- 1Department of Chemistry, Korea Advanced Institute of Science and Technology (KAIST), Daejeon, Republic of Korea
- 2Center for Catalytic Hydrocarbon Functionalizations, Institute for Basic Science (IBS), Daejeon, Republic of Korea
The synthesis of heterobiaryl compounds holds significant value in organic chemistry due to their extensive range of applications. Herein, we report a highly efficient strategy for conducting C4-selective (hetero) arylation of pyridines using N-aminopyridinium salts. The reaction proceeds readily at room temperature in the presence of a base, thus eliminating the requirement for catalysts or oxidants. This method allows for the installation of various electron-rich (hetero) aryl groups on pyridines, resulting in the streamlined synthesis of highly valuable C4-(hetero) aryl pyridine derivatives, which are otherwise challenging to acquire via conventional methods. This simple and straightforward method will facilitate access to a range of heterobiaryl compounds thereby promoting their application in various scientific disciplines.
Introduction
Pyridine, a prominent component in the realms of agrochemicals, pharmaceuticals, and functional materials (Vitaku et al., 2014; Zhong, et al., 2014), is often a key constituent of N-heterobiaryl scaffolds due to their rigid and adaptable three-dimensional structures (Wu and Chan, 2006; Ghoteimi et al., 2023; Yang et al., 2016; Lee, H. et al., 2019; Jo, W. et al., 2020; Li, H. et al., 2021; Shao et al., 2021; Nguyen, N. H. et al., 2022; Takahashi, F., and Yorimitsu, H., 2023). These structures are frequently used in the fabrication of therapeutic agents or as ligands for metal catalyst complexes. For instance, LJH685, a selective RSK inhibitor, has been designed to treat triple-negative breast cancer (TNBC) (Cui et al., 2022), and Etoricoxib (Chauret et al., 2001), a selective cyclooxygenase-2 inhibitor, has been developed for its anti-inflammatory effects. As a result, the synthesis of arylated pyridine scaffolds has become a hot topic, with a majority of strategies employing transition metal catalysts (Li et al., 2014; Lee, D. et al., 2015; Zuo et al., 2015; Jiao et al., 2016; Lutz et al., 2016; Jia et al., 2018; Gu, X. et al., 2022). The Baran group, for example, introduced a Minisci-type Ag-catalyzed arylation of pyridine and other heteroarenes (Seiph et al., 2010) (Scheme 1A). In addition, modified versions of the Suzuki-Miyaura reaction using heteroaryl halides and Pd catalysts have been reported (Ichikawa et al., 2017) (Scheme 1B). However, despite their synthetic versatility, these methods demand high temperatures and exhibit limited functional group tolerance. The advent of photocatalyzed reactions utilizing diazonium salts (Bartolomeu et al., 2019) and diaryliodonium salts (Tobisu et al., 2013) as aryl radical precursors marked a significant advance (Scheme 1C). Although these reactions are achievable at room temperature, the poor site selectivity of pyridine continues to pose a challenge.
Recently, pyridinium salts have emerged as versatile synthetic tools for pyridine functionalization, delivering mild conditions and superior site selectivity (Kim et al., 2021; Shin et al., 2021; Choi et al., 2022; Kim et al., 2022a; Kim et al., 2022b; Kim et al., 2022c). As pyridine surrogates, N-substituted pyridinium salts have bolstered the development of numerous visible-light induced reactions where radicals are produced via photocatalysts or electron donor-acceptor (EDA) complex formations. These radicals initiate a hydrogen atom transfer (HAT) or react with unsaturated carbon species, giving rise to complex radical species that can be sequestered in the pyridinium salts (Kim et al., 2019; Lee et al., 2020; Mathi et al., 2020). Of particular interest is the site-selective functionalization controlled by the N-substituents attached to the pyridinium salts (Jung et al., 2019). Beyond radical pathways, two-electron pathways have also been investigated, with pyridine or other heteroarene salts acting as electrophiles, demonstrating impressive reactivity and site selectivity (Okamoto et al., 1963; Okamoto et al., 1966; Lemire et al., 2004; Donohoe et al., 2009; Chupakhin et al., 2017). While one reference exists on pyridine C4 selective arylation using Grignard reagents, there are inherent compatibility challenges with various functional groups during the arylation process. As a result, the substrate scope is constrained, with only a single example reported (Katritzky and Beltrami, 1979). Despite these advances, the direct cross-coupling of pyridinium salts with other (hetero) arenes under mild conditions is still an underexplored field. Motivated by the versatile reactivity and excellent selectivity of N-substituted pyridinium salts, we aimed to design a method for C4-selective (hetero) arylation of pyridine under mild reaction conditions. We hypothesized that electron-rich (hetero) arenes, such as indoles and naphthol, would undergo nucleophilic addition to electrophilic pyridinium salts, leading to the formation of N-(hetero) biaryl compounds incorporating pyridine. This new approach enables the synthesis of a variety of N-(hetero) biaryl building blocks, known to be invaluable scaffolds in the sphere of medicinal chemistry (Marchais-Oberwinkler et al., 2008; Guo et al., 2011; Tsuji, T. et al., 2020; Yuan et al., 2020; Chaudhary, 2021).
