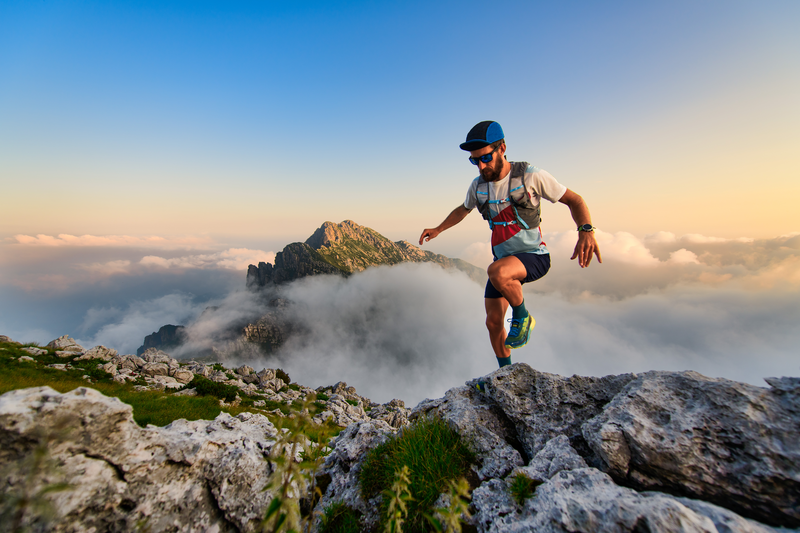
95% of researchers rate our articles as excellent or good
Learn more about the work of our research integrity team to safeguard the quality of each article we publish.
Find out more
ORIGINAL RESEARCH article
Front. Chem. , 13 July 2023
Sec. Green and Sustainable Chemistry
Volume 11 - 2023 | https://doi.org/10.3389/fchem.2023.1234763
This article is part of the Research Topic Chemistry and The Circular Economy View all 5 articles
Due to its excellent properties, poly(ethylene terephthalate) (PET) is one of the most produced and consumed polymers. Among plastics, it represents the main contributor to environmental pollution. Following the circular economy model, the chemical upcycling of PET reduces the amount of waste generated and transforms it into high-value products. The depolymerization of poly(ethylene terephthalate) into oligomers or monomers leads to forming a library of reactive molecules involved in different polymerization processes to obtain compounds with improved properties. Herein, several β-hydroxy amines were synthesized and used for the chemical recycling of water bottle waste by an environmental benefit aminolysis process to get very useful new terephthalamide diol monomers. The recycled diol monomers were subsequently exploited to synthesize poly(urethane acrylates) (PUAs) UV-curable coatings, and their chemical, thermal and mechanical characterizations were performed. The results show the great potential of the developed synthesis protocols to obtain PUAs with final properties that can be modulated to meet the requirements of different applications.
Plastic pollution has become one of the most critical environmental issues, as the rapidly increasing production of plastic products overcomes the ability to deal with them. The world produces around 400 million metric tons of plastic waste every year. Globally, only 10% of plastic waste is successfully recycled, 14% is incinerated, and the remaining 74% is still accumulated in landfills, dumps or the natural environment (Geyer, 2020). That is why a circular approach to the design and synthesis of polymers has become one of the main focuses, from existing materials to new and more tunable chemical feedstocks (Li K. L. et al., 2023). The most evident purposes of polymers are within packaging, construction, or textiles. Furthermore, they are present in many other forms, such as consumer or industrial formulations (e.g., cosmetics, pharmaceuticals, detergents, lubricants, and agrochemicals). In these applications, there is still the need to enhance and optimize performances by changing the polymer chains’ molecular structure. This could include molecular weight control, adding functional groups or making polymers where the monomer is designed for a specific purpose with tunable organic structures. Control over the chemical structure of monomers is significant since it can affect the thermal and physical properties of the subsequent polymer, from thermal shifts and mechanical properties (Begley et al., 2004; Hopewell et al., 2009; Li et al., 2016). The most prominent polymeric material in the last decades is poly (ethylene terephthalate) (PET), which has excellent chemical and physical properties for many applications. PET is one of the most important in world production. Its global consumption is increasing yearly due to its outstanding chemical resistance, excellent thermoformability, optical transparency, and good quality tensile and impact strength. These properties make PET suitable for many applications, such as coatings, food packaging, water bottles, textile fibres, etc. (Achilias et al., 2010; Jamdar et al., 2018). The global demand and consumption of PET will reach high values by 2025, increasing the production of post-consumer plastic waste that ends up in landfills or is incinerated. This waste treatment leads to severe threats from an environmental point of view (Ghosal and Nayak, 2022), being the main contributor to the accumulation of plastic waste in the environment while only 28.4% is recycled into bottles, films and fibres, the remaining landfilled (Taniguchi et al., 2019). Researchers, consultants and agencies are also working on these PET products’ environmental impact using the Life Cycle Assessment tool (Marathe et al., 2019) to assess the environmental impact of several processes and products. With this in mind, it appears very clear how the generation rate of PET waste is too fast, and its reduction is possible only using chemical and mechanical recycling techniques. Thus, the challenge for researchers and technologists is to join the two ideologies to reduce and recycle waste and convert it into high-value-added products (Coates and Getzler. 