- 1Zigong First People’s Hospital, Zigong, Sichuan, China
- 2West China Hospital, Sichuan University, Chengdu, Sichuan, China
- 3Sichuan North Medical College, Nanchong, Sichuan, China
Lung cancer (LC) is one of the leading causes of high cancer-associated mortality worldwide. Non-small cell lung cancer (NSCLC) is the most common type of LC. The mechanisms of NSCLC evolution involve the alterations of multiple complex signaling pathways. Even with advances in biological understanding, early diagnosis, therapy, and mechanisms of drug resistance, many dilemmas still need to face in NSCLC treatments. However, many efforts have been made to explore the pathological changes of tumor cells based on specific molecular signals for drug therapy and targeted delivery. Nano-delivery has great potential in the diagnosis and treatment of tumors. In recent years, many studies have focused on different combinations of drugs and nanoparticles (NPs) to constitute nano-based drug delivery systems (NDDS), which deliver drugs regulating specific molecular signaling pathways in tumor cells, and most of them have positive implications. This review summarized the recent advances of therapeutic targets discovered in signaling pathways in NSCLC as well as the related NDDS, and presented the future prospects and challenges.
1 Introduction
Cancer is the main cause of death worldwide, and lung cancer (LC) is the disease with the highest mortality rate (Ferlay et al., 2018; Ferlay et al., 2019). According to histology, LC is generally divided into two categories: small cell lung cancer (SCLC) and non-small cell lung cancer (NSCLC). Here, NSCLC is the most common type of LC (85%), with high incidence rate and high mortality (Ferlay et al., 2019). Moreover, the average survival rate of patients is 10%–20% (Ferlay et al., 2018). Nowadays, targeted drugs for gene mutations and immune loci have become the preferred option for NSCLC patients, but the current clinical route of drug delivery still has major drawbacks, such as low drug utilization and significant side effects, which reduce the survival benefits for clinical patients (Hirsch et al., 2017; de Scordilli et al., 2022). In addition, there are many problems inherent in the traditional route of drug delivery. The bioavailability of oral and intravenous dosing is low, and these systemic dosing regimens can cause a variety of toxic reactions in the body, including severe vomiting, seizures, vasculitis, and even death, clearly doing more harm than good in low-risk stage IA NSCLC (NSCLC Meta-analysis Collabora tive Group, 2014; Argilés et al., 2023). Therefore, developing new therapeutic interventions which focus on more microscopic and detailed levels like the signaling pathways of disease onset to overcome these limitations is of great significance. Scientists have done a great deal of work in exploring the evolution of tumor cells based on specific molecular signals for drug treatment and targeted delivery. Targeted therapy has become a hot term in tumor treatment with a broad meaning that includes not only the targeting of drugs but also the precise delivery of drugs.
For the proliferation and invasion process of LC cells, abnormal cell signaling pathways, which exist complex regulatory mechanisms, are closely related to genetic mutations. Mutations of RAS gene are common in NSCLC, and most of them are KRAS mutations (Punekar et al., 2022). Mitogen-activated protein kinase (MAPK) is one of the major signals stimulated by RAS (Ferlay et al., 2018; Ferlay et al., 2019). The RAS rapid fibrosarcoma (RAF)-MAPK-extracellular signal-regulated kinase (ERK) pathway and phosphatidylinositol 3-kinase (PI3K)-protein kinase B (AKT) pathway control cell survival and proliferation in NSCLC (Ferlay et al., 2019). Epidermal growth factor receptor (EGFR) gene mutations are important in the development of NSCLC. It can increase EGFR expression when mutations in kinase, resulting in the functional upregulation of the EGFR pathway and uncontrolled proliferation of mutant tumor cells (Passaro et al., 2021). In malignant cells, vascular endothelial growth factor (VEGF) and VEGF receptor (VEGFR) promote cell proliferation, survival, and angiogenesis in, and inhibition of VEGF and VEGFR retards tumor growth (Niu et al., 2022). Also important in cell differentiation, proliferation, and cancer progression are the pathways NTRK/ROS1 and JAK-STAT (Lai and Johnson, 2010; Song et al., 2023). In summary, molecular signaling has a strong connection with tumor progression, which is also can modulate drug resistance. To fully understand the functions of these pathways, it is necessary to examine their upstream and downstream node. As evidence accumulates, strategies that target these pathways may hold promise for NSCLC treatment. However, the complexity of signaling makes it difficult to understand the complete regulatory pathway of a particular signaling target in tumor cells. At the same time, all mutated genes may become therapeutic targets, while there are still many unknown mutated genes, so that continuing in-depth screening of mutated genes has a positive effect on drug development.
Accompanied by the discovery of more and more signaling pathways, a great victory has been achieved in the precise molecular therapy of NSCLC, which established in regulating signaling pathways to overcome the drug resistance. For example, activation of NF-κB/Bcl-2/Snail pathway increases chemotherapy resistance in NSCLC, and thus targeted drug delivery of this pathway would behave with good specificity and pharmacokinetic characteristics which could inhibit tumor cell proliferation (McCubrey et al., 2008; Chen et al., 2015; Asati et al., 2016). Targeted drugs can reduce resistance to chemotherapy drugs, but are prone to cause new genetic mutations during treatment, leading to resistance to targeted drugs (Cabanos and Hata, 2021). Targeting EGFR tyrosine kinase inhibitors (EGFR-TKIs) for NSCLC promotes the emergence of acquired drug resistance, a major barrier to EGFR-targeted therapy (Johnson et al., 2022). EGFR resistance mechanisms are mainly classified as dependent resistance and non-dependent resistance. Dependent resistance mechanisms include T790M mutation, C797 mutation and G796R mutation, while non-dependent resistance mechanisms are divided into mesenchymal-epithelial transition (MET) factor amplification, (human epidermal growth factor receptor 2) HER2 amplification and gene fusion (Passaro et al., 2021; Nie et al., 2022). Apart from that, the PI3K/AKT/mTOR signaling pathway can activate EGFR and mutate among various malignant tumors, including leukemia and NSCLC (Yan et al., 2021; Chen et al., 2022). Studies have confirmed that the knockdown of miR-126, miR-203, and miR-34a genes can regulate drug resistance through PI3K/AKT signaling pathway (Zhong et al., 2010; Garofalo et al., 2011). It has to be acknowledged that some unknown signaling pathways may have direct or indirect effects of the drug resistance.
In addition to the drug resistance of cancer cells, the positive and negative role of the signaling molecules in the immune system in drug therapy is also being paid attention to. Immune-related regulators play key roles in autoimmunity, self-tolerance, and the malignant microenvironment, such as the co-suppressor receptor programmed death 1 (PD-1) and its ligand (PD-L1) (Reck et al., 2022). Furthermore, there is substantial evidence that cancer cells may use these immunomodulatory factors to evade the immune response (Muenst et al., 2015). PD-1/PD-L1 pathway immunologic drugs have been widely used in the treatment of NSCLC (Suresh et al., 2018; Reck et al., 2022). At the same time, immunotherapeutic drugs, when applied to the human body, are distributed to various tissues and organs throughout the body and cannot achieve precise focal targeting, thus causing a series of immune-related adverse reactions, which greatly hinders their clinical application potential (Jing et al., 2021; Zhou et al., 2023). To overcome these problems and improve patient outcomes, nanoparticles (NPs) with penetrating and slow release properties have been proposed to successfully treat drug-resistant cancer cells of NSCLC in vitro or in vivo models (Palazzolo et al., 2018; Patra et al., 2018; Wang et al., 2018). The results of these studies have stimulated the interest of researchers in nanomaterials, and more research is still in progress.
The emergence of drug resistance and apparent toxicity is currently an important reason for the unsustainable treatment of NSCLC patients. NPs are endowed with special functions due to their microstructure, which bring more hope to address these problems. In particular, the modified NPs have the advantage of targeting and carrying multiple drugs. Nanotechnology requires multidisciplinary cooperation, involving various types of NPs as well as new nanodevices and applications of nanomaterials in different fields (Molina et al., 2008; Sung et al., 2021). NPs have a wide surface area and can be modified by bonding or encapsulation (Rosell et al., 2020). As carriers of antineoplastic drugs, NPs have greatly improved efficacy, safety, stability and pharmacokinetics of drugs (Naylor et al., 2016; Stater et al., 2021; Haider et al., 2022; Tian et al., 2022; Detappe et al., 2023; Fang et al., 2023; Nguyen et al., 2023). The researches on tumor targeted therapy via NPs focus on the size and the impact of encapsulation techniques on the bioavailability of drugs in vivo (Raju et al., 2021; Ezhilarasan et al., 2022; Huang et al., 2022). Biocompatible nanocarriers can be tailored to tumor characteristics to improve their physical and chemical properties, permeability, and metabolism, such as the smaller structures can penetrate tissue barriers more easily (Seeta Rama Raju et al., 2015). Furthermore, researchers can limit the uptake of drugs in healthy tissues by wrapping appropriate NPs to enhance drug targeting, thereby shield normal cells from the cytotoxic effects of anticancer drugs, and reduce adverse drug reaction (Kalyane et al., 2019; Haider et al., 2020; Duan et al., 2022; Haider et al., 2022).
Nano-based drug delivery systems (NDDS) formed by NPs have become a hot issue for research. NDDS has a wide range of promising applications in NSCLC treatment and has the potential to save patients’ lives. Targeted therapy aiming at mutated genes and immune targets is of epoch-making significance in NSCLC treatment. So far, the abundant studies on signaling targets based on NDDS have performed well in the delivery of targeted and immune drugs, and the mechanism is shown in Figure 1. NDDS is a new therapeutic concept, which is theoretically well synergistic with the drug itself, providing benefits to patients, and makes the goal of long-term coexistence with malignancy the technical and theoretical basis. It will break through the limitations of traditional treatments and promotes NSCLC patients to live longer.
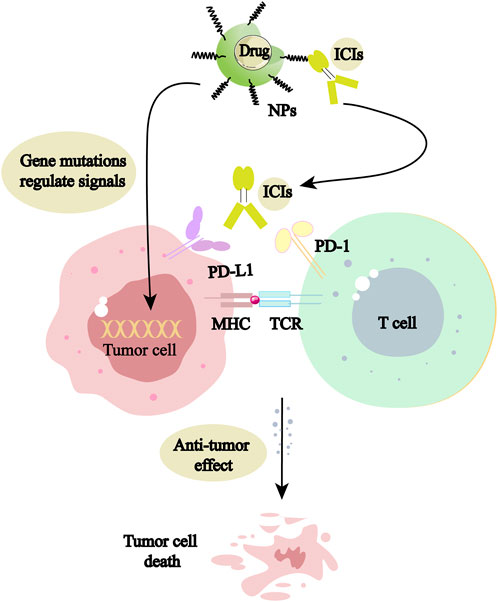
FIGURE 1. NPs can be used as delivery vehicles for mutation-targeting drugs and immune checkpoint inhibitors (ICIs), acting directly on tumor cells or activating living immune cells, respectively, to promote tumor cell death.
In this paper, we summarize the results achieved in recent years regarding the discovery of therapeutic targets in signaling pathways of NSCLC and related NDDS, and present the future prospects and challenges.
