- 1Solar Cell Applications Research Lab, Department of Physics, Government College University Lahore, Lahore, Punjab, Pakistan
- 2Department of Physics, Lahore Garrison University, Lahore, Punjab, Pakistan
- 3Punjab University College of Pharmacy, University of the Punjab, Lahore, Pakistan
- 4Core Research Facilities, Research Institute, King Fahd University of Petroleum and Minerals, Dhahran, Saudi Arabia
- 5Department of Clinical Sciences, Faculty of Veterinary and Animal Sciences, Muhammad Nawaz Shareef University of Agriculture, Multan, Punjab, Pakistan
- 6Departament d’Enginyeria Química, Universitat Rovira i Virgili, Tarragona, Spain
In this study, MoO3 nanostructures were prepared, doped with various concentrations of graphene oxide (2 and 4% GO) and a fixed amount of polyvinylpyrrolidone (PVP) using the co-precipitation method. The motive of this study was to examine the catalytic and antimicrobial efficacy with evidential molecular docking analyses of GO/PVP-doped MoO3. GO and PVP were utilized as doping agents to reduce the exciton recombination rate of MoO3 by providing more active sites that increase the antibacterial activity of MoO3. The prepared binary dopant (GO and PVP)-dependent MoO3 was used as an effective antibacterial agent against Escherichia coli (E. coli). Notably, 4% GO/PVP-doped MoO3 showed good bactericidal potential against E. coli at higher concentrations in comparison to ciprofloxacin. Furthermore, in silico docking revealed the possible inhibitory impact of the synthesized nanocomposites on folate and fatty acid synthesis enzymes, dihydrofolate reductase and enoyl-[acyl carrier protein] reductase, respectively.
1 Introduction
A shortage of water resources has occurred internationally as a result of the quick rate of industrialization and the massive growth of the population (Santhosh et al., 2016). Numerous types of waste products are discharged in water, such as pesticides, plastics, textile dyes, and organic and inorganic contaminants, endangering freshwater resources and the ecological environment (Nasrollahzadeh et al., 2021). Heavy metal ions and dyes are two significant categories of aquatic pollutants, and if these substances are present in water, it is no longer good for health. Thoroughly cleaning up the contaminated water can also be challenging (Rafiq et al., 2021). According to the World Bank, dyeing textiles contribute significantly to about 17%–20% of water contamination. Dyes have been used industrially in leather, plastics, printing, cosmetics, and pharmaceuticals (Rafiq et al., 2021). Approximately 7 million metric tons of dyes are produced annually, with over 10,000 commercially accessible dyes (Kapoor et al., 2021). It is challenging to degrade dyes because of their diverse chemical structures, especially when mixed with azo, heterocyclic, and cationic forms (Zhou et al., 2021) Researchers are interested in nanomaterials because of their distinctive physiochemical characteristics and advanced dye-contaminated wastewater treatment techniques (Bari et al., 2022). Small nanostructures (NSs) have splendid surface-to-volume ratios, significantly enhancing chemical (biological and catalytic activity) and physical properties. Among all techniques, the catalytic process has been employed to address the problems of organic impurity (Bari et al., 2022), (Khan et al., 2022). Nano-sized metal oxides (MOs), such as ZnO, MoO3, TiO2, La2O3, and CeO2, have many applications since they are employed in catalysis and to monitor the antibacterial activity used to degrade industrial wastewater contamination (Ikram et al., 2022). MoO3 has attracted attention from different fields, including solar cells, optoelectronic devices, catalysis, electrochromic system dye degradation, oxidative catalysts, antibacterial activities, gas sensors, and photocatalysis. Recent research studies investigated the role of MoO3-based materials for antibacterial activities against several types of harmful bacteria. The cell membrane and cell wall are the major defensive boundaries for resisting pathogens in antibacterial actions (Ashraf et al., 2020). MoO3 disrupts the bacterial cell, which causes immediate cell death. Its main benefit is that it does not generate bacteria that are resistant to antibiotics. Due to its non-toxicity, it is secure and harmless for human health (Zhao et al., 2020). However, MoO3 causes a high recombination rate of electrons and holes. Also, the decrease in the catalytic activity of MoO3 was attributed to the self-aggregation of nanoadsorbents, which may reduce the adsorption property and restrict their wide-scale practical application (Li et al., 2022). The addition of dopants (2D materials, rare earth metal or metal oxides, etc.) should be an effective approach for enhancing the properties of MoO3. Graphene oxide (GO) gained a lot of attention due to its large surface area and excellent electrochemical and mechanical capabilities. The structure of graphene can effectively prevent nanoparticle aggregation in the liquid-based catalytic process (Yao et al., 2019). The 2D graphitic property of graphene, in contrast to 0D fullerene, 1D carbon nanotubes, and 3D graphite basic components, has been the subject of much research for its photocatalytic properties (Li et al., 2016). These materials have enormous surface areas that give them useful characteristics, including high thermal and mechanical stability and good chemical resistance. To digest hazardous MB in industrially contaminated water, GO and reduced graphene oxide (rGO) are interesting options that may boost the effectiveness of photocatalysis (Xiang et al., 2012). The efficiency of GO/rGO photocatalysts in converting light into chemical energy may be improved by blocking the recombination of electron–hole pairs (Qumar et al., 2021). Polymers may form complexes or ion pairs with metal ions, making them a potential alternative to stabilizers that can be manipulated into achieving the desired results in the physicochemical characterization of NSs (Adzitey et al., 2022). Polymeric materials’ potential uses in biology and ecology have garnered a lot of attention from scientists (NCCLS, 2007). Metal oxide doping is utilized to achieve significant results for a variety of applications, and several polymers (including polyvinyl alcohol, polyvinyl chloride, polyvinylpyrrolidone, and chitosan) are employed (Weidmann et al., 1995; Sampson and de Korte, 2011; Lewis, 2012). PVP, an artificial polymer, is one among them and is widely regarded as an efficient capping material for metal oxide NSs. Carbonyl units and functional groups that reinforce metal oxide NSs are responsible for the composite’s unique characteristics (V Jadhav et al., 2013), (Lu et al., 2012). Because of its high-quality physicochemical qualities, it is employed as a stabilizer for NSs and as an additive in other materials 48–51. PVP has been proven to be biocompatible, minimally toxic, water soluble, and having antibacterial actions that show promise in recent investigations (Shahmiri et al., 2013; Karpuraranjith and Thambidurai, 2017; Hu et al., 2018; Naz et al., 2020; Al Mogbel et al., 2021). Numerous methods (hydrothermal, sol–gel, thermal deposition, co-precipitation, physiochemical techniques, etc.) can be used to prepare MoO3 NSs. Co-precipitation is the most practical technique due to its low cost and eco-friendly nature (Ikram et al., 2022). Among the several suggested approaches, co-precipitation has the characteristics of being easily repeatable, low in impurity, and easily processable (Naz et al., 2020). As direct precipitation is unable to separate the desired metallic species because of its low concentration in the sample solution, co-precipitation can be adopted. It is less time consuming, uses basic laboratory equipment that makes it easily processable, and is a low-cost technique (Bader et al., 2014). The goal of this study is to synthesize GO/PVP-doped MoO3 NSs via the co-precipitation method for the breakdown of organic dyes from contaminated water and to examine their bactericidal effect against E. coli. As PL intensity decreases, the electron charge efficiency increases, which leads to the generation of reactive oxygen species that may produce more active sites resulting in good antibacterial activity The majority of bacterium cell walls (including those of E. coli and S. aureus) are known to be negatively charged. In fact, due to an electrostatic interaction with a negatively charged bacterium surface, some chemicals (quaternary ammonium compounds, molecules, and polymers) can trigger membrane rupture and, ultimately, lead to subsequent death. Active substances are more likely to bind and be transferred through the skin if they are able to construct a hydrogen-bonded network with water molecules, which is facilitated by the existence of hydroxyl groups (Kupnik et al., 2020).
