- Laboratory of Organic and Metal-Organic Nitrogen-Oxygen Systems, N. D. Zelinsky Institute of Organic Chemistry, Russian Academy of Sciences, Moscow, Russia
β-Hydrazonophosphine oxides are precursors of useful organophosphorus compounds, including phosphorylated N-heterocycles, α-aminophosphonates, and vinylphosphonates. In this work, a general transition metal-free synthesis of β-hydrazonophosphine oxides was developed. The method relies on the Michael addition of phosphine oxides R2P(O)H to reactive azoalkenes (1,2-diaza-1,3-butadienes), which are generated in situ from α-halohydrazones and Hunig’s base. The reaction stereoselectively leads to Z-isomers of β-hydrazonophosphine oxides that are stabilized by intramolecular hydrogen bonding. The conversion of the products thus obtained into potential chelating ligands was showcased.
1 Introduction
Organophosphorus compounds containing nitrogen are extensively used as pharmaceutical ingredients (Rodriguez and Gallo-Rodriguez, 2019; Yu et al., 2020) and pesticides (Ajiboye et al., 2022). Although the majority of phosphorous-containing drugs correspond to derivatives of phosphoric and phosphonic acids (esters/ amides), phosphine oxides are also applied in clinical practice (Yu et al., 2020). Well-known examples include fosazepam (Nicholson and Wright, 1977), brigatinib (Huang et al., 2016), and fosenazide (Zainkonnikova et al., 1980) (Scheme 1, A). Apart from a biological use, phosphine oxides having additional nitrogen functionality can serve as ligands for transition metal catalysis (Minghetti et al., 1998; Pailloux et al., 2011; Zhang et al., 2012; Aleksanyan et al., 2013; Nyamato et al., 2015; Junges et al., 2019; Enikeeva et al., 2023) and as building blocks for organic synthesis via Wittig and HWE-type reactions (Palacios et al., 1994) (Scheme 1B).
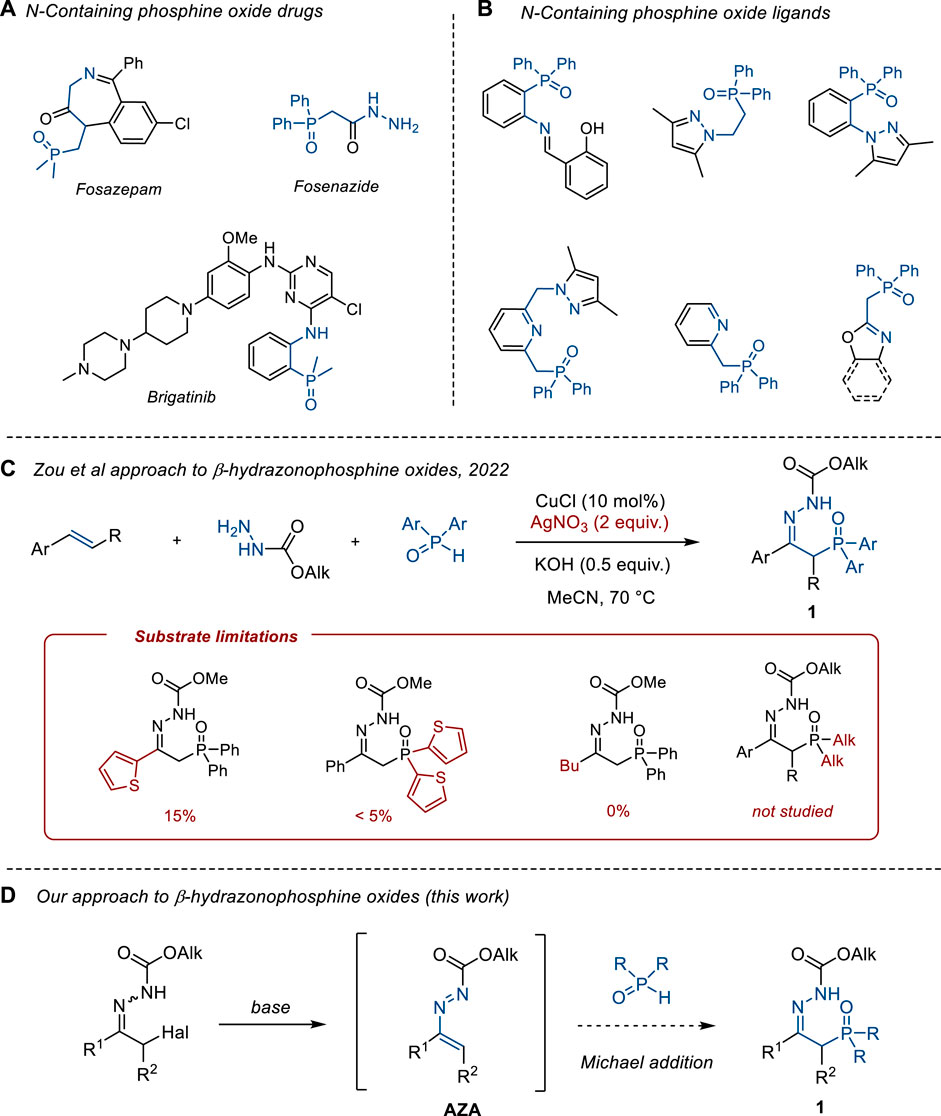
Scheme 1. Background of this study. (A) N-Containing phosphine oxide drugs. (B) N-Containing phosphine oxide ligands. (C) Zou et al approach to hydrazonophosphine oxides 1. (D) Our approach to hydrazonophosphine oxides 1.