Result and discussion
First, we conducted a comprehensive screening of reaction conditions to facilitate the incorporation of an indole moiety onto pyridine, employing pyridinium salt 1a and 5-methoxyindole 2a. We assessed the efficiency of various organic and inorganic bases (Table 1, entries 1–5). Cs2CO3 failed to show any significant conversion, while DBU and NaOtBu generated moderate yields. Notably, alongside the desired product 3b, we also detected minor side product N1, which exhibited a bond formation between the nitrogen of the indole and the C4 position of the pyridine. Specifically, side product 3b′ resulted from an additional nucleophilic addition of the free N–H from the desired product, 3b, to another pyridinium salt. When a stronger base, NaH, was used, the yield of the desired product 3b was slightly improved. Prior research has suggested that the choice of base cation can affect the reactivity and selectivity (Kobayashi et al., 2015; Chen and Wu, 2017). To investigate the potential influence of base cations on our reaction, we further scrutinized various tert-butoxide species with differing cations, due to the similar conversion but milder basicity of NaOtBu in comparison with NaH (entries 5 and 6). Remarkably, LiOtBu yielded a result similar to NaOtBu but demonstrated significantly superior selectivity for the indole C3 position. Subsequently, we explored the effects of ratio of 2a and LiOtBu (entries 7–13). A lower base quantity resulted in a decreased yield, and it was concluded that an optimal condition involved the use of 2.0 equiv of base (entry 10). When 2.0 equiv of 2a was used, the yield significantly improved (entry 13). This enhancement can be attributed to the unfavorable formation of 3b′ in the presence of excess 2a. Additionally, we evaluated other solvents with varying polarities, but DMSO proved to be superior (entries 14 and 15). Without the base, the reaction did not proceed (entry 16). Considering these optimization results, we selected entry 13 as the optimal condition for the introduction of the indole group at the pyridine C4 position.