2 Experimental section
2.1 Materials
Ammonium molybdate (NH4)6 Mo7O24.4H2O and PVP (C6H9)n were purchased from Sigma-Aldrich, Germany.
2.2 Synthesis of GO
Refined graphite was used to synthesize GO via the modified Hummers technique. Graphite (5 g) and NaNO3 (2.5 g) were integrated into H2SO4 (108 mL) with H3PO4 (12 mL), and the solution was vigorously stirred for 10 min in a reaction flask (immersed in an ice bath). The filtrate solution was poured into a muffle furnace for 2 h (60°C) to remove moisture. Later, KMnO4 (15 g) was incorporated slowly, while the temperature was kept below 5°C and vigorously stirred; the solution color turned from purple to yellow. After the addition of H2O2 (12 mL), the suspension was then centrifuged (7,000 rpm) and repeatedly washed with deionized water (DI water) to achieve a residue. Afterward, the resulting precipitates were dried for 12 h and ground to get a fine powder (Figure 1A).
2.3 Synthesis of GO/PVP-doped MoO3
To synthesize MoO3 by the co-precipitation technique, 0.1 M of ammonium molybdate was prepared under continuous stirring at 90°C for 40 min. The desired amount of HCl was added dropwise in the aforementioned solution to keep the pH ∼2. Afterward, the colloidal solution was centrifuged two times (7,000 rpm front 7 min) with DI water, dried for 12 h at 150°C, and ground to attain a fine powder. Similarly, different concentrations of GO (2 and 4 wt%) and fixed amounts of PVP-doped MoO3 NSs were prepared (Figure 1B).
2.4 Catalysis (CA)
The catalytic efficacy of the host and doped MoO3 for the reduction of rhodamine B (RhB) was analyzed in the presence of sodium borohydride (NaBH4). First, 0.1 M NaBH4 was added into the RhB solution followed by the integration of 200 μL of MoO3 and GO/PVP-doped MoO3. The change of the color of the dye from pink to light pink indicated the reduction of RhB into leuco-rhodamine B (LRhB). The obtained supernatant was examined using a UV–Vis spectrometer to measure the degree of dye degradation in a periodic time interval. The percentage of dye degradation for each sample was computed via the followingequation:
where Co is the initial concentration of the dye at t0 and Ct is the final concentration of the dye at t after the incorporation of composite materials.
2.5 Isolation and identification of MDR E. coli
2.5.1 Sample collection
The selected nursing cows were milked directly into sterilized glassware, available at several markets, veterinary clinics, and farms in Punjab, Pakistan. After being collected at 4°C, raw milk was immediately brought to the lab. We examined the number of coliforms in raw milk on MacConkey agar. 48 h were spent incubating all the plates at 37°C.
2.5.2 Identification and characterization of bacterial isolates
Using Bergey’s Manual of Determinative Bacteriology as a reference, the first identification of E. coli was based on the colonial morphology detected after Gram staining and many biochemical tests (Sinclair, 1939).
2.5.3 Antibiotic susceptibility
The Bauer disc diffusion method was used to perform the antibiotic susceptibility test on Mueller Hinton agar (MHA) (Bauer et al., 1966). The test was performed to check whether E. coli was resistant to the following antibiotics (classes): azithromycin (Azam) 15 µg (macrolides), gentamicin (Gm) 10 µg (aminoglycosides), ciprofloxacin (Cip) 5 µg (quinolones), amoxicillin (A) 30 μg (penicillin), tetracycline (Te) 30 µg (tetracyclines), imipenem (Imi) 10 µg (carbapenem), and ceftriaxone (Cro) 30 µg (cephalosporins) (Adzitey et al., 2022). E. coli purified cultures were grown and adjusted to 0.5 McFarland turbidity. The spread plate was placed on MHA (Oxoid Limited, Basingstoke, United Kingdom). The antibiotic discs were set away from the inoculation plate to prevent the overlap of inhibition zones. The Clinical and Laboratory Standards Institute evaluated the results after the plates were incubated at 37°C for 24 h (NCCLS, 2007). MDR was given to bacteria that were discovered to be resistant to at least three antibiotics (Iwalokun et al., 2004).
2.6 Antimicrobial activity
Using the agar well diffusion method, the in vitro antibacterial activity of MoO3 was assessed against 10 typical isolates of MDR E. coli obtained from mastitic milk. Petri dishes were swabbed with 1.5 × 108 CFU/mL (0.5 McFarland standard) MDR E. coli on MacConkey agar. Using a sterile cork borer, 6 mm-diameter wells were created. 2 and 4% GO/PVP-MoO3 were applied as 500 μg/0.05 mL and 1,000 μg/0.05 mL, respectively. Ciprofloxacin (5 μg/0.05 mL) was used as the positive control and DI water as the negative control (0.05 mL) (Haider et al., 2020a).
