- 1Institut für Neurowissenschaften und Medizin, INM-5: Nuklearchemie, Forschungszentrum Jülich (FZJ), Jülich, Germany
- 2Department of Physics, Government College University Lahore (GCUL), Lahore, Pakistan
- 3Department of Physics, COMSATS University Islamabad, Lahore, Pakistan
Copper has several clinically relevant radioisotopes and versatile coordination chemistry, allowing attachment of its radionuclides to biological molecules. This characteristic makes it suitable for applications in molecular imaging or radionuclide targeted therapy. Of particular interest in nuclear medicine today is the theranostic approach. This brief review considers five radionuclides of copper. These are Cu-60, Cu-61, Cu-62, Cu-64, and Cu-67. The first four are positron emitters for imaging, and the last one Cu-67 is a β–-emitting radionuclide suitable for targeted therapy. The emphasis here is on theory-aided evaluation of available experimental data with a view to establishing standardised cross-section database for production of the relevant radionuclide in high purity. Evaluated cross section data of the positron emitters have been already extensively reported; so here they are only briefly reviewed. More attention is given to the data of the 68Zn(p,2p)67Cu intermediate energy reaction which is rather commonly used for production of 67Cu.
1 Introduction
Copper is an essential trace element in all living systems. It has versatile coordination chemistry (Blower et al., 1996; Wadas et al., 2007) which allows its metallation with various chelators, such as DOTA (1,4,7,10-tetraazacyclododecane tetraacetic acid), NOTA (1,4,7-triazacyclononane triacetic acid) etc., that can be conjugated to biological targeting molecules like peptides, proteins and antibodies (Blower et al., 1996; Wadas et al., 2007; Aluicio-Sarduy et al., 2018). Thus suitable radionuclides of copper attached to those molecules have great potential in molecular imaging and/or radionuclide targeted therapy, i.e., in following the theranostic approach, which entails the use of two radionuclides of the same element in identical chemical form, one a positron emitter for measuring the distribution kinetics of the radioactivity in the body via Positron Emission Tomography (PET), and the other a radionuclide emitting corpuscular radiation (β–, α or Auger electrons) useful for internal radiotherapy. The two nuclides are denoted as “matched pair” (Herzog et al., 1993; Rösch et al., 2017; Qaim et al., 2018). The radionuclides of copper of theranostic interest are listed in Table 1. They consist of four positron-emitting radionuclides, namely, 60Cu(T1/2 = 23.7 min), 61Cu(T1/2 = 3.33 h), 62Cu(T1/2 = 9.67 min) and 64Cu(T1/2 = 12.7 h), and the β–emitting radionuclide 67Cu (T1/2 = 61.83 h). Whereas 60Cu and 62Cu, being rather short-lived, have found only limited application in PET measurements (Wallhaus et al., 1998; McCarthy et al., 1999; Ng et al., 2014), 61Cu and 64Cu are more widely used. In particular 64Cu is gaining increasing significance due to its almost ideal decay characteristics for PET imaging (Williams et al., 2005). The counterpart radionuclide 67Cu is of considerable interest in internal radiotherapy because of its suitable half-life and β–energy. The “matched pairs” 61Cu/67Cu and 64Cu/67Cu thus constitute very important theranostic pairs. Furthermore, because of its β–and Auger electron emission component, the radionuclide 64Cu is also of interest in radionuclide targeted therapy.
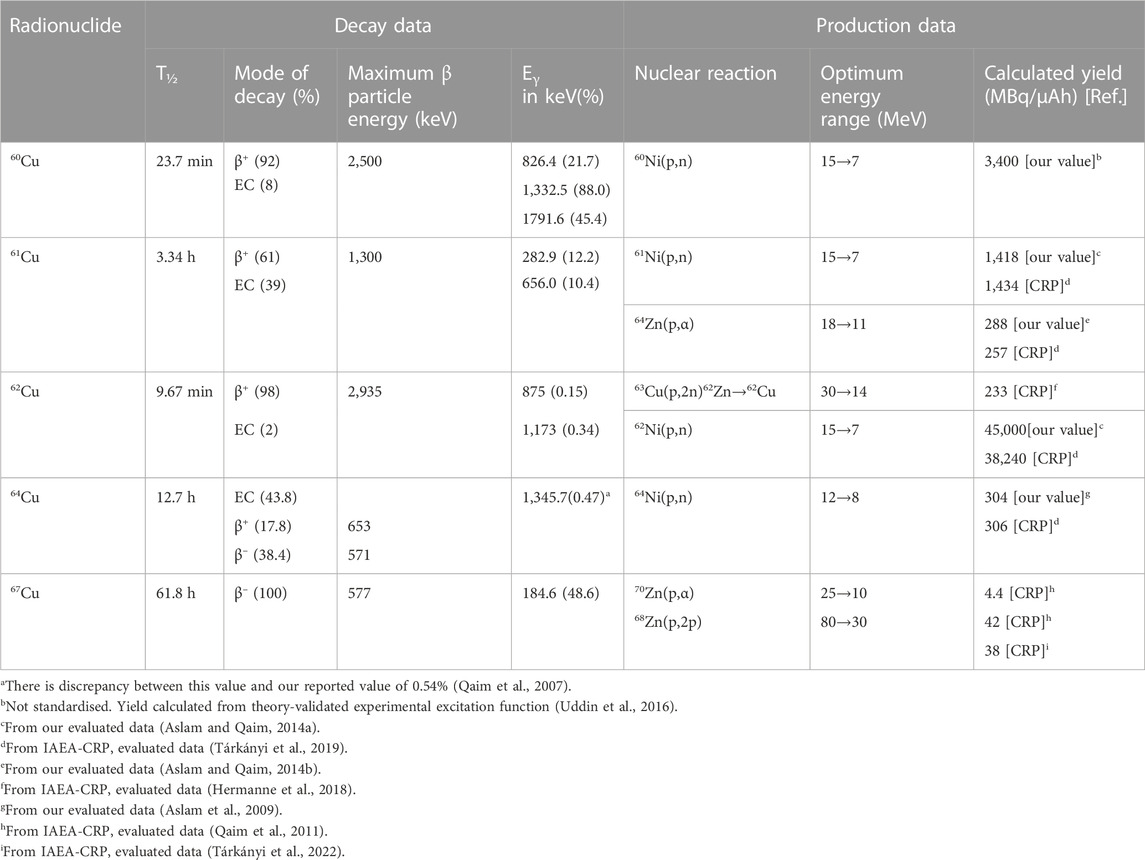
TABLE 1. Standardised decay and production data of some copper radionuclides of theranostic interest.