2020; Junk et al., 2023). For this purpose, chemical recycling, which involves the depolymerization of PET, is of great interest as an alternative feedstock to prepare innovative monomers (Karayannidis and Achillas, 2007). They can be further used as precursors suitable for chemical upcycling, obtaining new value-added products. On the contrary, mechanical recycling produces lower-quality products due to the deterioration of the physical and chemical properties of PET during reprocessing (Koo et al., 2013). The most used chemical processes are hydrolysis (Karayannidis et al., 2002; Carta et al., 2003; Sinha et al., 2010; Aguado et al., 2014), glycolysis (Vaidya and Nadkarni, 1989), methanolysis (Sako et al., 2000; Kurokawa et al., 2003), aminolysis and ammonolysis (Paszun and Spichaj, 1997; Al-Sabagh et al., 2016). The aminolysis of PET yields diamides of terephthalic acid. However, this process is less exploited on an industrial scale than other chemical recycling methods. Only the partial aminolytic degradation of PET fibres has been the subject of numerous research studies and is currently applied on a large-scale production (Maurya et al., 2017). Ethanolamine is generally employed for aminolytic degradation of PET waste to obtain bis(2-hydroxyethylene) terephthalamides (BHETAs), which can be further used as starting material for chemical upcycling (Sadeghi et al., 2011). There are few studies concerning the use of other terephthalamide diols as synthons for the synthesis of polymers. They are obtained by aminolysis of PET using commercially available β-hydroxy amines. For instance, Demarteau et al. (2020) proposed an aminolytic method for PET recycling using these amino-alcohols to produce poly (ester imide)s. Recently, terephthalamide diols have been investigated as raw materials for synthesizing polymers such as polyurethane (Shamsi et al., 2008), polyesters (Vaidya and Nadkarni, 1987; Gioia et al., 2014), poly (ester imide)s (Elsaeed and Farag, 2009), epoxy (Atta et al., 2007; Bal et al., 2017), acrylic (Karayannidis et al., 2006) and alkyd resins (Atta et al., 2013). To our knowledge, only one study uses chemically recycled PET-derived products to synthesize poly (urethane acrylates) (PUAs). Liu et al. (2023) reported the glycolysis of PET with cardanol diol to synthesize a PUA coating. Herein we proposed a microwave-assisted recycling process for innovative aminolysis of PET waste to synthesize PUAs derivative coatings. Several substituted β-hydroxy amines were synthetized and further used for the chemical depolymerization of PET waste, exploiting a well-known and healthy catalyst (sodium acetate). The first step allowed the complete and efficient depolymerization of PET with terephthalimde diols formation in just 1 h. This environmental benefit method allows the synthesis of a library of new and innovative diol monomers with tunable organic structures suitable for PUA UV-curable coatings synthesis (Figure 1). Furthermore, we performed a whole chemical, thermal and mechanical characterization of PUAs to evaluate the PUAs-derived structure and performances by modulating the final properties changing the nature of β-hydroxy amines.
All reagents such as butyraldehyde, dodecanal, octanal, hydro cinnamaldehyde, benzaldehyde, furfural, nitromethane, 1,1,3,3-tetramethylguanidine, nichel chloride hexahydrate (NiCl2.6H2O), sodium borohydride (NaBH4), ethanolamine, sodium acetate (NaOAc), cerium trichloride heptahydrate (CeCl3.7H2O), sodium iodide (NaI), isophorone diisocyanate (IPDI), PEG 400, 2-hydroxyethyl methacrylate (HEMA), benzophenone and N-methyl diethanolamine were all purchased from Merck. Polyethylene terephthalate (PET) are 2–5 mm particle size pellets obtained by crushing PET bottle scraps. As the first treatment, diols and diisocyanate were dried at 50°C under vacuum conditions for 24 h to remove residual water, and all the aldehydes were previously distilled. In the case of air and moisture-sensitive reactions, the glassware was oven dried at 100°C for more than 2 hours before use, when the reactions were performed under an inert atmosphere (nitrogen gas). For thin-layer chromatography (TLC) analysis, Merck pre-coated TLC plates (silica gel 60 GF254 0.25 mm) were used. Products were observed under UV light or stained in 2,4-dinitrophenyl hydrazine or potassium permanganate solutions.
According to Yamanaka et al. (2015) the Henry Reaction was carried out: 1,1,3,3-tetramethylguanidine (tetramethylguanidine 5.0 mol %), and different aldehydes (2a-g, 1 eq.) were added to a solution of nitromethane (5 eq.) in diethyl ether at 0°C. The mixture was stirred for 6 h at room temperature. The reaction mixture was quenched by saturated aqueous NH4Cl and extracted with Et2O (20mlx3). The combined organic layers were washed with brine, dried over Na2SO4, filtered, and concentrated under reduced pressure. Compounds 3a-e were purified by silica gel chromatography using hexanes:EtOAc, 9:1 as eluent. Compounds 3f and 3g were purified using DCM:hexane, 3:1 and hexanes:EtOAc, 8:2 as eluent, respectively. Yields are reported in Table 1.
After purification, NaBH4 (5 eq.) was slowly added to a solution of 3a-g and NiCl2.6H2O (1 eq.) in MeOH at 0 °C under an N2 atmosphere to obtain compounds 4a-g. The mixture was stirred for 30 min at 0°C and then was allowed to warm to room temperature and stirred overnight. The reaction mixture was quenched with water and then extracted with THF and a solution of NaOH (10 M). The reaction mixture was saturated using K2CO3. The separation was allowed using a solvent extraction method. The extracted phase was dried over Na2SO4, filtered, and concentrated under reduced pressure. Compounds 4a-c were purified by silica gel chromatography using CHCl3:MeOH, 7:3 as eluent. Compounds 4d and 4e were purified using CHCl3:MeOH, 5:5 as eluent (Scheme 1). Yields are reported in Table 1.
SCHEME 1. The general procedure for β-hydroxylamines (4a-g) synthesis starting from nitro-derivatives (3a-g).
