- 1State Key Laboratory of Elemento-Organic Chemistry, College of Chemistry, Nankai University, Tianjin, China
- 2School of Physics and Electronic Information, Anhui Normal University, Wuhu, China
- 3Life and Health Intelligent Research Institute, Tianjin University of Technology, Tianjin, China
- 4Tianjin Key Laboratory of Biosensing and Molecular Recognition, College of Chemistry, Nankai University, Tianjin, China
- 5Frontiers Science Center for New Organic Matter, College of Chemistry, Nankai University, Tianjin, China
Although mass spectrometry (MS) has its unique advantages in speed, specificity and sensitivity, its application in quantitative chiral analysis aimed to determine the proportions of multiple chiral isomers is still a challenge. Herein, we present an artificial neural network (ANN) based approach for quantitatively analyzing multiple chiral isomers from their ultraviolet photodissociation mass spectra. Tripeptide of GYG and iodo-L-tyrosine have been applied as chiral references to fulfill the relative quantitative analysis of four chiral isomers of two dipeptides of L/DHisL/DAla and L/DAspL/DPhe, respectively. The results show that the network can be well-trained with limited sets, and have a good performance in testing sets. This study shows the potential of the new method in rapid quantitative chiral analysis aimed at practical applications, with much room for improvement in the near future, including selecting better chiral references and improving machine learning methods.
1 Introduction
Chirality exists in various types of organic and biological molecules, including amino acids, carbohydrates, DNAs and proteins. The chiral isomers may have quite different interactions with their surroundings in living systems, resulting in different pharmacological activities (Nguyen et al., 2006). At the early ages, it was still believed that D-amino acids only occurred in microorganisms and some peptides. Due to the development of analytical methods in the past decades, the presence of D-amino acids in higher organisms, including human beings, is proven (Genchi, 2017). Thus, chiral analysis is very important for both fundamental and applied research. Various methods have been developed and applied for effective chiral analysis, including circular dichroism (CD) spectroscopy, nuclear magnetic resonance (NMR), and chromatographic methods, such as liquid chromatography (LC) or gas chromatography (GC) (Nguyen et al., 2006; Ward and Baker, 2008; Lanucara et al., 2014; Wenzel, 2018; Hu et al., 2020). It should be mentioned that the selection of suitable reagent or ligand to fulfill chiral analysis or to assign the absolute configuration of particular compounds is very important in such experiments (Müller et al., 2008; Scaramuzzo et al., 2013).
Since the ions of enantiomers (or chiral isomers) have the same m/z, mass spectrometry (MS) was considered as a blind method for chiral analysis in the early years. However, the rapid development of mass spectrometry methods, especially in the field of soft ionization, has undergone great changes (Tao and Cooks, 2003; Wu et al., 2012; Awad and El-Aneed, 2013; Yu and Yao, 2017; Han and Yao, 2020; Shi et al., 2020). In recent years, MS based chiral analysis methods have attracted widespread attention due to its unique advantages in speed, specificity and sensitivity. Differentiation of chiral isomers can be achieved by comparing the formation, dissociation or reaction behavior of the diastereomers in the gas phase, or their mobilities when combined with the technique of ion mobility (Tao and Cooks, 2003; Wu et al., 2012; Awad and El-Aneed, 2013; Lanucara et al., 2014; Yu and Yao, 2017; Han and Yao, 2020; Hu et al., 2020; Shi et al., 2020). The method of infrared multiple photon dissociation (IRMPD) and ultraviolet photodissociation (UVPD) spectroscopic methods, have also applied to the field and have shown their advantages in providing spectral and structural information of corresponding chiral complexes (Filippi et al., 2012; Liao et al., 2013; Fujihara et al., 2016; Lee et al., 2017; Ren et al., 2017; Fujihara and Okawa, 2018; Lee et al., 2018; Ma et al., 2018; Shi et al., 2019; Shi et al., 2020; Sun et al., 2020). Among these MS-based methods, UVPD has been demonstrated to be able to yield abundant radicals in fragmentation processes, thus provides a unique means for generating novel dissociation pathways in tandem mass spectrometry (Ly and Julian, 2009).