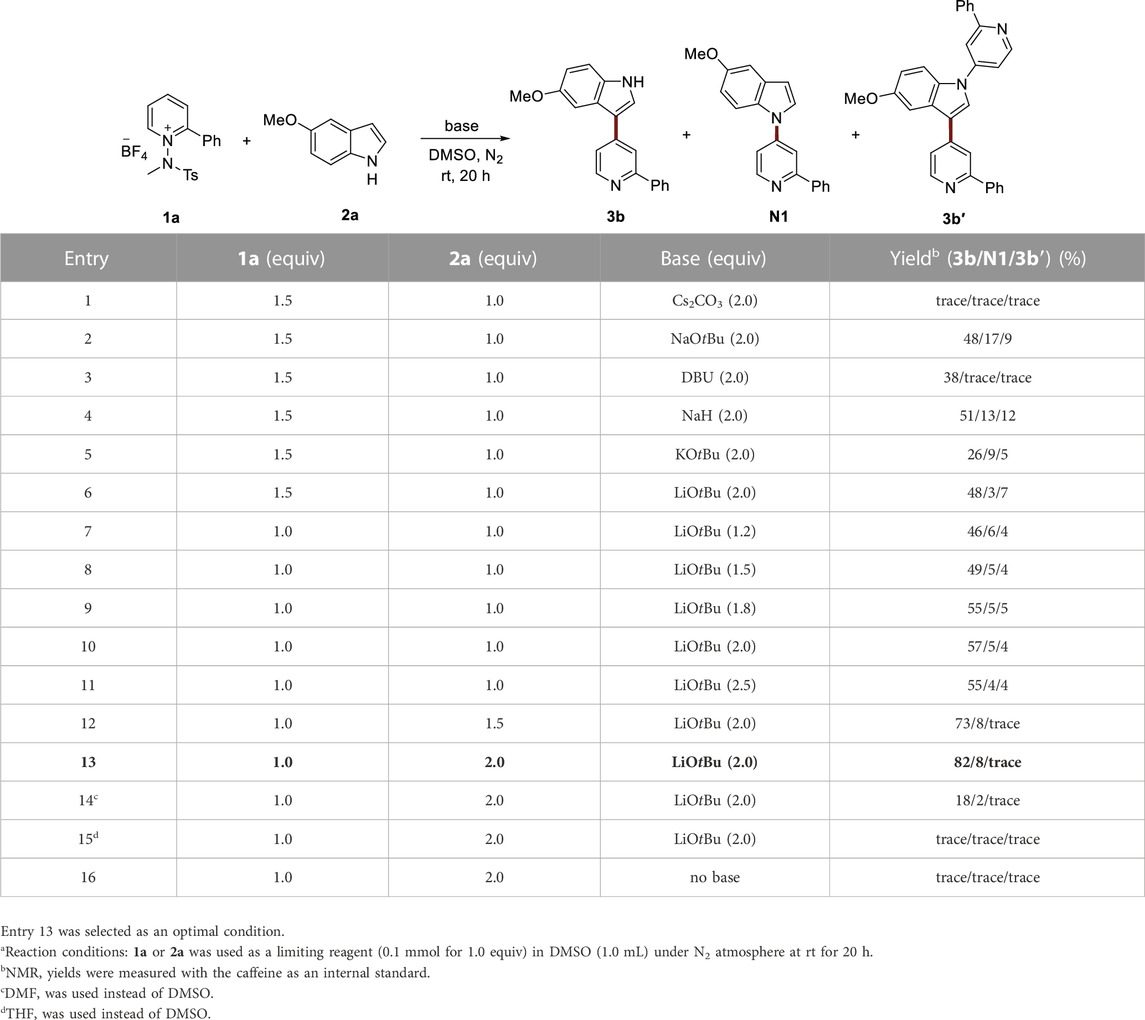
TABLE 1. Optimization of reaction conditions.a
Utilizing the optimized conditions for heteroarylations, we explored the scope of the reaction by including various functional groups on indoles (Table 2). The reactions showed consistent performance across a variety of indoles, from simple indoles to those substituted with electron-donating groups, such as methoxy, and methyl groups (3a–3e). Various halogen groups, spanning from iodo to fluoro, resulted in satisfactory conversions in the reaction (3f–3i). Electron-withdrawing groups, including nitro (3j), cyano (3k), amide (3l), and aldehyde (3m) groups, were successfully accommodated under the reaction conditions, leading to good conversions. Remarkably, even in the presence of a strong base, the hydroxyl group (3n) yielded a significant output. We also tested azaindole (3o) and pyrrolopyrimidine (3p), both of which are common derivatives of indoles found in medicinal chemistry. Pleasingly, the reactions proceeded well, highlighting the applicability of this method for the synthesis of these vital indole derivatives.
Subsequently, we systematically evaluated a series of pyridinium salts with diverse substituents to examine their reactivity and suitability with the established reaction conditions. The utilization of unsubstituted pyridinium salts resulted in comparatively lower yields (3q) than when 2-substituted pyridinium salts were used. When pyridinium salts carried an aryl substituent, the reaction progressed efficiently, suggesting that the aryl group present on the pyridinium salts enhances the reaction (3r–3t). The incorporation of other aryl groups, such as thiophene (3u) and pyridine (3v), into the pyridinium salt successfully yielded the corresponding products. We found that the reaction proceeded successfully when using C2-ester pyridinium salt 3w. Additionally, we expanded the applicability of our method by facilitating reactions with quinolinium salt (3x). When we used a pyridinium salt derived from a pharmaceutical compound, vismodegib, the reaction produced the desired product 3y in 40% yield.