The most significant decay data and the important production methods of the five radionuclides under consideration are also given in Table 1. Both decay data and production methodologies have been amply described (Qaim et al., 2018; Qaim, 2019; NUDAT 3.0, 2023; Nichols, 2022; Qaim, 2017; Qaim et al., 2019). In this brief review therefore we concentrate only on a special aspect, namely, the standardisation (also called “evaluation”) of nuclear data of those five radionuclides. As far as we know, to date such a review has not been written.
2 Standardised decay data: discrepancies and deficiencies
The decay data have conventionally received more attention with regard to their standardisation, and detailed evaluated mass decay chains are available for the above-mentioned five radionuclides (Junde et al., 2005; Nichols et al., 2012; Browne and Tuli, 2013; Zuber and Singh, 2015; Singh and Chen, 2021). The data given in Table 1 are all standardised, especially the half-lives, the γ-ray energies and their intensities, as well as the β+(β–) energies and their intensities. They were taken from NUDAT which is based on evaluated mass decay chains. Yet some uncertainties do exist. The intensity of the weak 1,345.7 keV γ-ray of 64Cu, for example, is slightly controversial. The reported standardised value is (0.47 ± 0.01) % (Bé et al., 2012). A later measurement using 64Cu in a solution volume of 5 mL gave the same value for the intensity of this γ-ray (Pibida et al., 2017). But an independent experiment done earlier at FZJ (Qaim et al., 2007), utilizing a cyclotron-produced, radiochemically separated, highly pure thin point source gave a value of (0.54 ± 0.03) %. Incidentally, in that work the intensities obtained for β+, β–and EC emissions were exactly the same as the standardised values. The discrepancy is thus specific to the determination of the 1,345.7 keV γ-ray. An independent experiment using a properly prepared thin sample of 64Cu is therefore suggested to solve the discrepancy.
An important consideration in quantification of a PET measurement is the intensity of the positrons emitted from the radionuclide (Iβ+). In most cases this intensity (% of decay) is derived from a balance of various γ-transitions described in the decay scheme. But often there are uncertainties in the reported values. A direct experimental determination of the positron emission intensity involving a spectrometric analysis of the 511 keV annihilation radiation and Kα X-rays, as developed at FZJ, appears to provide more accurate results (Qaim et al., 2007). The Iβ+ value for 64Cu obtained this way amounted to 17.8% and is now regarded as the standard value. For 60Cu, 61Cu, and 62Cu, so far such a direct measurement has not been performed. On the other hand, the Iβ+ values for 60Cu and 62Cu, being 93% and ∼100%, respectively, are rather strong; the uncertainty is therefore assumed to be small. Furthermore, due to very limited use of those radionuclides, the reported Iβ+ values appear to be satisfactory. Regarding the radionuclide 61Cu, on the other hand, the reported Iβ+ value of 61% is rather uncertain (Qaim, 2017; Qaim et al., 2019). In view of somewhat enhancing interest in this radionuclide, a direct measurement of the Iβ+ value, as done for 64Cu, appears to be of some urgency.
As far as the decay data of 67Cu are concerned, there appears to be no discrepancy (Junde et al., 2005) and the energies and intensities of all emitted radiations given in Table 1 may be regarded as standardised values.
3 Methodologies for standardisation of production data
3.1 General development
In contrast to neutron-induced reactions, the standardisation of charged-particle induced reaction cross sections, needed for production of radionuclides at cyclotrons/accelerators, remained initially somewhat neglected (Qaim, 2020). From 1995 onwards, however, the IAEA got interested in this field, and the relevant data compilation and evaluation efforts were intensified. The former led to improvement of the international EXFOR file and the standardisation of those data was initiated and followed through three successive Coordinated Research Projects (CRPs). Since no evaluation methodology for charged-particle data existed in the beginning, in the first CRP related to commonly used diagnostic radionuclides, the work was rather empirical and reliance was placed on statistical fitting of concordant set of data (Gul et al., 2001). In the second CRP dealing with therapeutic radionuclides, theory was also introduced to some extent. Calculations were done using the codes ALICE-IPPE and EMPIRE for comparison with the experimental data (Qaim et al., 2011). The third and last CRP in the series became a very extensive endeavour dealing with a large number of novel radionuclides (Tárkányi et al., 2019). However, the selection/rejection of the experimental data in the evaluation process remained rather empirical, and standardised curves were obtained by statistical fittings. For comparison, only the results of the global theoretical file TENDL were considered. All data for charged-particle production of medical radionuclides evaluated under those CRPs are available on the website of the IAEA [Medical Portal (iaea.org)].
