- 1International Advanced Functional Omics Platform, Scientific Experiment Center and Hainan General Hospital, College of Chinese Medicine, Hainan Medical University, Haikou, China
- 2Graduate School, Heilongjiang University of Chinese Medicine, Harbin, China
Natural products derived from herbal medicine are a fruitful source of lead compounds because of their structural diversity and potent bioactivities. However, despite the success of active compounds derived from herbal medicine in drug discovery, some approaches cannot effectively elucidate the overall effect and action mechanism due to their multi-component complexity. Fortunately, mass spectrometry-based metabolomics has been recognized as an effective strategy for revealing the effect and discovering active components, detailed molecular mechanisms, and multiple targets of natural products. Rapid identification of lead compounds and isolation of active components from natural products would facilitate new drug development. In this context, mass spectrometry-based metabolomics has established an integrated pharmacology framework for the discovery of bioactivity-correlated constituents, target identification, and the action mechanism of herbal medicine and natural products. High-throughput functional metabolomics techniques could be used to identify natural product structure, biological activity, efficacy mechanisms, and their mode of action on biological processes, assisting bioactive lead discovery, quality control, and accelerating discovery of novel drugs. These techniques are increasingly being developed in the era of big data and use scientific language to clarify the detailed action mechanism of herbal medicine. In this paper, the analytical characteristics and application fields of several commonly used mass spectrometers are introduced, and the application of mass spectrometry in the metabolomics of traditional Chinese medicines in recent years and its active components as well as mechanism of action are also discussed.
1 Introduction
Exploring the safety and effectiveness of herbal medicine, such as traditional Chinese medicine (TCM), is the key to its modernization and internationalization (Zhang et al., 2011; Ren et al., 2023). It is of great practical and long-term significance to excavate and utilize TCM resources for the development of China’s pharmaceutical industry (Wang X. et al., 2012; Zhang et al., 2012a). Especially in the large epidemic situation of novel coronavirus infection in the past 2 years, the advantages and characteristics of TCM are increasingly emerging. However, like almost all traditional medicine, TCM also faces severe challenges (Zhang et al., 2018a). The lack of scientific and technological means and the lack of modern research restrict the development of TCM worldwide (Zhang et al., 2012b). Therefore, building a communication bridge between TCM and modern medicine has become an important issue in the field of modern biological sciences (Zhang et al., 2010). The advent of metabolomics (Johnson et al., 2016) has gradually made the clear link between TCM and modern medicine. Metabolomics has become more prevalent in TCM research during the past few years, such as in cancer (Lv et al., 2019; Chen et al., 2020; Li et al., 2020), cardiovascular disease (Mcgarrah et al., 2018; Li et al., 2019; Wang X. et al., 2020), senile dementia (Zhang et al., 2018b; Wei et al., 2019; Yi et al., 2020), liver injury (Zhang et al., 2013a; Li et al., 2017; Wei et al., 2020), etc. The application of metabolomics to understand the process of action of TCM is consistent with the overall syndrome differentiation observation of TCM (Wang et al., 2017).
Terminal downstream products of the genome are known as the metabolome (Zamboni et al., 2015), which is composed of metabolites of all low-molecular-weight molecules in cells, tissues, or organisms, and the metabolome possesses a lot of information that is thought to best predict the phenotype. Metabolomics uses modern instrumental analysis methods, combined with pattern recognition, to analyze metabolite changes over time after a biological system is stimulated or disturbed (Schrimpe-Rutledge et al., 2016). In biochemistry, metabolomics is the ultimate end-point measure of biological events that link genotype to phenotype but also captures the effects of nutrition, environmental influences, drug response, etc. The importance of metabolomics in assessing health and treatment impact is also manifested in biological timing and biological probability: Genetics and genomics capture possible events. Proteomics captures ongoing events, and metabolomics captures events that have occurred (Amberg et al., 2017). In addition, due to the intrinsic sensitivity of metabolomics, small modifications in metabolic mechanisms and internal environments of organisms can be discovered to shed light on the mechanisms underlying a variety of physiological situations and abnormal phenomena (Johnson et al., 2016). Metabolomics was originally proposed as an approach to functional genomics (Przybyla and Gilbert, 2022), but its use is far more than that. It contributes greatly when changes in metabolite levels need to be assessed. The main advantage is that metabolomics methods can specifically compare the changes in the metabolic profile of the human body, elucidate the mechanism of action and action changes of TCM, make up for the lack of single components and single targets of TCM research methods, and provide a more scientific and reasonable comprehensive explanation for elucidating TCM research (Zhang et al., 2019a; Qiu et al., 2020; Zhang et al., 2020; Du et al., 2022). The experiment workflow of metabolomics is shown in Figure 1. However, even the most comprehensive approach is unable to provide a clear upper bound on the number of metabolites. The current capacity to detect and quantify metabolites is far from comprehensive enough (Qiu et al., 2023). In mass spectrometry-based metabolomics, there are two general approaches, namely, targeted metabolomics (Liu et al., 2023a) and untargeted metabolomics (Vinayavekhin and Saghatelian, 2010). Because it can provide a methodology for the absolute quantification of identified and prospective biomarkers, targeted metabolomics is an essential component of metabolomics. However, targeted metabolomics can only be used to quantify relatively few metabolites, so there is a deficiency in overall metabolome coverage (Di Minno et al., 2021). By comparison, untargeted metabolomics can measure up to thousands of molecules at a time and can be thousands of molecules of different molecular types. Untargeted metabolomics is extremely advantageous in that it provides a large amount of data (Qiu et al., 2022).
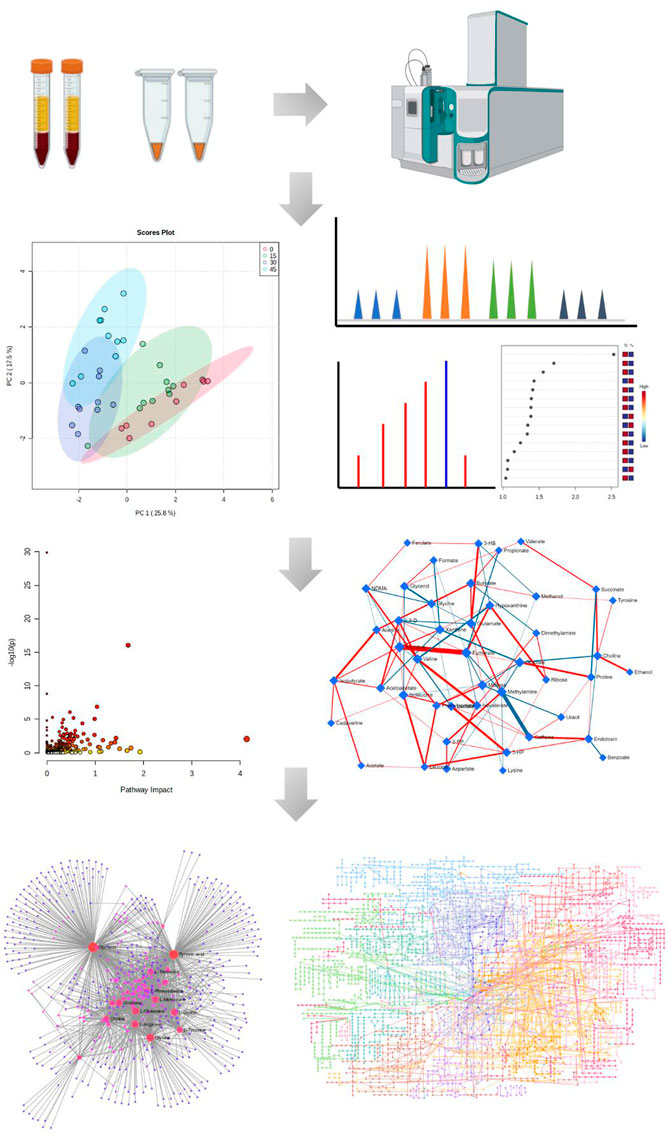
FIGURE 1. The experiment workflow of metabolomics. All images were obtained using the example data provided by the MetaboAnalyst 5.0 and figures created by BioRender.
At present, there are four main analytical instruments to analyze and identify the structure of compounds, which are nuclear magnetic resonance (NMR) spectroscopy, infrared (IR) spectroscopy, ultraviolet-visible (UV) spectroscopy and mass spectrometry (MS). NMR spectroscopy has the characteristics of the same sensitivity to all detected substances, simple sample processing and non-invasiveness, so it can be applied in isotope labeling to trace metabolic pathways (Markley et al., 2017). However, it has the disadvantages of low sensitivity and a narrow detection range. IR spectroscopy can realize the lossless qualitative analysis of almost all organic compounds and most inorganic compounds, and has the characteristics of fast analysis speed (Putzig et al., 1994; Morris et al., 2005). UV spectroscopy is only suitable for the analysis and identification of compounds containing unsaturated bonds and aromatic ring groups, with a small scope of application, low quantitative sensitivity, and usually only reaching the microgram level (Lourenço et al., 2012). MS is suitable for the study of numerous various substances, because it has highly selective analysis, good repeatability, high sensitivity, and a wide linear range (Király et al., 2016). In the research and development of metabolomics, the most common is the combination of various chromatograms and mass spectra, such as liquid chromatography mass spectrometry (LC-MS), gas chromatography mass spectrometry (GC-MS) and Capillary electrophoresis mass spectrometry (CE-MS). The structures of the above mass spectrometers are shown in Figure 2. MS is of great help for the panoramic analysis of the metabolome. With the continuous expansion of the field of metabolomics research and the gradual maturation of MS, the application of MS technology has evolved into an essential instrument for examining the metabolomics of TCM. Depending on the characteristics of the mass analyzer, mass spectra are divided into low-resolution mass spectrum and high-resolution mass spectrum. Low-resolution mass analyzers such as quadrupole analyzer (Q), ion trap (IT), triple quadrupole (QQQ) and quadrupole linear ion trap (Q-Trap) are slightly poor in the qualitative performance of compounds, but can greatly improve the quantitative sensitivity and stability of compounds through ion selection, and the cost is relatively low, which is a reliable method for compound quantification (Mlot et al., 2011).