Cut-pieces (2–5 mm) of PET bottles were washed with fresh acetone three times and then dried in an oven at 50°C. Using a Mixer Mill MM 500 nano, the PET pieces were ground to obtain dust scale particles. Depolymerization was performed at 180°C for 1 h under microwave irradiation using a Biotage® Initiator +. In a microwave vial, β-hydroxy amines and PET were reacted in a 2:1 wt. ratio using sodium acetate (1wt.%) as a catalyst. Finally, the depolymerisation products were purified by recrystallization using different solvents and dried in an oven at 50°C (Scheme 2). The compounds 6a, 6b and 6e’ were recrystallized from a water solution. The compound 6c was recrystallized from a solution of NaOH (10 M). The compound 6f’ was recrystallized from a solution of hot MeOH and EtOAc. The compound 6d was recrystallized from a solution of DMF and EtOH.
SCHEME 2. The general procedure for PET grinding and Mw irradiation to terephthalamide moieties 6a-f’, and preparation of UV-curable coating films 9a-g’.
Following our previous study (Pastore et al., 2022), in a 25 ml two-necked round bottom flask, 1eq. of polyol (0.8 eq. of PEG400 and 0.2 eq. of terephthalamides diol monomers previously dissolved in DMF) was reacted with IPDI (1.5 eq.) at 70°C in the presence of CeCl3.7H2O-NaI (0.1 wt.%) as a catalyst. After 2 h, the oligomer was cooled to 50°C and then end-capped with HEMA (50 wt.%), adding it dropwise to the reaction mixture. Then when the isocyanate was wholly reacted, the mixture was cooled down to room temperature and benzophenone (6 wt.%) with methyl diethanolamine (6 wt.%) was added. The polymer was deposed onto a glass plate and dried in an oven at 50°C and PUA films (Scheme 2) were obtained from a COATMASTER 509 MC after the exposition to the UV-lamp (10 min per side).
Compounds were characterized by FT-IR ATR analysis, carried out with a Perkin-Elmer Spectrum Two UATR equipped with ZnSe crystal. The measurements were performed in the frequency range 400–4,000 cm−1 at a 2 cm−1 resolution, four scans and processed by a Perkin-Elmer data manager (Spectrum). The measurements of molecular weights were performed by Agilent 1260 Infinity II Multi-Detector Suite (MDS) device, constituted by a thermostatic column compartment (G7116A), a 4-channel vacuum degasser (G7111B) an autosampler (G7129A) and three different detectors (G7800A): RI, VS and dual light scattering detector (15° and 90°). The flow rate was fixed at 1.0 ml/min, and the THF mobile phase contained 250 ppm of BHT (butylated hydroxytoluene). The system was equipped with a guard column (Agilent GPC/SEC Guard Column), followed by two columns in series (PLgel MIXED-C and PLgel MIXED-D) and the measurements were processed by Agilent GPC/SEC Software. The polystyrene standards (Mp values in the range of 580–283800 g/mol) were used for column calibration. The nuclear magnetic resonance was used to characterize PUAs, 1H-NMR and 13C-NMR spectra were recorded by Varian Mercury 400 (400 MHz or 100 MHz, respectively). The chemical shifts δ are in ppm and are referenced to residual protons in the deuterated solvent as the internal standard, such as CD3OD (3.31 ppm for 1H and 49.0 ppm for 13C) or CDCl3 (7.26 ppm for 1H and 77.0 ppm for 13C). Splitting patterns are s, for singlet; d, for doublet; t, for triplet; q, for quartet, and m, for multiplet. The UV-Lamp VL-215.G-2.15W with characteristic wavelength at 254 nm, filter size 495 × 120 mm and power [W]: 2 × 15 was used for the photo-polymerization process.
The glass transition temperature was measured by a DSC 214 Polyma (Netzsch GmbH, Selb, Germany). The samples (9–10 mg) were placed in an aluminium concave pan with a pierced lid and subjected to the subsequent thermal program: heating from −80°C to 140°C (5 min hold), cooling to −80°C (5 min hold) and heating to 140°C, all steps at 10°C/min. The thermal behaviour was determined by a Netzsch STA 2500 Regulus thermal analyzer equipped with Al2O3 crucibles. The samples (10 mg) were heated from room temperature to 900°C under an inert atmosphere with a heating rate of 10°C/min. Tensile properties of PUA films were evaluated at room temperature (23.5°C ± 1°C) according to ASTM D882 on a Z010 universal testing machine (Zwick/Roell, Ulm, Germany), and five specimens were tested for each PUA formulation. Films were cut into rectangular samples (6 mm × 80 mm), and exams were performed in displacement control with a 10 mm/min speed.
The depolymerization of PET has already been studied. Still, most works report the depolymerization step using commercially available amines (Shukla and Harad, 2006; Shah et al., 2013; Maurya et al., 2017). Therefore, to develop an innovative and complete method for the de/re-polymerization, we initially focused on the synthesis of β-hydroxy amines. Our idea was to synthesize a library of terephthalamide diols, by depolymerizing PET and then to investigate and compare their effect on the chemical, thermal and mechanical properties of the final poly (urethane acrylate) films. The synthetic approach involves a two-step process, starting from a first Henry reaction between nitromethane and organic aldehydes, providing the corresponding β-nitro alcohols, which could be further reduced to obtain the desired β-hydroxy amines. By following a synthetic procedure already reported by Yamanaka et al. (2015), we did the first step by choosing 1,1,3,3-tetramethylguanidine (TMG) as a catalyst. Although heterogeneous catalysis is usually preferred, a meagre amount of TMG allows us to obtain very high yields of products, even carrying out reactions in a gram scale. All reactions were performed up to 100 mmol scales, and we obtained yields up to 95% for the first step by using only 5mol% of the catalyst. Only a small change to the original procedure was necessary for hydro cinnamaldehyde 2d (Table 1, entry 4), which required 15mol% of TMG to provide an 90% yield of the corresponding β-nitro alcohol 3d. Regarding reactivity, we found that aliphatic linear aldehydes were more reactive towards this transformation with respect to their branched or aromatic counterparts (Concellón et al., 2006; Bosica and Polidano, 2017). We tested aromatic aldehydes, both benzaldehyde (Table 1, entry 5) and furfural (Table 1, entry 6), but they did not provide sufficient yields after the first transformation due to possible elimination of water and the formation of the corresponding nitroolefins. Further, furfural did not provide the following reduced product to allow the depolymerization step due to the instability of the furan ring under suitable acidic conditions. We also tested cinnamaldehyde (Table 1, entry 7); however, it did not convert to the corresponding β-hydroxy amine.