3.2 Theory-assisted selection of data in the standardisation process
Standardisation work on charged-particle induced reaction cross sections has also been done outside the IAEA-CRPs mentioned above. In those studies extensive use was made of nuclear model calculations to ascertain the most concordant set of data for a given nuclear reaction, followed by polynomial fitting of the selected data. Based on a suggestion made by Sudár et al. (2002), the standardisation methodology was extensively developed under a German-Pakistan cooperation (Hussain et al., 2009, 2010; Aslam and Qaim, 2014a; Aslam and Qaim, 2014b; Amjed et al., 2016, 2020; Ali et al., 2019). It consists of a comparison of the experimental data with the results of a nuclear model calculation, whereby the input parameters are adjusted within their recommended limits (RIPL-3). The basic relation for obtaining the evaluated cross section is developed as
where σev (E), σmodel (E) and f (E) are the evaluated cross section, model calculated cross section and the energy-dependent normalisation factor, respectively. The ratio of experimental to calculated data is plotted as a function of energy, followed by a polynomial fitting to estimate the
For nuclear model calculations, in our collaboration four major codes, namely, STAPRE, ALICE-IPPE, EMPIRE and TALYS (Koning et al. 2005) were used. With the exception of ALICE-IPPE, which is based purely on the exciton precompound model, the other codes entail a combination of compound and precompound processes, with some consideration of direct interactions. They reproduce the experimental data with varying degree of success. In each calculation therefore, the optical model parameters were varied within their recommended limits to obtain a fit as close as possible to the experimental data. The recommended data for the particular production reaction were then generated as outlined earlier. For uncertainty estimates, a confidence limit of 95% was adopted. With constant improvements in the code TALYS in recent years (Koning et al. 2023), its use has become more universal.
4 Standardised (recommended) cross-section data
For all 5 radionuclides under consideration, several production routes were studied (see below). However, in Table 1 we give for each radionuclide only the more commonly used production reactions, the optimum energy ranges derived and the thick target yields calculated from the standardised excitation functions. Each radionuclide is discussed below individually.
4.1 Positron emitters
4.1.1 60Cu
The most suitable production route for this radionuclide is the 60Ni(p,n)60Cu process. However, no attempt has been made to standardise its cross-section data either in the IAEA-CRP or by us. On the other hand, a detailed experimental and theoretical study of the excitation function of this reaction has been carried out by Uddin et al. (2016). We used those data as reference values to deduce the optimum energy range (Ep = 15→7 MeV) for the production of 60Cu, and also calculated its expected thick target yield. This process has been developed to produce high-purity 60Cu on a clinical scale (McCarthy et al., 1999).
4.1.2 61Cu
The cross-section data for the reactions 61Ni(p,n)61Cu, 62Ni(p,2n)61Cu, 60Ni(d,n)61Cu and 58Ni(α,p)61Cu were standardised by Aslam and Qaim (2014a) and those for the reactions 64Zn(p,α)61Cu, 64Zn(d,αn)61Cu, 59Co(3He,n)61Cu and 59Co(α,2n)61Cu by Aslam and Qaim (2014b). The standardised numerical data are given in those two publications. The 59Co(α,2n)61Cu reaction can be used if an α-particle beam of about 40 MeV is available (Fukumura et al., 2004). However, the reactions 61Ni(p,n)61Cu and 64Zn(p,α)61Cu have been more commonly used for production. The suitable energy ranges for those two reactions were deduced. The calculated yields of 61Cu from standardised curves by us (Aslam and Qaim, 2014a; Aslam and Qaim, 2014b) and those by the IAEA-CRP (Tárkányi et al., 2019) are given in Table 1. They agree within about 1% for the 61Ni(p,n)-reaction and about 10% for the 64Zn(p,α)-reaction. For both reactions, using highly-enriched targets, clinical scale production of high-purity 61Cu has been reported (McCarthy et al., 1999; Thieme et al., 2013).
4.1.3 62Cu
This short-lived positron emitting radionuclide is obtained via two routes:
a) natCu(p,xn)62Zn→62Cu (generator)
b) 62Ni(p,n)62Cu
The data for the reaction natCu(p,xn)62Zn have been very well standardised because it is an important monitor reaction (IAEA report, Gul et al., 2001) and the calculated yield of 62Zn over the suitable energy range (Ep = 30→14 MeV) is given in Table 1. Use of an enriched target is not necessary. This route is the method of choice for the production of 62Cu (Wallhaus et al., 1998; Ng et al., 2014). The data for the reaction 62Ni(p,n)62Cu were standardised by us (Aslam and Qaim, 2014a) and the IAEA-CRP (Tárkányi et al., 2019). The yields agree within about 15%. This route gives a very high yield of the product but, in order to achieve high radionuclidic purity, an enriched target is needed. It has seldom been used for production.
4.1.4 64Cu
Cross-section data of a large number of reactions leading to the formation of this radionuclide have been standardised both by us (Aslam et al., 2009) and under an IAEA-CRP (Qaim et al., 2011). They include the reactions 64Ni(p,n)64Cu, 64Ni(d,2n)64Cu, 68Zn(p,αn)64Cu, 66Zn(p,2pn)64Cu, 64Zn(d,2p)64Cu, 66Zn(d,α)64Cu, natZn(d,x)64Cu and a few others. Out of all those reactions, however, the 64Ni(p,n)64Cu process on highly-enriched target is the most interesting. Its thick target yields reported by us and the IAEA-CRP agree within 1%. Initially proposed by the Jülich group (Szelecsényi et al., 1993) and further developed by the St. Louis group (McCarthy et al., 1997), the technology was improved over the years (for a review cf. Qaim et al., 2018), and today this reaction has become the method of choice for large scale production of high-purity and high-specific-activity 64Cu.
