- 1Department of Chemistry, School of Science, Kwansei Gakuin University, Sanda, Japan
- 2JST-PRESTO, Chiyoda, Japan
Herein we report a copper-catalyzed synthesis of imidazolidine by employing the reaction of aziridine with imine. The reaction smoothly provided a diverse range of 2-substituted imidazolidines with high compatibility with various functional groups. Moreover, during our investigation, we discovered that isocyanate also reacted with aziridine to yield substituted imidazolidinones efficiently. The versatility of these reactions was further demonstrated by their application in the synthesis of hybrid molecules derived from two pharmaceutical compounds. This approach opens new possibilities for the discovery of novel classes of bioactive molecules.
1 Introduction
The construction of saturated nitrogen-containing heterocycles is of utmost importance as they are prevalent structures in a wide range of natural products and pharmaceuticals (Smith et al., 1999; Antonopoulos et al., 2008). Among such saturated azaheterocycles, the synthesis of substituted imidazolidines has received comparatively less attention than other mono-azaheterocycles, such as pyrrolidines. However, given that imidazolidine scaffolds have been identified in numerous bioactive molecules, the development of new synthetic methodologies for accessing these scaffolds is highly important (Figure 1A).
To access imidazolidine derivatives, aziridines have been widely employed as the starting materials (Figure 1B). Aziridines can be categorized into three types based on their reactivity: i) activated dipolar aziridines (Wu and Zhang, 2012; Shi et al., 2021), ii) activated vinyl aziridines (Lin et al., 2018; Spielmann et al., 2018), and iii) other substituted aziridines (Liu et al., 2017; Sengoden et al., 2017; Li et al., 2018; Tu et al., 2019; Pradhan et al., 2020). For type i), Shi et al. reported in 2021 the reaction of donor-accepter-type aziridines with 1,3,5-triazinanes through a [3 + 2] cycloaddition (Shi et al., 2021). This reaction demonstrated that aziridines can serve as efficient precursors for the synthesis of multi-substituted imidazolidines. Regarding type ii), Spielmann et al. reported the palladium-catalyzed [3 + 2] cycloaddition reaction of vinylaziridines with cyclic imines (Spielmann et al., 2018). The reaction proceeds through the formation of π-allylpalladium intermediate, which then reacts with the cyclic imine to yield the corresponding products. This approach allows for the diastereoselective synthesis of imidazolidine-fused tricyclic molecules. For type iii), Sengoden et al. reported copper-catalyzed reaction of aziridine with N-alkylanilines (Sengoden et al., 2017). This reaction involves the oxidation of aniline derivatives to generate an iminium intermediate in situ, which allows the efficient preparation of N-aryl-N-sulfonylimidazolidines. Despite the availability of various methods for accessing imidazolidines from aziridines, copper-catalyzed reactions of aziridines with imines or isocyanates have remained relatively underdeveloped (Zhu and Chiba, 2016; Bozorov et al., 2019; Pal et al., 2020; Pineschi, 2020; Zhang et al., 2022).
In this context, our previous study demonstrated the reaction of aziridine with diazetidine (Figure 1C), which led to the formation of imidazolidines (Higuchi et al., 2023). While the scope of this reaction was broad to construct various imidazolidines, the synthetic limitation associated with diazetidine hindered its widespread use for accessing imidazolidine derivatives. During our mechanistic investigation, we disclosed that an in situ formed imine reacted with aziridine to form the corresponding imidazolidine. This discovery motivated us to explore the reaction of aziridines with substituted imines to expand the scope of this transformation. Herein, we report the copper-catalyzed synthesis of imidazolidines through the reaction of aziridines with imines (Figure 1D). This transformation exhibits good functional group compatibility to afford the desired products efficiently. Additionally, we have successfully demonstrated that the use of isocyanates instead of imines allows accessing imidazolidinones. We have shown its application in the synthesis of hybrid molecules derived from Celecoxib and NLRP3 inflammasome inhibitor.