After β-hydroxy amines had been obtained, we proceeded towards the aminolysis of poly (ethylene terephthalate) from water bottle waste using microwave irradiation technology, slightly changing an already reported procedure (Shah et al., 2013). Thus, chemical recycling provides terephthalamides diols and a tiny fraction of oligomers through a chain scission mechanism of the polymer matrix. We added two commercially available β-hydroxy amines to the original scaffold, namely 2-aminoethanol 4e’ and diethanolamine 4f.’ These two examples were chosen to demonstrate the goodness of our protocol based on the use of different waste PET (Shukla and Harad, 2006; Ghosal and Sarkar, 2019). The chosen catalyst is an easy-to-handle and high-safety sodium acetate (NaOAc) in 1wt.%, which gave encouraging results (Shukla and Harad, 2006). Depolymerization occurs in a microwave apparatus at 180°C with a β-hydroxy amine:PET ratio of 2:1 in solvent-free conditions. We discovered that PET is completely depolymerized and the highest BHETA yield was achieved after 1 h (Supplementary Figure S1 e 2). Aminolysis reaction proceeded well with synthesized compounds starting from 4a, 4b and 4d, whose behaviour resulted like that shown by 2-aminoethanol 4e’ (Table 2, entry 5) and less with diethanolamine 4f’ (Table 2, entry 6), both in terms of PET conversion and final yield of the product (Table 2, entries 1, 2 and 4). A slight erosion in the chemical yield has been observed for β-hydroxy amine 4c, probably due to the difficulties in reaching the target site on the polymer by the highly hindering alkyl chain, providing an isolated yield of 62% (Table 2, entry 3). Along with this, the secondary amine 4f’ resulted in rather unreactive depolymerization process (Demarteau et al., 2020) despite the total conversion of PET. The high conversion rate, while obtaining low yields, confirmed that under these reaction conditions, partial depolymerization of PET occurs, providing oligomers along with a plausible formation of ethylene glycol, thus not resulting in complete depolymerization to the desired terephthalamide diol monomers.
The NMR and FT-IR analyses (Supplementary Figures S13–S18) confirm the formation of compounds 6a-d and 6e’-f’. In particular, the FT-IR spectrum of 6a (Figure 2) shows the peaks at 3,360 and 3,305 cm−1 relating to the stretching vibrations –NH and –OH. The signals at 2,955, 2,929, 2,908 and 2,870 cm−1 are associated with aliphatic –CH3, –CH and –CH2 stretching, respectively. The peak at 1617 cm−1 is related to the –C=O stretching of the amide linkage, and the peak at 1555 cm−1 corresponds to the secondary amide bending. Finally, 1,501, 1,453, and 864 cm−1 signals denote the aromatic C–C bending, –CH bending, and the para-substituted benzene ring stretching. This spectrum does not show the presence of characteristic PET signals (1,713 cm−1 –C=O stretching, 1,050 cm−1 stretching vibrations –C-O ester bonds), confirming the success of the depolymerization process and the importance of the purification step, which eliminates all traces of undecomposed PET.
Following our previous study, we developed an efficient and environmental benefit synthesis of poly (urethane acrylates) (Pastore et al., 2022). We disclosed a procedure for synthesizing PUA starting from different terephtalamide derivatives obtained from chemically recycled PET. The polymer-to-polymer approach to PUAs permits us to demonstrate the upcycling process and to evaluate the effect of compound 6a-f’ on the final properties of PUAs. First, we performed the reaction between polyethylene glycol (PEG 400, 7), diol terephthalamide (6) and isophorone diisocyanate (IPDI, 8) in the presence of CeCl3·7H2O/NaI as catalyst at 70°C. The NCO-terminated urethane prepolymer (10) was further reacted with 2-hydroxyethyl methacrylate (HEMA, 11) to obtain the urethane acrylate oligomer (UAO, 12, Scheme 3).
Finally, the photo-polymerization reaction in the presence of benzophenone (photo-initiator) and methyl diethanolamine as co-initiator was performed, obtaining the final PUA 9. To study whether the presence of compounds 6a-f’ could affect PUA properties, one blank sample was synthesized starting from PEG 400 and IPDI, without any terephthalamide diol monomer into the polymeric backbone (Table 3, entry 7). From GPC analysis, it was observed that the compound 10g’ thus obtained shows a value of Mw around 2,500 Da. Similar results were obtained for the bulkier compounds 10d (Table 3, entry 4) and 10f’ (Table 3, entry 6). NCO-terminated urethane prepolymers 10a-c and 10e’ show an Mw value of around 3,500 Da, confirming that it is possible to increase the molecular weight of the final prepolymer by changing the structure of terephthalamide diol monomers. A similar trend has been observed after the reaction between compounds 10a-g’ and HEMA (11). In particular, the best result is obtained with UAO 12a-12c and 12e’, where a Mw value of around 3,800–4,000 Da was achieved (Table 3, entries 1–3, 5).