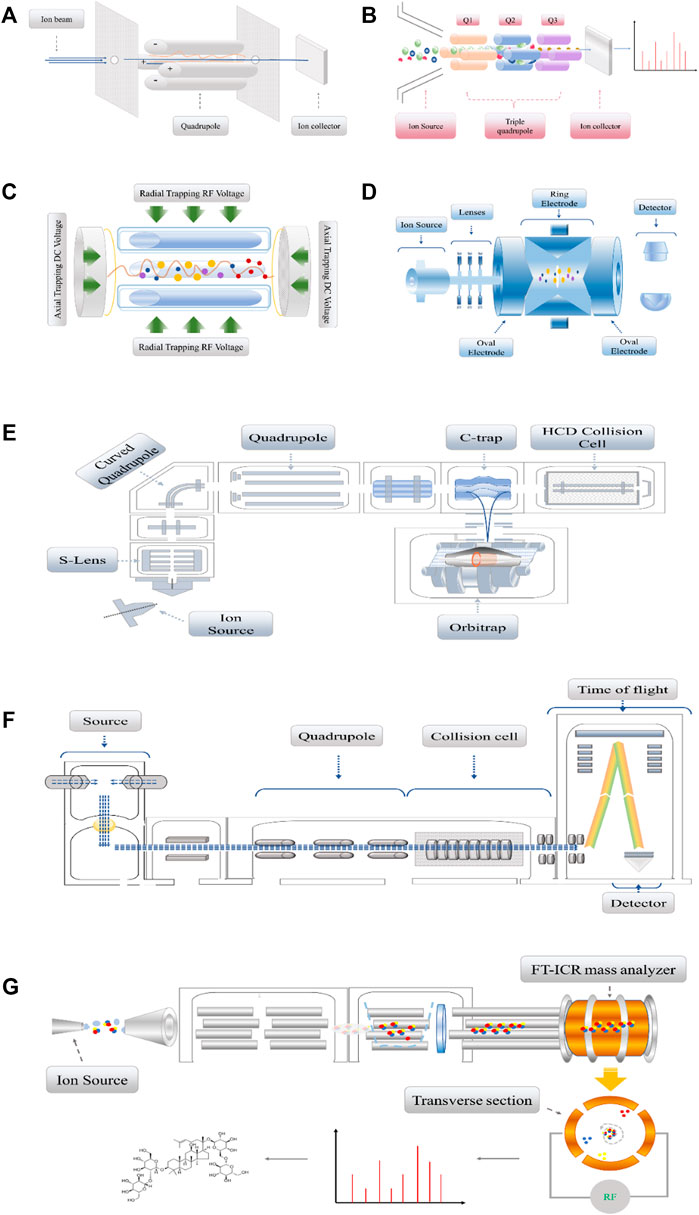
FIGURE 2. (A). The single quadrupole detector consists of four parallel cylindrical or hyperbolic cylindrical electrodes which are equally spaced with the central axis to form two groups of positive and negative electrodes. The DC voltage and RF voltage in the x and y directions are applied to generate a dynamic electric field. (B). Triple quadrupole detector, which by breaking the sample in the ion source to obtain specific daughter ions, daughter ions by Q1, Q2, and Q3 after the selection of the receiver into electrical signals. (C). Linear ion trap, which are designed as three segments in their entirety, apply Radial Trapping RF Voltage and Axial Trapping DC Voltage between electrodes. (D). 3D ion trap diagram is composed of a ring electrode and two oval electrodes. Two oval electrodes have small holes as ion channels. Generally, RF AC voltage or DC voltage is applied on the ring electrode. (E). The Orbitrap detector, after ionization of the analytes in the Ion Source, will sequentially enter the Quadrupole, the C-trap, and the Orbitrap. If debris is also collected, the detected material will also be fragmented in a high-energy collision cell. It works like electrons rotate around the nucleus. (F). The schematic diagram of a substance passing through the Q-TOF mass spectrometer. According to the equation of kinetic energy with mass and velocity: E = mv2, ions with smaller mass-to-charge ratio will obtain higher velocity, shorter flight time, and then convert into mass spectra. (G). The FT-ICR mass spectrometer is a cavity with uniform superconducting magnetic field. Ions move in a circular orbit perpendicular to the magnetic field. When the cyclotron ion beam approaches a pair of traps, the image current signal will be detected on the traps, and the original data is transformed by Fourier transform to form a mass spectrum.
High-resolution mass spectrometers such as Orbitrap, time of flight (TOF) and Fourier transform ion cyclotron resonance (FT-ICR) can attain a mass resolution of nearly 10 million and are suited for correctly determining the precise molecular mass and molecular structure of substances. Although high-resolution mass spectrometry is better at quantifying chemicals, the costs of both its purchase and maintenance are substantial (Marshall and Hendrickson, 2008). The most common mass spectrometry analyzers that are based on metabolomics in the area of TCM are the analytical instruments of Q and TOF and their combinations. Besides, instruments such as Orbitrap and FT-ICR-MS were used. In this paper, the role of mass spectrometry in the metabolomics of TCM is described, and the future development of mass spectrometry in metabolomics is prospected.
2 Mass analyzers
2.1 Triple quadrupole mass spectrometer
The quadrupole mass spectrometer (QMS) is the most classical mass spectrometer (Li et al., 2018) with outstanding quantitative capabilities, and QMS accounts for the vast majority of GC-MS. QMS belongs to dynamic mass spectrometry, which does not require a magnetic field because it only uses a pure electric field to work. Consequently, the instrument’s sensitivity and resolution can indeed be altered by modifying its electrical parameters, allowing it to fulfill various analysis requirements (Ens and Standing, 2005). QMS has the advantages of straightforward structure, light weight, inexpensive maintenance, simple operation, strong quantitative ability and fast scanning speed. However, in accurate mass determination, samples are required to have relatively high purity, and the chemical background of impurities cannot exist, which can cause undetectable interference (Liu et al., 2023b), so it is less used in drug metabolomics. The most common multistage tandem MS system is the tertiary tandem mass spectrometer-QQQ. In three-stage tandem quadrupole mass spectrometry, three quadrupole analyzers are connected in series to form a QQQ sequence, which can also be called TQMS. This allows QMS to have serial functionality and gain much higher chemical specificity than single-stage, while maintaining significant quantitative power.
The most common multistage tandem MS system is a three-stage tandem mass spectrometer--QQQ. In three-level tandem quadrupole mass spectrometry, three quadrupole analyzers are connected in series to form a QQQ sequence, which can also be called TQMS (Yost and Enke, 1978). This allows QMS to have serial functionality and gain much higher chemical specificity than single-stage, while maintaining significant quantitative power. The TQMS workflow diagram is shown in Figure 3. In this way, the qualitative ability of mass spectrometry is strengthened, and it is widely utilized as a confirmatory detection approach for QMS in the detection standards. TQMS includes a wide range of capabilities beyond the standard product ion scanning operation, including SRM, MRM, precursor scan, neutral loss, and others (Rubino, 2020). Therefore, it is particularly suitable for combination with LC and is now widely used in metabolomics for the screening and identification of metabolites and the metabolic transformation of active components of TCM.
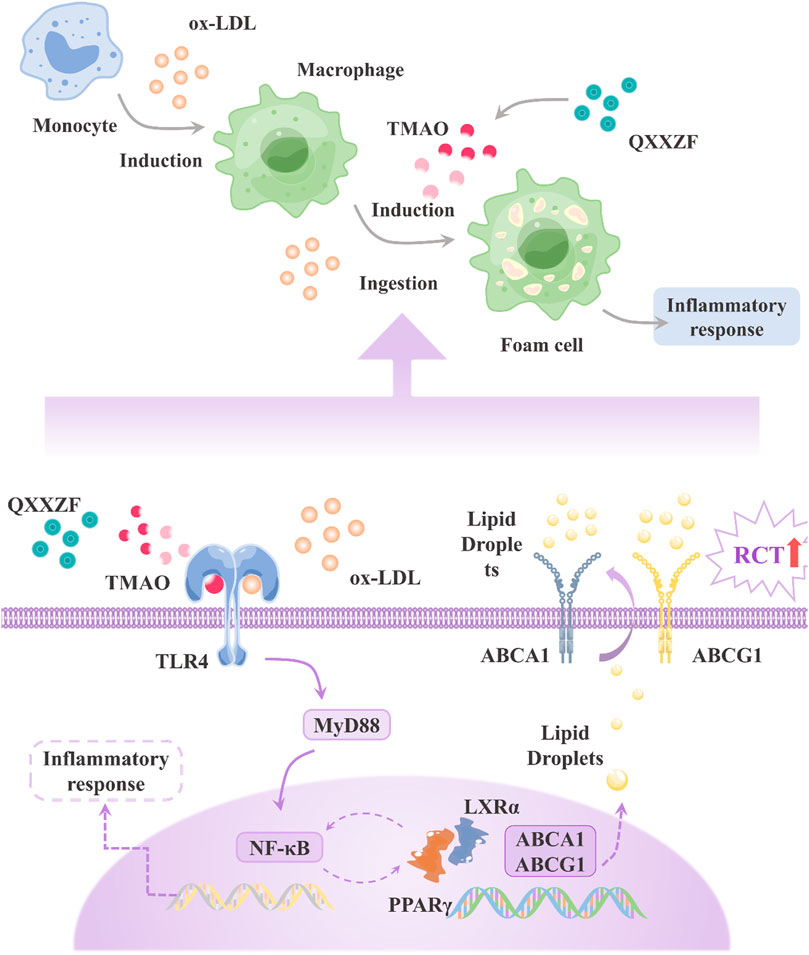
FIGURE 3. Mechanism of Qing-Xue-Xiao-Zhi formula in attenuating atherosclerosis. QXXZF, Qing-Xue-Xiao-Zhi formula; ox-LDL, oxidized low-density lipoprotein; TMAO, trimethylamine N-oxide; RCT, reverse cholesterol transport; ABCA1, ATP-binding cassette A1; ABCG1, ATP-binding cassette G1; PPARγ, peroxisome proliferator-activated receptor γ; LXRα, liver X receptor α; TLR4, Toll-like receptor 4; MyD88, Myeloid Differentiation primary response 88; NF-κB, Nuclear Factor kappa B.
2.2 Quadrupole time-of-flight tandem mass spectrometer
TOF-MS performs well in terms of fast scanning capability, wide mass range, and high resolution (Liang et al., 2015). Q-TOF combines the stability of QSM with TOF functionality by using QMS as a quality filter and TOF-MS as a mass analyzer for coupling. The most common arrangement involves three independent quadrupoles executing the sequential tasks of collision-induced dissociation, parent ion selection, and collision cooling. Then the TOF spectrometer is filled with orthogonal ions for the purpose of obtaining m/z measurements (Ens and Standing, 2005). Q-TOF offers superior resolution spectra as well as MS/MS experiments. At the same time, it has the characteristics of a fast running speed, which creates the conditions for providing comparable sensitivity and a shorter method development time. Q-TOF-MS coupled to liquid chromatography is one of the most commonly used instruments in metabolomics research. In spite of its moderate mass resolution and mass precision, it can be compensated by the very fast scan rate, thus better defining the chromatographic peak (Zhang et al., 2014). However, the flight tube vacuum cavity of Q-TOF MS is bulky and has poor quality stability, and most instruments require real-time correction. It has advantages over QQQ configuration in high performance MS/MS measurements of biomolecules (Zhang et al., 2013b). TOF/MS also has the advantages of a wide mass analysis range and a large data set, making it particularly suitable for use in tandem analysis with ultra-high performance liquid chromatography and tandem analysis with quadrupole mass spectrometry, which can obtain secondary mass spectra of low-abundance chemical components and is suitable for the analysis of complex TCM system components. However, because Q-TOF MS lacks a multi-stage mass spectrometry function, it must be combined with other tandem mass spectrometry when inferring or confirming the structure of TCM components. In the experiment, except for the identification and analysis of TCM components using UHPLC-Q/TOF-MS alone, some components will also be quantified and further elucidated by Q/TOF-MS combined with TQ-MS (Liu et al., 2018a; Lin et al., 2020). At the same time, the in vivo metabolites of TCM can also be identified and characterized by UPLC-QTOF/MS (Mi et al., 2019).
2.3 Ion trap mass spectrometry
IT-MS is a three-dimensional quadrupole field composed of a toroidal electrode with a hyperboloid section and a pair of upper and lower end electrodes (Elschner, 1953). The principle of IT is to store ions in the trap, and then change the electric field to push ions out of the trap according to different mass-to-charge ratios for detection. Due to their uncomplicated construction, compact size, outstanding sensitivity, and relatively low vacuum demands, IT mass analyzers have emerged as the preferred choice for the creation of miniature mass spectrometers (Snyder et al., 2016). Two-dimensional linear ion trap (LIT), which is an improved form of conventional 3D ion trap, is not constrained by radiofrequency potential in the axial direction as is the case with 3D ion trap (Li et al., 2021a). TCM metabolites are emerging as important sources for the discovery of pharmacologically active substances and new drugs. However, the large internal destruction and small amounts of metabolites in biological matrices make it challenging for TCM to identify and discriminate between in vivo components. The advent of IT mass spectrometry has provided solutions to this challenge. However, the disadvantage of IT is that it has a fair resolution and cannot give accurate molecular weight information. Optimization is therefore achieved by coupling with other types of mass analyzers. The quadrupole ion trap (Q-Trap) that adds auxiliary radiofrequency to the QQQ can do selective excitation and provide the functional advantage of multistage serial for the QQQ (Douglas et al., 2005). Ion trap-time-of-flight (IT-TOF-MS) mass spectrometry uses a 3D ion trap as a mass selector and reactor, which combines the multi-stage mass spectrometry ability of the IT with the excellent resolution ability of TOF-MS (Liu, 2012). Linear ion trap-time-of-flight (LIT-TOF-MS) mass spectrometry uses a LIT as a mass selector and reactor, which combines the highly sensitive multistage serial capacity of the LIT with the high-resolution capacity of TOF mass spectrometry (Kosma et al., 2021). Combining the information of IT and TOF mass spectra for structure identification can improve the accuracy of the results and is a common strategy for the identification of TCM components. The elemental composition of the components was deduced by determining the exact molecular weight of TOF. The fragmentation information was then obtained using the multi-stage mass spectrometry function of IT to confirm the structure. LIT-Orbitrap mass spectrometers have the ability to screen and confirm compounds with multiple residues in complex substances (Kosma et al., 2021). LIT-Orbitrap has the dual confirmation function and precise molecular weight of MSn, which can explain the molecular cleavage process, and is also an important tool in the field of metabonomics of TCM.
