sulfate (−6.5), nonic acid (−5.6), annuionone B (−5.4), L-galactose (−5.9), purine (−5.4), curcumenol (−6.4), theobromine (−5.7), ethyl 4-methylphenoxyacetate (−6.3), 3-eydroxy-3-methyl-glutaric acid (−5.7), and curcumenol (−6.4) are presented in Table 9. The higher negative values of the binding energy score represent the higher binding affinity of the ligand with the receptor molecule, which may be due to the chemical structures and interactions of compounds with amino acid residues (Ahmed et al., 2022a). The 2D interactions of α-glucosidase with ligands having the highest docking score are shown in Figure 7. The standard used for α-glucosidase was quercetin. The previous study also showed that the fruit and female flower extracts of T. domingensis are promising sources of glucosidase inhibitors (Chai et al., 2014).
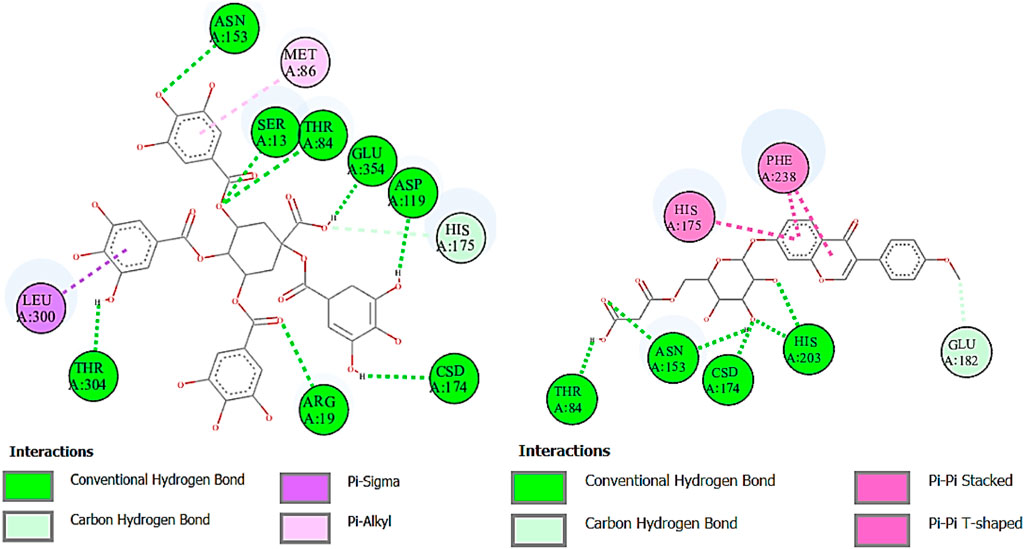
FIGURE 7. Two-dimensional interactions of 1,3,4,5-tetracaffeoylquinic acid (left) and formononetin 7-O-glucoside-6″-O-malonate (right) against α-glucosidase.
Urease is used to determine the H. pylori infection, a common cause of gastritis and gastrointestinal ulcers. In-depth research on diverse classes of urease inhibitors has been provoked by the need to treat such illnesses (Kosikowska and Berlicki, 2011). A lot of ligands showed the strongest binding affinity like formononetin 7-O-glucoside-6″-O-malonate (8.7), deoxymiroestrol (−8.1), 1.3,4,5-tetracaffeoylquinic acid (−7.7), stipitatonate (−6.7), 6-(alpha-D-glucosaminyl)-1D-myo-inositol (−6.7), vanillic acid 4-sulfate (−6.6), codonopsine (6.5), l5-acetylamino-6-formylamino-3-methylurssacil (−6.4), emmotin A (−6.4), nonic acid (−4.9), annuionone B (−6.0), L-galactose (−5.5), purine (−5.1), gurcumenol (−6.7), theobromine (−5.4), ethyl 4-methylphenoxyacetate (−5.8), 3-eydroxy-3-methyl-glutaric acid (−5.0), and nigerose (6.3), as presented in Table 9. The 2D interactions of ligands with the highest docking score against urease are presented in Figure 8.
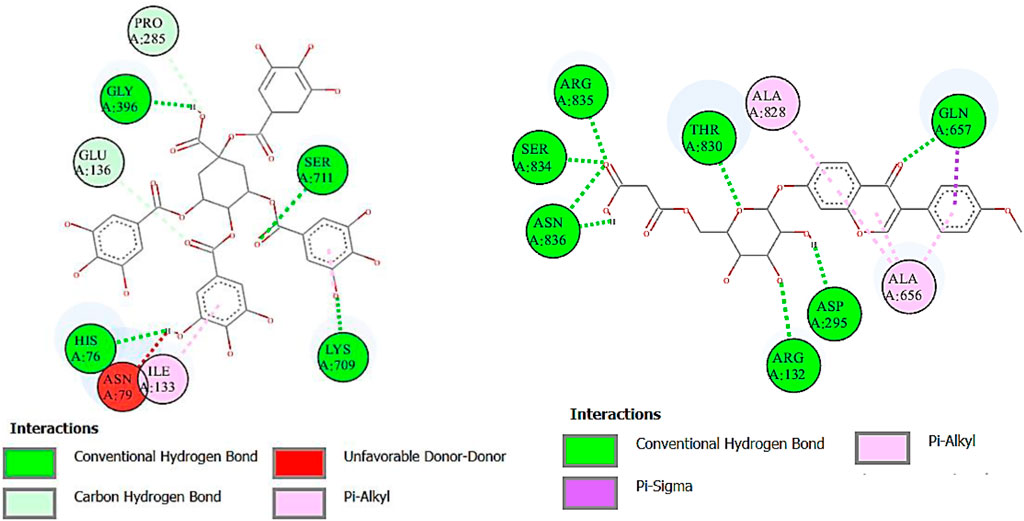
FIGURE 8. Two-dimensional interactions of 1,3,4,5-tetracaffeoylquinic acid (left) and formononetin 7-O-glucoside-6″-O-malonate (right) against urease.
Lipoxygenase (LOX) is an oxidoreductase enzyme that is found in both plants and animals. Its enzymatic action results in the formation of aromatic molecules, making it useful in the production of pleasant fragrances. Furthermore, LOX regulates the synthesis of volatile compounds, making it a great natural taste enhancer in the food preparation process (Steele et al., 2000). The binding affinity of deoxymiroestrol (−8.6), N-cyclohexanecarbonylpentadecylamine (−7.3), emmotin A (−7.0), and vanillic acid 4-sulfate (−7.4) showed good results. Indomethacin standard was used for LOX. Formononetin 7-O-glucoside-6″-O-malonate and 1,3,4,5-tetracaffeoylquinic acid showed better binding affinity as compared to the standard compound. The 2D interactions of ligands with the highest docking score against lipoxygenase are presented in Figure 9.