The FT-IR analysis confirms PUA film formation (Figure 3). The pre-polymer shows the characteristic peaks at 3,331, 2,263 and 1,705 cm−1 relative to –NH, –NCO, –C=O stretching, respectively. After the reaction of compound 10a with HEMA, the peak at 2,263 cm−1 disappears, and a new signal at 1664 cm−1 related to the acrylate double bond (–C=C– stretching) is observed. Finally, the photo-polymerization leads to the formation of PUA, where the double bonds are involved in the cross-linking reaction, and the characteristic peak of the acrylate double bond (1664 cm−1) is not detected.
The effect of compounds 6a-f’ on the thermal and mechanical properties of the PUAs synthesized was evaluated. TGA analysis of UV-cured films shows two weight losses, the first related to the thermal degradation of the hard segments in the temperature range of 200°C–370°C (Figure 4). The second decomposition step, instead, is due to the degradation of soft segments and occurs in the temperature range of 370°C–500°C (Gabrielli et al., 2021).
The final polymers show a variation in thermal behaviour only in the first decomposition step. Therefore, the chemical structure of the terephtalamide diols only affects the thermal stability of the hard domains. The highest values were obtained for compounds 9e’, 9f’ and 9d (Table 4). The higher aggregation of hard segments with a better phase separation between soft and hard domains might account for the difference when the less hindered terephtalamide diol (6e’) was used. In the case of the tetrafunctional diol (6f’), the organization and packing of hard segment domains changed due to the formation of a branched network (Chattopadhyay et al., 2005). This structure contributes to slightly increase the crosslink density of compound 9f’, and the maximum decomposition temperature in the first step reached 342.1°C. The same trend was observed for compound 9d, due to the presence of an aromatic side chain. The lowest value was obtained for compound 9c, where terephtalamide diol with a longer alkyl chain was used. We hypothesize that this behavior is related to these side chains, which reduce the interaction between the hard domains, leading to lower thermal stability.
DSC was used to investigate the potential microphase separation status of PUA films by evaluating the glass transition temperature (Tg). One major glass transition (inflection point) in the DSC curves was detected for each PUA film (Figure 5A), therefore in Figure 5B only the DSC traces around the glass transition have been reported, along with the average values. Micro-phase separation is typical of polyurethane acrylate films, because of the existence of urethane groups producing hydrogen bonding in hard domains (Yong et al., 2018). In the present case, the glass transition temperature driven by the hard segment of the PUA films ranges from 41.3°C to 52.6°C, depending on the specific diol terephthalamide compound. The higher values were registered for compounds 6f’ and 6d, while in the other cases the glass transition temperature remained lower than 50°C. The presence of branched network and aromatic side chain might account for such differences. It is worth noting that the hard segment glass transition temperature of all PUA films is above room temperature, meaning that the hard segment part of these films is in a glassy state which will affect the resulting mechanical properties. In this regard, uniaxial tensile tests highlighted that a wide range of properties combinations can be achieved depending on the different segmental mobility of polymer chains, molecular weight, and testing temperature (23.5°C ± 1°C). Typical tensile stress-strain curves are collected in Figure 6A, while the corresponding tensile strength and Young’s modulus values are shown in Figure 6B. The mechanical properties of PUAs are mostly governed by multiple factors such as crosslinking density, overall phase-segregated morphology, molecular weight, crystallinity, and temperature. All samples featured a ductile behavior, but PUA films 9b, 9c, 9f’ showed a distinct yield point with quite high elastic modulus, a behavior that can be interpreted in terms of marked tensile yielding with increasing soft segment length (Kim et al., 1996). Among all the formulations investigated, the PUA film 9b exhibited the best combination of strength and ductility, though it is to be emphasized that the mechanical properties of these films compared favorably with those reported in literature (Xu et al., 2012; Dong et al., 2018; Khasarghi et al., 2019; Fu et al., 2020).
FIGURE 5. (A) Typical DSC thermogram over the entire temperature range and (B) close-up views of the DSC traces of all PUA films. In figure (B) the average value ± standard deviation of the glass transition temperature is included.
FIGURE 6. (A) Typical tensile stress-strain curves and (B) relevant tensile characteristics of all PUA films.
With this work, we demonstrated the chemical upcycling of PET using newly synthesized therephthalamide diol monomers to prepare PUA UV-curable coatings. This is important from a recycling point of view due to plastic pollution, one of the most crucial issues in our daily life. Chemical recycling is a pioneering alternative method to reduce waste and obtain innovative monomers. The challenge is not only to recycle scraps but also to convert them into high-value-added products. The aminolysis of PET by synthesising new valuable monomers can be efficiently and wholly achieved within 1 h using a biocompatible catalyst, such as sodium acetate, under solvent-free conditions at 180°C, with a microwaves assisted method. Several β-hydroxy amines were synthetized in a two-step process, further used for the chemical recycling of water bottle waste to achieve innovative monomers suitable for obtaining different chemically structured poly (urethane acrylate) UV curable coatings that could be useful in wood applications because it leads to higher adhesion, anti-ageing, wear and weather resistance and increased optical properties. Finally, we performed the chemical, thermal and mechanical characterization of PUA films. The results confirm the quality of the polymerization process and show the great potential of the developed synthesis protocols for tuning the thermal and mechanical response of PUA films to meet the requirements of diverse applications. The proposed approach can lead to various PET upcycled materials through different functionalized therephtalamides derivatives, which will extend the application scenarios of PET upcycling. We are still working on different PET waste samples, having different molecular weights, aiming to develop a general methodology. Furthermore, we want to enlarge the chemical derivatives from waste materials and consider the ethylene glycol obtained during this process.