4.2 Therapeutic radionuclide 67Cu
This β–-emitting therapeutic radionuclide has been of interest for more than 40 years and its production methods have been reviewed by several groups (Qaim et al., 2011; Qaim, 2012; IAEA-report; Smith et al., 2012; Qaim et al., 2018; Mou et al., 2022). Considerable industrial efforts are underway to produce it via the 68Zn(γ,p)67Cu process. Its excitation function is known fairly well. We concentrated on four charged-particle induced reactions. Three of them have been investigated in the low-energy range. They are 70Zn(p,α)67Cu (Levkovskii, 1991; Kastleiner et al., 1999; Dellepiane et al., 2023), 70Zn(d,αn)67Cu (Kozempel et al., 2012; Nigron et al., 2021) and 64Ni(α,p)67Cu (Skakun and Qaim, 2004; Ohya et al., 2018; Uddin et al., 2018; Takács et al., 2020). An evaluation of the cross section data was, however, carried out only for the 70Zn(p,α)67Cu reaction under an IAEA-CRP (Qaim et al., 2011). The new data by Delllepiane et al. (2023) up to 18 MeV fit well in the evaluated curve. Thus a reliable standardised database is available for this reaction up to 30 MeV. This method has been used for 67Cu production in MBq quantities at 24–30 MeV cyclotrons (Hilgers et al., 2003; Lee et al., 2022). Very recently some new data have been reported for the 70Zn(p,x)67Cu process up to proton energy of about 70 MeV (Pupillo et al., 2020). The cross section increases sharply beyond 40 MeV. An evaluation of the data would be meaningful when more information is available. The fourth reaction, namely, 68Zn(p,2p)67Cu, is presently often used for production purposes. The standardisation of production cross sections was attempted under an IAEA-CRP (Qaim et al., 2011). Another IAEA-CRP version has also been presented (Tárkányi et al., 2022). Jung et al. (2023) reported extensive new cross-section measurements in the higher energy range. We discuss critically all reported data.
For this reaction, twelve experiments have been reported in the EXFOR library of the IAEA (http://www-nds.iaea.org/exfor/exfor.htm) over the proton energy range up to 430 MeV. For evaluation, however, the data only up to 100 MeV are interesting, i.e., leaving out some data points in the higher energy region (Morrison et al., 1962; Morrison et al., 1964; Mirzadeh et al., 1986). Cohen et al. (1955) measured only one cross section value at 21.5 MeV which was found to be very discrepant. McGee et al. (1970) performed measurements at eight energies covering the range from 30 to 85 MeV. They used the reference of Meadows (1953) for the monitor reaction. The present status of that monitor reaction led us to correct those data. Similarly the Levkovskii data (1991) were reduced by 25% because of the use of wrong monitor cross section (Qaim et al., 2014). Stoll et al. (2002) performed the experiment over a wide energy range of 24.9–70.8 MeV at two cyclotrons (JULIC and PSI accelerator) using enriched 68Zn (98.3%) thin target samples and radiochemical separation of 67Cu. The experimental data were generally consistent but a few data points showed a systematic lower trend in the energy range of 35–45 MeV investigated at the cyclotron JULIC. Bonardi et al. (2005) studied this reaction up to 141 MeV using thin target foils of Zn. The data were consistent but with large uncertainties in all energy regions. Szelecsényi et al. (2009) reported the data for this reaction up to 40 MeV. They used enriched 68Zn (≥99%) as the target material. Recently two detailed measurements have been reported for this reaction using enriched 68Zn targets, one by Pupillo et al. (2018) and the other by Jung et al. (2023). Pupillo et al. (2018) measured the cross sections after radiochemical separation while Jung et al. (2023) used two analytical methods without radiochemical separation. Both datasets were found to be consistent. All the normalised data are plotted in Figure 1 as a function of proton energy. The IAEA evaluated data curves (Medical Portal (iaea.org)) are also shown in Figure 1. It is evident that those two evaluations are not fully supported by the new data. In particular the updated curve (Tárkányi et al., 2022) appears to be too low. Considering all the data published we conclude that a new evaluation is necessary using the theory-assisted selection of data. For practical production of 67Cu via this route, however, the energy range Ep = 80→30 MeV remains the most suitable (Qaim, 2012). This method has been practically used in clinical scale production of 67Cu (Katabuchi et al., 2008; Medvedev et al., 2012).
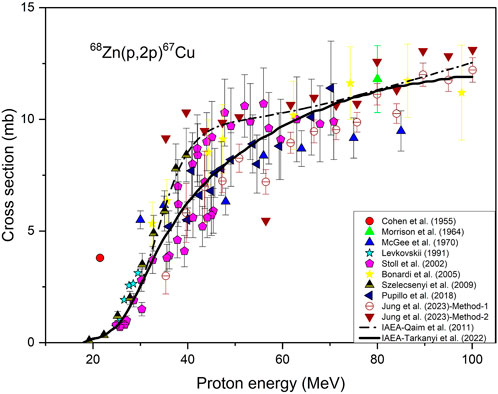
FIGURE 1. Normalised experimental data and IAEA-evaluated reaction cross section curves for the 68Zn(p,2p)67Cu process.
5 Discussion
The decay data of the 5 radionuclides of copper relevant for theranostic applications are well standardised, except for the intensity of the weak γ-line of 64Cu at 1,345.7 keV where some discrepancy exists. This discrepancy needs to be solved, especially because some radionuclide producers use this γ-ray for determination of the total radioactivity of 64Cu.
The data for production of 61Cu, 62Cu and 64Cu via the more common routes are well evaluated. For the standardisation of production cross sections of 60Cu, however, more measurements are needed. With regard to the data for the production of the therapeutic radionuclide 67Cu, presently considerable efforts are underway. Standardised data for the reaction 70Zn(p,x)67Cu are available up to 30 MeV which consists of the (p,α) reaction. In the higher energy region up to 70 MeV, however, the cross section increases rapidly, possibly due to the onset of the many nucleon emission processes like 70Zn(p,2p2n)67Cu. But more measurements and a critical evaluation are needed to obtain standardised data for this process. The presently rather commonly used intermediate reaction 68Zn(p,2p)67Cu was evaluated under two IAEA-CRPs. The newest measurements, however, show some deviations from the evaluated data. A new critical evaluation should thus be very meaningful. In order to estimate the specific activity of the radionuclide produced, it is also imperative to determine via model calculation the inactive material, i.e., 65Cu, co-produced with the respective radionuclide.