Although progresses have been achieved in qualitative chiral analysis, quantitative analysis aimed to determine the value of enantiomeric excess (ee) is more difficult and complicated than qualitative analysis aimed to recognition (Fujihara et al., 2017). On the other hand, most of the MS-based chiral analysis studies still focused on enantiomers with one chiral center. For the complicated compounds with multiple chiral centers, the recognition of the multiple isomers including both enantiomers and diastereomers in a single experiment will be more difficult, not to mention the quantitative analysis of these compounds. Among the D-amino acids, D-Ala is an unusual endogenous amino acid present in invertebrates and vertebrates, and its function in the mammalian nervous and endocrine systems is significant (Lee et al., 2020). And D-Asp is one of the major regulators of adult neurogenesis and plays an important role in the development of endocrine function (Genchi, 2017). In this paper, two dipeptides including L/D-Ala and L/D-Asp were selected here as the sample molecules to establish a new analytical method. Herein, we show that the quantitative analysis of four chiral isomers of dipeptides can be achieved by combining the methods of UVPD MS performed with a tunable UV laser and artificial neural network (ANN).
2 Experimental
The experimental setup has been described in our previous paper (Shi et al., 2019; Zhang et al., 2020). Briefly, a 7.0 T Fourier transform ion cyclotron resonance (FT ICR) mass spectrometer (IonSpec, Varian, Inc., Lake Forest, CA) was applied here, combined with one commercial UV-Vis tunable laser (NT-342C, EKSPLA, Lithuania). The laser was operated in normal mode in the range of 210–300 nm, with a typical output energy of 1–2 mJ/pulse in this experiment. The laser was introduced coaxially to the ICR cell through a CaF2 window and the irradiation time was set as 4s that was controlled though a mechanical shutter controller (SSH, Sigma-Koki, Tokyo, Japan).
Chiral dipeptides of L/DHisL/DAla and L/DAspL/DPhe were ordered from Ontores and Shanghai Apeptide companies, respectively. And the chiral reference molecules of tripeptide GYG and 3-Iodo-L-tyrosine were brought from GL Bopchem (Shanghai) and DAMAS-BETA, respectively. Each sample was prepared in deionized water with a concentration of 10 mmol/L and then diluted to 1 mmol/L aqueous solutions with 49% methanol and 2% acetic acid before mixing. The solution of chiral reference was then mixed with the solution of dipeptide in a volume ratio of 1:1, and the later was prepared by premixing the 4 kinds of chiral isomers as designed. In the MS experiments, a Zspray electrospray ionization (ESI) source was applied with a probe biased at 3.6 kV. The complex ions were selected by the method of stored waveform inverse Fourier transform (SWFIT), followed by the UV irradiation (Cody et al., 1987; Shi et al., 2019). After the UVPD process, the product ions were detected and the mass spectrum was recorded. The wavelengths of the UV laser can be readily selected and changed with the commercial program provided by the laser manufactory.
For the applied artificial neural network, a three-layered backpropagation (BP) network was designed with MATLAB mathematical software, in which sigmoid transfer function at hidden layer was used (Figure 1). The input variables were peak intensities of ions with specified m/z’s under different wavenumbers. And the corresponding molar ratios of the four chiral isomers were chosen as the target. The data sets were divided into training and test subsets, respectively.