2.4 Orbitrap
The Orbitrap, the first high-performance mass spectrometer introduced in early 2000, which uses electrostatic fields to capture ions, is another mass analyzer of the Fourier transform series and can usually be used in combination with other mass analyzers (Zubarev and Makarov, 2013). Two exterior electrodes and an electrode in the center of the Orbitrap mass spectrometer serve as both an analyzer and a detector. Ions enter the Orbitrap and are trapped by electrokinetic extrusion, then they oscillate around at the center electrode and among the two external electrodes. When ions are tangentially introduced into the logarithmic DC field between these two electrodes, they begin to oscillate radially around the lead and are eventually ejected at the end of the trap. Different ions oscillate at different frequencies and thus get separated. By determining the oscillation frequency that the ion generates on the external electrode, image flow monitoring can be utilized to acquire the mass spectrum of an ion (Hu et al., 2005; Perry et al., 2008; Makarov and Scigelova, 2010). The first Orbitrap mass analyzer was developed to build a new spectrometer avoiding the disadvantages of existing instruments, such as the complexity and size of the FT-ICR analyzer; the limitations of resolution, dynamic range, and sensitivity of the TOF analyzer; and the limited mass accuracy of the IT analyzer (Eliuk and Makarov, 2015). Orbitrap closes the gap between TOF and FT-ICR quality analyzers. And this instrument is currently more suitable for targeted analysis. However, Orbitrap-MS has a lower acquisition rate than Q-TOF MS, and its resolution interacts with the scan rate, and the resolution decreases significantly at high scan rates. It is difficult to obtain the secondary spectra of low-abundance chemical constituents in TCM under the traditional data-dependent scanning mode (DDA). Therefore, this instrument is currently more suitable for non-target analysis and target analysis of active components of TCM. The Orbitrap-MS, in combination with the LC, provides approximately 500,000 resolution capabilities (Pan et al., 2020; Stettin et al., 2020). In contrast, since its development, Orbitrap-MS integrated with GC has had few applications in the field of metabolomics. It is possible that LC separating has been shown to offer the optimum balance between uncomplicated sample processing and metabolome coverage, making it the preferred method for MS-based metabolomics.
2.5 Fourier transform ion cyclotron resonance mass spectrometer
Ion cyclotron resonance spectrometry (ICR) and contemporary digital technology were combined to create Fourier transform mass spectrometry (FT-MS), also known as Fourier transform ion cyclotron resonance mass spectrometry (FTICR-MS). FTICR-MS has the highest resolving power and is often used as equipment for high-end scientific research, and its unique analytical characteristics make it an essential tool for studies on proteomics, metabolomics and complex mixtures. A common method for micromolecule and proteomic analysis uses FTICR-MS in conjunction with ion preselection and fragmentation equipment and its bonding with reversed-phase LC. The high fidelity of detection linearity and frequency determination are inherent properties of FTICR-MS, which can realize the resolution, mass accuracy and dynamic range (Scigelova et al., 2011; Nikolaev et al., 2016). Since its invention, the fundamental principle of FT-ICR-MS has remained the same: Internally or externally generated ions are radially captured inside the strong electromagnetic field in conjunction with the poor axial electromagnetic current, and image currents are observed from connectedly energized captured ions, culminating in time-domain signals that are imaged and transformed into frequency-domain spectra using FT before being converted into mass spectra. Additionally, cooling circulation is provided for the cryogenic preamplifier within the FT-ICR, increasing sensitivity (Comisarow and Marshall, 1974; Nicolardi et al., 2015; Choi et al., 2012; Qi and O’connor, 2014). FT-ICR-MS has a strong resolving power and can be used in combination with different ionization sources to detect compounds with different polarities. Its ultra-high resolution and mass accuracy can provide accurate elemental composition of compounds, giving it significant potential and advantages in the composition and structure of unknowns, as well as the rapid analysis of complex mixtures. However, the comprehensive grasp of the data machining methods used tends to lag behind that of the instrument, and the majority of data machining algorithms used in FT-ICR have not been thoroughly studied; as a result, expert skills and experience in FT-ICR procedure and data analysis are still essential to achieving the high performance of FT-ICR.
2.6 Ion mobility mass spectrometer
In the traditional MS, the new separation and measurement factor of ion mobility is added, thus constituting the IM-MS system. IM-MS has multidimensional separation, which increases peak capacity, shortens analysis time, separates structural analogues or isomers, and has multiple fragmentation modes and acquisition modes. Experimental data that cannot be provided from MS alone when IM is coupled with MS. Ion-size isomers can be measured by adding an ion migration cell to the MS. Isobaric lines and conformational isomers are separated, and chemical noise is decreased, which are characteristics of IMS. Additionally, ions with a similar charge state and those with structural similarities can be grouped into categories according to the lines where their mass-mobility relationships appear (Kanu et al., 2008). At present, there are four ion mobility separation methods used with MS, including drift time ion mobility spectrometry (DTIMS) (Latif et al., 2021), traveling wave ion mobility spectrometry (TWIMS) (Shliaha et al., 2014), differential migration spectrometry (DMS), also known as field asymmetric waveform ion mobility spectrometry (FAIMS) (Costanzo et al., 2017) and aspiration ion mobility spectrometry (AIMS) (Ratiu et al., 2017). Especially for compounds that are not easily separated chromatographically, IM-MS has great separation advantages. Combined with high resolution mass spectrometry, it has significant advantages in the study of the material basis of TCM. IM has been linked to additional mass spectrometers such as QQQ, IT, and Orbitrap in addition to TOF, which is typically the main mass instrument associated with IM-MS. IM-MS can be readily combined with other cutting-edge technologies, such as capillary electrophoresis and supercritical fluid chromatography. The characteristic parameter ion mean collision cross-sectional area (CCS) determined by IM-MS is an inherent property of compound ions and has significant advantages in compound characterization. However, the CCS database of chemical components of TCM is still blank, and the construction of the CCS database of chemical components of TCM is of great significance for the analysis and research of TCM. The advantages and disadvantages of the above mass spectrometers and their scope of application in the field of TCM research are shown in Supplementary Table S1.
3 Application
3.1 Pharmacodynamic evaluation
Effectiveness is a fundamental property of a drug. Despite the fact that TCM is frequently used in clinical settings, it is still challenging to demonstrate its usefulness through science (Cao et al., 2015; Ma et al., 2016). Further consolidating and improving the efficacy of TCM and greatly enhancing its contribution to medical and health security in China has become a key issue for the sustainable development of TCM. TCM effectiveness evaluation is a necessary step in determining TCM quality markers and pharmacodynamic material basis. However, the clinical effectiveness of TCM is often related to biochemical indicators; in addition, mass spectrometry should be used to prove that the effectiveness of TCM is directly related to clinical indicators from a molecular point of view and at a microscopic level. Combining in vivo and in vitro studies, the effectiveness of TCM was scientifically expressed, and the overall pharmacodynamic action process of TCM was efficiently analyzed under the premise of effectiveness. Tang et al. (2020) used UPLC-Q-TOT-MS to quantitatively analyze the multiple biotransformation products of Xian-Ling-Gu-Bao (XLGB) with rat intestinal bacteria, and this study method successfully described the dynamic contour of thirty-one biotransformation substances of XLGB. He et al. (2022) studied the role of Jigucao capsule (JGCC) in the treatment of Dampness-heat Jaundice syndrome (DHJS) using a classical strategy, which is a research technique of UPLC-QTOF-MS united with pattern identification along with metabolomics applications and digital databases JGCC has been found to call back twenty-five potential biomarkers, including arachidonic acid, L-urobilin, etc. Yao et al. (2022) used UPLC-QTOF-MS to find that Longzuantongbi granules effectively regulated 11 biomarkers associated with lipid metabolism and amino acid metabolism, such as lysophosphatidylcholine, alpha-3-hydroxybutyric acid, alpha-linolenic acid, arachidonic acid, and 12-HETE. Wang et al. (2021) demonstrated that Liu Shen capsule treats respiratory diseases by altering airway microbiota, such as Bacteroidetes and Proteobacteria, based on UPLC-QTOF-MS. Yang et al. (2022) explored the antidiabetic effect of red ginseng on type Ⅱ diabetes with a focus on UPLC-Q Exactive-MS Technology and found that disturbances in regulatory pathways such as D-arginine and D-ornithine metabolic activities, tryptophan bioconversion, taurine, and hypotaurine metabolism were improved after red ginseng intervention. Liu et al. (2022) used UPLC-IT-TOF-MS/MS to qualitatively analyze the metabolites of berberine in human hepatocellular carcinoma cells to elucidate the biodistribution and pharmacokinetic profile of berberine and its metabolites in hepatocytes. Zhao et al. (2022) targeted analysis of 29 kinds of energy metabolites in myocardial tissue based on UPLC-QQ-MS, and verified that Naoxintong Capsules played a role in treating myocardial infarction by affecting part of energy metabolism.
3.2 Quality markers and quality control
In the clinical setting, the effectiveness of TCM is crucial. Contrary to modern medicine, TCM’s multiple active ingredients are affected by a variety of parameters, including the source, provenance, and processing methods of medicinal ingredients (Bai et al., 2018). The non-systematic nature of TCM quality control also seriously affects its efficacy and credibility. The idea of quality-marker (Q-marker), according to TCM features was proposed by Professor Liu ChangXiao, providing new research opportunities for TCM quality management (Liu et al., 2018b; Wu et al., 2018). Q-markers of TCM are substances that are naturally occurring in medicinal materials and components, such as processed goods, extractions, and formulations, or that are created during manufacturing and preparation. The Q-marker approach essentially emphasizes the core meaning of TCM’s quality qualities, which not only indicate security but also represent the effectiveness of treatment and represent a fundamental advance in standard evaluation models and ideas (Yang et al., 2017; Ren et al., 2020). To investigate the potential Q-marker of the Periplocae Cortex, UPLC/Q-TOF MS and network pharmacology approaches were integrated. A total of nine components, such as periplocin, periplogenin, periplocymarin, etc. were selected as Q-markers for the Periplocae Cortex (Li et al., 2021b). Li et al. (2022) analyzed the Q-markers of Wutou decoction (WTD) according to Pearson correlation analysis and UPLC-Q/TOF-MS and identified 12 compounds, including aconitine, ephedrine, quercetin, astragaloside IV, paeoniflorin, and glycyrrhizic acid, etc. as Q-markers of WTD. Zhang et al. (2015) used Carbonized Typhae Pollen (CTP) as an example to establish a discovery strategy for Q-markers of carbonized TCM. Using UPLC-QTOF-MS, six Q-markers of CTP were found to include kaempferol-3-O-neohesperidoside, isorhamnetin-3-O-neohesperidoside, sorhamnetin, naringenin, quercetin, isorhamnetin-3-O-rutinoside and isorhamnetin. Liang et al. (2021) identified eight compounds, including emodin, chrysophanol, magnolol, hesperidin, geniposide, etc. as potential Q-markers for Chaiqin chengqi decoction (CQCQD) based on UPLC-QQQ-MS. Chu et al. (2022) found sweroside, paeoniflorin, liquiritigenin, chlorogenic acid, calycosin-7-glucoside, formononetin and 3-butylephthalide to be Q-markers of the Mailuoshutong pill based on TCM theory and metabolomics techniques. Wang et al. (2023) used UPLC-Q-Exactive Orbitrap MS to identify five active ingredients in Bushen Huoxue Prescription as potential Q markers against diabetic retinopathy, tanshinone IIA, puerarin, ajugol, protocatechuic acid and panaxatriol. Li et al. (2023) used UHPLC-LTQ-Orbitrap MS to analyze and identify the active components in Panax notoginseng and found a new efficacy grading marker alkynol related to Panax notoginseng, which can be used as a new grading quality marker.