2.6.1 Statistical analyses
The inhibition zone (mm) size was used to detect the antimicrobial efficiency and was analyzed statistically by one-way analysis of variance (ANOVA) using SPSS 20 (Haider et al., 2020b)
2.7 Molecular docking analysis
Enzyme targets involved in fatty acid and folate biosynthesis pathways, specifically enoyl-[acyl carrier protein] reductase (FabI) and dihydrofolate reductase (DHFR) from E. coli, were subjected to molecular docking. DHFR (PDB ID: 2ANQ; resolution: 2.13 Å) (Summerfield et al., 2006) and FabI (PDB ID: 1MFP; resolution: 2.33 Å) (Seefeld et al., 2003) have been acquired from the Protein Data Bank. SYBYL-X 2.0 (Mehmood et al., 2022) was used to predict the results of the molecular docking analysis. The SYBYL-X 2.0/SKETCH module was used to create 3D structures of selected compounds, followed by energy minimization using the Tripos force field with the Gasteiger–Hückel atomic charge (Clark et al., 1989). The Surflex–Dock module of molecular modeling software program SYBYL-X 2.0 (Shahzadi et al., 2023) was used to analyze flexible molecular docking simulations to study binding interactions of nanoparticles with active site residues of the selected proteins. The missing hydrogens were introduced. According to the AMBER 7 ff99 force field, the atomic types were allocated and atomic charges were applied. Finally, using the Powell algorithm with a convergence gradient of 0.5 kcal/(molA) for 1,000 cycles, the energy was reduced to avoid steric clashes. For each ligand-receptor complex system, at least 20 of the finest docked poses were saved conclusively. The Hammerhead scoring system was used to rate the best putative ligand poses. The Surflex–Dock module generates and ranks putative poses of ligand fragments using an empirically generated consensus scoring (cScore) (Blount et al., 2002) function that combines Hammerhead’s empirical scoring function (Jain, 1996), comprised of the D score (dock score), G score (gold score), ChemScore, potential mean force (PMF) score, and/or complete score, with a molecular similarity method (morphological similarity).
3 Results and discussion
The modified Hummers method was used to prepare GO, and (2 and 4 wt%) GO/PVP-doped MoO3 was obtained using the co-precipitation technique.
The XRD analysis was used to investigate the phase purity, crystalline structure, and inter-planar properties of pure and GO/PVP-doped MoO3, as shown in Figure 2A. Diffraction peaks were observed at 25.84°, 29.46°, 35.52°, 45.57°, 56.19°, and 67.25°, along with matching crystal planes, (210), (300), (310), (410), (218), and (610), respectively. Furthermore, the hexagonal crystal structure of MoO3 was confirmed by JCPDS file no. 00-021-0569. Minor shifting toward a lower angle can be seen after doping. The crystallinity of prepared NSs was reduced by the incorporation of GO, manifested for anchoring of MoO3 on nanosheets (Munawar et al., 2022). Additionally, the SAED analysis of MoO3 and GO/PVP-doped MoO3 exhibited circular rings corresponding to various planes, (310), (300), (210), (218), and (410), of XRD (Figures 2B, C).
UV–Vis spectroscopy was employed to examine the optical characteristic of pure and (2 and 4 wt%) GO- and PVP-doped MoO3 (Figure 3A). MoO3 exhibited the absorption range between 300 and 340 nm (Gowtham et al., 2018). Upon doping of GO and PVP, a red shift was observed that led to the decrease in the band gap energy, which might be the quantum confinement effects. The band gap of pure MoO3 NSs was calculated to be 2.7 eV, which decreases upon doping. PL spectroscopy was employed to investigate the optical characteristics, quantum confinement processes, and energy levels of the material. A photoluminescence signal is produced when electrons in the valence band (VB) are excited with the conduction band (CB) at an excitation wavelength and then returned to the VB. MoO3 NSs yield broad emission peaks in the visible range from 415 to 430 nm at an exciton wavelength of 300 nm (Klinbumrung et al., 2012). Upon doping of GO and PVP, the intensity of MoO3 was reduced, attributed to a decrease in the exciton recombination rate (Figure 3B).
The FTIR technique was used to investigate the existence of the functional group in a host and doped MoO3, with a wavenumber range of 4,000–500 cm-1 (Figure 3C). The transmittance band at 610 cm−1 was assigned as O–Mo–O stretching and bending vibrations (Song et al., 2007), and the band at 886 cm−1 in the range of 875–885 cm−1 was attributed to Mo–O–Mo vibrations of Mo (Chiang and Yeh, 2013). The bands at 1,633 and 3,351 cm−1 were manifested for the bending and stretching of the absorbed hydroxyl function group (Bari et al., 2022).
EDS analysis was performed to evaluate the elemental composition of the pure and (2 and 4 wt%) GO/PVP-doped MoO3. Intense peaks of Mo and O confirmed the purity of the synthesized material, as shown in Supplementary Figure S1. Gold (Au) peaks can be seen in the spectra because the sample has a Au coating applied to it to reduce the influence of charge. Small Cu peaks may have been caused by the brass sample holder used for the EDS observation. TEM was used to determine the morphological properties of the prepared samples, as shown in Figures 4A–E. MoO3 showed randomly oriented nanorod-like (NR) structures with nanoparticles, as demonstrated in Figure 4A. The addition of PVP into MoO3 showed an aggregation of nanoparticles (Figure 4B). Figure 4C confirmed the nanosheets of pristine GO, and the incorporation of GO in the binary system (PVP–MoO3) showed that GO nanosheets overlapped the nanoparticles and agglomeration increased with the increase in the amount of GO (Figure 4D). Using Gatan software, interlayer d-spacing was determined from HRTEM images, as shown in Supplementary Figure S2. The d-spacing for GO/PVP-doped MoO3 was recorded as 0.20, 0.16, 0.25, and 0.13 nm, which was well matched with the XRD results.
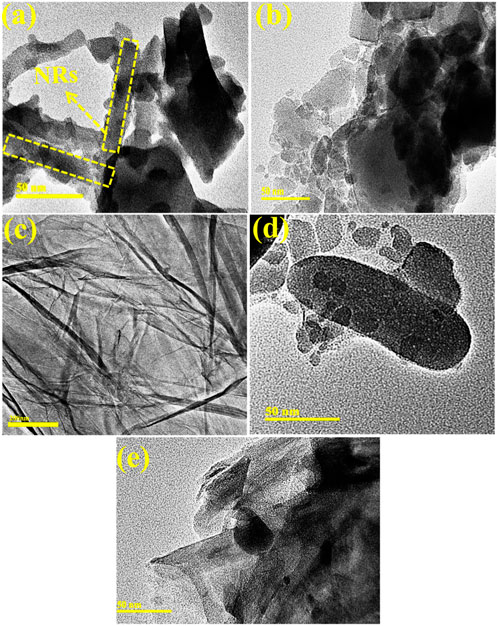
FIGURE 4. (A–E) TEM images of (A) MoO3, (B) PVP–MoO3, (C) GO, (D) 2% GO/PVP–MoO3, and (E) 4% GO/PVP–MoO3.
The degradation efficacy of dopant-free and (2 and 4 wt%) GO/PVP-doped MoO3 was investigated for a time interval of 10 min for acidic values of 92.38, 57, 54, and 56%, shown in Figure 5A, for basic values of 84.61, 45.85 52, and 60.42%, shown in Figure 5B, and for neutral values as 90.0, 56.85, 83.28, and 74.14%, shown in Figure 5C. In all media, MoO3 NSs resulted in the highest concentrations of catalytic activity, while the addition of dopants decreased the efficiency of catalysis. The decreased degradation efficiency of dopants may be due to the self-aggregation of the nanoadsorbents, which may reduce the adsorption property and restrict their wide-scale practical application (Li et al., 2022).