Despite the tremendous recent progress in the assembly of the carbon–phosphorus bond via transition metal and photoredox catalysis (Tappe et al., 2010; Luo et al., 2017), the development of practical methods for the synthesis of N-containing organophosphorus compounds is still in high demand. For instance, this applies to the synthesis of β-hydrazonophosphine oxides 1, which were previously shown to be convenient precursors of phosphorylated pyrazoles (Palacios et al., 1996; Hassen and Hajjem, 2006), pyrroles (Palacios et al., 1999), pyrrolones (Palacios et al., 2001), pyridinones (Palacios et al., 2001), pyridazines (Palacios et al., 2005b), phosphinyl-substituted ketones (Corbel et al., 1985; Ogundipe et al., 2022b), 1-aza- and 1,2-diaza-1,3-butadienes (Palacios et al., 2006; Hassen et al., 2008), α-aminophosphonates (Palacios et al., 2004; Palacios et al., 2005a), α,β-unsaturated hydrazones (Palacios et al., 1993; Palacios et al., 1994), azaprolines (de los Santos et al., 2008), and vinylphosphonates (He et al., 2020). Recently, Zou et al. developed a Cu-catalyzed hydrazono-phosphinoylation of alkenes with a diarylphosphine oxide approach to β-hydrazonophosphine oxide 1 (Ogundipe et al., 2022a) (Scheme 1C). However, the substrate scope of this method is mostly limited to α-C and P-aryl-substituted products. Heteroaryl- and alkyl-substituted hydrazones 1 are not accessible via this reaction, as exemplified in Scheme 1C. Also, the method requires the use of excess silver salt (2 equiv.) as an oxidant, which makes the procedure inapplicable for large-scale synthesis. Similar limitations apply to a related Cu-catalyzed phosphono-hydrazonation of alkynes (Ogundipe et al., 2022b). Other approaches to β-hydrazonophosphine oxide 1 rely on the Arbuzov reaction of α-chlorohydrazones with alkyl phosphinites (Corbel et al., 1985; Delarue-Cochin et al., 2007) and the Michael addition of hydrazines to phosphinyl-substituted allenes (Palacios et al., 1993) or vinyl phosphonium salts (Ovakimyan et al., 2002). These methods require either sophisticated precursors or drastic reaction conditions, thus limiting the substrate scope.
Based on our recent studies on azoalkenes (1,2-diaza-1,3-butadienes, AZA) as enolates umpolung synthons (Semakin et al., 2016; Ushakov et al., 2019; Kokuev et al., 2021), we envisioned that this approach can be efficiently used to access hydrazones of type 1 in a transition metal-free fashion. Being highly active Michael acceptors, azoalkenes react with nucleophiles (Grignard reagents, enolates, amines, silyl azides, ylides, etc.) to give α-substituted hydrazones (Attanasi and Caglioti, 1986; Schantl, 1996; Attanasi et al., 2002; Attanasi et al., 2009; Lemos, 2009; Lopes et al., 2018; Ushakov et al., 2022). Surprisingly, the addition of P-nucleophiles to azoalkenes remains underexplored (Attanasi et al., 1992; Attanasi et al., 2005; Attanasi et al., 2008), and no examples of the addition of disubstituted phosphine oxides R2P(O)H have been reported to the best of our knowledge. Thus, here, we wish to report our studies on the reaction of phosphine oxides R2P(O)H with azoalkenes AZA that led to the development of a general and practical method for the synthesis of β-hydrazonophosphine oxides 1.
2 Results and discussion
2.1 Optimization of reaction conditions
Being of a labile species liable to dimerization and other side reactions, azoalkenes are usually generated in situ by dehydrohalogenation of α-halohydrazones (Zhang et al., 2016; Lopes et al., 2018). Due to instability issues, finding suitable conditions for efficient coupling is often challenging and requires special research for each particular nucleophile. The nature of the base was shown to be essential as it controls the stationary concentrations of the AZA intermediate and the nucleophile (if the nucleophile is taken in the H-form) (Kokuev et al., 2021). Thus, initially, the reaction of model acetophenone-derived hydrazone 2a (Z-isomer) and diphenylphosphine oxide 3a in the presence of different bases was studied (Table 1). In these experiments, diphenylphosphine oxide 3a was initially treated with a base in THF, and then the solution of hydrazone 2a in THF was slowly added via a syringe pump.
In the absence of a base, no desired hydrazone 1aa was formed (Table 1, entry 1). Alkali metal carbonates are commonly applied as bases to generate azoalkenes from α-halohydrazones (Lopes et al., 2018). Indeed, reactions with potassium and cesium carbonates afforded the desired phosphorylated hydrazone 1aa, yet only in moderate yields (Table 1, entries 2, 3). The use of stronger bases, such as tBuOK and NaH, did not lead to any improvement in the product yield (Table 1, entries 4, 5). We then switched to organic amine bases that are rarely used to generate azoalkenes. While DBU showed poor performance (Table 1, entry 6), triethylamine gave the desired product in 54% yield (Table 1, entry 7). We reasoned that these amines may undergo a competitive Michael addition to the transient AZA, leading to side products (Vuillermet et al., 2023). Gratifyingly, the use of a more sterically encumbered Hunig’s base (iPr2NEt) resulted in the highest yield (84%) of hydrazone 1aa (Table 1, entry 8). The amount of diphenylphosphine oxide 3a, the base, and the reaction time could be reduced while maintaining the efficiency of the reaction (Table 1, entry 9).
2.2 Substrate scope studies
The substrate scope of the reaction was studied under optimized conditions (Scheme 2). α-Bromohydrazones 2a−g derived from substituted acetophenones delivered the desired products 1a−g in the reaction with diphenylphosphine oxide 3a in high yields. Both electron-rich and electron-poor substituents in the aromatic ring were tolerated in the reaction. It is noteworthy that the challenging thiophenyl-substituted product 1ha was obtained in 38% yield, which is higher than that in the literature method (Scheme 1C) (Ogundipe et al., 2022a). Tetralone-derived product 1ia was prepared in 70% yield, demonstrating that β-substituted azoalkenes efficiently enter Michael addition with diphenylphosphine oxide 3a. The method proved to be applicable to aliphatic substrates, as exemplified by the successful synthesis of products 1ja and 1ka. Apart from Boc-hydrazones, the corresponding Cbz-derivatives were shown to enter the process efficiently (as demonstrated by the successful preparation of product 1la).