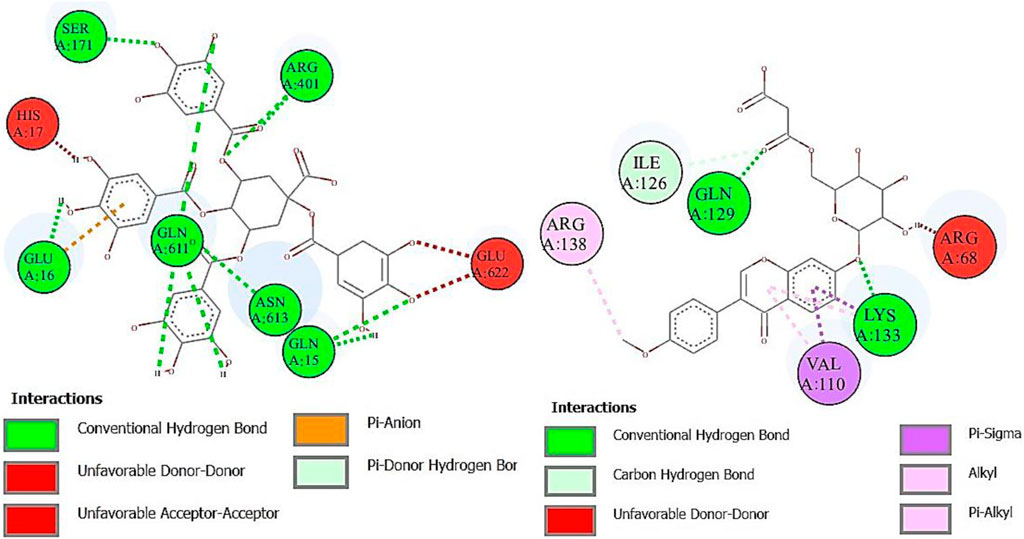
FIGURE 9. Two-dimensional interactions of 1,3,4,5-tetracaffeoylquinic acid (left) and formononetin 7-O-glucoside-6″-O-malonate (right) against lipoxygenase (5-LOX).
Cyclooxygenase COX-1 inhibitors are used for the reduction of inflammation and also anti-platelet properties. Cyclooxygenase COX-2 inhibitors are used to alleviate pain and inflammation while minimizing gastrointestinal side effects compared to traditional nonsteroidal anti-inflammatory drugs (NSAIDs) (Ahmad et al., 2018). The binding affinity of deoxymiroestrol (COX-1, -8.0 and COX-2, -8.7), N-cyclohexanecarbonylpentadecylamine (COX-1, -7.0 and COX-2, -8.3), emmotin A (COX-1, -7.4 and COX-2, -7.3), and vanillic acid 4-sulfate (COX-1, -6.5 and COX-2, -6.8) is shown. The standard used for cyclooxygenase was indomethacin. Many compounds showed results higher than standard. The 2D interactions of ligands with the highest docking score against COX-1 and COX-2 are presented in Figure 10 and Figure 11, respectively.
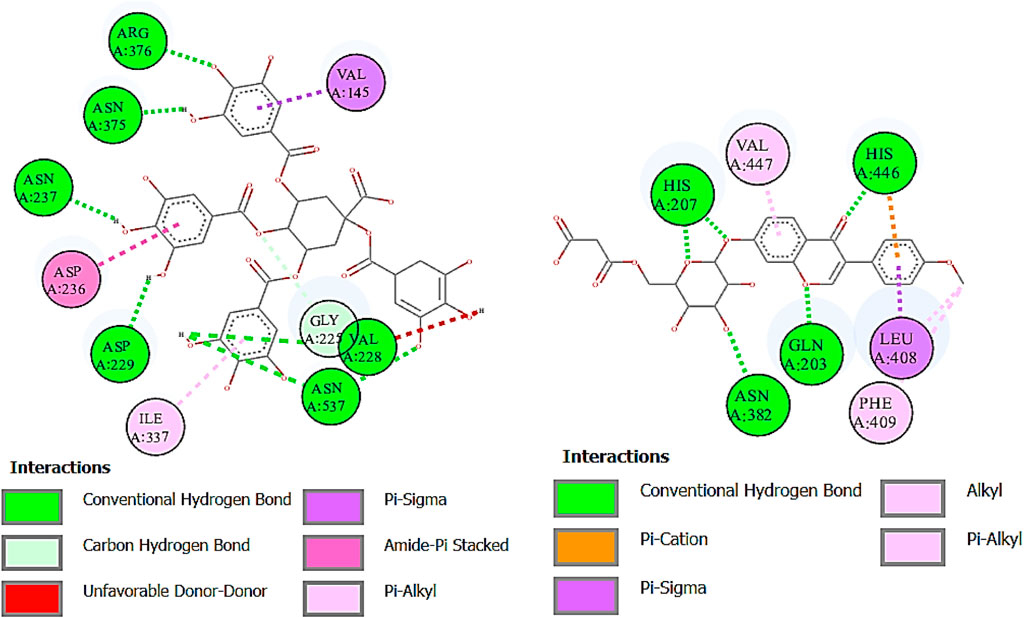
FIGURE 10. Two-dimensional interactions of 1,3,4,5-tetracaffeoylquinic acid (left) and formononetin 7-O-glucoside-6″-O-malonate (right) against cyclooxygenase-1 (COX-1).