The raw data supporting the conclusion of this article will be made available by the authors, without undue reservation.
GP: investigation, validation, writing—original draft- review and editing; RG: investigation, validation. GL: investigation, writing—original draft- review and editing; FrS: investigation, validation; RR: resources, review and editing; RB: supervision; FaS: investigation, validation, writing—original draft; JT: investigation, validation; EM: supervision, conceptualization; SG: supervision, conceptualization, methodology, writing—original draft- review and editing. All authors contributed to the article and approved the submitted version.
The work was supported by RR, University La Sapienza of Rome, Italy.
The authors are thank the Marche Applied Research Laboratory for Innovative Composites (MARLIC) of Marche Region for the post-doc fellowship to GP, and thanks HP Composites Ascoli Piceno and iGuzzini for doctoral fellowships to FrS and RG.
The authors declare that the research was conducted in the absence of any commercial or financial relationships that could be construed as a potential conflict of interest.
All claims expressed in this article are solely those of the authors and do not necessarily represent those of their affiliated organizations, or those of the publisher, the editors and the reviewers. Any product that may be evaluated in this article, or claim that may be made by its manufacturer, is not guaranteed or endorsed by the publisher.
The Supplementary Material for this article can be found online at: https://www.frontiersin.org/articles/10.3389/fchem.2023.1234763/full#supplementary-material
Achilias, D. S., Redhwi, H. H., Siddiqui, M. N., Nikolaidis, A. K., Bikiaris, D. N., and Karayannidis, G. P. (2010). Glycolytic depolymerization of PET waste in a microwave reactor. J. Appl. Polym. Sci. 118, 3066–3073. doi:10.1002/app.32737
Aguado, A., Martínez, L., Becerra, L., Arieta-araunabeña, M., Arnaiz, S., Asueta, A., et al. (2014). Chemical depolymerisation of PET complex waste: Hydrolysis vs. glycolysis. J. Mat. Cycles Waste Manag. 16, 201–210. doi:10.1007/s10163-013-0177-y
Al-Sabagh, A. M., Yehia, F. Z., Eshaq, G., Rabie, A. M., and ElMetwally, A. E. (2016). Greener routes for recycling of polyethylene terephthalate. Egypt. J. Pet., 25 (1), 53–64. doi:10.1016/j.ejpe.2015.03.001
Atta, A. M., El-Ghazawy, R. A., and El-Saeed, A. M. (2013). Corrosion protective coating based on alkyd resins derived from recycled poly (ethylene terephthalate) waste for carbon steel. Int. J. Electrochem. Sci. 8, 5136–5152. doi:10.1016/s1452-3981(23)14668-2
Atta, A. M., El-Kafrawy, A. F., Aly, M. H., and Abdel-Azim, A.-A. A. (2007). New epoxy resins based on recycled poly(ethylene terephthalate) as organic coatings. Prog. Org. Coat. 58 (1), 13–22. doi:10.1016/j.porgcoat.2006.11.001
Bal, K., Ünlü, K. C., Acar, I., and Güçlü, G. (2017). Epoxy-based paints from glycolysis products of postconsumer PET bottles: Synthesis, wet paint properties and film properties. J. Coat. Technol. Res. 14, 747–753. doi:10.1007/s11998-016-9895-0
Begley, T. H., Biles, J. E., Cunningham, C., and Piringer, O. (2004). Migration of a UV stabilizer from polyethylene terephthalate (PET) into food simulants. Food Addit. Contam. 21, 1007–1014. doi:10.1080/02652030400010447
Bosica, G., and Polidano, K. (2017). Solvent-free Henry and michael reactions with nitroalkanes promoted by potassium carbonate as a versatile heterogeneous catalyst. J. Chem. 1-9, 1–9. doi:10.1155/2017/6267036
Carta, D., Cao, G., and D’Angeli, C. (2003). Chemical recycling of poly(ethylene terephthalate) (PET) by hydrolysis and glycolysis. Environ. Sci. Pollut. Res. Int. 10 (6), 390–394. doi:10.1065/espr2001.12.104.8
Chattopadhyay, D. K., Sreedhar, B., and Raju, K. V. S. N. (2005). Effect of chain extender on phase mixing and coating properties of polyurethane ureas. Ind. Eng. Chem. Res. 44 (6), 1772–1779. doi:10.1021/ie0492348
Coates, G. W., and Getzler, Y. D. Y. L. (2020). Chemical recycling to monomer for an ideal circular polymer economy. Nat. Rev. Mat. 5, 501–516. doi:10.1038/s41578-020-0190-4
Concellón, J. M., Rodríguez-Solla, H., Concellón, C., García-Granda, S., and Díaz, M. R. (2006). Efficient addition reaction of bromonitromethane to aldehydes catalyzed by NaI: A new route to 1-Bromo-1-nitroalkan-2-ols under very mild conditions. Org. Lett. 8 (26), 5979–5982. doi:10.1021/ol062451f
Demarteau, J., Olazabal, I., Jehanno, C., and Sardon, H. (2020). Aminolytic upcycling of poly(ethylene terephthalate) wastes using a thermally-stable organocatalyst. Polym. Chem. 11, 4875–4882. doi:10.1039/D0PY00067A
Dong, F., Maganty, S., Meschter, S. J., and Cho, J. (2018). Effects of curing conditions on structural evolution and mechanical properties of UV-curable polyurethane acrylate coatings. Prog. Org. Coat. 114, 58–67. doi:10.1016/j.porgcoat.2017.09.018
