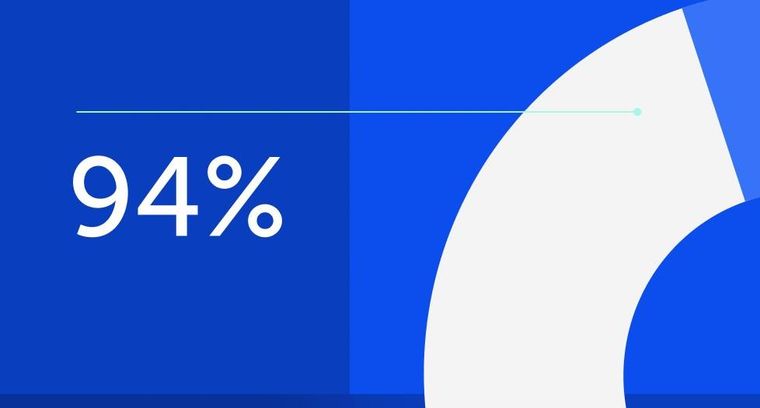
94% of researchers rate our articles as excellent or good
Learn more about the work of our research integrity team to safeguard the quality of each article we publish.
Find out more
MINI REVIEW article
Front. Chem., 19 October 2023
Sec. Green and Sustainable Chemistry
Volume 11 - 2023 | https://doi.org/10.3389/fchem.2023.1225073
This article is part of the Research TopicApplication of Emerging Technologies Aiming at The Recovery of BiomoleculesView all 7 articles
The global annual production of rice is over 750 million tons, and generates a huge amount of biomass waste, such as straw, husk, and bran, making rice waste an ideal feedstock for biomass conversion industries. This review focuses on the current progress in the transformation of rice waste into valuable products, including biochar, (liquid and gaseous) biofuels, valuable chemicals (sugars, furan derivatives, organic acids, and aromatic hydrocarbons), and carbon/silicon-based catalysts and catalyst supports. The challenges and future prospectives are highlighted to guide future studies in rice waste valorization for sustainable production of fuels and chemicals.
The excessive use of non-renewable fossil fuels causes enormous challenges, such as energy crisis and global warming (Binder and Raines, 2009; Putten et al., 2013). Biomass is the main renewable carbon resource that could replace fossil feedstocks in producing fuels and valuable chemicals (Hu et al., 2015; Sun et al., 2018; Zhang and Huber, 2018; Dodds and Gross, 2019). Compared to edible biomass such as corn, sugarcane and sweet potato, the conversion of biomass waste is promising due to non-edible, low cost and low environmental harm.
Agricultural waste, including corn stoves (leaves, stalk, and cobs), wheat straw, rice straw, rice husk, and bagasse (sugarcane), etc., is abundant with a world production of 5.1 billion tons per year in dry weight (Ho et al., 2014; Biswas et al., 2017). Particularly, the utilization of rice waste (Oryza sativa) is highly desired in many Asia countries such as China, India, Indonesia, Vietnam, etc., where rice is the main food crop (Wanninayake et al., 2021). In general, the rice waste mainly includes the rice husk and the rice straw. As reported in previous studies, 1 kg of harvested rice grain is accompanied by 1–1.5 kg of rice straw, and an ideal milling process can produce around 20% of rice husk (Abraham et al., 2016; Wanninayake et al., 2021; Shaheen et al., 2022). That means over 750–1,125 million tons of rice straw and around 150 million tons of rice husk are produced as rice waste every year around the world. Cellulose, hemicellulose, and lignin are the main components of rice waste (Chieng and Kuan, 2022). In rice straw, the lignocellulosic composition is 32%–47% of cellulose, 19%–27% of hemicellulose, and 5%–24% of lignin, and in rice husk, the predominant composition is 25%–35% of cellulose, 18%–21% of hemicellulose, and 26%–31% of lignin (Binod et al., 2010; Santos et al., 2016). Besides the organic components, SiO2 (>80%) is dominant with other oxides containing Al, Fe, Ca, Na, Mg, K, etc., that can be utilized for silica production or other materials based on these elements (Chieng and Kuan, 2022).
Rice waste can be converted into fuels and valuable chemicals, such as biochar, biofuels and platform molecules (e.g., glucose) (Figure 1) (Uddin et al., 2021; Shaheen et al., 2022; Shukla et al., 2022). Various methodologies have been developed for the utilization of rice waste, such as combustion, fast and slow pyrolysis, gasification and microbial fermentation. Herein, the synthesis and applications of rice waste as biochar and biofuels are firstly introduced, which act as carbon-neutral alternatives to fossil fuels. Then, the transformation of rice waste into platform chemicals is highlighted in providing renewable carbon feedstocks for sustainable chemical production with the existing industrial facilities. Furthermore, other applications of rice waste, such as in the synthesis of carbon catalyst or support materials, are also introduced. The summary of the valuable products derived from rice waste in recent years is presented in Table 1. At the end, the challenges and perspectives for the potential sustainable applications and the related chemical processes are discussed to guide future development.
Biochar is a carbonaceous product that can be produced from the thermal decomposition of biomass, such as pyrolysis or gasification, in an oxygen-limited environment (Zou et al., 2022). Biochar derived from rice waste can feature several advantages including low cost, high carbon content, highly porous structure and rich functional groups which make it suitable for various applications in energy fuels, environmental science and new materials (Zou et al., 2022).
Biochar is utilized as excellent adsorbents for water treatment (Foong et al., 2022). To remove lead from wastewater, Pham et al. used alginate to modify biochar derived from rice husk, which provides higher surface area and more surface oxygen-contained functional groups, resulting in the uptake capacity increased from 41.2 to 112.3 mg/g, compared to the origin biochar (Pham et al., 2022). Zhang et al. increased the surface area of biochar through applying a higher pyrolysis temperature, which can significantly improve the absorption performance of biochar towards heavy metal, such as to reduce the concentration of arsenic and cadmium in the aqueous phase of sediment (Zhang et al., 2020). Yi et al. utilized stainless steel pickling waste liquor and rice straw to synthesize magnetic biochar (Yi et al., 2021). The adhered iron oxides (Fe3O4, Fe2O3, FeO) induced π-π interaction, hydrogen bonding and electrostatic interaction, which could remarkably enhance the adsorption capacity of biochar, e.g., up to ∼111.5 mg/g toward crystal violet in wastewater (Yi et al., 2021).