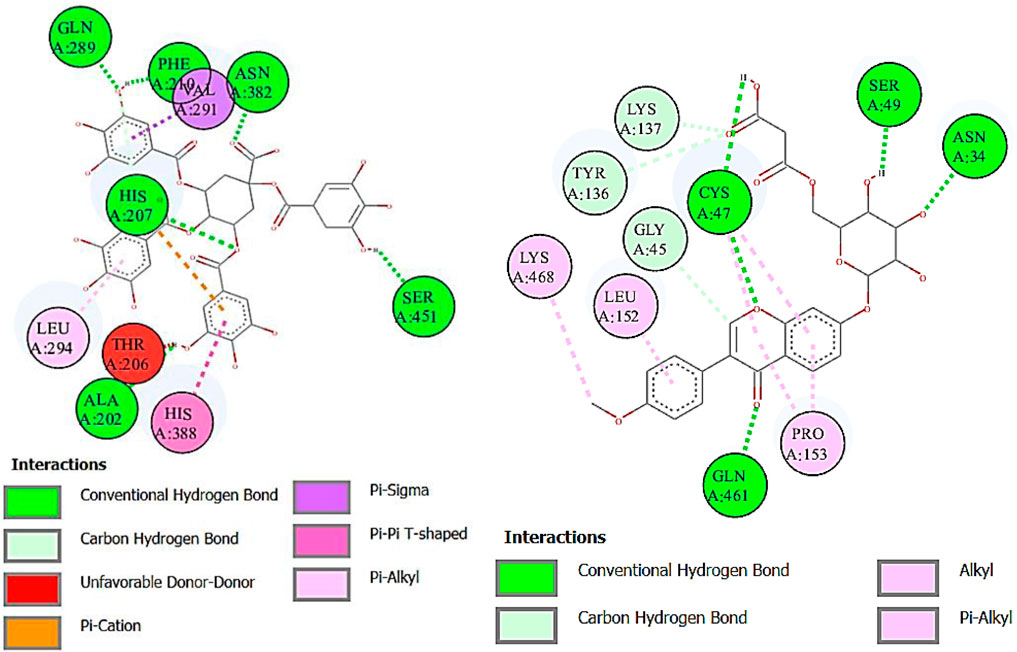
FIGURE 11. Two-dimensional interactions of 1,3,4,5-tetracaffeoylquinic acid (left) and formononetin 7-O-glucoside-6″-O-malonate (right) against cyclooxygenase-2 (COX-2).
3.5.2 In silico absorption, distribution, metabolism, excretion, and toxicological characterization of compounds
All dots showed within pink color which is near to target. So, this is called an optimized drug. Smaller TPSA values (usually below 140–160 Å2) are frequently chosen for oral bioavailability in drug development, for instance, as bigger TPSA values may result in decreased permeability through cell membranes. On the other hand, compounds designed to engage with particular targets or receptors may benefit from having higher TPSA values (over 80–100 Å2). Typically, the molar refractivity range is 0–100 cm³/mol. The range of consensus Log P o/w is between −3 and +6. The solubility range is < −10 < poorly < −6 < moderately < −4 < soluble < -2 very <0 < highly. Skin permeation range is −1.2 to +1.32. If any compound follows at least three rules of drug-likeness, it can act as an oral drug (Shahid et al., 2023). To determine pharmacokinetic drug features, such as absorption, distribution, metabolism, and excretion, the Lipinski rule makes use of certain physicochemical qualities. The product’s molecular weight should not exceed 500 Da. The number of atoms in its molecules should range between 20 and 70, with an average of 50. The polar surface area must be less than 140 Å2. Furthermore, the product should not contain more than five hydrogen bond donor sites and not surpass 10 hydrogen bond acceptor sites (Lipinski et al., 2012). The results of ADME and toxicological studies are presented in Table 10 and 11, respectively.
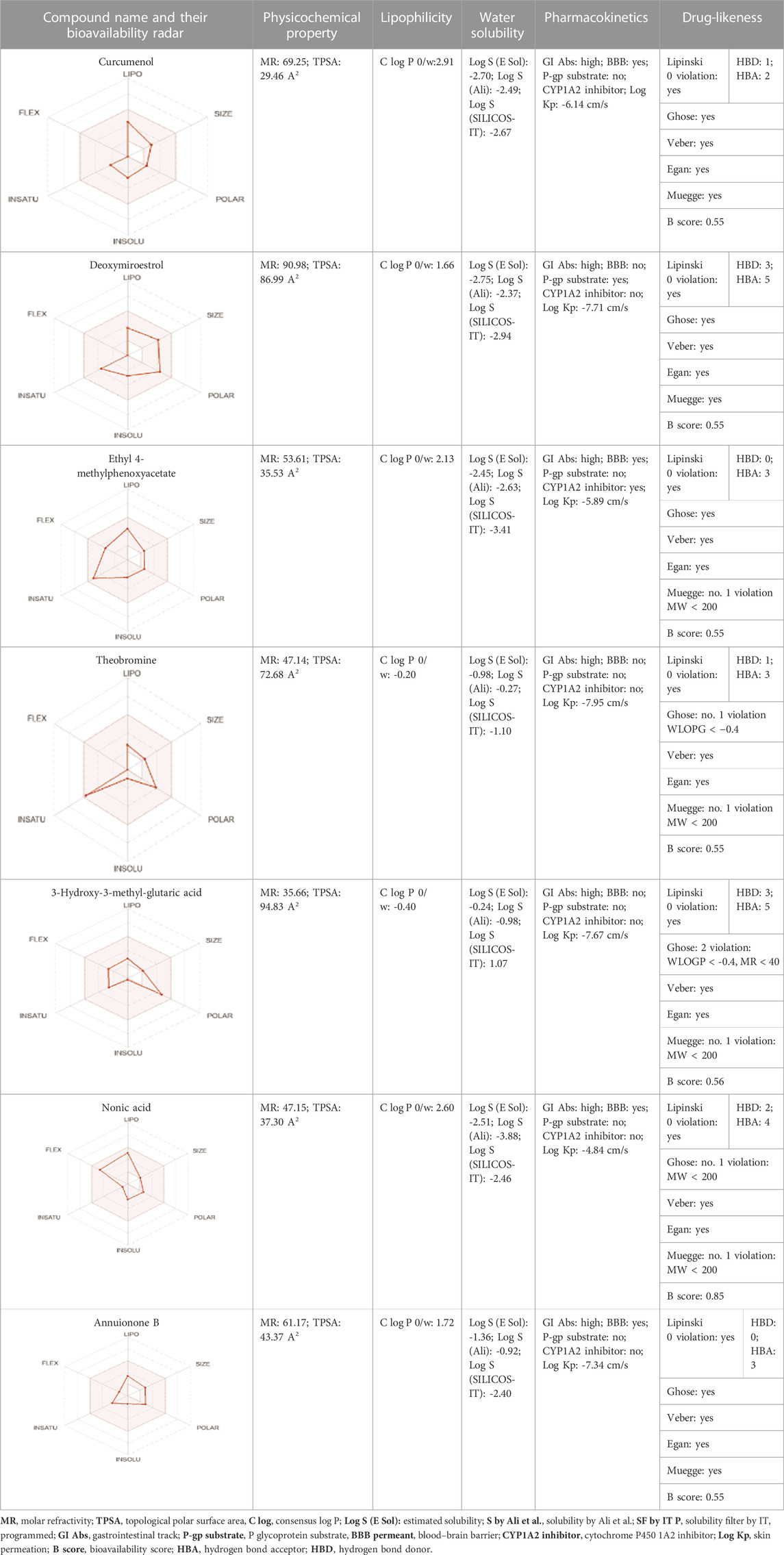
TABLE 10. Pharmacokinetics, bioavailability, structural parameters, Lipinski rule violation count, and solubility predictions of major compounds from TDME.