We discovered that 2-naphthol could be effectively installed at the C4 position of pyridine in the presence of a base. Following the screening of reaction conditions using pyridinium salt 1a and 2-naphthol 4a as model substrates, we identified an optimized reaction condition that led to high conversion yields towards compound 5a (see Supplementary Table S1 for details). In the naphthol scope (Table 3), we noted a promising tolerance towards a variety of functional groups. The reaction progressed smoothly for substrates containing electron-donating groups such as methyl and methoxy groups (5a–5e). Halogen groups, including bromo (5f), chloro (5g), and fluoro (5h), were also compatible with the reaction conditions. Furthermore, electron-withdrawing groups, like cyano, amide, ester, ketone, and aldehyde groups, resulted in excellent conversion (5i–5m). The nucleophilic alcohol group also showed favorable conversion (5n). Motivated by this broad functional group tolerance, we extended our investigation to other heteroarenes as naphthol derivatives (5o–5r). Pleasingly, the reactions involving quinoline (5o), isoquinoline (5p), and benzothiophene (5r) proceeded well. However, benzofuran (5q) exhibited lower compatibility with the reaction conditions. Within the pyridine scope, improved conversion was observed with pyridine salts bearing a phenyl group compared to simple pyridine salts (5s–5v). Pyridinium salts substituted with thiophene (5w) and pyridine (5x) resulted in high conversions. Pleasingly, excellent yields were observed with the pyridinium salt carrying an ester group (5y) and the quinolinium salt (5z). Furthermore, a promising conversion was demonstrated when using the pyridinium salt derived from vismodegib (5aa), indicating the efficiency of this method in introducing a naphthol moiety even in the late stage of complex, pyridine-containing molecules.
To further elucidate the reaction mechanism, we conducted several control experiments. When the hydroxyl group of 2-naphthol was protected with an acyl group, the desired product was not observed, implying that the deprotonation of the hydroxyl group is a critical step (Scheme 2A). Blocking the C1 position of 2-naphthol with a methyl group halted the reaction. This evidence, together with the synthesis of 5e, distinctly indicates that under the optimal reaction conditions, the most reactive site of 2-naphthol towards the pyridinium salt is the C1 position (Scheme 2B). In the context of indoles, when N-methylated indole was used as a substrate, no reaction was observed, highlighting that a free N–H is vital for the reaction to proceed (Scheme 2D). When the C3 position of indole was blocked, the only significant product observed was 3z, which resulted from the nucleophilic attack of nitrogen from 2ab on the pyridinium salt (Scheme 2E). The addition of a radical scavenger, (2,2,6,6-tetramethylpiperidine-1-oxyl) (TEMPO) did not inhibit reactivity, suggesting that the reaction proceeded via an ionic pathway (Schemes 2C, F).
Given the experimental evidence, a plausible reaction mechanism for the arylation of pyridine with indole is depicted in Scheme 3. The reaction commences with the base-induced deprotonation of indole, followed by nucleophilic addition to the pyridinium salt 1. This addition is succeeded by the base-assisted aromatization of the pyridine moiety, leading to the release of a tosyl amine anion. Intriguingly, this anionic tosyl amine could potentially function as a base for the rearomatization of indole. Upon completion of the reaction, the reaction mixture is subjected to mild acidic workup, leading to the formation of the desired product 3. A similar pathway is expected for naphthol and is outlined in Supplementary Scheme S1.
Conclusion
In summary, we have developed a mild and selective nucleophilic (hetero) arylation of pyridine with exclusive C4 selectivity at the pyridine ring. This method employs N-aminopyridinium salts as electrophiles, enabling the facile and efficient synthesis of (hetero) arylated pyridine derivatives incorporating a wide range of aromatic functionalities. With the assistance of a base, indoles, naphthols, and their respective heteroarene derivatives can undergo nucleophilic addition onto the pyridine, eliminating the need for additional protection or deprotection steps. Interestingly, these nucleophilic (hetero) arenes exhibit unique and selective reactivity when engaging with the pyridinium salt. This selective behavior allows for meticulous control over the regiochemistry of the arylation reaction, facilitating the introduction of (hetero) aryl groups at the C4 position of pyridine. Given the observed extensive functional group tolerance, the developed protocols have the potential to yield a vast array of heterobiaryl scaffolds, which are indispensable building blocks in medicinal chemistry. These insights will contribute to the ongoing evolution of synthetic methods, simultaneously unlocking new possibilities for the discovery and development of innovative pharmaceutical compounds.