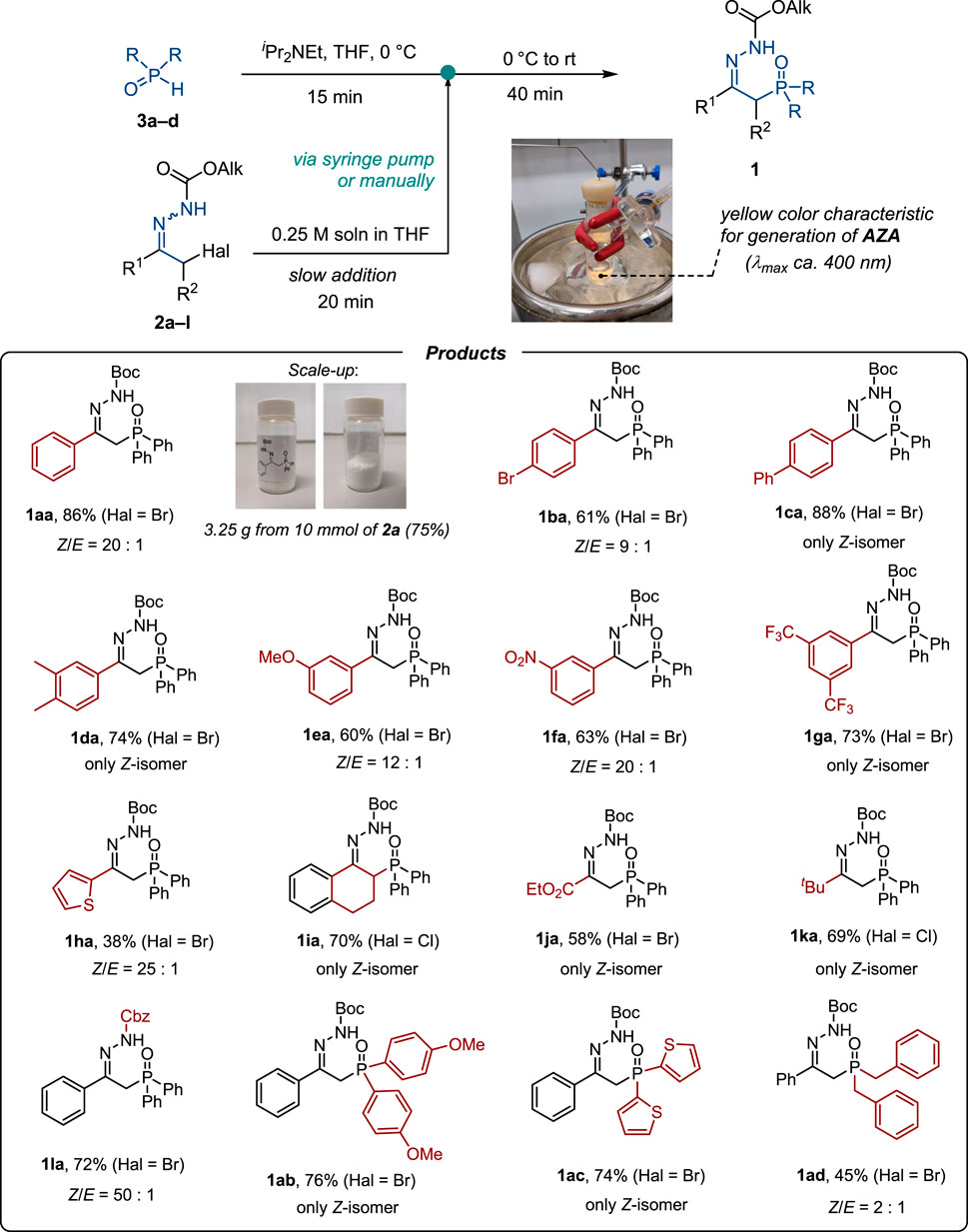
Scheme 2. Substrate scope studies. Boc–tBuOC(O), Cbz–PhCH2OC(O). Yields and Z/E ratios are given for isolated products.
The scope of phosphine oxides was also studied. Apart from diphenylphosphine oxide 3a, di(4-methoxyphenyl)phosphine oxide 3b, di(2-thienyl)phosphine oxide 3c, and dibenzylphosphine oxide 3d afforded the corresponding P-substituted hydrazones 1ab−1ad in moderate to high yields (Scheme 2). It is of note that di(2-thienyl)phosphine oxide 3d was not tolerated in Zou’s method; thus, products of type 1ac could not be accessed (cf. with Scheme 1C) (Ogundipe et al., 2022a).
The process is scalable, as demonstrated by the synthesis of 3.25 g of product 1aa without a significant loss of efficiency. It is of note that the required slow addition of a solution of hydrazone 3 could be performed manually without the need for the syringe pump technique.
Studies on the substrate scope show that the developed approach to β-hydrazonophosphine oxides 1 is more general than the methods reported previously. To further explore the scope and limitations of our method, the Glorius additive approach was followed (Collins and Glorius, 2013). In this approach, the functional group tolerance and reaction robustness are assessed by performing the model reaction in the presence of simple and commercially available additives bearing common functionalities. A set of seventeen additives was chosen for this study, including those possessing alkene, alkyne, hydroxyl (alcohol, phenol), thiol, amine (primary, secondary, tertiary, heterocyclic), nitrile, aldehyde, ketone, electron-rich aromatics, and tertiary phosphine moieties (Scheme 3).