4 Conclusion
The study represents the chemical constitution and biological activities (in vitro, in vivo, and in silico) of the methanolic extract of T. domingensis (TDME). The result of RP-UHPLC–QTOF-MS screening revealed the presence of various classes of bioactive compounds. HPLC quantification offered a reliable and adaptable approach, enabling precise analysis and facilitating a diversity of academic and industrial applications. The inhibition of lipoxygenase, urease, and α-glucosidase offer considerable potential in the development of innovative treatment approaches for gastrointestinal diseases and metabolic disorders. The detected hemolytic activity of the TDME has disruptive effects on RBCs and suggests its importance in both research and therapeutic settings to validate the safety of the analyte, which was further confirmed by the in vivo acute toxicity method. Both in vitro and in vivo experiments showed significant analgesic and anti-inflammatory effects of TDME. Hence, this recent research provides support for the plant’s efficacy in alleviating pain and addressing inflammatory diseases. This research highlights the therapeutic potential of TDME, which could be further investigated for its applications in the nutraceutical and pharmaceutical industries.
Data availability statement
The original contributions presented in the study are included in the article/Supplementary Material; further inquiries can be directed to the corresponding authors.
Ethics statement
The animal study was approved by the Pharmacy Animal Ethics committee with reference no PAEC 20/30 issued by the Faculty of Pharmacy, IUB Punjab, Pakistan. This study was conducted in accordance with the local legislation and institutional requirements.
Author contributions
RD: methodology, writing–original draft, conceptualization, data curation, and investigation. K-u-RK: conceptualization, supervision, validation, writing–review and editing. SA: project administration, supervision, resources, and writing–review and editing. HR: data curation, formal analysis, validation, and writing–review and editing. MA: conceptualization, data curation, formal analysis, software, validation, writing–review and editing, and writing–original draft. MB: funding acquisition, investigation, and writing–review and editing.
Funding
The author(s) declare financial support was received for the research, authorship, and/or publication of this article. The authors extend their appreciation to the Deanship of Scientific Research at King Khalid University for funding this work through the Small group Research Project under grant number RGP1/196/44.
Acknowledgments
The authors extend their appreciation to the Deanship of Scientific Research at King Khalid University for funding this work through the Small group Research Project under grant number RGP1/196/44.
Conflict of interest
The authors declare that the research was conducted in the absence of any commercial or financial relationships that could be construed as a potential conflict of interest.
Publisher’s note
All claims expressed in this article are solely those of the authors and do not necessarily represent those of their affiliated organizations, or those of the publisher, the editors, and the reviewers. Any product that may be evaluated in this article, or claim that may be made by its manufacturer, is not guaranteed or endorsed by the publisher.
Supplementary material
The Supplementary Material for this article can be found online at: https://www.frontiersin.org/articles/10.3389/fchem.2023.1273191/full#supplementary-material
References
Abbas, S. Q., Muhammad, I., Wu, J.-J., Yan, S.-K., Ali, F., Majid, M., et al. (2022). Metals-triggered compound cdpdp exhibits anti-arthritic behavior by downregulating the inflammatory cytokines, and modulating the oxidative storm in mice models with extensive admet, docking and simulation studies. Front. Pharmacol. 13, 1053744. doi:10.3389/fphar.2022.1053744
Abdullahi, M., Das, N., Adeniji, S. E., Usman, A. K., and Sani, A. M. (2021). In-silico design and admet predictions of some new imidazo [1, 2-A] pyridine-3-carboxamides (ipas) as anti-tubercular agents. J. Of Clin. Tuberc. And Other Mycobact. Dis. 25, 100276. doi:10.1016/j.jctube.2021.100276
Ahmad, A., Abuzinadah, M. F., Alkreathy, H. M., Banaganapalli, B., and Mujeeb, M. (2018). Ursolic acid rich ocimum sanctum L leaf extract loaded nanostructured lipid carriers ameliorate adjuvant induced arthritis in rats by inhibition of cox-1, cox-2, tnf-Α and il-1: pharmacological and docking studies. Plos One 13, E0193451. doi:10.1371/journal.pone.0193451
Ahmad, S., Abdel-Salam, N. M., and Ullah, R. (2016). In vitro antimicrobial bioassays, dpph radical scavenging activity, and ftir spectroscopy analysis of Heliotropium bacciferum. Biomed Res. Int. 2016, 1–12. doi:10.1155/2016/3818945
Ahmed, M., Ahmad, S., Aati, H. Y., Sherif, A. E., Ashkan, M. F., Alrahimi, J., et al. (2022a). Phytochemical, antioxidant, enzyme inhibitory, thrombolytic, antibacterial, antiviral and in silico studies of Acacia jacquemontii leaves. Arabian J. Of Chem. 15, 104345. doi:10.1016/j.arabjc.2022.104345