Elsaeed, S. M., and Farag, R. K. (2009). Synthesis and characterization of unsaturated polyesters based on the aminolysis of poly(ethylene terephthalate). J. Appl. Polym. Sci. 112, 3327–3336. doi:10.1002/app.29527
Fu, J., Yu, H., Wang, L., and Fahad, S. (2020). Preparation and properties of UV-curable diamine-based polyurethane acrylate hard coatings. Appl. Surf. Sci. 533, 147442. doi:10.1016/j.apsusc.2020.147442
Gabrielli, S., Pastore, G., Stella, F., Marcantoni, E., Sarasini, F., Tirillò, J., et al. (2021). Chemical and mechanical characterization of licorice root and palm leaf waste incorporated into poly(urethane-acrylate) (PUA). Molecules 26 (24), 7682. doi:10.3390/molecules26247682
Geyer, R. (2020). Production, use, and fate of synthetic polymers. Environ. Impact, Soc. Issues, Prev. Solutions - Plastic Waste & Recycl. Elsevier Inc 2, 13–32. doi:10.1016/B978-0-12-817880-5.00002-5
Ghosal, K., and Nayak, C. (2022). Recent advances in chemical recycling of polyethylene terephthalate waste into value added products for sustainable coating solutions – hope vs. hype. Mat. Adv. 3, 1974–1992. doi:10.1039/D1MA01112J
Ghosal, K., and Sarkar, K. (2019). Poly(ester amide) derived from municipal polyethylene terephthalate waste guided stem cells for osteogenesis. New J. Chem. 43, 14166–14178. doi:10.1039/C9NJ02940K
Gioia, C., Vannini, M., Marchese, P., Minesso, A., Cavalieri, R., Colonna, M., et al. (2014). Sustainable polyesters for powder coating applications from recycled PET, isosorbide and succinic acid. Green Chem. 16, 1807–1815. doi:10.1039/C3GC42122H
Hopewell, J., Dvorak, R., and Kosior, E. (2009). Plastics recycling: Challenges and opportunities. Philos. Trans. R. Soc. B Biol. Sci. 364, 2115–2126. doi:10.1098/rstb.2008.0311
Jamdar, V., Kathalewar, M., and Sabnis, A. (2018). Depolymerization study of PET waste using aminoethylethanolamine and recycled product application as polyesteramide synthesis. J. Polym. Environ. 26 (1), 2601–2618. doi:10.1007/s10924-017-1149-4
Jung, H., Shin, G., Kwak, H., Hao, L. T., Jegal, J., Kim, H. J., et al. (2023). Review of polymer technologies for improving the recycling and upcycling efficiency of plastic waste. Chemosphere 320, 138089. doi:10.1016/j.chemosphere.2023.138089
Karayannidis, G. P., and Achillas, D. S. (2007). Chemical recycling of poly(ethylene terephthalate). Macromol. Mat. Eng. 292, 128–146. doi:10.1002/mame.200600341
Karayannidis, G. P., Chatziavgoustis, A. P., and Achilias, D. S. (2002). Poly(ethylene terephthalate) recycling and recovery of pure terephthalic acid by alkaline hydrolysis. Adv. Polym. Technol. 21, 250–259. doi:10.1002/adv.10029
Karayannidis, G. P., Nikolaidis, A. K., Sideridou, I. D., Bikiaris, D. N., and Achillas, D. S. (2006). Chemical recycling of PET by glycolysis: Polymerization and characterization of the dimethacrylated glycolysate. Macromol. Mat. Eng. 291 (11), 1338–1347. doi:10.1002/mame.200600243
Khasarghi, S. S., Shojaei, A., and Sundararaj, U. (2019). Bio-based UV curable polyurethane acrylate: Morphology and shape memory behaviors. Eur. Polym. J. 118, 514–527. doi:10.1016/j.eurpolymj.2019.06.019
Kim, B. K., Lee, S. Y., and Xu, M. (1996). Polyurethanes having shape memory effects. Polymer 37 (26), 5781–5793. doi:10.1016/S0032-3861(96)00442-9
Koo, H. J., Chang, G. S., Kim, S. H., Hahm, W. G., and Park, S. Y. (2013). Effects of recycling processes on physical, mechanical and degradation properties of PET yarns. Fibers Polym. 14, 2083–2087. doi:10.1007/s12221-013-2083-2
Kurokawa, H., Ohshima, M., Sugiyama, K., and Miura, H. (2003). Methanolysis of polyethylene terephthalate (PET) in the presence of aluminium tiisopropoxide catalyst to form dimethyl terephthalate and ethylene glycol. Polym. Degrad. Stabil. 79 (3), 529–533. doi:10.1016/S0141-3910(02)00370-1
Li, B., Wang, Z.-W., Lin, Q.-B., and Hu, C.-Y. (2016). Study of the migration of stabilizer and plasticizer from polyethylene terephthalate into food simulants. J. Chromatogr. Sci. 54, 939–951. doi:10.1093/chromsci/bmw025
Li, X. L., Ma, K., Xu, F., and Xu, T. Q. (2023). Advances in the synthesis of chemically recyclable polymers. Chem. Asian J. 18, e202201167. doi:10.1002/asia.202201167
Liu, Z., Zhang, H., Liu, S., and Wang, X. (2023). Bio-based upcycling of poly(ethylene terephthalate) waste to UV-curable polyurethane acrylate. Polym. Chem. 14, 1110–1116. doi:10.1039/d2py01506d
Marathe, K. V., Chavan, K. R., and Nakhate, P. (2019). Life Cycle assessment (LCA) of PET bottles – recycling of polyethylene terephthalate bottles. Elsevier Inc. 8, 149–168. doi:10.1016/B978-0-12-811361-5.00008-0