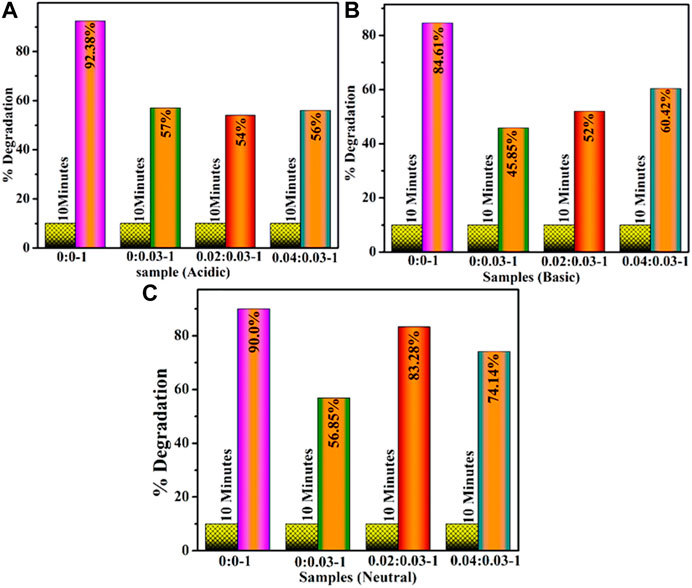
FIGURE 5. Catalytic activity of (2 and 4 wt%) of GO- and PVP-doped MoO3 in (A) acidic, (B) basic, and (C) neutral media.
The two main aspects that are thought to be crucial in the catalysis mechanism are adding a nanocatalyst and a reducing agent to the dye (Jana and Pal, 1999; Panigrahi et al., 2007; Khalavka et al., 2009; Maerzke and Siepmann, 2011). As nanoparticles have a large surface area, MoO3 NSs serve as an electron relay system for dye catalytic reduction (Hu et al., 2007). The catalytic process for reducing RhB from MoO3 NSs in the presence of BH−4 ions is shown in Supplementary Figure S3. Initially, BH−4 and RhB will absorb over a large surface area of MoO3 NSs. In general, BH−4 ions act nucleophilic and give electrons to MoO3, while RhB acts electrophilic and can take electrons away from MoO3 to generate a reduced form of leuco RhB. Leuco RhB and BH4 have been desorbed from the MoO3 NS surface. Because of their large surface area, sodium borohydride and MoO3 NSs must be loaded more frequently. The reaction rate of RhB degradation considerably increases when the concentration of MoO3 NSs rises (Cazaux and Tielens, 2004). A comparison of the antibacterial activity of the synthesized nanostructures in previous studies with the results of the current investigation (Table 2).
The stability of GO/PVP-MoO3 was tested by storing the degraded solution samples in the dark for 3 days to see if the dye degradation was stable. Dye degradation efficiency was monitored via UV–Vis spectrophotometry every 24 h, as shown in Figure 6. Using Eq. 1, the efficiency of percentage degradation was calculated.
The antibacterial behavior of GO/PVP-doped MoO3 is summarized in Table 1. Undoped MoO3 showed less antibacterial activity (Naseem and Durrani, 2021), while GO/PVP-doped MoO3 has an increased bactericidal action. E. coli showed an inhibitory zone at low and high doses ranging from (2.65–6.90) to (4.05–8.65). In addition, the ciprofloxacin inhibition zone against E. coli was measured as 9.65 mm (positive control), parallel to 0 mm of DI water (negative control). Oxidative stress produced by nanomaterials is proportional to their concentration, size, and shape. The antibacterial efficiency of the substance is inversely associated with its size. GO/PVP-doped MoO3 produces more reactive oxygen species, called ROS, due to its smaller size (Yu et al., 2020). These species, in turn, cause the extrusion of cytoplasmic components as seen from Supplementary Figure S4, which ultimately leads to the death of bacteria by the penetration of the micro-organism membrane. Establishing oxidative stress effectively supplements the fundamental antibacterial function, which is accomplished by intimate contact with GO and involves a non-oxidative electron exchange procedure triggered by interactions between GO and the metal substrate. The reactive oxygen species (e.g., H2O2) produced by GO have the potential to impair oxygen consumption, energy transduction, the energetic equilibrium of phospholipids, and the transport of physiologically active molecules, all of which might lead to serious structural degradation of cell membranes (Haider et al., 2020b).
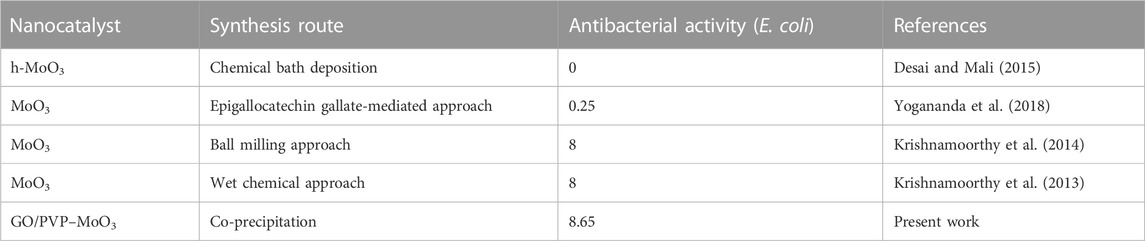
TABLE 2. Literature comparison of the antibacterial activity of synthesized NSs with the present study.
Several researchers (Seefeld et al., 2003; Summerfield et al., 2006; Mehmood et al., 2022) have investigated the microbicidal capability of metal ion-containing nanocomposites (Clark et al., 1989), (Shahzadi et al., 2023). The bioactivity of the nanocomposites depends on their tendency to interface bacteria via electrostatic, van der Waals, or hydrophobic forces. The enzymes associated with essential metabolic activities in bacterial metabolic processes have been identified, potentially promising antibiotic candidates. Thus, the fatty acid biosynthesis enzyme FabI and the folate biosynthesis enzyme DHFR from E. coli were adopted for viable species to examine the inhibitory response of PVP/MoO3 with GO/PVP/MoO3 against them.
Figures 7A, B illustrate the optimally docked conformation of PVP/MoO3 within the active domain of FabI E. coli, which disclosed an H-bond with Ile20, Ala21, Ser41, Lys163, and Thr194 and a total binding score of 5.05. The key binding contacts for GO/PVP/MoO3 were Ser19, Thr194, and Ala196, respectively, with a binding score of 7.20, as shown in (Figures 7A–C).
