- Institut für Organische Chemie und Makromolekulare Chemie, Heinrich-Heine-Universität Düsseldorf, Düsseldorf, Germany
Multicomponent reactions, conducted in a domino, sequential or consecutive fashion, have not only considerably enhanced synthetic efficiency as one-pot methodology, but they have also become an enabling tool for interdisciplinary research. The highly diversity-oriented nature of the synthetic concept allows accessing huge structural and functional space. Already some decades ago this has been recognized for life sciences, in particular, lead finding and exploration in pharma and agricultural chemistry. The quest for novel functional materials has also opened the field for diversity-oriented syntheses of functional π-systems, i.e. dyes for photonic and electronic applications based on their electronic properties. This review summarizes recent developments in MCR syntheses of functional chromophores highlighting syntheses following either the framework forming scaffold approach by establishing connectivity between chromophores or the chromogenic chromophore approach by de novo formation of chromophore of interest. Both approaches warrant rapid access to molecular functional π-systems, i.e. chromophores, fluorophores, and electrophores for various applications.
1 Introduction
Chromophores have increasingly become functional organic materials (Müller, 2007). As a consequence of their photophysical properties, such as fluorescence and aggregation-induced emission (AIE) (Hong et al., 2009; Hong et al., 2011; Hu et al., 2014; Mei et al., 2015; Mazumdar et al., 2016), and their electrochemical characteristics they find broad application in organic light-emitting diodes (OLEDs) (Müllen and Scherf, 2006; Park et al., 2011; Kalyani and Dhoble, 2012; Li, 2015), dye-sensitized solar cells (DSSCs) (Grätzel, 2001; Mishra et al., 2009), organic photovoltaics (OPVs) (Yam, 2010; Su et al., 2012; Ameri et al., 2013), organic field effect transistors (OFETs) (Kymissis, 2008; Torsi et al., 2013) and in bio- or environmental analytics (Chen et al., 1998; Nilsson et al., 2002; Wagenknecht, 2008; Kim and Park, 2009; Cairo et al., 2010), as well as in medicinal and pharmaceutical applications (Dua et al., 2011; Arora et al., 2012; Saini et al., 2013; Kumar and Kumar Jain, 2016; Taylor et al., 2016; Asif, 2017; Taek Han et al., 2017; Petronijevic et al., 2018; Soleymani and Chegeni, 2019). The ongoing quest for novel, efficient syntheses of new functional chromophores with well-defined features and thoroughly fine-tuned properties remains a challenge. Research on diversity-oriented syntheses concepts for functional chromophores has become highly relevant over the past one and a half decades (Müller and D'Souza, 2008; Briehn and Bäuerle, 2002; Zhu et al., 2014; D'Souza and Müller, 2007). In particular, multicomponent reactions (MCRs) (Weber et al., 1999; Bienaymé et al., 2000; Dömling and Ugi, 2000; Zhu and Bienaymé, 2006; Müller, 2016; Riva et al., 2016) have been established as a promising tool for the synthesis of functional
By definition MCRs proceed in a one-pot fashion, where more than two starting components react to form a product containing most of the deployed atoms. In sensu stricto, the process is not only conducted in a single reaction vessel, but also without changing the solvent, filtration or any other workup operations. Indeed, MCR represent a reactivity-based concept (Müller, 2014), where reactivities of functional groups can be employed in three different ways. In a domino MCRs, all compounds are present in the vessel from the beginning of the process. In a sequential MCR, reactants are added in a well-defined order while the reaction conditions maintained constant. Finally, MCRs taking advantage of defined order of transformation of the reactants, yet, with variably adjusting the reaction conditions from step to step are considered as consecutive processes. All MCR have in common high levels of functional and structural diversity with a minimum of purification operations at the end of the one-pot processes.
MCRs are generally well suited to generate acyclic or cyclic scaffolds that either bear chromophores as functional substituents (scaffold approach) or can be considered de novo formed chromophores (chromogenic approach) (Scheme 1) (Levi and Müller, 2016b). While the former approach allows placing multiple chromophores in close proximity with defined configuration and conformation by the constituted scaffold, also in an electronically non-conjugative fashion, the latter approach is chromogenic and forms the chromophore scaffold of interest with broad structural diversity in a one-pot fashion.
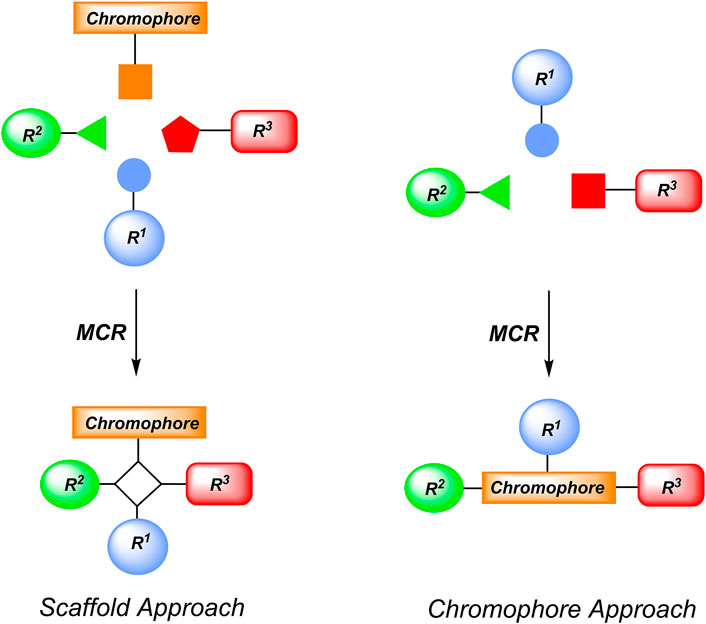
SCHEME 1. Conceptual MCR formation of functional chromophores by scaffold and chromophore approaches (Reprinted from Levi and Müller (2016b), Copyright (2016), with permission from The Royal Society of Chemistry).
Both approaches are advantageous for advanced chromophore research since large chromophore libraries are rapidly and convergently generated. This allows extensive exploration of electronic properties by electrochemical and photophysical investigation in combination with advanced computational chemistry. Ultimately, the evolving structure-property relationships lead to a more comprehensive understanding and to a rational experimentally founded design of functional chromophores, which are highly requested for high-tech applications.
Guided by the synthetic chromogenic concept of MCR we have structured this review, which updates our previous overview on MCR approaches to functional chromophores (Levi and Müller, 2016b), according to the chromophore classes as defined by their acyclic or (hetero)cyclic central structural elements. Starting from merocyanines, we span the arc from five-to six-membered heterocycles or (hetero)arene and azo chromophores to complexes, discussing predominantly chromophore approaches and some scaffold approaches for illustrating and highlighting in a flashlight fashion most recent developments.
2 Merocyanines
Merocyanines are donor-acceptor polyenes (Mishra et al., 2000; Kulinich and Ishchenko, 2009a; Kulinich and Ishchenko, 2009b; Hamer, 2009). Due to the polymethine chain and the resulting conjugated
It should be noted that all alkyl-substituted derivatives are characterized by a single extremely narrow absorption band in the visible (λmax,abs = 495–533 nm). All of the obtained indoline 2, benzothiazole 3 and benzoxazole dyes 4 display red or orange solid-state luminescence. Only a few of the thiazole dyes 1 fluoresce in solid state. Thiazole dyes 1 absorb light in a range of 528–555 nm. Because of their electronic peculiarity that contributions of the non-polar and the zwitterionic resonance structures are almost identical both in the electronic ground and excited state, these dyes find application in color copy (Gregory, 2012).
A variety of indolone-based merocyanines with a push-pull character on the basis of insertion-alkynylation Michael addition sequence can be synthesized (Muschelknautz et al., 2014; D'Souza et al., 2010; Muschelknautz et al., 2013). Recently, even a white light emitter generated by aggregation-induced double emission (AIDE) in combination with partial energy transfer is postulated (Denißen et al., 2020). Indolone-based merocyanines, with a Boc group, also exhibit interesting photophysical properties such as aggregation-induced emission (AIE) or crystallization-induced emission enhancement (CIEE) (Denissen et al., 2017a).
The Boc-substituted 3-arylallylidene indolones 5 can be synthesized via a consecutive four-component approach following the chromophore concept. The synthesis via Heck condensation sequence is not stereoselective regarding the allylidene double bond adjacent to the indolone, which exhibits photoisomerization in daylight (Scheme 2A) (Wilbert and Müller, 2022).
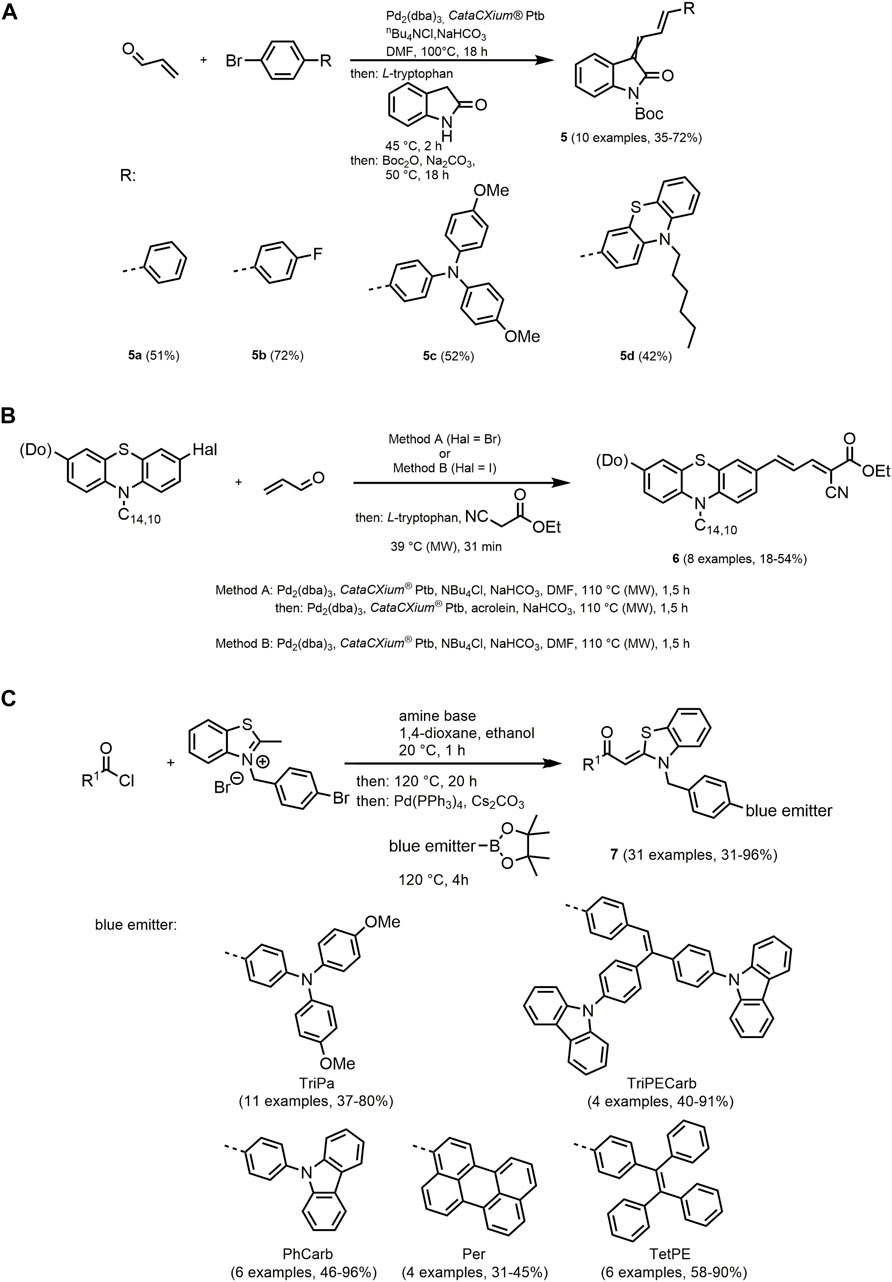
SCHEME 2. (A) Consecutive one pot four-component synthesis of allylidene indolone chromophores 5 by a Heck condensation sequence and selected examples (Wilbert and Müller, 2022). (B) Consecutive three-component Heck-Knoevenagel synthesis of merocyanine esters 6 (Stephan et al., 2022). (C) Consecutive three-component reaction to synthesize N-benzyl aroyl-S,N-keteneacetal bichromophores 7 and an insight into the compound library sorted by the employed blue emitters (Biesen et al., 2021).
Notably, the respective diastereomers of compound 5 share very similar photophysical properties. In general, most of the derivatives display exclusively solid-state emission. Among them, compounds 5a and 5b possess the highest fluorescence quantum yields reaching 18%. Furthermore, the oxindole-based merocyanines 5c and 5d are also luminescent in solution and exhibit positive emission solvatochromism. Additionally, 5c exhibits aggregation-induced emission enhancement (AIEE) (Supplementary Material S2).
Using a Heck-Knoevenagel sequence, 7-donor-substituted phenothiazinyl merocyanines 6 are prepared with yields up to 54%. The optimal reaction conditions of the three-component reaction involving heteroaryl halides, acrolein and ethyl cyanoacetate were developed by statistical design of experiments and Bayesian optimization (Scheme 2B) (Stephan et al., 2022).
The phenothiazine-based merocyanines 6 are red-orange dyes that reveal longest absorption bands in a range from 466 to 491 nm. Using the Hammett plot, a correlation of the red shift of the absorption band with increasing donor strength can be proven, which indicates an essential charge transfer character. The fluorescence of the chromophores is located in a range from 730 to 780 nm with Stokes shifts of 6,500–8,200 cm−1. The carboxylic acids obtained by saponification of merocyanine esters can be implemented in DSSC and achieve solar cell performance with relative efficiencies up to 93% compared to standard ruthenium dye N3.
Further comparably small merocyanines with outstanding photophysical properties such as AIE behavior and tunable solid-state emission are proposed by chromophores with aroyl-S,N-ketene acetal building blocks (Biesen et al., 2020). The previous reported systems were modified by synthesis of bichromophores. For this purpose, blue emitters were introduced after condensing aroyl chlorides and a N-(p-bromobenzyl) 2-methyl-benzothiazolium salt by a Suzuki coupling of pinacolboronic esters. This three-component one-pot procedure enabled an extraordinarily large substance library of N-benzyl aroyl-S,N-keteneacetal bichromophores 7 (Scheme 2C) (Biesen et al., 2021).
The synthesized fluorophores show a rainbow-like tuning of the solid-state emission color. Depending on the substitution pattern, a color impression from blue to orange-red can be achieved (Supplementary Material S3).
The variation of both chromophores allows different communication pathways between them. This can result in full or partial energy transfer processes leading to dual emission, or aggregation-induced switching of fluorescence. This selective excitation of either the blue emitter or the aroyl S,N-ketene acetal provides specific emission behavior in solution or in aggregates, which can be exploited for application as a pH sensor or as a detector of water content in alcoholic beverages.
The bichromophore systems could be further developed by preparing asymmetrically bridged aroyl-S,N-ketene acetals and aroyl-S,N-ketene acetal multichromophores 8 via multiple Suzuki one-pot sequences starting from electron donating p-bromo substituted benzylaroyl-S,N-ketene acetals. Phenyl and more complex dye systems were accepted as linker systems within the three-component Suzuki reaction. In order to synthesize the multichromophore systems, triborylated benzene and triphenylamine as well as tetraborylated tetraphenylethene were used as linkers (Supplementary Material S4) (Biesen et al., 2022).
Similar to previous synthesized bichromophores, the bridged compounds 8 exhibit AIE and ET properties. The emission behavior in solution and in the solid state as well as starting point for the formation of aggregates can be determined by the employed linker molecule. The interaction of the aroyl S,N-ketene acetals and the linker influences photophysical properties such as emission color and intensity, fluorescence quantum yield and lifetime. Furthermore, due to the different combination of the linker and aroyl S,N-ketene acetal, partial and complete energy transfer processes as well as different emission behavior upon aggregation (AIE, AIEE and ACQ) is observed. Interestingly, the emission properties of bichromophores 7 and multichromophores 8 can be enhanced by encapsulation in polystyrene particles.
Just recently a Suzuki-Knoevenagel condensation sequence was applied to synthesize merocyanines with good DSSC performance in a one-pot fashion (Meyer and Müller, 2020). The consecutive three-component reaction of boronic acids/esters, (hetero)aromatic bromoaldehydes and methylene-active compounds was expanded to a library of 60 donor-
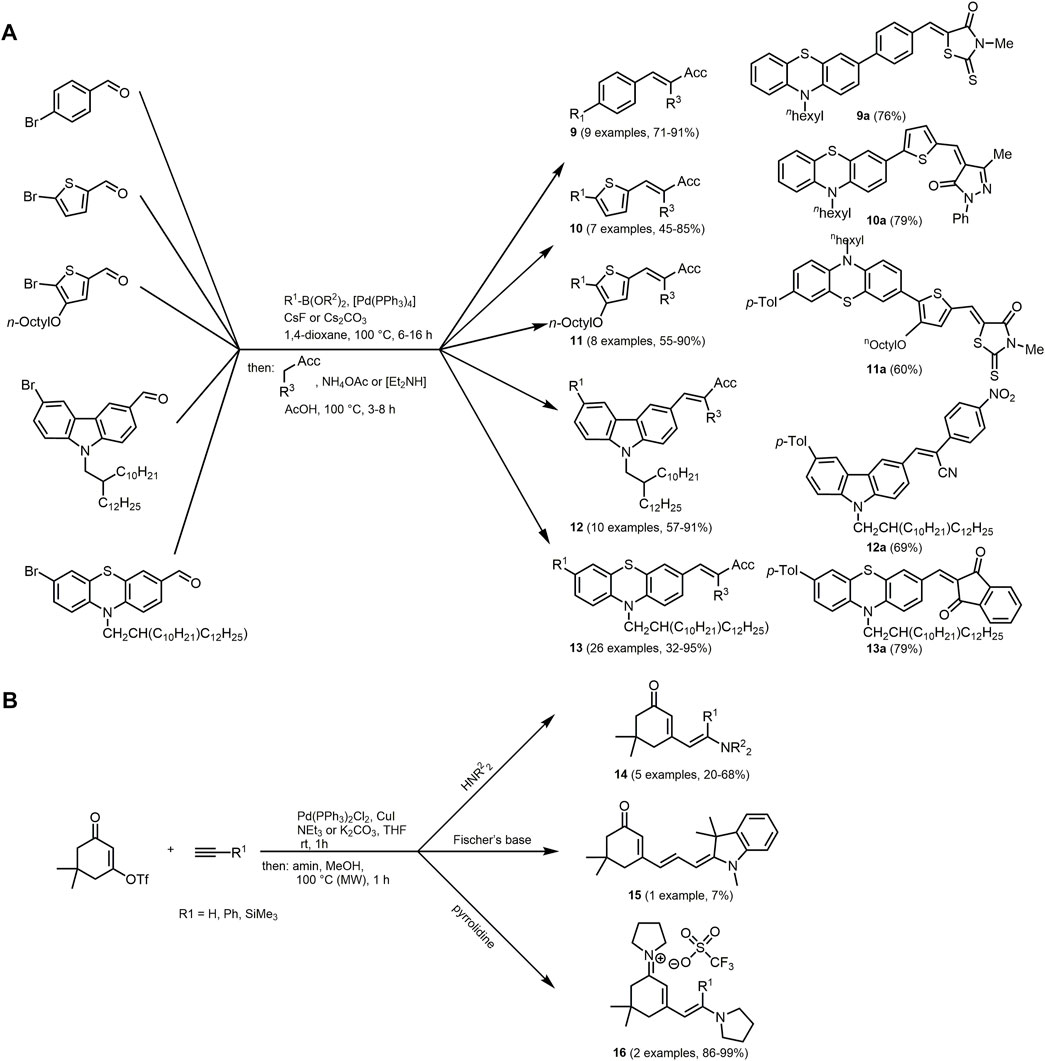
SCHEME 3. (A) Three-component Suzuki–Knoevenagel synthesis of merocyanines 9–13 as well as representative derivatives of the substance libraries. The position of the acceptor group is indicated by Acc (Meyer et al., 2021). (B) One-pot synthesis of a triflate compound, terminal alkyne and various amines to form cyclohexene-embedded merocyanines 14 and 15 as well as cyanines 16 (Papadopoulos et al., 2022).
The UV/Vis spectra of merocyanine 9–13 exhibit the longest wavelength absorption maxima in a broad spectral range of λmax,abs = 367–580 nm with molar extinction coefficients ε between 21,000 and 52,000 M−1 cm−1, which accounts to a dominant charge transfer transition from the donor to the acceptor part. The emission maxima can be detected in a range of 412–668 nm with Stokes shifts ranging from 1,200 to 8,000 cm−1 (0.147–0.990 eV). The energy of the hypothetical E0-0 transition determined from the intersection of the absorption and emission bands represents the optical band gap and lies between 2.083 and 3.197 eV. A correlation analyses by plotting the optical band gaps E0-0 against the first oxidation potentials E1/2 of redox active systems of consanguineous series furnishes linear correlations and, by extension, two parameter correlations (oxidation potential and emission maximum) with the optical band gaps. Thus, given this planar correlation, for a number of merocyanines optical band gaps can be predicted based on the first oxidation potentials and emission maxima.
Consecutive three-component alkynylation addition sequences can be performed to synthesize cyclohexene-embedded merocyanines 14 and 15. A stronger nucleophilic amine moiety like pyrrolidine can be employed to synthesize cyanines 16 in excellent yields via a pseudo four-component synthesis by carbonyl condensation of the heterocyclic amine (Scheme 3B) (Papadopoulos et al., 2022).
Merocyanines 14 and 15 and cyanines 16 are obtained as yellow to orange solids. The prepared merocyanine derivatives are found to be non-luminescent in solution and in solid state, whereas the cyanines exhibit luminescence with low quantum yield of 1%. The absorption maxima of 16 are located at 443 and 459 nm with absorption coefficients over 110·103 M−1cm−1 for the two derivates. The absorption maxima of the merocyanines 14/15 and component 16 are located at 365–390 nm and 442 nm, respectively. Due to the extension of the system, the red shift of the absorption band can be explained.