Biochar with excellent adsorption capacity can also serve in soil amendment to enhance soil organic carbon content, bulk density and microbial activity (Shaheen et al., 2022). Singh et al. prepared rice husk biochar with high surface area, rich micropores and various composition (N, P, K, Si, etc.), which can effectively increase the total N, C, and P elements in soil, regarding as a potential amendment for nutrient-deficient soils (Singh et al., 2018). Bu et al. further revealed that N leaching in biochar could be effectively reduced through synthesis rice waste biochar at high pyrolysis temperature (∼650 °C), which is attributed to the improvement of the net negative surface charge and the anion exchange capacity of biochar (Bu et al., 2019). Furthermore, biochar can immobilize heavy metals and herbicides to reduce their bioavailability in the soil (Asadi et al., 2021). For example, Lu et al. found that when 5% rice straw biochar treated the sandy loam soil, it could effectively immobilize heavy metals of Cd, Cu, Pb and Zn, thus reducing their mobility and bioavailability in contaminated soils (Lu et al., 2017). Liu et al., found that the addition of proper amount of biochar can reduce the concentration of Cd in lettuce shoots in the lightly heavy-metal-polluted greenhouse soils by immobilize the Cd in the biochar (Zhang et al., 2017).
As discussed above, the conversion of rice waste into biochar provides a sustainable production of excellent adsorbents for water treatment, the immobilization of heavy metals and herbicides, as well as soil amendment for nutrient-deficient soils. Besides increasing the nutrient elements in soil, biochar can be utilized for water and fertilizer retention and adsorption of organic matter. Moreover, the cost-effective biochar could be activated, and utilized as activated carbon in water treatment, air purification, medical, food industry and other fields, to remove pollutants, purify water and air, detoxification and so on. Most of these applications of biochar are strongly depends on its high surface area, which can be enhanced via increasing the pyrolysis temperature but is energy consuming. The preparation of biochar with high surface area at relative low temperature by optimizing synthesis conditions, such as gaseous atmosphere, could be interesting for future investigations. Decoration of biochar with surface functional groups or introducing metal active sites is promising to enhance their performance and expand their applications, such as catalysts or beyond.
Biofuels are fuels derived directly or indirectly from renewable biomass (Sharma et al., 2020). Liquid biofuels are of particular interest in replacing fossil fuels, especially for transportation. The two most common types of biofuels are bioethanol and biodiesel. Other biofuels, such as methane gas and biogas are gaseous biofuels, which can be derived from the decomposition of biomass in the absence of oxygen. Through thermochemical and biotechnological pathways, rice waste can be converted into liquid (biodiesel, bio-oil, bioethanol, biobutanol, etc.) or gaseous (biohydrogen and biomethane) biofuels (Abraham et al., 2016; Gupte et al., 2022).
Zullaikah et al. conducted a non-catalytic method to produce biodiesel in situ from rice bran using a subcritical water/methanol mixture, achieving 67.4% of fatty acid methyl esters yield (200°C, 4 MPa). The high yield has been proposed by the subcritical conditions promoting the hydrolysis of rice bran oil into free fatty acids, followed by methyl-esterification (Zullaikah et al., 2017). Generally, introducing catalysts can speeds up a chemical reaction, or lowers the reaction conditions. Therefore, Chen et al. employed a series of Ni/CeO2 catalysts to catalyze the hydro-liquefaction of rice straw for the production of bio-oil, achieving a maximum rice straw conversion of 89.08% and a bio-oil yield of 66.7% (290°C, 2 MPa) (Chen D. et al., 2018). Compared to thermocatalytic process, microbial fermentation can produce liquid biofuels from biomass at relative milder conditions. Mihajlovski et al. processed waste rice grains through a pre-saccharification step, followed by simultaneous saccharification and fermentation using waste brewer’s yeast, achieving a bioethanol yield of 4.69% (30°C, under atmospheric pressure) (Mihajlovski et al., 2018). Valles et al. compared the efficiency of the simultaneous saccharification and fermentation (SSF) process and the hydrolysis and fermentation (SHF) process for biobutanol production from rice straw by Clostridium beijerinckii DSM 6422, revealing that SSF was more efficient than SHF with a biobutanol productivity of 0.114 g L−1h−1 (37°C, under atmospheric pressure) (Valles et al., 2020).
Biohydrogen and biomethane are the major renewable gaseous biofuels generated from biomass. Xu et al. produced renewable biohydrogen from catalytic reforming/cracking of rice husk, using a Fe-Ni catalyst supported on the rice husk pyrolysis carbon, which provides a biohydrogen concentration of 50.5% (Xu et al., 2018). Recently, Zeng et al. prepared a bifunctional catalyst, Ce0.7Ni1Ca5, for the efficient production of hydrogen-rich gas from steam gasification of rice husk, achieving a higher biohydrogen concentration of 85.81 ± 0.39 vol% and yield of 35.82 ± 0.28 mmol/g biomass (Zeng et al., 2022). Fermentation is a cost-effective process that often be utilized in the production of biogases in large scale. Solid-state fermentation was applied in producing biohydrogen from rice husk by Tosuner et al., and they found that the volume and yield of biohydrogen formation increased with decreasing particle size of rice husk, the highest biohydrogen formation volume was 29.26 mL under the particle size of <74 μm (yield: 5.9 mL/g substrate) (Tosuner et al., 2019). In producing biomethane from rice waste, optimizing the thermal pretreatment, ozonolysis induction and anaerobic co-digestion can enhance the productivity (He et al., 2017; Patil et al., 2021). In anaerobic digestion process, high thermal pretreatment temperature (e.g., 210°C) may cause longer biogasification period due to the formation of byproducts, thus He et al. found a proper thermal pretreatment temperature (150°C) achieving biomethane yield of 134 mL/(g-Volatile solid added) (He et al., 2017). Ozonolysis induction could help in reducing the complexity of the substrates and increasing accessibility to cellulose and hemicellulose during thermal hydrolysis rice straw (Patil et al., 2021). The co-digestion process can treat multiple waste in one facility that can keep a balanced nutrient and sufficient feedstock supply, thus improving the net biogas yield (Chen et al., 2015; Jha et al., 2021).
Biofuels is a renewable and carbon-neutral alternative to fossil fuels; however, the direct use of biofuels also have shortcomings. For example, ethanol has a lower energy density than gasoline, and the use of ethanol to partially replace gasoline creates a net energy loss. Therefore, using biofuels as feedstocks for producing bulk chemicals, such as synthesis ethylene from bioethanol dehydration, are value-added strategy (Wang et al., 2019). Hydrogen and methane are also key feedstocks in various chemical processes, such as ammonia synthesis and Fischer-Tropsch process. Exploring efficient and energy saving process for converting rice waste into biofuels is another important strategy. Using renewable energies (e.g., solar) and developing high-performance catalysts to reduce the reaction conditions are promising. Moreover, synthesis microbial by biotechnology for the efficient and selective conversion of rice wastes could promote their applications in large-scale as renewable feedstocks.
Besides biochar and biofuels, rice waste can also be converted into valuable carbon-based chemicals through hydrolysis, dehydration, oligomerization, decarboxylation/decarbonylation, etc. The desired products include sugars, furan derivatives, organic acids, and aromatic hydrocarbons that can be applied in the pharmaceutical, biological, medical, energy, and chemical industries.