2 Results and discussions
We initiated our study by investigating the ligand effect for the reaction. The treatment of aziridine 1a with imine 2a in the presence of 10 mol% of CuBr and L1 in toluene at 120 °C for 20 h afforded the corresponding imidazolidine 3a in 72% isolated yield (Figure 2A). It is noteworthy that the ligand effect is critical where 6% of 3a was obtained without the addition of L1. Furthermore, CuBr was found to be essential for the reaction, and no product was observed in the absence of both CuBr and L1. We then investigated the effect of ligands (Figure 2B). Although no clear correlation between ligand structure and catalytic activity was observed, 1,10-phenanthroline derivatives showed good results (1H NMR yields using various ligands L2: 74% L3: 49%, L4: 43%). Similarly, 2,2-bipyridine derivatives also promoted the reaction (L5–L8: 14%–72% 1H NMR yields.). After the screening, we decided to use L1 as the optimal ligand because it provided the highest isolated yield.
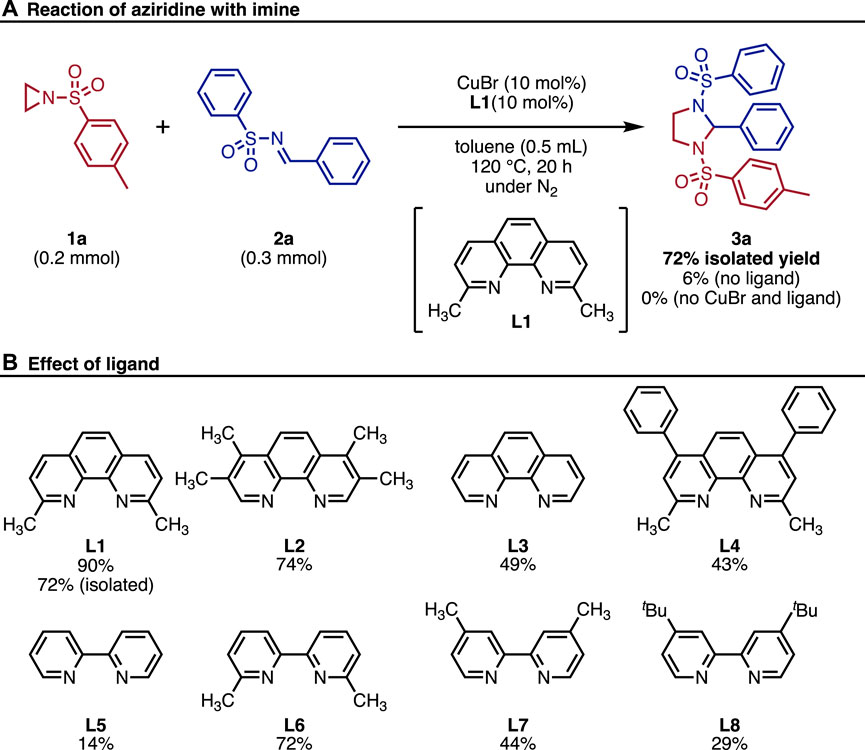
FIGURE 2. (A) Reaction of aziridine with imine. (B) Effect of ligand. 1H NMR yields were shown using 1,1,2,2-tetrachloroethane.
The scope of the reaction was investigated (Figure 3). Initially, we examined the substituent effect on imines. The use of electron-withdrawing p-trifluoromethylphenyl group at the R1 position allowed an efficient transformation to give 3b in 80% yield. Other electron-withdrawing groups, such as p-bromo- or p-chlorophenyl groups, provided the corresponding products 3c and 3d in 88% and 84% yields, respectively. It is noteworthy that such halogen moieties can serve as footholds for further transformation. The reactions of electron-donating p-methyl- or p-methoxyphenyl-substituted imines were relatively sluggish, however, both starting materials were completely consumed. Not only p-substituted one but also m-substituted phenylsulfonylimine reacted smoothly converted to 3g in 81% yield. Despite the bulky o-bromo-, or o-nitrophenyl groups, the corresponding products 3h and 3i were obtained in moderate to high yields. Although the yield was not high, imine 2j derived from cinnamaldehyde, was applicable to the reaction to afford 3j in 34% yield. A variety of sulfonyl groups were tolerated under the reaction conditions, where methylsulfonyl (Ms) or o-nitrophenylsulfonyl (Ns)-substituted imines provided 3k and 3l in 84% and 67% yields without the loss of these sulfonyl groups. When electron-donating group-substituted arylsulfonylaziridines were employed, the reactions were less effective and gave 3m and 3n in 25% and 49% yields, respectively. Furthermore, the reaction was applicable to cyclic imine to give 3o in almost quantitative yield. Unsuccessful combinations of substrates are summarized at the bottom of Figure 3. The reactions of N-phenylimine or N-sulfoxyimine with 1a failed to give the corresponding products. Although the reaction of unsubstituted aziridine 1a with imine 2a smoothly provided the products, the reaction of diphenylmethanimine or substituted aziridine failed. This can be attributed to steric hindrance, as the reaction of substituted aziridine proceeded smoothly with methanimine derivatives (Higuchi et al., 2023).