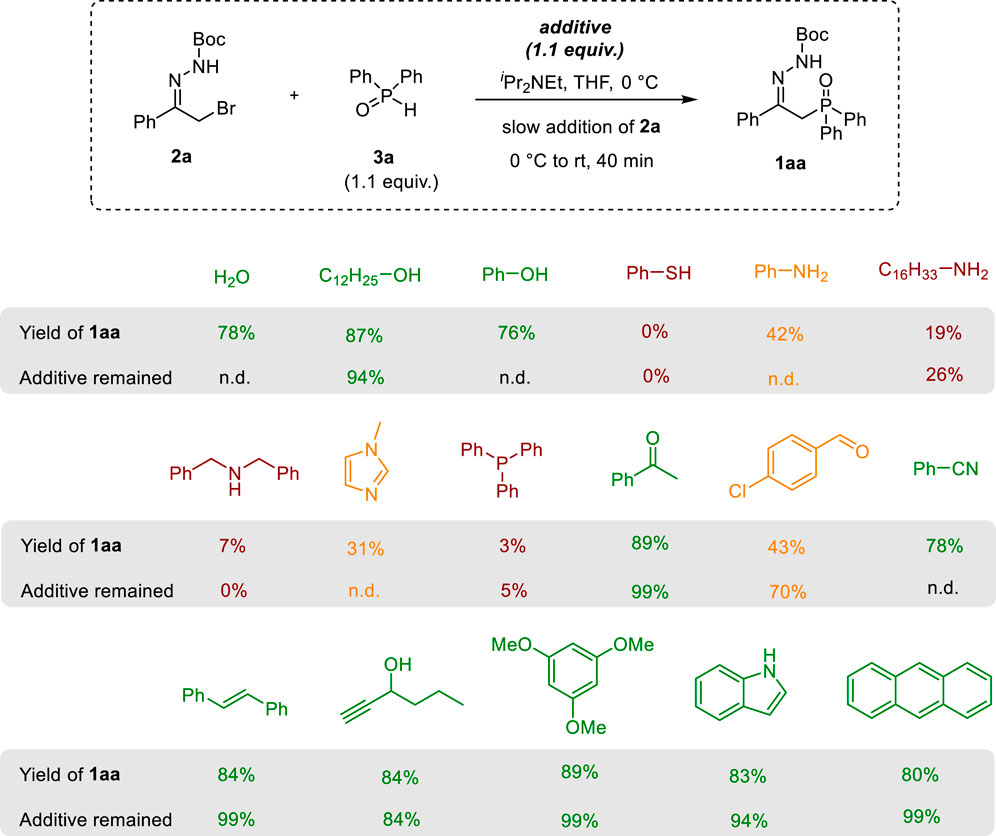
Scheme 3. Study of the effect of additives on the reaction efficiency. Yields were determined by 1H NMR with an internal standard (trichloroethylene) in the reaction mixtures. n.d.—not determined, Boc–tBuOC(O). The following color coding is used: green–additives that are tolerated; red–additives inhibiting the reaction to a large extent; orange–additives showing a moderate effect on the product yield.
Accordingly, the reaction of model bromohydrazone 2a with diphenylphosphine oxide 3a was performed in the presence of each of these additives. The yields of the Michael addition product 1aa and the additive were measured as indicators of the effect of the functional group on the reaction efficiency and tolerance of the functional group under the reaction conditions (the results are summarized in Scheme 3). As seen from this study, water, aliphatic alcohols, and phenols are well tolerated in the reaction. In contrast, thiophenol completely inhibited the formation of the desired product. Amines, including aniline and imidazole, were tolerated poorly. In a similar fashion, triphenylphosphine had a dramatic negative effect on the yield of product 1aa. In all cases, these additives were consumed under the reaction conditions. These results are logical as thiols, amines, and phosphines are strong nucleophiles that can compete with Ph2P(O)H in the Michael addition to azoalkene intermediates (Semakin et al., 2016; Vuillermet et al., 2023). Aromatic ketones and nitriles did not interfere with the reaction, whereas the addition of p-chlorobenzaldehyde led to a substantial decrease in the yield of 1aa. In the latter case, the nucleophilic addition of Ph2P(O)H to aldehyde is likely to compete with the Michael addition to the AZA intermediate (Zheng et al., 2013).
Since azoalkenes can act as heterodienes in the Diels–Alder reaction (Lopes et al., 2018), additives containing activated π bonds were also studied. We were pleased to find that alkenes, alkynes, electron-rich aromatic rings, and indole were fully tolerated. High yields of product 1aa and excellent recovery of the additives were observed in all these experiments. Thus, the addition of Ph2P(O)H to AZA occurs faster than the Diels–Alder reaction and the Michael addition of π-nucleophiles.
2.3 Stereochemistry and reaction mechanism
For all the β-hydrazonophosphine oxides 1 obtained, the Z-isomer was selectively formed (assignment of the C=N bond configuration was performed on the basis of 2D NOESY and characteristic 13C and 31P NMR shifts, see Supplementary Material for details). We believe that the Z-isomer is thermodynamically more stable than the E-isomer due to the intramolecular hydrogen bond between the C(O)N−H and phosphine oxide moieties [no isomerization of (Z)-1aa was detected upon prolonged heating at 60°C]. The existence of this H-bond is corroborated by a strong downfield NMR shift (ca. 10–11 ppm in CDCl3) of the NH hydrogen in (Z)-1 [ca. 8–9 ppm in (E)-1] and the appearance of νN−H stretching as a broad band at ca. 3,200 cm-1 in the FT-IR spectra. According to DFT calculations at wB97M-D4/def2-TZVP (CPCM: CH2Cl2) level of theory, the more stable (Z)-1aa differs from (E)-1aa by 3.5 kcal/mol (ΔG°). DFT simulation also supports the formation of the intramolecular H-bond (d N(H)…O 2.90 Å, see Supplementary Material for details).
Yet another possible reason for the preferential formation of Z-isomers is of kinetic origin. Both transoid and cisoid conformations of azoalkenes AZA are known to participate in cycloadditions and Michael-type reactions (Clarke et al., 1983; Ferguson et al., 1991). However, the observed Z-isomers of products 1 originate from the addition of diphenylphosphine oxide tautomer Ph2POH to the cisoid conformation of AZA. We believe that the corresponding transition state (Z)-TS is additionally stabilized by the formation of an intramolecular H-bond, as shown in Scheme 4. NMR analysis of crude reaction mixtures resulting from the synthesis of hydrazone 1aa (before chromatographic separation) showed only trace amounts of the (E)-isomer, thus confirming that the (Z)-isomer is a kinetic product [E/Z-isomerization is relatively slow in acyl hydrazones, see Benassi et al. (1982)].
The intermediacy of AZA in the reaction was evidenced by the appearance of yellow color (λmax ca. 400 nm), which is characteristic of azo compounds (see Scheme 2 and the Supplementary Material for the UV-Vis spectra). Also, dimer of AZA resulting from a self [4 + 2]-cycloaddition reaction was detected by HRMS and 1H NMR in the reaction of 2a with Hunig’s base.
2.4 Post-transformations of hydrazone 1aa
As mentioned in the introduction, the reactivity of β-hydrazonophosphine oxides was extensively explored in previous studies. However, since Boc-protected derivatives 1 obtained by our method were not reported previously, some useful post-transformations were showcased on the model substrate 1aa (Scheme 5). Deprotection of (Z)-1aa with TFA gave free hydrazone 4aa in 90% yield as a 2:1 mixture of Z/E-isomers. The latter was then brought in the reaction with salicylic aldehyde to give azine 5aa, which is a structural analog of known chelating phosphine oxide ligands (cf. with Scheme 1) (Hii et al., 1992). Remarkably, only the Z-isomer of hydrazone 4aa underwent condensation with salicylic aldehyde, while the (E)-4aa isomer remained unreacted and was recovered. Another potential hydrazide-type ligand 6aa was obtained by hydrogenation of the C=N bond in 1aa over a Pd/C catalyst. It is of note that the nitrogen–nitrogen bond remained intact under these reductive conditions.