3 Five-membered heterocycles
3.1 Oxygen heterocycles
Furans are found in many natural products (Keay and Dibble, 1996) and as a result of their biological activity, they are often structural moieties in pharmaceuticals (Iyer and Gopalachari, 1973; Dikshit et al., 1974; Durani et al., 1989; Dikshit et al., 1991; Nieves-Neira et al., 1999; Mortensen et al., 2001; Chène, 2003; Issaeva et al., 2004; Thuita et al., 2008; Wenzler et al., 2009; Barrett and Croft, 2012). The Molisch test is a colometric detection method that can be used to detect carbohydrates that have been converted into furfuraldehydes by thermal acid degradation. This detection is carried out via reaction with α-naphthol and results in a violet coloration (Molisch, 1886). But aside from their pharmacological importance, they can also be considered as photonic chromophores (Liu and Luh, 2002). In general, many furan heterocycles exhibit strong fluorescence. Due to their excellent semiconducting properties, they are found in organic solar cells (Zheng and Huo, 2021). In addition, furan possesses strong non-linear optical properties which makes it useful in a number of non-linear optical applications (Kamada et al., 2000; Gündüzalp et al., 2016). Classic synthetic routes for the production of substituted furans rely either on cyclocondensation of dicarbonyl compounds or on furan ring substitution (Zeni and Larock, 2004; Brown, 2005; Duc, 2019). The preparation of 2,5-disubstituted furans is commonly carried out via a Paal-Knorr reaction in the sense of a cyclocondensation of 1,4-diketones (Amarnath and Amarnath, 1995), yet a consecutive multicomponent approach to symmetrically 2,5-disubstituted furans via a Sonogashira-Glaser addition-cyclization sequence is known (Klukas et al., 2014).
Butenolides are unsaturated lactones consisting of a dihydrofuran-2-one group. They are important structural building blocks in natural products (Seitz and Reiser, 2005; Roethle and Trauner, 2008; Kitson et al., 2009; Igarashi et al., 2011) and often possess high biological activity such as antibiotic, anticancer and antitumor (Shiomi et al., 2005; Wu et al., 2005; Fei et al., 2007; Liu et al., 2008; Isaka et al., 2009; Wang et al., 2009; Kitani et al., 2011; Uchida et al., 2011; Chen et al., 2012; Csuk et al., 2012). The coupling of a chromium methoxycarbene complex with a ketone or an imide lithium enolate followed by a propargylic organomagnesium reagent lead to the synthesis of novel hydroxy-substituted bicyclic [4.3.0]-galkylidene-2-butenolides 17 and 18 (Scheme 4A) (Suero et al., 2012). Upon modulating substituents on the starting materials, high diversity and complexity of the bicycles can be realized. For instance, alkyl, aryl, heteroaryl, alkenyl and alkynyl groups are well tolerated in the bicyclic compound 17 as a group R2 resulting from the ketone component. The synthesis of compound 18 proceeds enantioselectively due to the inserted chiral imide enolate auxiliary.
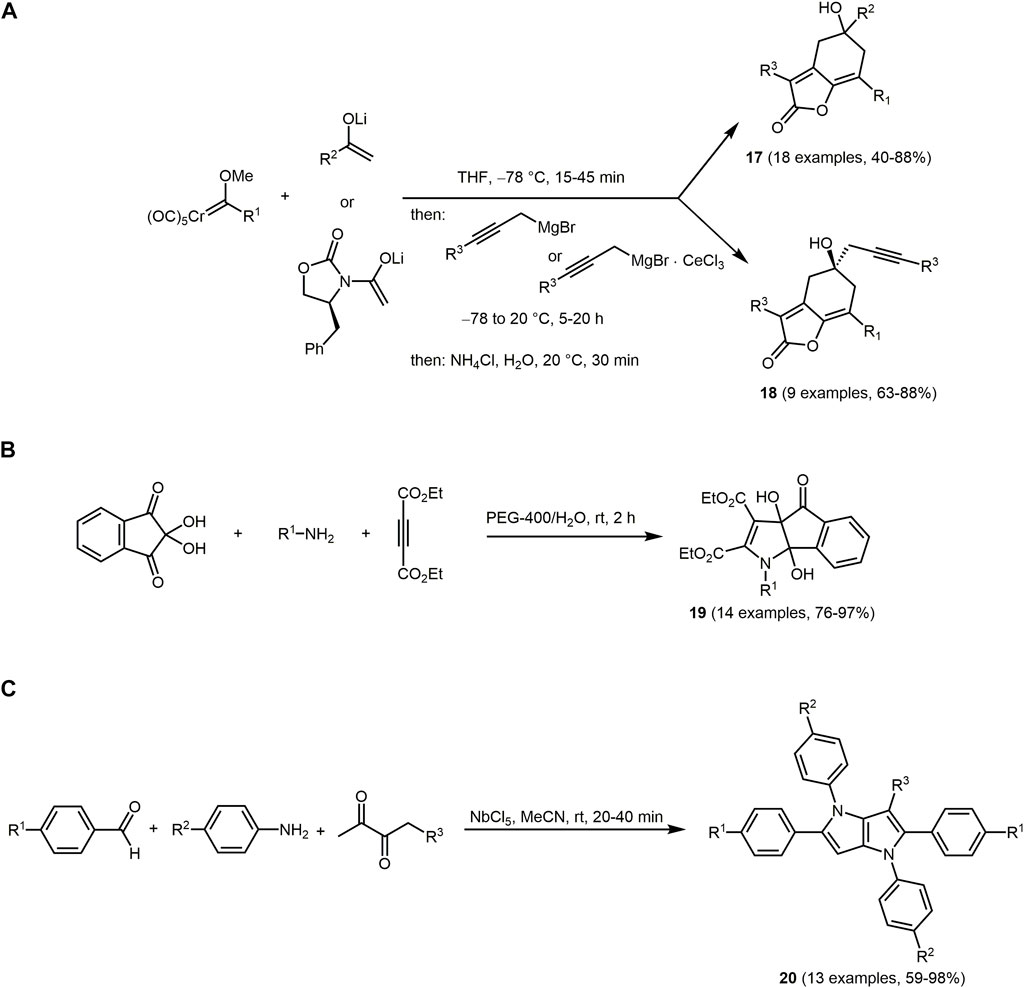
SCHEME 4. (A) One-pot synthesis of 6-5-bicyclic γ-alkylidene-2-butenolides 17 and 18 from ketone lithium enolates or from imide lithium enolates (Suero et al., 2012). (B) Three-component synthesis of highly functionalized dihydroindeno[1,2-b]pyrrole fluorophores 19 (Mal et al., 2018). (C) Pseudo five-component synthesis of tetraaryl-1,4-dihydropyrrolo-[3,2-b]pyrrole derivatives 20 (Martins et al., 2018).
Both heterocycles embed the γ-(2-arylalkylidene)-2-butenolide fluorophore and the bicycles 17 and 18 fluoresce intensively blue in solution with large Stokes shifts.
3.2 Nitrogen heterocycles
Pyrrole is the five-membered azol and appears as a structural core in many biologically active molecules with antibacterial (Daidone et al., 1990), antifungal (Kaiser and Glenn, 1972), anti-inflammatory (Battilocchio et al., 2013), antioxidant (Meshram et al., 2010), antitumour and ionotropic properties (Jonas et al., 1993). Besides, pyrroles naturally and non-naturally occurring pyrroles are per se interesting as functional dyes (Jonas et al., 1993; Fürstner et al., 1998; Fürstner, 2003; Jones and Bean, 2013; Khajuria et al., 2016). Thus, aryl-substituted pyrrole derivatives with pronounced AIE properties are known (Feng et al., 2010; Han et al., 2012; Shi et al., 2012) and in addition, for instance, indenopyrrole derivatives can serve as Al(III) selective OFF-ON chemosensors for bioimaging in live human hepatocellular carcinoma cells (Mal et al., 2018). For this purpose, the reaction of ninhydrin, primary amines and dialkyl acetylenedicarboxylates furnishes a series of dihydroindeno[1,2-b]pyrrole derivatives utilizing the chromophore approach (Scheme 4B). The selected green solvent is water and PEG-400 [polyethylene glycol (400)] acts as a phase transfer catalyst. First, a nucleophilic enamine diester is formed upon reaction of the amine and dialkyl acetylenedicarboxylate, which nucleophilically attacks the carbonyl center of the ninhydrin. After water elimination, intramolecular cyclization and tautomerization results in the formation of indeno[1,2-b]pyrrole derivatives 19.
Chromophores 19 exhibit a weak emission in the range of around 485 and 515 nm. However, the fluorescence intensity increases selectively in the presence of Al(III) ions due to CHEF (chelation-enhanced fluorescence). Complexation ensues between the Al(III) ions and presumably the two syn-O-atoms of the functional alcohol groups, which can be experimentally verified by 1H NMR as well as via calculations (Sun et al., 2012; Jo et al., 2016; Naskar et al., 2018).
Several multicomponent syntheses of pyrroles have been reported (Balme, 2004; Estevez et al., 2010; Estévez et al., 2014). For instance, tetraaryl-1,4-dihydropyrrolo[3,2-b] pyrroles 20 are formed via Mannich reaction of benzaldehydes, anilines and β-diketone derivatives in the sense of a chromophore approach (Scheme 4C) (Martins et al., 2018). The in situ generated Schiff base reacts with the enol form of butane-2,3-dione. Then, the cyclic enamine intermediate adds to another Schiff base. The pyrrolopyrrole is finally formed cyclization and subsequent oxidation (Janiga et al., 2013). These compounds can be classified as heteropentalenes which consist of two fused heterocyclic five-membered rings (Gribble and Joule, 2009) with 10 π-electrons and, thus, elevating them for further usage in electronic devices (Biswas et al., 2016). Chromophores 20 are promising candidates as sensitizing dyes in optoelectronic applications. For the MCR synthesis of compounds 20, niobium pentachloride is used as a Lewis acid catalyst. The reaction proceeds via two competing mechanisms, which can be controlled by the reactivity of the selected benzaldehyde and aniline derivatives.
Most components absorb between 294 and 382 nm and emit around 420 nm. As said, the photophysical properties suggest that these materials can be used as sensitizing dyes in optoelectronic devices. The modulation of the optical properties can be particularly controlled by the substituent R1 benzaldehyde derivative. For the electron-withdrawing substituent NO2, the absorption bands redshifts to the region between 500 and 600 nm. In contrast, the electron-releasing p-methyl substituent CH3 causes a blueshift of the absorption bands.
Imidazole is a key structural motif of considerable importance in biomolecules as represented by the essential amino acid histidine (Grimmett et al., 1984; Luca, 2006; Bellina et al., 2007). Imidazoles have been shown to possess antibacterial (Khabnadideh et al., 2003; Khalafi-Nezhad et al., 2005), antifungal (Pestellini et al., 1987; Jones et al., 1990; Silvestri et al., 2004), farnesyltransferase inhibitory (Curtin et al., 2003; Lin et al., 2003; Tong et al., 2003), and anti-inflammatory activity properties (Raingeaud et al., 1995; Beyaert et al., 1996) or function as selective ligands at histamine receptors (Ganellin et al., 1996; De Esch et al., 1999; Elz et al., 2000). Imidazole-fused heterocycles can exhibit interesting fluorescence properties such as excited-state intramolecular proton transfer (ESIPT) (Heller and Williams, 1970; Goodman and Brus, 1978; Kasha, 1986; Park et al., 2005; Skonieczny et al., 2012; Ali et al., 2015). In addition, some non-linear optical (NLO) imidazole-based chromophores (Stähelin et al., 1992; Bu et al., 1996; Santos et al., 2001; Wang et al., 2002) as well as donor-acceptor chromophores are known (Murata et al., 2007; Chang et al., 2008; Chang et al., 2009; Kulhánek et al., 2011). The Groebke-Blackburn-Bienaymé reaction (GBBR) represents an MCR approach to imidazoazines by acid-catalyzed condensation of aminoazines, aldehydes and isocyanides (Bienaymé and Bouzid, 1998; Blackburn et al., 1998; Groebke et al., 1998). Using the concept of GBBR, heterocyclic scaffolds with adjustable properties can be prepared. Chromophores 21 are synthesized via a double GBBR of 2,4-diaminopyrimidine and a variety of aldehydes and isocyanides in moderate to good yields following the chromophore concept (Scheme 5AI) (Ghashghaei et al., 2018). The process can also be conducted in a sequential fashion to give unsymmetrically substituted chromophores 23 via the intermediate GBB monoadducts 22 (Scheme 5A II). The high selectivity is rationalized by preferential formation of an imine from the amino group at 2-position in the initial step of the GBB. Likewise, various polyaminopolyazines such as 2,4-diaminoquinazolines and 3,6-diaminopyridazines as substrates are equally successfully transformed.
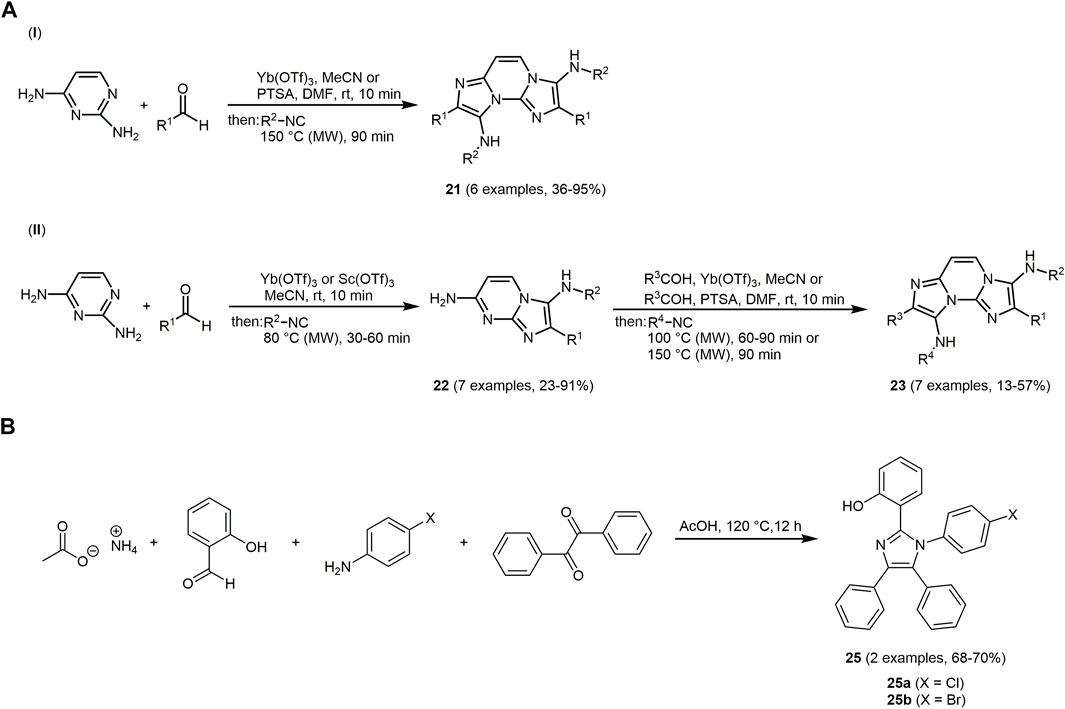
SCHEME 5. (A) Synthesis of GBB products 21 and 23 via twofold GBBR of 2,4-diaminopyrimidine in a direct (I) and a sequential approach (II) (Ghashghaei et al., 2018). (B) Debus-Radziszwski multicomponent reaction to form imidazole-based fluorophores 25 (Somasundaram et al., 2018).
The emission maxima of selected GBBR dyes 21 and 23 are found in a narrow range from 447 to 460 nm. By the substituent diversity on the GBB cores, the emission can be tuned. Thus, a redshift in absorption and emission wavelengths occurs by expanding the π-electron conjugation with conjugated (hetero)aryl groups and their modification. For instance, the orange bispyridinium salt 24 is prepared by alkylation of the pyridine ring of yellow chromophore 21a (Supplementary Material S5). While compound 21a shows no specific interaction with quadruplex DNA structures, the bispyridinium salt 24 reveals significant strong interaction and can be potentially applied as selective binder for the further development of new anticancer drugs.
A further established approach for the synthesis of imidazoles is the Debus-Radziszwski reaction (Debus, 1858; Radzisewski, 1882). Upon reaction of ammonium acetate, salicylaldehyde, 4-chloro/bromoaniline and benzil in acetic acid, 2-(1-(4-chlorophenyl)-4,5-diphenyl-1H-imidazol-2-yl)phenol 25a and 2-(1-(4bromophenyl)-4,5-diphenyl-1H-imidazol-2-yl)phenol 25b are obtained in good yields (Scheme 5B) (Somasundaram et al., 2018).
The synthesized imidazole chromophores 25 are blue fluorescent and show excited state proton transfer (ESIPT). In the solid state as well as in solution, intense fluorescence is exhibited due to the four phenyl rings on the imidazole core limiting the intermolecular interactions between neighboring molecules.
Isoxazoles rarely occur in nature, yet, these heterocycles possess enormous bioactive properties (Palmer and Venkatraman, 2003) and are therefore used in therapeutics (Kumbhare et al., 2012; Joshi et al., 2017; Pairas et al., 2017). However, their photophysical properties are still largely unexplored (Irfan et al., 2016; de Brito et al., 2018; Sato et al., 2020). Some one-pot methods for the formation of isoxazoles have been reported (Merkul and Müller, 2006; Kaim et al., 2009; Wachenfeldt et al., 2013). Just recently, a sequentially palladium catalyzed consecutive four-component coupling-cyclocondensation-coupling (C3) synthesis of isoxazoles from aroyl chloride, alkyne, hydroxylamine and boronic acids has been disclosed (Scheme 6A) (Deden et al., 2020). Fluorescent biaryl-substituted isoxazoles 26 and 27 are obtained in the sense of a chromophore approach. Indeed, p-bromophenyl acetylene or p-bromobenzoyl chloride can be successfully employed in a sequential catalyzed Sonogashira-cyclocondensation-Suzuki process.
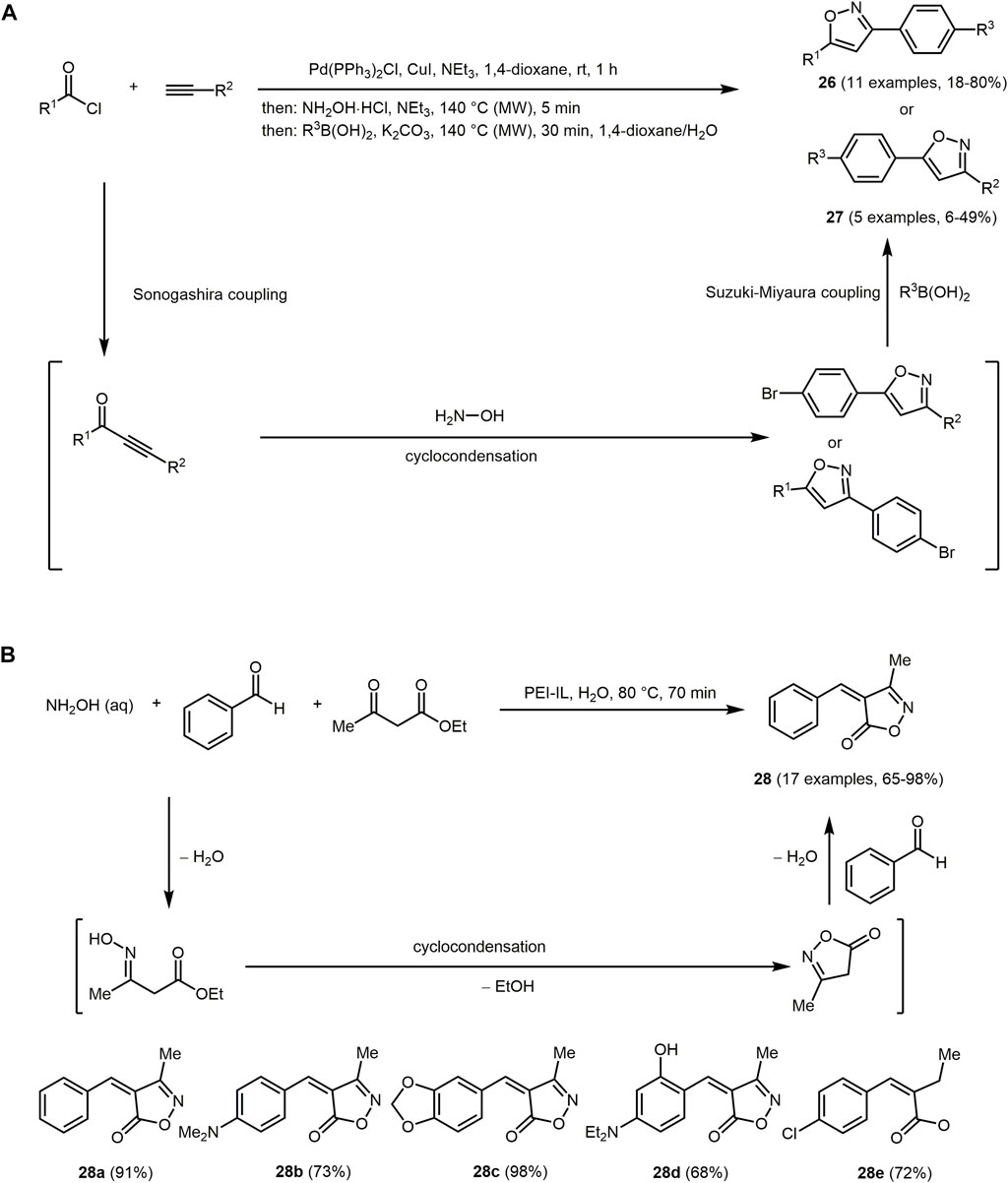
SCHEME 6. (A) Synthesis of biaryl-substituted isoxazoles 26 and 27 via a coupling-cyclocondensation-coupling (C3) sequence (Deden et al., 2020). (B) Enzyme-catalyzed three-component isoxazol-5(4H)-one 28 synthesis and selected fluorophores (Oliveira et al., 2021).
The representatives with donor-acceptor substitution pattern strongly fluoresce in solution (Supplementary Material S6). The absorption spectrum of 26a, which contains the biaryl substituent in 3-position, is hypsochromically shifted (λmax,abs = 294 nm). Compound 26a exhibits the highest molecular absorption coefficient (ε = 66,000 M−1cm−1) and Stokes shift (Δ
Another interesting catalytic approach for the generation of isoxazol-5(4H)-one is catalysis by enzymes. Polyethylene imine (PEI) derivatives are synthetic enzymes with good catalytic activities for various reactions (Vasylyev et al., 2006; Abu-Reziq et al., 2008; Avenier and Hollfelder, 2009). The benefit of this catalyst system is that it can be recycled up to 15 times without any noticeable loss of catalyst activity. Mechanistically, the condensation reaction of hydroxylamine and ethyl acetoacetate is followed by cyclization. The resulting isoxazolones undergo a Knoevenagel-type condensation with aromatic aldehydes to form isoxazol-5(4H)-ones 28 in good to excellent yields (Scheme 6B) (Oliveira et al., 2021).