2 Strengths of nanodrug in NSCLC treatment
The pathogenesis of NSCLC is mediated by multiple intercellular molecular signaling pathways, and the targeted therapeutically relevant signaling targets are also receiving more attention. Gene-targeted therapy is widely used in the treatment of NSCLC, where EGFR classical mutations and KRAS G12C/D/V occur more frequently, while ROS1 rearrangements, RET rearrangements, NTRK fusions, MET14 exon skipping mutation, BRAF V600E mutations are relatively infrequent. They can be classified as “rare” mutations, but all of them have corresponding targeted drugs and should not be ignored (Harada et al., 2023). However, the physicochemical properties of these targeted drugs are always poor. Besides, the complex multi-order biological barriers in the body often lead to treatment that does not achieve the desired efficacy and also brings certain side effects on the organism (Boolell et al., 2015). More seriously, some side effects can even hasten the patient’s death.
In the immune system, T cell-mediated cellular immunity is the “main legion” for tumor eradication, where T cell activation is required to exert anti-tumor effects (Tanaka and Sakaguchi, 2017; Liu et al., 2020). But some “cunning” tumor cells can bypass immune surveillance and grow uncontrollably, thus endangering the health of the body. These tumor cells manipulate the immune cells by using the characteristics of immune checkpoints, and express PD-L1 protein on the surface, which causes T cells to lose the activity of killing tumor cells, thus realizing the immune escape (Jiang et al., 2019; Kim et al., 2019; Liu Z. et al., 2021). Immune checkpoint inhibitors (ICIs) can effectively overcome tumor immune evasion, and antagonists targeting immune checkpoint ligands can effectively activate tumor-specific T cells (Zhang et al., 2020; Liu et al., 2022). But traditional immune checkpoint blockade therapies have disadvantages, such as low immunogenicity, weak targeting, easy drug resistance, and accidental cytokine storms (Vincent et al., 2022). NDDS can effectively enhance ICIs’ blocking efficiency. At the same time, it can achieve synergistic tumor treatment in combination with other therapeutic tools (Vincent et al., 2022).
NDDS can control the distribution and metabolic behavior at different levels of tissues, cells, and organelles by improving the stability and physicochemical properties of drugs, as well as by overcoming multi-level biological barriers (Chen et al., 2014; Wang J. et al., 2021). Similarly, it has significant implications for the regulation of drug resistance (Vincent et al., 2022). Based on the anatomical and pathophysiological differences between normal and tumor cells, the NPs have strong enhanced permeability and retention (EPR) effect, as shown in Figure 2 (Ikeda-Imafuku et al., 2022; Wu et al., 2023). Nanocarriers can be divided into natural polymer and synthetic polymer materials, most of which have excellent biocompatibility, stability, safety, non-toxicity and modifiability, mainly including virus-like particles, poly lactic glycolic acid (PLGA), liposomes, dendrimers, metal organic framework compounds, as shown in Figure 3 (Guo and Jiang, 2020; Ji et al., 2023). Meanwhile, many biomaterials are inherently targeted, so that nanomaterial delivery systems are always partnered with nucleic acid drugs (Zhang et al., 2013). Due to the emergence of drug resistance, targeting related signaling pathways based on nanomaterials has become a new direction to treat NSCLC in recent years, as shown in Table 1.
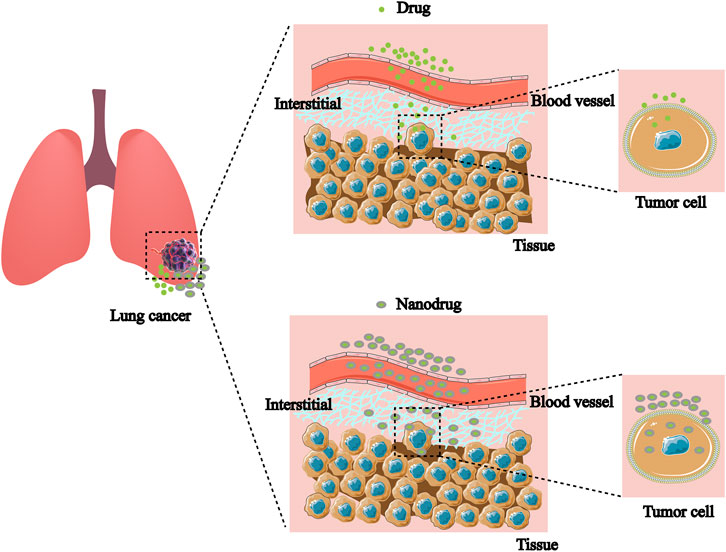
FIGURE 2. Nanodrugs are more likely to break through cellular and tissue barriers to accumulate in target cells than non-nanodrugs.
3 Nanodrug targeted epidermal growth factor receptor in NSCLC
The EGFR is one of the most common mutation driver oncogenes. Among Asian female nonsmokers with NSCLC, the mutation rate was as high as 59.4%, with exon 19 deletions and L858R point mutations located in the receptor tyrosine kinase (RTK) domain accounting for 90% of mutations (Li et al., 2016; Bílek et al., 2019). The stimulation of MAPK and PI3K is highly associated with increased cancer risk, and the downstream signaling pathways are associated with cell proliferation, metastasis, and drug resistance (Villaruz et al., 2013; Ciuffreda et al., 2014). Drugs targeting the EGFR have been developed rapidly, such as gefitinib, afatinib, and osimertinib (Yang et al., 2020; Lai-Kwon et al., 2021; Li et al., 2022; Liam et al., 2023). Despite the significant effect of initial treatment, patients often develop acquired resistance after a period of time, by mechanisms including dependence or non-dependence on the EGFR pathway (Taniguchi et al., 2019; Chmielecki et al., 2023). To solve these problems, NPs with special properties such as slow release have been shown initial success (Huang et al., 2022).
The latest monotherapies to address resistance to EGFR-TKIs mainly include nanoconjugates viral delivery, nucleic acid therapy, and targeted EGFR-TKIs loaded in nanostructures. Viruses as natural carrier materials take advantage of the biochemical and physical properties, such as solubility and nanometer size, making them an important choice for NDDS (Sainsbury and Steinmetz, 2023). Arrieta’s team constructed a novel type of magnetic lentivirus that can infect EGFR-TKIs-resistant cells in the model and realize microscale RNA interference (RNAi) by inhibiting EGFR expression, causing apoptosis of drug-resistant cells (Song et al., 2009; Li et al., 2021). In addition, pH affects the effect of nanocarriers mainly by microenvironment/nanomaterial surface charge interconversion, tumor penetration size, and swelling or disintegration upon drug release (Song et al., 2008). The lactate accumulation method was used to design lactate-responsive vectors containing lactate oxidase (LOX) and AAV2, which reduced pH and viral infection, as well as increased apoptosis when both LOX and lactate were presented in the formulation (Tseng et al., 2018).
Nanocarriers can carry nucleic acids through the phospholipid bilayer of cell membranes, which promotes easier drug accumulation within the target cells (Bishop et al., 2015). Li et al. reported that exosomes loaded with small interfering RNA (siRNA) were used for the suppression of NSCLC (Li et al., 2021). Cholesterol is used to anchor ligands targeting EGFR onto secretions that load siRNA to silence the anti-apoptotic factor survivin. Cytoplasmic delivery of siRNA resolves the problem of endosomal capture and leads to effective gene knockdown, chemosensitization and tumor regression. In NSCLC patients, the knockdown of expression of selected appropriate targets restores sensitivity to EGFR-targeted drugs (Li et al., 2021). Thereby, the progress has been made in combining RNA nanotechnology with exon-delivery platforms, which can improve the targeting of cancer therapies.
The discovery of ligands that bind specifically to cancer cells is essential for NDDS delivery, and peptide binding to liposomes has been used to selectively deliver drugs to kill tumor cells with EGFR mutations (Song et al., 2008; Song et al., 2009). The study was performed by small peptides with phosphorylation sites (AEYLR, EYINQ, and PDYQQD), which were labeled with fluorescein isothiocyanate (FITC), in tumor cells of NSCLC with or without EGFR expression. It showed that AEYLR recognizes EGFR protein with high selectivity (Han et al., 2013). This confirmed that NDDS bound to AEYLP can accumulate more easily in tumor cells. Li et al. prepared lipid-containingNPs loaded with anti-EGFR DNA aptamer, which can make NDDS reach the target cells more easily by exploiting the specific binding of EGFR DNA aptamer, thus increasing the drug concentration and exerting better anti-tumor efficacy of targeted drugs (Li et al., 2017). Additionally, Han et al. loaded cyclosporine A and targeted drugs in a nanostructured poly (ethylene glycol)-poly (lactic acid) (PEG-PLA) and found a significant effect of cyclosporine A in reversing resistance to EGFR-TKIs (Han et al., 2018). Compared to EGFR-TKIs alone, NPs loaded with EGFR TKI not only reduce systemic toxicity but also improve intracellular delivery and increase bioavailability (Ahlawat et al., 2022). Upadhyay et al. demonstrated the efficiency of transferrin-modified PLGA thymoquinone NPs combined with gefitinib (NP-DUAL-3) in treating resistant NSCLC cells for the first time (Upadhyay et al., 2021). The results indicated that NP-DUAL-3 may restore the MET phenomenon, thereby making drug-resistant NSCLC cells re-sensitive to gefitinib. Therefore, the combination of NPs and gefitinib may be effective in treating NSCLC patients in the future.
4 Nanodrug targeted vascular endothelial growth factor receptor in NSCLC
VEGF is mainly secreted by vascular endothelial cells, as well as tumor microenvironment (TME) cells, such as tumor-associated macrophages (TAMs) (Hwang et al., 2020), tumor-associated neutrophils (TANs) (Guimarães-Bastos et al., 2022), mast cells (MCs) (Komi et al., 2020), myelogenous suppressor cells (MDSCs) (Mortezaee, 2021) and natural killer cells (NKs) (Eisinger et al., 2020). VEGF is the main mediator of tumor microangiogenesis and closely associated with the development and progression of NSCLC. In addition, VEGF stimulates regulatory immune cells by inhibiting antigen presentation, thus promoting immunosuppression of the TME. It is an important manifestation of VEGF’s involvement in immune regulation (Zhao Y. et al., 2022).
The binding of VEGF ligands to VEGFR-2 and the PI3K/AKT signaling pathway control the survival of endothelial cells (Wang et al., 2022). The activation of endothelial nitrogen monoxide (NO) synthase by c-Src and phospholipase C1 (PLC1), and the activation of prostacyclin synthase by Raf1-MEK1/2ERK1/2 lead to an increase of NO and PGI2 in endothelial cells, respectively (Socinski et al., 2018). This pathway is the core of endothelial cell proliferation. The upregulation of NO induced by VEGF may also participate in the generation and mobilization of endothelial progenitor cells (Aicher et al., 2003).
Kandasamy et al. explored the efficiency of poly (1-vinyl imidazole) (PVI) as an effective siRNA carrier for VEGF gene silencing (Kandasamy et al., 2020). They found that the individual PVI polymer was safe to the cells, and the polymer exhibited good internalization and effectively escaped the inner body, indicating that the vector may be a biocompatible system for gene therapy. In terms of silencing VEGF in tumors, the polymerase is more effective than free siRNA, and the silencing of VEGF leads to changes in the gene expression responsible for cancer cell proliferation and metastasis. Peptide silencing of VEGF can enhance the cytotoxicity of chemotherapy drug 5-fluorouracil, suggesting that it could be used as an adjuvant treatment strategy for cancer. Meanwhile, VEGF-targeted RNAi using poly-siRNA/tGC NPs in combination with chemotherapeutic agents can control tumor growth by increasing anti-angiogenic efficacy while minimizing toxicity and drug resistance (Kwak et al., 2017). Chemically polymerized siRNAs complexed with thiolated-glycol chitosan (psi (VEGF)/tGC) NPs mediated suppression of VEGF which exerted anti-tumor effects. Furthermore, the combination of bevacizumab can better perform the drug’s efficacy (Kim et al., 2017).