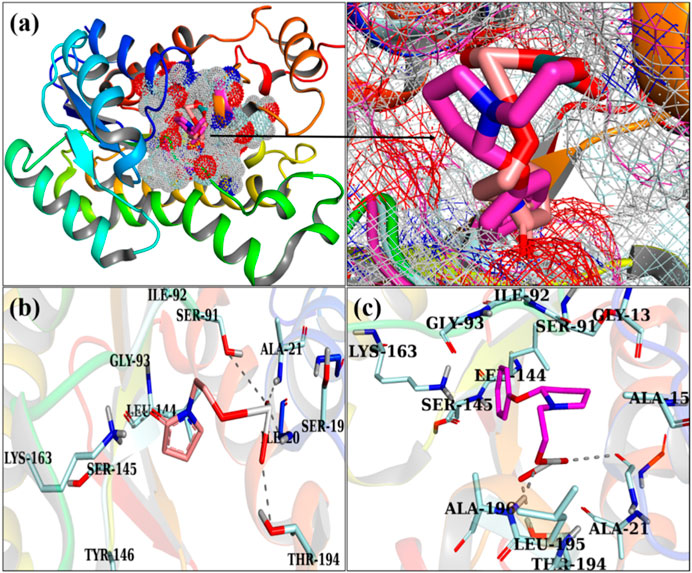
FIGURE 7. 3D view of a binding pocket (A) and the binding interaction pattern of PVP/MoO3 (B) and GO/PVP/MoO3 (C) inside the binding site of FabI.
Figures 8A, B exhibit the optimally docked conformation of PVP/MoO3 in the active pocket of DHFR E. coli, which exhibited an H-bond interaction with Asn18, Ser49, and Thr123 and a total binding score of 3.91. The primary binding interactions for GO/PVP/MoO3 were with Ser49, which had a binding score of 5.91 (Figures 8A–C).
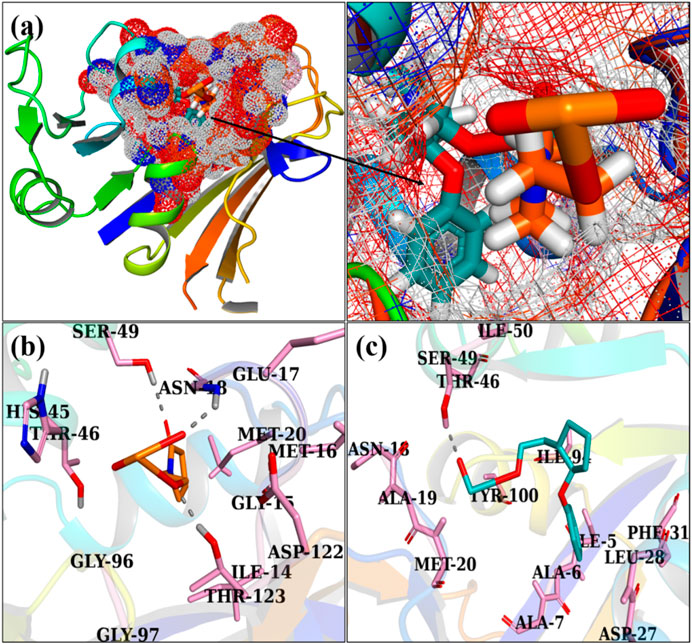
FIGURE 8. 3D view of a binding pocket (A) and the binding interaction pattern of PVP/MoO3 (B) and GO/PVP/MoO3 (C) inside the binding site of FabI.
4 Conclusion
In this study, GO/PVP-doped MoO3 NSs were effectively synthesized using a low-cost co-precipitation method. The XRD pattern confirmed the presence of the hexagonal structure of synthesized NSs. A rod-like morphology of MoO3 was recorded by TEM, while a higher concentration of GO revealed the encapsulation of nanorods to nanosheets. FTIR has confirmed the existence of O–Mo–O stretching vibrations of the synthesized NSs. Moreover, EDS spectra have confirmed the presence of Mo, PVP, and GO. UV–Vis spectroscopy revealed absorption peaks for MoO3 and GO/PVP-doped MoO3; however, the red shift was observed due to the quantum confinement effect. Compared to the doped samples, the undoped MoO3 nanostructure showed the highest catalytic potential. Additionally, the prepared nanostructures were highly efficient in inhibiting E. coli. In conclusion, MoO3 NSs with natural and synthetic polymers may be inexpensive and effective against microbes, but they are resistant to the degradation of industrial dyes. This study suggests that 4% GO/PVP-doped MoO3 NSs could be used as effective antibacterial agents against E. coli (Dakal et al., 2016; Altaf et al., 2020; Arularasu et al., 2020; Ikram et al., 2020; Shahzadi et al., 2022).
Data availability statement
The original contributions presented in the study are included in the article/Supplementary Material; further inquiries can be directed to the corresponding authors.
Author contributions
MI: conceptualization, investigation, writing—original draft preparation, supervision, funding acquisition, and visualization. IA: methodology, formal analysis, and writing—review and editing. AR: conceptualization and resources. IS: conceptualization, writing—review and editing, and investigation. AU-H: methodology and formal analysis. AH: data curation and resources. WN: formal analysis, conceptualization, and funding acquisition. FM: data analysis, interpretation, conceptualization, review and editing, investigation, formal analysis, and fund acquisition.
Acknowledgments
Authors are grateful to HEC, Pakistan through NRPU 20-17615, Proyectos de Generación de Conocimiento AEI/MCIN (PID2021-123665OB-I00), and the project reference number of TED2021–129343B-I00.
Conflict of interest
The authors declare that the research was conducted in the absence of any commercial or financial relationships that could be construed as a potential conflict of interest.
Publisher’s note
All claims expressed in this article are solely those of the authors and do not necessarily represent those of their affiliated organizations, or those of the publisher, the editors, and the reviewers. Any product that may be evaluated in this article, or claim that may be made by its manufacturer, is not guaranteed or endorsed by the publisher.