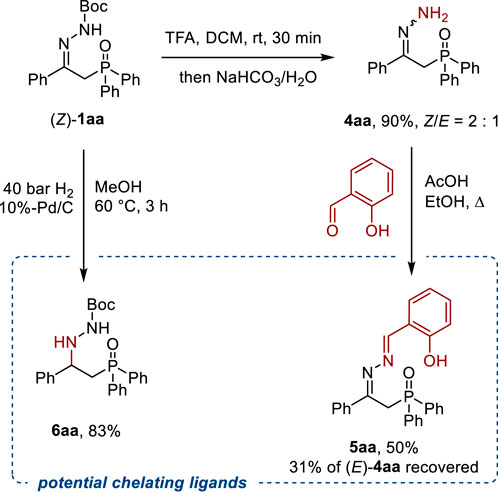
Scheme 5. Post-transformations of Boc-protected β-hydrazonophosphine oxide 1aa and synthesis of potential chelating ligands for transition metals. Boc–tBuOC(O).
3 Materials and methods
For general experimental, instrumental, and computational methods, synthetic procedures, and full compound characterization, see the Supplementary Material.
3.1 General procedure for the synthesis of β-hydrazonophosphine oxide 1
To a stirred solution of phosphine oxide 3 (0.275 mmol) in THF (1 mL) was added iPr2NEt (0.625 mmol, 108 μL) at 0°C under an argon atmosphere. The mixture was stirred for 15 min at the same temperature. Then, a solution of α-halohydrazone 2 (0.25 mmol) in THF (1 mL) was slowly added in small portions over 20 min (manually or via a syringe pump). The mixture was stirred for 10 min at 0°C, and the cooling bath was removed. After stirring for additional 30 min, the mixture was concentrated in a vacuum. The residue was subjected to column chromatography on silica gel to give the corresponding β-hydrazonophosphine oxide 1.
3.2 Gram-scale synthesis of β-hydrazonophosphine oxide 1aa
To a stirred solution of diphenylphosphine oxide 3a (10.9 mmol, 2.2 g) in THF (40 mL) was added iPr2NEt (25 mmol, 4.32 mL) at 0°C. The mixture was stirred for 15 min at the same temperature. Then, a solution of α-bromohydrazone 2a (10 mmol, 3.13 g) in THF (40 mL) was slowly added in small portions over 20 min. The mixture was stirred for 10 min at 0°C, and the cooling bath was removed. After stirring for additional 30 min, the mixture was concentrated in a vacuum. The residue was dissolved in ethyl acetate (500 mL) and extracted with water. The organic layer was concentrated in a vacuum, and the residue was triturated with methyl tert-butyl ether to give 2.92 g of product 1aa. The mother liquor residue was subjected to column chromatography on silica gel to give an additional 330 mg of product 1aa. Overall yield: 3.25 g (75%).
3.3 Tert-butyl 2-[2-(diphenylphosphoryl)-1-phenylethylidene]hydrazinecarboxylate (1aa)
White crystals. Mp 188°C–190°C (AcOEt). Mixture of Z/E isomers (ratio 20:1). 1H NMR (300 MHz, chloroform-d, Z-isomer) δ 10.93 (s, 1 H, NH), 7.68 (dd, J = 12.0, 8.3, 4 H, o-CHPh-P), 7.57 – 7.03 (m, 11 H, Ph), 3.81 (d, J = 14.7 Hz, 2 H, CH2), 1.56 (s, 9 H, t-Bu). 13C NMR (76 MHz, DEPT, HMBC, chloroform-d, Z-isomer) δ 154.6 (C=O), 141.4 (C=N), 137.9 (d, J = 3.0 Hz, CPh), 132.7 (d, J = 2.8 Hz, 2 CHPh-P), 131.1 (d, J = 10.0 Hz, 4 CHPh-P), 130.5 (d, J = 106 Hz, 2 C−P), 128.9 (CHPh), 128.8 (d, J = 12.2 Hz, 4 CHPh-P), 128.0 (2 CHPh), 126.5 (2 CHPh), 80.8 (CMe3), 33.4 (d, J = 63.7 Hz, CH2P), 28.3 (3 Me). 31P NMR (122 MHz, chloroform-d, HMBC, Z-isomer) δ 33.50. Characteristic 2D NOESY correlations (Z-isomer): NH/CH2P, NH/o-CHPh-P. 1H NMR (300 MHz, chloroform-d, E-isomer, characteristic signals) δ 8.86 (br s, 1 H, NH), 4.14 (d, J = 15.2 Hz, 2 H, CH2P). 31P NMR (122 MHz, chloroform-d, E-isomer) δ 29.07. HRMS: m/z [M + H]+ calcd. for [C25H28N2O3P]+: 435.1832; found: 435.1825. Anal. Calcd. for C25H27N2O3P: C, 69.11%; H, 6.26%; N, 6.45%. Found: C, 68.84%; H, 6.47%; N, 6.18%.
4 Conclusion
In conclusion, a convenient transition metal-free method for the synthesis of useful β-hydrazonophosphine oxides from readily available α-halohydrazones was developed. The method features a broad substrate scope, mild reaction conditions, scalability, and stereoselectivity (Z-isomers are formed). The reaction tolerates numerous functional groups (alkene, alkyne moieties, electron-rich aromatic rings, indole, aliphatic and aromatic hydroxyl groups, and ketone and nitrile functionalities), as shown using the Glorius additive approach. The reaction mechanism most likely involves the generation of azoalkenes from α-halohydrazones followed by a hydrogen bond-assisted Michael addition of R2POH. Conversion of the β-hydrazonophosphine oxides thus obtained into potential chelating ligands was showcased through a chemoselective reduction of the C=N bond and deprotection/azine formation strategies.
Data availability statement
The original contributions presented in the study are included in the article/Supplementary Material; further inquiries can be directed to the corresponding author.
Author contributions
AK performed the experimental part and prepared the supporting information; AS designed the work and wrote the manuscript.