Data availability statement
The original contributions presented in the study are included in the article/Supplementary Material, further inquiries can be directed to the corresponding author.
Author contributions
KK: Investigation, Methodology, Writing–original draft, Writing–review and editing. EY: Investigation, Methodology, Writing–review and editing. SH: Conceptualization, Funding acquisition, Project administration, Resources, Supervision, Visualization, Writing–original draft, Writing–review and editing.
Funding
This research was supported financially by Institute for Basic Science (IBS-R010-A2).
Conflict of interest
The authors declare that the research was conducted in the absence of any commercial or financial relationships that could be construed as a potential conflict of interest.
Publisher’s note
All claims expressed in this article are solely those of the authors and do not necessarily represent those of their affiliated organizations, or those of the publisher, the editors and the reviewers. Any product that may be evaluated in this article, or claim that may be made by its manufacturer, is not guaranteed or endorsed by the publisher.
Supplementary material
The Supplementary Material for this article can be found online at: https://www.frontiersin.org/articles/10.3389/fchem.2023.1254632/full#supplementary-material
References
Bartolomeu, A. A., Silva, R. C., Brocksom, T. J., Noel, T., and de Oliveira, K. T. (2019). Photoarylation of pyridines using aryldiazonium salts and visible light: An EDA approach. J. Org. Chem. 84, 10459–10471. doi:10.1021/acs.joc.9b01879
Chaudhary, A. (2021). Recent development in the synthesis of heterocycles by 2-naphthol-based multicomponent reactions. Mol. Divers. 25, 1211–1245. doi:10.1007/s11030-020-10076-4
Chauret, N., Yergey, J. A., Brideau, C., Friesen, R. W., Mancini, J., Riendeau, D., et al. (2001). In vitro metabolism considerations, including activity testing of metabolites, in the discovery and selection of the COX-2 inhibitor Etoricoxib (MK-0663). Bioorg. Med. Chem. Lett. 11, 1059–1062. doi:10.1016/s0960-894x(01)00135-4
Chen, J., and Wu, J. (2017). Transition-metal-free C3 arylation of indoles with aryl halides. Angew. Chem. Int. Ed. 56, 3951–3955. doi:10.1002/anie.201612311
Choi, W., Kim, M., Lee, K., Park, S., and Hong, S. (2022). C4-Selective C–H borylation of pyridinium derivatives driven by electron donor-acceptor complexes. Org. Lett. 24, 9452–9457. doi:10.1021/acs.orglett.2c03882
Chupakhin, O. N., Shchepochkin, A. V., and Charushin, V. N. (2017). Atom- and step-economical nucleophilic arylation of azaaromatics via electrochemical oxidative cross C–C coupling reactions. Green Chem. 19, 2931–2935. doi:10.1039/c7gc00789b
Cui, Y. M., Li, W., Shen, T. Z., Tao, Y. X., Liu, B. Q., Li, X. L., et al. (2022). Design, synthesis and anti-breast cancer evaluation of biaryl pyridine analogues as potent RSK inhibitors. Bioorg. Med. Chem. Lett. 59, 128565. doi:10.1016/j.bmcl.2022.128565
Donohoe, T. J., Connolly, M. J., and Walton, L. (2009). Regioselective nucleophilic addition to pyridinium salts: A new route to substituted dihydropyridones. Org. Lett. 11, 5562–5565. doi:10.1021/ol902402v