Typically, sugars such as glucose, fructose, xylose, etc., are the primary products generated from the fermentation/hydrolysis of cellulose, hemicellulose, and lignin that are the main components of rice waste (Karimi et al., 2006). For the hydrolysis behavior of rice straw using dilute sulfuric acid, Karimi et al. found that in the first stage of the process, the acid-catalyzed hydrolysis of rice straw mainly depolymerized xylan into xylose with a maximum yield of 80.8% (0.5% acid, 10 min, 15 bar). Without further acid addition, the yield of glucose from glucan only increased up to 26.6% in the second stage. With the addition of more sulfuric acid (0.5%) prior to the second stage, it can achieve a glucose yield of 46.6% from glucan (3 min, 30 bar) (Karimi et al., 2006). Moreover, the pretreatment of alkali could effectively remove lignin and increase the accessibility of the substrate, and it can also change the structure of lignocellulosic biomass and make it easier to be hydrolyzed (Qi et al., 2019). Therefore, pretreatment via alkali could reduce the acid content needed for the reaction. Qi et al. found that using alkali-pretreated rice straw could significantly reduce the acid addition to 0.015 wt% HCl required for hydrolysis, and also promote the formation of glucose at a yield of 52.1% at 200°C for 60 min (un-pretreated: 13.06%) (Qi et al., 2019). Compared to the harsh conditions using liquid acids, enzyme could convert rice waste into sugars under milder conditions. For example, Park et al. have developed a facile approach to recover glucose and fructose from enzymatic saccharification of rice straw at 50°C under atmospheric pressure, resulting in yields of 40.1% for glucose and 43.5% for fructose, respectively (Park et al., 2009).
Furan derivatives can be directly produced from rice waste as well (Bariani et al., 2020). For example, Bariani et al. synthesis furfural from sulphuric acid-catalyzed hydrolysis of rice husk, obtaining a furfural yield of 6.0 w/w% based on oven-dried rice husk weight under optimized conditions (200°C, 0.1% w/w of acid/rice husk, and 40 min) (Bariani et al., 2020). By using boron-doped biochar catalysts, Ofrasio et al. investigated the production of furan and 5-hydroxymethylfurfural (HMF) from one-pot hydrolysis-dehydration of rice straw, wheat straw or corncob, respectively, and the highest yields of furan (12%) and HMF (21%) were achieved with rice straw (Ofrasio et al., 2020). Yu et al. studied the one-pot catalysis of food waste into HMF using tri/tetravalent metal catalysts (Yu et al., 2017). SnCl4 offers the highest HMF yield of 22.7 wt% generated from cooked rice, which is attributed to the high activity of SnCl4 in the acid-catalyzed hydrolysis of starch into glucose, followed by glucose isomerization and dehydration into HMF (Yu et al., 2017; Wang et al., 2021). Further, Yu et al. reported a higher HMF yield of 35.2 mol% through Al(Ⅲ)-organic acid system in the conversion of rice waste to HMF, and the addition of maleic acid can increase the HMF selectivity by suppressing the loss of sugars and side reactions (Yu et al., 2019).
The conversion of rice waste to organic acids usually starts from the degradation of rice waste to hemicellulose or oligosaccharides, then transformed into sugars. The sugars, as the platform chemicals, undergo a series of processes including isomerization, dehydration, rehydration, etc. to reach the target products of organic acids. Ukarde et al. utilized polyethyleneimine-functionalized acidic ionic liquid catalysts for the conversion of rice straw into organic acids (formic, acetic, and levulinic acids), and it was observed that the catalyst with a [HSO4]− counter ions exhibited superior efficiency compared to other tested catalysts since it has the highest hydrophilicity and Hammett acidity (Ukarde and Pawar, 2022). Moreover, the sodium hydroxide pretreated rice straw was found to provide higher yields of levulinic acid (65.5%) and formic acid (75.8%) than untreated rice straw (levulinic acid: 49.8%; formic acid: 50.5%) (Ukarde and Pawar, 2022). Typically, these organic acids are produced along with the furan derivatives (Wanninayake et al., 2021). To convert these derivatives into acids, Peng et al. developed a one-pot chemo-enzymatic reaction system utilizing Sn-sepiolite catalyst and immobilized E. coli TS whole-cell biocatalyst for the efficient synthesis of furoic acids from alkali pretreated rice straw (Peng et al., 2019). The furfural generated at the initial stage under Sn-loaded sepiolite at a yield of 42.2%, followed by the completely converted into furoic acid under immobilized E. coli TS whole-cell biocatalyst (Peng et al., 2019).
Aromatic hydrocarbons, such as benzene, toluene, and xylene, are commonly produced from rice waste through the catalytic fast pyrolysis process (Zhang et al., 2018a; Li et al., 2019). H-ZSM-5 has strong acidity and heat resistance as well as excellent selective cracking catalysis and isomerisation catalytic properties, and thus, is the most frequently used catalyst in the conversion of rice wastes into aromatic hydrocarbon with high activity and selectivity (Zheng et al., 2017; Zhang et al., 2018b; Li et al., 2020). The alkali modification of ZSM-5 by potassium hydroxide could increase mesoporosity and improve the mass transfer of ZSM-5, which can significantly enhance the formation of aromatics during pyrolysis of microcrystalline cellulose extracted from rice straw, with a total aromatics relative peak area of 33.05% (0.4 M NaOH) that was higher than ZSM-5 without modification (24%) (Nishu et al., 2021). Li et al. compared the performance of HZSM-5 treated using different types of bases in the microwave-assisted catalytic fast pyrolysis of rice husk, which shows that the organic base treatment outperformed the inorganic base treatment, resulting in a 4.3% increase in monocyclic aromatic hydrocarbons and 4.6% reduction in coke formation (Li et al., 2020). Besides the alkali pretreatment, the aromatic product distribution and coke formation strongly depends on the control of hierarchical mesoporous structure (Zhang et al., 2018a; Li et al., 2019). It was found that hierarchical structure is formed by adding a certain amount of hexadecyl trimethyl ammonium bromide (0.01%) into ZSM-5, which enhanced the yield of aromatics (26.8%) and decreased the coke formation (39.2%) compared with the bare ZSM-5 (23.6%, 45.1%). (Zhang et al., 2018b). Moreover, incorporating suitable metals or other ligands into mesoporous catalysts has been shown to have an effect on the active sites of the catalysts which may influence the production of aromatic hydrocarbons. For instance, Nishu et al. synthesized Ni modified ZSM-5 for the pyrolysis of rice straw, which offers a higher selectivity to aromatics (47%) than unmodified catalyst (44%) due to the improved acidity (Nishu et al., 2023). Nevertheless, the introduction of ammonium ions in the organosilanes had a negative effect on the catalytic property of ZSM-5 that led to a lower yield (19.7%) of aromatics compared to unmodified catalyst (25.6%) due to the decreased acidity (Chen H. et al., 2018).