Ahmed, M., Khan, K.-U.-R., Ahmad, S., Aati, H. Y., Ovatlarnporn, C., Rehman, M. S.-U., et al. (2022b). Comprehensive phytochemical profiling, biological activities, and molecular docking studies of pleurospermum candollei: an insight into potential for natural products development. Molecules 27, 4113. doi:10.3390/molecules27134113
Akhter, N., Aqeel, M., Hameed, M., Alhaithloul, H. A. S., Alghanem, S. M., Shahnaz, M. M., et al. (2021). Foliar architecture and physio-biochemical plasticity determines survival of Typha domingensis pers. Ecotypes in nickel and salt affected soil. Environ. Pollut. 286, 117316. doi:10.1016/j.envpol.2021.117316
Akkol, E. K., Süntar, I., Keles, H., and Yesilada, E. (2011). The potential role of female flowers inflorescence of Typha domingensis pers. In wound management. J. Of Ethnopharmacol. 133, 1027–1032. doi:10.1016/j.jep.2010.11.036
Al-Qahtani, J., Abbasi, A., Aati, H. Y., Al-Taweel, A., Al-Abdali, A., Aati, S., et al. (2023). Phytochemical, antimicrobial, antidiabetic, thrombolytic, anticancer activities, and in silico studies of Ficus palmata forssk. Arabian J. Of Chem. 16, 104455. doi:10.1016/j.arabjc.2022.104455
Aljazy, N. A. S., Abdulstar, A. R., and Alrakabi, J. M. F. (2021). Analytical study of phytochemicals and antioxidant activity of pollen (Typha domingensis pers.) extracted from the papyrus plant and its use in cake enrichment. Al-Qadisiyah J. Agric. Sci. 11, 126–136. doi:10.33794/qjas.2021.132392.1017
Aqeel, M. T., Rahman, N.-U., Khan, A.-U., Khan, M. T., Ashraf, Z., Ul Hassan, S. S., et al. (2023). Cardioprotective effect of 2-methoxy phenol derivatives against oxidative stress-induced vascular complications: an integrated in vitro, in silico, and in vivo investigation. Biomed. Pharmacother. 165, 115240. doi:10.1016/j.biopha.2023.115240
Basit, A., Ahmad, S., Naeem, A., Usman, M., Ahmed, I., Shahzad, M. N., et al. (2022). Chemical profiling of Justicia vahlii roth.(Acanthaceae) using uplc-qtof-ms and gc-ms analysis and evaluation of acute oral toxicity, antineuropathic and antioxidant activities. J. Of Ethnopharmacol. 287, 114942. doi:10.1016/j.jep.2021.114942
Basit, A., Ovatlarnporn, C., Rao, H., Ahmad, S., Sajomsang, W., Singkhonrat, J., et al. (2023). Protective effect of chemically characterized extract of viola stocksii boiss. Against breast cancer and vincristine induced neuropathic pain by alleviation of oxidative stress and inflammatory markers. Food Biosci. 56, 103058. doi:10.1016/j.fbio.2023.103058
Beare, P. A., and Zedler, J. B. (1987). Cattail invasion and persistence in A coastal salt marsh: the role of salinity reduction. Estuaries 10, 165–170. doi:10.2307/1352181
Beg, Z., and Lupien, P. (1972). In vitro and in vivo inhibition of hepatic cholesterol synthesis by 3-hydroxy-3-methylglutaric acid. Biochimica Biophysica Acta (Bba)-Lipids And Lipid Metabolism 260, 439–448. doi:10.1016/0005-2760(72)90059-8
Briscoe, B., Mustafaev, V., and Tabor, D. (1972). Lubrication of polythene by oleamide and stearamide. Wear 19, 399–414. doi:10.1016/0043-1648(72)90314-6
Chai, T., Mohan, M., Ong, H., and Wong, F. (2014). Antioxidant, Iron-chelating and Anti-glucosidase Activities of <i>Typha domingensis</i> Pers (Typhaceae). Trop. J. Of Pharm. Res. 13, 67–72. doi:10.4314/tjpr.v13i1.10
Chen, A. Y., and Chen, Y. C. (2013). A review of the dietary flavonoid, kaempferol on human health and cancer chemoprevention. Food Chem. 138, 2099–2107. doi:10.1016/j.foodchem.2012.11.139
Chen, L., Deng, H., Cui, H., Fang, J., Zuo, Z., Deng, J., et al. (2018). Inflammatory responses and inflammation-associated diseases in organs. Oncotarget 9, 7204–7218. doi:10.18632/oncotarget.23208
Chirumamilla, P., Vankudoth, S., Dharavath, S. B., Dasari, R., and Taduri, S. (2022). In vitro anti-inflammatory activity of green synthesized silver nanoparticles and leaf methanolic extract of solanum khasianum clarke. Proc. Of Natl. Acad. Of Sci. India Sect. B Biol. Sci. 92, 301–307. doi:10.1007/s40011-021-01337-9
Chowdhury, S. K., Dutta, T., Chattopadhyay, A. P., Ghosh, N. N., Chowdhury, S., and Mandal, V. (2021). Isolation of antimicrobial tridecanoic acid from Bacillus sp. Lbf-01 and its potentialization through silver nanoparticles synthesis: a combined experimental and theoretical studies. J. Of Nanostructure Chem. 11, 573–587. doi:10.1007/s40097-020-00385-3
Conte, F., Van Buuringen, N., Voermans, N. C., and Lefeber, D. J. (2021). Galactose in human metabolism, glycosylation and congenital metabolic diseases: time for A closer look. Biochimica Biophysica Acta (Bba)-General Subj. 1865, 129898. doi:10.1016/j.bbagen.2021.129898
Cutler, B. R., Gholami, S., Chua, J. S., Kuberan, B., and Babu, P. V. A. (2018). Blueberry metabolites restore cell surface glycosaminoglycans and attenuate endothelial inflammation in diabetic human aortic endothelial cells. Int. J. Of Cardiol. 261, 155–158. doi:10.1016/j.ijcard.2018.03.027
Dave, G. S., Rakholiya, K. D., Kaneria, M. J., Galvadiya, B. P., Vyas, S. R., Kanbi, V. H., et al. (2020). High affinity interaction of <i>Solanum tuberosum</i> and <i>Brassica juncea</i> residue smoke water compounds with proteins involved in coronavirus infection. Phytotherapy Res. 34, 3400–3410. doi:10.1002/ptr.6796