Author contributions
SQ: Writing–original draft, Conceptualization, Supervision, Writing–review and editing. IS: Investigation, Writing–original draft. MH and MA: Data curation, Writing–original draft. BN: Funding acquisition, Writing–review and editing.
Funding
The author(s) declare that no financial support was received for the research, authorship, and/or publication of this article.
Acknowledgments
MH thanks the Alexander von Humboldt Foundation of Germany for a fellowship to carry out this study at FZJ Germany.
Conflict of interest
The authors declare that the research was conducted in the absence of any commercial or financial relationships that could be construed as a potential conflict of interest.
Publisher’s note
All claims expressed in this article are solely those of the authors and do not necessarily represent those of their affiliated organizations, or those of the publisher, the editors and the reviewers. Any product that may be evaluated in this article, or claim that may be made by its manufacturer, is not guaranteed or endorsed by the publisher.
References
Ali, W., Tashfeen, M., and Hussain, M. (2019). Evaluation of nuclear reaction cross sections via proton induced reactions on 55Mn for the production of 52Fe: A potential candidate for theranostic applications. Appl. Radiat. Isot. 144, 124–129. doi:10.1016/j.apradiso.2018.11.016
Aluicio-Sarduy, E., Ellison, P. A., Barnhart, T. E., Cai, W., Nickles, R. J., and Engle, J. W. (2018). PET radiometals for antibody labeling. J. Label. Comp. Radiopharm. 61, 636–651. doi:10.1002/jlcr.3607
Amjed, N., Hussain, M., Aslam, M. N., Tárkányi, F., and Qaim, S. M. (2016). Evaluation of nuclear reaction cross sections for optimization of production of the emerging diagnostic radionuclide 55 Co. Appl. Radiat. Isot. 108, 38–48. doi:10.1016/j.apradiso.2015.11.058
Amjed, N., Wajid, A. M., Ahmad, N., Ishaq, M., Aslam, M. N., Hussain, M., et al. (2020). Evaluation of nuclear reaction cross sections for optimization of production of the important non-standard positron emitting radionuclide 89Zr using proton and deuteron induced reactions on 89Y target. Appl. Radiat. Isot. 165, 109338. doi:10.1016/j.apradiso.2020.109338
Aslam, M. N., and Qaim, S. M. (2014b). Nuclear model analysis of excitation functions of proton and deuteron induced reactions on 64Zn and 3He- and α-particle induced reactions on 59Co leading to the formation of copper-61: Comparison of major production routes. Appl. Radiat. Isot. 94, 131–140. doi:10.1016/j.apradiso.2014.08.001
Aslam, M. N., and Qaim, S. M. (2014a). Nuclear model analysis of excitation functions of proton, deuteron and α-particle induced reactions on nickel isotopes for production of the medically interesting copper-61. Appl. Radiat. Isot. 89, 65–73. doi:10.1016/j.apradiso.2014.02.007
Aslam, M., Sudár, S., Hussain, M., Malik, A. A., Shah, H. A., and Qaim, S. M. (2009). Charged particle induced reaction cross section data for production of the emerging medically important positron emitter 64Cu: A comprehensive evaluation. Radiochim. Acta 97, 669–686. doi:10.1524/ract.2009.1670
Bé, M.-M., Cassette, P., Lépy, M. C., Amiot, M.-N., Kossert, K., Nähle, O., et al. (2012). Standardization, decay data measurements and evaluation of 64Cu. Appl. Radiat. Isot. 70, 1894–1899. doi:10.1016/j.apradiso.2012.02.056
Blower, P. J., Lewis, J. S., and Zweit, J. (1996). Copper radionuclides and radiopharmaceuticals in nuclear medicine. Nucl. Med. Biol. 23, 957–980. doi:10.1016/s0969-8051(96)00130-8
Bonardi, M. L., Groppi, F., Mainardi, H. S., Kokhanyuk, V. M., Lapshina, E. V., Mebel, M. V., et al. (2005). Cross section studies on 64Cu with zinc target in the proton energy range from 141 down to 31 MeV. J. Radioanal. Nucl. Chem. 264, 101–105. doi:10.1007/s10967-005-0681-1
Browne, E., and Tuli, J. K. (2013). Nuclear data sheets for A = 60. Nucl. Data Sheets 114, 1849–2022. doi:10.1016/j.nds.2013.11.002
Cohen, B. L., Newman, E., and Handley, T. H. (1955). (p, pn)+(p, 2n) and (p, 2p) cross sections in medium weight elements. Phys. Rev. 99, 723–727. doi:10.1103/physrev.99.723
Dellepiane, G., Casolaro, P., Gottstein, A., Mateu, I., Scampoli, P., and Braccini, S. (2023). Optimized production of 67Cu based on cross section measurements of 67Cu and 64Cu using an 18 MeV medical cyclotron. Appl. Radiat. Isot. 195, 110737. doi:10.1016/j.apradiso.2023.110737
EXFOR database (2023). Available at: http://www-nds.iaea.org/exfor/exfor.htm.
Fukumura, T., Okada, K., Szelecsényi, F., Kovács, Z., and Suzuki, K. (2004). Practical production of 61Cu using natural Co target and its simple purification with a chelating resin for 61Cu-ATSM. Radiochim. Acta 92, 209–214. doi:10.1524/ract.92.4.209.35593
Gul, K., Hermanne, A., Mustafa, M. G., Nortier, F. M., Oblozinsky, P., and Qaim, S. M. (2001). Charged particle cross section database for medical radioisotopes production: Diagnostic radioisotopes and monitor reactions, IAEA technical report series, IAEA-TECDOC-1211.