3 Results and discussion
Considering the success of peptide as the ligand applied in the chiral analysis of short peptides (Tao, et al., 2001), the tripeptide of GYG (Mr) is selected as a reference molecule for the four analytes of L/DHisL/DAla. The complex ions of [HisAla + Mr]H+ were generated by ESI from corresponding mixed solutions. The target ions were then selected and trapped in the FT ICR cell, followed by irradiation of UV laser at suitable wavelengths. As an example, the 225 nm UVPD mass spectra of the four complex ions are shown in Figure 2. The UVPD mass spectra are readily to be read. For all cases, three fragment ions can be found: the radical cations of [HisAla + Mr]+• formed by the loss of hydrogen atom, the protonated dipeptide ions of [HisAla]+ and their dehydrated forms. The relative intensities of the three fragment ions are kinds of different from each other, but can be hardly direct applied for chiral analysis for the four chiral compounds. The difference among them can be made clearer if three-dimensional dissociation mass spectra obtained under different UV wavelengths were applied (Figure 3).
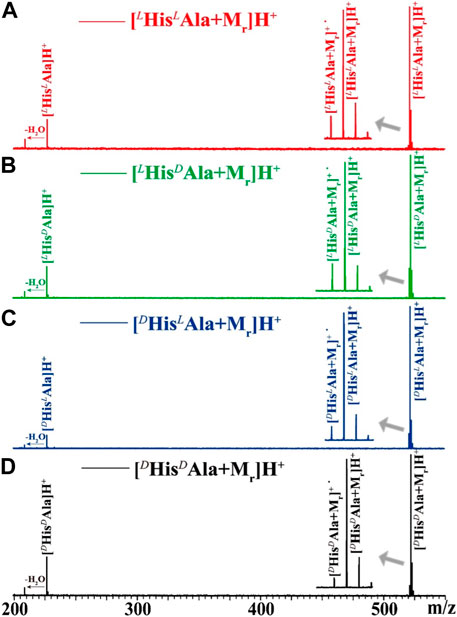
FIGURE 2. 225 nm UV photodissociation mass spectra of (A) [LHisLAla + Mr]H+, (B) [LHisDAla + Mr]H+, (C) [DHisLAla + Mr]H+, and (D) [DHisDAla + Mr]H+, in which the Mr indicates the reference molecule of tripeptide GYG. Details of the mass spectra near the precursor ions are further shown in the insets.
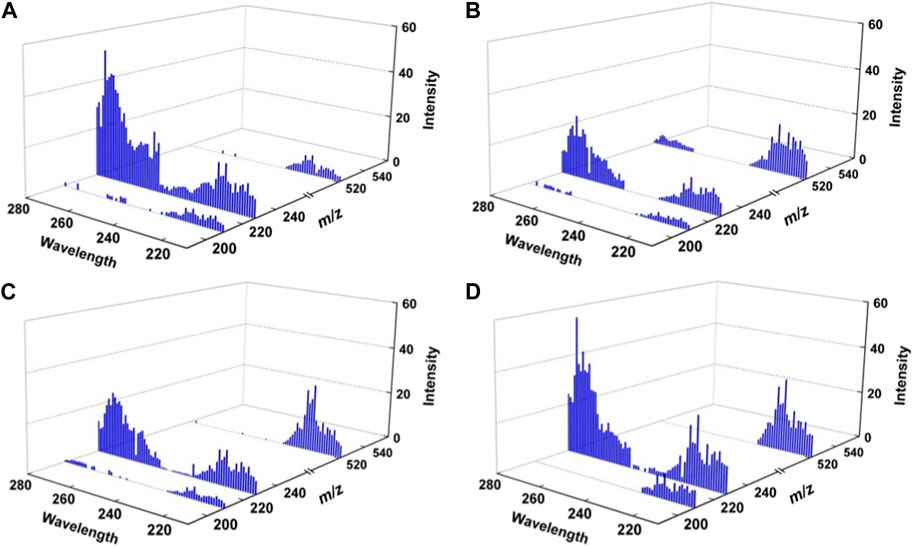
FIGURE 3. Three-dimensional UV photodissociation mass spectra of (A) [LHisLAla + Mr]H+, (B) [LHisDAla + Mr]H+, (C) [DHisLAla + Mr]H+, and (D) [DHisDAla + Mr]H+.