Ghoteimi, R., Braka, A., Rodriguez, C., Cros-Perrial, E., Duvauchelle, V., Uttaro, J. P., et al. (2023). Second-generation CD73 inhibitors based on a 4,6-Biaryl-2-thiopyridine scaffold. ChemMedChem 18, e202200594. doi:10.1002/cmdc.202200594
Gu, X., Liu, K., Yang, L., Xie, C., Li, M., and Wang, J. J. (2022). Nickel-catalyzed enantioselective alpha-heteroarylation of ketones via C–F bond activation to construct all-carbon quaternary stereocenters. Chem. Sci. 13, 12498–12502. doi:10.1039/d2sc03409c
Guo, P., Joo, J. M., Rakshit, S., and Sames, D. (2011). C–H arylation of pyridines: High regioselectivity as a consequence of the electronic character of C–H bonds and heteroarene ring. J. Am. Chem. Soc. 133, 16338–16341. doi:10.1021/ja206022p
Ichikawa, T., Netsu, M., Mizuno, M., Mizusaki, T., Takagi, Y., Sawama, Y., et al. (2017). Development of a unique heterogeneous palladium catalyst for the suzuki-miyaura reaction using (Hetero)aryl chlorides and chemoselective hydrogenation. Adv. Synth. Catal. 359, 2269–2279. doi:10.1002/adsc.201700156
Jia, F., Yin, C., Zeng, Y., Sun, R., Ge, Y. C., Xu, D., et al. (2018). Mechanism of direct C–H arylation of pyridine via a transient activator strategy: A combined computational and experimental study. J. Org. Chem. 83, 10389–10397. doi:10.1021/acs.joc.8b01480
Jiao, J., Murakami, K., and Itami, K. (2016). Palladium-catalyzed C–H arylation of pyridines with aryl triflates. Chem. Lett. 45, 529–531. doi:10.1246/cl.160133
Jo, W., Baek, S. Y., Hwang, C., Heo, J., Baik, M. H., and Cho, S. H. (2020). ZnMe2-Mediated, direct alkylation of electron-deficient N-heteroarenes with 1,1-diborylalkanes: Scope and mechanism. J. Am. Chem. Soc. 142, 13235–13245. doi:10.1021/jacs.0c06827
Jung, S., Lee, H., Moon, Y., Jung, H.-Y., and Hong, S. (2019). Site-selective C–H acylation of pyridinium derivatives by photoredox catalysis. ACS Catal. 9, 9891–9896. doi:10.1021/acscatal.9b03367
Katritzky, A. R., Beltrami, H., and Sammers, M. P. (1979). Preparation of 4-aryl-ad 4-Heteroaryl-pyridines: Regiospecific nucleophilic attack γ to a quaternaryl nitrogen atom. J. Chem. Soc. Chem. Commun. 137. doi:10.1039/C39790000137
Kim, C., Jeong, J., Vellakkaran, M., and Hong, S. (2022a). Photocatalytic decarboxylative pyridylation of carboxylic acids using in situ-generated amidyl radicals as oxidants. ACS Catal. 12, 13225–13233. doi:10.1021/acscatal.2c04417
Kim, M., Koo, Y., and Hong, S. (2022b). N-functionalized pyridinium salts: A new chapter for site-selective pyridine C–H functionalization via radical-based processes under visible light irradiation. Acc. Chem. Res. 55, 3043–3056. doi:10.1021/acs.accounts.2c00530
Kim, M., Shin, S., Koo, Y., Jung, S., and Hong, S. (2022c). Regiodivergent conversion of alkenes to branched or linear alkylpyridines. Org. Lett. 24, 708–713. doi:10.1021/acs.orglett.1c04156
Kim, M., You, E., Park, S., and Hong, S. (2021). Divergent reactivity of sulfinates with pyridinium salts based on one-versus two-electron pathways. Chem. Sci. 12, 6629–6637. doi:10.1039/d1sc00776a
Kim, N., Lee, C., Kim, T., and Hong, S. (2019). Visible-light-induced remote C(sp3)–H pyridylation of sulfonamides and carboxamides. Org. Lett. 21, 9719–9723. doi:10.1021/acs.orglett.9b03879
Kobayashi, S., Yoo, W.-J., Nguyen, V. Q., and Guiteras Capdevila, M. (2015). Lithium tert-butoxide-mediated carboxylation reactions of unprotected indoles and pyrroles with carbon dioxide. Heterocycles 90, 1196–1204. doi:10.3987/com-14-s(k)94