To expand the scope of the reaction, we employed isocyanate 4 as the coupling partner (Figure 4). The reaction of tosylaziridine 1a with phenylisocyanate 4a under the same optimized conditions afforded imidazolidinone 5a in 74% yield. A variety of arylisocyanate was applicable to the reaction where electron-withdrawing p-acetyl-, p-chloro-, or electron-donating p-methoxyphenylisocyanate gave products 5b–5d in 60%–83% yields. When tosylisocyanate was employed as the substrate, product 5e was obtained in 64% yield. We then investigated the scope of substituted aziridines. Compared with the reaction with imine, the reaction with isocyanate showed a broader substrate scope of aziridines. The reaction of vinylaziridine afforded 5f in 43% yield, and no isolable byproduct that reacted with the olefin was observed, leaving less than 20% of vinylaziridine remaining. Other substituents, such as methoxycarbonyl, benzyl, or biphenyl groups, were applicable to the reaction to give 5g–5i in 36%–56% yields. Bicyclic aziridine 1h, which can be prepared from cyclohexene, reacted to give 5j in 22% yield. Although the yield was not high, the formal cis-diamination product can be obtained through this transformation (Minakata et al., 2021).
A proposed mechanism is shown in Figure 5. The reaction initiates with the coordination of aziridine 1 to copper catalyst A to give the corresponding intermediate B (or B′). Subsequently, imine 2 attacks to open the aziridine ring to give intermediate C (or C’). Finally, a cyclization reaction takes place from C to give product 3. From the result of the competitive experiment, the electron-donating group might reduce the reactivity of intermediate C, which hampers the cyclization and eventually the turnover of the catalyst. Note that another reaction mechanism can be depicted (see Supplementary Figure S1). At this stage, we considered that the reaction with isocyanate also proceeded through a similar reaction mechanism. Because it is difficult to elucidate the full reaction mechanism, further investigation is ongoing.
Finally, we applied the developed reaction to synthesize pharmaceutical hybrids (Figure 6). Firstly, we synthesized aziridine 1i from Celecoxib (Ozaki et al., 2021). Aziridine 1i was then reacted with imine 2m, derived from an NLRP3 inflammasome inhibitor, to give 3p in 51% yield. Furthermore, the reaction of aziridine 1i with bis-isocyanate 4f afforded product 5k in 95% yield. These applications clearly demonstrate the excellent functional group compatibility that allows connecting various pharmaceuticals.
3 Conclusion
In conclusion, we have successfully reported the copper-catalyzed reaction of aziridine with imine and isocyanate, which afforded imidazolidine and imidazolidinone respectively. These developed reactions exhibited broad functional group compatibility to access a diverse array of potential bioactive 5-membered azaheterocycles. Furthermore, to demonstrate the practical applicability of these reactions, we prepared hybrids of pharmaceutical molecules using the developed reactions. These reactions surely facilitate the syntheses of complex azaheterocycles, which will accelerate the discovery of structurally new bioactive molecules in the future.
4 Materials and methods
For experimental procedures and compound characterization data, see the Supplementary Materials.
4.1 Experimental section
4.1.1 Experimental procedures and characterization data
4.1.1.1 General procedure for reaction of aziridine with imine
Aziridine (0.20 mmol, 1.0 equiv.), imine (0.30 mmol, 1.5 equiv.), copper bromide (3.0 mg, 0.020 mmol, 10 mol%), and 2,9-dimethyl-1,10-phenanthroline (4.2 mg, 0.020 mmol, 10 mol%) were added with a stirring bar to a dried Schlenk tube. The tube was filled with nitrogen. Toluene (0.5 mL) was added to the tube and the mixture was stirred at 120°C for 20 h. The crude mixture was passed through a short pad of silica gel and concentrated in vacuo. Purification by chromatography on silica gel provided the desired product.