5 Nanodrug regulated PI3K/AKT/mTOR signaling pathway in NSCLC
The PI3K/AKT/mTOR pathway is vital in regulating cell growth and metabolism, which is significantly activated in NSCLC (50%–73%) (Papadimitrakopoulou, 2012). Meanwhile, persistent activation of this pathway can contribute to the development of resistance to anticancer therapy. PI3K is regulated by numerous upstream factors, such as HER2 (Tan, 2020). Under stress or ligand binding, AKT is readily activated to regulate the phosphorylation of phosphatidylinositol 4,5-bisphosphate (PIP2) to phosphatidylinositol 3,4,5-trisphosphate (PIP3) (Papadimitrakopoulou, 2012). Activation of AKT will cause changes in downstream signaling molecules, which can inhibit Bcl-2-associated death promoter (BAD) and Bcl-2-associated X protein (BAX), members of the Bcl-2 family, and promote apoptosis (Cantley, 2002). Activation of nuclear factor-κB (NF-κB) light chain enhancer plays a role in immune regulation and biological behavior (Sonenshein, 1997). Another important downstream pathway is the activation of protein kinase mTOR. The mTOR can cause activation of the eukaryotic translation initiation factor 4 complex, which subsequently promotes tumor development, regulates cell cycle, and inhibits cell apoptosis (Engelman et al., 2006).
In recent years, photothermal therapy (PTT) and photodynamic therapy (PDT) based on nanomaterials have made remarkable progress as an anticancer strategy, as shown in Figure 4 (Hou et al., 2020; Sun et al., 2020). Quantum dots (QDs) have good biocompatibility, solubility, excellent photostability, and easy surface functionalization properties, making them new promising nanomaterials (McHugh et al., 2018; Fan et al., 2019). Kuo et al. improved the efficiency of PDT by functionalizing nitrogen doped QDs with amino molecules (Kuo et al., 2018). Liu’s team has constructed a novel CoFe2O4 with excellent synergistic PTT/PDT properties, which can effectively inhibit NSCLC without significant toxicity. In addition, CoFe2O4 treatment also increases reactive oxygen species by regulating the PI3K/AKT pathway, thereby triggering cell death (Liu J. et al., 2021). Based on the current results, the safe, non-toxic NPs may have a positive effect on NSCLC treatment.
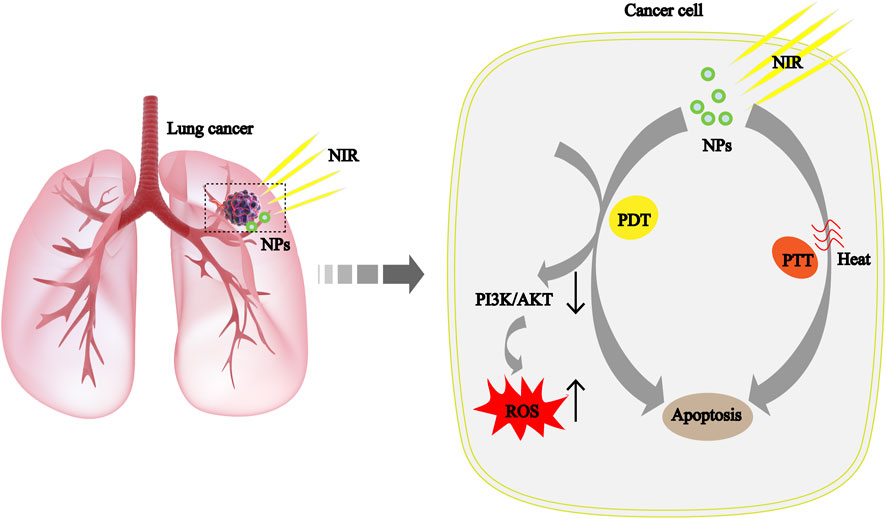
FIGURE 4. The mechanism of cancer cell apoptosis caused by the syergistic treatment of PTT and PDT based on NPs is explained scientifically in NSCLC. ROS, reactive oxygen species; NIR, near-infrared light.
6 Nanodrug regulated JAK2/STAT3 signaling pathway in NSCLC
Janus kinase (JAK) is a non-receptor tyrosine kinase that mediates the cascade activation of signaling molecules after cytokine and receptor binding (Johnson et al., 2018). The JAK family consists of four members (JAK1-3 and TYK2) (Lin et al., 2018). Abnormal JAK2 signaling plays an important role in solid tumors (Lee et al., 2006). The signal transducer and activator of transcription (STAT) protein family consists of seven members (STAT1-4, STAT5a, STAT5b, and STAT6) (Fang et al., 2017). STAT3 is a transcription factor that has been extensively studied in cancer. Generally, the JAK2/STAT3 pathway mediates signal transduction through a variety of cytokine receptors, such as interleukin-6 (IL-6) (Zhong et al., 1994) or granulocyte colony-stimulating factor (G-CSF) (Thorn et al., 2016), and EGFR (Shi and Kehrl, 2004), which makes JAK2 phosphorylate on its tyrosine residues (called autophosphorylation) and activate its kinase domain. This signaling pathway regulates not only different cancer cell biological habits, including oncogenesis, proliferation, and distant transfer, but also the development and maintenance of tumor stem cells (CSCs). Phosphorylation levels of STAT3 are associated with poor prognosis in NSCLC, and aberrant STAT3 activity has been observed in malignant cells of other tumors. Therefore, there is a broad prospect of the STAT3 signaling pathway in the treatment of NSCLC. NPs are up-and-coming delivery systems for small-molecule drugs and nucleic acid. Consequently, the nanodrugs targeted signaling pathways are more conducive to uptake and utilization.
The TME contains a vast array of TAMs, which are classified into M1 (anti-tumor) and M2 (tumor-promoting) phenotypes (Mills, 2012; Sica and Mantovani, 2012; Komohara et al., 2016). The activation of STAT3 enhances M2-type polarization, and contributes to the formation of tumor-related phenotypes (Kortylewski et al., 2009). Chen et al. developed dual-targeting delivery system by using siRNA to target both tumor cells and M2 macrophages to silence STAT3 (Chen et al., 2020). The dual targeting system used for siRNA packaging was constructed through electronic self-assembly, consisted of carboxymethyl chitosan, and coupled with folic acid. Compared with non-targeted NPs, the dual-targeted delivery system led to a significant decrease in expression of STAT3, with a successful transition of macrophages from the M2 phenotype to the M1 phenotype, while increased apoptosis and inhibited proliferation of LC cells. In addition, Shobaki’s group used a lipid NPs (LNPs) to targeted deliver siRNA to TAMs, which can silence STAT3 and hypoxia-inducible factor 1α (HIF-1α) (Shobaki et al., 2020). It led to an increase of M1 macrophages, thus obtaining well anti-tumor therapeutic effects. On the whole, the novel nanodrugs targeting macrophages and STAT3 behave with good clinical and pharmaceutical application prospects.
RNAi is a powerful tool to downregulate the level of STAT3, which can make drug-resistant cancer cells re-sensitive (Alshamsan et al., 2011; Kotmakçı et al., 2017). To deliver RNAi-mediated plasmid DNA, Kotmakç et al. developed and evaluated a kind of cationic solid lipid NPs (SLNs). It showed that the expression level of STAT3 mRNA decreased by approximately five-fold while cisplatin-resistant cancer cells were re-sensitive after SLNs treatment (Kotmakçı et al., 2017). Chen et al. used the PEG-PLA NPs to deliver small molecule drugs (Chen et al., 2018). The results showed a synergistic anti-tumor effect on resistant NSCLC in vitro. The nanodrug significantly downregulated the level of biomolecules in signaling pathways, such as p-JAK2, which can cause drug resistance. In summary, the NPs-mediated drug co-delivery method can overcome drug resistance by regulating specific signaling pathways.
7 Nanodrug induced p53 and Bax/Bcl-2 pathway Dysfunction in NSCLC
As a well-known tumor suppressor, p53 can inhibit cell proliferation and promote apoptosis (Cui and Guo, 2016). Dysfunction of the p53 pathway is particularly common, accounting for approximately 68% of NSCLC patients (Kong et al., 2019). A variety of downstream signal molecules are regulated by p53 in tumor, such as B-cell lymphoma 2 protein (Bcl-2) and Bcl-2 related X protein (Bclx). P53 can downregulate the anti-apoptosis factor Bcl-2 and upregulate the pro-apoptosis factor Bclx (Rasheduzzaman et al., 2018).
The main problems with the application of Bcl-2 antagonists in cancer treatment are their poor water solubility and toxicity to normal cells (Schimmer et al., 2008; Paik et al., 2010). NPs can be used to improve the solubility of drugs to enhance anti-tumor efficiency (Li et al., 2018). Chen et al. prepared RBCm-OM/PLGA NPs by combining PLGA with red blood cell membrane (RBCm) to wrap obatok mesylate (OM) (Chen S et al., 2020). The results showed that their NDDS could effectively stop the growth of malignant tumor cells in vitro and in vivo by inducing apoptosis related to the high accumulation of nanodrugs. Similarly, tetrandrine (Tet) was used to make NPs (Tet NPs), which can enhance cell apoptosis by down-regulating the expression of Bcl-2. What’s more, Tet NPs can inhibit cell migration and invasion more effectively than free Tet by down-regulating matrix metalloproteinases 2 (MMP2) and matrix metalloproteinases 9 (MMP9), and upregulating tissue inhibitor of metalloproteinase-3 (TIMP-3) (Xu et al., 2014). The above results indicate that uniting anti-Bcl-2 drugs and NPs might have good prospects in the field of NSCLC treatment by improving the anti-cancer efficiency.
8 Nanodrug remodeled tumor immune microenvironment in NSCLC
During the process of cell differentiation and proliferation, the immunogenicity of tumor cells is reduced, which leads to immune escape (Guo et al., 2022). ICIs can influence the activation and depletion of T cells by interacting with PD-1 or PD-L1, and ultimately inhibit the occurrence of tumor immune escape. (Gordon et al., 2017). This kind of drugs have the potential to improve the survival rate of cancer patients, which are regarded as a desirable choice for the tumor treatment.
To improve the response rate of ICIs therapies, Zhao et al. successfully constructed a nanodrug consisting of fluorinated dendrimer and HSP70 promoter-driven CRISPR/Cas9 (Zhao L. et al., 2022). In this system, the activated HSP70 promoter facilitated the expression of Cas9 protein, leading to permanent genomic destruction of PD-L1. Consequently, it effectively hindered the immune escape of tumor cells. In another study, Zhou et al. found that integrin β3 (β3-INT) is highly expressed in NSCLC and further observed that the inhibitor RGDyK facilitates the process of PD-L1 ubiquitination (Zhou et al., 2022). Based on this, their group prepared RGDyK-modified mesoporous silica NPs loaded with zinc protoporphyrin (ZnPP@MSN-RGDyK). This nanodrug showed high photodynamic treatment efficiency and good immunotherapeutic effect by precisely targeting β3-INT to weaken the function of PD-L1 in preclinical tumor models.