Supplementary material
The Supplementary Material for this article can be found online at: https://www.frontiersin.org/articles/10.3389/fchem.2023.1191849/full#supplementary-material
References
Adzitey, F., Yussif, S., Ayamga, R., Zuberu, S., Addy, F., Adu-Bonsu, G., et al. (2022). Antimicrobial susceptibility and molecular characterization of Escherichia coli recovered from milk and related samples. Microorganisms 10 (7), 1335. doi:10.3390/microorganisms10071335
Al Mogbel, M. S., Elabbasy, M. T., Mohamed, R. S., Ghoniem, A. E., El-Kader, M. F. H. A., and Menazea, A. A. (2021). Improvement in antibacterial activity of Poly Vinyl Pyrrolidone/Chitosan incorporated by graphene oxide NPs via laser ablation. J. Polym. Res. 28 (474). doi:10.1007/s10965-021-02838-x
Altaf, S., Haider, A., Naz, S., Ul-Hamid, A., Haider, J., Imran, M., et al. (2020). Comparative study of selenides and tellurides of transition metals (Nb and Ta) with respect to its catalytic, antimicrobial, and molecular docking performance. Nanoscale Res. Lett. 15 (1), 144. doi:10.1186/s11671-020-03375-0
Arularasu, M. V., Harb, M., and Sundaram, R. (2020). Synthesis and characterization of cellulose/TiO2 nanocomposite: Evaluation of in vitro antibacterial and in silico molecular docking studies. Carbohydr. Polym. 249, 116868. doi:10.1016/j.carbpol.2020.116868
Ashraf, M. A., Yang, Y., and Fakhri, A. (2020). Synthesis of NiS–MoO3 nanocomposites and decorated on graphene oxides for heterogeneous photocatalysis, antibacterial and antioxidant activities. Ceram. Int. 46 (6), 8379–8384. doi:10.1016/j.ceramint.2019.12.070
Bader, N. R., Edbey, K., and Telgheder, U. (2014). Cloud point extraction as a sample preparation technique for trace element analysis: An overview. J. Chem. Pharm. Res. 6 (2), 496–501. https://www.researchgate.net/profile/Nabil-Bader-3/publication/287302682_Co-precipitation_as_a_sample_preparation_technique_for_trace_element_analysis_An_overview/links/58d68f65458515337888623f/Co-precipitation-as-a-sample-preparation-technique-for-trace-. Accessed: Apr. 09, 2023. [Online]. Available
Bari, A., Ikram, M., Haider, A., Ul-Hamid, A., Haider, J., Shahzadi, I., et al. (2022). Evaluation of bactericidal potential and catalytic dye degradation of multiple morphology based chitosan/polyvinylpyrrolidone-doped bismuth oxide nanostructures. Nanoscale Adv. 4 (12), 2713–2728. doi:10.1039/d2na00105e
Bauer, A. W., Kirby, W. M., Sherris, J. C., and Turck, M. (1966). Antibiotic susceptibility testing by a standardized single disk method. Am. J. Clin. Pathol. 45 (4), 493–496. doi:10.1093/ajcp/45.4_ts.493
Blount, K. F., Grover, N. L., Mokler, V., Beigelman, L., and Uhlenbeck, O. C. (2002). Steric interference modification of the hammerhead ribozyme. Chem. Biol. 9 (9), 1009–1016. doi:10.1016/S1074-5521(02)00218-1
Cazaux, S., and Tielens, A. G. G. M. (2004). H 2 formation on grain surfaces. Astrophys. J. 604 (1), 222–237. doi:10.1086/381775
Chiang, T. H., and Yeh, H. C. (2013). The synthesis of α-MoO3 by ethylene glycol. Mater. (Basel) 6 (10), 4609–4625. doi:10.3390/ma6104609
Clark, M., Cramer, R. D., and Van Opdenbosch, N. (1989). Validation of the general purpose tripos 5.2 force field. J. Comput. Chem. 10 (8), 982–1012. doi:10.1002/jcc.540100804
Dakal, T. C., Kumar, A., Majumdar, R. S., and Yadav, V. (2016). Mechanistic basis of antimicrobial actions of silver nanoparticles. Front. Microbiol. 7, 1831. doi:10.3389/fmicb.2016.01831
Desai, N., and Mali, S. (2015). Chemically grown MoO3 nanorods for antibacterial activity study. J. Nanomed. Nanotechnol. 6 (6), 338. doi:10.4172/2157-7439.1000338
Gowtham, B., Ponnuswamy, V., Pradeesh, G., Chandrasekaran, J., and Aradhana, D. (2018). MoO3 overview: Hexagonal plate-like MoO3 nanoparticles prepared by precipitation method. J. Mat. Sci. Mat. Electron. 29 (8), 6835–6843. doi:10.1007/s10854-018-8670-7
Haider, A., Ijaz, M., Ali, S., Haider, J., Imran, M., Majeed, H., et al. (2020). Green synthesized phytochemically (zingiber officinale and allium sativum) reduced nickel oxide nanoparticles confirmed bactericidal and catalytic potential. Nanoscale Res. Lett. 15 (1), 50. doi:10.1186/s11671-020-3283-5
Haider, A., Ijaz, M., Imran, M., Naz, M., Majeed, H., Khan, J. A., et al. (2020). Enhanced bactericidal action and dye degradation of spicy roots’ extract-incorporated fine-tuned metal oxide nanoparticles. Appl. Nanosci. 10 (4), 1095–1104. doi:10.1007/s13204-019-01188-x
Hu, H., Shao, M., Zhang, W., Lu, L., Wang, H., and Wang, S. (2007). Synthesis of layer-deposited silicon nanowires, modification with Pd nanoparticles, and their excellent catalytic activity and stability in the reduction of methylene blue. J. Phys. Chem. C 111 (8), 3467–3470. doi:10.1021/jp066440f
Hu, M., Li, C., Li, X., Zhou, M., Sun, J., Sheng, F., et al. (2018). Zinc oxide/silver bimetallic nanoencapsulated in PVP/PCL nanofibres for improved antibacterial activity. Artif. Cells, Nanomedicine Biotechnol. 46 (6), 1248–1257. doi:10.1080/21691401.2017.1366339
Ikram, M., Abid, N., Haider, A., Ul-Hamid, A., Haider, J., Shahzadi, A., et al. (2022). Toward efficient dye degradation and the bactericidal behavior of Mo-doped La2O3nanostructures. Nanoscale Adv. 4 (3), 926–942. doi:10.1039/d1na00802a
Ikram, M., Hassan, J., Raza, A., Haider, A., Naz, S., Ul-Hamid, A., et al. (2020). Photocatalytic and bactericidal properties and molecular docking analysis of TiO2nanoparticles conjugated with Zr for environmental remediation. RSC Adv. 10 (50), 30007–30024. doi:10.1039/d0ra05862a
Iwalokun, B. A., Ogunledun, A., Ogbolu, D. O., Bamiro, S. B., and Jimi-Omojola, J. (2004). In vitro antimicrobial properties of aqueous garlic extract against multidrug-resistant bacteria and Candida species from Nigeria. J. Med. Food 7 (3), 327–333. doi:10.1089/jmf.2004.7.327