4.1.1.2 General procedure for reaction of aziridine with isocyanate
Aziridine (0.20 mmol, 1.0 equiv.), isocyanate (0.30 mmol, 1.5 equiv.), copper bromide (3.0 mg, 0.020 mmol, 10 mol%), and 2,9-dimethyl-1,10-phenanthroline (4.2 mg, 0.020 mmol, 10 mol%) were added with a stirring bar to a dried Schlenk tube. The tube was filled with nitrogen. Toluene (0.5 mL) was added to the tube and the mixture was stirred at 120°C for 20 h. The crude mixture was passed through a short pad of silica gel and concentrated in vacuo. Purification by chromatography on silica gel provided the desired product.
Data availability statement
The original contributions presented in the study are included in the article/Supplementary Material, further inquiries can be directed to the corresponding author.
Author contributions
KH: Writing–review and editing. DH: Writing–review and editing. SM: Writing–review and editing. KM: Writing–original draft, Writing–review and editing.
Funding
The authors declare financial support was received for the research, authorship, and/or publication of this article. This work was supported by Japan Science and Technology Agency (JST) PRESTO Grant Number JPMJPR20D8 and the Kobayashi Foundation.
Conflict of interest
The authors declare that the research was conducted in the absence of any commercial or financial relationships that could be construed as a potential conflict of interest.
Publisher’s note
All claims expressed in this article are solely those of the authors and do not necessarily represent those of their affiliated organizations, or those of the publisher, the editors and the reviewers. Any product that may be evaluated in this article, or claim that may be made by its manufacturer, is not guaranteed or endorsed by the publisher.
Supplementary material
The Supplementary Material for this article can be found online at: https://www.frontiersin.org/articles/10.3389/fchem.2023.1272034/full#supplementary-material
References
Antonopoulos, S., Kokkoris, S., Gerakari, S., Mikros, S., Nitsotolis, T., Vikeli, D., et al. (2008). Comparison of monotherapy versus combination antihypertensive therapy in elderly patients with essential hypertension. Angiology 59, 230–235. doi:10.1177/0003319707304580
Bozorov, K., Zhao, J., and Aisa, H. A. (2019). 1,2,3-Triazole-containing hybrids as leads in medicinal chemistry: A recent overview. Bioorg. Med. Chem. 27, 3511–3531. doi:10.1016/j.bmc.2019.07.005
Higuchi, D., Matsubara, S., Kadowaki, H., Tanaka, D., and Murakami, K. (2023). Copper-catalyzed heterocyclic recombination of aziridine and diazetidine for the synthesis of imidazolidine. Chem. Eur. J. 29, e202301071. doi:10.1002/chem.202301071
Li, H., Huang, S., Wang, Y., and Huo, C. (2018). Oxidative dehydrogenative [2 + 3]-cyclization of Glycine esters with aziridines leading to imidazolidines. Org. Lett. 20, 92–95. doi:10.1021/acs.orglett.7b03448
Lin, T.-Y., Wu, H.-H., Feng, J.-J., and Zhang, J. (2018). Chirality transfer in rhodium(I)-Catalyzed [3 + 2]-cycloaddition of vinyl aziridines and oxime ethers: Atom-economical synthesis of chiral imidazolidines. Org. Lett. 20, 3587–3590. doi:10.1021/acs.orglett.8b01378
Liu, P., Xu, G., and Sun, J. (2017). Metal-free [2 + 1 + 2]-cycloaddition of tosylhydrazones with hexahydro-1,3,5-triazines to form imidazolidines. Org. Lett. 19, 1858–1861. doi:10.1021/acs.orglett.7b00600
Minakata, S., Miwa, H., Yamamoto, K., Hirayama, A., and Okumura, S. (2021). Diastereodivergent intermolecular 1,2-diamination of unactivated alkenes enabled by iodine catalysis. J. Am. Chem. Soc. 143, 4112–4118. doi:10.1021/jacs.1c00228