Maurya, S. D., Kurmvanshi, S. K., Mohanty, S., and Nayak, S. K. (2017). A review on acrylate-terminated urethane oligomers and polymers: Synthesis and applications. Polym. Plast. Technol. Eng. 57 (7), 625–656. doi:10.1080/03602559.2017.1332764
Pastore, G., Gabrielli, S., Giacomantonio, R., Lupidi, G., Capodaglio, S., Stella, F., et al. (2022). An efficient synthesis of bio-based Poly(urethane-acrylate) by SiO2-Supported CeCl3·7H2O–NaI as recyclable Catalyst. Results Mater. 15, 100294. doi:10.1016/j.rinma.2022.100294
Paszun, D., and Spychaj, T. (1997). Chemical recycling of poly(ethylene terephthalate). Ind. Eng. Chem. Res. 36 (4), 1373–1383. doi:10.1021/ie960563c
Sadeghi, G. M. M., Shamsi, R., and Sayaf, M. (2011). From aminolysis product of PET waste to novel biodegradable polyurethanes. J. Polym. Environ. 19 (2), 522–534. doi:10.1007/s10924-011-0283-7
Sako, T., Okajima, I., Sugeta, T., Otake, K., Yoda, S., Takebayashi, Y., et al. (2000). Recovery of constituent monomers from polyethylene terephthalate with supercritical methanol. Polym. J. 32, 178–181. doi:10.1295/polymj.32.178
Shah, R. V., Borude, V. S., and Shukla, S. R. (2013). Recycling of PET waste using 3-Amino-1-propanol by conventional or microwave irradiation and synthesis of bis-oxazin therefrom. J. Appl. Polym. Sci. 127 (1), 323–328. doi:10.1002/app.37900
Shamsi, R., Abdouss, M., Sadeghi, G. M. M., and Taromi, F. A. (2008). Synthesis and characterization of novel polyurethanes based on aminolysis of poly(ethylene terephthalate) wastes, and evaluation of their thermal and mechanical properties. Polym. Int. 58, 22–30. doi:10.1002/pi.2488
Shukla, S. R., and Harad, A. M. (2006). Aminolysis of polyethylene terephthalate waste. Polym. Degrad. Stab. 91, 1850–1854. doi:10.1016/j.polymdegradstab.2005.11.005
Sinha, V., Patel, M. R., and Patel, J. V. (2010). Pet waste management by chemical recycling: A review. J. Polym. Environ. 18, 8–25. doi:10.1007/s10924-008-0106-7
Taniguchi, I., Yoshida, S., Hiraga, K., Miyamoto, K., Kimura, Y., and Oda, K. (2019). Biodegradation of PET: Current status and application aspects. ACS Catal. 9 (5), 4089–4105. doi:10.1021/acscatal.8b05171
Vaidya, U. R., and Nadkarni, V. M. (1989). Polyester polyols from glycolyzed PET waste: Effect of glycol type on kinetics of polyesterification. J. Appl. Polym. Sci. 38 (6), 1179–1190. doi:10.1002/app.1989.070380615
Vaidya, U. R., and Nadkarni, V. M. (1987). Unsaturated polyester resins from poly(ethylene terephthalate) waste. 1. Synthesis and characterization. Ind. Eng. Chem. Res. 26 (2), 194–198. doi:10.1021/ie00062a003
Xu, H., Qiu, F., Wang, Y., Wu, W., Yang, D., and Guo, Q. (2012). UV-Curable waterborne polyurethane-acrylate: Preparation, characterization and properties. Prog. Org. Coat. 73 (1), 47–53. doi:10.1016/j.porgcoat.2011.08.019
Yamanaka, T., Kondoh, A., and Terada, M. (2015). Kinetic resolution of racemic amino alcohols through intermolecular acetalization catalyzed by a chiral brønsted acid. J. Am. Chem. Soc. 137 (3), 1048–1051. doi:10.1021/ja512238n
Keywords: circular economy, chemical recycling, PET, PUA, characterization
Citation: Pastore G, Giacomantonio R, Lupidi G, Stella F, Risoluti R, Papa E, Ballini R, Sarasini F, Tirillò J, Marcantoni E and Gabrielli S (2023) Novel terephthalamide diol monomers synthesis from PET waste to Poly(Urethane acrylates). Front. Chem. 11:1234763. doi: 10.3389/fchem.2023.1234763
Received: 05 June 2023; Accepted: 04 July 2023;
Published: 13 July 2023.
Edited by:
Alessio Zuliani, Consorzio Interuniversitario Per Lo Sviluppo Dei Sistemi A Grande Interfase, ItalyReviewed by:
Sinisa Marinkovic, Agro-Industrie Recherches et Développements, SA, FranceCopyright © 2023 Pastore, Giacomantonio, Lupidi, Stella, Risoluti, Papa, Ballini, Sarasini, Tirillò, Marcantoni and Gabrielli. This is an open-access article distributed under the terms of the Creative Commons Attribution License (CC BY). The use, distribution or reproduction in other forums is permitted, provided the original author(s) and the copyright owner(s) are credited and that the original publication in this journal is cited, in accordance with accepted academic practice. No use, distribution or reproduction is permitted which does not comply with these terms.
*Correspondence: Serena Gabrielli, c2VyZW5hLmdhYnJpZWxsaUB1bmljYW0uaXQ=
Disclaimer: All claims expressed in this article are solely those of the authors and do not necessarily represent those of their affiliated organizations, or those of the publisher, the editors and the reviewers. Any product that may be evaluated in this article or claim that may be made by its manufacturer is not guaranteed or endorsed by the publisher.
Research integrity at Frontiers
Learn more about the work of our research integrity team to safeguard the quality of each article we publish.