NSCLC patients often develop drug resistance in ICIs monotherapy (Dempke et al., 2018). Kim’s team constructed an investigational tumor-targeted nanodrug named SGT-53 (Kim et al., 2022). They found that SGT-53 can restore an effective immune response to tumor cells by modulating immunosuppressive cells, including T cells and macrophages, and downregulate the expression of immunosuppressive molecule Galectin-1. Furthermore, the study revealed that the intensity of macrophage infiltration was highly correlated with the emergence of ICIs resistance (Cui et al., 2020; Kim et al., 2020; Zhu et al., 2020). Besides, Xu et al. bound nano-diamond doxorubicin conjugate (Nano-DOX) to a PD-L1 blocking agent named BMS-1, which can effectively reactivate the M1-type macrophages to kill tumor cells and inhibit tumor growth (Xu et al., 2021).
In summary, the combination of NPs and ICIs can not only help in the precise targeting of drugs, but also restore the immune surveillance function.
9 Challenges and prospects of nanodrug in targeted NSCLC therapy
Targeted therapies and immunotherapies have been widely used in clinical practice. These drugs improve the prognosis of patients with a variety of advanced cancers. However, most of the targeted drugs are unable to achieve the expected therapeutic effects due to their low solubility, low bioavailability and severe adverse reactions (de Scordilli et al., 2022). Furthermore, systemic administration of immunotherapy can also cause immune damage to respiratory and cardiovascular systems or other systems, and can even be life-threatening (Lahiri et al., 2023). Nanotechnology is a rapidly evolving field that offers new strategies related to drug delivery. Here, the modifiability and micro-size properties enable nanomatrials to play a synergistic therapeutic role with drugs. From this, NDDS can regulate the drug concentration of target cells by controlling the release rate, which eventually overcome the shortcomings of anti-cancer drugs, including the drug resistance, systemic toxicity and rapid metabolism (Abdelaziz et al., 2018; Wang X. et al., 2021). The intersection of disciplines brings a broader prospect for the development of nanomedicine.
Despite the encouraging successes, there are still many problems that remain to be solved, which seem impossible to accomplish in a short period of time. Currently, many studies are dedicated to develop safe and effective nanodrugs in NSCLC, but very few drugs can pass clinical trials (Liao et al., 2020). There are still many unknown metabolic pathways of nanodrugs in the human body, which may bring unpredictable drug side effects (Abdelaziz et al., 2018). They are easily absorbed by healthy cells through active transport because the nanodrugs have small size, which may cause damage on normal histiocytes (Ferrari, 2005; Wang X. et al., 2021). There is still a long way to go for nanodrugs to enter clinical treatment, which requires close cooperation between disciplines. For example, multi-radionuclide imaging can personalize treatment by stratifying patients, and the use of artificial intelligence algorithms can help select specific nano parameters in these highly complex cases to improve the biological function (Arrieta et al., 2012; Mahmoud et al., 2020). Nanodrug plays an active role in the regulation of various signaling pathways in tumors. Many signaling pathways in TME have been shown to play important roles in tumor cell growth, and it is necessary to develop specific drug and delivery devices for these signaling pathways (Wang F. et al., 2021; Lei et al., 2022).
In summary, both nanotechnology and signaling pathway-regulated drugs are ushering in a new era of disease treatment, and their synergistic effects have broad clinical application prospects. However, there are still many problems need to be solved. Accordingly, it is necessary to combine the advantages of complementary multidisciplinary to solve these problems, and this will bring benefits to tumor patients as soon as possible.
10 Conclusion and future prospects
In recent years, the global incidence of NSCLC is the highest among all cancer incidence rates. Currently, precision molecular therapy for NSCLC has been widely used, and many drugs have entered the clinic, but more signal-regulated pathways of tumor development are still unclear. It is the goal of medical development to find more effective and safer therapeutic targets. NDDS brings more convenience to drug therapy, but the defects of the nanodrug itself still need to be overcome. The most important problems come from the stability, effectiveness, and safety of the nanomaterials themselves, in addition to the type of carrier material, preparation technology, and cost issues (Ji et al., 2022). At present, the pharmacokinetic behaviors of more nanodrugs are unclear, which is also an important reason for the low success rate of clinical transformation (Cao and Chen, 2022). Multidisciplinary intersection brings the advantage of solving these problems, and therefore the exchange of new technologies among various disciplines needs to be enhanced (Boehnke et al., 2022; Xu C. et al., 2023). Besides, studies on the regulation of signaling pathways by nanodrugs are mostly conducted in vitro or in animal models. Due to the heterogeneity of cancers in animal models and clinical patients, the therapeutic effects of nanodrugs have large differences between the preclinical therapy and clinical trials, which reduces their clinical application (Xu W. et al., 2023). Therefore, it will be beneficial to improve clinical feasibility of nanodrugs by developing humanized animal models (May, 2018). Meanwhile, we need clinical trials to validate and evaluate their efficacy and safety.
The combination of drugs and nanomaterials will provide hope for patient survival (Van der Meel et al., 2019; Jia et al., 2023). Multi-drug combination is one of the important means to improve anti-cancer efficacy, and it is also a general trend in the field of drug research and development (Detappe et al., 2023). Exploring more therapeutic targets for molecular signaling is also an important prerequisite for promoting multi-drug combination therapy (You et al., 2023). Nanocarriers provide a safe, fast and effective platform for multi-drug combination therapy (Yang et al., 2023). Hence, NDDS combined with multi-target drugs synergistic therapy may be the focus of future research.
Designing efficient and safe nanodrugs and exhaustively investigating their pharmacokinetics in vivo are key to their application and development. The development of humanised animal models will also greatly improve the success rate of clinical translation of novel nanodrugs. Exploring more molecular signalling targets and multi-target combination therapy based on nanocarriers are important research directions. With the development of nanotechnology, NPs will also be updated, and their obvious advantages as drug carriers will be played more in practical applications. Eventually, they will become powerful tools for patients to overcome NSCLC. For the next phase of research on nanodrug, researchers should spend more time and effort to provide clear evidence for existing mechanisms rather than creating many new and complex nanocarriers for similar concepts as a way to advance the clinical translation of nanomedicines.
In conclusion, the research of nanodrugs in regulating signaling pathways is still in its infancy, and many practical problems still need to be solved.
Author contributions
YL and ZD designed this study. WL, ML, XHe, and QH drafted the manuscript. WL performed drawing and organization of figures. ML, CS, XH, FX, and YL revised the manuscript. All authors contributed to the article and approved the submitted version.
Funding
This work was funded by the Zigong Academy of Medical Sciences 2022, Nos ZGYKY22KF008, ZGYKY22KF009, and ZGYKY22KF005; the National Natural Science Foundation of China, No. 82003262; the Key R&D Program of Sichuan Provincial Department of Science and Technology (Major Science and Technology Project), No. 2022YFS0095; China Postdoctoral Science Foundation, Nos 2019TQ0221 and 2019M663517; Post-Doctor Research Project, West China Hospital, Sichuan University, Nos 2019HXBH059 and 2021HXBH009; Sichuan University Postdoctoral Interdisciplinary Innovation Fund.
Acknowledgments
The authors would like to gratefully acknowledge Dr. Yi Li (West China Hospital), Dr. Quan Yuan and Dr. Youli Wen (Zigong First People’s Hospital) for their instructive suggestions to refine the manuscript.
Conflict of interest
The authors declare that the research was conducted in the absence of any commercial or financial relationships that could be construed as a potential conflict of interest.
Publisher’s note
All claims expressed in this article are solely those of the authors and do not necessarily represent those of their affiliated organizations, or those of the publisher, the editors and the reviewers. Any product that may be evaluated in this article, or claim that may be made by its manufacturer, is not guaranteed or endorsed by the publisher.
References
Abdelaziz, H. M., Gaber, M., Abd-Elwakil, M. M., Mabrouk, M. T., Elgohary, M. M., Kamel, N. M., et al. (2018). Inhalable particulate drug delivery systems for lung cancer therapy: nanoparticles, microparticles, nanocomposites and nanoaggregates. J. Control Release 269, 374–392. doi:10.1016/j.jconrel.2017.11.036
Ahlawat, P., Phutela, K., Bal, A., Singh, N., and Sharma, S. (2022). Therapeutic potential of human serum albumin nanoparticles encapsulated actinonin in murine model of lung adenocarcinoma. Drug Deliv. 29 (1), 2403–2413. doi:10.1080/10717544.2022.2067600