Dilshad, R., Ahmad, S., Aati, H. Y., Al-Qahtani, J. H., Sherif, A. E., Hussain, M., et al. (2022). Phytochemical profiling, in vitro biological activities, and in-silico molecular docking studies of Typha domingensis. Arabian J. Of Chem. 15, 104133. doi:10.1016/j.arabjc.2022.104133
El-Nezhawy, A. O., Alrobaian, M., Khames, A., El-Badawy, M. F., and Abdelwahab, S. F. (2019). Design and total synthesis of (-)-Codonopsinine,(-)-Codonopsine and codonopsinine analogues by O-(2-Oxopyrrolidin-5-Yl) trichloroacetimidate as amidoalkylating agent with improved antimicrobial activity via solid lipid nanoparticle formulations. Bioorg. Med. Chem. 27, 1263–1273. doi:10.1016/j.bmc.2019.02.021
Hamadou, W. S., Bouali, N., Badraoui, R., Hadj Lajimi, R., Hamdi, A., Alreshidi, M., et al. (2022). Chemical composition and the anticancer, antimicrobial, and antioxidant properties of Acacia honey from the hail region: the in vitro and in silico investigation. Evidence-Based Complementary And Altern. Med. 2022, 1–16. doi:10.1155/2022/1518511
He, D., Simoneit, B. R., Jara, B., and Jaffé, R. (2015). Gas chromatography mass spectrometry based profiling of Alkyl coumarates and ferulates in two species of Cattail (Typha domingensis P., and Typha latifolia L.). Phytochem. Lett. 13, 91–98. doi:10.1016/j.phytol.2015.05.010
Herath, H. D., Preston, S., Jabbar, A., Garcia-Bustos, J., Taki, A. C., Addison, R. S., et al. (2019). Identification of fromiamycalin and halaminol A from Australian marine sponge extracts with anthelmintic activity against Haemonchus contortus. Mar. Drugs 17, 598. doi:10.3390/md17110598
Hinderer, W., Köster, J., and Barz, W. (1987). Further characterization of chickpea isoflavone 7-O-Glucoside-6"-O-malonate: malonylesterase: evidence for A highly specific, membrane-bound enzyme in roots of cicer arietinum L. Z. Für Naturforsch. C 42, 251–257. doi:10.1515/znc-1987-0314
Huang, Z., Xie, N., Illes, P., Di Virgilio, F., Ulrich, H., Semyanov, A., et al. (2021). From purines to purinergic signalling: molecular functions and human diseases. Signal Transduct. And Target. Ther. 6, 162. doi:10.1038/s41392-021-00553-z
Ilavenil, S., Kim, D. H., Srigopalram, S., Arasu, M. V., Lee, K. D., Lee, J. C., et al. (2016). Potential application of P-coumaric acid on differentiation of C2c12 skeletal muscle and 3t3-L1 preadipocytes—an in vitro and in silico approach. Molecules 21, 997. doi:10.3390/molecules21080997
Javed, F., Jabeen, Q., Aslam, N., and Awan, A. M. (2020). Pharmacological evaluation of analgesic, anti-inflammatory and antipyretic activities of ethanolic extract of Indigofera argentea Burm. f. Burm. F. J. Of Ethnopharmacol. 259, 112966. doi:10.1016/j.jep.2020.112966
Kahkeshani, N., Farzaei, F., Fotouhi, M., Alavi, S. S., Bahramsoltani, R., Naseri, R., et al. (2019). Pharmacological effects of gallic acid in health and diseases: a mechanistic review. Iran. J. Of Basic Med. Sci. 22, 225–237. doi:10.22038/ijbms.2019.32806.7897
Kashtoh, H., and Baek, K.-H. (2022). Recent updates on phytoconstituent alpha-glucosidase inhibitors: an approach towards the treatment of type two diabetes. Plants 11, 2722. doi:10.3390/plants11202722
Khalid, A., Algarni, A. S., Homeida, H. E., Sultana, S., Javed, S. A., Abdalla, H., et al. (2022). Phytochemical, cytotoxic, and antimicrobial evaluation of tribulus terrestris L., Typha domingensis pers., and ricinus communis L.: scientific evidences for folkloric uses. Evidence-Based Complementary And Altern. Med. 2022, 1–11. doi:10.1155/2022/6519712
Khan, D. A., Shahid, A., Sherif, A. E., Aati, H. Y., Abdullah, M., Mehmood, K., et al. (2023). A detailed biochemical characterization, toxicological assessment and molecular docking studies of launaea fragilis: an important medicinal xero-halophyte. Saudi Pharm. J. 31, 1047–1060. doi:10.1016/j.jsps.2023.04.028
Khan, K., Alhar, M. S. O., Abbas, M. N., Abbas, S. Q., Kazi, M., Khan, S. A., et al. (2022a). Integrated bioinformatics-based subtractive genomics approach to decipher the therapeutic drug target and its possible intervention against brucellosis. Bioengineering 9, 633. doi:10.3390/bioengineering9110633
Khan, N. U., Qazi, N. G., Khan, A.-U., Ali, F., Hassan, S. S. U., and Bungau, S. (2022b). Anti-diabetic activity of brucine in streptozotocin-induced rats: in silico, in vitro, and in vivo studies. Acs Omega 7, 46358–46370. doi:10.1021/acsomega.2c04977
Khatri, S., Paramanya, A., and Ali, A. (2019). Phenolic acids and their health-promoting activity. Plant And Hum. Health 2. doi:10.1007/978-3-030-03344-6_27
Konishi, Y., and Shindo, K. (1997). Production of nigerose, nigerosyl glucose, and nigerosyl maltose by acremonium sp. S4g13. Biosci. Biotechnol. And Biochem. 61, 439–442. doi:10.1271/bbb.61.439
Kosikowska, P., and Berlicki, Ł. (2011). Urease inhibitors as potential drugs for gastric and urinary tract infections: a patent review. Expert Opin. Ther. Pat. 21, 945–957. doi:10.1517/13543776.2011.574615
Krishnaveni, M., Kalaivani, M., Banu, C. R., and Kumari, G. K. (2015). Gc-ms/ms study of parthenium hysterophorus L (N. Am) stem, antimicrobial activity. Res. J. Of Pharm. And Technol. 8, 517–519. doi:10.5958/0974-360x.2015.00086.4
Lalitha, P., Sripathi, S. K., and Jayanthi, P. (2012). Acute toxicity study of extracts of Eichhornia crassipes (mart.) solms. Asian J. Pharm. Clin. Res. 5, 59–61.