Sugars, furan derivatives, organic acids, aromatic hydrocarbons, etc., are generally produced from rice waste via thermocatalytic process. Most of these chemicals are platform chemicals, such as sugars, which could connect the conversion of rice wastes with the current chemical process for producing valuable chemicals. For example, glucaric acid or HMF derived from glucose can act as renewable precursors to synthesize adipic acid (Wan and Lee, 2021). The conversion efficiency strongly depends on the activity of catalysts, such as acids, alkalis, enzymes, metal oxides, etc. However, developing highly selective catalysts for specific products production is still a challenge in biomass conversion, including rice wastes, which often suffers from the lack understanding of reaction mechanisms due to the comprehensive reactions occurred.
Besides the catalytic conversion of rice waste into valuable products, various catalysts can also be synthesized from rice waste to drive chemical reactions. Carbon materials can be synthesized from rice waste via hydrothermal carbonization, which can be used as catalysts or as supports for functional groups and metals (Wataniyakul et al., 2018). In some cases, rice waste derived products could also be applied as feedstocks and catalysts at the same time.
Sulfonate treatment is a common method used to introduce strong acid sites on the hydrothermal carbon. For instance, sulfonated rice husk has been utilized for the in-situ transesterification of microalgae at ambient temperature, obviated the steps for oil extraction after the reaction and pre-treatment of biodiesel before its application (Wadood et al., 2019). In the hydrolysis of cellulose, sulfonated hydrothermal carbon catalysts derived from defatted rice bran exhibits higher activity than the commercial Amberlyst 16 WT catalyst, with glucose and HMF yields of 19% and 46%, respectively (Wataniyakul et al., 2018).
The incorporation of active metals is another commonly used method to introduce active sites on hydrothermal carbon. Ni-loaded rice husk derived biochar was utilized as catalysts for steam gasification of food waste, exhibiting superior catalytic performance (∼68.88% gas yield) compared to Ni-loaded commercial α-alumina support (∼43.7% gas yield) (Farooq et al., 2021). This is attributed to its high reducibility, high nickel dispersion, abundant inherent K and Ca as co-catalysts, and moderate surface area (Farooq et al., 2021). Similarly, the humins generated from the reaction of starch-rich rice waste to HMF, can be valorized as biochar-supported Lewis acid catalysts after impregnation with AlCl3 followed by carbonization, which can catalyze glucose-to-fructose isomerization with a fructose yield of up to 14 Cmol% (Xiong et al., 2021). Moreover, Yuan et al. synthesized a N-doped biochar supported MgFe2O4 catalyst through one-pot pyrolysis of N-containing precursors, cooked rice waste, and metal salts (Yuan et al., 2023). The catalysts exhibit superior capacity (1,052.83 mg/g) for efficient low-temperature desulfurization of H2S with high tolerance and stability under harsh environmental conditions (Yuan et al., 2023).
The ash obtained from the combustion of rice waste contains a high concentration of silica which can be utilized as an excellent catalyst support. Franco et al. utilized microwave-assisted acid dissolution to effectively remove metal ions from rice husk, resulting in a highly pure amorphous mesoporous silica (>95%) (Franco et al., 2018). The incorporation of iron oxide in this silica framework could efficiently promote toluene alkylation with 100% selectivity toward monoalkylated products (Franco et al., 2018). Azat et al. developed an eco-friendly method for the production of high-purity silica from rice husk, utilizing dry citric acid pre-treatment and direct thermal treatment, which results in a silica yield of 20% with a purity as high as 99% (Azat et al., 2019). Kamari and Ghorbani synthesized magnetic MCM-41 with a highly ordered hexagonal structure using the silica extracted from rice husk through leaching with HCl (Kamari and Ghorbani, 2020). Benefitting from the high silica content in rice husk, the Si-Fe/S/N-RH3 electrocatalyst exhibited high proportions of pyridine N, graphite N and especially Fe-Nx and thiophene S for efficient oxygen reduction reaction, demonstrating superior activity compared to Pt/C benchmark electrocatalyst and other Fe-based electrocatalysts (Wang et al., 2022). Additionally, self-doped Si can improve the graphitization degree of the catalyst, leading to enhanced stability and methanol tolerance ability (Wang et al., 2022).
The advantage of the rice waste derived catalysts is due to their abundant and low cost. However, the purity limited their use as catalysts or supports. Seeking suitable reactions requiring low catalyst purity maybe expand their applications. Moreover, these supports consists of other elements, such as K. Developing high-performance catalysts with utilization of these elements as co-catalyts could be promising.
Biomass has attracted significant attentions in the fields of biofuel and chemical conversions, as well as environmental studies. In particular, utilizing rice waste as a biomass resource holds tremendous promise for the agricultural industry. The conversion of rice waste into valuable products not only maximizes the economic benefits of agricultural biomass resources but also plays a crucial role in mitigating energy and environmental issues. This review provides a comprehensive overview of the current advancements in converting rice waste into useful products. Despite the progress made, commercializing rice waste still encounters significant challenges.
Typically, the utilization of rice waste requires multiple pretreatment processes including physical methods such as mechanical crushing and grinding, ultrasound, microwave, etc., as well as chemical methods including catalytic hydrothermal process with catalysts or enzymes and acid/alkali pretreatment. The physical pretreatment methods can reduce the size and increase the uniformity of rice waste, as well as enhance its surface area. In comparison, chemical pretreatment methods such as hydrothermal processing, acid-base treatment, and oxidation treatment are essential for degrading organic substances in rice waste. These methods break down complex organic compounds into simpler or more easily processed substances to facilitate subsequent processing steps. Despite the fact that chemical pretreatment can enhance waste availability, the processing process requires a significant amount of energy and water resources, resulting in substantial wastewater generation. This necessitates post-treatment, which increases treatment costs and environmental pressure. Additionally, high-temperature and high-pressure operating conditions pose equipment and safety risks. In order to reduce energy and water costs while addressing environmental concerns, the exploration of novel pretreatment methods utilizing regenerated high-performance catalysts or biochemical processes shows promise in achieving the pretreatment at lower costs and milder conditions.