The shown derivatives 28 fluoresce in solution and they also display positive solvatochromicity. Thus, small Stokes shifts are observed in non-polar solvents and medium to large Stokes shifts in polar solvents. In general, the chromophores have large molar extinction coefficients and low fluorescence quantum yields. In particular, the fluorescent derivative 28d represents a promising candidate as a probe for bioimaging due to its ability to selectively stain early endosomes in living cells.
Another push-pull chromophore, where the isoxazole scaffold acts as the electron acceptor and the aromatic ring as the donor, is 4-(arylmethylene)-5-oxo-4,5-dihydroisoxazole-3-carboxylic acid 29. The reaction proceeds via a two-step one-pot reaction starting from aromatic aldehydes, diethyl acetylenedicarboxylate and hydroxylamine-O-sulfonic acid (Scheme 7A) (Tasior et al., 2021). The reaction is initiated by a Michael addition of hydroxylamine to diethyl acetylenedicarboxylate. The formed enamine then reacts with aromatic aldehydes and the isoxazole derivatives are obtained by elimination of the sulfur trioxide and subsequent transesterification.
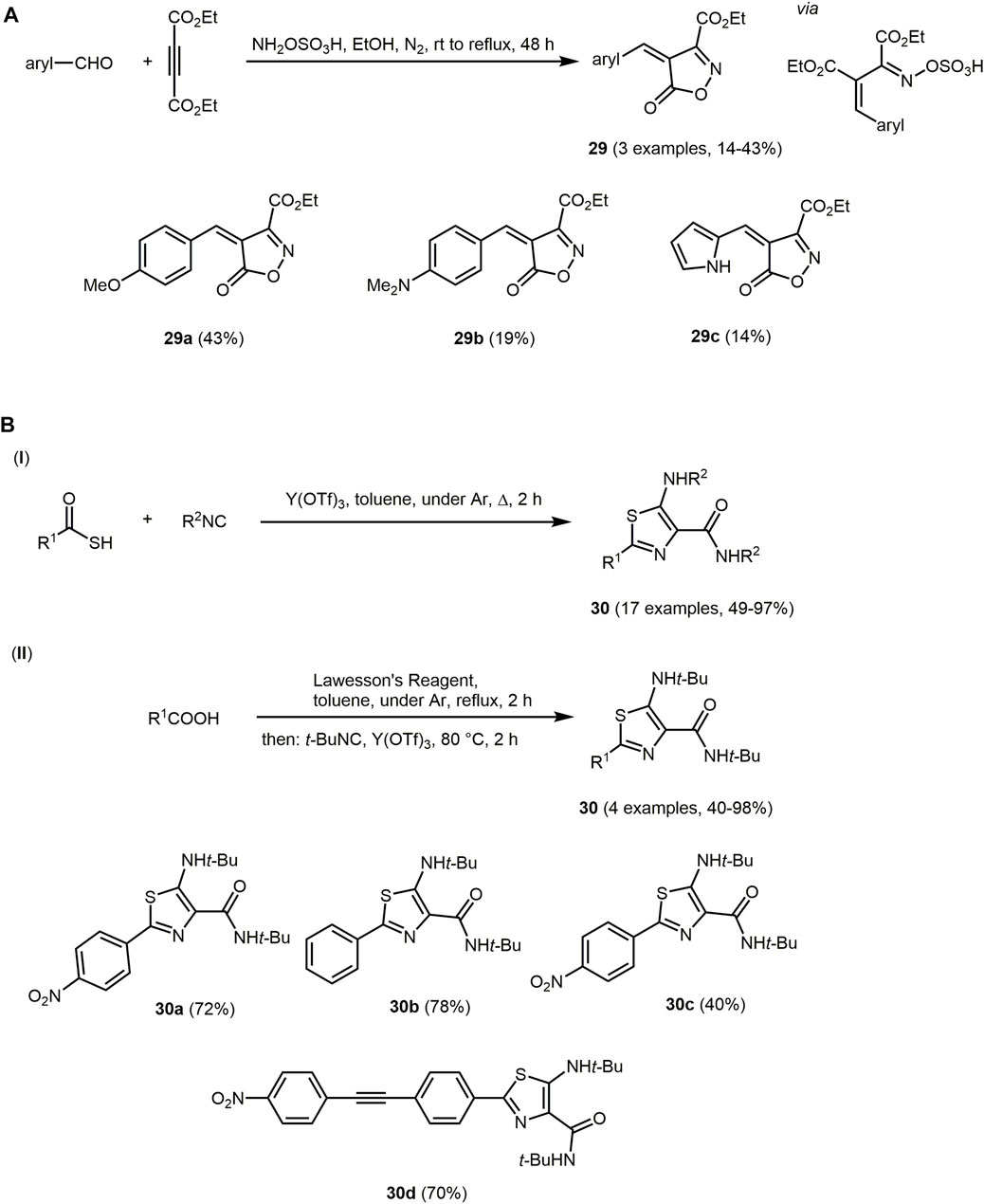
SCHEME 7. (A) Synthesis of isoxazol-5-one 29 via a two-step one-pot reaction and the three obtained fluorophores (Tasior et al., 2021). (B) One-step synthesis of 5-amino-4-carboxamidothiazoles 30 based on the chromophore approach and the synthesized chromophores (Tong et al., 2017).
All isoxazole derivates 29 are non-emissive, probably due to thermal relaxation caused by rotation of the methine bridge. The absorption maxima of 29b and 29c are bathochromically shifted (λmax,abs = 491 and 435 nm, respectively) in comparison to the maxima of 29a (λmax,abs = 400 nm) caused their stronger electron-donating character.
Another five-membered ring containing a nitrogen and a sulfur atom is thiazole. The thiazole core is found in naturally occurring compounds such as vitamin B1, penicillins and dolastatin analogues. The latter are cytostatic drugs (Maderna et al., 2014), which can possibly be used in the treatment of cancer (Bhat et al., 2009; Halasi et al., 2010; Anuradha et al., 2019; Sharma et al., 2020). In general, thiazoles show biological activity and are therefore of great interest for medicinal chemistry (Kashyap et al., 2012; Mahmoodi and Ghanbari Pirbasti, 2016; Hussein and Turan, 2018). Furthermore, organic semiconductors based on thiazole for organic electronics are known (Lin et al., 2012a; Lin et al., 2012b). The synthesis of thiazoles proceeds via MCR, for example via the Hantzsch reaction or via cyclization/oxidation of a corresponding peptide precursor in a biomimetic approach (Hantzsch, 1881; Evans et al., 1979; Boden and Pattenden, 1994; Videnov et al., 1996). Thiazoles can also be prepared via condensation of thiocarboxylic acids with isocyanides (Scheme 7B I) (Tong et al., 2017). 5-Amino-4-carboxamidothiazoles 30 obtained by the triflate-catalyzed reaction display interesting fluorescence properties as potential ESIPT chromophores. Alternatively, thiazole-based chromophores 30 are also produced by reacting a suspension of carboxylic acid with Lawesson’s reagent in boiling toluene followed by addition of an isocyanide and Y(OTf)3 in a one-pot fashion (Scheme 7B II).
5-Amino-4-carboxamidothiazoles 30 (λmax,abs = 333–460 nm) possess high extinction coefficients (ε > 104 M−1 cm−1) and fluoresce with violet, blue, green to yellow color depending on the substitution pattern (λmax,em = 393–558 nm). Upon UV excitation, compound 30a emits in the green region, 30b in the in violet to blue region and 30c as well as 30d in the yellow region. An aryl substituent in 2-position with electron-withdrawing and electron-donating antiauxochromes and auxochromes can significantly increase the quantum yield. The quantum yield can also be increased by expanding the conjugated system via group R1. The insertion of a multiple bond between the thiazole ring and further arenes in substituent R1 causes a bathochromic shift. In particular, nitro-phenyl substituted thiazoles can be excited with visible light and fluoresce with large Stokes shifts, high quantum yields and pronounced solvatochromism. These dyes have potential for the double ESIPT process.
Indoles are desirable due to their widespread medical use (Kang et al., 2009; Kochanowska-Karamyan and Hamann, 2010; Kaushik et al., 2013; Álvarez et al., 2013; Zhang et al., 2015a; Zhang et al., 2015b; Parisi et al., 2015; Hu et al., 2017) and their occurrence in many natural products (Lindquist et al., 1991; Pettit et al., 2002; Cruz-Monserrate et al., 2003; Simon and Petrášek, 2011). For instance, indole constitutes the core of many alkaloids, some hormones and also dyes. Among indole dyes indigo is the most prominent representative (Amat et al., 2011). Considering indoles photophysically, the heterocycle displays fluorescence and strong phosphorescence (Bünau and Birks, 1970). The absorption and emission maxima of indole derivatives in aqueous solution lie typically at 270 and 355 nm, respectively (Bridges and Williams, 1968). Similar absorption and emission values are found for indolylmalonamides 31. In addition, when irradiated with long-wave UV light (
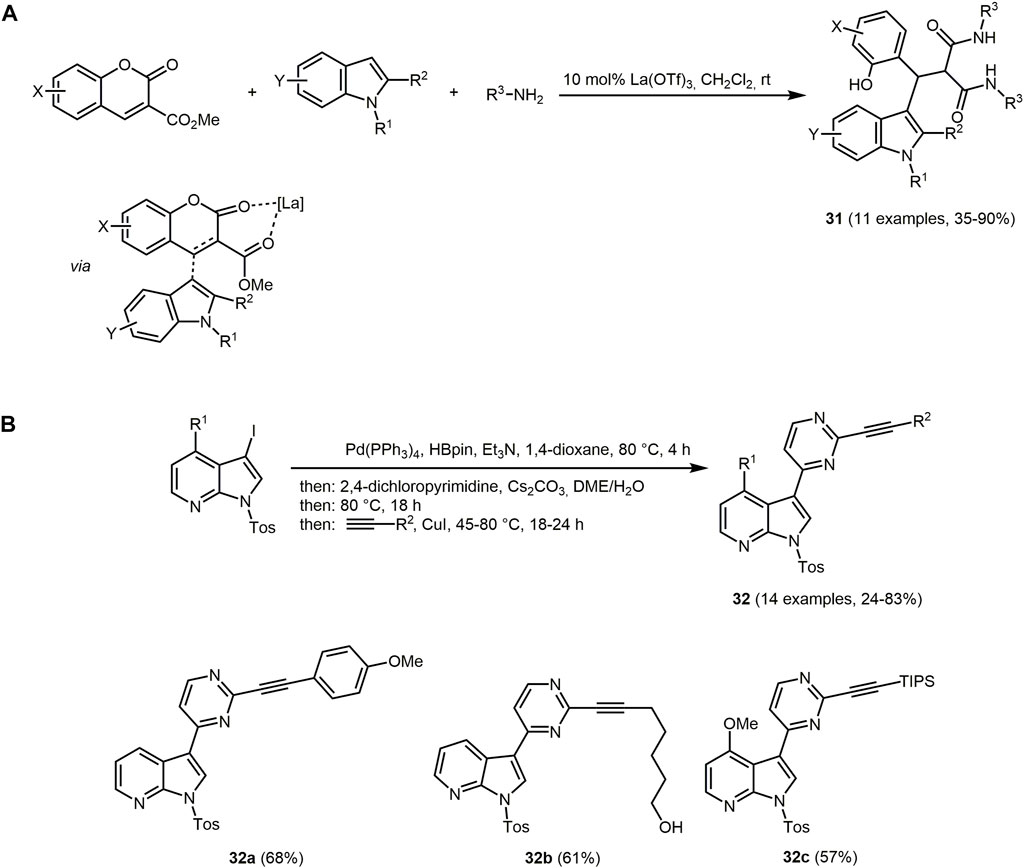
SCHEME 8. (A) Three-component synthesis of indolylmalonamides 31 (Jennings et al., 2016). (B) Sequentially catalyzed consecutive three-component Masuda–Suzuki–Sonogashira synthesis of 2-alkynyl-4-(7-azaindol-3-yl) pyrimidines 32 and selected fluorophores (Drießen et al., 2021).
The sequentially Pd-catalyzed three-component Masuda-Suzuki-Sonogashira reaction combines three Pd-catalyzed processes (borylation, arylation, and alkynylation) in a one-pot fashion to give fluorescent 2-alkynyl-4-(7-azaindol-3-yl) pyrimidines 32 as illustrated with N-tosyl 3-iodo-7-azaindoles, 2,4-dichloropyrimidines and terminal alkynes as starting materials (Scheme 8B) (Drießen et al., 2021).
The UV/Vis spectra of 2-alkynyl-4-(7-azaindol-3-yl) pyrimidines 32 show absorption maxima at λmax,abs = 293–296 nm with molar extinction coefficients ranging between 23,100 and 43,900 L mol−1 cm−1 for aliphatic substituents and 48,000 and 77,000 L mol−1 cm−1 for aromatic substituents, respectively. Electron-donating substituents R2 red-shift the absorption maxima to longer wavelengths. Most of the compound fluoresce with emission maxima at about 447 nm with high Stokes shifts (Δ
Amine-appended spiro[indoline-3,4′-pyridines] 33, for example, can be applied as ON-OFF chemosensors for Cu(II) ions via a fluorescence response. These chemosensors show high selectivity and have already been applied for imaging Cu(II) ions in human hepatocellular liver carcinoma cells. Spiro[indoline-3,4'-pyridine] 33 are prepared via a one-pot four-component reaction involving dialkyl but-2-ynedioate, primary amines, isatin, and malononitrile (Scheme 9A) (Mondal et al., 2018). The environmental benign reaction is catalyzed by iodine and carried out in aqueous ethanol solution. The proposed mechanism suggests activation of the C-3 carbon of isatin by iodine. The isatin-iodine complex reacts with malononitrile via Knoevenagel condensation. In the presence of the iodine catalyst, the nucleophilic attack of the primary amine occurs. Addition of dialkyl but-2-ynedioate initiates the intramolecular Michael addition. Subsequently, chromophores 32 are formed by proton tautomerization.
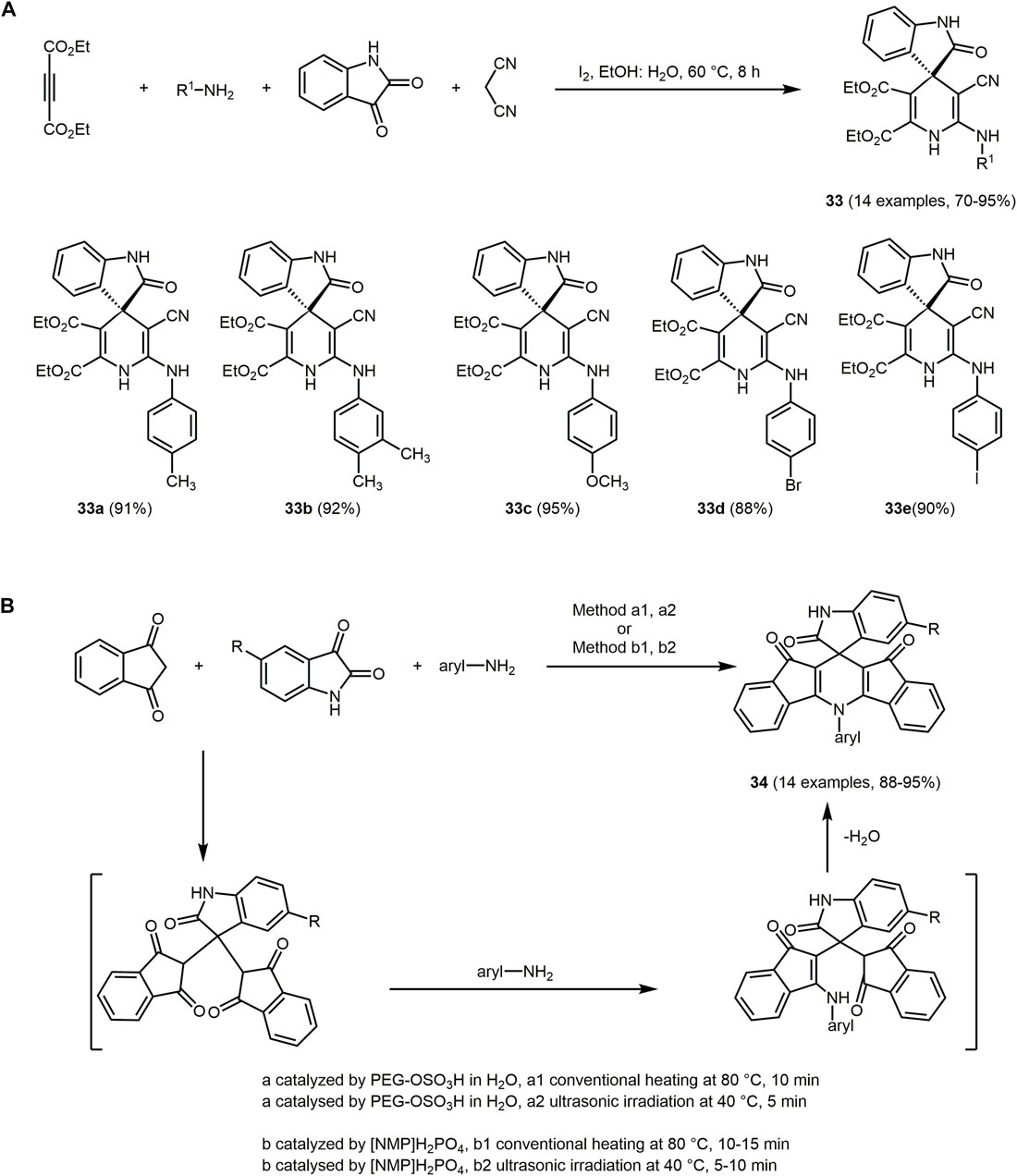
SCHEME 9. (A) Amine-appended spiro[indoline-3,4′-pyridine] ON–OFF chemosensor 33 and selected derivates (Mondal et al., 2018). (B) MCR synthesis of spiro[diindenopyridine-indoline]triones 34 with different catalyst systems (PEG-OSO3H or [NMP]H2PO4) under conventional heating and ultrasonic irradiation (Sindhu et al., 2015).
The chromophores 33 display strong emission in DMSO in a range of 458–493 nm with large Stokes shifts (Δ
Further spiro indoles 34 can also be generated under sustainable conditions. The three-component reaction of 1,3-indanediones, isatins, and aromatic amines is catalyzed either by PEG-OSO3H or by [NMP]H2PO4 to produce spiro[diindenopyridine-indoline]triones 34 under conventional heating and ultrasonication (Scheme 9B) (Sindhu et al., 2015). The PEG-OSO3H is a polymeric acid-surfactant based catalyst that can be recycled and reused without significant loss of activity. Implementing of acidic ionic liquid [NMP]H2PO4 as a catalyst, solvents are not required as it also functions as the medium. The condensation reaction proceeds with a variety of aromatic amines and different isatins with excellent yields (88%–95%) following the chromophore approach.
All dyes 34 are deep red with broad absorption bands with maxima between 264 and 274 nm and they show strong fluorescence in methanol in a range of 282–596 nm with large Stokes shifts (Δ
As indoles, also oxindoles show considerable biological activity (Silva and Pinto, 2001; Peddibhotla, 2009; Kaur et al., 2016; Saraswat et al., 2016). Thus, fluorescent triazolylspirocyclic oxindole derivatives 35 and 36 containing pyran and 1,2,3-triazole moieties possess antimicrobial activity (Kerru et al., 2021). The synthesis proceeds via an one-pot domino reaction of 1-(prop-2-ynyl)indoline-2,3-dione, cyclic 1,3-diketones, malononitrile, and various arylazides in diazabicycloundecene-based ionic liquids ([DBU-H]OAc or [DBU-Bu]OH) under ultrasonic irradiation (Scheme 10A) (Singh et al., 2014). Mechanistically, a triazole intermediate is first formed by Cu-catalyzed alkyne-azide cycloaddition between 1-(prop-2-ynyl)indoline-2,3-dione and arylazide in the presence of in situ generated Cu(I). Knoevenagel condensation with malononitrile and subsequent Michael addition with the cyclic 1,3-dicarbonyl compound and cyclocondensation gives rise to the target structures in excellent yields.
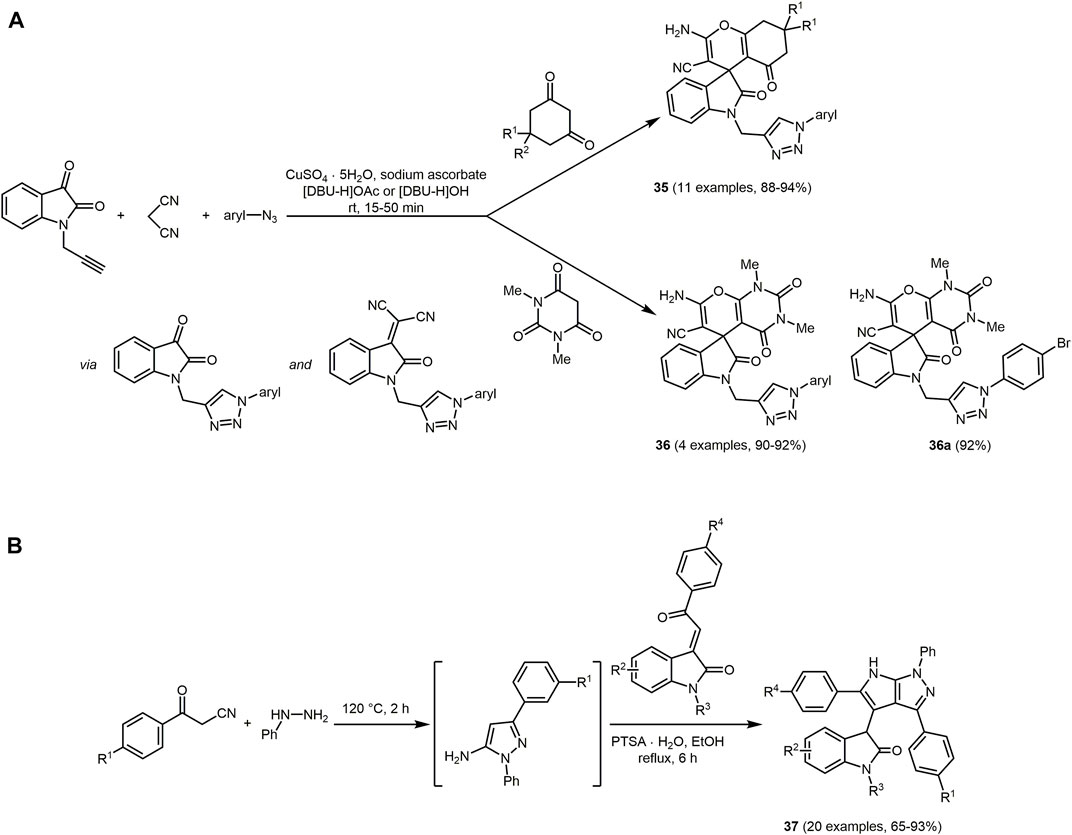
SCHEME 10. (A) Domino reaction for synthesis of triazolyl spirocyclic oxindoles 35 and 36 in the sense of the chromophore concept and the most potent fluorescent, antibacterial triazolyl spirocyclic oxindole 36a (Singh et al., 2014) (B) Sequential one-pot synthesis of oxindole bearing pyrrolo[2,3-c]pyrazoles 37 (Nazeri et al., 2020).