Jain, A. N. (1996). Scoring noncovalent protein-ligand interactions: A continuous differentiable function tuned to compute binding affinities. J. Comput. Aided. Mol. Des. 10 (5), 427–440. doi:10.1007/BF00124474
Jana, N. R., and Pal, T. (1999). Redox catalytic property of still-growing and final palladium particles: A comparative study. Langmuir 15 (10), 3458–3463. doi:10.1021/la981512i
Kapoor, R. T., Danish, M., Singh, R. S., Rafatullah, M., and Abdul, A. K. (2021). Exploiting microbial biomass in treating azo dyes contaminated wastewater: Mechanism of degradation and factors affecting microbial efficiency. J. Water Process Eng. 43, 102255. doi:10.1016/j.jwpe.2021.102255
Karpuraranjith, M., and Thambidurai, S. (2017). Chitosan/zinc oxide-polyvinylpyrrolidone (CS/ZnO-PVP) nanocomposite for better thermal and antibacterial activity. Int. J. Biol. Macromol. 104, 1753–1761. doi:10.1016/j.ijbiomac.2017.02.079
Khalavka, Y., Becker, J., and Sönnichsen, C. (2009). Synthesis of rod-shaped gold nanorattles with improved plasmon sensitivity and catalytic activity. J. Am. Chem. Soc. 131 (5), 1871–1875. doi:10.1021/ja806766w
Khan, A. D., Ikram, M., Haider, A., Ul-Hamid, A., Nabgan, W., and Haider, J. (2022). Polyvinylpyrrolidone and chitosan-doped lanthanum oxide nanostructures used as anti-bacterial agents and nano-catalyst. Appl. Nanosci. 12 (7), 2227–2239. doi:10.1007/s13204-022-02471-0
Klinbumrung, A., Thongtem, T., and Thongtem, S. (2012). Characterization of orthorhombic α-MoO 3 microplates produced by a microwave plasma process. J. Nanomater. 2012, 1–5. doi:10.1155/2012/930763
Krishnamoorthy, K., Premanathan, M., Veerapandian, M., and Jae Kim, S. (2014). Nanostructured molybdenum oxide-based antibacterial paint: Effective growth inhibition of various pathogenic bacteria. Nanotechnology 25 (31), 315101. doi:10.1088/0957-4484/25/31/315101
Krishnamoorthy, K., Veerapandian, M., Yun, K., and Kim, S. J. (2013). New function of molybdenum trioxide nanoplates: Toxicity towards pathogenic bacteria through membrane stress. Colloids Surfaces B Biointerfaces 112, 521–524. doi:10.1016/j.colsurfb.2013.08.026
Kupnik, K., Primožič, M., Kokol, V., and Leitgeb, M. (2020). Nanocellulose in drug delivery and antimicrobially active materials. Polymers 12 (12), 2825–2840. doi:10.3390/polym12122825
Lewis, G. (2012). Nucleus pulposus replacement and regeneration/repair technologies: Present status and future prospects. J. Biomed. Mater. Res. - Part B Appl. Biomaterials 100 B (6), 1702–1720. doi:10.1002/jbm.b.32712
Li, X., Yu, J., Wageh, S., Al-Ghamdi, A. A., and Xie, J. (2016). Graphene in photocatalysis: A review. Small 12 (48), 6640–6696. doi:10.1002/smll.201600382
Li, Y., Shaheen, S. M., Azeem, M., Zhang, L., Feng, C., Peng, J., et al. (2022). Removal of lead (Pb+2) from contaminated water using a novel MoO3-biochar composite: Performance and mechanism. Environ. Pollut. 308, 119693. doi:10.1016/j.envpol.2022.119693
Lu, G., Li, S., Guo, Z., Farha, O. K., Hauser, B. G., Qi, X., et al. (2012). Imparting functionality to a metal-organic framework material by controlled nanoparticle encapsulation. Nat. Chem. 4 (4), 310–316. doi:10.1038/nchem.1272
Maerzke, K. A., and Siepmann, J. I. (2011). Transferable potentials for phase equilibria - coarse-Grain description for linear alkanes. J. Phys. Chem. B 115 (13), 3452–3465. doi:10.1021/jp1063935
Mehmood, Z., Ikram, M., Imran, M., Shahzadi, A., Haider, A., Ul-Hamid, A., et al. (2022). Z. officinale-doped silver/calcium oxide nanocomposites: Catalytic activity and antimicrobial potential with molecular docking analysis. Process Biochem. 121, 635–646. doi:10.1016/j.procbio.2022.07.035
Munawar, T., Nadeem, M. S., Mukhtar, F., Rehman, M. N. u., Riaz, M., Batool, S., et al. (2022). Transition metal-doped SnO2 and graphene oxide (GO) supported nanocomposites as efficient photocatalysts and antibacterial agents. Environ. Sci. Pollut. Res. 29 (60), 90995–91016. doi:10.1007/s11356-022-22144-3
Naseem, T., and Durrani, T. (2021). The role of some important metal oxide nanoparticles for wastewater and antibacterial applications: A review. Environ. Chem. Ecotoxicol. 3, 59–75. doi:10.1016/j.enceco.2020.12.001
Nasrollahzadeh, M., Sajjadi, M., Iravani, S., and Varma, R. S. (2021). Green-synthesized nanocatalysts and nanomaterials for water treatment: Current challenges and future perspectives. J. Hazard. Mater. 401, 123401. doi:10.1016/j.jhazmat.2020.123401
Naz, S., Javid, I., Konwar, S., Surana, K., Singh, P. K., Sahni, M., et al. (2020). A simple low cost method for synthesis of SnO2 nanoparticles and its characterization. SN Appl. Sci. 2 (5), 975. doi:10.1007/s42452-020-2812-2
Nccls, (2007). Performance standards for antimicrobial susceptibility testing. Clin. Lab. Standars Inst. - NCCLS 27 (1), 1–182. https://agris.fao.org/agris-search/search.do?recordID=US201300057088.Accessed: Mar. 05, 2023. [Online]. Available:
Panigrahi, S., Basu, S., Praharaj, S., Pande, S., Jana, S., Pal, A., et al. (2007). Synthesis and size-selective catalysis by supported gold nanoparticles: Study on heterogeneous and homogeneous catalytic process. J. Phys. Chem. C 111 (12), 4596–4605. doi:10.1021/jp067554u
Qumar, U., Hassan, J., Naz, S., Haider, A., Raza, A., Ul-Hamid, A., et al. (2021). Silver decorated 2D nanosheets of GO and MoS2serve as nanocatalyst for water treatment and antimicrobial applications as ascertained with molecular docking evaluation. Nanotechnology 32 (25), 255704. doi:10.1088/1361-6528/abe43c