Although the difference, it is still too difficult to apply the method to quantitative analysis of these chiral isomers directly since the quantitative evaluation of the difference is difficult. In order to overcome the obstacle, the method of machine learning based on artificial neural network (ANN) was introduced here. To fulfill it, four wavelengths of 210, 213, 225 and 230 nm were selected here. A total of 19 mixed solutions with different proportions of the four chiral isomers were prepared and mixed with the reference molecule. The generated complex ions were further isolated and irradiated under the four different UV wavelengths. All the UVPD mass spectra were recorded. The data from the first 15 samples were applied as the training sets for the network, followed by training under supervision. Figure 4 shows the training results of the artificial neural network applied here (Figure 1). The error between the training output value and the real value is small, which indicates that the artificial neural network has been trained. To prevent possible over-fitting in the training process, two methods were applied here: 1) to design the training sets carefully and to ensure its proper population, 2) to adopt the early stopping method (Deng et al., 2015). After the training, the last four data sets are tested. And the results are shown in Figure 5. The predicted values are in good agreement with the real ones, and the standard deviations are less than 8%.
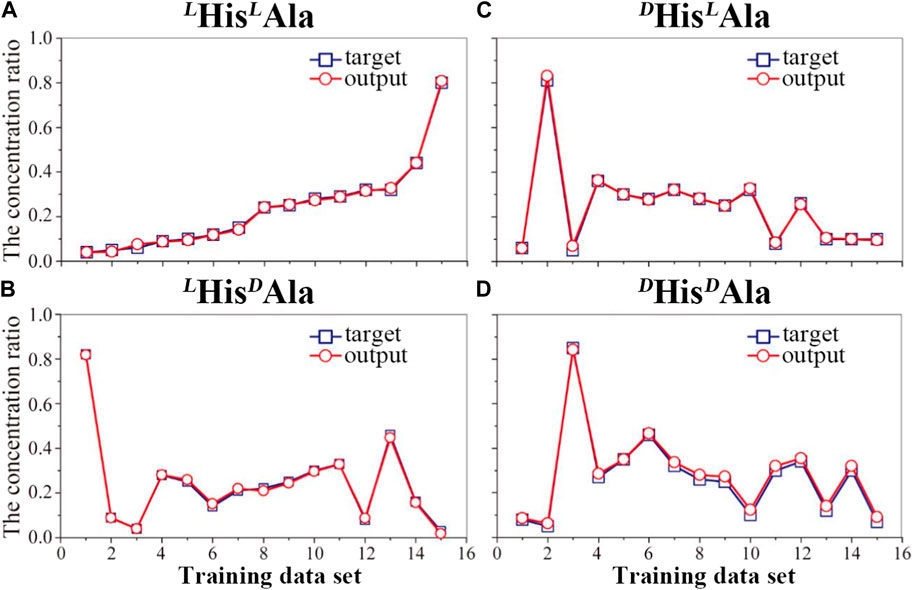
FIGURE 4. Trained results of the artificial neural network for the quantitative analysis of the four peptides: (A) LHisLAla, (B) LHisDAla, (C) DHisLAla and (D) DHis/DAla in mixture samples.