All dyes 35 and 36 (λmax,abs = 239–287 nm) exhibit fluorescence in methanol in a range from 366 to 417 nm with large Stokes shifts (Δ
Hybridizing several biologically active moieties in a single molecule via MCR also applies to chromophore 37, a scaffold consisting of oxindole, pyrrole, and pyrazole (Jamwal et al., 2013; Karrouchi et al., 2018). Oxindole bearing pyrrolo[2,3-c]pyrazole 37 is prepared by an acid-promoted sequential three-component reaction between benzoylacetonitriles, phenylhydrazine, and 3-phenacylideneoxindoles in the sense of the chromophore approach (Scheme 10B) (Nazeri et al., 2020). In the first step, phenylhydrazine reacts with benzoylacetonitrile via cyclocondensation. This is followed by Michael addition with 3-phenacylideneoxindoles and concluded by cyclocondensation to give the desired products.
Absorption maxima of the violet fluorescent compounds 37 can be detected at around 220 and 355 nm. Electron-donating groups on the aromatic ring of the pyrrolo[2,3-c]pyrazoles display a slight redshift causing a decrease of the absorption and photoluminescence intensity.
Isoindoles are likewise important scaffolds of natural products and pharmaceuticals (Speck and Magauer, 2013; Kaur Bhatia, 2017; Csende and Porkoláb, 2018). The 3-substituted isoindolinone derivatives 38 and 39 have potential as cell sensors or drug carriers. The reaction proceeds as a Lewis acid-catalyzed, solid-phase MCR between chiral β-keto lactam, an aldehyde, an isocyanide, and a dienophile mediated by microwave energy. The synthesis of chromophore 38 employs immobilized aldehydes on a solid phase, whereas 39 uses immobilized dienophiles (Supplementary Material S7) (Massarano et al., 2020).
The 3-substituted isoindolinone derivatives 38 and 39 display significant fluorescence with large Stokes shifts (Δ
Blue-luminescent 4-aryl-1H-benzo[f]isoindole-1,3(2H)-diones 40 are formed by consecutive pseudo three-component synthesis of arylpropiolic acids and amines in the sense of the chromophore concept (Scheme 11A) (Denissen et al., 2017b). Arylpropiolic acids are in situ activated by n-propylphosphonic acid anhydride (T3P®) to give anhydrides. Intramolecular Diels–Alder reaction followed by aromatization forms the tricyclic anhydrides that are reacted with amines to give a substance library of tricyclic imide target compounds in 26% and 95% yield.
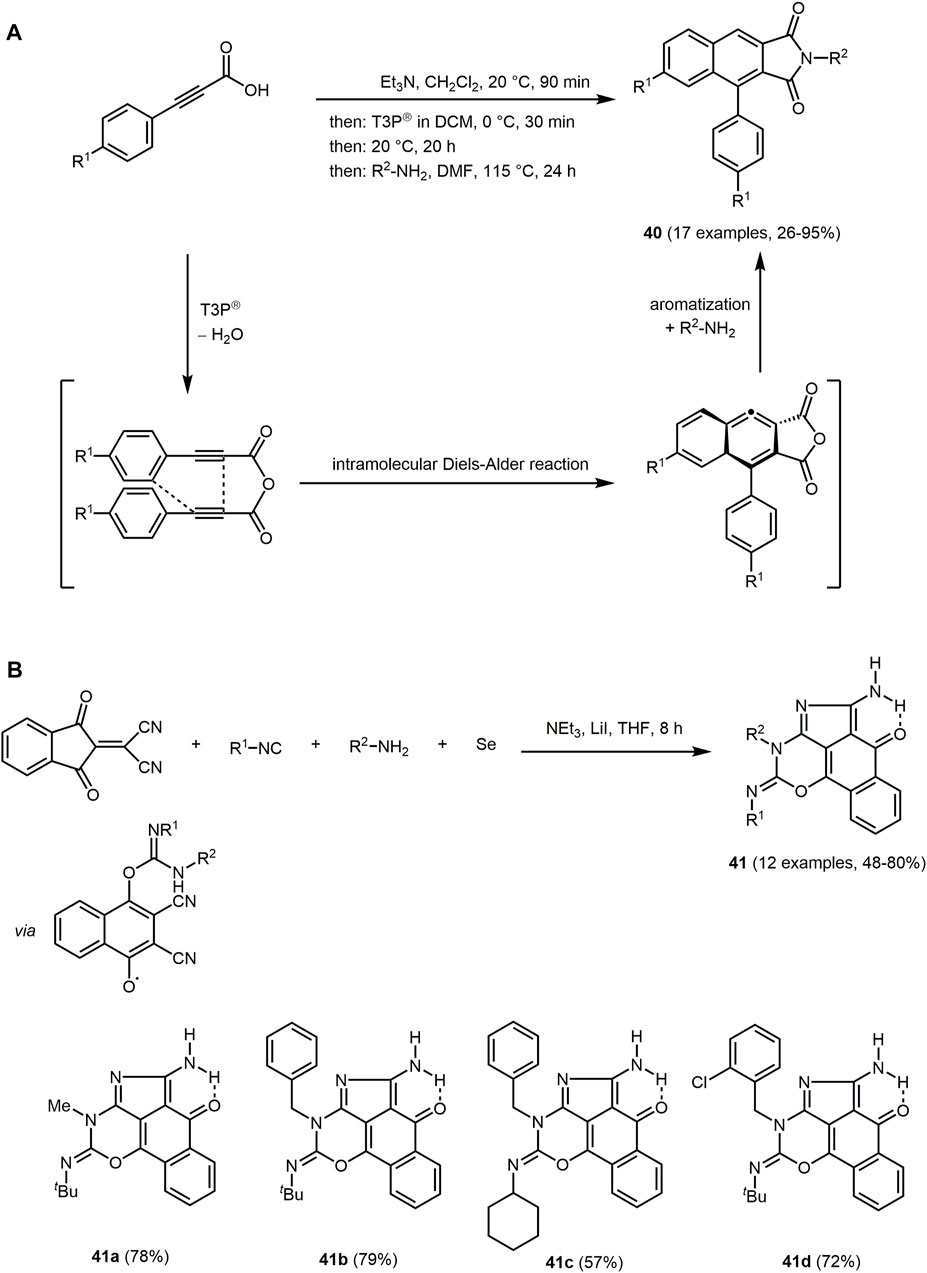
SCHEME 11. (A) Diversity-oriented one-pot process for the synthesis of 4-aryl-1H-benzo[f]isoindole-1,3(2H)-diones 40 (Denissen et al., 2017b). (B) Iodine catalyzed, selenium assisted sequential multicomponent synthesis of benzo-oxazino-isoindoles 41 and selected examples (Sedighian et al., 2021).
Intensive blue and greenish luminesce can by observed for several dyes 40 in solution at low concentration (Supplementary Material S8).
The UV/Vis spectra of the 4-aryl-1H-benzo[f]isoindole-1,3(2H)-diones 40 display two distinct absorption maxima in a range from 259 to 274 nm (ε ≈ 55,000 L mol−1 cm−1) and 359–379 nm (ε ≈ 3,500 L mol−1 cm−1). Electron-donating substituents R1 shift the absorption and emission maxima to longer wavelengths, accompanied by a significant increase in luminescence as seen for dye 40b (Supplementary Material S8). Suppression of luminescence can also be caused by free rotation of the aryl substituent on the imide. Therefore, 4-aryl-1H-benzo[f]isoindole-1,3(2H)-diones 40 also possess AIE, where the intramolecular motion is suppressed upon aggregation. For instance, the fluorescence quantum yield of compound 40a thereby increases more than eightfold in the solid state compared to emission in solution.
Another approach to access chromophores with an isoindole core proceeds via sequential MCR of the Knoevenagel adduct of ninhydrin and malononitrile, isocyanide, amine, and elemental selenium. The iodide-catalyzed process very likely involves a radical ring enlargement and furnishes luminescent benzo-oxazino-isoindole derivates 41 under mild reaction condition according to the chromophore concept (Scheme 11B) (Sedighian et al., 2021). Due to intramolecular hydrogen bonding, compounds 41 exhibits keto-enol tautomerism.
The dyes 41 are intensively luminescent. The absorption behavior in different protic and aprotic solvents shows minor changes in the absorption maxima (as detected at about 490 nm for derivative 41b). In comparison, the emission spectrum displays a clear blueshift in methanol compared to the emission maxima in aprotic solvents. This shift might be attributed to hydrogen bonding of dyes 41 with hydrogen bond accepting solvents, lowering the ground state and increasing the excited state energies of the chromophores, resulting in a hypsochromic shift (Zakerhamidi and Sorkhabi, 2015).
Pyridoindolizine are particularly interesting to their biological properties as also indolizines (Sharma and Kumar, 2014; Venugopala et al., 2017). Pyrido[2,3-b]indolizine-10-carbonitriles 42 and 43 are formed by pseudo three-component reactions of N-(cyanomethyl)pyridinium salts with enaminones or vinamidinium perchlorates, respectively (Supplementary Material S9) (Sokolova et al., 2019). The proposed mechanism commences with a base-promoted dimerization of pyridinium salt. The elimination of pyridinium hydrochloride and aromatization leads to the formation of aminoindolizine, which condenses with 1,3-dielectrophiles to produce pyridoindolizines 42 or 43.
Both pyridoindolizines exhibit strong green fluorescence with maxima in a range from 448 to 490 nm and with quantum yields of up to 0.81.
4 Six-membered heterocycles
4.1 Oxygen heterocycles
Chromenes can be regarded as benzo[b]pyrans. Depending on the positioning of the double bond in the pyran ring, two isomers arise, 2H-chromene and 4H-chromene. Coumarins are derived from 2H-chromenes, however, by oxidation of the 2-position to the oxidation state of an acid derivative, they are lactons. Coumarins absorb blue-green light and have found application as laser dyes (Winters et al., 1974; Abdel-Mottaleb et al., 1992; Yang et al., 2007), in chemosensors (Yao et al., 2009; Jung et al., 2011), in OLEDs (Puthumana and Damodaran, 2018), or in DSSCs (Hara et al., 2003; Wang et al., 2007; Seo et al., 2011). In addition, 4H-chromenes and coumarins are represented in a wide spectrum of pharmaceuticals (Montagner et al., 2008; Smyth et al., 2009; Thakur et al., 2015; Rawat and Verma, 2016; Ngabaza et al., 2017; Soni et al., 2019; Mishra et al., 2020). Due to their applications in the biomedical field, their preparation by sustainable methods for minimizing the usage and generation of toxic organic substances has become increasingly important. Therefore, several green methods have been reported for the synthesis of compounds with a coumarin or chromene core (Molnar et al., 2020; da Silveira Pinto et al., 2018; Ghosh et al., 2015; Mekheimer et al., 2010).
An interesting sustainable approach is the three-step one-pot synthesis of α-acyloxy carboxamides 44 by Passerini reaction in sodium phosphate buffer solution as a medium. The three steps of the sequence starting from salicylic alcohol derivatives include a Trametes versicolor laccase catalyzed aerobical oxidation, aldol condensation and 6-O-glucose ester promoted Passerini MCR. By modification of alcohols, aldehydes, or isocyanides, α-acyloxy carboxamides containing a coumarin scaffold 44 are obtained in variable yields (Scheme 12A) (Paprocki et al., 2020). The synthesis can also be started with the third step employing coumarin-3-carboxylic acids as starting materials in the terminal Passerini reaction.
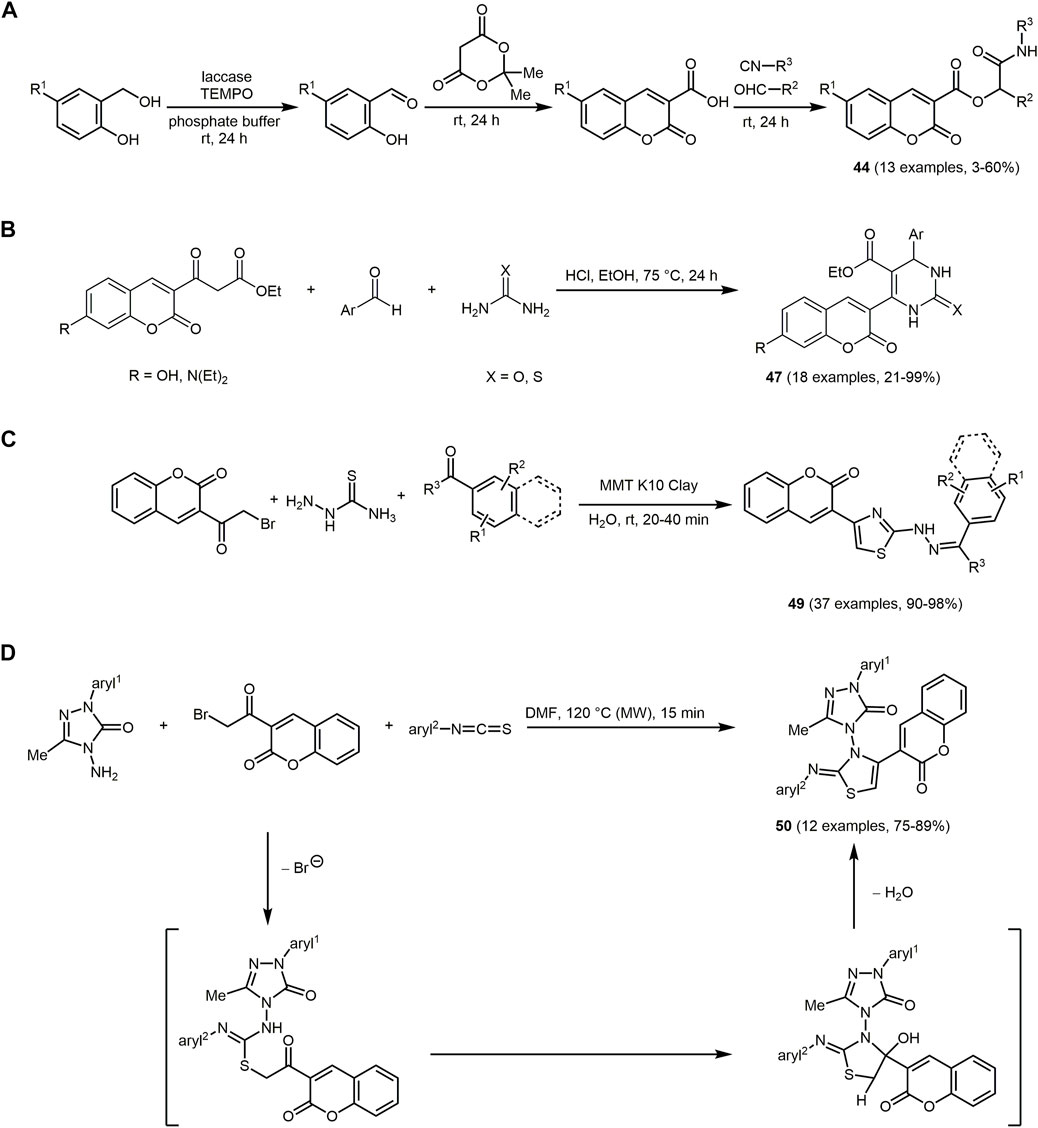
SCHEME 12. (A) Three-step one-pot synthesis of α-acyloxy carboxamides 44 (Paprocki et al., 2020). (B) Biginelli reaction to synthesize 3,4-dihydropyrimidin-2(1H)-one/thiones 47 in the sense of the scaffold concept (Vitório et al., 2015). (C) MMT K10 clay catalyzed three-component synthesis of diversified HTC derivatives 49 (Godugu et al., 2020). (D) Microwave-induced synthesis of coumarin-3-yl-thiazol-3-yl-1,2,4-triazolin-3-ones 50 (Shaikh et al., 2018).
The obtained fluorescent α-acyloxy carboxamides 44 (λmax,em = 415 nm) are well-suited as probes for enzyme activity profiling.
Another approach to produce coumarin-based dyes proceeds via condensation of 3-acetyl coumarin with acetyl chloride, an aromatic aldehyde, and acetonitrile to obtain blue light emitting fluorescent dyes named ‘Beta Fluors’ 45 (Supplementary Material S10) (Soumya et al., 2014). Phenyl boronic acid acts as a green catalyst for this reaction.
All chromophores 45 absorb with longest wavelength maxima between exhibit 303–346 nm and emit blue light from λmax,em = 382–436 nm with large Stokes shifts.
The three-component reaction of 3-acetyl-7-(diethylamino)-2Hchromen-2-one, 4-chlorobenzaldehyde, ethyl cyanoacetate provides D-π-A based coumarin-pyridone conjugate (CPC) 46 with a yield of 87% (Supplementary Material S11) (Manjunatha et al., 2022).
The absorption and emission spectra of the prepared CPC 46 are located in the range of 451–460 nm and 532–549 nm, respectively. Apart from the large Stokes shift, the molecule exhibits positive solvatochromic behavior. The peak at 490–510 nm in the solvatochromic absorption spectrum can be attributed to intramolecular charge transfer (ICT) from the donor (coumarin building block) to the acceptor (pyridone building block). Due to its special photophysical and electrochemical properties, this could be utilized as a fluorescent labeling agent not only for the visualization of latent fingerprints on various surfaces, but also as a detection of nitrite (NO2) via cyclic voltammetry (CV) and chronoamperometry (CA).
Via a Biginelli multicomponent reaction coumarin–dihydropyrimidinone dyads 47 starting from coumarin β-ketoester derivatives, various aldehydes and (thio)urea can be formed with yields ranging from 21% to 99% (Scheme 12B) (Vitório et al., 2015).
The synthesized chromophores 47 exhibit blue fluorescent properties, which can be slightly affected by the electronic properties of the aryl residue on the dihydropyrimidinone moiety, although there is no direct conjugation with the coumarin core. Based on the internal charge transfer process, the 3,4-dihydropyrimidin-2(1H)-one/thione, are promising candidates for novel chemical and biological probes in addition as useful pH indicators. As a selective Hg2+ chemosensor, chromenone-pyrazoles derivatives 48a and 48b can be applied, which are formed via a solvent-free one-pot sequence of salicylaldehyde derivative, 4-hydroxy-6-methyl-2H-pyran-2-one and hydrazine formed using SrFe12O19 as a catalyst. Through an one-pot multicomponent reaction using SrFe12O19 chromenone-pyrazole derivatives can be synthesized (Supplementary Material S12) (Ziarani et al., 2022).
The fluorescence properties of the prepared chromenone-pyrazole derivatives 48 are strongly determined by the residue on the pyrazole group. Thus, only the prepared non-aromatic introduced residues reveal fluorescence properties. The fluorescence emission of 48a and 48b in ethanol are at λex = 348 nm and λex = 300 nm, respectively.
Also, via simple work-up which even does not require purification via column chromatography, donor–acceptor type hydrazinyl thiazolyl coumarins (HTCs) 49 can be obtained (Scheme 12C). HTCs exhibits antioxidant, antimicrobial, and antibacterial activities (Kalluraya et al., 2001; Arshad et al., 2011; Osman et al., 2012; KhanYusufzai et al., 2017). Only a few MCRs to form HTCs are reported to date (Ibrar et al., 2016; Sujatha and Vedula, 2018). The water-mediated three-component reaction is catalyzed by a reusable, solid acid catalyst (montmorillonite (MMT) K10 clay) (Godugu et al., 2020). The wide variation of aromatic/hetero-aromatic aldehydes and aromatic ketones allows establishing a considerable substance library with excellent yields.
Most HTC derivatives 49 display bright fluorescence in chloroform (λmax,em = 409–511 nm) with large Stokes shifts. Electron-donating groups in para/meta positions of the aromatic ring stemming from aldehydes/ketones result in bathochromic shifts. Furthermore, the electrochemical properties indicate that these compounds can be used as hole transporting materials.
The dyes 50 are chromophores that also contain coumarin and thiazole moieties and can be produced by an efficient microwave-induced, uncatalyzed one-pot reaction following the chromophore concept (Scheme 12D) (Shaikh et al., 2018). Components 50 also possess anticancer activity.
Fluorescence of compounds 50 is detected in the visible blue to green region. The chromophores 50 exhibit a bathochromic shift for both emission and absorption maxima with increasing solvent polarity. In non-protic solvents, the emission maxima is redshifted due to an intermolecular charge transfer process (ICT).
Further access to coumarin-based chromophores can be achieved by Ugi reaction of aromatic aldehydes, diamines, coumarin-3-carboxylic acid, and alkyl isocyanides producing coumarin-3-carboxamides containing lipophilic spacers 51 in the sense of the scaffold approach (Supplementary Material S13) (Balalaie et al., 2012). The Ugi 4CR adducts exhibit bright fluorescence at 544 nm in chloroform.
Chromenopyridinones derivatives are also bioactive (Fayed et al., 2021) and find application in the treatment of bronchial asthma (Ukawa et al., 1985) and they show anticancer activities (Bizarro et al., 2018). Furthermore, these heterocycles can be applied for the construction of chemosensors (Kozhevnikov et al., 2003). The one-pot three-component condensation of 4-hydroxycoumarins with ammonium acetate and 3-formylchromones gives highly substituted chromenopyridinone derivatives 51 in an environmentally benign solid-state melt reaction catalyzed by L-proline (Supplementary Material S14) (Paul and Lee, 2016). Mechanistically, the process can be rationalized by L-proline catalyzed Knoevenagel condensation of 4-hydroxycoumarin and 3-formylchromones via an iminium ion intermediate, followed by cyclocondensation with NH4OAc. The product 50 is thus obtained by a ring opening-ring closing sequence. The process follows the chromophore concept and produces the dyes in high yields (75%–93%).
Dye 52a strongly emits in the range of 450–550 nm and the highest fluorescence intensity was detected in MeOH and the lowest in non-polar solvents. The methyl group in the 6-position of the coumarin ring accounts for the strong emission of component 52a. In addition, electron-donating components as R1 and R2 groups are instrumental for ICT.