Lipinski, C. A., Lombardo, F., Dominy, B. W., and Feeney, P. J. (2012). Experimental and computational approaches to estimate solubility and permeability in drug discovery and development settings. Adv. Drug Deliv. Rev. 64, 4–17. doi:10.1016/j.addr.2012.09.019
Locatelli, M., Zengin, G., Uysal, A., Carradori, S., De Luca, E., Bellagamba, G., et al. (2017). Multicomponent pattern and biological activities of seven asphodeline taxa: potential sources of natural-functional ingredients for bioactive formulations. J. Of Enzyme Inhibition And Med. Chem. 32, 60–67. doi:10.1080/14756366.2016.1235041
Macıas, F. A., Lopez, A., Varela, R. M., Torres, A., and Molinillo, J. M. (2004). Bioactive apocarotenoids annuionones F and G: structural revision of annuionones A, B and E. Phytochemistry 65, 3057–3063. doi:10.1016/j.phytochem.2004.08.048
Meng, X.-Y., Zhang, H.-X., Mezei, M., and Cui, M. (2011). Molecular docking: a powerful approach for structure-based drug discovery. Curr. Computer-Aided Drug Des. 7, 146–157. doi:10.2174/157340911795677602
Mohamed-Smati, S. B., Faraj, F. L., Becheker, I., Berredjem, H., Le Bideau, F., Hamdi, M., et al. (2021). Synthesis, characterization and antimicrobial activity of some new azo dyes derived from 4-hydroxy-6-methyl-2h-pyran-2-one and its dihydro derivative. Dyes And Pigments 188, 109073. doi:10.1016/j.dyepig.2020.109073
Morton, J. F. (1975). Cattails (Typha spp.)—weed problem or potential crop? Econ. Bot. 29, 7–29. doi:10.1007/bf02861252
Nazir, N., Nisar, M., Zahoor, M., Uddin, F., Ullah, S., Ullah, R., et al. (2021). Phytochemical analysis, in vitro anticholinesterase, antioxidant activity and in vivo nootropic effect of Ferula ammoniacum (dorema ammoniacum) D. Don. In scopolamine-induced memory impairment in mice. Brain Sci. 11, 259. doi:10.3390/brainsci11020259
Ngu, E.-L., Tan, C.-Y., Lai, N. J.-Y., Wong, K.-H., Lim, S.-H., Ming, L. C., et al. (2022). Spirulina platensis suppressed inos and proinflammatory cytokines in lipopolysaccharide-induced Bv2 microglia. Metabolites 12, 1147. doi:10.3390/metabo12111147
Nile, S. H., Nile, A. S., Keum, Y. S., and Sharma, K. (2017). Utilization of quercetin and quercetin glycosides from onion (allium cepa L.) solid waste as an antioxidant, urease and xanthine oxidase inhibitors. Food Chem. 235, 119–126. doi:10.1016/j.foodchem.2017.05.043
Nisar, R., Ahmad, S., Khan, K.-U.-R., Sherif, A. E., Alasmari, F., Almuqati, A. F., et al. (2022). Metabolic profiling by gc-ms, in vitro biological potential, and in silico molecular docking studies of verbena officinalis. Molecules 27, 6685. doi:10.3390/molecules27196685
Palakkal, L., Hukuman, N. Z., and Mullappally, J. (2017). Antioxidant activities and chemical composition of various crude extracts of lepidagathis keralensis. J. Of Appl. Pharm. Sci. 7, 182–189. doi:10.7324/JAPS.2017.70626
Pandey, A., and Verma, R. (2018). Taxonomical and pharmacological status of Typha: a review. Ann. Of Plant Sci. 7, 2101–2106. doi:10.21746/aps.2018.7.3.2
Rao, M. R. K., Saranya, Y., Divya, D., and Linn, A. (2016). Preliminary phytochemical analysis of Typha domingensis rhizome aqueous extracts. Int. J. Pharm. Sci. Rev. Res. 37, 30–32.
Sajid-Ur-Rehman, M., Ishtiaq, S., Aati, H. Y., Sherif, A. E., Abbas Khan, M., Hussain, M., et al. (2023). Antiarthritic potential of the butanol fraction of Sesuvium sesuvioides: an in vitro, in vivo, and in silico evaluation. Front. Pharmacol. 14, 1136459. doi:10.3389/fphar.2023.1136459
Sajid-Ur-Rehman, M., Ishtiaq, S., Khan, M. A., Alshamrani, M., Younus, M., Shaheen, G., et al. (2021). Phytochemical profiling, in vitro and in vivo anti-inflammatory, analgesic and antipyretic potential of Sesuvium sesuvioides (fenzl) verdc.(aizoaceae). Inflammopharmacology 29, 789–800. doi:10.1007/s10787-021-00824-9
Saleem, H., Htar, T. T., Naidu, R., Nawawi, N. S., Ahmad, I., Ashraf, M., et al. (2019). Biological, chemical and toxicological perspectives on aerial and roots of filago germanica (L.) huds: functional approaches for novel phyto-pharmaceuticals. Food And Chem. Toxicol. 123, 363–373. doi:10.1016/j.fct.2018.11.016
Sewell, R., and Spencer, P. (1976). Antinociceptive activity of narcotic agonist and partial agonist analgesics and other agents in the tail-immersion test in mice and rats. Neuropharmacology 15, 683–688. doi:10.1016/0028-3908(76)90037-x
Shahid, A., Rao, H., Aati, H. Y., Sherif, A. E., Khan, D. A., Basit, A., et al. (2023). Phytochemical profiling of the ethanolic extract of zaleya pentandra L. Jaffery and its biological activities by in-vitro assays and in-silico molecular docking. Appl. Sci. 13, 584. doi:10.3390/app13010584
Shahzad, M. N., Ahmad, S., Tousif, M. I., Ahmad, I., Rao, H., Ahmad, B., et al. (2022). Profiling of phytochemicals from aerial parts of Terminalia neotaliala using lc-esi-ms2 and determination of antioxidant and enzyme inhibition activities. Plos One 17, E0266094. doi:10.1371/journal.pone.0266094
Shams Ul Hassan, S., Abbas, S. Q., Hassan, M., and Jin, H.-Z. (2022). Computational exploration of anti-cancer potential of guaiane dimers from xylopia vielana by targeting B-raf kinase using chemo-informatics, molecular docking, and Md simulation studies. Anti-Cancer Agents Med. Chem. Former. Curr. Med. Chemistry-Anti-Cancer Agents) 22, 731–746. doi:10.2174/1871520621666211013115500
Singh, A. R. (2010). Modern medicine: towards prevention, cure, well-being and longevity. Mens. Sana Monogr. 8, 17. doi:10.4103/0973-1229.58817
Singsai, K., Charoongchit, P., Chaikaew, W., Boonma, N., Fhanjaksai, P., and Chaisatan, K. (2020). Antilipoxygenase and anti-inflammatory activities of streblus asper leaf extract on xylene-induced ear edema in mice. Adv. Pharmacol. And Pharm. Sci. 2020, 1–5. doi:10.1155/2020/3176391
Sinha, M., Kaur, B., Kaur, A., Kuletha, S., Singh, K., and Bhatia, R. (2019). Anticancer activity of aminoacid linked novel 4-methylumbelliferone derivatives. Curr. Bioact. Compd. 15, 51–62. doi:10.2174/1573407213666170210143503
Sofowora, A., Ogunbodede, E., and Onayade, A. (2013). The role and place of medicinal plants in the strategies for disease prevention. Afr. J. Of Traditional, Complementary And Altern. Med. 10, 210–229. doi:10.4314/ajtcam.v10i5.2
Song, M., Liu, Y., Zhou, J., Shi, H., Su, X., Shao, M., et al. (2023). Potential plasma biomarker panels identification for the diagnosis of first-episode schizophrenia and monitoring antipsychotic monotherapy with the use of Metabolomics analyses. Psychiatry Res. 321, 115070. doi:10.1016/j.psychres.2023.115070
Steele, V. E., Holmes, C. A., Hawk, E. T., Kopelovich, L., Lubet, R. A., Crowell, J. A., et al. (2000). Potential use of lipoxygenase inhibitors for cancer chemoprevention. Expert Opin. Investigational Drugs 9, 2121–2138. doi:10.1517/13543784.9.9.2121
Tabassum, S., Ahmad, S., Ali, B., Usman, F., Jabeen, Q., Sajid-Ur-Rehman, M., et al. (2023). Chemical profiling and evaluation of toxicological, antioxidant, anti-inflammatory, anti-nociceptive and tyrosinase inhibitory potential of portulacaria afra using in-vitro, in-vivo and in-silico studies. Arabian J. Of Chem. 16, 104784. doi:10.1016/j.arabjc.2023.104784
Tabassum, S., Ahmad, S., Rehman Khan, K. U., Tabassum, F., Khursheed, A., Zaman, Q. U., et al. (2022). Phytochemical profiling, antioxidant, anti-inflammatory, thrombolytic, hemolytic activity in vitro and in silico potential of portulacaria afra. Molecules 27, 2377. doi:10.3390/molecules27082377
Tanaka, K., Kuba, Y., Ina, A., Watanabe, H., and Komatsu, K. (2008). Prediction of cyclooxygenase inhibitory activity of curcuma rhizome from chromatograms by multivariate analysis. Chem. And Pharm. Bull. 56, 936–940. doi:10.1248/cpb.56.936
Tang, B., Grant, D., and Kalow, W. (1983). Isolation and identification of 5-acetylamino-6-formylamino-3-methyluracil as A major metabolite of caffeine in man. Drug Metabolism And Dispos. 11, 218–220.