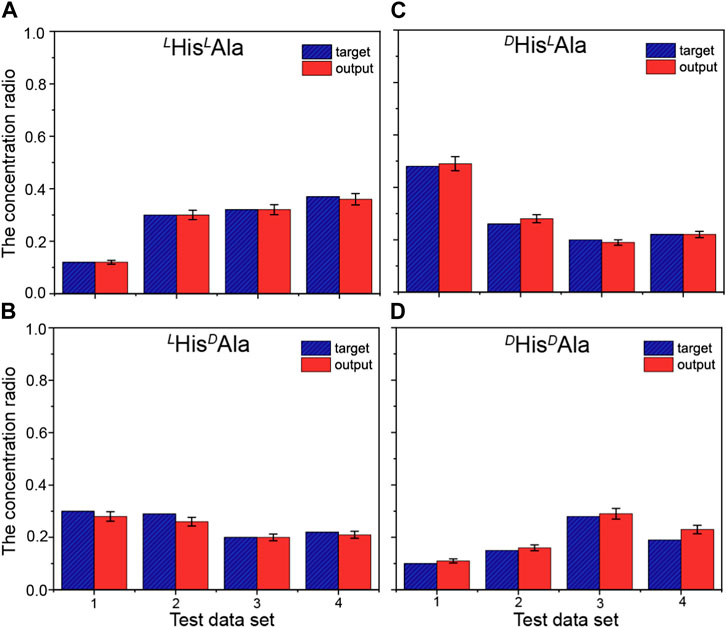
FIGURE 5. Comparisons between the true concentration ratios (blue striped columns) and predicted results (red columns) for the quantitative analysis of the four peptides: (A) LHisLAla, (B) LHisDAla, (C) DHisLAla and (D) DHis/DAla. The predictions were performed for four date sets, using the artificial neural network built based on the training set shown in Figure 4. The standard deviations of the predicted results based on three independent experimental data are less than 8%.
The most valuable variables in such an experiment are the distributions of fragment ions and how the distributions depend on the applied wavelengths. Thus, the selection of chiral ligands is important. For the second case, a small chiral reference of iodo-L-tyrosine is selected. The molecule has been previously studied in the gas phase. Ranka et al. have studied the radical rearrangement chemistry of the molecule using 193 nm UVPD mass spectrometry, IR ion spectroscopy and calculations, discovered that the high-energy radicals generated by UVPD engaged in following hydrogen/proton rearrangement (Ranka et al., 2018). The molecule has been also successfully applied as a good chiral reference for differentiation of enantiomeric pairs of amino acids and some pharmaceutically important drugs (Kumari et al., 2007; Karthikraj1 et al., 2012). Similarly, the 280 nm UVPD mass spectra of the four complex ions are shown in Supplementary Figure S1. The main fragment ions observed in the spectra include: the radical cations of [AspPhe + Mr-I]H+• formed by the loss of iodine atom, and its product of [AspPhe + Mr-I]+, the protonated ions of [AspPhe]H+, its radical cations of [AspPhe]+• and its dehydrated ions of [AspPhe-H2O]H+. By tuning the applied UV wavelength, the intensities of those fragment ions vary differently for the four chiral isomers. A similar artificial neural network described in the upper case has been built, and relative quantitative analysis of the multiple chiral isomers has been fulfilled using very limited training sets (10 samples), and the results are shown in the Supplementary Figures S2, S3.
4 Conclusion
In summary, we herein present an ANN approach for analyzing four chiral isomers from their UVPD mass spectra obtained under different wavelengths. Chiral reference molecules of tripeptides and iodo-L-tyrosine, have been successfully applied here to fulfill the relative quantitative analysis of four chiral isomers of dipeptides. The results show that the ANN can be well-trained with limited training sets, and have a quite good performance in testing sets. Meanwhile, the combination of the multiple UVPD mass spectra and ANN still has a lot of room for improvement in quantitative chiral analysis aimed at practical applications, such as searching better chiral references (Yu and Yao, 2017), finding possible derivatization methods (Will et al., 2021), and choosing more advanced machine learning methods.Fujihara and Maeda, 2017
Data availability statement
The raw data supporting the conclusion of this article will be made available by the authors, without undue reservation.
Author contributions
XK and XZ designed the study. YS and MZ participated in its experiment execution. YS, MZ, MK, and KZ collected the data and draw the figure draft. XK polished and financially supported the manuscript. All authors elaborated and discussed the results.
Funding
The authors gratefully acknowledge financial support from the National Natural Science Foundation of China (Grant Nos. 22174076 and 21627801).
Conflict of interest
The authors declare that the research was conducted in the absence of any commercial or financial relationships that could be construed as a potential conflict of interest.