Further approaches to sustainable syntheses of chromenopyridine fluorophores following the chromophore concept are one-pot condensation sequences with salicylaldehyde derivatives, various malononitriles and selected O- or N-nucleophiles (Supplementary Material S15) (Zonouzi et al., 2013). This process can be applied to produce chromenopyridine derivatives such as dialkylamino-5H-chromeno[2,3-d]pyrimidin-2-yl-phenols 53, dialkylamino-5H-chromeno[2,3-d]pyrimidin-2-yl-phenols 54 and 4-alkoxy-5H-chromeno[2,3-d]pyrimidines 55.
All dye 53–55 show blue to green fluorescence with broad maxima upon excitation at 290 nm. Furthermore, the structures of the derivates 53a and 54a exhibit interesting, exchangeable, intramolecular H-bonding, which open an access to new phenol containing pharmacophores.
Compounds consisting of a phenazine and chromene core have found application in medicinal chemistry (Laursen and Nielsen, 2004). Blue emission is also observed from regioisomeric benzo[a]chromeno-phenazines 56 and 55 (Harichandran et al., 2018). The condensation of 1,2-phenylenediamine, 2-hydroxynaphthalene-1,4-dione, 2-hydroxy benzaldehydes, and 1,3-diketones catalyzed by IRA-400 Cl exclusively produces products 56, whereas amberlite IR 120 H+ resin as a catalyst leads to a mixture of 56 and 57 (Supplementary Material S16). Advantageously, anion and cation exchange amberlite resins are both reusable. The intermediates are formed by the Knoevenagel condensation of phenylenediamine and 2-hydroxynaphthalene-1,4-dione, 2-hydroxy benzaldehydes and 1,3-diketones. The sequence finally concludes by Michael addition and condensation in the sense of the chromophore approach.
The absorption spectra of dyes 56 and 57 are characterized by two to three distinct maxima, with the longest wavelength absorptions in a range from 414 to 422 nm. The emission maxima of the chromophores 56 and 57 can be detected at wavelengths ranging from 462 to 498 nm and 450–468 nm, respectively. Most dyes 56 give fluorescence quantum yields ranging from less than 0.01 up to 0.10, as also the chromphores 57 reveal quantum yields of around or less than 0.1. The compounds 56a and 57a have shown to be suitable for the detection of Fe(III) and Cu(II) ions and can thus be used as chemical sensors.
An approach to increase sustainability of common reactions is the use of solvent-free syntheses to reduce organic solvents. As mentioned before, the multicomponent synthesis of fluorescent 4H-chromene derivatives 58 can be mediated by amberlite IRA-400 Cl resin, which functions both as the solvent and the reusable catalyst (Supplementary Material S17) (Harichandran et al., 2017). 2-Hydroxybenzaldehydes, 1,3-diketones, and nucleophiles (Nu) react involving by Knoevenagel condensation and subsequent Michael addition in excellent yields.
The UV/Vis spectra of the 4H-chromen dyes 58 display absorption maxima ranging from 252 to 394 nm and emission maxima in a range from 405 to 462 nm, blue fluorescence. Chromophore 58b has been found to be the best fluorophore in the series. Compounds 58a and 58b are the only derivatives containing an electron donating amino group at the R1 position. These chromophores exhibit smaller Stokes shifts and higher fluorescence quantum yield (Δ
Furo[3,2-g]chromen-7-one, better known as psoralen, is a tricyclic donor-acceptor heterocycle consisting of an electron-rich furan and electron-poor α-pyrone fused to a central benzene core. Starting from bromo-triflato-functionalized psoralen donors and acceptors can be specifically coupled by sequentially Pd-catalyzed consecutive Suzuki-Suzuki and Sonogashira-Sonogashira multicomponent reactions to give donor-acceptor psoralens 59 and 60, i.e. orthogonally oriented cruciform structures (Scheme 13) (Geenen et al., 2020). Cross-shaped molecules allow access to functional chromophores with interesting properties resulting from significant changes in the orientation of the transition dipole vector (Zucchero et al., 2010; Le et al., 2015; Xu et al., 2018).
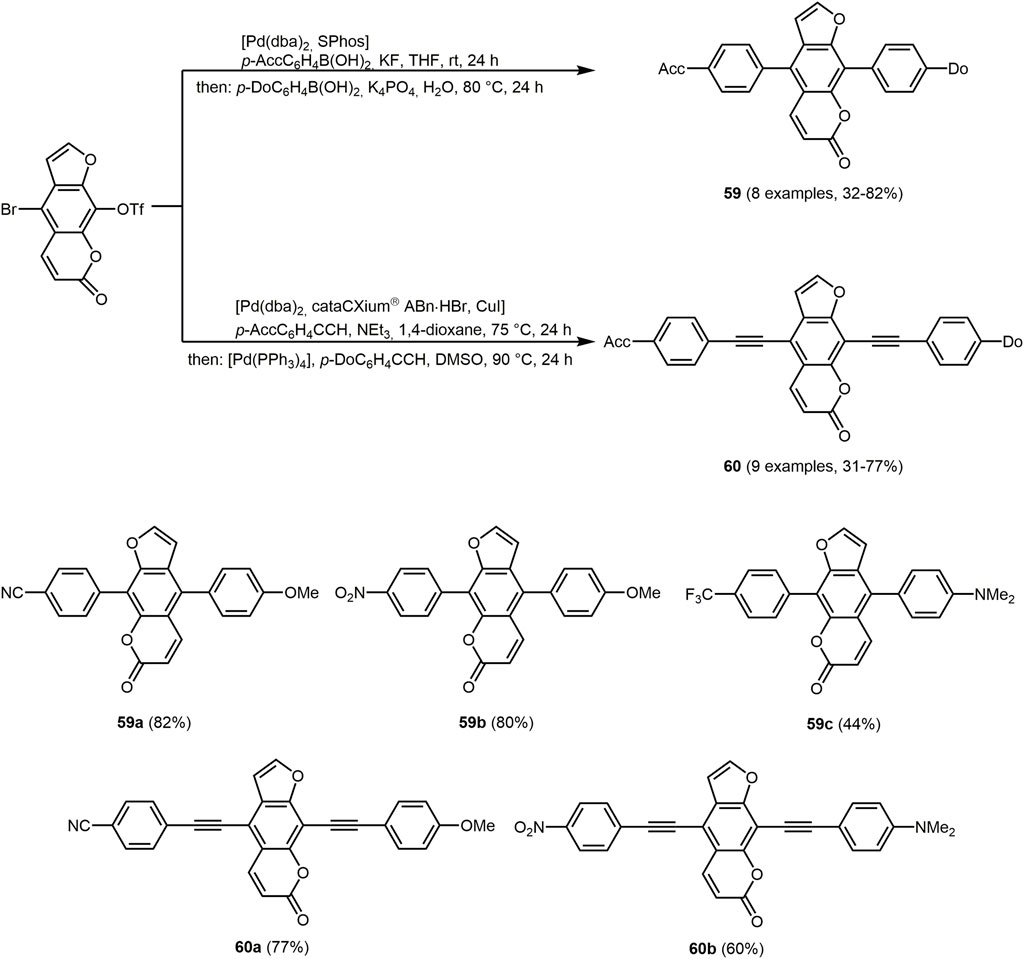
SCHEME 13. Consecutive three-component Suzuki−Suzuki and Sonogashira−Sonogashira synthesis of 8-donor-5-acceptor-substituted psoralens 59 and 60 in the sense of the scaffold concept and selected derivates (Geenen et al., 2020).
Depending on the selection of the donor and acceptor components, the photophysical properties of the 8-donor-5-acceptor-substituted psoralens 59 and 60 can be fine-tuned. The optical properties of the donor-acceptor psoralens are characterized by large Stokes shifts, partially high fluorescence quantum yield in solution, and strong solid-state fluorescence. Of particular interest are observed solvatochromism, acidochromism, and aggregation-induced emission (Supplementary Material S18). In addition, the pronounced charge transfer character of the longest wavelength absorption band is confirmed experimentally and computationally.
Fluorescence of xanthene-containing chromophores 61 can also be observed in natural products (Bhowmik and Ganguly, 2005). Due to their photophysical properties, they are mainly applied in dyes and can function for instance as pH-sensitive fluorescent materials for visualization of biomolecules (Knight and Stephens, 1989; Shabir et al., 2018). In addition, these compounds have various biologically active properties, such as anticancer, antibacterial and antioxidant (Kidwai et al., 2005; Giri et al., 2010; Raschip et al., 2020). The antioxidant activity of 14-aryl-14H-dibenzo[a,i]xanthene-8,13-diones 61 containing a hydroxy group at R2 or R3 was tested using the 2,2-diphenyl-1-picrylhydrazyl radical scavenging assay (Khurana et al., 2012). The electron-donating groups on the aldehyde (R1), as dyes 61b and 61c, enhance this antioxidant characteristics. Possess antioxidant features. 14-Aryl-14H-dibenzo[a,i]xanthene-8,13-diones 61 can be prepared via condensation of aldehydes, 2-hydroxynaphthalene-1,4-diones, and 2-naphthol/2,7-dihydroxynaphthalenes/2,6-dihydroxynaphthalenes catalyzed either by H2SO4 or ionic liquid 1-butyl-3-methylimidazolium hydrogen sulfate ([bmim]HSO4) (Scheme 14A). For xanthenes in general, many green approaches have been reported to involve environmentally benign catalysts such as ionic liquids (Burange et al., 2021). Another advantage of the domino MCR following the chromophore approach is the simple purification by crystallization in ethanol. The synthesized compounds 61 exhibit absorption bands with maxima in the range from 333 to 355 nm with extinction coefficients between 10,800 and 27,500 L mol−1 cm−1.
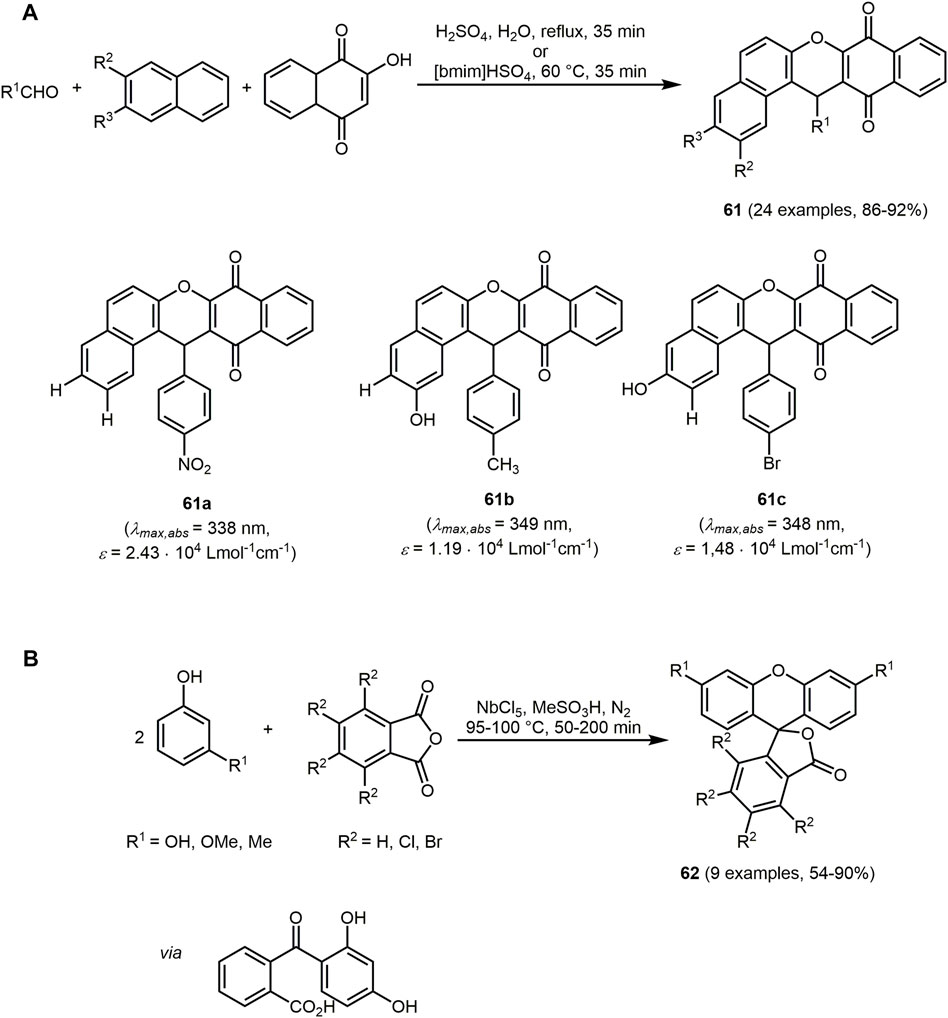
SCHEME 14. (A) Domino synthesis of 14-aryl-14H-dibenzo[a,i]xanthene-8,13-diones 61 and selected fluorophores (Khurana et al., 2012). (B) One-pot synthesis of fluoresceins 62 using NbCl5 as a catalyst (Sacoman Torquato da Silva et al., 2017).
Fluoresceins can also be categorized as xanthene dyes. These are characterized by interesting photophysical properties such as high molar absorptivity, high fluorescence quantum yields, and high photostability. Typically, fluoresceins absorb in the range from 400 to 700 nm (Sjöback et al., 1995; Song et al., 1998). Depending on the pH value, fluorescein is present as an anion, cation, or in its neutral form, which influences the photophysical properties (Martin and Lindqvist, 1975; Diehl and Markuszewski, 1989). This pH dependency can be exploited for the application as an indicator (Kim et al., 2011). The UV/Vis spectra of the fluorescein derivatives 62 were measured in NaOH so that the fluorescein derivatives are present in the dianionic form (pH > 8) (Sacoman Torquato da Silva et al., 2017). The dianionic form shows longest wavelength absorption and emission maxima and highest fluorescence quantum yields due to its conjugated system. The absorption (λmax,abs = 476–512 nm) and emission (λmax,em = 515–525 nm) of dyes 62 lie in the typical range of fluorescein and high fluorescence quantum yields are realized (ΦF = 0.60–0.93). Derivatives containing halogens show a bathochromic shift in emission and absorption behavior as well as a decrease in fluorescence intensity. The fluorescein dye derivatives can be formed by a pseudo three-component reaction of phenol and phthalic anhydride derivatives in the sense of the chromophore approach (Scheme 14B). This MCR can be catalyzed by the electrophilic NbCl5. The reaction proceeds in two steps via twofold Friedel-Crafts reaction and subsequent carbonyl addition. In addition, the interesting photophysical of fluoresceins 62 make them suitable for application in DSSC.
4.2 Nitrogen heterocycles
Pyridine (azine) is found in many bioactive natural products, such as vitamin B6 (Hill et al., 1996) or nicotinamide adenine dinucleotide, which is a pivotal coenzyme in the metabolism (Noctor et al., 2006). Furthermore, pyridine is an important synthetic building block for pharmaceuticals (Chang et al., 2005; Reddy et al., 2006; Altaf et al., 2015; Nagham Mahmood Aljamali, 2021). Pyridine is an excellent moiety for the assembly of fluorescent compounds due to its strong electron withdrawing properties, good rigid structure, and strong coordination ability (Xie et al., 2021). Numerous chemosensors with a pyridine core are reported (Hirano et al., 2000; Jiang and Guo, 2004; Machado et al., 2014; Ma et al., 2017). For instance, a fluorescent chemosensors can be used for the detection of Fe(III) and Hg(II) ions based on an ON-OFF mechanism. The fluorescent 2-amino-6-methyl-4-phenyl-nicotinonitrile 63 is synthesized via multicomponent condensation following the chromophore concept (Supplementary Material S19) (Koner et al., 2012).
The coordination of Fe(III) ions and 2-aminopyridine-based compound 63 results in an increase of the absorption intensity of both bands λmax,abs = 246 and 335 nm. In contrast, Hg(II) ions lead to a decrease in absorption intensity with a slight bathochromic shift. In the presence of Fe(III) and Hg(II) ions, the fluorescence spectrum shows quenching of emission intensity by 81% and 55%, respectively. Quenching is very likely caused by the paramagnetic nature of the Fe(III) ions and by the heavy metal ion effect for Hg(II). Due to the small spectral shift upon emission decrease, photo-induced electron transfer (PET) is a plausible mechanism. It is noteworthy that selective quenching occurs only for Fe(III) and Hg(II) ions, which makes the chromophore a suitable chemosensor for these two metal ions. Iron is involved in many biological processes and its selective detection can be a valuable tool for biological studies (Sigel and Sigel, 1998; Que et al., 2008).
The Groebke-Blackburn-Bienaymé (GBB) three-component reaction of heterocyclic amidines, aldehydes, and isocyanides furnishes α,β-substituted imidazo[1,2-a]pyridines 64 (Scheme 15A) (Burchak et al., 2011), an important source of bioimaging probes due to their pharmacophore. Imidazo[1,2-a]pyridines have been shown to possess antiviral (Gueiffier et al., 1998), antiulcer (Starrett et al., 1989), antipsychotic (Marcinkowska et al., 2016), and antidiabetic activities (Kercher et al., 2007).
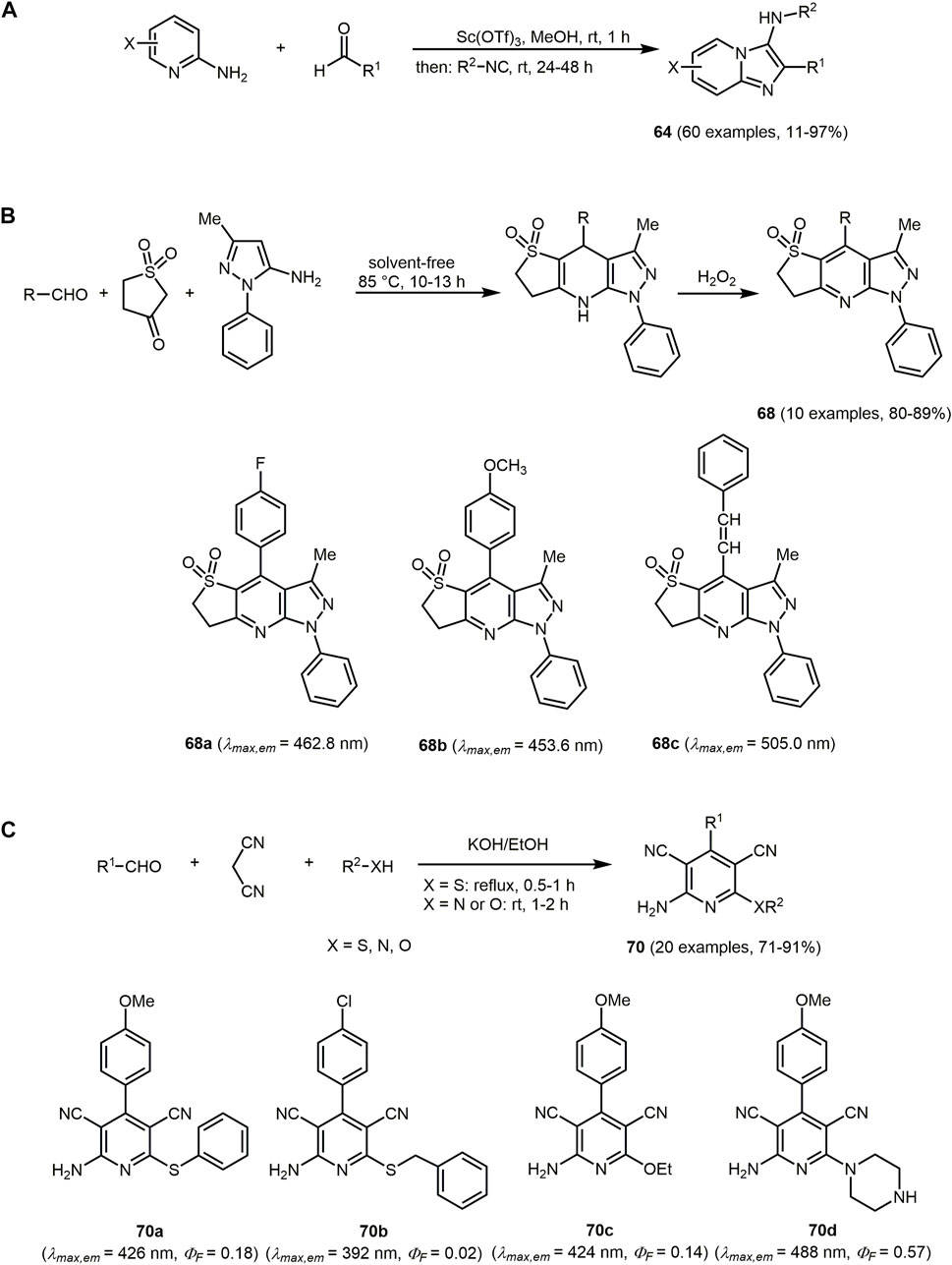
SCHEME 15. (A) Groebke-Blackburn-Bienaymé synthesis of fluorescent α,β-substituted imidazo[1,2-a]pyridines 64 via the chromophore approach (Burchak et al., 2011). (B) Solvent-free MCR synthesis of pyrazole[3,4-b]thieno[2,3-e]pyridine derivatives 68 in the sense of the chromophore approach and selected fluorophores (Yao et al., 2014). (C) Pseudo four-component synthesis of functionalized 2-amino pyridine dyes 70 and selected derivates (Khan et al., 2012b).
In general, the absorption band of imidazo[1,2-a]pyridine is located near 280 nm and an efficient fluorescence band is observed at 370 nm (Stasyuk et al., 2012). The emission properties of the dyes 64 are mainly affected by the amidine and aldehyde building blocks. Thus, the products starting from methyl 2-aminoisonicotinate, methyl 2-aminoisonicotinate, pyrimidin-2-amine, and pyrazin-2-amine fluoresce intensively in solution. All the mentioned imidazo[1,2-a]pyridines exhibit intrinsic fluorescence with a broad range of colors and they are also promising for use in chemosensors. The fluorescence mainly depends on the electron-donating effect and the degree of conjugation of aldehyde moiety (Supplementary Material S20).
The GBB synthesis of imidazo[1,2-a]pyridines 65 proceeds under mild conditions and short reaction times giving good yields after simple non-aqueous workup (Supplementary Material S21) (Khan et al., 2012a; Khan et al., 2012b). In this variant of the three-component condensation, bromodimethylsulfonium bromide (BDMS) serves as the catalyst. Thereby, even sterically demanding amidines can be transformed with good yields. The released water from condensation reacts with BDMS to liberate HBr which protonates the imine. Mechanistically, the key step of the formation of imidazo[1,2-a]pyridines 65 is a [4 + 1] cycloaddition followed an aromatizing 1,3-H shift.