Aicher, A., Heeschen, C., Mildner-Rihm, C., Urbich, C., Ihling, C., Technau-Ihling, K., et al. (2003). Essential role of endothelial nitric oxide synthase for mobilization of stem and progenitor cells. Nat. Med. 9 (11), 1370–1376. doi:10.1038/nm948
Alshamsan, A., Hamdy, S., Haddadi, A., Samuel, J., El-Kadi, A. O., Uludağ, H., et al. (2011). STAT3 knockdown in B16 melanoma by siRNA lipopolyplexes induces bystander immune response in vitro and in vivo. Transl. Oncol. 4 (3), 178–188. doi:10.1593/tlo.11100
Argilés, J. M., López-Soriano, F. J., and Stemmler Bbusquets, S. (2023). Cancer-associated cachexia - understanding the tumour macroenvironment and microenvironment to improve management. Nat. Rev. Clin. Oncol. 20 (4), 250–264. doi:10.1038/s41571-023-00734-5
Arrieta, Ó., Medina, L. A., Estrada-Lobato, E., Hernández-Pedro, N., Villanueva-Rodríguez, G., Martínez-Barrera, L., et al. (2012). First-line chemotherapy with liposomal doxorubicin plus cisplatin for patients with advanced malignant pleural mesothelioma: phase II trial. Br. J. Cancer 106 (6), 1027–1032. doi:10.1038/bjc.2012.44
Asati, V., Mahapatra, D. K., and Bharti, S. K. (2016). PI3K/Akt/mTOR and Ras/Raf/MEK/ERK signaling pathways inhibitors as anticancer agents: structural and pharmacological perspectives. Eur. J. Med. Chem. 109, 314–341. doi:10.1016/j.ejmech.2016.01.012
Bílek, O., Holánek, M., Berkovcová, J., Horký, O., Kazda, T., Čoupková, H., et al. (2019). Uncommon EGFR mutations in non-small cell lung cancer and their impact on the treatment. Klin. Onkol. 32 (3), 6–12. doi:10.14735/amko20193S6
Bishop, C. J., Kozielski, K. L., and Green, J. J. (2015). Exploring the role of polymer structure on intracellular nucleic acid delivery via polymeric nanoparticles. J. Control Release 219, 488–499. doi:10.1016/j.jconrel.2015.09.046
Boehnke, N., Straehla, J. P., Safford, H. C., Kocak, M., Rees, M. G., Ronan, M., et al. (2022). Massively parallel pooled screening reveals genomic determinants of nanoparticle delivery. Science 377 (6604), eabm5551. doi:10.1126/science.abm5551
Boolell, V., Alamgeer, M., Watkins, D. N., and Ganju, V. (2015). The evolution of therapies in non-small cell lung cancer. Cancers (Basel) 7 (3), 1815–1846. doi:10.3390/cancers7030864
Cabanos, H. F., and Hata, A. N. (2021). Emerging insights into targeted therapy-tolerant persister cells in cancer. Cancers (Basel) 13 (11), 2666. doi:10.3390/cancers13112666
Cantley, L. C. (2002). The phosphoinositide 3-kinase pathway. Science 296 (5573), 1655–1657. doi:10.1126/science.296.5573.1655
Cao, M., and Chen, C. (2022). Bioavailability of nanomaterials: bridging the gap between nanostructures and their bioactivity. Natl. Sci. Rev. 9 (10), nwac119. doi:10.1093/nsr/nwac119
Chen, D., Zhang, F., Wang, J., He, H., Duan, S., Zhu, R., et al. (2018). Biodegradable nanoparticles mediated Co-delivery of erlotinib (ELTN) and fedratinib (FDTN) toward the treatment of ELTN-resistant non-small cell lung cancer (NSCLC) via suppression of the JAK2/STAT3 signaling pathway. Front. Pharmacol. 9, 1214. doi:10.3389/fphar.2018.01214
Chen, J., Dou, Y., Tang, Y., and Zhang, X. (2020). Folate receptor-targeted RNAi nanoparticles for silencing STAT3 in tumor-associated macrophages and tumor cells. Nanomedicine 25, 102173. doi:10.1016/j.nano.2020.102173
Chen, N., Wei, M., Sun, Y., Li, F., Pei, H., Li, X., et al. (2014). Self-assembly of poly-adenine-tailed CpG oligonucleotide-gold nanoparticle nanoconjugates with immunostimulatory activity. Small 10 (2), 368–375. doi:10.1002/smll.201300903
Chen, P. M., Cheng, Y. W., Wu, T. C., Chen, C. Y., and Lee, H. (2015). MnSOD overexpression confers cisplatin resistance in lung adenocarcinoma via the NF-κB/Snail/Bcl-2 pathway. Free Radic. Biol. Med. 79, 127–137. doi:10.1016/j.freeradbiomed.2014.12.001
Chen, Q., Lai, Q., Jiang, Y., Yao, J., Chen, Q., Zhang, L., et al. (2022). Anlotinib exerts potent antileukemic activities in Ph chromosome negative and positive B-cell acute lymphoblastic leukemia via perturbation of PI3K/AKT/mTOR pathway. Transl. Oncol. 25, 101516. doi:10.1016/j.tranon.2022.101516
Chen S, S., Ren, Y., and Duan, P. (2020). Biomimetic nanoparticle loading obatoclax mesylate for the treatment of non-small-cell lung cancer (NSCLC) through suppressing Bcl-2 signaling. Biomed. Pharmacother. 129, 110371. doi:10.1016/j.biopha.2020.110371
Chmielecki, J., Mok, T., Wu, Y. L., Han, J. Y., Ahn, M. J., Ramalingam, S. S., et al. (2023). Analysis of acquired resistance mechanisms to osimertinib in patients with EGFR-mutated advanced non-small cell lung cancer from the AURA3 trial. Nat. Commun. 14 (1), 1071. doi:10.1038/s41467-023-35962-x
Ciuffreda, L., Incani, U. C., Steelman, L. S., Abrams, S. L., Falcone, I., Curatolo, A. D., et al. (2014). Signaling intermediates (MAPK and PI3K) as therapeutic targets in NSCLC. Curr. Pharm. Des. 20 (24), 3944–3957. doi:10.2174/13816128113196660763
Cui, X., Ma, C., Vasudevaraja, V., Serrano, J., Tong, J., Peng, Y., et al. (2020). Dissecting the immunosuppressive tumor microenvironments in Glioblastoma-on-a-Chip for optimized PD-1 immunotherapy. Elife 9, 52253. doi:10.7554/eLife.52253
Cui, Y., and Guo, G. (2016). Immunomodulatory function of the tumor suppressor p53 in host immune response and the tumor microenvironment. Int. J. Mol. Sci. 17 (11), 1942. doi:10.3390/ijms17111942
de Scordilli, M., Michelotti, A., Bertoli, E., De Carlo, E., and Del Conte Abearz, A. (2022). Targeted therapy and immunotherapy in early-stage non-small cell lung cancer: current evidence and ongoing trials. Int. J. Mol. Sci. 23 (13), 7222. doi:10.3390/ijms23137222
Dempke, W. C. M., Fenchel, K., and Dale, S. P. (2018). Programmed cell death ligand-1 (PD-L1) as a biomarker for non-small cell lung cancer (NSCLC) treatment-are we barking up the wrong tree? Transl. Lung Cancer Res. 7 (3), S275–s279. doi:10.21037/tlcr.2018.04.18
Detappe, A., Nguyen, H. V., Jiang, Y., Agius, M. P., Wang, W., Mathieu, C., et al. (2023). Molecular bottlebrush prodrugs as mono- and triplex combination therapies for multiple myeloma. Nat. Nanotechnol. 18 (2), 184–192. doi:10.1038/s41565-022-01310-1
Duan, Y., Shen, C., Zhang, Y., and Luo, Y. (2022). Advanced diagnostic and therapeutic strategies in nanotechnology for lung cancer. Front. Oncol. 12, 1031000. doi:10.3389/fonc.2022.1031000
Eisinger, S., Sarhan, D., Boura, V. F., Ibarlucea-Benitez, I., Tyystjarvi, S., Oliynyk, G., et al. (2020). Targeting a scavenger receptor on tumor-associated macrophages activates tumor cell killing by natural killer cells. Proc. Natl. Acad. Sci. U. S. A. 117 (50), 32005–32016. doi:10.1073/pnas.2015343117
Engelman, J. A., Luo, J., and Cantley, L. C. (2006). The evolution of phosphatidylinositol 3-kinases as regulators of growth and metabolism. Nat. Rev. Genet. 7 (8), 606–619. doi:10.1038/nrg1879
Ezhilarasan, D., Lakshmi, T., and Mallineni, S. K. (2022). Nano-based targeted drug delivery for lung cancer: therapeutic avenues and challenges. Nanomedicine (Lond) 17 (24), 1855–1869. doi:10.2217/nnm-2021-0364
Fan, H. Y., Yu, X. H., Wang, K., Yin, Y. J., Tang, Y. J., Tang, Y. L., et al. (2019). Graphene quantum dots (GQDs)-based nanomaterials for improving photodynamic therapy in cancer treatment. Eur. J. Med. Chem. 182, 111620. doi:10.1016/j.ejmech.2019.111620
Fang, R. H., Gao, W., and Zhang, L. (2023). Targeting drugs to tumours using cell membrane-coated nanoparticles. Nat. Rev. Clin. Oncol. 20 (1), 33–48. doi:10.1038/s41571-022-00699-x
Fang, X., Hong, Y., Dai, L., Qian, Y., Zhu, C., Wu, B., et al. (2017). CRH promotes human colon cancer cell proliferation via IL-6/JAK2/STAT3 signaling pathway and VEGF-induced tumor angiogenesis. Mol. Carcinog. 56 (11), 2434–2445. doi:10.1002/mc.22691
Ferlay, J., Colombet, M., Soerjomataram, I., Dyba, T., Randi, G., Bettio, M., et al. (2018). Cancer incidence and mortality patterns in Europe: estimates for 40 countries and 25 major cancers in 2018. Eur. J. Cancer 103, 356–387. doi:10.1016/j.ejca.2018.07.005
Ferlay, J., Colombet, M., Soerjomataram, I., Mathers, C., Parkin, D. M., Piñeros, M., et al. (2019). Estimating the global cancer incidence and mortality in 2018: GLOBOCAN sources and methods. Int. J. Cancer 144 (8), 1941–1953. doi:10.1002/ijc.31937
Ferrari, M. (2005). Cancer nanotechnology: opportunities and challenges. Nat. Rev. Cancer 5 (3), 161–171. doi:10.1038/nrc1566
Garofalo, M., Romano, G., Di Leva, G., Nuovo, G., Jeon, Y. J., Ngankeu, A., et al. (2011). Retracted article: EGFR and MET receptor tyrosine kinase–altered microRNA expression induces tumorigenesis and gefitinib resistance in lung cancers. Nat. Med. 18 (1), 74–82. doi:10.1038/nm.2577
Gordon, S. R., Maute, R. L., Dulken, B. W., Hutter, G., George, B. M., McCracken, M. N., et al. (2017). PD-1 expression by tumour-associated macrophages inhibits phagocytosis and tumour immunity. Nature 545 (7655), 495–499. doi:10.1038/nature22396
Guimarães-Bastos, D., Frony, A. C., Barja-Fidalgo, C., and Moraes, J. A. (2022). Melanoma-derived extracellular vesicles skew neutrophils into a pro-tumor phenotype. J. Leukoc. Biol. 111 (3), 585–596. doi:10.1002/jlb.3a0120-050rr
Guo, Q., and Jiang, C. (2020). Delivery strategies for macromolecular drugs in cancer therapy. Acta Pharm. Sin. B 10 (6), 979–986. doi:10.1016/j.apsb.2020.01.009
Guo, Y., Xie, Y., and Luo, Y. (2022). The role of long non-coding RNAs in the tumor immune microenvironment. Front. Immunol. 13, 851004. doi:10.3389/fimmu.2022.851004
Haider, M., Abdin, S. M., Kamal, L., and Orive, G. (2020). Nanostructured lipid carriers for delivery of chemotherapeutics: a review. Pharmaceutics 12 (3), 288. doi:10.3390/pharmaceutics12030288