Hermanne, A., Ignatyuk, A. V., Capote, R., Carlson, B. V., Engle, J. W., Kellett, M. A., et al. (2018). Reference Cross Sect. Charged-particle Monit. React. Nucl. Data Sheets 148, 1338–1382. doi:10.1016/j.nds.2018.02.009
Herzog, H., Rösch, F., Stöcklin, G., Lueders, C., Qaim, S. M., and Feinendegen, L. E. (1993). Measurement of pharmacokinetics of yttrium-86 radiopharmaceuticals with PET and radiation dose calculation of analogous yttrium-90 radiotherapeutics. J. Nucl. Med. 34, 2222–2226.
Hilgers, K., Stoll, T., Skakun, Y., Coenen, H. H., and Qaim, S. M. (2003). Cross-section measurements of the nuclear reactions natZn(d, x)64Cu, 66Zn(d,α)64Cu and 68Zn(p,αn)64Cu for production of 64Cu and technical developments for small-scale production of 67Cu via the 70Zn(p,α)67Cu process. Appl. Radiat. Isot. 59, 343–351. doi:10.1016/s0969-8043(03)00199-4
Hussain, M., Sudár, S., Aslam, M. N., Malik, A. A., Ahmad, R., and Qaim, S. M. (2010). Evaluation of charged particle induced reaction cross section data for production of the important therapeutic radionuclide 186Re. Radiochim. Acta 98, 385–395. doi:10.1524/ract.2010.1733
Hussain, M., Sudár, S., Aslam, M. N., Shah, H. A., Ahmad, R., Malik, A. A., et al. (2009). A comprehensive evaluation of charged-particle data for production of the therapeutic radionuclide 103Pd. Appl. Radiat. Isot 67, 1842–1854. doi:10.1016/j.apradiso.2009.06.010
Junde, H., Xiaolong, H., and Tuli, J. K. (2005). Nuclear data sheets for A = 67. Nucl. Data Sheets 106, 159–250. doi:10.1016/j.nds.2005.10.006
Jung, M., Cho, W., Jang, H. M., Chun, K., Lee, J. S., Kim, S. W., et al. (2023). Cross-section measurements for 68Zn(p,2p)67Cu and 68Zn(p,2n)67Ga reactions using a newly developed separation method for the superposed γ-ray spectra. Nat. Sci. Rep. 13, 11. doi:10.1038/s41598-023-38483-1
Kastleiner, S., Coenen, H. H., and Qaim, S. M. (1999). Possibility of production of 67Cu at a small-sized cyclotron via the (p,α)-reaction on enriched 70Zn. Radiochim. Acta 84, 107–110. doi:10.1524/ract.1999.84.2.107
Katabuchi, T., Watanabe, S., Ishioka, N. S., Lida, Y., Hanaoka, H., Endo, K., et al. (2008). Production of 67Cu via the 68Zn(p,2p)67Cu reaction and recovery of 68Zn target. J. Radioanal. Nucl. Chem. 277, 467–470. doi:10.1007/s10967-007-7144-9
Koning, A. J., Hilaire, S., and Duijvestijn, M. C. (2005). TALYS: Comprehensive nuclear reaction modeling. AIP Conf. Proc. 769, 1154–1159. doi:10.1063/1.1945212
Koning, A. J., Hilaire, S., and Goriely, S. (2023). TALYS: modelling of nuclear reactions. Eur. Phys. J. A. 59, 131
Kozempel, J., Abbas, K., Simonelli, F., Bulgheroni, A., Holzwarth, U., and Gibson, N. (2012). Preparation of 67Cu via deuteron irradiation of 70Zn. Radiochim. Acta 100, 419–424. doi:10.1524/ract.2012.1939
Lee, J. V., Chae, J. H., Hur, M. G., Yang, S. D., Kong, Y. B., Lee, J., et al. (2022). Theranostic 64Cu/67Cu radioisotopes production with RFT-30 cyclotron. Front. Med. 9, 889640. doi:10.3389/fmed.2022.889640
Levkovskii, V. N. (1991). Activation cross sections by medium energy (E = 10-50 MeV) protons and alpha-particles. Moscow, Russia: Intervesi, 215.Medium mass nuclides (A = 40-100)
McCarthy, D. W., Bass, L. A., Cutler, P. D., Shefer, R. E., Klinkowstein, R. E., Herrero, P., et al. (1999). High purity production and potential applications of copper-60 and copper-61. Nucl. Med. Biol. 26, 351–358. doi:10.1016/s0969-8051(98)00113-9
McCarthy, D. W., Shefer, R. E., Klinkowstein, R. E., Bass, L. A., Margeneau, W. H., Cutler, C. S., et al. (1997). Efficient production of high specific activity 64Cu using a biomedical cyclotron. Nucl. Med. Biol. 24, 35–43. doi:10.1016/s0969-8051(96)00157-6
McGee, T., Rao, C. L., Saha, G. B., and Yaffe, L. (1970). Nuclear interactions of 45Sc and 68Zn with protons of medium energy. Nucl. Phys. A 150, 11–29. doi:10.1016/0375-9474(70)90451-3
Meadows, J. W. (1953). Excitation functions for proton-induced reactions with copper. Phys. Rev. 91, 885–889. doi:10.1103/physrev.91.885
Medvedev, D. G., Mausner, L. F., Meinken, G. E., Kurczak, S. O., Schnakenberg, H., Dodge, C. J., et al. (2012). Development of a large scale production of 67Cu from 68Zn at the high energy proton accelerator: Closing the 68Zn cycle. Appl. Radiat. Isot. 70, 423–429. doi:10.1016/j.apradiso.2011.10.007