Absorption maxima of the fluorescent compounds 65 can be detected at around 250 and around 335 nm. A chlorine substituent at C2-position of the imidazo[1,2-a]pyridine results in a slight redshift of the absorption maxima. A 2,4-methoxy substituent on the aromatic ring shifts the emission maximum to a longer wavelength (λmax,em = 470 nm).
To synthesize tosylmethyl isocyanide (TOSMIC) fused imidazo[1,2-a]pyridine 66, the GBB reaction is carried out with 2-amino pyridine, TOSMIC and a wide variation of aldehydes in the sense of the chromophore concept (Supplementary Material S22) (Shukla et al., 2022).
The obtained imidazo[1,2-a]pyridine derivatives 66 display a blue color under the UV lamp. Based on the aldehyde component, the fluorescence properties can be modified.
The pseudo three-component reaction of mucobromic acid and two molecules of a series of 2-substituted benzimidazoles provides benzo[4,5]imidazo[1,2-a]pyridine derivates 67 in moderate to good yields. Mechanistically, a nucleophilic substitution of benzimidazoles with mucobromic acid occurs in the presence of the base potassium carbonate. After decarboxylation, Michael addition of another benzimidazole is performed. With the aid of the base, a dehydrohalogenation reaction proceeds and the desired product is obtained by cyclization (Supplementary Material S23) (Yang et al., 2022). Via Michael addition, other NH-containing heterocyclic nucleophiles can also be introduced to the system.
The introduced heterocyclic rings in C1 position of benzo[4,5]imidazo[1,2-a]pyridine 67 lead to the cancellation of the coplanarity of the whole molecule and hinder the strong intermolecular π-π interaction and the tight π-π stacking between the neighboring molecules. These electrostatic interactions are able to constrain intramolecular motion in the solid state, causing an enhancement of fluorescence in the aggregation state. 67a could be used to detect picric acid, a nitroaromatic explosive.
Pyrazole[3,4-b]thieno[2,3-e]pyridines 68 contain pyrazoles and thienyl moieties fused to a central pyridine core and can be accessed by Hantzsch dihydropyridine synthesis followed by a solvent-free oxidative aromatization with H2O2 (Scheme 15B) (Yao et al., 2014). The aromatic substituent of the aldehyde can be electron-withdrawing or electron-releasing and it influences the emission behavior. The dyes 68 feature a blue to green fluorescent donor-π-conjugated acceptor system with emission maxima in a range from 430 to 505 nm.
Pyrene moieties can be placed to pyridines by the chromogenic one-pot pseudo four-component pyridine synthesis starting from 1-acetylpyrene, arylaldehydes, and ammonium acetate in acetic acid to form 4-aryl-2,6-di (pyren-1-yl)pyridines 69 in good yields ranging from 65% to 86% (Supplementary Material S24) (Asaadi et al., 2019).
The dyes fluoresce in a range of 434–464 nm with quantum yields of 0.10–0.17. The fluorescence is increased by electron-donating groups (69c) due to the conjugation of the system, where electron-withdrawing groups (69d) diminish the fluorescence.
Highly substituted pyridines can be formed via potassium hydroxide catalyzed pseudo four-component reaction of aldehydes, malononitrile and nucleophiles, such as thiols, alcohols or amines in the sense of the chromophore concept (Scheme 15C) (Khan et al., 2012b). Mechanistically, one molecule of malononitrile reacts via a Knoevenagel condensation with aldehyde and the other malononitrile undergoes a Michael addition with the previously formed electrophile followed by a concomitant nucleophilic addition to the cyano-substituent of the adduct. The functionalized 2-amino pyridines 70 are formed via cyclization and oxidative aromatization.
The UV/Vis spectra of 2-amino-3,5-dicarbonitrile-6-thio-pyridines 70 display absorption maxima in a range from 329 to 356 nm and emission maxima in a range from 386 to 451 nm. The highest quantum yield in comparison to other thio-pyridine derivatives (ΦF = 0.00–0.05) is detected for compound 70a (ΦF = 0.18), which possesses a strongly electron-donating group at the C4 aryl substituent of the pyridine ring. The amino and oxo pyridine derivatives exhibit quantum yields of 0.27–0.57 and 0.10 to 0.14, respectively. The chromophores are potential candidates as new fluorescent probes or luminescence materials. Furthermore, it was reported that when sterically hindered aldehydes are used not the expected thio-pyridine derivatives but the corresponding 1,4-dihydropyridines (DHPs) were obtained.
Pyridine is the oxidation (dehydrogenation) product of 1,4-dihydropyridine (1,4-DHP), which also occurs in coenzymes nicotinamide adenine dinucleotide (NADH) and nicotinamide adenine dinucleotide phosphate (NADPH). Synthetic derivatives of 1,4-DHP are ubiquitous and cover a wide spectrum of biological activities, including vasodilators (Schachinger et al., 2001; Safak and Simsek, 2006), antibacterials (Huang et al., 2009; Sellamuthu et al., 2017) and antioxidatives. As a drug, DHP is used as a calcium channel blocker in the treatment of hypertension (Young, 1984; Edraki et al., 2009; Poondra et al., 2013). 1,4-DHPs, which are available by Hantzsch synthesis, are also used as dyads in photoinduced electron-transfer systems (Fasani et al., 2006; Jimenez et al., 2009; Al-Awadi et al., 2012).
1,4-Dihydropyridines 71 and 72 are formed via catalyst-free MCR of amine hydrochloride salts or ammonium chloride, aldehydes, and acetals (Scheme 16A) (Sueki et al., 2014). Ammonium chloride and 3,3-diethoxypropionate activated by protons react to give an imine intermediate that tautomerizes to the enamine. This enamine intermediate forms with an aldehyde upon elimination of ethanol an α,β-unsaturated imine. Subsequently, the enamine intermediate reacts with the α,β-unsaturated imine via Michael anellation to form tetrahydropyridine. Upon elimination of ammonia 1,4-DHP is generated. Based on the two approaches A and B, a wide range of various 3,4,5-trisubstituted 1,4-DHPs with ethoxycarbonyl groups at the 3- and 5-positions 71 can be formed (Scheme 16A I). Further modification can be achieved by changing the ethoxycarbonylgroups to other electron-withdrawing groups (EWGs) and the corresponding 3,4,5-substituted 1,4-DHPs 71 are obtained in moderate to good yields in the sense of the chromophore concept (Scheme 16A II).
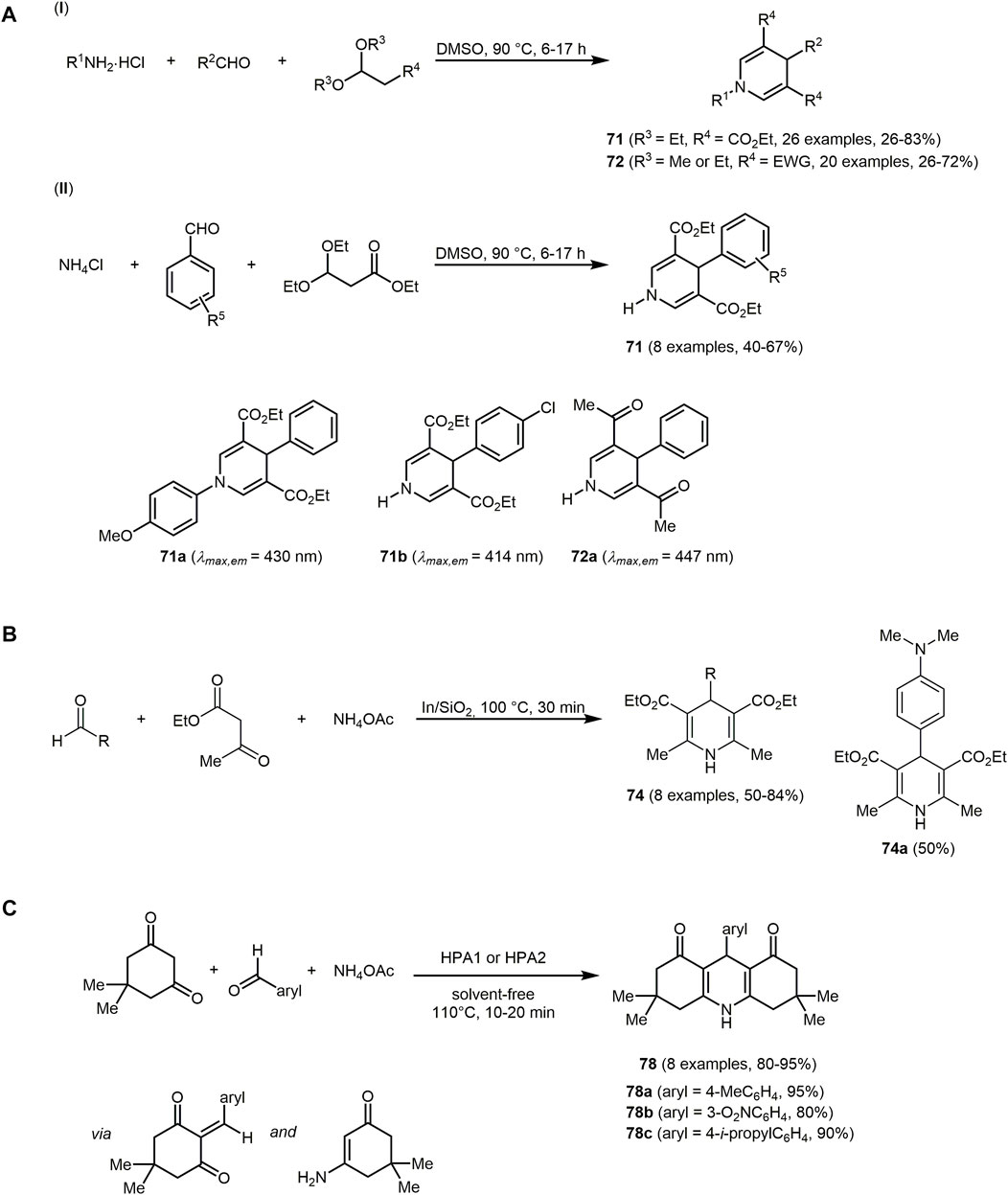
SCHEME 16. (A) Catalyst-free pseudo four-component synthesis of 3,4,5-substituted 1,4-dihydropyridines 71 and 72 and selected fluorophores (Sueki et al., 2014). (B) Synthesis of photoactiv Hantzsch 1,4-DHPs 74 as well as one selected derivate 74a (Affeldt et al., 2012). (C) Pseudo four-component synthesis of 1,8-dioxodecahydroacridines 78 with the green catalysts HPA1 or HPA2 (Baradaran-Sirjani et al., 2018).
The MCR products 71 and 72 exhibit fluorescence in the range from 403 to 542 nm and quantum yields up to 0.94. 1,4-DHPs with extended π-conjugation exhibit emission maxima at longer wavelengths. Photophysical properties such as the fluorescence wavelength and the quantum yield can be varied by modifying the substituents of the 1,4-DHPs.
A green approach to 1,4-DHPs is presented by the one-pot five-component synthesis of 1,2,3-triazole-linked pentasubstituted 1,4-DHPs 73 under ultrasonic irradiation at room temperature or under MW irradiation with PEG-400 as a medium (Supplementary Material S25) (Singh et al., 2013). PEG-400 is an inexpensive, biologically compatible, non-toxic and recyclable solvent. Initially, a CuAAC occurs between aryl azide and the propargylated benzaldehyde derivative, forming a 1,2,3-triazole derivative. Subsequently, the disubstituted 1,2,3-triazole-linked DHPs are produced by the Hantzsch condensation involving Knoevenagel condensation and Michael addition.
All chromophores 73 display three distinct absorption maxima in the UV/Vis spectra, the first around λmax,abs = 230 nm, the second around 255 nm, and the third around 365 nm. Also, the 1,2,3-triazole-linked pentasubstituted 1,4-DHPs show strong fluorescence in solution with emission maxima between 439 and 451 nm. It is worth noting that the substituent on the phenyl ring has only a minor influence on the fluorescence properties. Moreover, the compounds 73 are proven to possess antibacterial, antifungal and antioxidant properties.
The In/SiO2 catalyzed Hantzsch reaction of various substituted aryl aldehydes provides access to blue-green fluorescent 1,4-DHPs 74 (Scheme 16B) (Affeldt et al., 2012)
The UV/Vis spectra of 74 exhibit the absorption maxima at around 350 nm. The emission maxima can be detected in a range of 402–516 nm with large Stokes shifts ranging from 4,200 to 11,000 cm−1. In particular, for derivatives 74a the largest Stokes shift is measured, due to intramolecular charge transfer mechanism in the excited state from the dimethylaniline to the dihydropyridine chromophores.
Similarly, hydroxyphenylbenzoxazole, a heterocycle with interesting photopyhsical properties such as large Stokes shift, ESIPT or dual fluorescence emission, can be introduced as an aldehyde component in the Hantzsch reaction (Rodembusch et al., 2007; Grando et al., 2009). The addition of 5,5-dimethylcyclohexane-1,3-dione and/or ethyl acetoacetate provide 1,4-DHPs 75–77 (Supplementary Material S26) (Affeldt et al., 2014).
The three synthesized 1,4-DHPS 75, 76 and 77 display absorption in the UV region and emission in the blue-green region. The photophysical study reveals that the hydroxyphenylbenzoxazole and DHP fluorophores in the hydroxyphenylbenzoxazole-DHP structure behave independently after excitation. In addition, ESIPT emission can be observed.
The pseudo four-component reaction for the synthesis of polyfunctionalized derivatives of 1,4-DHP and 1,8-dioxodecahydroacridines can be efficiently catalyzed by two Preyssler heteropolyacids, H14[NaP5W29MoO110] (HPA1) and H14[NaP5W30O110] (HPA2), under solvent-free conditions (Baradaran-Sirjani et al., 2018). The reaction is catalyzed by both polyoxometalate anions and cations. The cations activate the carbonyl groups in aromatic aldehydes and dimedones due to their Lewis acidic nature and the anions abstract the α-proton of the dimedone furnishing 1,8-dioxodecahydroacridines 78 in excellent yields based on the chromophore concept (Scheme 16C).
The emission spectra of 78c recorded in different solvents and at different temperatures exhibit a maximum at around 520 nm under all conditions.
Based on a pseudo five-component reaction of cyclopentanone, two molecules of aromatic aldehyde, N-pyridinium substituted ortho-hydroxyaryl methyl ketone and ammonium acetate 2-(ortho-hydroxyaryl)cyclopenta[b]pyridines 79 and 80 are synthesized with yields ranging from 19% to 87% (Supplementary Material S27) (Batalin et al., 2021). For the aromatic aldehyde either benzaldehyde or up to 3 methoxy groups with various substitution patterns on the aromatic ring were furnished, which influenced the yields and the amount of pyridium salt added. The reaction proceeds via a modified Kröhnke reaction (Yan et al., 2007), in which the first step is an aldol condensation between the cyclopentanone and the aromatic aldehyde in the presence of ammonium acetate. The resulting E-cross conjugated dienone undergoes a Michael reaction with the pyridime salt and forms the intermediate illustrated in the Supplementary Material S27, which after elimination of the pyridinium cation forms the unsaturated 1,5-diketone. In the final step, the nucleophilic addition of ammonia leads to the compounds 79 or 80.
The derivatives exhibit interesting fluorescence properties such as excited-state intramolecular proton transfer (ESIPT) in solution. Excitation maxima are measured at 374 nm and emission maxima at 434 nm under neutral conditions. In acidic conditions, excitation maxima and emission maxima for compounds 79 and 80 are found at 332–476 nm and 484–606 nm, respectively. An increase in acid concentration results in either an increase in fluorescence intensity or quenching of fluorescence in the case of newly formed fluorophores in solution, according to the specific derivative. In the crystalline state, double emission due to ESIPT was observed with fluorescence maxima of the enolimine tautomers ranging from 414 to 426 nm. The fluorescence maxima of the keto amine tautomers are within the region of 594–624 nm with high Stokes shifts.
Pyrimidine derivatives display biological and pharmacological characteristics, including antitumor (Atwal et al., 1989; El-Subbagh et al., 2000), antibacterial (Cieplik et al., 2011; Selvam et al., 2015) and anticancer (Joshi et al., 2016; Mangal and Jangid, 2016) properties. Pyrimidine nucleosides as well as alkaloids and antibiotics have been isolated from natural sources (Lagoja, 2005). Access to different pyrimidine derivatives is possible for example via Biginelli MCR of aldehydes, urea and methylene active compounds under dielectric heating (Dabiri et al., 2007; Tu et al., 2009). In addition, some chromophores with a pyrimidine core show interesting photophysical properties. C6 unsubstituted tetrahydropyrimidines 81 exhibit aggregation-induced emission enhancement (AIEE) and size-independent emission (SIE) characteristics (Zhu et al., 2013). They emit blue or green fluorescence in aggregates (λmax,em = 399–491 nm) with fluorescence quantum yields of up to 0.93. For derivatives with aromatic substituents in the R2 and R4 position, a strong emission in aggregates can be observed compared to substituents with alkyl groups. The derivative 81a shows SIE characteristics since emission maximum of suspension particles, powder, film and crystals are identical at and appear at 434 and 484 nm, respectively. The synthesis is performed under mild conditions and catalyzed by the organocatalyst urea. The five-component reaction allows the construction of a substance library in which all starting materials except aldehyde are varied giving yields between 21% and 63% in the sense of the chromophore approach (Scheme 17A). The reaction contains four elementary steps starting with an aminovinylation, followed by aza-ene-type reaction, nucleophilic addition, and cyclization.
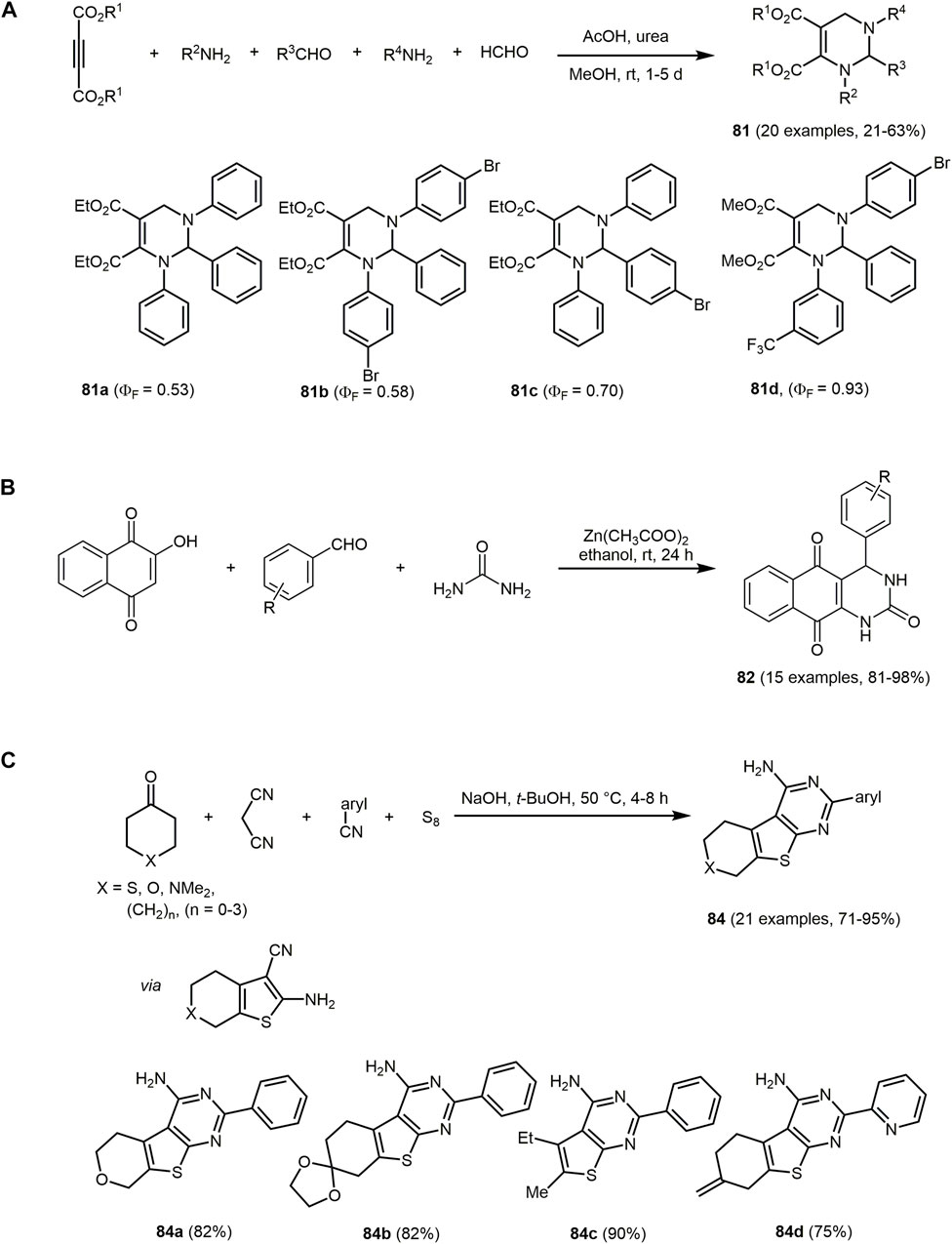
SCHEME 17. (A) Five-component reaction of tetrahydropyrimidines 81 and selective dyes with quantum yields above 0.50 (Zhu et al., 2013). (B) Synthesis of disperse dyes with a dihydropyrimidinone scaffold 82 via one-pot multicomponent reaction (Patel et al., 2022). (C) Modified Gewald synthesis of 2-arylthieno[2,3-d]pyrimidin-4-amines 84 and selected examples (Abaee et al., 2017).
Recently, via Biginelli reaction 3,4-dihydropyrimidin-2-(1H)-one derivatives 82 with excellent yields are formed. The reaction of Lawson, various aromatic aldehydes and urea is catalyzed using zinc acetate (Scheme 17B) (Patel et al., 2022).
The 3,4-dihydropyrimidin-2-(1H)-ones derivative 82 display well-defined color regions such as red, orange and yellow with good intensity. The UV-visible absorption spectra in DMF exhibit absorption maxima in the region from 399 to 493 nm.
Further dihyropyrimidines derivatives can also be prepared via the Biginelli reaction. By employing fluorescent β-ketoamides, hybrid fluorescent 3,4-dihydropyrimidine-2-(thi)ones 83 can be obtained (Supplementary Material S28) (de Souza et al., 2020).