Haider, M., Zaki, K. Z., El Hamshary, M. R., Hussain, Z., and Orive Gibrahim, H. O. (2022). Polymeric nanocarriers: a promising tool for early diagnosis and efficient treatment of colorectal cancer. J. Adv. Res. 39, 237–255. doi:10.1016/j.jare.2021.11.008
Han, C. Y., Yue, L. L., Tai, L. Y., Zhou, L., Li, X. Y., Xing, G. H., et al. (2013). A novel small peptide as an epidermal growth factor receptor targeting ligand for nanodelivery in vitro. Int. J. Nanomedicine 8, 1541–1549. doi:10.2147/ijn.S43627
Han, W., Shi, L., Ren, L., Zhou, L., Li, T., Qiao, Y., et al. (2018). A nanomedicine approach enables co-delivery of cyclosporin A and gefitinib to potentiate the therapeutic efficacy in drug-resistant lung cancer. Signal Transduct. Target Ther. 3, 16. doi:10.1038/s41392-018-0019-4
Harada, G., Yang, S. R., Cocco, E., and Drilon, A. (2023). Rare molecular subtypes of lung cancer. Nat. Rev. Clin. Oncol. 20 (4), 229–249. doi:10.1038/s41571-023-00733-6
Hirsch, F. R., Scagliotti, G. V., Mulshine, J. L., Kwon, R., Curran, W. J., Wu, Y. L., et al. (2017). Lung cancer: current therapies and new targeted treatments. Lancet 389 (10066), 299–311. doi:10.1016/s0140-6736(16)30958-8
Hou, Y. J., Yang, X. X., Liu, R. Q., Zhao, D., Guo, C. X., Zhu, A. C., et al. (2020). <p>Pathological mechanism of photodynamic therapy and photothermal therapy based on nanoparticles</p>. Int. J. Nanomedicine 15, 6827–6838. doi:10.2147/ijn.S269321
Huang, J., Zhuang, C., Chen, J., Chen, X., Li, X., Zhang, T., et al. (2022). Targeted drug/gene/photodynamic therapy via a stimuli-responsive dendritic-polymer-based nanococktail for treatment of EGFR-TKI-resistant non-small-cell lung cancer. Adv. Mater 34 (27), e2201516. doi:10.1002/adma.202201516
Hwang, I., Kim, J. W., Ylaya, K., Chung, E. J., Kitano, H., Perry, C., et al. (2020). Tumor-associated macrophage, angiogenesis and lymphangiogenesis markers predict prognosis of non-small cell lung cancer patients. J. Transl. Med. 18 (1), 443. doi:10.1186/s12967-020-02618-z
Ikeda-Imafuku, M., Wang, L. L., Rodrigues, D., Shaha, S., Zhao, Z., and Mitragotri, S. (2022). Strategies to improve the EPR effect: a mechanistic perspective and clinical translation. J. Control Release 345, 512–536. doi:10.1016/j.jconrel.2022.03.043
Ji, D., Zhang, Y., Sun, J., Zhang, B., Ma, W., Cheng, B., et al. (2023). An engineered influenza virus to deliver antigens for lung cancer vaccination. Nat. Biotechnol. 2023, 01796. doi:10.1038/s41587-023-01796-7
Ji, X., Li, Q., Song, H., and Fan, C. (2022). Protein-mimicking nanoparticles in biosystems. Adv. Mater 34 (37), e2201562. doi:10.1002/adma.202201562
Jia, Y., Jiang, Y., He, Y., Zhang, W., Zou, J., Magar, K. T., et al. (2023). Approved nanomedicine against diseases. Pharmaceutics 15 (3), 774. doi:10.3390/pharmaceutics15030774
Jiang, X., Wang, J., Deng, X., Xiong, F., Ge, J., Xiang, B., et al. (2019). Role of the tumor microenvironment in PD-L1/PD-1-mediated tumor immune escape. Mol. Cancer 18 (1), 10. doi:10.1186/s12943-018-0928-4
Jing, Y., Zhang, Y., Wang, J., Li, K., Chen, X., Heng, J., et al. (2021). Association between sex and immune-related adverse events during immune checkpoint inhibitor therapy. J. Natl. Cancer Inst. 113 (10), 1396–1404. doi:10.1093/jnci/djab035
Johnson, D. E., O'Keefe, R. A., and Grandis, J. R. (2018). Targeting the IL-6/JAK/STAT3 signalling axis in cancer. Nat. Rev. Clin. Oncol. 15 (4), 234–248. doi:10.1038/nrclinonc.2018.8
Johnson, M., Garassino, M. C., Mok, T., and Mitsudomi, T. (2022). Treatment strategies and outcomes for patients with EGFR-mutant non-small cell lung cancer resistant to EGFR tyrosine kinase inhibitors: focus on novel therapies. Lung Cancer 170, 41–51. doi:10.1016/j.lungcan.2022.05.011
Kalyane, D., Raval, N., Maheshwari, R., Tambe, V., Kalia, K., and Tekade, R. K. (2019). Employment of enhanced permeability and retention effect (EPR): nanoparticle-based precision tools for targeting of therapeutic and diagnostic agent in cancer. Mater Sci. Eng. C Mater Biol. Appl. 98, 1252–1276. doi:10.1016/j.msec.2019.01.066
Kandasamy, G., Danilovtseva, E. N., Annenkov, V. V., and Krishnan, U. M. (2020). Poly(1-vinylimidazole) polyplexes as novel therapeutic gene carriers for lung cancer therapy. Beilstein J. Nanotechnol. 11, 354–369. doi:10.3762/bjnano.11.26
Kim, D. H., Kim, H., Choi, Y. J., Kim, S. Y., Lee, J. E., Sung, K. J., et al. (2019). Exosomal PD-L1 promotes tumor growth through immune escape in non-small cell lung cancer. Exp. Mol. Med. 51 (8), 1–13. doi:10.1038/s12276-019-0295-2
Kim, M. G., Jo, S. D., Yhee, J. Y., Lee, B. S., Lee, S. J., Park, S. G., et al. (2017). Synergistic anti-tumor effects of bevacizumab and tumor targeted polymerized VEGF siRNA nanoparticles. Biochem. Biophys. Res. Commun. 489 (1), 35–41. doi:10.1016/j.bbrc.2017.05.103
Kim, S. S., Harford, J. B., Moghe, M., Doherty, C., and Chang, E. H. (2022). A novel P53 nanomedicine reduces immunosuppression and augments anti-PD-1 therapy for non-small cell lung cancer in syngeneic mouse models. Cells 11 (21), cells11213434. doi:10.3390/cells11213434
Kim, Y. J., Won, C. H., Lee, M. W., Choi, J. H., Chang, S. E., and Lee, W. J. (2020). Correlation between tumor-associated macrophage and immune checkpoint molecule expression and its prognostic significance in cutaneous melanoma. J. Clin. Med. 9 (8), 2500. doi:10.3390/jcm9082500
Komi, D. E. A., Khomtchouk, K., and Santa Maria, P. L. (2020). A review of the contribution of mast cells in wound healing: involved molecular and cellular mechanisms. Clin. Rev. Allergy Immunol. 58 (3), 298–312. doi:10.1007/s12016-019-08729-w
Komohara, Y., Fujiwara, Y., Ohnishi, K., and Takeya, M. (2016). Tumor-associated macrophages: potential therapeutic targets for anti-cancer therapy. Adv. Drug Deliv. Rev. 99, 180–185. doi:10.1016/j.addr.2015.11.009
Kong, N., Tao, W., Ling, X., Wang, J., Xiao, Y., Shi, S., et al. (2019). Synthetic mRNA nanoparticle-mediated restoration of p53 tumor suppressor sensitizes p53-deficient cancers to mTOR inhibition. Sci. Transl. Med. 11 (523), eaaw1565. doi:10.1126/scitranslmed.aaw1565
Kortylewski, M., Swiderski, P., Herrmann, A., Wang, L., Kowolik, C., Kujawski, M., et al. (2009). In vivo delivery of siRNA to immune cells by conjugation to a TLR9 agonist enhances antitumor immune responses. Nat. Biotechnol. 27 (10), 925–932. doi:10.1038/nbt.1564
Kotmakçı, M., Çetintaş, V. B., and Kantarcı, A. G. (2017). Preparation and characterization of lipid nanoparticle/pDNA complexes for STAT3 downregulation and overcoming chemotherapy resistance in lung cancer cells. Int. J. Pharm. 525 (1), 101–111. doi:10.1016/j.ijpharm.2017.04.034
Kuo, W. S., Shao, Y. T., Huang, K. S., Chou, T. M., and Yang, C. H. (2018). Antimicrobial amino-functionalized nitrogen-doped graphene quantum dots for eliminating multidrug-resistant species in dual-modality photodynamic therapy and bioimaging under two-photon excitation. ACS Appl. Mater Interfaces 10 (17), 14438–14446. doi:10.1021/acsami.8b01429
Kwak, G., Jo, S. D., Kim, D., Kim, H., Kim, M. G., Kim, K., et al. (2017). Synergistic antitumor effects of combination treatment with metronomic doxorubicin and VEGF-targeting RNAi nanoparticles. J. Control Release 267, 203–213. doi:10.1016/j.jconrel.2017.08.015
Lahiri, A., Maji, A., Potdar, P. D., Singh, N., Parikh, P., Bisht, B., et al. (2023). Lung cancer immunotherapy: progress, pitfalls, and promises. Mol. Cancer 22 (1), 40. doi:10.1186/s12943-023-01740-y
Lai, S. Y., and Johnson, F. M. (2010). Defining the role of the JAK-STAT pathway in head and neck and thoracic malignancies: implications for future therapeutic approaches. Drug Resist Updat 13 (3), 67–78. doi:10.1016/j.drup.2010.04.001
Lai-Kwon, J., Tiu, C., Pal, A., Khurana, S., and Minchom, A. (2021). Moving beyond epidermal growth factor receptor resistance in metastatic non-small cell lung cancer - a drug development perspective. Crit. Rev. Oncol. Hematol. 159, 103225. doi:10.1016/j.critrevonc.2021.103225
Lee, J. W., Soung, Y. H., Kim, S. Y., Nam, S. W., Park, W. S., Lee, J. Y., et al. (2006). Absence of JAK2 V617F mutation in gastric cancers. Acta Oncol. 45 (2), 222–223. doi:10.1080/02841860500341223
Lei, R., Zhou, M., Zhang, S., Luo, J., Qu, C., Wang, Y., et al. (2022). Potential role of PRKCSH in lung cancer: bioinformatics analysis and a case study of nano ZnO. Nanoscale 14 (12), 4495–4510. doi:10.1039/d1nr08133k
Li, F., Mei, H., Xie, X., Zhang, H., Liu, J., Lv, T., et al. (2017). Aptamer-Conjugated chitosan-anchored liposomal complexes for targeted delivery of erlotinib to EGFR-mutated lung cancer cells. Aaps J. 19 (3), 814–826. doi:10.1208/s12248-017-0057-9
Li, H. S., Wang, S. Z., Xu, H. Y., Yan, X., Zhang, J. Y., Lei, S. Y., et al. (2022). Afatinib and dacomitinib efficacy, safety, progression patterns, and resistance mechanisms in patients with non-small cell lung cancer carrying uncommon EGFR mutations: a comparative cohort study in China (afanda study). Cancers (Basel) 14 (21), cancers14215307. doi:10.3390/cancers14215307
Li, R., He, Y., Zhang, S., Qin, J., and Wang, J. (2018). Cell membrane-based nanoparticles: a new biomimetic platform for tumor diagnosis and treatment. Acta Pharm. Sin. B 8 (1), 14–22. doi:10.1016/j.apsb.2017.11.009
Li, C., Hu, J., Li, W., Song, G., and Shen, J. (2016). Combined bortezomib-based chemotherapy and p53 gene therapy using hollow mesoporous silica nanospheres for p53 mutant non-small cell lung cancer treatment. Biomater. Sci. 5 (1), 77–88. doi:10.1039/c6bm00449k
Li, S., Choi, Y. L., Gong, Z., Liu, X., Lira, M., Kan, Z., et al. (2016). Comprehensive characterization of oncogenic drivers in asian lung adenocarcinoma. J. Thorac. Oncol. 11 (12), 2129–2140. doi:10.1016/j.jtho.2016.08.142