Publisher’s note
All claims expressed in this article are solely those of the authors and do not necessarily represent those of their affiliated organizations, or those of the publisher, the editors and the reviewers. Any product that may be evaluated in this article, or claim that may be made by its manufacturer, is not guaranteed or endorsed by the publisher.
Supplementary material
The Supplementary Material for this article can be found online at: https://www.frontiersin.org/articles/10.3389/fchem.2023.1129671/full#supplementary-material
References
Awad, H., and El-Aneed, A. (2013). Enantioselectivity of mass spectrometry: Challenges and promises. Mass Spectrom. Rev. 32, 466–483. doi:10.1002/mas.21379
Cody, R. B., Hein, R. E., Goodman, S. D., and Marshall, A. G. (1987). Stored waveform inverse fourier transform excitation for obtaining increased parent ion selectivity in collisionally activated dissociation: Preliminary results. Rapid Commun. Mass Spectrom. 1, 99–102. doi:10.1002/rcm.1290010607
Deng, B. C., Yun, Y. H., Liang, Y. Z., Cao, D. S., Xu, Q. S., Yi, L. Z., et al. (2015). A new strategy to prevent over-fitting in partial least squares models based on model population analysis. Anal. Chim. Acta 880, 32–41. doi:10.1016/j.aca.2015.04.045
Filippi, A., Fraschetti, C., Piccirillo, S., Rondino, F., Botta, B., D’Acquarica, I., et al. (2012). Chirality effects on the IRMPD spectra of basket resorcinarene/nucleoside complexes. Chem. Eur. J. 18, 8320–8328. doi:10.1002/chem.201200614
Fujihara, A., Maeda, N., Doan, T. N., and Hayakawa, S. (2017). Enantiomeric excess determination for monosaccharides using chiral transmission to cold gas-phase tryptophan in ultraviolet photodissociation. J. Am. Soc. Mass Spectrom. 28, 224–228. doi:10.1007/s13361-016-1519-5
Fujihara, A., Maeda, N., and Hayakawa, S. (2016). Chiral recognition between l-alanine peptides and tryptophan enantiomers probed by ultraviolet photodissociation in the gas phase. J. Mass Spectrom. 51, 257–260. doi:10.1002/jms.3750
Fujihara, A., and Maeda, N. (2017). Quantitative chiral analysis of amino acids in solution using enantiomer-selective photodissociation of cold gas-phase tryptophan via chiral recognition. Anal. Chim. Acta. 979, 31–35. doi:10.1016/j.aca.2017.04.027
Fujihara, A., and Okawa, Y. (2018). Chiral and molecular recognition of monosaccharides by photoexcited tryptophan in cold gas-phase noncovalent complexes as a model for chemical evolution in interstellar molecular clouds. Anal. Bioanal. Chem. 410, 6279–6287. doi:10.1007/s00216-018-1238-9
Genchi, G. (2017). An overview on d-amino acids. Amino Acids 49, 1521–1533. doi:10.1007/s00726-017-2459-5
Han, D. Q., and Yao, Z. P. (2020). Chiral mass spectrometry: An overview. Trends Anal. Chem. 123, 115763. doi:10.1016/j.trac.2019.115763