Mirzadeh, S., Mausner, L. F., and Srivastava, S. C. (1986). Production of no-carrier added 67Cu. Appl. Radiat. Isot. A. 37, 29–36. doi:10.1016/0883-2889(86)90192-9
Morrison, D. L., Albert, A., and Caretto, A. A. (1962). Excitation functions of (p, xp) reactions. Phys. Rev. 127, 1731–1738. doi:10.1103/physrev.127.1731
Morrison, D. L., Albert, A., and Caretto, A. A. (1964). Recoil study of the 68Zn(p,2p)67Cu reaction. Phys. Rev. B 133, 1165–B1170. doi:10.1103/physrev.133.b1165
Mou, L., Martini, P., Pupillo, G., Cieszykowska, I., Cutler, C. S., and Mikołajczak, R. (2022). 67Cu production capabilities: A mini review. Molecules 27, 1501. doi:10.3390/molecules27051501
Ng, Y., Lacy, J. L., Fletcher, J. W., and Green, M. A. (2014). Performance of a 62Zn/62Cu microgenerator in kit-based synthesis and delivery of [62Cu]Cu–ETS for PET perfusion imaging. Appl. Radiat. Isot. 91, 38–43. doi:10.1016/j.apradiso.2014.05.006
Nichols, A. L. (2022). Status of the decay data for medical radionuclides: Existing and potential diagnostic γ emitters, diagnostic β+ emitters and therapeutic radioisotopes. Radiochim. Acta 110, 609–644. doi:10.1515/ract-2022-0004
Nichols, A. L., Singh, B., and Tuli, J. K. (2012). Nuclear data sheets for A = 62. Nucl. Data Sheets 113, 973–1114. doi:10.1016/j.nds.2012.04.002
Nigron, E., Guertin, A., Haddad, F., and Sounalet, T. (2021). Is 70Zn(d, x)67Cu the best way to produce 67Cu for medical applications? Front. Med. 8, 674617. doi:10.3389/fmed.2021.674617
NUDAT-3.0 (2023). Available at: https://www.nndc.bnl.gov/nudat3/.
Ohya, T., Nagatsu, K., Suzuki, H., Fukada, M., Minegishi, K., Hanyu, M., et al. (2018). Small-scale production of 67Cu for a preclinical study via the 64Ni(α, p)67Cu channel. Nucl. Med. Biol. 59, 56–60. doi:10.1016/j.nucmedbio.2018.01.002
Pibida, L., Zimmerman, B., Bergeron, D. E., Fitzgerald, R., Cessna, J. T., and King, L. (2017). Determination of photon emission probability for the main gamma ray and half-life measurements of 64Cu. Appl. Radiat. Isot 129, 6–12. doi:10.1016/j.apradiso.2017.07.001
Pupillo, G., Mou, L., Martini, P., Pasquali, M., Boschi, A., Cicoria, G., et al. (2020). Production of 67Cu by enriched 70Zn targets: First measurements of formation cross sections of 67Cu, 64Cu, 67Ga, 66Ga, 69mZn and 65Zn in interactions of 70Zn with protons above 45 MeV. Radiochim. Acta 108, 593–602. doi:10.1515/ract-2019-3199
Pupillo, G., Sounalet, T., Michel, N., Mou, L., Esposito, J., and Haddad, F. (2018). New production cross sections for the theranostic radionuclide 67Cu. Nucl. Instr. Meth. B 415, 41–47. doi:10.1016/j.nimb.2017.10.022
Qaim, S. M. (2020). Medical radionuclide production-science and technology. Berlin/Boston: Walter de Gruyter.ISBN 978-3-11-060156-5
Qaim, S. M. (2017). Nuclear data for production and medical application of radionuclides: Present status and future needs. Nucl. Med. Bio 44, 31–49. doi:10.1016/j.nucmedbio.2016.08.016
Qaim, S. M. (2012). The present and future of medical radionuclide production. Acta 100, 635–651. doi:10.1524/ract.2012.1966
Qaim, S. M. (2019). Theranostic radionuclides: Recent advances in production methodologies. J. Radioanal. Nucl. Chem. 322, 1257–1266. doi:10.1007/s10967-019-06797-y
Qaim, S. M., Bisinger, T., Hilgers, K., Nayak, D., and Coenen, H. H. (2007). Positron emission intensities in the decay of 64Cu, 76Br and 124I. Radiochim. Acta 95, 67–73. doi:10.1524/ract.2007.95.2.67
Qaim, S. M., Scholten, B., and Neumaier, B. (2018). New developments in the production of theranostic pairs of radionuclides. J. Radioanal. Nucl. Chem. 318, 1493–1509. doi:10.1007/s10967-018-6238-x
Qaim, S. M., Scholten, B., Spahn, I., and Neumaier, B. (2019). Positron-emitting radionuclides for applications, with special emphasis on their production methodologies for medical use. Radiochim. Acta 107, 1011–1026. doi:10.1515/ract-2019-3154
Qaim, S. M., Sudár, S., Scholten, B., Koning, A. J., and Coenen, H. H. (2014). Evaluation of excitation functions of 100Mo(p,d+pn)99Mo and 100Mo(p,2n)99mTc reactions: Estimation of long-lived Tc-impurity and its implication on the specific activity of cyclotron-produced 99mTc. Appl. Radiat. Isot 85, 101–113. doi:10.1016/j.apradiso.2013.10.004
Qaim, S. M., Tárkányi, F., and Capote, R. (2011). Nuclear data for the production of therapeutic radionuclides. IAEA Tech. Rep. Ser. No. 473, 145–375.