Acknowledgments
The authors are grateful to Dr. Andrey Tabolin for measuring NMR spectra and to Mr. Roman S. Malykhin for performing DFT calculations.
Conflict of interest
The authors declare that the research was conducted in the absence of any commercial or financial relationships that could be construed as a potential conflict of interest.
Publisher’s note
All claims expressed in this article are solely those of the authors and do not necessarily represent those of their affiliated organizations, or those of the publisher, the editors, and the reviewers. Any product that may be evaluated in this article, or claim that may be made by its manufacturer, is not guaranteed or endorsed by the publisher.
Supplementary material
The Supplementary Material for this article can be found online at: https://www.frontiersin.org/articles/10.3389/fchem.2023.1177680/full#supplementary-material
References
Ajiboye, T. O., Oladoye, P. O., Olanrewaju, C. A., and Akinsola, G. O. (2022). Organophosphorus pesticides: Impacts, detection and removal strategies. Environ. Nanotechnol. Monit. Manag. 17, 100655. doi:10.1016/j.enmm.2022.100655
Aleksanyan, D. V., Aleksenko, V. Y., Nelyubina, Y. V., Vasil’ev, A. A., Aysin, R. R., Klemenkova, Z. S., et al. (2013). Monoanionic salicylaldimine ligands with (thio)phosphoryl pendant arms: Synthesis and complexing features. Inorg. Chim. Acta 404, 167–174. doi:10.1016/j.ica.2013.04.022
Attanasi, O. A., Baccolini, G., Boga, C., De Crescentini, L., Filippone, P., and Mantellini, F. (2008). Carbon–phosphorus bond formation and transformation in the reaction of 1,2-diaza-1,3-butadienes with alkyl phenylphosphonites. Tetrahedron 64, 6724–6732. doi:10.1016/j.tet.2008.05.007
Attanasi, O. A., Baccolini, G., Boga, C., De Crescentini, L., Filippone, P., and Mantellini, F. (2005). Solvent-free reaction of some 1,2-diaza-1,3-butadienes with phosphites: Environmentally friendly access to new diazaphospholes and E-hydrazonophosphonates. J. Org. Chem. 70, 4033–4037. doi:10.1021/jo050242n
Attanasi, O. A., and Caglioti, L. (1986). Conjugated azoalkenes: Attractive products and versatile intermediates. Org. Prep. Proced. Int. 18, 299–327. doi:10.1080/00304948609356836
Attanasi, O. A., De Crescentini, L., Favi, G., Filippone, P., Mantellini, F., Perrulli, F. R., et al. (2009). Cultivating the passion to build heterocycles from 1,2-diaza-1,3-dienes: The force of imagination. Eur. J. Org. Chem. 2009, 3109–3127. doi:10.1002/ejoc.200900243
Attanasi, O. A., De Crescentini, L., Filippone, P., Mantellini, F., and Santeusanio, S. (2002). 1, 2-Diaza-1,3-butadienes; just a nice class of compounds, or powerful tools in organic chemistry? Reviewing an experience. ARKIVOC 11, 274–292. doi:10.3998/ark.5550190.0003.b25
Attanasi, O. A., Filippone, P., and Mei, A. (1992). Conjugated azoalkenes. Part XI. Direct synthesis of substituted pyrazoles and 4-triphenylphosphoranylidene-4,5-dihydropyrazol-5-ones by heterocyclization of some zwitterionic 1,4-adducts between conjugated azoalkenes and triphenylphosphine. Tetrahedron 48, 1707–1714. doi:10.1016/s0040-4020(01)88729-9
Benassi, R., Benedetti, A., Taddei, F., Cappelletti, R., Nardi, D., and Tajana, A. (1982). Conformational analysis of hydrazones. 1H dynamic nuclear magnetic resonance and solvent effects in aryl- and 2-furylaldehyde ethylaminoacetylhydrazones. Org. Magn. Reson. 20, 26–30. doi:10.1002/mrc.1270200107
Clarke, S. J., Davies, D. E., and Gilchrist, T. L. (1983). Competing [4 + 2] and [3 + 2] cycloaddition in the reactions of nucleophilic olefins with ethyl 3-(toluene-p-sulphonylazo)but-2-enoate. J. Chem. Soc. Perkin Trans. 1, 1803–1807. doi:10.1039/p19830001803
Collins, K. D., and Glorius, F. (2013). A robustness screen for the rapid assessment of chemical reactions. Nat. Chem. 5, 597–601. doi:10.1038/nchem.1669
Corbel, B., Medinger, L., Haelters, J. P., and Sturtz, G. (1985). An efficient synthesis of dialkyl 2-oxoalkanephosphonates and diphenyl-2-oxoalkylphosphine oxides from 1-chloralkyl ketones. Synthesis 1985, 1048–1051.