The conversion of rice waste into valuable chemicals has been extensively studied. The current biofuel production process requires harsh reaction conditions such as high temperature, high pressure, catalyst participation and a non-oxygen environment, which is energy-intensive. Furthermore, the yields of liquid and gaseous biofuels are significantly lower than their theoretical yield. To achieve efficient biofuel production from rice waste under milder conditions, the development of high-performance catalysts and the integrated application of multiple processes could be imperative. In this case, an example of practical application could be the integration of rice waste pyrolysis into liquid fuels at a relatively low temperature and catalytic reforming of gaseous fuels for high hydrogen density. In the conversion of rice waste to chemicals, compared to solid acids, liquid acids, such as H2SO4 and HCl, can directly contact and react with rice waste to promote catalytic reactions, but this results in wastewater and environmental issues. Ionic liquid catalysts possess high accessibility and activity, making them a potential candidate for this process, however, they have not been extensively tested. Reducing the cost and enhancing reusability are pivotal factors in utilizing ionic liquids. Another viable approach could involve downsizing or breaking down waste into smaller components, thereby augmenting the surface mass transfer of waste on solid surfaces.
In practice, tailoring catalysts with high activity and stability is essential, especially in complex processes like catalytic reforming where multiple reactions occur simultaneously and rapid deactivation of catalysts is common. To enhance catalyst activity and stability, it is crucial to uncover transformation pathways and coke generation mechanisms. However, due to the complexity of reaction conditions, understanding of the reaction mechanism remains limited. Therefore, greater emphasis should be placed on investigating these reactions under in situ conditions to facilitate the development of high-performance catalysts for large-scale applications. With the development of efficient pretreatment techniques and an improved understanding of reaction mechanisms for designing catalysts with high activity and stability, we firmly believe that sustainable production of valuable chemicals and fuels from rice waste can be achieved, benefiting our society in the near future.
WZ: Investigation, Conceptualization, Visualization, Writing-original draft, Funding acquisition. XX: Writing-original draft, Formal analysis, Validation. YY: Formal analysis, Validation. ZW: Funding acquisition, Project administration, Supervision, Writing-review and editing. All authors contributed to the article and approved the submitted version.
The authors thank the support of Fundamental Research Funds for the Central Universities (buctrc202231), and the Talent Project from Xihua University (Z211038).
The authors declare that the research was conducted in the absence of any commercial or financial relationships that could be construed as a potential conflict of interest.
All claims expressed in this article are solely those of the authors and do not necessarily represent those of their affiliated organizations, or those of the publisher, the editors and the reviewers. Any product that may be evaluated in this article, or claim that may be made by its manufacturer, is not guaranteed or endorsed by the publisher.
Abraham, A., Mathew, A. K., Sindhu, R., Pandey, A., and Binod, P. (2016). Potential of rice straw for bio-refining: an overview. Bioresour. Technol. 215, 29–36. doi:10.1016/j.biortech.2016.04.011
Asadi, H., Ghorbani, M., Rezaei-Rashti, M., Abrishamkesh, S., Amirahmadi, E., Chengrong, C., et al. (2021). Application of rice husk biochar for achieving sustainable agriculture and environment. Rice Sci. 28 (4), 325–343. doi:10.1016/j.rsci.2021.05.004
Azat, S., Korobeinyk, A. V., Moistakas, K., and Inglezakis, V. J. (2019). Sustainable production of pure silica from rice husk waste in Kazakhstan. J. Clean. Prod. 217, 352–359. doi:10.1016/j.jclepro.2019.01.142
Bariani, M., Boix, E., Cassella, F., and Cabrera, M. N. (2020). Furfural production from rice husks within a biorefinery framework. Biomass Convers. bior. 11 (3), 781–794. doi:10.1007/s13399-020-00810-1
Binder, J. B., and Raines, R. T. (2009). Simple chemical transformation of lignocellulosic biomass into furans for fuels and chemicals. J. Am. Chem. Soc. 131, 1979–1985. doi:10.1021/ja808537j
Binod, P., Sindhu, R., Singhania, R. R., Vikram, S., Devi, L., Nagalakshmi, S., et al. (2010). Bioethanol production from rice straw: an overview. Bioresour. Technol. 101 (13), 4767–4774. doi:10.1016/j.biortech.2009.10.079
Biswas, B., Pandey, N., Bisht, Y., Singh, R., Kumar, J., and Bhaskar, T. (2017). Pyrolysis of agricultural biomass residues: comparative study of corn cob, wheat straw, rice straw and rice husk. Bioresour. Technol. 237, 57–63. doi:10.1016/j.biortech.2017.02.046
Bu, X. L., Su, J., Xue, J., Wu, Y., Zhao, C., and Wang, L. (2019). Effect of rice husk biochar addition on nutrient leaching and microbial properties of Calcaric Cambisols. J. Soil Water Conserv. 74 (2), 172–179. doi:10.2489/jswc.74.2.172
Chen, D., Chen, D., Ma, Q., Wei, L., Li, N., Shen, Q., et al. (2018a). Catalytic hydroliquefaction of rice straw for bio-oil production using Ni/CeO2 catalysts. J. Anal. Appl. Pyrol. 130, 169–180. doi:10.1016/j.jaap.2018.01.012
Chen, H., Cheng, H., Zhou, F., Chen, K., Qiao, K., Lu, X., et al. (2018b). Catalytic fast pyrolysis of rice straw to aromatic compounds over hierarchical HZSM-5 produced by alkali treatment and metal-modification. J. Anal. Appl. Pyrol. 131, 76–84. doi:10.1016/j.jaap.2018.02.009
Chen, X., Yuan, H. R., Zou, D. X., Liu, Y. P., Zhu, B. N., Chufo, A., et al. (2015). Improving biomethane yield by controlling fermentation type of acidogenic phase in two-phase anaerobic co-digestion of food waste and rice straw. Chem. Eng. J. 273, 254–260. doi:10.1016/j.cej.2015.03.067