Lee, D. J., Ko, D., and Yoo, E. J. (2015). Rhodium(II)-Catalyzed cycloaddition reactions of non-classical 1,5-dipoles for the formation of eight-membered heterocycles. Angew. Chem. Int. Ed. 54, 13715–13718. doi:10.1002/anie.201506764
Lee, H. E., Lee, M. J., and Park, J. K. (2019). Rapid base- and metal-free oxidative C-H cyclization for the synthesis of benzo[4,5]imidazo[1,2-a]pyridine derivatives. Asian J. Org. Chem. 8, 1659–1663. doi:10.1002/ajoc.201900347
Lee, K., Lee, S., Kim, N., Kim, S., and Hong, S. (2020). Visible-light-enabled trifluoromethylative pyridylation of alkenes from pyridines and triflic anhydride. Angew. Chem. Int. Ed. 59, 13379–13384. doi:10.1002/anie.202004439
Lemire, A., Grenon, M., Pourashraf, M., and Charette, A. B. (2004). Nucleophilic addition to 3-substituted pyridinium salts: Expedient syntheses of (-)-L-733,061 and (-)-CP-99,994. Org. Lett. 6, 3517–3520. doi:10.1021/ol048624n
Li, H., Tang, X., Pang, J. H., Wu, X., Yeow, E. K. L., Wu, J., et al. (2021). Polysulfide anions as visible light photoredox catalysts for aryl cross-couplings. J. Am. Chem. Soc. 143, 481–487. doi:10.1021/jacs.0c11968
Li, Y., Liu, W., and Kuang, C. (2014). Direct arylation of pyridines without the use of a transition metal catalyst. Chem. Commun. 50, 7124–7127. doi:10.1039/c4cc02546f
Lutz, J. P., Chau, S. T., and Doyle, A. G. (2016). Nickel-catalyzed enantioselective arylation of pyridine. Chem. Sci. 7, 4105–4109. doi:10.1039/c6sc00702c
Marchais-Oberwinkler, S., Kruchten, P., Frotscher, M., Ziegler, E., Neugebauer, A., Bhoga, U., et al. (2008). Substituted 6-Phenyl-2-naphthols. Potent and selective nonsteroidal inhibitors of 17β-hydroxysteroid dehydrogenase type 1 (17β-HSD1): Design, synthesis, biological evaluation, and pharmacokinetics. J. Med. Chem. 51, 4685–4698. doi:10.1021/jm800367k
Mathi, G. R., Jeong, Y., Moon, Y., and Hong, S. (2020). Photochemical carbopyridylation of alkenes using N-alkenoxypyridinium salts as bifunctional reagents. Angew. Chem. Int. Ed. 59, 2049–2054. doi:10.1002/anie.201913320
Nguyen, N. H., Oh, S. M., Park, C. M., and Shin, S. (2022). Ortho-selective C–H arylation of phenols with N-carboxyindoles under brønsted acid- or Cu(I)-Catalysis. Chem. Sci. 13, 1169–1176. doi:10.1039/d1sc06157g
Okamoto, T., Hirobe, M., Mizushima, C., and Ohsawa, A. (1963). Reaction of N-aminopyridinium derivatives. II. The reactions of 1-(N-acylalkylamino) pyridinium salt derivatives with cyanide ion, A new synthesis of primary amines. Chem. Pharm. Bull. 11, 780–785. doi:10.1248/cpb.11.780
Okamoto, T., Hirobe, M., and Ohsawa, A. (1966). Reaction of N-aminopyridinium derivatives. V. Syntheses of 1-(N-methylacetamido) alkylpyridinium salts and their reaction with cyanide ion. Chem. Pharm. Bull. 14, 518–523. doi:10.1248/cpb.14.518
Seiph, I. B., Su, S., Rodriguez, R. A., Fujiwara, Y., Sobel, A. L., Baran, P. S., et al. (2010). Direct C–H arylation of electron-deficient heterocycles with arylboronic acids. J. Am. Chem. Soc. 132, 13194–13196. doi:10.1021/ja1066459
Shao, Y.-D., Han, D.-D., Dong, M.-M., Yang, X.-R., and Cheng, D.-J. (2021). A one-pot stepwise approach to axially chiral quinoline-3-carbaldehydes enabled by iminium–allenamine cascade catalysis. Org. Chem. Front. 8, 605–612. doi:10.1039/d0qo01339k