Rösch, F., Herzog, H., and Qaim, S. M. (2017). The beginning and development of the theranostic approach in nuclear medicine, as exemplified by the radionuclide pair 86Y and 90Y. Pharmaceuticals 10, 56. doi:10.3390/ph10020056
Singh, B., and Chen, J. (2021). Nuclear data sheets for A=64. Nucl. Data Sheets 178, 41–537. doi:10.1016/j.nds.2021.11.002
Skakun, Y., and Qaim, S. M. (2004). Excitation function of the 64Ni(α, p)67Cu reaction for production of 67Cu. Appl. Radiat. Isot. 60, 33–39. doi:10.1016/j.apradiso.2003.09.003
Smith, N. A., Bowers, D. L., and Ehst, D. A. (2012). The production, separation, and use of 67Cu for radioimmunotherapy: A review. Appl. Radiat. Isot. 70, 2377–2383. doi:10.1016/j.apradiso.2012.07.009
Stoll, T., Kastleiner, S., Shubin, N., Coenen, H. H., and Qaim, S. M. (2002). Excitation functions of proton induced reactions on 68Zn from threshold up to 71 MeV, with specific reference to the production of 67Cu. Radiochim. Acta 90, 309–313. doi:10.1524/ract.2002.90.6.309
Sudár, S., Cserpák, F., and Qaim, S. M. (2002). Measurements and nuclear model calculations on proton-induced reactions on 103Rh up to 40 MeV: Evaluation of the excitation function of the 103Rh(p, n)103Pd reaction relevant to the production of the therapeutic radionuclide 103Pd. Appl. Radiat. Isot. 56, 821–831. doi:10.1016/s0969-8043(02)00054-4
Szelecsényi, F., Blessing, G., and Qaim, S. M. (1993). Excitation functions of proton induced nuclear reactions on enriched 61Ni and 64Ni: Possibility of production of no-carrier-added 61Cu and 64Cu at a small cyclotron. Appl. Radiat. Isot. 44, 575–580. doi:10.1016/0969-8043(93)90172-7
Szelecsényi, F., Steyn, G. F., Dolley, S. G., Kovács, Z., Vermeulen, C., and Van der Walt, T. N. (2009). Investigation of the 68Zn(p,2p)67Cu nuclear reaction: New measurements up to 40 MeV and compilation up to 100 MeV. Nucl. Instrum. Meth. B 267, 1877–1881. doi:10.1016/j.nimb.2009.03.097
Takács, S., Aikawa, M., Haba, H., Komori, Y., Ditrói, F., Szűcs, Z., et al. (2020). Cross sections of alpha-particle induced reactions on natNi: Production of 67Cu. Nucl. Instrum. Meth. B 479, 125–136. doi:10.1016/j.nimb.2020.06.022
Tárkányi, F., Hermanne, A., Ignatyuk, A. V., and Capote, R. (2022). Upgrade of recommended nuclear cross section data base for production of therapeutic radionuclides. J. Radioanal. Nucl. Chem. 331, 1163–1206. doi:10.1007/s10967-022-08189-1
Tárkányi, F., Ignatyuk, A. V., Hermanne, A., Capote, R., Carlson, B. V., Engle, J. W., et al. (2019). Recommended nuclear data for medical radioisotope production: Diagnostic positron emitters. J. Radioanal. Nucl. Chem. 319, 533–666. doi:10.1007/s10967-018-6380-5
Thieme, S., Walther, M., Preusche, S., Rajander, J., Pietzsch, H. J., Lill, J. O., et al. (2013). High specific activity 61Cu via 64Zn(p,α)61Cu reaction at low proton energies. Appl. Radiat. Isot. 72, 169–176. doi:10.1016/j.apradiso.2012.11.007
Uddin, M. S., Kim, K., Nadeem, M., Sudár, S., and Kim, G. (2018). Measurements of excitation functions of α-particle induced reactions on natNi: Possibility of production of the medical isotopes 61Cu and 67Cu. Radiochim. Acta 106, 87–93. doi:10.1515/ract-2017-2837
Uddin, M. S., Sudár, S., Spahn, I., Shariff, M. A., and Qaim, S. M. (2016). Excitation function of the 60Ni(p,γ)61Cu reaction from threshold to 16 MeV. Phys. Rev. C 93, 044606. doi:10.1103/physrevc.93.044606
Wallhaus, T. R., Lacy, J., Whang, J., Green, M. A., Nickles, R. J., and Stone, C. K. (1998). Human biodistribution and dosimetry of the PET perfusion agent copper-62-PTSM. J. Nucl. Med. 39, 1958–1964.
Wadas, T. J., Wong, E. H., Weisman, G. R., and Anderson, C. J. (2007). Copper chelation chemistry and its role in copper radiopharmaceuticals. Curr. Pharm. Des. 13, 3–16. doi:10.2174/138161207779313768
Williams, H. A., Robinson, S., Julyan, P., Zweit, J., and Hastings, D. (2005). A comparison of PET imaging characteristics of various copper radioisotopes. Eur. J. Nucl. Med. Mol. Imag. 32, 1473–1480. doi:10.1007/s00259-005-1906-9
Keywords: radionuclides of copper, theranostic approach, nuclear reaction cross section, nuclear model calculation, standardisation of nuclear data, thick target yield
Citation: Hussain M, Qaim SM, Spahn I, Aslam MN and Neumaier B (2023) Copper radionuclides for theranostic applications: towards standardisation of their nuclear data. A mini-review. Front. Chem. 11:1270351. doi: 10.3389/fchem.2023.1270351
Received: 31 July 2023; Accepted: 08 September 2023;
Published: 29 September 2023.
Edited by:
Susanta Lahiri, Saha Institute of Nuclear Physics (SINP), IndiaReviewed by:
Zoltan Szucs, Institute for Nuclear Research, Debrecen, HungaryCopyright © 2023 Hussain, Qaim, Spahn, Aslam and Neumaier. This is an open-access article distributed under the terms of the Creative Commons Attribution License (CC BY). The use, distribution or reproduction in other forums is permitted, provided the original author(s) and the copyright owner(s) are credited and that the original publication in this journal is cited, in accordance with accepted academic practice. No use, distribution or reproduction is permitted which does not comply with these terms.
*Correspondence: Syed M. Qaim, cy5tLnFhaW1AZnotanVlbGljaC5kZQ==