Rafiq, A., Ikram, M., Ali, S., Niaz, F., Khan, M., Khan, Q., et al. (2021). Photocatalytic degradation of dyes using semiconductor photocatalysts to clean industrial water pollution. J. Industrial Eng. Chem. 97, 111–128. doi:10.1016/j.jiec.2021.02.017
Sampson, A., and de Korte, D. (2011). DEHP-Plasticised PVC: Relevance to blood services. Annu. Dep. Antiq. Jordan 55, 73–83. doi:10.1111/j.1365-3148.2010.01056.xhttps://ixtheo.de/Record/1670668762.Accessed: Apr. 09, 2023. [Online]. Available:
Santhosh, C., Velmurugan, V., Jacob, G., Jeong, S. K., Grace, A. N., and Bhatnagar, A. (2016). Role of nanomaterials in water treatment applications: A review. Chem. Eng. J. 306, 1116–1137. doi:10.1016/j.cej.2016.08.053
Seefeld, M. A., Miller, W. H., Newlander, K. A., Burgess, W. J., DeWolf, W. E., Elkins, P. A., et al. (2003). Indole naphthyridinones as inhibitors of bacterial enoyl-ACP reductases FabI and FabK. J. Med. Chem. 46 (9), 1627–1635. doi:10.1021/jm0204035
Shahmiri, M., Ibrahim, N. A., Shayesteh, F., Asim, N., and Motallebi, N. (2013). Preparation of PVP-coated copper oxide nanosheets as antibacterial and antifungal agents. J. Mat. Res. 28 (22), 3109–3118. doi:10.1557/jmr.2013.316
Shahzadi, I., Islam, M., Saeed, H., Haider, A., Shahzadi, A., Haider, J., et al. (2022). Formation of biocompatible MgO/cellulose grafted hydrogel for efficient bactericidal and controlled release of doxorubicin. Int. J. Biol. Macromol. 220, 1277–1286. doi:10.1016/j.ijbiomac.2022.08.142
Shahzadi, I., Islam, M., Saeed, H., Shahzadi, A., Haider, J., Haider, A., et al. (2023). Facile synthesis of copolymerized cellulose grafted hydrogel doped calcium oxide nanocomposites with improved antioxidant activity for anti-arthritic and controlled release of doxorubicin for anti-cancer evaluation. Int. J. Biol. Macromol. 235, 123874. doi:10.1016/j.ijbiomac.2023.123874
Sinclair, C. G. (1939). Bergey’s manual of determinative Bacteriology. Am. J. Trop. Med. Hyg. s1-19 (6), 605–606. doi:10.4269/ajtmh.1939.s1-19.605
Song, J., Ni, X., Gao, L., and Zheng, H. (2007). Synthesis of metastable h-MoO3 by simple chemical precipitation. Mat. Chem. Phys. 102 (2–3), 245–248. doi:10.1016/j.matchemphys.2006.12.011
Summerfield, R. L., Daigle, D. M., Mayer, S., Mallik, D., Hughes, D. W., Jackson, S. G., et al. (2006). A 2.13 Å structure of E. coli dihydrofolate reductase bound to a novel competitive inhibitor reveals a new binding surface involving the M20 loop region. J. Med. Chem. 49 (24), 6977–6986. doi:10.1021/jm060570v
V Jadhav, S., Nikam, D. S., Khot, V. M., Thorat, N. D., Phadatare, M. R., Ningthoujam, R. S., et al. (2013). Studies on colloidal stability of PVP-coated LSMO nanoparticles for magnetic fluid hyperthermia. New J. Chem. 37 (10), 3121–3130. doi:10.1039/c3nj00554b
Weidmann, P., Schneider, M., and Bohlen, L. (1995). Therapeutic efficacy of different antihypertensive drugs in human diabetic nephropathy: An updated meta-analysis. Nephrol. Dial. Transplant. 10 (9), 39–45. doi:10.1093/ndt/10.supp3.39https://pubmed.ncbi.nlm.nih.gov/8643207/.Accessed: Apr. 09, 2023. [Online]. Available:
Xiang, Q., Yu, J., and Jaroniec, M. (2012). Graphene-based semiconductor photocatalysts. Chem. Soc. Rev. 41 (2), 782–796. doi:10.1039/c1cs15172j
Yao, S., Zhou, S., Wang, J., Li, W., and Li, Z. (2019). Optimizing the synthesis of SnO2/TiO2/RGO nanocomposites with excellent visible light photocatalytic and antibacterial activities. Photochem. Photobiol. Sci. 18 (12), 2989–2999. doi:10.1039/c9pp00242a
Yogananda, H. S., Nagabhushana, H., Darshan, G., Basavaraj, R., Daruka Prasad, B., Sateesh, M., et al. (2018). MoO3 nanostructures from EGCG assisted sonochemical route: Evaluation of its application towards forensic and photocatalysis. J. Alloys Compd. 745, 874–891. doi:10.1016/j.jallcom.2017.11.278
Yu, C. H., Chen, G. Y., Xia, M. Y., Xie, Y., Chi, Y. Q., He, Z. Y., et al. (2020). Understanding the sheet size-antibacterial activity relationship of graphene oxide and the nano-bio interaction-based physical mechanisms. Colloids Surfaces B Biointerfaces 191, 111009. doi:10.1016/j.colsurfb.2020.111009
Zhao, Y., Xu, J., Li, Z., Fu, T., and Jiang, S. (2020). In vitro antibacterial properties of MoO3/SiO2/Ag2O nanocomposite coating prepared by double cathode glow discharge technique. Surf. Coatings Technol. 397, 125992. doi:10.1016/j.surfcoat.2020.125992
Keywords: MoO3 nanorods, catalytic activity, antibacterial activity, molecular docking, analysis
Citation: Ikram M, Atiq I, Rafiq Butt A, shahzadi I, Ul-Hamid A, Haider A, Nabgan W and Medina F (2023) Graphene oxide/polyvinylpyrrolidone-doped MoO3 nanocomposites used for dye degradation and their antibacterial activity: a molecular docking analysis. Front. Chem. 11:1191849. doi: 10.3389/fchem.2023.1191849
Received: 22 March 2023; Accepted: 21 April 2023;
Published: 09 May 2023.
Edited by:
Suresh Neethirajan, Farmworx Research Institute, NetherlandsReviewed by:
Murugan Veerapandian, Central Electrochemical Research Institute (CSIR), IndiaXuan Weng, University of Electronic Science and Technology of China, China
Copyright © 2023 Ikram, Atiq, Rafiq Butt, shahzadi, Ul-Hamid, Haider, Nabgan and Medina. This is an open-access article distributed under the terms of the Creative Commons Attribution License (CC BY). The use, distribution or reproduction in other forums is permitted, provided the original author(s) and the copyright owner(s) are credited and that the original publication in this journal is cited, in accordance with accepted academic practice. No use, distribution or reproduction is permitted which does not comply with these terms.
*Correspondence: Muhammad Ikram, ZHIubXVoYW1tYWRpa3JhbUBnY3UuZWR1LnBr; Ali Haider, YWxpLmhhaWRlckBtbnN1YW0uZWR1LnBr; Walid Nabgan, d2FsaWQubmFiZ2FuQHVydi5jYXQ=