de los Santos, J. M., López, Y., Aparicio, D., and Palacios, F. (2008). A convenient synthesis of substituted pyrazolidines and azaproline derivatives through highly regio- and diastereoselective reduction of 2-pyrazolines. J. Org. Chem. 73, 550–557. doi:10.1021/jo702050t
Delarue-Cochin, S., Pan, J.-J., Dauteloup, A., Hendra, F., Angoh, R. G., Joseph, D., et al. (2007). Asymmetric Michael reaction: Novel efficient access to chiral β-ketophosphonates. Tetrahedron Asymmetry 18, 685–691. doi:10.1016/j.tetasy.2007.02.023
Enikeeva, K. R., Shamsieva, A. V., Kasimov, A. I., Litvinov, I. A., Lyubina, A. P., Voloshina, A. D., et al. (2023). Pyridyl-containing dialkylphosphine oxides and their chelate copper(II) complexes. Inorg. Chim. Acta 545, 121286. doi:10.1016/j.ica.2022.121286
Ferguson, G., Lough, A. J., Mackay, D., and Weeratunga, G. (1991). Diversity of reaction of azoalkenes in cycloadditions. J. Chem. Soc. Perkin Trans. 1, 3361–3369. doi:10.1039/p19910003361
Hassen, Z., Chihi, A., and Hajjem, B. (2008). Action de l'Acide iodique sur les 2-pentafluorophenylhydrazonoalkylphosphonates et oxydes de phosphine: Synthèse d'azoalcènes phosphorylès. Phosphorus Sulfur Silicon Relat. Elem. 183, 1152–1160. doi:10.1080/10426500701589040
Hassen, Z., and Hajjem, B. (2006). Action des hydrazines fluoroalkylées sur les α-cetophosphonates: Synthèse des hydrazones α-phosphonylées N-fluoroalkylées. Phosphorus Sulfur Silicon Relat. Elem. 181, 987–993. doi:10.1080/10426500500272277
He, J., Feng, Y., Yang, F., Dai, B., and Liu, P. (2020). Palladium-catalyzed olefination of N-tosylhydrazones as β-diazo phosphonate precursors with arylhalides. Eur. J. Org. Chem. 2020, 5857–5861. doi:10.1002/ejoc.202000981
Hii, K. K., Perera, S. D., Shaw, B. L., and Thornton-Pett, M. (1992). New bidentate ligands PPh2CH2C(But)=NNR2 (R = H or Me) and PPh2CH2C(But)=NN=CHPh and their complexes with Group 6 metal carbonyls. J. Chem. Soc. Dalton Trans., 2361–2366. doi:10.1039/dt9920002361
Huang, W.-S., Liu, S., Zou, D., Thomas, M., Wang, Y., Zhou, T., et al. (2016). Discovery of brigatinib (AP26113), a phosphine oxide-containing, potent, orally active inhibitor of anaplastic lymphoma kinase. J. Med. Chem. 59, 4948–4964. doi:10.1021/acs.jmedchem.6b00306
Junges, C. H., Dresch, L. C., Da Costa, M. T., Tirloni, B., Casagrande, O. L., and Stieler, R. (2019). Pyrazolyl-phosphinoyl nickel (II) complexes: Synthesis, characterization and ethylene dimerization studies. Appl. Organomet. Chem. 33, e4887. doi:10.1002/aoc.4887
Kokuev, A. O., Ioffe, S. L., and Sukhorukov, A. Y. (2021). Addition of malonic esters to azoalkenes generated in situ from α-bromo- and α-chlorohydrazones. Tetrahedron Lett. 83, 153414. doi:10.1016/j.tetlet.2021.153414
Lemos, A. (2009). Addition and cycloaddition reactions of phosphinyl- and phosphonyl-2H-azirines, nitrosoalkenes and azoalkenes. Molecules 14, 4098–4119. doi:10.3390/molecules14104098
Lopes, S. M. M., Cardoso, A. L., Lemos, A., and Pinho E Melo, T. M. V. D. (2018). Recent advances in the chemistry of conjugated nitrosoalkenes and azoalkenes. Chem. Rev. 118, 11324–11352. doi:10.1021/acs.chemrev.8b00375
Luo, K., Yang, W.-C., and Wu, L. (2017). Photoredox catalysis in organophosphorus chemistry. Asian J. Org. Chem. 6, 350–367. doi:10.1002/ajoc.201600512
Minghetti, G., Stoccoro, S., Agostina Cinellu, M., Zucca, A., Manassero, M., and Sansoni, M. (1998). Palladium(II) and platinum(II) derivatives with N,O ligands. J. Chem. Soc. Dalton Trans., 4119–4126. doi:10.1039/a805842c
Nicholson, A., and Wright, C. (1977). Activity of fosazepam, a soluble analogue of diazepam. Br. J. Clin. Pharmacol. 4, 494–496. doi:10.1111/j.1365-2125.1977.tb00773.x
Nyamato, G. S., Alam, M. G., Ojwach, S. O., and Akerman, M. P. (2015). (Pyrazolyl)-(phosphinoyl)pyridine iron(II), cobalt(II) and nickel(II) complexes: Synthesis, characterization and ethylene oligomerization studies. J. Organomet. Chem. 783, 64–72. doi:10.1016/j.jorganchem.2015.02.015
Ogundipe, O. O., Shoberu, A., Xiao, M., and Zou, J.-P. (2022a). Copper-catalyzed radical hydrazono-phosphorylation of alkenes. J. Org. Chem. 87, 15820–15829. doi:10.1021/acs.joc.2c01832
Ogundipe, O. O., Shoberu, A., and Zou, J.-P. (2022b). Copper-catalyzed stereoselective radical phosphono-hydrazonation of alkynes. J. Org. Chem. 87, 14555–14564. doi:10.1021/acs.joc.2c01959
Ovakimyan, M. Z., Barsegyan, S. K., Karapetyan, A. A., Panosyan, G. A., and Indzhikyan, M. G. (2002). Phosphonioethylation of phenylhydrazine and hydroxylamine and selected properties of the resulting derivatives. Russ. Chem. Bull. 51, 1744–1747. doi:10.1023/a:1021372009930
Pailloux, S., Shirima, C. E., Duesler, E. N., Smith, K. A., and Paine, R. T. (2011). Synthesis and lanthanide coordination chemistry of 2-[(phosphinoyl)methyl]-4,5-dihydrooxazole and 2-[(phosphinoyl)methyl]benzoxazole ligands. Polyhedron 30, 2746–2757. doi:10.1016/j.poly.2011.08.012
Palacios, F., Aparicio, D., and De Los Santos, J. M. (1993). A simple and efficient synthesis of αβ-unasaturated hydrazones from functionalized ylides and phosphine oxides. Tetrahedron Lett. 34, 3481–3484. doi:10.1016/s0040-4039(00)79189-1
Palacios, F., Aparicio, D., and De Los Santos, J. M. (1996). An efficient and general strategy for the synthesis of 4-phosphorylated pyrazoles from β-hydrazono phosphine oxides. Tetrahedron 52, 4123–4132. doi:10.1016/s0040-4020(96)00073-7
Palacios, F., Aparicio, D., and De Los Santos, J. M. (1999). An efficient strategy for the regioselective synthesis of 3-phosphorylated-1-aminopyrroles from β-hydrazono phosphine oxides and phosphonates. Tetrahedron 55, 13767–13778. doi:10.1016/s0040-4020(99)00860-1