Hu, M., Yuan, Y. X., Wang, W., Li, D. M., Zhang, H. C., Wu, B. X., et al. (2020). Chiral recognition and enantiomer excess determination based on emission wavelength change of AIEgen rotor. Nat. Comm. 11, 161. doi:10.1038/s41467-019-13955-z
Karthikraj1, R., Prabhakar, S., and Vairamani, M. (2012). Differentiation of enantiomeric drugs by iodo-substituted L-amino acid references under electrospray ionization mass spectrometric conditions. Rapid Commun. Mass Spectrom. 26, 1385–1391. doi:10.1002/rcm.6237
Kumari, S., Prabhakar, S., Vairamani, M., Devi, C. L., Chaitany, G. K., and Bhanuprakashb, K. (2007). Chiral discrimination of D- and L-amino acids using iodinated tyrosines as chiral references: Effect of iodine substituent. J. Am. Soc. Mass Spectrom. 18, 1516–1524. doi:10.1016/j.jasms.2007.05.006
Lanucara, F., Holman, S. W., Gray, C. J., and Eyers, C. E. (2014). The power of ion mobility-mass spectrometry for structural characterization and the study of conformational dynamics. Nat. Chem. 6, 281–294. doi:10.1038/NCHEM.1889
Lee, C. J., Qiu, T. A., and Sweedler, J. V. (2020). D-alanine: Distribution, origin, physiological relevance, and implications in disease. Biochim. Biophys. Acta Proteins Proteom 1868 (11), 140482. doi:10.1016/j.bbapap.2020.140482
Lee, S. S., Lee, J. U., Oh, J. H., Park, S., Hong, Y., Min, B. M., et al. (2018). Chiral differentiation of d- and l-isoleucine using permethylated β-cyclodextrin: Infrared multiple photon dissociation spectroscopy, ion-mobility mass spectrometry, and DFT calculations. Phys. Chem. Chem. Phys. 20, 30428–30436. doi:10.1039/c8cp05617j
Lee, S. S., Park, S., Hong, Y., Lee, J. U., Kim, J. H., Yoon, D., et al. (2017). Chiral differentiation of d- and l-alanine by permethylated β-cyclodextrin: IRMPD spectroscopy and DFT methods. Phys. Chem. Chem. Phys. 19, 14729–14737. doi:10.1039/c7cp01085k
Liao, G., Yang, Y., and Kong, X. (2013). Chirality effects on proline-substituted serine octamers revealed by infrared photodissociation spectroscopy. Phys. Chem. Chem. Phys. 16, 1554–1558. doi:10.1039/c3cp53469c
Ly, T., and Julian, R. (2009). Ultraviolet photodissociation: Developments towards applications for mass-spectrometry-based proteomics. Angew. Chem. Int. Ed. 48, 7130–7137. doi:10.1002/anie.200900613
Ma, L., Ren, J., Feng, R., Zhang, K., and Kong, X. (2018). Structural characterizations of protonated homodimers of amino acids: Revealed by infrared multiple photon dissociation (IRMPD) spectroscopy and theoretical calculations. Chin. Chem. Lett. 29, 1333–1339. doi:10.1016/j.cclet.2018.02.008
Müller, C., Pidko, E., Staring, A., Lutz, M., Spek, A., van Santen, R., et al. (2008). Developing a new class of axial chiral phosphorus ligands: Preparation and characterization of enantiopure atropisomeric phosphinines. Chem. Eur. J. 14, 4899–4905. doi:10.1002/chem.200800175
Nguyen, L. A., He, H., and Pham-Huy, C. (2006). Chiral drugs: An overview. Int. J. Biomed. Sci. 2, 85–100.