The synthesized dihydropyrimidines derivatives 83 exhibit fluorescence in solution with large Stokes shifts, due to a proton transfer process. Moreover, fluorophores reveal double fluorescence emission. The emission at short wavelengths is attributed to the excited enol forms and at longer wavelengths to the tautomeric species, which can be associated with the intramolecular excited state proton transfer process (ESIPT). Further studies confirm a cytotoxic activity of the compounds and their potential application as fluorescence probes.
Pyrimidines can also be employed as metal ion sensors. For example, an acetonitrile solution of 2-arylthieno[2,3-d]pyrimidyl-4-amine 84d changes color from colorless to yellow at higher concentrations of Pd(II) ions, which is visible to the naked eye. The absorption spectrum reveals a decrease of the three bands of the dye (λmax,abs = 221, 244 and 332 nm) on expense of a new band with an isosbestic point at 362 nm in presence Pd(II) ions. The synthesis of 2-arylthieno[2,3-d]pyrimidin-4-amines 84 proceeds via a modified Gewald reaction (Gewald et al., 1966) with four components, where the additional component exploits the reactivity of the two neighboring functional groups of the classical three-component product. The starting materials are various α-methylene bearing ketones, malononitrile, aryl or heteroarylnitrile derivatives and elemental sulfur, which form functionalized products in good to excellent yields in the sense of the chromophore approach (Scheme 17C) (Abaee et al., 2017).
Another chemosensor but for the detection of Cu(II) ions are furo[2,3-d]pyrimidines-2,4[1H,3H]-diones 85. The chromophores consist of fused furopyrimidine and are generated via multicomponent strategy with a chromophore approach (Supplementary Material S29) (Kumar et al., 2017). The MW assisted three-component reaction of 1,3-dimethylbarbituric acid, benzaldehyde and respective isocyanides corresponding isocyanides proceeds via Knoevenagel condensation, [4 + 1] cycloaddition, and a 1,3-H shift to form two furopyrimidinones in excellent yields.
The furo[2,3-d]pyrimidines-2,4[1H,3H]-diones 85 display dual channel sensing of Cu(II) ions in solution and in the membrane phase. The electroanalytic study exhibits an ion selective electrode response toward Cu(II) ion in membrane phase. In the presence of Cu(II) ions, the absorption maximum decreases at the longest wavelength of the four absorption maxima (λmax,abs = 210, 271, 325 and 375 nm) and results in a decolorization of the initially yellow solution. The emission spectra are not affected by Cu(II) ions. The proposed complex based on the 1H NMR study shows that the furan oxygen atom and the alkylamino NH group are directly involved in the coordination of Cu(II) ions (Supplementary Material S30). The coordination can be cleaved by sequestering agents such as EDTA. The chemical sensor is recoverable and efficiently reused several times.
Another MCR to synthesize pyrimidine chromophores is the three-component reaction of 5-amino-1H-pyrazole-4-carbonitrile, p-substituted benzoylacetonitriles and triethylorthoesters (Supplementary Material S31) (Ghotekar et al., 2009). The cyclocondensation forms pyrazolo[1,5-a]pyrimidines 86 and 87 via two methods in good yields in the sense of the chromophore concept. If the reaction proceeds in toluene with triethylamine as a catalyst 7-(4-aryl) pyrazolo[1,5-a]pyrimidine-3,6-dicarbonitriles 86 are obtained, whereas catalysis with HCl in ethanol furnishes 7-amino-6-(4-aroyl)pyrazolo[1,5-a]pyrimidine-3-carbonitriles 87.
All pyrazolo[1,5-a]pyrimidines display intense fluorescence. The compounds 86 show absorption maxima between 267 and 296 nm and emission maxima between 304 and 332 nm. The amino group at the C7-position of compound 87 enhances the optical properties (λmax,abs = 336–360 nm, λmax,em = 393–414 nm) in comparison to the aryl group of compounds 86. If dimedone is replaced by benzoylacetonitriles in the synthesis, pyrazolo[1,5-a]quinazolines can be prepared through cyclocondensation in refluxing toluene.
Quinoxaline can be considered as a benzo[b]fused pyrazine, which leads to a strongly electron-deficient π-system, enabling its application in chromophores for DSSCs (Chang et al., 2011; Pei et al., 2012; Wu and Zhu, 2013). A variety of quinoxaline derivatives show inherent fluorescence with significant solvatochromic shifts in the emission bands (Woody et al., 2011; Schaffroth et al., 2013). Quinoxaline derivatives can be accessed via various MCRs, which can proceed in a domino to sequential or consecutive manner. The activation-alkynylation-cyclocondensation (AACC) and glyoxylation-alkynylation-cyclocondensation (GACC) sequences provide a simple and elegant way to synthesize various quinoxalines via MCR (Gers-Panther et al., 2017; Merkt et al., 2018; Biesen and Müller, 2021).
Moreover, the condensation of aromatic aldehyde and substituted 2-hydroxyacetophenone or 2-aminoacetophenone and malononitrile gives rise to 5-amino-2-aryl-3H-chromeno[4,3,2-d,e][1,6]naphthyridine-4-carbonitriles 88 and 5-amino-2-aryl-3H-quinolino[4,3,2-d,e][1,6]naphthyridine-4-carbonitriles 89 in the sense of a pseudo five-component MCR (Scheme 18A) (Wu et al., 2010). The process proceeds in aqueous medium and is catalyzed by silica gel, an easily available, inexpensive, and non-toxic substance. First, a chalcone is formed by aldol condensation of the aldehyde and the 2-hydroxyacetophenone, which then reacts with malononitrile. Subsequent cyclization and condensation with another malononitrile take place to give the desired products upon aromatization.
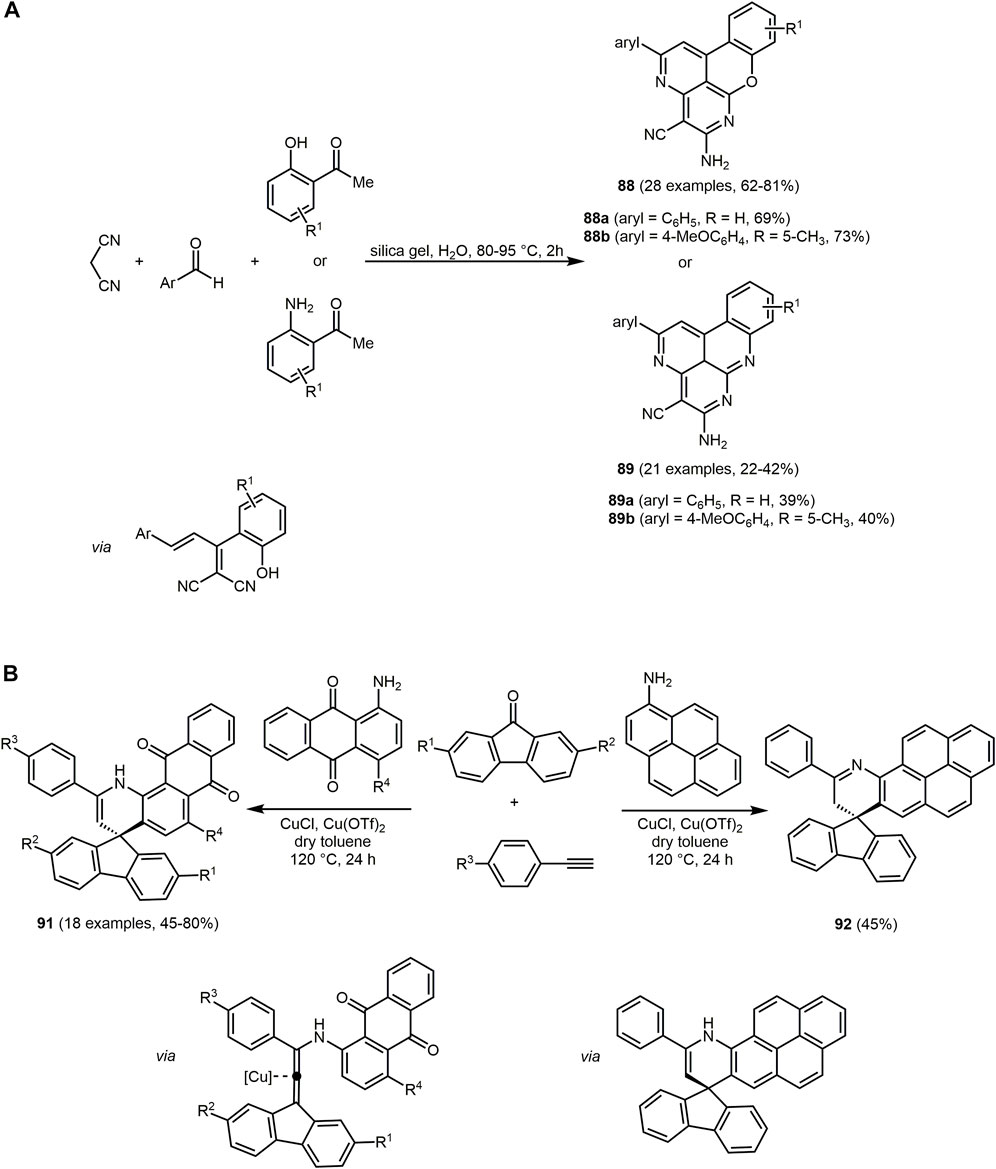
SCHEME 18. (A) Silica gel-catalyzed one-pot syntheses of 5-amino-2-aryl-3H-chromeno[4,3,2-de][1,6]naphthyridine-4-carbonitriles 88 and 5-amino-2-aryl-3H-quinolino[4,3,2-de][1,6]naphthyridine-4-carbonitriles 89 (Wu et al., 2010). (B) Synthesis of spirofluorenonaphthoquinolines 91 and 92 through MCR of 9-fluorenones, aryl alkynes, and aminoanthraquinones 1-aminopyrene (Meerakrishna et al., 2016).
The tetracycles 88 and 89 show strong fluorescence in EtOH upon irradiation with UV light (λexc = 360 nm), also with high fluorescence quantum yields. Moreover, emissions in the visible, allow applications as fluorescent probes, OLEDs, or luminescent materials. In fact, naphthyridine derivatives have already been used as luminescent materials for molecular recognition due to their planar rigid structure (Peng et al., 2005; Lu et al., 2006).
Nitroquinolines 90 can be produced by reacting p-nitroaniline, benzaldehydes and phenyl acetylene in presence of the Lewis acid niobium pentachloride under mild conditions (Supplementary Material S32) (dos Santos et al., 2017).
The optical properties of the compounds 90 can be altered by substituents on the benzaldehyde. The two absorption bands appear in a range of 250–280 nm and 325–393 nm, ascribed to the π-π*-transition and n-π*-transition. Electron-donating substituents cause a bathochromic shift in the absorption spectra. The nitroquinolines can be reduced with hydrazine monohydrate in the presence of 10% Pd/C to give aminoquinolines, which exhibit high quantum yields up to 0.83.
If ketones are used instead of aldehydes in the three-component reaction in the sense of the scaffold approach, access to substance libraries of highly conjugated, fluorescent spirofluorenonaphthoquinolines 91 are formed (Scheme 18B) (Meerakrishna et al., 2016). Using aryl alkynes with electron-donating groups in the copper-catalyzed reaction of ketones, alkynes, and amines (KA2 coupling) lead to higher yields due to the increased nucleophilicity of the copper acetylide. Mechanistically, the reaction proceeds by Cu(I)-catalyzed nucleophilic addition of phenylacetylide to 9-fluorenone to give a propargyl alcohol, which reacts with aminoanthraquinone forming an amino allene that undergoes intramolecular arylation and subsequent aromatization. Structurally different spirofluorenophenalenoquinoline derivatives 92 are obtained by using 1-aminopyrene as the amino component in an analogous reaction.
The absorption spectra of chromophores 91 are generally characterized by an absorption maximum between 537 and 614 nm, whereas dye 92 shows two absorption maxima 391 and 412 nm. The compounds 91 exhibit orange-red fluorescence, while pyrenospirofluoreno-naphthoquinoline 92 shows emission in the deep blue region with the highest Stokes shifts (Δ
The isatin-based spiro compounds 93 are formed via Knoevenagel condensation between isatins and malononitrile, followed by a Michael-type addition of meta-phenylenediamine, subsequent intramolecular cyclization and tautomerization (Supplementary Material S33) (Kundu et al., 2013).
All isatin-based spiro compounds 93 show similar absorption and emission spectra. A red shift of the emission maxima can be observed by increasing the solvent polarity and fluorescence quantum yields and lifetimes are also affected by the polarity of the solvent. The solvatochromism is characteristic for quinolines (Mataga and Tsuno, 1957; Atkinson and Speakman, 1971). Furthermore, the isatin-based spiro compounds 93 can be used as an ON-OFF switch chemosensor for Cu(II) ions.
A three-component reaction of 4-hydroxycoumarin, aldehydes and primary amines produces a huge substance library of quinoline chromophores 94 (Scheme 19A) (Ataee-Kachouei et al., 2019). The synthesis of chromeno[4,3-b]quinolin-6-ones and their symmetrical and unsymmetrical dyes 95–98 is catalyzed by naturally occurring halloysite nanotube (HNT) with the general formula of (Al2(OH)4Si2O5⋅2 H2O) (Rawtani and Agrawal, 2012). The solvent-free conditions, excellent yields, short reaction times and the low-cost, environmentally friendly and reusable catalyst characterize this reaction procedure as a green approach. The fluorophores 94–98 fluoresce blue and green and emission maxima can be detected in a range from 400 to 535 nm.
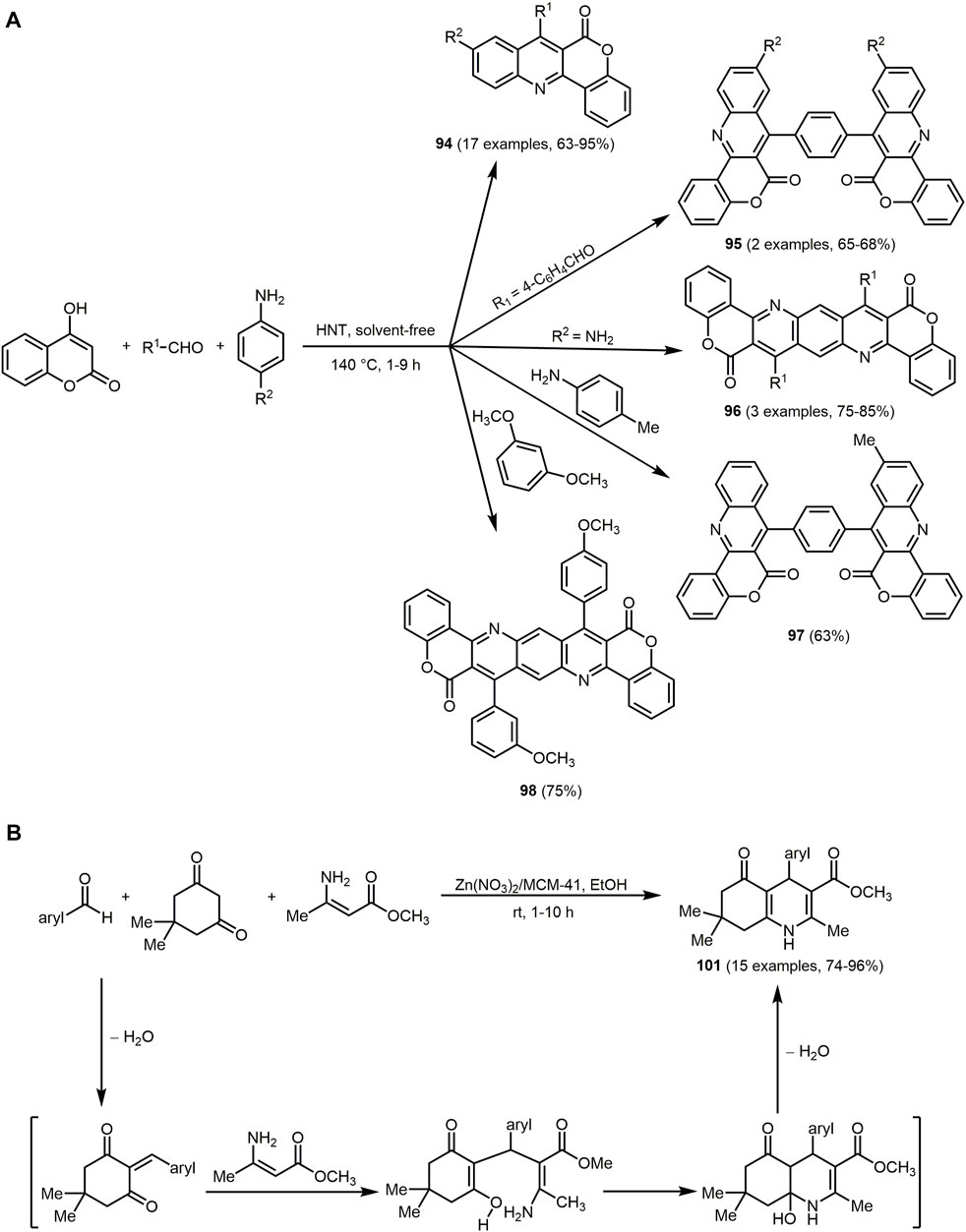
SCHEME 19. (A) Green one-pot three-component synthesis of chromeno[4,3-b]quinolin-6-ones 94–98 (Ataee-Kachouei et al., 2019). (B) Three-component synthesis of hexahydroquinolin-5-ones 101 (Oskuie et al., 2020).
Coumarin-fused dihydroquinolines 99 are available in excellent yields (82%–94%) in the sense of the chromophore approach from 4-hydroxycoumarin, aldehydes and aromatic amines with bismuth triflate as a catalyst in water under microwave irradiation (Supplementary Material S34) (Khan et al., 2014). Mechanistically, Schiff base formation followed by condensation with 4-hydroxycoumarin generates the intermediate that reacts by carbonyl condensation with the aromatic amine followed by 6
The catalyst-free three-component reaction of various α,β-unsaturated aldehydes, 2-hydroxy-1,4-naphthoquinone and several 5-aminopyrazoles afford pentacyclic pyran-fused pyrazolobenzo[h]quinoline derivatives 100, which incorporate four bioactive components such as pyran, pyridine, pyrazole and α-naphthol (Supplementary Material S35) (Yadav et al., 2022). It is suggested that the α,β-unsaturated aldehyde reacts with 2-hydroxy-1,4-naphthoquinone via a Knoevenagel reaction. This is followed by the C-nucleophilic attack of 5-aminopyrazole via the C4 position. The two subsequent cyclizations yield the desired product 100.
Nearly all of the synthesized derivatives fluoresce strongly under UV light. The quantum yields are in the range of 0.24–0.42 with Stokes shift of about 200 nm. The emission maxima are found at 490–514 nm.
The Zn/MCM-41-catalyzed (ZnNO3-impregnated MCM-41) unsymmetrical Hantzsch three-component condensation of various aryl aldehydes, dimedone and methyl-3-aminocrotonate proceeds under mild conditions and gives rise to the formation of hexahydroquinolin-5-ones 101 (Scheme 19B) (Oskuie et al., 2020).
The absorption maxima of the hexahydroquinolin-5-ones 101 can be detected at around 365 nm and emission at around 450 nm. The compounds 101 exhibit high Stokes shifts ranging from 4,800 to 5,800 cm−1 and moderate quantum yields up to 0.28. In addition, some of the synthesized hexahydroquinolines show anticancer activities.
The benzoanellated quinolinones 102 can be synthesized via one-pot reaction of 6-methoxy-1,2,3,4-tetrahydro-naphthalin-1-one, trimethoxybenzaldehyde, ethyl cyanoacetate and ammonium acetate under microwave irradiation (Supplementary Material S36) (Khan and Asiri, 2015; Zayed and Kumar, 2017). The 2-oxo-quinoline-3-carbonitrile derivative 102b also shows antibacterial properties.
The intramolecular charge transfer band in the absorption spectrum and emission solvatochromicity of the donor-acceptor quinoline dyes 102 account for polar excited states. The quinoline-based chromophores 102a and 102b achieve quantum yields of up to 0.40 and 0.59, respectively.
The sequential three-component synthesis of homophthalonitrile, o-hydroxybenzaldehyde, and a nucleophile gives rise to chromenoisoquinolines (Festa et al., 2017). If (aza)indole acts as the nucleophile, 12-(1H-indol-3-yl)12H-chromeno[2,3-c]isoquinolin-5-amines 103 are obtained in the sense of the chromophore approach (Supplementary Material S37) (Festa et al., 2019).
The UV/Vis spectra of indol-3-yl substituted dyes 103 show similar absorption and emission maxima at around 354 and 415 nm, respectively. The highest fluorescence quantum yields (ΦF = 0.42–0.70) are obtained in polar and protic solvents. In addition, the compounds 103 undergo reversible fluorescence quenching under acidic conditions. These optical properties result from the localized electron density of the frontier orbitals on the isoquinolinamine moiety and the equal energy gaps of the associated frontier molecular orbitals.
Phenothiazines are electron-rich heterocyclic organic π-systems and, thus, they are interesting donors in dyes. As a consequence of their substitution pattern they tunable with respect to reversible oxidation potentials and luminescence (Sun et al., 2005; Sasaki et al., 2007; Miura et al., 2010). In general, donor-acceptor systems are utilized in molecular electronics and photonics (Armstrong et al., 2001; Lai et al., 2001; Richter, 2004; Dini, 2005; Kulkarni et al., 2005), in OLEDs (Kraft et al., 1998; Mitschke and Bäuerle, 2000; Kulkarni et al., 2004; Walker et al., 2011), as well as in photovoltaic devices (Hoppe and Sariciftci, 2004; Walker et al., 2011; Lin et al., 2012b). Remarkably, N-substituted phenothiazines are used for the construction of AIE compounds (Zhang et al., 2015b; Okazaki et al., 2017; Chen et al., 2019; Gong et al., 2019; Ekbote et al., 2020) resulting from their non-planar “butterfly” shaped conformation, which is folded along the S,N axis (Bell et al., 1968; Klein et al., 1985). Access to phenothaizines in the sense of an MCR can be achieved, for example, via an Ugi reaction (Bay et al., 2013; Bay et al., 2014; Bay and Müller, 2014).
Just recently, 3,10-diaryl phenothiazines 104 were generated via a sequentially Pd-catalyzed three-component arylation-amination sequence in a one-pot fashion (Scheme 20A) (Mayer et al., 2020; Mayer and Müller, 2021). The consecutive Suzuki arylation-Buchwald-Hartwig amination sequence was applied to obtain 25 different examples of 3,10-diarylphenothiazines 104 in moderate to very good yields by varying arylboronic acids or esters as well as the aryl bromides with electron-donating and electron-withdrawing substituents in the para-position.