The quality marker of TCM is the basis of a modern TCM research system that combines macroscopical and microscopic aspects and includes the study of compatibility law of TCM compounds, the basic study of chemicals and the modern pharmacological study from the molecular point of view. The determination of TCM quality markers is based on the overall view of “pharmacodynamic components,”, that is, those that have clear chemical structure and biological activity. It is conducive to carrying out layer-by-layer analysis of the pharmacodynamic components that play an important role in TCM, and gradually elucidating its pharmacological effects and mechanism of action, which is the premise for traditional Chinese medicine to carry out subsequent research.
3.3 Formula compatibility
TCM formula has always been the most common type of medication in clinical research, and it is a group of drugs that are properly compatible according to the composition principle after selecting the appropriate dosage of drugs after determining the treatment method based on syndrome differentiation examination. TCM formulas containing two or more medicinal herbs tend to obtain better efficacy and fewer side effects than the single herbs (Zhou et al., 2017). Jun-Chen-Zuo-Shi in Chinese (king, minister, assistant and guide) is one of the most typical and significant theories, which vividly defines the diverse roles of various components in TCM (Luan et al., 2020). Herb pairings were crucial to the progress of TCM, and their appearance expanded its range of applications and established the organizing tenets of formulas. The compatibility principles of TCM formulae include considering herbal properties (hot, cold, warm, and cool), herbal taste (pungent, sweet, bitter, acidic, and salty), and pharmacodynamic trends (entering meridians, going up and down, and floating, etc.), as in modern medicine: Synergism and attenuation (Wang S. et al., 2012; Zhang et al., 2015).
Gu et al. (2021) investigated the synergistic effect of Jinlingzi San (JLZS) on Fructus Toosendan (FT) and Rhizoma Corydalis (RC) based on UPLC-QQQ-MS. Synergistic effects of the combination of FT and RC were observed at the pharmacokinetic level, slowing the clearance of tertiary alkaloids and improving their intake and bioavailability to some extent. Sun et al. (2021b) used UFLC-QTRAP-MS targeted metabolomics to elucidate the contribution of Salvia miltiorrhiza and Pueraria lobata in the treatment of acute myocardial ischemia (AMI) in Xin-Ke-Shu and found that the regulatory effect of cardioprotection during AMI was significantly lost in the deficient group lacking Salvia miltiorrhiza and Pueraria lobata, especially the mediation of FFA metabolism. Zhou et al. (2021) investigated the compatibility principle and salt treatment of salt-processed Foeniculi Fructus& Salt-processed Psoraleae Fructus (sFF&sPF) by UHPLC-QTOF-MS. The results showed that sFF&sPF group had the best efficacy in the treatment of diarrhea and polyuria, and salt treatment would briefly affect the correlation between pharmacodynamic components and endogenous metabolites. Chen et al. (2021) used pharmacokinetics and metabolomics, relying on UHPLC-Q Exactive Orbitrap-MS technology, to elucidate the compatibility mechanism of Radix Bupleuri-Radix Paeoniae Alba (RB-RPA). The results showed that the RB-RPA combination could dramatically enhance the bioavailability of 11 drug ingredients and raise neuroprotective and anti-inflammatory activity. Guo et al. (2023) used LC-IT-TOF-MS to compare the component differences between single herb and Zhi-Zi-Chi decoction (ZZCD) compounds, while the solubility of toxic substances and active components was found to affect the attenuation and synergy of the compound by quantitative characteristic compounds, laying the foundation for optimizing the compatibility of ZZCD. Dong et al. (2023) used UFLC—QTRAP-MS to compare the pharmacokinetic differences between Sijunzi decoction and “quewei” Sijunzi compound (one medicinal herb was randomly removed) and found that the compatibility of the four herbs could change the pharmacokinetic properties of the compound and justify oral administration of Sijunzi decoction.
The research on the chemical constituents of Chinese herbal compounds alone is not enough to clarify the compatibility law of Chinese herbal compounds and their basic efficacy and mechanism of action. As a result, using mass spectrometry, it is necessary to analyze the qualitative or quantitative changes in active ingredients of drugs before and after compatibility, study the pharmacokinetic interactions in vivo, that is, the effects of TCM compatibility on in vivo processes such as absorption, distribution, metabolism, and excretion, and finally reflect the synergistic or resistant biological effects in TCM compounds through effect indicators, bioavailability, and target recognition.
3.4 Mechanism of action and target exploration
Although there is only one drug, single herbs contain a variety of active ingredients. While TCM formulas are composed of several herbs and dozens of components, these components are decomposed into countless chemical molecules, so elucidating the mechanism of action of TCM is a very difficult thing (Hao et al., 2017). Metabolomics based on mass spectrometry combined with multivariate statistical analysis can analyze endogenous metabolites, study their species, quantities, and changes under the action of internal and external factors, and perform group index analysis through systematic integration to reflect the dynamic changes of endogenous metabolites in organisms. Li et al. (2021c) studied the mechanism of action of the Qing-Xue-Xiao-Zhi formula (QXXZF) in the treatment of atherosclerosis based on the serum metabolomics of UPLC-Q-TOF-MS. This paper has found that QXXZF can inhibit the development of atherosclerosis by reducing atherosclerotic plaques in the aorta and aortic root, reducing the content of oxidized low-density lipoprotein and trimethylamine N-oxide in serum. Moreover, QXXZF can also promote reverse cholesterol transport by regulating the expression of related genes (PPARγ/LXRα/ABCA1/ABCG1). Wang et al. (2022a) explored the mechanism of action of Qing-Xin-Jie-Yu Granule (QXJYG) in the treatment of atherosclerosis by LC-QTOF-MS. Finally, QXJYG was found to reduce mRNA levels of IL-6 and IL-1β in the aorta while remodeling gut microbiota and associated bile acid levels. Zhang et al. (2022c) investigated the mechanism of Sheng Mai San (SMS) in the treatment of liver injury by UHPLC-QTRAP-MS combined with the pharmacodynamics strategy. The results showed that SMS reconstructs mitochondria to achieve therapeutic effects by altering AST/ALT ratio, regulating glycolysis and TCA cycle. Luo et al. (2022) found that emodin ameliorates ulcerative colitis by modulating the PPARγ signaling pathway. Wang et al. (2022b) evaluated the metabolites of honeysuckle by UPLC-QTOF-MS isolation and found that chlorogenic acid and swertiamarin could regulate the main mediators of inflammation, which in turn affected the phosphatidylinositol-3-kinase-AKT and MAPK pathways. Taking QXXZF as an example, its mechanism of action is shown in Figure 3.
Many studies showed that the main active components of TCM can target one or more specific molecules and thus perform a variety of clinic treatment functions (Shen and Yin, 2021). Exploring TCM from the methodical sentiment and the molecular criterion changes the research paradigm from the previous “one-target and one-drug” model to a novel “multiple targets and multiple constituents” model. Based on the exploration of the drug-disease relationship in the comprehensive pharmacology of TCM, the network of “drug active ingredient-target-disease” was established and enriched, as shown in Figure 4, which was used to analyze the functions of key target genes, metabolic pathways and their “cores”. Construct a comprehensive network framework for TCM-target interactions. Based on the biological effect factors and the regulation mechanism of TCM on the targets and pathways of disease, strengthen the precise positioning and provide accurate treatment of TCM (Li and Zhang, 2013; Wang et al., 2018; Wei et al., 2021). Duan et al. (2021) studied the mechanism of Qing-Re-Ka-Sen granule (QRKSG) in the remedy for nephrotic syndrome (NS) via integrating UPLC-QTRAP-MS, GC-MS, Western blotting and molecular docking techniques. Ultimately, it was discovered that the main targets of the QRKSG for the therapy of NS were AKT1, MTOR, CASP3, and BCL2L1. Guo et al. (2022) found that Qifenggubiao granules achieved immunomodulatory effects by acting on targets such as HSP90AA1, PPARA, PTGS2, CASP3, AKT1, IL6, MAPK3, MAPK1, ESR1, and PPARG. Figure 5 illustrates the target action for QRKSG. Li et al. (2021d) used data mining technology combined with HPLC-Q-Exactive MS/MS to comprehensively analyze the key targets of Hydroxysaffloryellow A in the treatment of traumatic brain injury, as PTGS2, XDH, NOS1 and ACHE. Huang et al. (2022); Zhang et al. (2022d) used UPLC-QTOF-MS to discover potential targets of Chaigui Granule in the treatment of depression and key targets of Rheum officinale Baill. in the treatment of thrombotic diseases, respectively. Wang et al. (2020c) characterized 20 compounds in Zi-shen pill ultrafiltrate using FT-ICR MS and found four active ingredients that inhibit 5-lipoxygenase to treat benign prostatic hyperplasia.
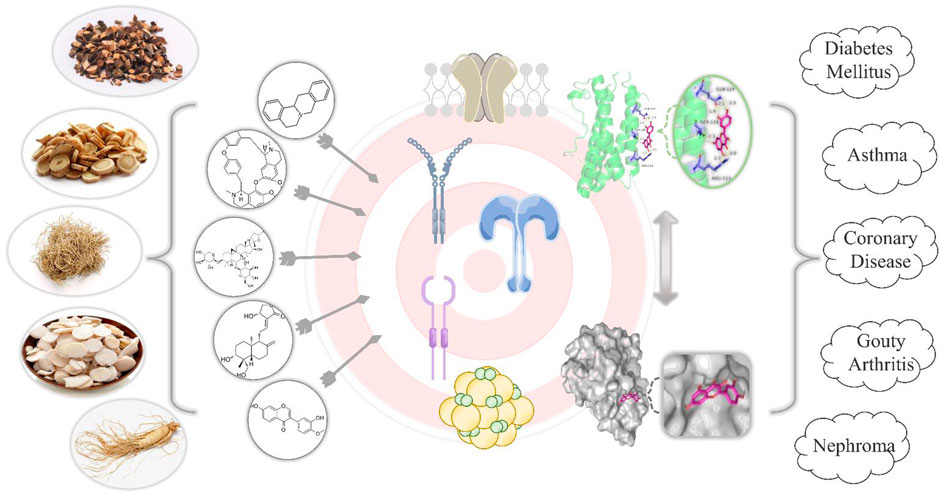
FIGURE 4. ‘Drug-Ingredient-Target-Disease’ Network Diagram. Drug ingredients bind to common targets such as enzymes, ion channels, receptors, or other biomolecules. In this figure, quercetin is used as an example to show its docking diagram with the stick structure and surface structure of IL6 target.