Chieng, S., and Kuan, S. H. (2022). Harnessing bioenergy and high value-added products from rice residues: a review. Biomass Convers. bior. 12 (8), 3547–3571. doi:10.1007/s13399-020-00891-y
Dodds, D. R., and Gross, R. A. (2019). Chemicals from biomass. Science 318, 1250–1251. doi:10.1126/science.1146356
Eduah, J. O., Nartey, E. K., Abekoe, M. K., Breuning-Madsen, H., and Andersen, M. N. (2019). Phosphorus retention and availability in three contrasting soils amended with rice husk and corn cob biochar at varying pyrolysis temperatures. Geoderma 341, 10–17. doi:10.1016/j.geoderma.2019.01.016
Farooq, A., Jang, S. H., Lee, S. H., Jung, S. C., Rhee, G. H., Jeon, B. H., et al. (2021). Catalytic steam gasification of food waste using Ni-loaded rice husk derived biochar for hydrogen production. Chemosphere 280, 130671. doi:10.1016/j.chemosphere.2021.130671
Foong, S. Y., Chan, Y. H., Chin, B. L. F., Lock, S. S. M., Yee, C. Y., Yiin, C. L., et al. (2022). Production of biochar from rice straw and its application for wastewater remediation-An overview. Bioresour. Technol. 360, 127588. doi:10.1016/j.biortech.2022.127588
Franco, A., De, S., Balu, A. M., Romero, A. A., and Luque, R. (2018). Integrated mechanochemical/microwave-assisted approach for the synthesis of biogenic silica-based catalysts from rice husk waste. ACS Sustain. Chem. Eng. 6 (9), 11555–11562. doi:10.1021/acssuschemeng.8b01738
Gupte, A. P., Basaglia, M., Casella, S., and Favaro, L. (2022). Rice waste streams as a promising source of biofuels: feedstocks, biotechnologies and future perspectives. Renew. Sust. Energ. Rev. 167, 112673. doi:10.1016/j.rser.2022.112673
He, L., Huang, H., Zhang, Z. Y., Lei, Z. F., and Bin-Le Lin., (2017). Energy recovery from rice straw through hydrothermal pretreatment and subsequent biomethane production. Energ. Fuel. 31 (10), 10850–10857. doi:10.1021/acs.energyfuels.7b01392
Ho, D. P., Ngo, H. H., and Guo, W. (2014). A mini review on renewable sources for biofuel. Bioresour. Technol. 169, 742–749. doi:10.1016/j.biortech.2014.07.022
Hoang, P. H., Dat, N. M., Cuong, T. D., and Tung, D. T. (2020). Production of 5-hydroxymethylfurfural (HMF) from rice-straw biomass using a HSO3-ZSM-5 zeolite catalyst under assistance of sonication. RSC Adv. 10 (23), 13489–13495. doi:10.1039/D0RA02037K
Hu, L., Lin, L., Wu, Z., Zhou, S., and Liu, S. (2015). Chemocatalytic hydrolysis of cellulose into glucose over solid acid catalysts. Appl. Catal. B Environ. 174-175, 225–243. doi:10.1016/j.apcatb.2015.03.003
Jha, B., Chandra, R., Vijay, V. K., Subbarao, P. M. V., and Isha, A. (2020). Utilization of de-oiled rice bran as a feedstock for renewable biomethane production. Biomass Bioenerg. 140, 105674. doi:10.1016/j.biombioe.2020.105674
Jha, B., Isha, A., Trivedi, A., Chandra, R., and Subbarao, R. M. V. (2021). Anaerobic co-digestion of rice straw and de-oiled rice bran for biomethane production. Energ. Rep. 7, 704–710. doi:10.1016/j.egyr.2021.01.032
Kamari, S., and Ghorbani, F. (2020). Extraction of highly pure silica from rice husk as an agricultural by-product and its application in the production of magnetic mesoporous silica MCM-41. Biomass Convers. bior. 11 (6), 3001–3009. doi:10.1007/s13399-020-00637-w
Karimi, K., Kheradmandinia, S., and Taherzadeh, M. J. (2006). Conversion of rice straw to sugars by dilute-acid hydrolysis. Biomass Bioenerg. 30 (3), 247–253. doi:10.1016/j.biombioe.2005.11.015
Li, Z., Zhong, Z., Zhang, B., Wang, W., Seufitelli, G. V. S., and Resende, F. L. P. (2020). Effect of alkali-treated HZSM-5 zeolite on the production of aromatic hydrocarbons from microwave assisted catalytic fast pyrolysis (MACFP) of rice husk. Sci. Total Environ. 703, 134605. doi:10.1016/j.scitotenv.2019.134605
Li, Z., Zhong, Z., Zhang, B., Wang, W., Wu, W., and Resende, F. L. (2019). Catalytic fast pyrolysis of rice husk over hierarchical micro-mesoporous composite molecular sieve: analytical Py-GC/MS study. J. Anal. Appl. Pyrolysis. 138, 561–568. doi:10.1016/j.wasman.2019.11.012
Lu, K., Yang, X., Gielen, G., Bolan, N., Ok, Y. S., Niazi, N. K., et al. (2017). Effect of bamboo and rice straw biochars on the mobility and redistribution of heavy metals (Cd, Cu, Pb and Zn) in contaminated soil. J. Environ. Manage. 186, 285–292. doi:10.1016/j.jenvman.2016.05.068
Mihajlovski, K., Radovanovi, Z., Carevi, M., and Dimitrijevic-Brankovic, S. (2018). Valorization of damaged rice grains: optimization of bioethanol production by waste brewer’s yeast using an amylolytic potential from the Paenibacillus chitinolyticus CKS1. Fuel 224, 591–599. doi:10.1016/j.fuel.2018.03.135
Mohammad, G., Hossein, A., and Sepideh, A. (2019). Effects of rice husk biochar on selected soil properties and nitrate leaching in loamy sand and clay soil. ISWCR 7, 258–265. doi:10.1016/j.iswcr.2019.05.005
Nishu, L., Liu, R., Rahman, M. M., Li, C., Chai, M., Sarker, M., et al. (2021). Catalytic pyrolysis of microcrystalline cellulose extracted from rice straw for high yield of hydrocarbon over alkali modified ZSM-5. Fuel 285, 119038. doi:10.1016/j.fuel.2020.119038
Nishu, L., Li, C., Yellezuome, D., Li, Y., and Liu, R. (2023). Catalytic pyrolysis of rice straw for high yield of aromatics over modified ZSM-5 catalysts and its kinetics. Renew. Energ. 209, 569–580. doi:10.1016/j.renene.2023.04.025
Nyambura, S. M., Nyambura, S. M., Jufei, W., Hua, L., Xuebin, F., Xingjia, P., et al. (2022). Microwave co-pyrolysis of kitchen food waste and rice straw for waste reduction and sustainable biohydrogen production: thermo-kinetic analysis and evolved gas analysis. Sustain. Energ. Technol. 52, 102072. doi:10.1016/j.seta.2022.102072
Ofrasio, B. I. G., de Luna, M. D. G., Chen, Y. C., Abarca, R. R. M., Dong, C. D., and Chang, K. L. (2020). Catalytic conversion of sugars and biomass to furanic biofuel precursors by boron-doped biochar in ionic liquid. Bioresour. Technol. Rep. 11, 100515. doi:10.1016/j.biteb.2020.100515