Tsuboi, K., Hilligsmann, C., Vandevoorde, S., Lambert, D. M., and Ueda, N. (2004). N-cyclohexanecarbonylpentadecylamine: a selective inhibitor of the acid amidase hydrolysing N-acylethanolamines, as A tool to distinguish acid amidase from fatty acid amide hydrolase. Biochem. J. 379, 99–106. doi:10.1042/bj20031695
Uddin, S. J., Grice, I. D., and Tiralongo, E. (2011). Cytotoxic effects of Bangladeshi medicinal plant extracts. Evidence-Based Complementary And Altern. Med. 2011, 1–7. doi:10.1093/ecam/nep111
Udomsuk, L., Juengwatanatrakul, T., Putalun, W., and Jarukamjorn, K. (2011). Down regulation of gene related sex hormone synthesis pathway in mouse testes by miroestrol and deoxymiroestrol. Fitoterapia 82, 1185–1189. doi:10.1016/j.fitote.2011.08.005
Uritu, C. M., Mihai, C. T., Stanciu, G.-D., Dodi, G., Alexa-Stratulat, T., Luca, A., et al. (2018). Medicinal plants of the family lamiaceae in pain therapy: a review. Pain Res. And Manag. 2018, 1–44. doi:10.1155/2018/7801543
Usmani, O. S., Belvisi, M. G., Patel, H. J., Crispino, N., Birrell, M. A., Korbonits, M., et al. (2005). Theobromine inhibits sensory nerve activation and cough. Faseb J. 19, 1–16. doi:10.1096/fj.04-1990fje
Vázquez, B., Avila, G., Segura, D., and Escalante, B. (1996). Antiinflammatory activity of extracts from aloe vera gel. J. Of Ethnopharmacol. 55, 69–75. doi:10.1016/s0378-8741(96)01476-6
Vinayagam, R., Jayachandran, M., and Xu, B. (2016). Antidiabetic effects of simple phenolic acids: a comprehensive review. Phytotherapy Res. 30, 184–199. doi:10.1002/ptr.5528
Wangchuk, P., Pyne, S. G., Keller, P. A., Taweechotipatr, M., and Kamchonwongpaisan, S. (2014). Phenylpropanoids and furanocoumarins as antibacterial and antimalarial constituents of the Bhutanese medicinal plant pleurospermum amabile. Nat. Product. Commun. 9, 1934578X1400900. doi:10.1177/1934578x1400900719
Wei, W., Qi, D., Zhao, H.-Z., Lu, Z.-X., Lv, F., and Bie, X. (2013). Synthesis and characterisation of galactosyl glycerol by Β-galactosidase catalysed reverse hydrolysis of galactose and glycerol. Food Chem. 141, 3085–3092. doi:10.1016/j.foodchem.2013.05.145
Yeboah, G. N., Owusu, F. W. A., Archer, M.-A., Kyene, M. O., Kumadoh, D., Ayertey, F., et al. (2022). Bridelia ferruginea benth.; an ethnomedicinal, phytochemical, pharmacological and toxicological review. Heliyon 8, E10366. doi:10.1016/j.heliyon.2022.e10366
Zeng, G., Wu, Z., Cao, W., Wang, Y., Deng, X., and Zhou, Y. (2020). Identification of anti-nociceptive constituents from the pollen of Typha angustifolia L. Using effect-directed fractionation. Nat. Prod. Res. 34, 1041–1045. doi:10.1080/14786419.2018.1539979
Keywords: T. domingensis extract, phytochemical profiling by UHPLC–MS, phenolic quantification by HPLC, in vitro biological activities, in vivo anti-inflammatory and analgesic, in silico docking and ADMET predictions
Citation: Dilshad R, Khan K-u-R, Ahmad S, Shaik Mohammad AA, Sherif AE, Rao H, Ahmad M, Ghalloo BA and Begum MY (2023) Phytochemical characterization of Typha domingensis and the assessment of therapeutic potential using in vitro and in vivo biological activities and in silico studies. Front. Chem. 11:1273191. doi: 10.3389/fchem.2023.1273191
Received: 05 August 2023; Accepted: 09 October 2023;
Published: 08 November 2023.
Edited by:
Syed Shams ul Hassan, Shanghai Jiao Tong University, ChinaReviewed by:
Maqsood Rehman, University of Malakand, PakistanJiajia Wu, Shanghai Jiao Tong University, China
Copyright © 2023 Dilshad, Khan, Ahmad, Shaik Mohammad, Sherif, Rao, Ahmad, Ghalloo and Begum. This is an open-access article distributed under the terms of the Creative Commons Attribution License (CC BY). The use, distribution or reproduction in other forums is permitted, provided the original author(s) and the copyright owner(s) are credited and that the original publication in this journal is cited, in accordance with accepted academic practice. No use, distribution or reproduction is permitted which does not comply with these terms.
*Correspondence: Kashif-ur-Rehman Khan, a2FzaGlmdXIucmFobWFuQGl1Yi5lZHUucGs=; M. Yasmin Begum, eWJhamVuQGtrdS5lZHUuc2E=