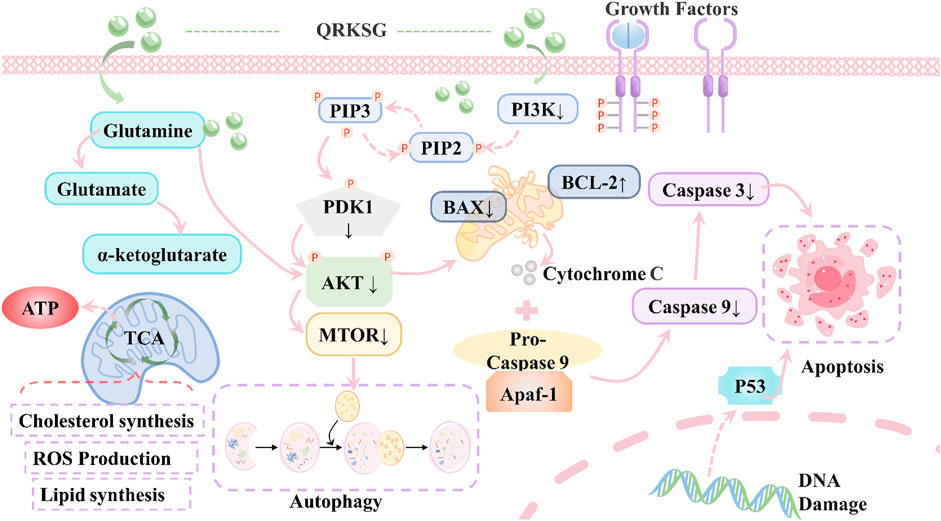
FIGURE 5. Mechanism of Qing-Re-Ka-Sen Granule in Treating Nephrotic Syndrome. QRKSG, Qing-Re-Ka-Sen granule; PI3K, Phosphatidylinositol3-kinase; PIP2, Phosphoinositide 2 kinase; PIP3, Phosphoinositide 3 kinase; PDK1, Pyruvate dehydrogenase kinase 1; AKT, AKT Serine/Threonine Kinase; mTOR, mammalian target of rapamycin; BAX, BCL2-associated X protein; BCL2, Apoptosis Regulator; TCA, tricarboxylic acid cycle; ROS, reactive oxygen species; P53, tumor suppressor gene.
3.5 Pharmacophoric substance discovery
A large number of research studies have shown the utility of metabolomics in discovering the pharmacodynamic material basis of TCM and TCM formulations (Han et al., 2020). The pharmacodynamic material basis of TCM refers to the general term for the chemical components contained in TCM that can express the clinical efficacy of drugs. All the effective components in the Chinese herbal formula were separated and identified by mass spectrometry, and the changes in endogenous metabolites after the compound acted on specific diseases were further studied to elucidate the relevant metabolic pathways. Finally, the pharmacodynamic components that could prevent and treat specific diseases were selected. To find the active constituents of Xiaoxuming decoction (XXMD) in the therapy of ischemic epilepsy, Luo et al. (2019) used UPLC/Q-TOF MS in conjunction with fast separation liquid chromatography-triple quadrupole linear ion trap mass spectrometry (RRLC-QTRAP MS(n)). 48 different substances were found in XXMD by qualitative examination; 33 of these substances underwent quantitative study, and the most prevalent substances were found to be monoterpenes, flavonoids, and cyanophoric glycosides. Huang et al. (2021) compared the metabolic profiles of Uncaria rhynchophylla. (Miq.) Miq. ex Hvail. and Uncaria hirsute Havil. based on UHPLC/Q-Orbitrap-MS. In this study, six potential vasodilator compounds with relaxant effects on mesenteric arteries were selected using multivariate statistical analysis, including corynoxeine, isocorynoxeine, isorhynchophylline, rhynchophylline, and hirudin. Sun et al. (2022) identified glycyrrhizic acid, kaempferol, cinnamaldehyde, catechin daidzein, and caffeic acid as the primary therapeutic efficacy components of Lingguizhugan decoction in the treatment of heart failure using UHPLC-Q-TOF-MS/MS in conjunction with cell experiments and digital storage mining; Kong et al. (2022) used UPLC-QTOF-MS to explore the core active ingredients of Keluoxin (KLX) in the treatment of diabetic retinopathy. The results showed that rhein, astragaloside IV, emodin, chrysophanol and other compounds may be the pharmacodynamic basis of KLX. Liao et al. (2023) isolated and identified the specific binding components of Huanglian Jiedu Decoction to human umbilical vein endothelial cells by a combination of UPLC-Orbitrap-MS and found 13 active components such as geniposide, thapsigin, baicalin, and berberine as pharmacodynamic components that promote angiogenesis. Gao et al. (2023) isolated and identified 45 compounds from the leaves of Amomumvillosum Lour., with the help of HPLC-QTOF-MS technology and further experimented that 14 compounds (one unknown compound and thirteen known compounds) could prevent and treat diseases caused by inflammation and oxidative stress. Liu et al. (2023b) analyzed the components of Danshen decoction by UHPLC-Q-Orbitrap-MS technology, combined with network pharmacology, to elucidate that 35 compounds in its active components are potential pharmacodynamic materials for the treatment of cardiovascular diseases.
4 Future perspectives and conclusion
Metabolomics, as a science that can comprehensively analyze the metabolome, can elucidate the mechanism of action and the change rule of action of TCM by specifically comparing the changes in metabolic profile. It provides a powerful grasp of “multi-component-multi-target” for TCM, and provides a more scientific and reasonable comprehensive explanation for elucidating TCM. As the most important instrument for the analysis and identification of compound structure, MS lays the foundation for the scientific elaboration of the metabolome change contour, pharmacodynamic material basis, mechanism of action, safety, and compatibility principles of TCM. Metabolomics also emerged as a result of the development of MS. With the addition of high-tech and more MS databases, the characterization and identification of metabolites are also more accurate.
With the advancement of science and technology and the passage of time, the types of MS and MS-based analysis technology have been continuously updated, reformed and broken through, and the sensitivity, scope of application, throughput and identification technology of instruments have been greatly improved. The development of MS has contributed to the promotion of metabolomics applications, giving researchers a more comprehensive understanding of small molecule metabolites. Although mass spectrometry has the traits of high resolution, good precision, and maximum throughput, the research progress of metabolomics is still slow due to the complexity of organisms, the huge number of metabolites, and unrepeatable experimental protocols in different laboratories. Different types of MS have their own advantages, and appropriate mass analyzers are selected according to different sample characteristics and requirements. Compared with the acquisition of data, the subsequent processing and mining of data are the bottleneck problems facing metabolomics. Although the existing metabolome database has shortcomings in terms of scale, the open MS database and shared metabolome dataset provide convenience for the qualitative identification of metabolomics. Introducing the quick algorithms of computers into the field of metabolomics is of great help to the annotation as well as the accuracy of the data. The research direction of multi-omics conforms to the root of the overall regulation of organisms, and multidisciplinary cross-fusion can promote the development of metabolomics.
Author contributions
SG, SQ, YC, ZW, YX, and AZ analyzed the data; SG, SQ, YC, and ZW wrote the paper. YX, ST, and AZ revised the paper. All the authors read and approved the final manuscript.
Funding
The authors are grateful for the generous support from the Program of Natural Science Foundation of State (Grant Nos. 81973745, 82104733, 81302905), Talent Lift Engineering Project of China Association of TCM (QNRC2-B06), Natural Science Foundation of Heilongjiang Province (YQ2019H030).
Acknowledgments
We also thank BioRender and MetaboAnalyst for the figure preparation.
Conflict of interest
The authors declare that the research was conducted in the absence of any commercial or financial relationships that could be construed as a potential conflict of interest.
Publisher’s note
All claims expressed in this article are solely those of the authors and do not necessarily represent those of their affiliated organizations, or those of the publisher, the editors and the reviewers. Any product that may be evaluated in this article, or claim that may be made by its manufacturer, is not guaranteed or endorsed by the publisher.
Supplementary material
The Supplementary Material for this article can be found online at: https://www.frontiersin.org/articles/10.3389/fchem.2023.1142287/full#supplementary-material
References
Amberg, A., Riefke, B., Schlotterbeck, G., Ross, A., Senn, H., Dieterle, F., et al. (2017). NMR and MS methods for metabolomics. Methods Mol. Biol. 1641, 229–258. doi:10.1007/978-1-4939-7172-5_13
Bai, G., Zhang, T., Hou, Y., Ding, G., Jiang, M., and Luo, G. (2018). From quality markers to data mining and intelligence assessment: A smart quality-evaluation strategy for traditional Chinese medicine based on quality markers. Phytomedicine 44, 109–116. doi:10.1016/j.phymed.2018.01.017
Cao, H., Zhang, A., Zhang, H., Sun, H., and Wang, X. (2015). The application of metabolomics in traditional Chinese medicine opens up a dialogue between Chinese and Western medicine. Phytotherapy Res. 29 (2), 159–166. doi:10.1002/ptr.5240
Chen, C., Yin, Q., Tian, J., Gao, X., Qin, X., Du, G., et al. (2021). Studies on the changes of pharmacokinetics behaviors of phytochemicals and the influence on endogenous metabolites after the combination of Radix Bupleuri and Radix Paeoniae Alba based on multi-component pharmacokinetics and metabolomics. Front. Pharmacol. 12, 630970. doi:10.3389/fphar.2021.630970
Chen, H., Zhang, F., Zhang, J., Zhang, X., Guo, Y., and Yao, Q. (2020). A holistic view of berberine inhibiting intestinal carcinogenesis in conventional mice based on microbiome-metabolomics analysis. Front. Immunol. 11, 588079. doi:10.3389/fimmu.2020.588079
Choi, M. C., Lee, J. M., Lee, S. G., Choi, S. H., Choi, Y. S., Lee, K. J., et al. (2012). First signal from a broadband cryogenic preamplifier cooled by circulating liquid nitrogen in a 7 T Fourier transform ion cyclotron resonance mass spectrometer. Anal. Chem. 84 (24), 10543–10548. doi:10.1021/ac302049c
Chu, Y. J., Wang, M. L., Wang, X. B., Zhang, X. y., Liu, L. w., Shi, Y. y., et al. (2022). Identifying quality markers of Mailuoshutong pill against thromboangiitis obliterans based on chinmedomics strategy. Phytomedicine 104, 154313. doi:10.1016/j.phymed.2022.154313
Comisarow, M. B., and Marshall, A. G. (1974). Fourier transform ion cyclotron resonance spectroscopy. Chem. Phys. Lett. 25 (2), 282–283. doi:10.1016/0009-2614(74)89137-2
Costanzo, M. T., Boock, J. J., Kemperman, R. H. J., Wei, M. S., Beekman, C. R., and Yost, R. A. (2017). Portable FAIMS: Applications and future perspectives. Int. J. mass Spectrom. 422, 188–196. doi:10.1016/j.ijms.2016.12.007