Park, J., Seyama, T., Shiroma, R., Ike, M., Srichuwong, S., Nagata, K., et al. (2009). Efficient recovery of glucose and FructoseviaEnzymatic saccharification of rice straw with soft carbohydrates. Biosci. Biotechnol. Biochem. 73, 1072–1077. doi:10.1271/bbb.80840
Patil, R., Cimon, C., Eskicioglu, C., and Goud, V. (2021). Effect of ozonolysis and thermal pre-treatment on rice straw hydrolysis for the enhancement of biomethane production. Renew. Energ. 179, 467–474. doi:10.1016/j.renene.2021.07.048
Peng, B., Ma, C. L., Zhang, P. Q., Wu, C. Q., Wang, Z. W., Li, A. T., et al. (2019). An effective hybrid strategy for converting rice straw to furoic acid by tandem catalysis via Sn-sepiolite combined with recombinant E. coli whole cells harboring horse liver alcohol dehydrogenase. Green Chem. 21 (21), 5914–5923. doi:10.1039/c9gc02499a
Pham, T. H., Chu, T. T. H., Nguyen, D. K., Le, T. K. O., Obaid, S. A., Alharbi, S. A., et al. (2022). Alginate-modified biochar derived from rice husk waste for improvement uptake performance of lead in wastewater. Chemosphere 307, 135956. doi:10.1016/j.chemosphere.2022.135956
Putten, R. J., Wall, J. C., Jong, E., Rasrendra, C. B., Heeres, H., and Vries, J. G. (2013). Hydroxymethylfurfural, a versatile platform chemical made from renewable resources. Chem. Rev. 113 (3), 1499–1597. doi:10.1021/cr300182k
Qi, X., Yan, L., Shen, F., and Qiu, M. (2019). Mechanochemical-assisted hydrolysis of pretreated rice straw into glucose and xylose in water by weakly acidic solid catalyst. Bioresour. Technol. 273, 687–691. doi:10.1016/j.biortech.2018.11.011
Sahu, O. (2021). Characterisation and utilization of heterogeneous catalyst from waste rice-straw for biodiesel conversion. Fuel 287, 119543. doi:10.1016/j.fuel.2020.119543
Santos, F., Machado, G., Faria, D., Lima, J., Marcal, N., Dutra, E., et al. (2016). Productive potential and quality of rice husk and straw for biorefineries. Biomass Convers. bior. 7 (1), 117–126. doi:10.1007/s13399-016-0214-x
Saravanan, P., Josephraj, J., Thillainayagam, B. P., and Ravindiran, G. (2021). Evaluation of the adsorptive removal of cationic dyes by greening biochar derived from agricultural bio-waste of rice husk. Biomass Convers. bior. 13, 4047–4060. doi:10.1007/s13399-021-01415-y
Shaheen, S. M., Antoniadis, V., Shahid, M., Yang, Y., Abdelrahman, H., Zhang, T., et al. (2022). Sustainable applications of rice feedstock in agro-environmental and construction sectors: a global perspective. Renew. Sust. Energ. Rev. 153, 111791. doi:10.1016/j.rser.2021.111791
Sharma, A., Singh, G., and Arya, S. K. (2020). Biofuel from rice straw. J. Clean. Prod. 277, 124101. doi:10.1016/j.jclepro.2020.124101
Shukla, S. S., Chava, R., Appari, S., Bahurudeen, A., and Kuncharam, B. V. R. (2022). Sustainable use of rice husk for the cleaner production of value-added products. J. Environ. Chem. Eng. 10 (1), 106899. doi:10.1016/j.jece.2021.106899
Singh, C., Tiwari, S., Gupta, V. K., and Singh, J. S. (2018). The effect of rice husk biochar on soil nutrient status, microbial biomass and paddy productivity of nutrient poor agriculture soils. Catena 171, 485–493. doi:10.1016/j.catena.2018.07.042
Sun, Z., Bottari, G., Afanasenko, A., Stuart, M. C. A., Deuss, P. J., Fridrich, B., et al. (2018). Complete lignocellulose conversion with integrated catalyst recycling yielding valuable aromatics and fuels. Nat. Catal. 1 (1), 82–92. doi:10.1038/s41929-017-0007-z
Sutanto, S., Go, A. W., Ismadji, S., and Ju, Y. H. (2017). Hydrolyzed rice bran as source of lipids and solid acid catalyst during in situ (trans)esterification. Biofuel 11 (2), 221–227. doi:10.1080/17597269.2017.1348190
Tosuner, Z. V., Taylan, G. G., and Özmıhçı, S. (2019). Effects of rice husk particle size on biohydrogen production under solid state fermentation. Int. J. Hydrogen Energ. 44 (34), 18785–18791. doi:10.1016/j.ijhydene.2018.10.230
Uddin, I., Wang, G., Gao, D., Hussain, Z., Naz, M. Y., Hou, B., et al. (2021). Conventional and cement-catalyzed co-pyrolysis of rice straw and waste polyethylene into liquid and gaseous fuels by using a fixed bed reactor. Biomass Convers. bior. 13, 5307–5316. doi:10.1007/s13399-021-01470-5
Ukarde, T. M., and Pawar, H. S. (2022). PolyE-IL, an efficient and recyclable bronsted acid catalyst for conversion of rice straw into levulinic and other organic acids. Energ. Fuel. 36 (3), 1592–1603. doi:10.1021/acs.energyfuels.1c03773
Valles, A., Alvarez-Hornos, F. J., Martínez-Soria, V., Marzal, P., and Gabaldón, C. (2020). Comparison of simultaneous saccharification and fermentation and separate hydrolysis and fermentation processes for butanol production from rice straw. Fuel 282, 118831. doi:10.1016/j.fuel.2020.118831
Wadood, A., Rana, A., Basheer, C., Razzaq, S. A., and Farooq, W. (2019). In situ transesterification of microalgae parachlorella kessleri biomass using sulfonated rice husk solid catalyst at room temperature. Bioenerg. Res. 13 (2), 530–541. doi:10.1007/s12155-019-10060-3
Wan, Y., and Lee, J. M. (2021). Toward value-added dicarboxylic acids from biomass derivatives via thermocatalytic conversion. ACS Catal. 11, 2524–2560. doi:10.1021/acscatal.0c05419
Wang, F., Li, Q., Xiao, Z., Jiang, B., Ren, J. W., Jin, Z., et al. (2022). Conversion of rice husk biomass into electrocatalyst for oxygen reduction reaction in Zn-air battery: effect of self-doped Si on performance. J. Colloid Interf. Sci. 606 (2), 1014–1023. doi:10.1016/j.jcis.2021.08.117
Wang, Z. C., Buechel, R., Jiang, Y., Wang, L., Xu, H., Castignolles, P., et al. (2021). Engineering the distinct structure interface of subnano-alumina domains on silica for acidic amorphous silica-alumina toward biorefining. JACS Au 1, 262–271. doi:10.1021/jacsau.0c00083
Wang, Z. C., O’Dell, L., Zeng, X., Liu, C., Zhao, S. F., Zhang, W. W., et al. (2019). Insight into three-coordinate aluminum species on ethanol-to-olefin conversion over ZSM-5 zeolites. Angew. Chem. Int. Ed. 58, 18061–18068. doi:10.1002/anie.201910987