Palacios, F., Aparicio, D., De Los Santos, J. M., and Vicario, J. (2001). Regioselective alkylation reactions of hydrazones derived from phosphine oxides and phosphonates. Synthesis of phosphorus substituted 1-amino-pyrrolones, pyridinones and pyrroles. Tetrahedron 57, 1961–1972. doi:10.1016/s0040-4020(01)00033-3
Palacios, F., Aparicio, D., and De Los Santos, M. J. (1994). An effective strategy for the preparation of (α,β-unsaturated hydrazones and pyrazole derivatives. Synthetic applications of β-functionalized phosphorus compounds. Tetrahedron 50, 12727–12742. doi:10.1016/s0040-4020(01)89404-7
Palacios, F., Aparicio, D., López, Y., and De Los Santos, J. M. (2004). Addition of amine derivatives to phosphorylated 1,2-diaza-1,3-butadienes. Synthesis of α-aminophosphonates. Tetrahedron Lett. 45, 4345–4348. doi:10.1016/j.tetlet.2004.03.192
Palacios, F., Aparicio, D., López, Y., De Los Santos, J. M., and Alonso, C. (2005b). Cycloaddition reactions of phosphorylated 1,2-diaza-1,3-butadienes with olefins: Regioselective synthesis of pyridazine derivatives. Eur. J. Org. Chem. 2005, 1142–1147. doi:10.1002/ejoc.200400675
Palacios, F., Aparicio, D., López, Y., De Los Santos, J. M., and Ezpeleta, J. M. (2006). Preparation and reactions of 3-phosphinyl-1-aza-1,3-butadienes. Synthesis of phosphorylated pyridine and pyrazole derivatives. Tetrahedron 62, 1095–1101. doi:10.1016/j.tet.2005.11.003
Palacios, F., Aparicio, D., López, Y., and De Los Santos, J. M. (2005a). Synthesis of functionalized α-amino-phosphine oxides and -phosphonates by addition of amines and aminoesters to 4-phosphinyl- and 4-phosphonyl-1,2-diaza-1,3-butadienes. Tetrahedron 61, 2815–2830. doi:10.1016/j.tet.2005.01.081
Rodriguez, J. B., and Gallo-Rodriguez, C. (2019). The role of the phosphorus atom in drug design. ChemMedChem 14, 190–216. doi:10.1002/cmdc.201800693
Schantl, J. G. (1996). Hydrazine-derived heterocycles by conversion of azo-alkenes. Molecules 1, 212–222. doi:10.1007/s007830050040
Semakin, A. N., Kokuev, A. O., Nelyubina, Y. V., Sukhorukov, A. Y., Zhmurov, P. A., Ioffe, S. L., et al. (2016). Construction of bis-tris- and tetrahydrazones by addition of azoalkenes to amines and ammonia. Beilstein J. Org. Chem. 12, 2471–2477. doi:10.3762/bjoc.12.241
Tappe, F. M. J., Trepohl, V. T., and Oestreich, M. (2010). Transition-metal-catalyzed C−P cross-coupling reactions. Synthesis 2010, 3037–3062.
Ushakov, P. Y., Ioffe, S. L., and Sukhorukov, A. Y. (2022). Recent advances in the application of ylide-like species in [4 + 1]-annulation reactions: An updated review. Org. Chem. Front. 9, 5358–5382. doi:10.1039/d2qo00698g
Ushakov, P. Y., Tabolin, A. A., Ioffe, S. L., and Sukhorukov, A. Y. (2019). In situ generated magnesium cyanide as an efficient reagent for nucleophilic cyanation of nitrosoalkenes and parent nitronates. Eur. J. Org. Chem. 2019 1888–1892. doi:10.1002/ejoc.201801761
Vuillermet, F., Dahab, M. A., Abdelhamid, D., Polat, D. E., Gill, M. A., and Beauchemin, A. M. (2023). Synthesis of 1,2,4-triazinones exploiting the reactivity of diazadiene and N-isocyanate intermediates. J. Org. Chem. 88, 2095–2102. doi:10.1021/acs.joc.2c02494
Yu, H., Yang, H., Shi, E., and Tang, W. (2020). Development and clinical application of phosphorus-containing drugs. Med. Drug Discov. 8, 100063. doi:10.1016/j.medidd.2020.100063
Zainkonnikova, I. V., Val'dman, A. V., Kozlovskaia, M. M., and Rzhevskaia, G. F. (1980). Pharmacological characteristics of the tranquilizing action of hydiphen. Farmakol. Toksikol. 43, 334–338.
Zhang, S., Pattacini, R., Jie, S., and Braunstein, P. (2012). A phosphino-oxazoline ligand as a P,N-bridge in palladium/cobalt or P,N-chelate in nickel complexes: Catalytic ethylene oligomerization. Dalton Trans. 41, 379–386. doi:10.1039/c1dt11352f
Zhang, Z., Zhang, L., Chen, Q., Lu, T., and Zhou, Q. (2016). Synthesis of functionalized tetrahydropyridazines via catalyst-free self [4 + 2] cycloaddition of in situ generated 1,2-diaza-1,3-dienes. RSC Adv. 6, 61680–61685. doi:10.1039/c6ra13985j
Keywords: organophosphorus compounds, phosphine oxides, azoalkenes, Michael addition, hydrazones, reactive intermediates, chelating ligands
Citation: Kokuev AO and Sukhorukov AY (2023) Michael addition of P-nucleophiles to azoalkenes provides simple access to phosphine oxides bearing an alkylhydrazone moiety. Front. Chem. 11:1177680. doi: 10.3389/fchem.2023.1177680
Received: 01 March 2023; Accepted: 27 March 2023;
Published: 13 April 2023.
Edited by:
Gaurav Bhargava, I. K. Gujral Punjab Technical University, IndiaReviewed by:
Alejandro Bugarin, Florida Gulf Coast University, United StatesSatish Gandhesiri, Massachusetts Institute of Technology, United States
Gianfranco Favi, University of Urbino Carlo Bo, Italy
Jia-Qi Li, China Agricultural University, China
Ülkü Yilmaz, Malatya Turgut Özal University, Türkiye
Copyright © 2023 Kokuev and Sukhorukov. This is an open-access article distributed under the terms of the Creative Commons Attribution License (CC BY). The use, distribution or reproduction in other forums is permitted, provided the original author(s) and the copyright owner(s) are credited and that the original publication in this journal is cited, in accordance with accepted academic practice. No use, distribution or reproduction is permitted which does not comply with these terms.
*Correspondence: Alexey Yu. Sukhorukov, sukhorukov@ioc.ac.ru