Shin, S., Lee, S., Choi, W., Kim, N., and Hong, S. (2021). Visible-light-induced 1,3-aminopyridylation of [1.1.1]Propellane with N-aminopyridinium salts. Angew. Chem. Int. Ed. 60, 7873–7879. doi:10.1002/anie.202016156
Takahashi, F., and Yorimitsu, H. (2023). Stereoselective synthesis of tetraarylethylenes enabled by reductive anti-1,2-dimetallation of alkynes. Chem. Eur. J. 29, e202203988. doi:10.1002/chem.202203988
Tobisu, M., Furukawa, T., and Chatani, N. (2013). Visible light-mediated direct arylation of arenes and heteroarenes using diaryliodonium salts in the presence and absence of a photocatalyst. Chem. Lett. 42, 1203–1205. doi:10.1246/cl.130547
Tsuji, T., Tanaka, T., Tanaka, T., Yazaki, R., and Ohshima, T. (2020). Catalytic Aerobic Cross-Dehydrogenative Coupling of Azlactones en Route to α,α-Disubstituted α-Amino Acids. Org. Lett. 22, 4164–4170. doi:10.1021/acs.orglett.0c01248
Vitaku, E., Smith, D. T., and Njardarson, J. T. (2014). Analysis of the structural diversity, substitution patterns, and frequency of nitrogen heterocycles among U.S. FDA approved pharmaceuticals. J. Med. Chem. 57, 10257–10274. doi:10.1021/jm501100b
Wu, J., and Chan, A. S. C. (2006). P-phos: A family of versatile and effective atropisomeric dipyridylphosphine ligands in asymmetric catalysis. Acc. Chem. Res. 39, 711–720. doi:10.1021/ar0680015
Yang, C., Mehmood, F., Lam, T. L., Chan, S. L., Wu, Y., Yeung, C. S., et al. (2016). Stable luminescent iridium(III) complexes with bis(N-heterocyclic carbene) ligands: Photo-stability, excited state properties, visible-light-driven radical cyclization and CO2 reduction, and cellular imaging. Chem. Sci. 7, 3123–3136. doi:10.1039/c5sc04458h
Yuan, S., Chang, J., and Yu, B. (2020). Construction of biologically important biaryl scaffolds through direct C–H bond activation: Advances and prospects. Top. Curr. Chem. 378, 23. doi:10.1007/s41061-020-0285-9
Zhong, M., Peng, E., Huang, N., Huang, Q., Huq, A., Lau, M., et al. (2014). Discovery of functionalized bisimidazoles bearing cyclic aliphatic-phenyl motifs as HCV NS5A inhibitors. Bioorg. Med. Chem. Lett. 24, 5731–5737. doi:10.1016/j.bmcl.2014.10.057
Keywords: C-H (hetero) arylation, pyridinium salt, C4-selectivity, heterobiaryl, metal-free
Citation: Kim K, You E and Hong S (2023) Nucleophilic C4-selective (hetero) arylation of pyridines for facile synthesis of heterobiaryls. Front. Chem. 11:1254632. doi: 10.3389/fchem.2023.1254632
Received: 07 July 2023; Accepted: 17 August 2023;
Published: 01 September 2023.
Edited by:
Takashi Ohshima, Kyushu University, JapanReviewed by:
Tao Shi, The Scripps Research Institute, United StatesMatsubara Ryosuke, Kobe University, Japan
Jin Kyoon Park, Pusan National University, Republic of Korea
Copyright © 2023 Kim, You and Hong. This is an open-access article distributed under the terms of the Creative Commons Attribution License (CC BY). The use, distribution or reproduction in other forums is permitted, provided the original author(s) and the copyright owner(s) are credited and that the original publication in this journal is cited, in accordance with accepted academic practice. No use, distribution or reproduction is permitted which does not comply with these terms.
*Correspondence: Sungwoo Hong, hongorg@kaist.ac.kr