Wanninayake, P., Rathnayake, M., Thushara, D., and Gunawardena, S. (2021). Conversion of rice straw into 5-hydroxymethylfurfural: review and comparative process evaluation. Biomass Convers. bior. 12 (3), 1013–1047. doi:10.1007/s13399-021-01351-x
Wataniyakul, P., Boonnoun, P., Quitain, A. T., kida, T., Laosiripojana, N., and Shotipruk, A. (2018). Preparation of hydrothermal carbon acid catalyst from defatted rice bran. Ind. Crop. Prod. 117, 286–294. doi:10.1016/j.indcrop.2018.03.002
Xiong, X., Yu, I. K. M., Dutta, S., Masek, O., and Tsang, D. C. W. (2021). Valorization of humins from food waste biorefinery for synthesis of biochar-supported lewis acid catalysts. Sci. Total. Environ. 775, 145851. doi:10.1016/j.scitotenv.2021.145851
Xu, X., Li, Z. Y., Tu, R., Sun, Y., and Jiang, E. (2018). Hydrogen from rice husk pyrolysis volatiles via non-noble Ni-Fe catalysts supported on five differently treated rice husk pyrolysis carbon supports. ACS Sustain. Chem. Eng. 6 (7), 8325–8339. doi:10.1021/acssuschemeng.8b00343
Yi, Y., Tu, G., Ying, G., Fang, Z., and Tsang, E. P. (2021). Magnetic biochar derived from rice straw and stainless steel pickling waste liquor for highly efficient adsorption of crystal violet. Bioresour. Technol. 341, 125743. doi:10.1016/j.biortech.2021.125743
Yu, I. K. M., Tsang, D. C. W., Yip, A. C. K., Chen, S. S., Ok, Y. S., and Poon, C. S. (2017). Valorization of starchy, cellulosic, and sugary food waste into hydroxymethylfurfural by one-pot catalysis. Chemosphere 184, 1099–1107. doi:10.1016/j.chemosphere.2017.06.095
Yu, I. K. M., Tsang, D. C. W., Yip, A. C. K., Su, Z., Vigier, K. D. O., Jerome, F., et al. (2019). Organic acid-regulated Lewis acidity for selective catalytic hydroxymethylfurfural production from rice waste: an experimental-computational study. ACS Sustain. Chem. Eng. 7 (1), 1437–1446. doi:10.1021/acssuschemeng.8b05141
Yuan, Y., Huang, L., Yilmaz, M., Zhang, T. C., Wang, Y., and Yuan, S. (2023). MgFe2O4-loaded N-doped biochar derived from waste cooked rice for efficient low-temperature desulfurization of H2S. Fuel 339, 127385. doi:10.1016/j.fuel.2022.127385
Zealand, A. M., Roskilly, A. P., and Graham, D. W. (2017). Effect of feeding frequency and organic loading rate on biomethane production in the anaerobic digestion of rice straw. Appl. Energ. 207, 156–165. doi:10.1016/j.apenergy.2017.05.170
Zeng, X., Fang, M. X., Tong, L. V., Tian, J. G., Xia, Z. X., Cen, J. M., et al. (2022). Hydrogen-rich gas production by catalytic steam gasification of rice husk using CeO2-modified Ni-CaO sorption bifunctional catalysts. Chem. Eng. J. 441, 136023. doi:10.1016/j.cej.2022.136023
Zhang, R., Li, Z., Liu, X., Wang, B., Zhou, G., Huang, X., et al. (2017). Immobilization and bioavailability of heavy metals in greenhouse soils amended with rice straw-derived biochar. Ecol. Eng. 98, 183–188. doi:10.1016/j.ecoleng.2016.10.057
Zhang, W., Tan, X., Gu, Y., Liu, S., Liu, Y., Hu, X., et al. (2020). Rice waste biochars produced at different pyrolysis temperatures for arsenic and cadmium abatement and detoxification in sediment. Chemosphere 250, 126268. doi:10.1016/j.chemosphere.2020.126268
Zhang, Z., Cheng, H., Chen, H., Chen, K., Lu, X., Ouyang, P., et al. (2018b). Enhancement in the aromatic yield from the catalytic fast pyrolysis of rice straw over hexadecyl trimethyl ammonium bromide modified hierarchical HZSM-5. Bioresour. Technol. 256, 241–246. doi:10.1016/j.biortech.2018.02.036
Zhang, Z., Cheng, H., Chen, H., Li, J., Chen, K., Lu, X., et al. (2018a). Catalytic fast pyrolysis of rice straw to aromatics over hierarchical HZSM-5 treated with different organosilanes. Energ. Fuel. 33 (1), 307–312. doi:10.1021/acs.energyfuels.8b03213
Zhang, Z., and Huber, G. W. (2018). Catalytic oxidation of carbohydrates into organic acids and furan chemicals. Chem. Soc. Rev. 47 (4), 1351–1390. doi:10.1039/C7CS00213K
Zheng, Y., Wang, F., Yang, X., Huang, Y., Liu, C., Zheng, Z., et al. (2017). Study on aromatics production via the catalytic pyrolysis vapor upgrading of biomass using metal-loaded modified H-ZSM-5. J. Anal. Appl. Pyrol. 126, 169–179. doi:10.1016/j.jaap.2017.06.011
Zou, R., Qian, M., Wang, C., Mateo, W., Wang, Y., Dai, L., et al. (2022). Biochar: from by-products of agro-industrial lignocellulosic waste to tailored carbon-based catalysts for biomass thermochemical conversions. Chem. Eng. J. 441, 135972. doi:10.1016/j.cej.2022.135972
Keywords: rice waste conversion, biochar, biofuel, valuable chemical, carbon/silicon-based catalyst, catalyst support
Citation: Zhang W, Xu X, Yuan Y and Wang Z (2023) Sustainable application of rice-waste for fuels and valuable chemicals-a mini review. Front. Chem. 11:1225073. doi: 10.3389/fchem.2023.1225073
Received: 18 May 2023; Accepted: 09 October 2023;
Published: 19 October 2023.
Edited by:
Kong Xiangjin, Liaocheng University, ChinaReviewed by:
Shanhui Zhu, Chinese Academy of Sciences (CAS), ChinaCopyright © 2023 Zhang, Xu, Yuan and Wang. This is an open-access article distributed under the terms of the Creative Commons Attribution License (CC BY). The use, distribution or reproduction in other forums is permitted, provided the original author(s) and the copyright owner(s) are credited and that the original publication in this journal is cited, in accordance with accepted academic practice. No use, distribution or reproduction is permitted which does not comply with these terms.
*Correspondence: Zichun Wang, emljaHVuLndhbmdAYnVjdC5lZHUuY24=
†These authors have contributed equally to this work
Disclaimer: All claims expressed in this article are solely those of the authors and do not necessarily represent those of their affiliated organizations, or those of the publisher, the editors and the reviewers. Any product that may be evaluated in this article or claim that may be made by its manufacturer is not guaranteed or endorsed by the publisher.
Research integrity at Frontiers
Learn more about the work of our research integrity team to safeguard the quality of each article we publish.