Li, Z., Yang, L., Wang, H., Binzel, D. W., Williams, T. M., and Guo, P. (2021). Non-small-cell lung cancer regression by siRNA delivered through exosomes that display EGFR RNA aptamer. Nucleic Acid. Ther. 31 (5), 364–374. doi:10.1089/nat.2021.0002
Liam, C. K., Ahmad, A. R., Hsia, T. C., Zhou, J., Kim, D. W., Soo, R. A., et al. (2023). Randomized trial of tepotinib plus gefitinib versus chemotherapy in EGFR-mutant NSCLC with EGFR inhibitor resistance due to MET amplification: INSIGHT final analysis. Clin. Cancer Res. 29 (10), 1879–1886. doi:10.1158/1078-0432.CCR-22-3318
Liao, Z., Wong, S. W., Yeo, H. L., and Zhao, Y. (2020). Smart nanocarriers for cancer treatment: clinical impact and safety. NanoImpact 20, 100253. doi:10.1016/j.impact.2020.100253
Lin, T. E., HuangFu, W. C., Chao, M. W., Sung, T. Y., Chang, C. D., Chen, Y. Y., et al. (2018). A novel selective JAK2 inhibitor identified using pharmacological interactions. Front. Pharmacol. 9, 1379. doi:10.3389/fphar.2018.01379
Liu, B., Hu, X., Feng, K., Gao, R., Xue, Z., Zhang, S., et al. (2022). Temporal single-cell tracing reveals clonal revival and expansion of precursor exhausted T cells during anti-PD-1 therapy in lung cancer. Nat. Cancer 3 (1), 108–121. doi:10.1038/s43018-021-00292-8
Liu, J., Shi, X., Zhang, R., Zhang, M., He, J., Chen, J., et al. (2021b). CoFe(2)O(4)-Quantum dots for synergistic photothermal/photodynamic therapy of non-small-cell lung cancer via triggering apoptosis by regulating PI3K/AKT pathway. Nanoscale Res. Lett. 16 (1), 120. doi:10.1186/s11671-021-03580-5
Liu, Q., Sun, Z., and Chen, L. (2020). Memory T cells: strategies for optimizing tumor immunotherapy. Protein Cell 11 (8), 549–564. doi:10.1007/s13238-020-00707-9
Liu, Z., Wang, T., She, Y., Wu, K., Gu, S., Li, L., et al. (2021a). N(6)-methyladenosine-modified circIGF2BP3 inhibits CD8(+) T-cell responses to facilitate tumor immune evasion by promoting the deubiquitination of PD-L1 in non-small cell lung cancer. Mol. Cancer 20 (1), 105. doi:10.1186/s12943-021-01398-4
Mahmoud, M. E., Allam, E. A., El-Sharkawy, R. M., Soliman, M. A., Saad, E. A., and El-Khatib, A. M. (2020). Nano-manganese oxide-functionalized-oleyl amine as a simple and low cost nanosorbent for remediation of Zn(II)/Co(II) and their radioactive nuclides (65)Zn and (60)Co from water. Appl. Radiat. Isot. 159, 108989. doi:10.1016/j.apradiso.2019.108989
May, M. (2018). Cancer research with a human touch. Nature 556 (7700), 259–261. doi:10.1038/d41586-018-04161-w
McCubrey, J. A., Steelman, L. S., Abrams, S. L., Bertrand, F. E., Ludwig, D. E., Basecke, J., et al. (2008). Targeting survival cascades induced by activation of Ras/Raf/MEK/ERK, PI3K/PTEN/Akt/mTOR and Jak/STAT pathways for effective leukemia therapy. Leukemia 22 (4), 708–722. doi:10.1038/leu.2008.27
McHugh, K. J., Jing, L., Behrens, A. M., Jayawardena, S., Tang, W., Gao, M., et al. (2018). Biocompatible semiconductor quantum dots as cancer imaging agents. Adv. Mater 30 (18), e1706356. doi:10.1002/adma.201706356
Mills, C. D. (2012). M1 and M2 macrophages: oracles of health and disease. Crit. Rev. Immunol. 32 (6), 463–488. doi:10.1615/critrevimmunol.v32.i6.10
Molina, J. R., Yang, P., Cassivi, S. D., Schild, S. E., and Adjei, A. A. (2008). Non-small cell lung cancer: epidemiology, risk factors, treatment, and survivorship. Mayo Clin. Proc. 83 (5), 584–594. doi:10.4065/83.5.584
Mortezaee, K. (2021). Myeloid-derived suppressor cells in cancer immunotherapy-clinical perspectives. Life Sci. 277, 119627. doi:10.1016/j.lfs.2021.119627
Muenst, S., Soysal, S. D., Tzankov, A., and Hoeller, S. (2015). The PD-1/PD-L1 pathway: biological background and clinical relevance of an emerging treatment target in immunotherapy. Expert Opin. Ther. Targets 19 (2), 201–211. doi:10.1517/14728222.2014.980235
Naylor, E. C., Desani, J. K., and Chung, P. K. (2016). Targeted therapy and immunotherapy for lung cancer. Surg. Oncol. Clin. N. Am. 25 (3), 601–609. doi:10.1016/j.soc.2016.02.011
Nguyen, P. H. D., Jayasinghe, M. K., Le, A. H., Peng, B., and Le, M. T. N. (2023). Advances in drug delivery systems based on red blood cells and their membrane-derived nanoparticles. ACS Nano 17 (6), 5187–5210. doi:10.1021/acsnano.2c11965
Nie, M., Chen, N., Pang, H., Jiang, T., Jiang, W., Tian, P., et al. (2022). Targeting acetylcholine signaling modulates persistent drug tolerance in EGFR-mutant lung cancer and impedes tumor relapse. J. Clin. Invest. 132 (20), jci160152. doi:10.1172/jci160152
Niu, Z., Jin, R., Zhang, Y., and Li, H. (2022). Signaling pathways and targeted therapies in lung squamous cell carcinoma: mechanisms and clinical trials. Signal Transduct. Target Ther. 7 (1), 353. doi:10.1038/s41392-022-01200-x
NSCLC Meta-analysis Collaborative Group (2014). Preoperative chemotherapy for non-small-cell lung cancer: a systematic review and meta-analysis of individual participant data. Lancet 383 (9928), 1561–1571. doi:10.1016/s0140-6736(13)62159-5
Paik, P. K., Rudin, C. M., Brown, A., Rizvi, N. A., Takebe, N., Travis, W., et al. (2010). A phase I study of obatoclax mesylate, a Bcl-2 antagonist, plus topotecan in solid tumor malignancies. Cancer Chemother. Pharmacol. 66 (6), 1079–1085. doi:10.1007/s00280-010-1265-5
Palazzolo, S., Bayda, S., Hadla, M., Caligiuri, I., Corona, G., Toffoli, G., et al. (2018). The clinical translation of organic nanomaterials for cancer therapy: a focus on polymeric nanoparticles, micelles, liposomes and exosomes. Curr. Med. Chem. 25 (34), 4224–4268. doi:10.2174/0929867324666170830113755
Papadimitrakopoulou, V. (2012). Development of PI3K/AKT/mTOR pathway inhibitors and their application in personalized therapy for non-small-cell lung cancer. J. Thorac. Oncol. 7 (8), 1315–1326. doi:10.1097/JTO.0b013e31825493eb
Passaro, A., Jänne, P. A., Mok, T., and Peters, S. (2021). Overcoming therapy resistance in EGFR-mutant lung cancer. Nat. Cancer 2 (4), 377–391. doi:10.1038/s43018-021-00195-8
Patra, J. K., Das, G., Fraceto, L. F., Campos, E. V. R., Rodriguez-Torres, M. D. P., Acosta-Torres, L. S., et al. (2018). Nano based drug delivery systems: recent developments and future prospects. J. Nanobiotechnology 16 (1), 71. doi:10.1186/s12951-018-0392-8
Punekar, S. R., Velcheti, V., Neel, B. G., and Wong, K. K. (2022). The current state of the art and future trends in RAS-targeted cancer therapies. Nat. Rev. Clin. Oncol. 19 (10), 637–655. doi:10.1038/s41571-022-00671-9
Raju, G. S. R., Dariya, B., Mungamuri, S. K., Chalikonda, G., Kang, S. M., Khan, I. N., et al. (2021). Nanomaterials multifunctional behavior for enlightened cancer therapeutics. Semin. Cancer Biol. 69, 178–189. doi:10.1016/j.semcancer.2019.08.013
Rasheduzzaman, M., Jeong, J. K., and Park, S. Y. (2018). Resveratrol sensitizes lung cancer cell to TRAIL by p53 independent and suppression of Akt/NF-κB signaling. Life Sci. 208, 208–220. doi:10.1016/j.lfs.2018.07.035
Reck, M., Remon, J., and Hellmann, M. D. (2022). First-Line immunotherapy for non-small-cell lung cancer. J. Clin. Oncol. 40 (6), 586–597. doi:10.1200/jco.21.01497
Rosell, R., Karachaliou, N., and Arrieta, O. (2020). Novel molecular targets for the treatment of lung cancer. Curr. Opin. Oncol. 32 (1), 37–43. doi:10.1097/CCO.0000000000000590
Sainsbury, F., and Steinmetz, N. F. (2023). Nanotechnology meets virology. Virology 581, 56–57. doi:10.1016/j.virol.2023.02.015
Schimmer, A. D., O'Brien, S., Kantarjian, H., Brandwein, J., Cheson, B. D., Minden, M. D., et al. (2008). A phase I study of the pan bcl-2 family inhibitor obatoclax mesylate in patients with advanced hematologic malignancies. Clin. Cancer Res. 14 (24), 8295–8301. doi:10.1158/1078-0432.Ccr-08-0999
Seeta Rama Raju, G., Benton, L., Pavitra, E., and Yu, J. S. (2015). Multifunctional nanoparticles: recent progress in cancer therapeutics. Chem. Commun. (Camb) 51 (68), 13248–13259. doi:10.1039/c5cc04643b
Shi, C. S., and Kehrl, J. H. (2004). Pyk2 amplifies epidermal growth factor and c-Src-induced Stat3 activation. J. Biol. Chem. 279 (17), 17224–17231. doi:10.1074/jbc.M311875200
Shobaki, N., Sato, Y., Suzuki, Y., Okabe, N., and Harashima, H. (2020). Manipulating the function of tumor-associated macrophages by siRNA-loaded lipid nanoparticles for cancer immunotherapy. J. Control Release 325, 235–248. doi:10.1016/j.jconrel.2020.07.001
Sica, A., and Mantovani, A. (2012). Macrophage plasticity and polarization: in vivo veritas. J. Clin. Invest. 122 (3), 787–795. doi:10.1172/jci59643
Socinski, M. A., Jotte, R. M., Cappuzzo, F., Orlandi, F., Stroyakovskiy, D., Nogami, N., et al. (2018). Atezolizumab for first-line treatment of metastatic nonsquamous NSCLC. N. Engl. J. Med. 378 (24), 2288–2301. doi:10.1056/NEJMoa1716948
Sonenshein, G. E. (1997). Rel/NF-kappa B transcription factors and the control of apoptosis. Semin. Cancer Biol. 8 (2), 113–119. doi:10.1006/scbi.1997.0062
Song, H., Wu, J., Tang, Y., Dai, Y., Xiang, X., Li, Y., et al. (2023). Diverse rescue potencies of p53 mutations to ATO are predetermined by intrinsic mutational properties. Sci. Transl. Med. 15 (690), eabn9155. doi:10.1126/scitranslmed.abn9155
Song, S., Liu, D., Peng, J., Deng, H., Guo, Y., Xu, L. X., et al. (2009). Novel peptide ligand directs liposomes toward EGF-R high-expressing cancer cells in vitro and in vivo. Faseb J. 23 (5), 1396–1404. doi:10.1096/fj.08-117002
Song, S., Liu, D., Peng, J., Sun, Y., Li, Z., Gu, J. R., et al. (2008). Peptide ligand-mediated liposome distribution and targeting to EGFR expressing tumor in vivo. Int. J. Pharm. 363 (1-2), 155–161. doi:10.1016/j.ijpharm.2008.07.012