Ranka, K., Zhao, N., Yu, L., Stanton, J. F., and Polfer, N. C. (2018). Radical rearrangement chemistry in ultraviolet photodissociation of iodotyrosine systems: Insights from metastable dissociation, infrared ion spectroscopy, and reaction pathway calculations. J. Am. Soc. Mass Spectrom. 29, 1791–1801. doi:10.1007/s13361-018-1959-1
Ren, J., Wang, Y., Feng, R., and Kong, X. (2017). Investigation of l/d-threonine substituted l-serine octamer ions by mass spectrometry and infrared photodissociation spectroscopy. Chin. Chem. Lett. 28, 537–540. doi:10.1016/j.cclet.2016.10.032
Scaramuzzo, F. A., Licini, G., and Zonta, C. (2013). Determination of amino acid enantiopurity and absolute configuration: Synergism between configurationally labile metal-based receptors and dynamic covalent interactions. Chem. Eur. J. 19, 16809–16813. doi:10.1002/chem.201302721
Shi, Y., Du, M., Ren, J., Zhang, K., Xu, Y., and Kong, X. (2020). Application of infrared multiple photon dissociation (IRMPD) spectroscopy in chiral analysis. Molecules 25, 5152. doi:10.3390/molecules25215152
Shi, Y., Zhou, M., Zhang, K., Ma, L., and Kong, X. (2019). Chiral differentiation of non-covalent diastereomers based on multichannel dissociation induced by 213-nm ultraviolet photodissociation. J. Am. Soc. Mass. Spectrom. 30, 2297–2305. doi:10.1007/s13361-019-02302-7
Sun, L., Huang, F., Liu, W., Lin, L., Hong, Y., and Kong, X. (2020). Chiral differentiation of - and -penicillamine by β-cyclodextrin: Investigated by IRMPD spectroscopy and theoretical simulations. Spectrochim. Acta. A Mol. Biomol. Spectrosc. 241, 118653. doi:10.1016/j.saa.2020.118653
Tao, W. A., and Cooks, R. G. (2003). Peer reviewed: Chiral analysis by MS. Anal. Chem. 75, 25A–31A A. doi:10.1021/ac0312110
Tao, W. A., Wu, L., and Cooks, R. G. (2001). Differentiation and quantitation of isomeric dipeptides by low-energy dissociation of copper (II)-bound complexes. J. Am. Soc. Mass Spectrom. 12, 490–496. doi:10.1016/S1044-0305(01)00237-9
Ward, T. J., and Baker, B. A. (2008). Chiral separations. Anal. Chem. 80, 4363–4372. doi:10.1021/ac800662y
Wenzel, T. J. (2018). Differentiation of chiral compounds using NMR spectroscopy. 2nd ed. Hoboken, NJ, USA: John Wiley & Sons. doi:10.1002/9781119324782
Will, J. M., Behrens, A., Macke, M., Quarles, C. D., and Karst, U. (2021). Automated chiral analysis of amino acids based on chiral derivatization and trapped ion mobility–mass spectrometry. Anal. Chem. 93 (2), 878–885. doi:10.1021/acs.analchem.0c03481
Wu, L., and Vog, F. G. (2012). A review of recent advances in mass spectrometric methods for gas-phase chiral analysis of pharmaceutical and biological compounds. Pharm. Biomed. Anal. 69, 133–147. doi:10.1016/j.jpba.2012.04.022
Yu, X., and Yao, Z. P. (2017). Chiral recognition and determination of enantiomeric excess by mass spectrometry: A review. Anal. Chim. Acta. 968, 1–20. doi:10.1016/j.aca.2017.03.021
Keywords: chiral analysis, multiple isomers, mass spectrometry, ultraviolet photodissociation, dipeptide
Citation: Shi Y, Zhou M, Kou M, Zhang K, Zhang X and Kong X (2023) Simultaneous quantitative chiral analysis of four isomers by ultraviolet photodissociation mass spectrometry and artificial neural network. Front. Chem. 11:1129671. doi: 10.3389/fchem.2023.1129671
Received: 22 December 2022; Accepted: 02 March 2023;
Published: 09 March 2023.
Edited by:
Wei-Lung Tseng, National Sun Yat-Sen University, TaiwanReviewed by:
Iker León, University of Valladolid, SpainChi-Yu Lu, Kaohsiung Medical University, Taiwan
Copyright © 2023 Shi, Zhou, Kou, Zhang, Zhang and Kong. This is an open-access article distributed under the terms of the Creative Commons Attribution License (CC BY). The use, distribution or reproduction in other forums is permitted, provided the original author(s) and the copyright owner(s) are credited and that the original publication in this journal is cited, in accordance with accepted academic practice. No use, distribution or reproduction is permitted which does not comply with these terms.
*Correspondence: Xianglei Kong, kongxianglei@nankai.edu.cn