Di Minno, A., Gelzo, M., Stornaiuolo, M., Ruoppolo, M., and Castaldo, G. (2021). The evolving landscape of untargeted metabolomics. Nutr. metabolism, Cardiovasc. Dis. NMCD 31 (6), 1645–1652. doi:10.1016/j.numecd.2021.01.008
Dong, B., Ma, P., Chen, X., Peng, Y., Peng, C., and Li, X. (2023). Drug-polysaccharide/herb interactions and compatibility rationality of Sijunzi decoction based on comprehensive pharmacokinetic screening for multi-components in rats with spleen deficiency syndrome. J. Ethnopharmacol. 302, 115871. doi:10.1016/j.jep.2022.115871
Douglas, D. J., Frank, A. J., and Mao, D. (2005). Linear ion traps in mass spectrometry. Mass Spectrom. Rev. 24 (1), 1–29. doi:10.1002/mas.20004
Du, W. Z., Zhang, A. H., Ren, J. L., Lyu, K., Tuo, L. Y., and Xu, W. (2022). Study of differential serum metabolites in patients with adenomatous polyps of colon and yang-deficiency constitution based on ultra-performance liquid chromatography-mass spectrometry. Chin. J. Integr. Med. 28 (5), 403–409. doi:10.1007/s11655-019-3181-9
Duan, Y., Zhang, D., Ye, Y., Zheng, S., Huang, P., Zhang, F., et al. (2021). Integrated metabolomics and network pharmacology to establish the action mechanism of qingrekasen granule for treating nephrotic syndrome. Front. Pharmacol. 12, 765563. doi:10.3389/fphar.2021.765563
Eliuk, S., and Makarov, A. (2015). Evolution of orbitrap mass spectrometry instrumentation. Annu. Rev. Anal. Chem. (Palo Alto, Calif) 8, 61–80. doi:10.1146/annurev-anchem-071114-040325
Elschner, H. (1953). Ein neues Massenspektrometer ohne Magnetfeld. Ztschrift Fur Naturforsch. A 8, 448. doi:10.1515/zna-1953-0710
Ens, W., and Standing, K. G. (2005). Hybrid quadrupole/time-of-flight mass spectrometers for analysis of biomolecules. Methods Enzym. 402, 49–78. doi:10.1016/S0076-6879(05)02002-1
Gao, C., Zhu, M., Xu, W., Wang, Y., Xiong, L., Sun, D., et al. (2023). Chemical constituents from the stems and leaves of Amomum villosum Lour. and their anti-inflammatory and antioxidant activities. Bioorg. Chem. 131, 106281. doi:10.1016/j.bioorg.2022.106281
Gu, X., Wu, C., Zhang, M., Wang, X., Liu, Y., and Di, X. (2021). Rapid determination of seven bioactive components in rat plasma by UPLC-MS/MS and its application to pharmacokinetic compatibility study of Jinlingzi San. J. Pharm. Biomed. analysis 198, 198. doi:10.1016/j.jpba.2021.114014
Guo, B., Dong, W., Huo, J., Sun, G., Qin, Z., Liu, X., et al. (2022). Integrated metabolomics and network pharmacology analysis immunomodulatory mechanisms of Qifenggubiao granules. Front. Pharmacol. 13, 828175. doi:10.3389/fphar.2022.828175
Guo, H., Liu, X., Jiang, Y., He, J., Ge, W., Hao, H., et al. (2023). Characterization and quantification of the Chinese medical formula Zhi-Zi-Chi decoction, a systematic strategy for the attenuation and synergy of compatibility mechanism. J. Pharm. Biomed. analysis 223, 115130. doi:10.1016/j.jpba.2022.115130
Han, Y., Sun, H., Zhang, A., Yan, G., and Wang, X. j. (2020). Chinmedomics, a new strategy for evaluating the therapeutic efficacy of herbal medicines. Pharmacol. Ther. 216, 216. doi:10.1016/j.pharmthera.2020.107680
Hao, P., Jiang, F., Cheng, J., Ma, L., Zhang, Y., and Zhao, Y. (2017). Traditional Chinese medicine for cardiovascular disease: Evidence and potential mechanisms. J. Am. Coll. Cardiol. 69 (24), 2952–2966. doi:10.1016/j.jacc.2017.04.041
He, Y., Zhang, M., Li, T., Tan, Z., Zhang, A., Ou, M., et al. (2022). Metabolomics analysis coupled with UPLC/MS on therapeutic effect of Jigucao capsule against dampness-heat Jaundice syndrome. Front. Pharmacol. 13, 822193. doi:10.3389/fphar.2022.822193
Hu, Q., Noll, R. J., Li, H., Makarov, A., Hardman, M., and Graham Cooks, R. (2005). The orbitrap: A new mass spectrometer. J. mass Spectrom. 40 (4), 430–443. doi:10.1002/jms.856
Huang, D., Wang, L., Wu, Y., Qin, X., Du, G., and Zhou, Y. (2022). Metabolomics based on peripheral blood mononuclear cells to dissect the mechanisms of Chaigui granules for treating depression. ACS Omega 7 (10), 8466–8482. doi:10.1021/acsomega.1c06046
Huang, W., Zhang, Z., Niu, L., Hu, X., Teka, T., Han, L., et al. (2021). Rapid discovery of potentially vasodilative compounds from Uncaria by UHPLC/Q-Orbitrap-MS based metabolomics and correlation analysis. J. Pharm. Biomed. analysis 206, 206. doi:10.1016/j.jpba.2021.114384
Johnson, C. H., Ivanisevic, J., and Siuzdak, G. (2016). Metabolomics: Beyond biomarkers and towards mechanisms. Nat. Rev. Mol. Cell. Biol. 17 (7), 451–459. doi:10.1038/nrm.2016.25
Kanu, A. B., Dwivedi, P., Tam, M., Matz, L., and Hill, H. H. (2008). Ion mobility-mass spectrometry. J. mass Spectrom. JMS 43 (1), 1–22. doi:10.1002/jms.1383
Király, M., Dalmadiné Kiss, B., Vékey, K., Antal, I., and Ludanyi, K. (2016). Mass spectrometry: Past and present. Acta Pharm. Hung 86 (1), 3–11.
Kong, L., Sun, Y., Sun, H., Zhang, A. h., Zhang, B., Ge, N., et al. (2022). Chinmedomics strategy for elucidating the pharmacological effects and discovering bioactive compounds from Keluoxin against diabetic retinopathy. Front. Pharmacol. 13, 728256. doi:10.3389/fphar.2022.728256
Kosma, C. I., Koloka, O. L., Albanis, T. A., and Konstantinou, I. K. (2021). Accurate mass screening of pesticide residues in wine by modified QuEChERS and LC-hybrid LTQ/Orbitrap-MS. Food Chem. 2021, 360. doi:10.1016/j.foodchem.2021.130008
Latif, M., Zhang, D., and Gamez, G. (2021). Flowing atmospheric-pressure afterglow drift tube ion mobility spectrometry. Anal. Chim. acta 1163, 338507. doi:10.1016/j.aca.2021.338507
Li, C., Chen, W., Zhang, M., Zhang, C., Cao, B., Dong, B., et al. (2020). Modulatory effects of Xihuang Pill on lung cancer treatment by an integrative approach. Biomed. Pharmacother. = Biomedecine Pharmacother. 130, 110533. doi:10.1016/j.biopha.2020.110533
Li, C., Chu, S., Tan, S., Yin, X., Jiang, Y., Dai, X., et al. (2021). Towards higher sensitivity of mass spectrometry: A perspective from the mass analyzers. Front. Chem. 9, 813359. doi:10.3389/fchem.2021.813359
Li, M., Gao, W., Wang, H., Yang, J., Zhang, Y., Guo, S., et al. (2023). The grading quality markers identification of Panax notoginseng under the guidance of traditional experience using untargeted metabolomics and anti-myocardial ischemia evaluation of zebrafish. Phytomedicine 111, 154674. doi:10.1016/j.phymed.2023.154674
Li, S., and Zhang, B. (2013). Traditional Chinese medicine network pharmacology: Theory, methodology and application. Chin. J. Nat. Med. 11 (2), 110–120. doi:10.3724/sp.j.1009.2013.00110
Li, T., Wu, F., Zhang, A., Dong, H., Ullah, I., Lin, H., et al. (2022). High-throughput chinmedomics strategy discovers the quality markers and mechanisms of Wutou decoction therapeutic for rheumatoid arthritis. Front. Pharmacol. 13, 854087. doi:10.3389/fphar.2022.854087
Li, T., Zhang, W., Hu, E., Sun, Z., Li, P., Yu, Z., et al. (2021). Integrated metabolomics and network pharmacology to reveal the mechanisms of hydroxysafflor yellow A against acute traumatic brain injury. Comput. Struct. Biotechnol. J. 19, 1002–1013. doi:10.1016/j.csbj.2021.01.033
Li, Y. X., Gong, X. H., Liu, M. C., Peng, C., Li, P., and Wang, Y. T. (2017). Investigation of liver injury of polygonum multiflorum thunb. In rats by metabolomics and traditional approaches. Front. Pharmacol. 8, 791. doi:10.3389/fphar.2017.00791
Li, Y., Zhang, L., Ren, P., Yang, Y., Li, S., Qin, X., et al. (2021). Qing-Xue-Xiao-Zhi formula attenuates atherosclerosis by inhibiting macrophage lipid accumulation and inflammatory response via TLR4/MyD88/NF-κB pathway regulation. Phytomedicine 93, 153812. doi:10.1016/j.phymed.2021.153812
Li, Y. F., Qiu, S., Gao, L. J., and Zhang, A. H. (2018). Metabolomic estimation of the diagnosis of hepatocellular carcinoma based on ultrahigh performance liquid chromatography coupled with time-of-flight mass spectrometry. RSC Adv. 8 (17), 9375–9382. doi:10.1039/c7ra13616a
Li, Z. T., Zhang, F. X., Fan, C. L., Ye, M. n., Chen, W., Yao, Z., et al. (2021). Discovery of potential Q-marker of traditional Chinese medicine based on plant metabolomics and network pharmacology: Periplocae Cortex as an example. Phytomedicine 85, 153535. doi:10.1016/j.phymed.2021.153535
Li, Z., Yang, L., Liu, Y., Xu, H., Wang, S., Liu, J., et al. (2019). Anti-inflammatory and antioxidative effects of Dan-Lou tablets in the treatment of coronary heart disease revealed by metabolomics integrated with molecular mechanism studies. J. Ethnopharmacol. 240, 111911. doi:10.1016/j.jep.2019.111911
Liang, G., Yang, J., Liu, T., Wang, S., Wen, Y., Han, C., et al. (2021). A multi-strategy platform for quality control and Q-markers screen of Chaiqin chengqi decoction. Phytomedicine 85, 153525. doi:10.1016/j.phymed.2021.153525
Liang, Q., Wang, C., Li, B., and Zhang, A. H. (2015). Metabolic fingerprinting to understand therapeutic effects and mechanisms of silybin on acute liver damage in rat. Pharmacogn. Mag. 11 (43), 586–593. doi:10.4103/0973-1296.160469
Liao, F., He, D., Liu, C., Vong, C. T., Zhong, Z., and Wang, Y. (2023). Isolation and identification of angiogenesis-promoting components in Huanglian Jiedu decoction using live cell bio-specific extraction. J. Ethnopharmacol. 303, 115961. doi:10.1016/j.jep.2022.115961
Lin, P., Wang, Q., Liu, Y., Qin, Z., Gao, H., Ye, M., et al. (2020). Characterization of chemical profile and quantification of representative components of DanLou tablet, a traditional Chinese medicine prescription, by UHPLC-Q/TOF-MS combined with UHPLC-TQ-MS. J. Pharm. Biomed. analysis 180, 113070. doi:10.1016/j.jpba.2019.113070
Liu, C., Guo, D. A., and Liu, L. (2018). Quality transitivity and traceability system of herbal medicine products based on quality markers. Phytomedicine 44, 247–257. doi:10.1016/j.phymed.2018.03.006