Ozaki, T., Yorimitsu, H., and Perry, G. J. P. (2021). Primary sulfonamide functionalization via sulfonyl pyrroles: Seeing the N-ts bond in a different light. Chem. Eur. J. 27, 15387–15391. doi:10.1002/chem.202102748
Pal, T., Lahiri, G. K., and Maiti, D. (2020). Copper in efficient synthesis of aromatic heterocycles with single heteroatom. Eur. J. Org. Chem. 2020, 6859–6869. doi:10.1002/ejoc.202000688
Pineschi, M. (2020). The binomial copper-catalysis and asymmetric ring opening of strained heterocycles: Past and future challenges. Eur. J. Org. Chem. 2020, 2643–2649. doi:10.1002/ejoc.201901853
Pradhan, S., Chauhan, N., Shahi, C. K., Bhattacharyya, A., and Ghorai, M. K. (2020). Stereoselective synthesis of hexahydroimidazo[1,2-a]quinolines via SN2-type ring-opening hydroarylation–hydroamination cascade cyclization of activated aziridines with N-propargylanilines. Org. Lett. 22, 7903–7908. doi:10.1021/acs.orglett.0c02801
Sengoden, M., Bhowmick, A., and Punniyamurthy, T. (2017). Stereospecific copper-catalyzed domino ring opening and sp3 C−H functionalization of activated aziridines with N-alkylanilines. Org. Lett. 19, 158–161. doi:10.1021/acs.orglett.6b03458
Shi, Z., Fan, T., Zhang, X., Zhan, F., Wang, Z., Zhao, L., et al. (2021). Synthesis of diversely substituted imidazolidines via [3+2] cycloaddition of 1,3,5-triazinanes with donor-acceptor aziridines and their anti-tumor activity. Adv. Synth. Catal. 363, 2619–2624. doi:10.1002/adsc.202001569
Smith, A. L., Doershuk, C., Goldmann, D., Gore, E., Hilman, B., Marks, M., et al. (1999). Comparison of a β-lactam alone versus β-lactam and an aminoglycoside for pulmonary exacerbation in cystic fibrosis. J. Pediatr. 134, 413–421. doi:10.1016/s0022-3476(99)70197-6
Spielmann, K., van der Lee, A., de Figueriedo, R. M., and Campagne, J.-M. (2018). Diastereoselective palladium-catalyzed (3 + 2)-cycloadditions from cyclic imines and vinyl aziridines. Org. Lett. 20, 1444–1447. doi:10.1021/acs.orglett.8b00228
Tu, L., Li, Z., Feng, T., Yu, S., Huang, R., Li, J., et al. (2019). Access to imidazolidines via 1,3-dipolar cycloadditions of 1,3,5-triazinanes with aziridines. J. Org. Chem. 84, 11161–11169. doi:10.1021/acs.joc.9b01959
Wu, X., and Zhang, J. (2012). Y(OTf)3-Catalyzed diastereoselective [3+2] cycloaddition of N-tosylaziridines and imines; efficient synthesis of multisubstituted imidazolidines. Synthesis 44, 2147–2154. doi:10.1055/s-0031-1290816
Zhang, Z., Chen, P., and Liu, G. (2022). Copper-catalyzed radical relay in C(sp3)–H functionalization. Chem. Soc. Rev. 51, 1640–1658. doi:10.1039/d1cs00727k
Keywords: copper catalyst, aziridine, imine, isocyanate, imidazolidine, imidazolidinone, cyclization
Citation: Hashimoto K, Higuchi D, Matsubara S and Murakami K (2023) Copper-catalyzed reaction of aziridine for the synthesis of substituted imidazolidine and imidazolidinone. Front. Chem. 11:1272034. doi: 10.3389/fchem.2023.1272034
Received: 03 August 2023; Accepted: 18 September 2023;
Published: 29 September 2023.
Edited by:
Takashi Ohshima, Kyushu University, JapanReviewed by:
Tao Shi, The Scripps Research Institute, United StatesMaría De Gracia Retamosa, University of Alicante, Spain
Copyright © 2023 Hashimoto, Higuchi, Matsubara and Murakami. This is an open-access article distributed under the terms of the Creative Commons Attribution License (CC BY). The use, distribution or reproduction in other forums is permitted, provided the original author(s) and the copyright owner(s) are credited and that the original publication in this journal is cited, in accordance with accepted academic practice. No use, distribution or reproduction is permitted which does not comply with these terms.
*Correspondence: Kei Murakami, a2VpLm11cmFrYW1pQGt3YW5zZWkuYWMuanA=