Stater, E. P., Sonay, A. Y., Hart, C., and Grimm, J. (2021). The ancillary effects of nanoparticles and their implications for nanomedicine. Nat. Nanotechnol. 16 (11), 1180–1194. doi:10.1038/s41565-021-01017-9
Sun, Y., Zhang, Y., Gao, Y., Wang, P., He, G., Blum, N. T., et al. (2020). Six birds with one stone: versatile nanoporphyrin for single-laser-triggered synergistic phototheranostics and robust immune activation. Adv. Mater 32 (48), e2004481. doi:10.1002/adma.202004481
Sung, H., Ferlay, J., Siegel, R. L., Laversanne, M., Soerjomataram, I., Jemal, A., et al. (2021). Global cancer statistics 2020: GLOBOCAN estimates of incidence and mortality worldwide for 36 cancers in 185 countries. CA Cancer J. Clin. 71 (3), 209–249. doi:10.3322/caac.21660
Suresh, K., Naidoo, J., Lin, C. T., and Danoff, S. (2018). Immune checkpoint immunotherapy for non-small cell lung cancer: benefits and pulmonary toxicities. Chest 154 (6), 1416–1423. doi:10.1016/j.chest.2018.08.1048
Tan, A. C. (2020). Targeting the PI3K/Akt/mTOR pathway in non-small cell lung cancer (NSCLC). Thorac. Cancer 11 (3), 511–518. doi:10.1111/1759-7714.13328
Tanaka, A., and Sakaguchi, S. (2017). Regulatory T cells in cancer immunotherapy. Cell Res. 27 (1), 109–118. doi:10.1038/cr.2016.151
Taniguchi, H., Yamada, T., Wang, R., Tanimura, K., Adachi, Y., Nishiyama, A., et al. (2019). AXL confers intrinsic resistance to osimertinib and advances the emergence of tolerant cells. Nat. Commun. 10 (1), 259. doi:10.1038/s41467-018-08074-0
Thorn, M., Guha, P., Cunetta, M., Espat, N. J., Miller, G., Junghans, R. P., et al. (2016). Tumor-associated GM-CSF overexpression induces immunoinhibitory molecules via STAT3 in myeloid-suppressor cells infiltrating liver metastases. Cancer Gene Ther. 23 (6), 188–198. doi:10.1038/cgt.2016.19
Tian, Y., Gao, Z., Wang, N., Hu, M., Ju, Y., Li, Q., et al. (2022). Engineering poly(ethylene glycol) nanoparticles for accelerated blood clearance inhibition and targeted drug delivery. J. Am. Chem. Soc. 144 (40), 18419–18428. doi:10.1021/jacs.2c06877
Tseng, S. J., Kempson, I. M., Huang, K. Y., Li, H. J., Fa, Y. C., Ho, Y. C., et al. (2018). Targeting tumor microenvironment by bioreduction-activated nanoparticles for light-triggered virotherapy. ACS Nano 12 (10), 9894–9902. doi:10.1021/acsnano.8b02813
Upadhyay, P., Ghosh, A., Basu, A., Pranati, P. A., Gupta, P., Das, S., et al. (2021). Delivery of gefitinib in synergism with thymoquinone via transferrin-conjugated nanoparticle sensitizes gefitinib-resistant non-small cell lung carcinoma to control metastasis and stemness. Biomater. Sci. 9 (24), 8285–8312. doi:10.1039/d1bm01148k
Van der Meel, R., Sulheim, E., Shi, Y., Kiessling, F., and Mulder Wjmlammers, T. (2019). Smart cancer nanomedicine. Nat. Nanotechnol. 14 (11), 1007–1017. doi:10.1038/s41565-019-0567-y
Villaruz, L. C., Burns, T. F., Ramfidis, V. S., and Socinski, M. A. (2013). Personalizing therapy in advanced non-small cell lung cancer. Semin. Respir. Crit. Care Med. 34 (6), 822–836. doi:10.1055/s-0033-1358552
Vincent, M. P., Navidzadeh, J. O., Bobbala, S., and Scott, E. A. (2022). Leveraging self-assembled nanobiomaterials for improved cancer immunotherapy. Cancer Cell 40 (3), 255–276. doi:10.1016/j.ccell.2022.01.006
Wang, F., Chen, X., Cheng, H., Song, L., Liu, J., Caplan, S., et al. (2021). MICAL2PV suppresses the formation of tunneling nanotubes and modulates mitochondrial trafficking. EMBO Rep. 22 (7), e52006. doi:10.15252/embr.202052006
Wang, H. J., Ran, H. F., Yin, Y., Xu, X. G., Jiang, B. X., Yu, S. Q., et al. (2022). Catalpol improves impaired neurovascular unit in ischemic stroke rats via enhancing VEGF-PI3K/AKT and VEGF-MEK1/2/ERK1/2 signaling. Acta Pharmacol. Sin. 43 (7), 1670–1685. doi:10.1038/s41401-021-00803-4
Wang, J., Ni, Q., Wang, Y., Zhang, Y., He, H., Gao, D., et al. (2021). Nanoscale drug delivery systems for controllable drug behaviors by multi-stage barrier penetration. J. Control Release 331, 282–295. doi:10.1016/j.jconrel.2020.08.045
Wang, X., Zhong, X., Li, J., Liu, Z., and Cheng, L. (2021). Inorganic nanomaterials with rapid clearance for biomedical applications. Chem. Soc. Rev. 50 (15), 8669–8742. doi:10.1039/d0cs00461h
Wang, Z., Liu, W., Shi, J., Chen, N., and Fan, C. (2018). Nanoscale delivery systems for cancer immunotherapy. Mater. Horizons 5 (3), 344–362. doi:10.1039/c7mh00991g
Wu, D., Zhang, Z., Li, X., Zhu, T., Wang, J., and Hu, Q. (2023). Supramolecular theranostic nanomedicine for in situ self-boosting cancer photochemotherapy. Biomacromolecules 24 (2), 1022–1031. doi:10.1021/acs.biomac.2c01469
Xu, C., Ji, X., Zhou, Y., Cheng, Y., Guo, D., Li, Q., et al. (2023). Slimming and reinvigorating tumor-associated dendritic cells with hierarchical lipid rewiring nanoparticles. Adv. Mater 35 (30), e2211415. doi:10.1002/adma.202211415
Xu, H., Hou, Z., Zhang, H., Kong, H., Li, X., Wang, H., et al. (2014). An efficient Trojan delivery of tetrandrine by poly(N-vinylpyrrolidone)-block-poly(ε-caprolactone) (PVP-b-PCL) nanoparticles shows enhanced apoptotic induction of lung cancer cells and inhibition of its migration and invasion. Int. J. Nanomedicine 9, 231–242. doi:10.2147/ijn.S55541
Xu, H. Z., Li, T. F., Wang, C., Ma, Y., Liu, Y., Zheng, M. Y., et al. (2021). Synergy of nanodiamond-doxorubicin conjugates and PD-L1 blockade effectively turns tumor-associated macrophages against tumor cells. J. Nanobiotechnology 19 (1), 268. doi:10.1186/s12951-021-01017-w
Xu, W., Yang, S., Lu, L., Xu, Q., Wu, S., Zhou, J., et al. (2023). Influence of lung cancer model characteristics on tumor targeting behavior of nanodrugs. J. Control Release 354, 538–553. doi:10.1016/j.jconrel.2023.01.026
Yan, S., Zhang, B., Feng, J., Wu, H., Duan, N., Zhu, Y., et al. (2021). FGFC1 selectively inhibits erlotinib-resistant non-small cell lung cancer via elevation of ROS mediated by the EGFR/PI3K/Akt/mTOR pathway. Front. Pharmacol. 12, 764699. doi:10.3389/fphar.2021.764699
Yang, C. Y., Yang, J. C., and Yang, P. C. (2020). Precision management of advanced non-small cell lung cancer. Annu. Rev. Med. 71, 117–136. doi:10.1146/annurev-med-051718-013524
Yang, Z., Gao, D., Zhao, J., Yang, G., Guo, M., Wang, Y., et al. (2023). Thermal immuno-nanomedicine in cancer. Nat. Rev. Clin. Oncol. 20 (2), 116–134. doi:10.1038/s41571-022-00717-y
You, M., Xie, Z., Zhang, N., Zhang, Y., Xiao, D., Liu, S., et al. (2023). Signaling pathways in cancer metabolism: mechanisms and therapeutic targets. Signal Transduct. Target Ther. 8 (1), 196. doi:10.1038/s41392-023-01442-3
Zhang, M., Yang, W., Wang, P., Deng, Y., Dong, Y. T., Liu, F. F., et al. (2020). CCL7 recruits cDC1 to promote antitumor immunity and facilitate checkpoint immunotherapy to non-small cell lung cancer. Nat. Commun. 11 (1), 6119. doi:10.1038/s41467-020-19973-6
Zhang, Y., Wang, Z., and Gemeinhart, R. A. (2013). Progress in microRNA delivery. J. Control Release 172 (3), 962–974. doi:10.1016/j.jconrel.2013.09.015
Zhao, L., Li, D., Zhang, Y., Huang, Q., Zhang, Z., Chen, C., et al. (2022). HSP70-Promoter-Driven CRISPR/Cas9 system activated by reactive oxygen species for multifaceted anticancer immune response and potentiated immunotherapy. ACS Nano 16 (9), 13821–13833. doi:10.1021/acsnano.2c01885
Zhao, Y., Guo, S., Deng, J., Shen, J., Du, F., Wu, X., et al. (2022). VEGF/VEGFR-targeted therapy and immunotherapy in non-small cell lung cancer: targeting the tumor microenvironment. Int. J. Biol. Sci. 18 (9), 3845–3858. doi:10.7150/ijbs.70958
Zhong, M., Ma, X., Sun, C., and Chen, L. (2010). MicroRNAs reduce tumor growth and contribute to enhance cytotoxicity induced by gefitinib in non-small cell lung cancer. Chem. Biol. Interact. 184 (3), 431–438. doi:10.1016/j.cbi.2010.01.025
Zhong, Z., Wen, Z., and Darnell, J. E. (1994). Stat3: a STAT family member activated by tyrosine phosphorylation in response to epidermal growth factor and interleukin-6. Science 264 (5155), 95–98. doi:10.1126/science.8140422
Zhou, L., Liang, H., Ge, Y., Ding, W., Chen, Q., Zhang, T., et al. (2022). Precisely targeted nano-controller of PD-L1 level for non-small cell lung cancer spinal metastasis immunotherapy. Adv. Healthc. Mater 11 (20), e2200938. doi:10.1002/adhm.202200938
Zhou, Y., Chen, H., Tang, L., Feng, Y., Tao, Y., Huang, L., et al. (2023). Association of immune-related adverse events and efficacy in advanced non-small-cell lung cancer: a systematic review and meta-analysis. Immunotherapy 15 (3), 209–220. doi:10.2217/imt-2022-0028
Keywords: nanodrug, signaling pathways, non-small cell lung cancer, drug resistance, targeted therapy
Citation: Li W, Li M, Huang Q, He X, Shen C, Hou X, Xue F, Deng Z and Luo Y (2023) Advancement of regulating cellular signaling pathways in NSCLC target therapy via nanodrug. Front. Chem. 11:1251986. doi: 10.3389/fchem.2023.1251986
Received: 03 July 2023; Accepted: 29 August 2023;
Published: 07 September 2023.
Edited by:
Dongdong Zeng, Shanghai University of Medicine and Health Sciences, ChinaReviewed by:
Saumya Nigam, Michigan State University, United StatesBing Chen, Fujian Medical University, China
Copyright © 2023 Li, Li, Huang, He, Shen, Hou, Xue, Deng and Luo. This is an open-access article distributed under the terms of the Creative Commons Attribution License (CC BY). The use, distribution or reproduction in other forums is permitted, provided the original author(s) and the copyright owner(s) are credited and that the original publication in this journal is cited, in accordance with accepted academic practice. No use, distribution or reproduction is permitted which does not comply with these terms.
*Correspondence: Yao Luo, bHVveWFvQHNjdS5lZHUuY24=; Zhiping Deng, ZGVuZ3pwMTAxNkAxNjMuY29t
†These authors have contributed equally to this work and share first authorship