Liu, C., Zong, W. J., Zhang, A. H., Zhang, H. M., Luan, Y. H., Sun, H., et al. (2018). Lipidomic characterisation discovery for coronary heart disease diagnosis based on high-throughput ultra-performance liquid chromatography and mass spectrometry. RSC Adv. 8 (2), 647–654. doi:10.1039/c7ra09353e
Liu, M., Li, Z., Ouyang, Y., Chen, M., Guo, X., Mazhar, M., et al. (2023). Material basis and integrative pharmacology of danshen decoction in the treatment of cardiovascular diseases. Phytomedicine 108, 154503. doi:10.1016/j.phymed.2022.154503
Liu, P., Cai, Y., Qiu, S., Yang, Q., Xie, Y., and Zhang, A. (2023). Critical roles of functional molecule metabolites. Front. Mol. Biosci. 10, 1119588. doi:10.3389/fmolb.2023.1119588
Liu, X., Li, W., Zhang, H., Wang, X., Huang, Y., Li, Y., et al. (2022). Biodistribution and pharmacokinetic profile of berberine and its metabolites in hepatocytes. Phytomedicine 104, 154288. doi:10.1016/j.phymed.2022.154288
Liu, Z. Y. (2012). An introduction to hybrid ion trap/time-of-flight mass spectrometry coupled with liquid chromatography applied to drug metabolism studies. J. mass Spectrom. JMS 47 (12), 1627–1642. doi:10.1002/jms.3126
Lourenço, N. D., Lopes, J. A., Almeida, C. F., Sarraguca, M. C., and Pinheiro, H. M. (2012). Bioreactor monitoring with spectroscopy and chemometrics: A review. Anal. Bioanal. Chem. 404 (4), 1211–1237. doi:10.1007/s00216-012-6073-9
Luan, X., Zhang, L. J., Li, X. Q., Rahman, K., Zhang, H., Chen, H. Z., et al. (2020). Compound-based Chinese medicine formula: From discovery to compatibility mechanism. J. Ethnopharmacol. 254, 112687. doi:10.1016/j.jep.2020.112687
Luo, S., He, J., Huang, S., Wang, X., Su, Y., Li, Y., et al. (2022). Emodin targeting the colonic metabolism via PPARγ alleviates UC by inhibiting facultative anaerobe. Phytomedicine 104, 154106. doi:10.1016/j.phymed.2022.154106
Luo, X., Chen, X., Shen, X., Yang, Z., and Du, G. (2019). Rapid identification and analysis of the active components of traditional Chinese medicine Xiaoxuming decoction for ischemic stroke treatment by integrating UPLC-Q-TOF/MS and RRLC-QTRAP MS(n) method. J. Chromatogr. B, Anal. Technol. Biomed. life Sci. 1124, 313–322. doi:10.1016/j.jchromb.2019.06.023
Lv, J., Jia, Y., Li, J., Kuai, W., Guo, F., Xu, X., et al. (2019). Gegen Qinlian decoction enhances the effect of PD-1 blockade in colorectal cancer with microsatellite stability by remodelling the gut microbiota and the tumour microenvironment. Cell. death Dis. 10 (6), 415. doi:10.1038/s41419-019-1638-6
Ma, Y., Zhou, K., Fan, J., and Sun, S. (2016). Traditional Chinese medicine: Potential approaches from modern dynamical complexity theories. Front. Med. 10 (1), 28–32. doi:10.1007/s11684-016-0434-2
Makarov, A., and Scigelova, M. (2010). Coupling liquid chromatography to Orbitrap mass spectrometry. J. Chromatogr. A 1217 (25), 3938–3945. doi:10.1016/j.chroma.2010.02.022
Markley, J. L., Brüschweiler, R., Edison, A. S., Eghbalnia, H. R., Powers, R., Raftery, D., et al. (2017). The future of NMR-based metabolomics. Curr. Opin. Biotechnol. 43, 34–40. doi:10.1016/j.copbio.2016.08.001
Marshall, A. G., and Hendrickson, C. L. (2008). High-resolution mass spectrometers. Annu. Rev. Anal. Chem. (Palo Alto, Calif) 1, 579–599. doi:10.1146/annurev.anchem.1.031207.112945
Mcgarrah, R. W., Crown, S. B., Zhang, G. F., Shah, S. H., and Newgard, C. B. (2018). Cardiovascular metabolomics. Circulation Res. 122 (9), 1238–1258. doi:10.1161/circresaha.117.311002
Mi, N., Cheng, T., Li, H., Yang, P., Mu, X., Wang, X., et al. (2019). Metabolite profiling of traditional Chinese medicine formula Dan Zhi Tablet: An integrated strategy based on UPLC-QTOF/MS combined with multivariate statistical analysis. J. Pharm. Biomed. analysis 164, 70–85. doi:10.1016/j.jpba.2018.10.024
Mlot, N. J., Tovey, C. A., and Hu, D. L. (2011). Fire ants self-assemble into waterproof rafts to survive floods. Proc. Natl. Acad. Sci. U. S. A. 108 (19), 7669–7673. doi:10.1073/pnas.1016658108
Morris, M. D., Berger, A., and Mahadevan-Jansen, A. (2005). Infrared and Raman spectroscopy. J. Biomed. Opt. 10 (3), 031101. doi:10.1117/1.1906246
Nicolardi, S., Bogdanov, B., Deelder, A. M., Palmblad, M., and van der Burgt, Y. (2015). Developments in FTICR-MS and its potential for body fluid signatures. Int. J. Mol. Sci. 16 (11), 27133–27144. doi:10.3390/ijms161126012
Nikolaev, E. N., Kostyukevich, Y. I., and Vladimirov, G. N. (2016). Fourier transform ion cyclotron resonance (FT ICR) mass spectrometry: Theory and simulations. Mass Spectrom. Rev. 35 (2), 219–258. doi:10.1002/mas.21422
Pan, Q., Zhuo, X., He, C., Zhang, Y., and Shi, Q. (2020). Validation and evaluation of high-resolution orbitrap mass spectrometry on molecular characterization of dissolved organic matter. ACS Omega 5 (10), 5372–5379. doi:10.1021/acsomega.9b04411
Perry, R. H., Cooks, R. G., and Noll, R. J. (2008). Orbitrap mass spectrometry: Instrumentation, ion motion and applications. Mass Spectrom. Rev. 27 (6), 661–699. doi:10.1002/mas.20186
Przybyla, L., and Gilbert, L. A. (2022). A new era in functional genomics screens. Nat. Rev. Genet. 23 (2), 89–103. doi:10.1038/s41576-021-00409-w
Putzig, C. L., Leugers, M. A., Mckelvy, M. L., Mitchell, G. E., Nyquist, R. A., Papenfuss, R. R., et al. (1994). Infrared spectroscopy. Anal. Chem. 66 (12), 26r–66r. doi:10.1021/ac00084a003
Qi, Y., and O'connor, P. B. (2014). Data processing in Fourier transform ion cyclotron resonance mass spectrometry. Mass Spectrom. Rev. 33 (5), 333–352. doi:10.1002/mas.21414
Qiu, S., Cai, Y., Wang, Z., Xie, Y., and Zhang, A. (2022). Decoding functional significance of small molecule metabolites. Biomed. Pharmacother. 158, 114188. doi:10.1016/j.biopha.2022.114188
Qiu, S., Guo, S., Yang, Q., Xie, Y., Tang, S., and Zhang, A. (2023). Innovation in identifying metabolites from complex metabolome—highlights of recent analytical platforms and protocols. Front. Chem. 11, 1129717. doi:10.3389/fchem.2023.1129717
Qiu, S., Zhang, A. H., Guan, Y., Sun, H., Zhang, T. L., Han, Y., et al. (2020). Functional metabolomics using UPLC-Q/TOF-MS combined with ingenuity pathway analysis as a promising strategy for evaluating the efficacy and discovering amino acid metabolism as a potential therapeutic mechanism-related target for geniposide against alcoholic liver disease. RSC Adv. 10 (5), 2677–2690. doi:10.1039/c9ra09305b
Ratiu, I. A., Bocos-Bintintan, V., Patrut, A., Moll, V. H., Turner, M., and Thomas, C. P. (2017). Discrimination of bacteria by rapid sensing their metabolic volatiles using an aspiration-type ion mobility spectrometer (a-IMS) and gas chromatography-mass spectrometry GC-MS. Anal. Chim. acta 982, 209–217. doi:10.1016/j.aca.2017.06.031
Ren, J. L., Zhang, A. H., Kong, L., Han, Y., Yan, G. L., Sun, H., et al. (2020). Analytical strategies for the discovery and validation of quality-markers of traditional Chinese medicine. Phytomedicine 67, 153165. doi:10.1016/j.phymed.2019.153165
Ren, J. L., Yang, L., Qiu, S., Zhang, A. H., and Wang, X. J. (2023). Efficacy evaluation, active ingredients, and multitarget exploration of herbal medicine. Trends Endocrinol. Metab. S1043-2760 (23), 146–157. doi:10.1016/j.tem.2023.01.005
Rubino, F. M. (2020). Center-of-Mass iso-energetic collision-induced decomposition in tandem triple quadrupole mass spectrometry. Mol. (Basel, Switz. 25 (9), 2250. doi:10.3390/molecules25092250
Schrimpe-Rutledge, A. C., Codreanu, S. G., Sherrod, S. D., and Mclean, J. A. (2016). Untargeted metabolomics strategies-challenges and emerging directions. J. Am. Soc. Mass Spectrom. 27 (12), 1897–1905. doi:10.1007/s13361-016-1469-y
Scigelova, M., Hornshaw, M., Giannakopulos, A., and Makarov, A. (2011). Fourier transform mass spectrometry. Mol. Cell. proteomics 10 (7), M111. doi:10.1074/mcp.o111.009431
Shen, X., and Yin, F. (2021). The mechanisms and clinical application of Traditional Chinese Medicine Lianhua-Qingwen capsule. Biomed. Pharmacother. 2021, 142. doi:10.1016/j.biopha.2021.111998
Shliaha, P. V., Jukes-Jones, R., Christoforou, A., Fox, J., Hughes, C., Langridge, J., et al. (2014). Additional precursor purification in isobaric mass tagging experiments by traveling wave ion mobility separation (TWIMS). J. Proteome Res. 13 (7), 3360–3369. doi:10.1021/pr500220g
Snyder, D. T., Pulliam, C. J., Ouyang, Z., and Cooks, R. G. (2016). Miniature and fieldable mass spectrometers: Recent advances. Anal. Chem. 88 (1), 2–29. doi:10.1021/acs.analchem.5b03070
Stettin, D., Poulin, R. X., and Pohnert, G. (2020). Metabolomics benefits from orbitrap GC-MS-comparison of low- and high-resolution GC-MS. Metabolites 10 (4), 143. doi:10.3390/metabo10040143
Sun, L., Jia, H., Yu, M., Yang, Y., Li, J., Tian, D., et al. (2021). Salvia miltiorrhiza and Pueraria lobata, two eminent herbs in Xin-Ke-Shu, ameliorate myocardial ischemia partially by modulating the accumulation of free fatty acids in rats. Phytomedicine Int. J. phytotherapy Phytopharm. 89, 153620. doi:10.1016/j.phymed.2021.153620
Sun, S., Xun, G., Zhang, J., Gao, Y., Ge, J., Liu, F., et al. (2022). An integrated approach for investigating pharmacodynamic material basis of Lingguizhugan Decoction in the treatment of heart failure. J. Ethnopharmacol. 295, 115366. doi:10.1016/j.jep.2022.115366
Tang, X. Y., Gao, M. X., Xiao, H. H., Yun, W. J., Dai, Y., Yao, Z. H., et al. (2020). Simultaneous quantitative analysis of multiple biotransformation products of xian-ling-gu-bao, a traditional Chinese medicine prescription, with rat intestinal microflora by ultra-performance liquid chromatography tandem triple quadrupole mass spectrometry. J. Chromatogr. Sci. 58 (6), 494–503. doi:10.1093/chromsci/bmaa012
Vinayavekhin, N., and Saghatelian, A. (2010). “Untargeted metabolomics,” in Current protocols in molecular biology (Hoboken: John Wiley & Sons). Chapter 30(Unit 30.1.1-24.
Wang, A., Guan, B., Shao, C., Zhao, L., Li, Q., Hao, H., et al. (2022). Qing-Xin-Jie-Yu Granule alleviates atherosclerosis by reshaping gut microbiota and metabolic homeostasis of ApoE-/- mice. Phytomedicine 103, 154220. doi:10.1016/j.phymed.2022.154220
Wang, B., Zhou, R. R., and Tang, S. H. (2018). Study on relevance mining of "core drug action target" in Dictionary of Traditional Chinese Medicine Prescriptions. Zhongguo Zhong Yao Za Zhi 43 (19), 3919–3926. doi:10.19540/j.cnki.cjcmm.20180710.002
Wang, H., Tian, L., Han, Y., Ma, X., Hou, Y., and Bai, G. (2022). Mechanism assay of honeysuckle for heat-clearing based on metabolites and metabolomics. Metabolites 12 (2), 121. doi:10.3390/metabo12020121
Wang, J., Wei, F., Wang, Y., Liu, Q., He, R., Huang, Y., et al. (2023). Exploring the quality markers and mechanism of Bushen Huoxue Prescription in prevention and treatment of diabetic retinopathy based on Chinmedomics strategy. J. Ethnopharmacol. 2023, 306. doi:10.1016/j.jep.2022.116131
Wang, M., Chen, L., Liu, D., Chen, H., Tang, D. D., and Zhao, Y. Y. (2017). Metabolomics highlights pharmacological bioactivity and biochemical mechanism of traditional Chinese medicine. Chemico-biological Interact. 273, 133–141. doi:10.1016/j.cbi.2017.06.011
Wang, X., Zhang, A., and Sun, H. (2012a). Future perspectives of Chinese medical formulae: Chinmedomics as an effector. OMICS 16 (7-8), 414–421. doi:10.1089/omi.2011.0138
Wang, S., Hu, Y., Tan, W., Wu, X., Chen, R., Cao, J., et al. (2012b). Compatibility art of traditional Chinese medicine: From the perspective of herb pairs. J. Ethnopharmacol. 143 (2), 412–423. doi:10.1016/j.jep.2012.07.033
Wang, X., Gao, Y., Tian, Y., Liu, X., Zhang, G., Wang, Q., et al. (2020a). Integrative serum metabolomics and network analysis on mechanisms exploration of Ling-Gui-Zhu-Gan Decoction on doxorubicin-induced heart failure mice. J. Ethnopharmacol. 250, 112397. doi:10.1016/j.jep.2019.112397
Wang, S., Huai, J., Shang, Y., Xie, L., Cao, X., Liao, J., et al. (2020c). Screening for natural inhibitors of 5-lipoxygenase from Zi-shen pill extract by affinity ultrafiltration coupled with ultra performance liquid chromatography-mass spectrometry. J. Ethnopharmacol. 254, 112733. doi:10.1016/j.jep.2020.112733
Wang, X., Xu, X., Chen, Y., Li, Z., Zhang, M., Zhao, C., et al. (2021). Liu shen capsule alters airway microbiota composition and metabolite profiles in healthy humans. Front. Pharmacol. 12, 824180. doi:10.3389/fphar.2021.824180
Wei, M., Liu, Z., Liu, Y., Hu, M., Yue, K., Liu, T., et al. (2019). Urinary and plasmatic metabolomics strategy to explore the holistic mechanism of lignans in S. chinensis in treating Alzheimer's disease using UPLC-Q-TOF-MS. Food & Funct. 10 (9), 5656–5668. doi:10.1039/c9fo00677j
Wei, R. L., Wang, L. X., and Xie, Y. M. (2021). [Cognition of traditional Chinese medicine properties and thinking of clinical rational use of drug]. Zhongguo Zhong Yao Za Zhi 46 (21), 5462–5467. doi:10.19540/j.cnki.cjcmm.20210330.501
Wei, S., Ma, X., Niu, M., Wang, R., Yang, T., Wang, D., et al. (2020). Mechanism of paeoniflorin in the treatment of bile duct ligation-induced cholestatic liver injury using integrated metabolomics and network pharmacology. Front. Pharmacol. 11, 586806. doi:10.3389/fphar.2020.586806
Wu, X., Zhang, H., Fan, S., Zhang, Y., Yang, Z., Fan, S., et al. (2018). Quality markers based on biological activity: A new strategy for the quality control of traditional Chinese medicine. Phytomedicine 44, 103–108. doi:10.1016/j.phymed.2018.01.016
Yang, W., Zhang, Y., Wu, W., Huang, L., Guo, D., and Liu, C. (2017). Approaches to establish Q-markers for the quality standards of traditional Chinese medicines. Acta Pharm. Sin. B 7 (4), 439–446. doi:10.1016/j.apsb.2017.04.012
Yang, Z., Dan, W., Li, Y., Zhou, X., Liu, T., Shi, C., et al. (2022). Untargeted metabolomics analysis of the anti-diabetic effect of Red ginseng extract in Type 2 diabetes Mellitus rats based on UHPLC-MS/MS. Biomed. Pharmacother. 146, 112495. doi:10.1016/j.biopha.2021.112495
Yao, L., Cheng, S., Yang, J., Xiang, F., Zhou, Z., Zhang, Q., et al. (2022). Metabolomics reveals the intervention effect of Zhuang medicine Longzuantongbi granules on a collagen-induced arthritis rat model by using UPLC-MS/MS. J. Ethnopharmacol. 2022, 294. doi:10.1016/j.jep.2022.115325
Yi, M., Zhang, C., Zhang, Z., Yi, P., Xu, P., Huang, J., et al. (2020). Integrated metabolomic and lipidomic analysis reveals the neuroprotective mechanisms of bushen tiansui formula in an aβ1-42-induced rat model of alzheimer's disease. Oxidative Med. Cell. Longev. 2020, 1–18. doi:10.1155/2020/5243453
Yost, R. A., and Enke, C. (1978). Selected ion fragmentation with a tandem quadrupole mass spectrometer. J. Am. Chem. Soc. 100, 2274–2275. doi:10.1021/ja00475a072
Zhang, A., Sun, H., Wang, X., Jiao, G., Yuan, Y., and Sun, W. (2012a). Simultaneous in vivo RP-HPLC-DAD quantification of multiple-component and drug-drug interaction by pharmacokinetics, using 6,7-dimethylesculetin, geniposide and rhein as examples. Biomed. Chromatogr. 26 (7), 844–850. doi:10.1002/bmc.1739
Zhang, A., Sun, H., and Wang, X. (2012b). Recent highlights of metabolomics for traditional Chinese medicine. Pharmazie 67 (8), 667–675.
Zhang, A., Sun, H., Dou, S., Sun, W., Wu, X., Wang, P., et al. (2013a). Metabolomics study on the hepatoprotective effect of scoparone using ultra-performance liquid chromatography/electrospray ionization quadruple time-of-flight mass spectrometry. Analyst 138 (1), 353–361. doi:10.1039/c2an36382h
Zhang, A. H., Sun, H., Qiu, S., and Wang, X. J. (2013b). Recent highlights of metabolomics in Chinese medicine syndrome research. Evid. Based Complement. Altern. Med. 2013, 1–4. doi:10.1155/2013/402159
Zamboni, N., Saghatelian, A., and Patti, G. J. (2015). Defining the metabolome: Size, flux, and regulation. Mol. Cell. 58 (4), 699–706. doi:10.1016/j.molcel.2015.04.021
Zhang, A., Sun, H., and Wang, X. (2018a). Mass spectrometry-driven drug discovery for development of herbal medicine. Mass Spectrom. Rev. 37 (3), 307–320. doi:10.1002/mas.21529
Zhang, A. H., Yu, J. B., Sun, H., Kong, L., Wang, X. Q., Zhang, Q. Y., et al. (2018b). Identifying quality-markers from Shengmai San protects against transgenic mouse model of Alzheimer's disease using chinmedomics approach. Phytomedicine Int. J. phytotherapy Phytopharm. 45, 84–92. doi:10.1016/j.phymed.2018.04.004
Zhang, A., Ma, Z., Sun, H., Zhang, Y., Liu, J. H., Wu, F. F., et al. (2019a). High-throughput metabolomics evaluate the efficacy of total lignans from acanthophanax senticosus stem against ovariectomized osteoporosis rat. Front. Pharmacol. 10, 553. doi:10.3389/fphar.2019.00553
Zhang, A., Sun, H., Wang, Z., Sun, W., Wang, P., and Wang, X. (2010). Metabolomics: Towards understanding traditional Chinese medicine. Planta medica. 76 (17), 2026–2035. doi:10.1055/s-0030-1250542
Zhang, A., Sun, H., Yuan, Y., Sun, W., Jiao, G., and Wang, X. (2011). An in vivo analysis of the therapeutic and synergistic properties of Chinese medicinal formula Yin-Chen-Hao-Tang based on its active constituents. Fitoterapia 82 (8), 1160–1168. doi:10.1016/j.fitote.2011.07.014
Zhang, A. H., Ma, Z. M., Kong, L., Gao, H. L., Sun, H., Wang, X. Q., et al. (2020). High-throughput lipidomics analysis to discover lipid biomarkers and profiles as potential targets for evaluating efficacy of Kai-Xin-San against APP/PS1 transgenic mice based on UPLC-Q/TOF-MS. Biomed. Chromatogr. 34 (2), e4724. doi:10.1002/bmc.4724
Zhang, A. H., Sun, H., Yan, G. L., Yuan, Y., Han, Y., and Wang, X. J. (2014). Metabolomics study of type 2 diabetes using ultra-performance LC-ESI/quadrupole-TOF high-definition MS coupled with pattern recognition methods. J. Physiol. Biochem. 70 (1), 117–128. doi:10.1007/s13105-013-0286-z
Zhang, J. H., Zhu, Y., Fan, X. H., and Zhang, B. l. (2015). Efficacy-oriented compatibility for component-based Chinese medicine. Acta Pharmacol. Sin. 36 (6), 654–658. doi:10.1038/aps.2015.8
Zhang, X., Jia, Y., Yuan, Z., Wen, Y., Zhang, Y., Ren, J., et al. (2022c). Sheng Mai San ameliorated heat stress-induced liver injury via regulating energy metabolism and AMPK/Drp1-dependent autophagy process. Phytomedicine 97, 153920. doi:10.1016/j.phymed.2021.153920
Zhang, Y. R., Liu, Y. R., Tang, Z. S., Song, Z. X., Zhang, J. W., Chang, B. J., et al. (2022d). Rheum officinale Baill. Treats zebrafish embryo thrombosis by regulating NOS3 expression in the arginine biosynthesis pathway. Phytomedicine Int. J. phytotherapy Phytopharm. 99, 153967. doi:10.1016/j.phymed.2022.153967
Zhao, J., Ouyang, Y., Wang, H., Lai, H., Hu, S., Tang, L., et al. (2022). An energy metabolism study on the efficacy of Naoxintong capsules against myocardial infarction in a rat model. Oxidative Med. Cell. Longev. 2022, 1–14. doi:10.1155/2022/3712500
Zhou, M., Hong, Y., Lin, X., Shen, L., and Feng, Y. (2017). Recent pharmaceutical evidence on the compatibility rationality of traditional Chinese medicine. J. Ethnopharmacol. 206, 363–375. doi:10.1016/j.jep.2017.06.007
Zhou, N., Li, Z., Wang, J. J., Wu, Q. T., Li, K., Zheng, X. K., et al. (2021). Correlation analysis between extracts and endogenous metabolites to characterise the influence of salt-processing on compatibility mechanism of 'Psoraleae Fructus & Foeniculi Fructus. J. Ethnopharmacol. 2021, 270. doi:10.1016/j.jep.2021.113782
Keywords: Metabolomic, mass spectrometry, herbal medicine, target, biomarker, mechanism
Citation: Guo S, Qiu S, Cai Y, Wang Z, Yang Q, Tang S, Xie Y and Zhang A (2023) Mass spectrometry-based metabolomics for discovering active ingredients and exploring action mechanism of herbal medicine. Front. Chem. 11:1142287. doi: 10.3389/fchem.2023.1142287
Received: 11 January 2023; Accepted: 20 March 2023;
Published: 31 March 2023.
Edited by:
Jiaqi Fu, Purdue University, United StatesReviewed by:
Kunming Qin, Jiangsu Ocean University, ChinaXiaowei Shi, Hebei Medical University, China
Copyright © 2023 Guo, Qiu, Cai, Wang, Yang, Tang, Xie and Zhang. This is an open-access article distributed under the terms of the Creative Commons Attribution License (CC BY). The use, distribution or reproduction in other forums is permitted, provided the original author(s) and the copyright owner(s) are credited and that the original publication in this journal is cited, in accordance with accepted academic practice. No use, distribution or reproduction is permitted which does not comply with these terms.
*Correspondence: Shi Qiu, cWl1c2hpaG55eEAxNjMuY29t; Songqi Tang, dGFuZ3NvbmdxaUBoYWlubWMuZWR1LmNuJiN4MDIwMGE7; Yiqiang Xie, eGlleWlxaWFuZ0BoYWlubWMuZWR1LmNuJiN4MDIwMGE7; Aihua Zhang, YWlodWF0Y21AMTYzLmNvbQ==