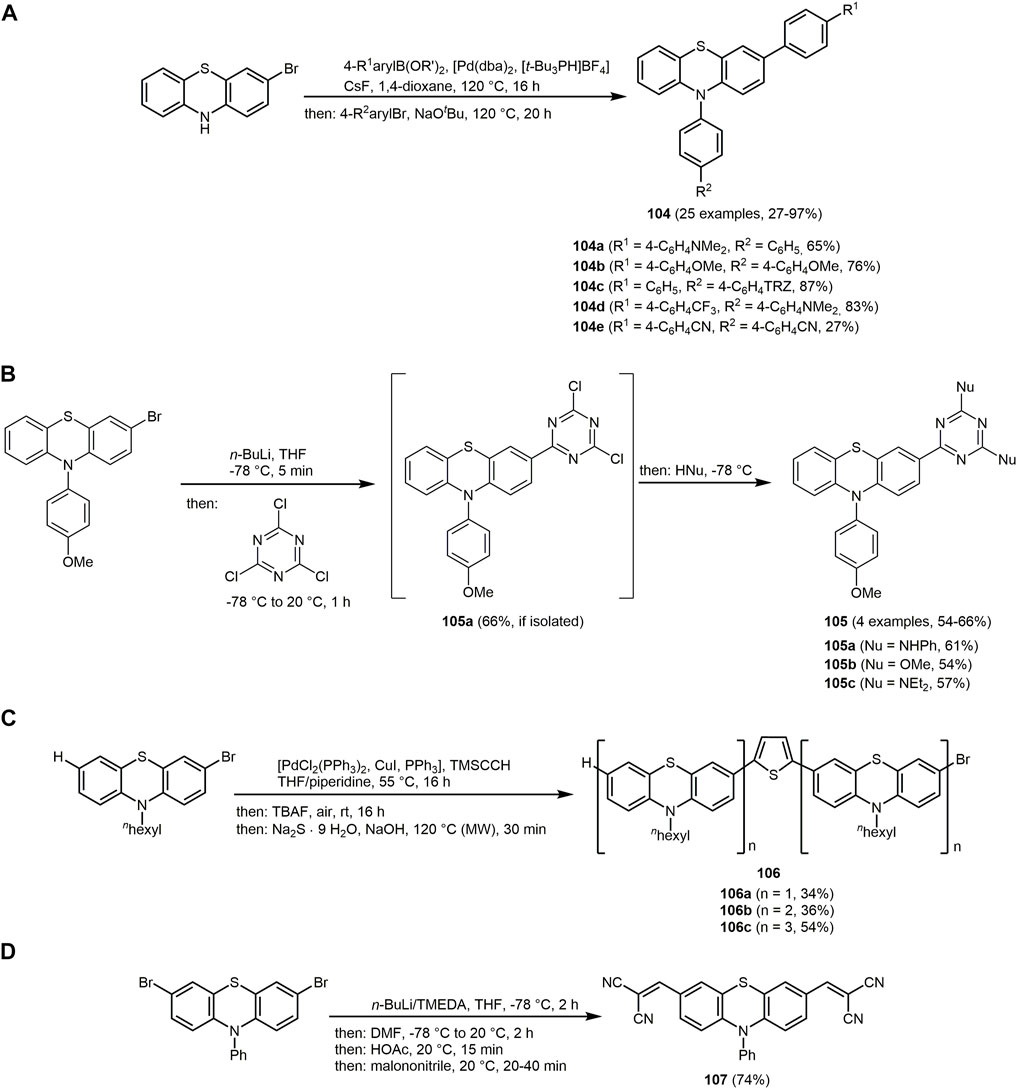
SCHEME 20. (A) Sequentially Pd-catalyzed arylation-amination consecutive three-component synthesis of 3,10-diaryl 10H-phenothiazines 104 (TRZ = 2,4-diphenyl-1,3,5-triazine) (Mayer et al., 2020; Mayer and Müller, 2021). (B) One-pot sequence to synthesize disubstituted phenothiazine-triazine dyes 105 (Kloeters et al., 2022). (C) Pseudo five-component Sonogashira–Glaser cyclization synthesis of thienyl-bridged oligophenothiazines 106 (Urselmann et al., 2016). (D) One-pot LiForK synthesis of a 3,7-diacceptor substituted phenothiazine 107 (May and Müller, 2020).
The electron properties of the 3,10-diaryl 10H-phenothiazines derivatives 104 can be fine-tuned by varying electronic substituents on the phenothiazine that extend the π-electron conjugation and the fundamental conformational change from intra- to extra-configuration. Using an elaborated structure-property relationship 3D diagram based on the correlation between the first oxidation potential (
Placing the heterocycle 1,3,5-triazine instead of the aryl radical in 3-position, also a considerable color spectrum can be covered by modulating the acceptor strength of the triazine moiety in the solid state under excitation under UV light (λexc = 365 nm). The triazine building blocks have immense importance due to their extraordinary biological activities, especially in chemical medicine (Verma et al., 2020). Synthetically, after brominelithium exchange of the starting component 3-bromo-N-anisyl-phenothiazine with BuLi and introduction of the trichloro-1,3,5-triazine core by subsequent double nucleophilic substitution, the phenothiazine-triazine chromophores 105 can be obtained (Scheme 20B) (Kloeters et al., 2022).
In general, compounds 105 show high fluorescence quantum yields in solution and in the solid state. By tuning the substitution pattern on the triazine, photophysical properties such as thermally activated delayed fluorescence (TADF) and white light emission can occure. Thus, solvatochromism studies of electron-deficient substituted triazine chromophores reveal strong charge transfer character and a small singlet-triplet energy gap, hence these derivatives in particular 105a identify as TADF candidates. While the electron rich triazine dyes show a reversible shift of the spectral emission upon protonation. Furthermore, a white light emission can be observed for derivatives 105d (Supplementary Material S38).
The phenothiazine moiety has also been implemented in linear (Sailer et al., 2008) or cyclic (Memminger et al., 2008) oligomer topologies as well as diphenothiazinyl dumbbells linked by heterocycles (Franz et al., 2009; Hauck et al., 2010; Jahnke et al., 2014). The synthesis of symmetrical thienyl-bridged oligophenothiazine dumbbells 106 is feasible via a consecutive pseudo five-component Sonogashira-Glaser cyclization sequence (Scheme 20C) (Urselmann et al., 2016).
The absorption spectra of compounds 106 exhibit four absorption bands. Three appear at shorter wavelengths, which can be assigned to the phenothiazinyl units, and the longest wavelength maximum can be attributed to the central 2,5-di (hetero)aryl-substituted thiophene moiety. The molar decadic extinction coefficient increases with the number of phenothiazinyl units. The thienyl-bridged oligophenothiazines 106 emit in a wavelength range from 506 to 521 nm with large Stokes shifts between 4,800 and 5,600 cm−1, which are characteristic for oligophenothiazines (Sailer et al., 2008). Fluorescence quantum yields of chromophores 106 range from 0.15 to 0.18. A cathodic shift of oxidation potentials is observed for the series with increasing number of phenothiazinyl electrophore units. A consistently reversible oxidation range can be demonstrated for compound 106c. Molecular modelling reveals lowest energy conformers that exhibit a sigmoidal and helical structure. TD-DFT calculations and even semiempirical ZINDO (Zerner’s intermediate neglect of differential overlap) calculations confirm the trends of the absorption bands with the longest wavelengths. Thus, the charge transfer can be largely assigned between the electrophore moieties from the neighboring phenothiazinyl moieties to the central thienyl unit.
Diacceptor substituted phenothiazine 107 can be accessed via a lithium formylation-Knoevenagel condensation (LiForK) sequence (May and Müller, 2020). The consecutive pseudo five-component reaction initiated by bromine-lithium exchange forms an acceptor-donor-acceptor conjugate (Scheme 20D) (May and Müller, 2020).
Via the same reaction sequence, the heterocyclic topologically analoguous 2,6-diacceptor-substituted dithieno[1,4]thiazines 108 and 109 can also be prepared (Supplementary Material S39). Further syn- and anti-anti dithieno[1,4]thiazine isomers with diacceptor (110a and 111a) and bisdonor (110b and 111b) substitution pattern can be synthesized via the pseudo three-component reaction of N-phenyl dithieno[1,4]thiazine and 4-bromobenzonitrile or 4-iodoanisole via dilithiation-lithium-zinc exchange-Negishi coupling in yields ranging from 20% to 83%.
The interactions between the substituents in the dithieno[1,4]thiazines (108 and 109) is stronger than in the corresponding phenothiazine 107 since dithieno[1,4]thiazines are generally higher polarizable. Dithieno[1,4]thiazines 108 and 109 are characterized by cathodically shifted oxidation potentials and red-shifted, more intense absorption bands compared to the corresponding phenothiazines 107. The analysis of the structure-property relationships points out that the photophysical and electrochemical properties as well as the electronic structure are significantly determined by the thiophene anellation mode of the products 108 and 109. Thus, strong acceptors in syn-syn-dithieno[1,4]thiazines 108 and 110a possess a rather folded structure as well as weak fluorescence (ΦF = 0.01). In contrast, equally substituted anti-anti isomers 109 and 111a show an almost planar ground state geometry and very intense near-infrared fluorescence (ΦF = 0.52). In principle these red light or NIR emitters can be promising for potential application in biomedical imaging (Hong et al., 2017) or OLED-devices (Qu et al., 2006).
5 (Hetero)Arene
Exciplexes (excited complexes) as well as excimers (excited dimers) are emitting charge transfer complexes, which are formed by excitation of one of the constituent chromophores, which collides with a second chromophore that is in the electronic ground state (Balzani, 2001; Balzani and Venturi, 2003). The Ugi four-component reaction provides an access to unimolecular exciplex emitting dyads 112 consisting of an N,N-dimethylaniline moiety as a donor and anthracene, naphthalene or pyrene as acceptor chromophores in the sense of the scaffold approach (Scheme 21A) (Ochs et al., 2019).
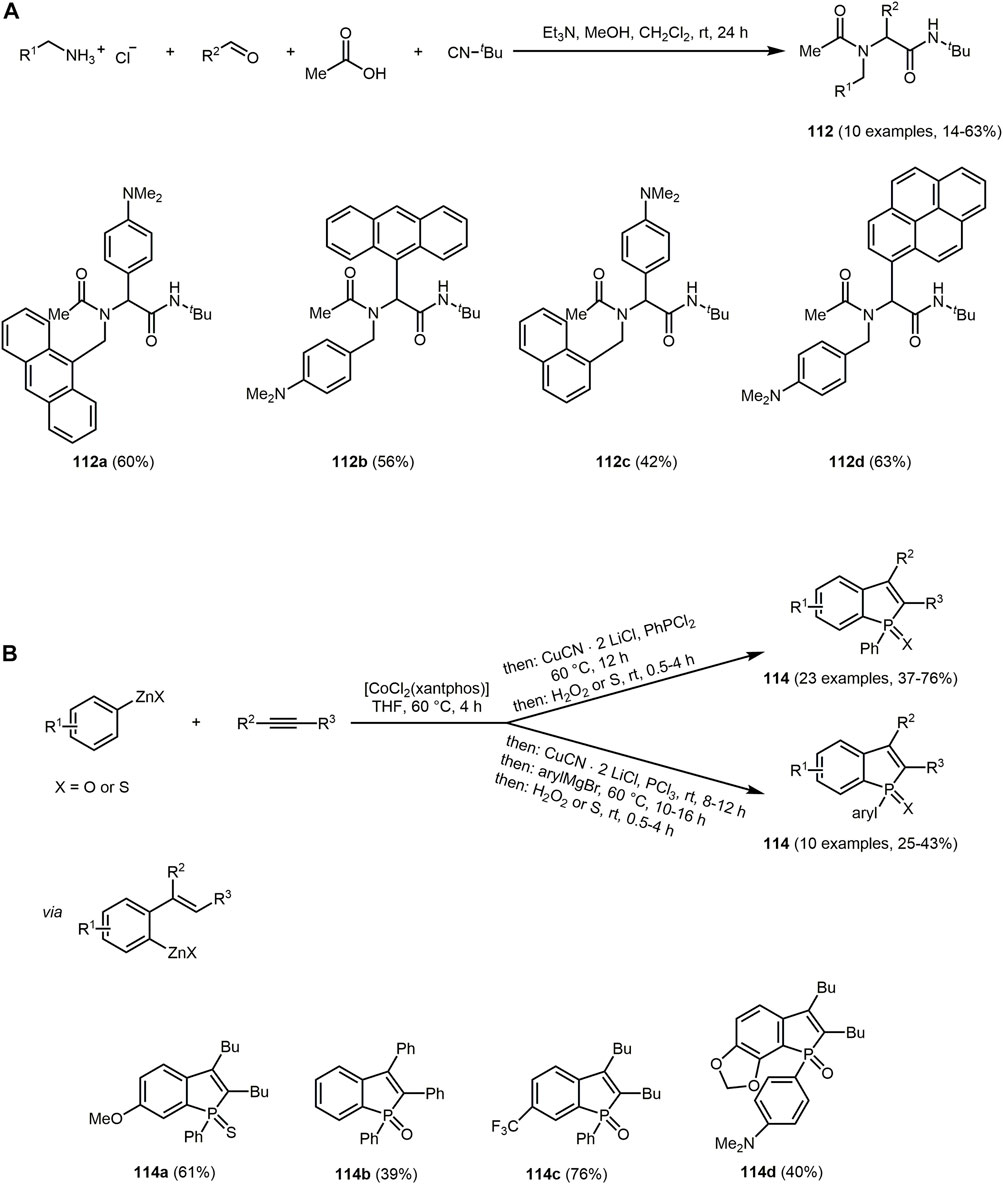
SCHEME 21. (A) One-pot Ugi 4CR synthesis of donor-acceptor dyads 112 and selected examples (Ochs et al., 2019). (B) One-pot synthesis of functionalized benzo[b]phosphole derivatives 114 and selected fluorophores (Wu et al., 2014).
The chromophores of the donor-acceptor dyads 112 are electronically decoupled in the ground state and electronically coupled in the excited state. This results in exciplex emissions due to the polar nature of the excited state. The formation of the exciplex depends on partial electron transfer with matching redox potentials and on spatial proximity of the donor and acceptor units. Furthermore, the N,N-dimethylaniline-acceptor chromophores 112 are capable of photoinduced intramolecular electron transfer (PIET) and exhibit emission solvatochromism with red-shifted emission upon increasing solvent polarity (Supplementary Material S40). Based on TD-DFT calculations, the qualitative assignment of the experimental absorption and emission bands is possible.
1,4-Diarylbuta-1,3-diene derivatives are applied in liquid crystals, illuminants, and non-linear optical materials (Bartkowiak et al., 2001; Davis et al., 2003; Davis et al., 2004; Denmark and Tymonko, 2005; Abraham et al., 2006; Davis et al., 2008; Das et al., 2010). In addition, some MCRs are known for the synthesis of these chromophores (Zhang and Larock, 2003; Shibata et al., 2005; Horiguchi et al., 2008). A palladium-catalyzed three-component reaction of aryl iodides, diarylacetylenes, and cinnamic acids furnishes 1,4-diarylbuta-1,3-dienes 113 in the sense of the chromophore concept (Supplementary Material S41) (Yamashita et al., 2011). The sequence is initiated by oxidative addition of aryliodide with in situ generated Pd (0) species followed by alkyne insertion and ligand exchange with cinnamic acid giving a vinylpalladium carboxylate intermediate. Subsequent decarboxylation and reductive elimination give the products. 1,4-Diarylbuta-1,3-dienes 113 show solid state emission with maxima in a range from 440 to 540 nm.
Structurally diverse benzo[b]phospholes 114 are synthesized via facile regiocontrolled one-pot sequential coupling of an arylzinc reagent, an alkyne, dichlorophenylphosphane (or phosphorus trichloride and a Grignard reagent), and an oxidant (hydrogen peroxide or sulfur) (Scheme 21B) (Wu et al., 2014). For this MCR two common approaches with similar initiation steps can be applied. Cobalt-catalyzed migratory arylzincation forms the intermediate that reacts after transmetalation to an organic copper species with PhPCl2 followed by oxidation with hydrogen peroxide or sulfur powder. Alternatively, the formation of the benzo[b]phosphole oxides or benzo[b]phosphole sulfides 114 proceeds by reaction of the copper species with PCl3, followed by addition of Grignard reagents and final oxidation.
Most benzo[b]phosphole derivatives 114, especially benzo[b]phosphole oxides, are fluorescent in solution. Electron-donating amino groups as substituents in the R1 position led to a significant redshift. In general, the longest wavelength absorption maxima appear between 317 and 394 nm. The emission maxima are located between 385 and 484 nm with fluorescence quantum yields of up to 0.93. In general, benzo[b]phospholes possess interesting optoelectronic properties and find application in organic electronic devices (Tsuji et al., 2009; Tsuji et al., 2010).
6 Azo chromophores
Azo dyes have a long history among synthetic dyes and are used in a wide range of applications, including in cosmetic, textile and paper industries (Şener et al., 2006; Benkhaya et al., 2017; Benkhaya et al., 2020). Azo dyes are characterized by one or more azo bridges as integral chromophore. The common preparation method of azo dyes is the diazotization of an aromatic primary amine followed by coupling with one or more electron-rich π-nucleophiles (Gürses et al., 2016). Due to the great importance and application of this dye class, several green syntheses of this class of compounds have been explored (Safari and Zarnegar, 2015; Nikpassand and Pirdelzendeh, 2016; Nikpassand et al., 2018; Nikpassand, 2020). For instance, the microwave assisted three-component reaction of arylazopyrazoles, benzaldehydes and dimedone proceed via Mannich cyclization and condensation to form pyrazoloquinazolinone azo dyes 115 (Supplementary Material S42) (Elgemeie et al., 2015). The absorption maxima of azo dyes 115 appear between 380 and 498 nm. In the presence of a nitro aryl substituent on the azo part, a bathochromic shift of the absorption maxima can be observed.
Azo pyrimido[4,5-b]quinoline derivatives 116 are prepared via an unsymmetrical Hantzsch synthesis with dimedone or 1,3-cyclohexadione, azo aldehydes, and 6-amino-1,3-dimethyluracil in the presence of choline chloride/oxalic acid (ChCl/Oxa) as a green solvent and recyclable substance catalyst (Scheme 22A) (Gholami et al., 2020). The UV/Vis spectra exhibit two absorption bands. The longest wavelength absorptions are found between 352 and 362 nm.
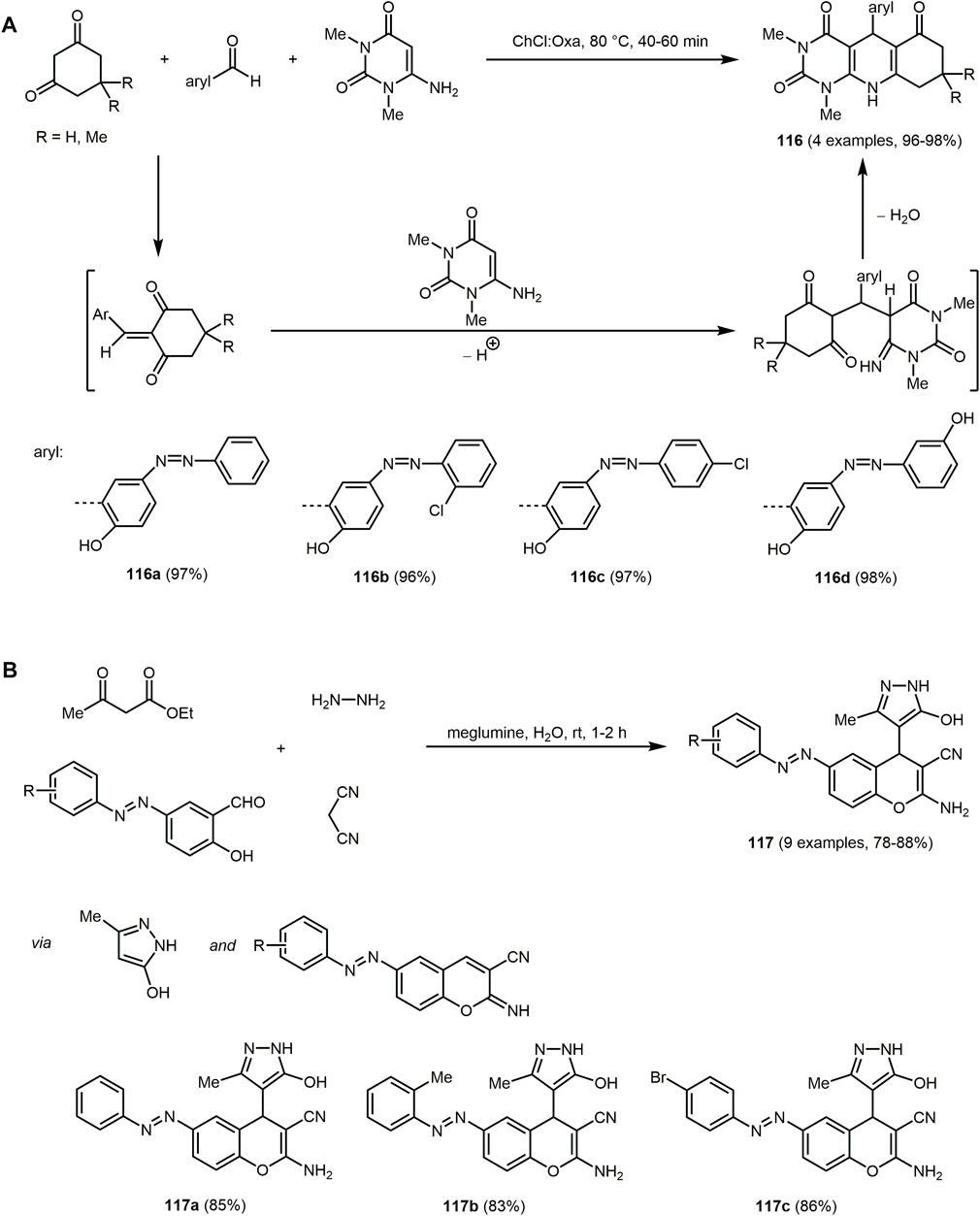
SCHEME 22. (A) Hantzsch synthesis of pyrimido[4,5-b]quinolines 116 and the four obtained derivates (Gholami et al., 2020). (B) Meglumine catalyzed one-pot synthesis of fluorescent 2-amino-4-pyrazolyl-6-aryldiazenyl-4H-chromene-3-carbonitriles 117 and selected derivates (Korade et al., 2021).
An energy efficient four-component synthesis starting from ethyl acetoacetate, hydrazine hydrate, azo salicylaldehydes, and malononitrile forms in the presence of meglumine (N-methyl-D-glucamine) as an organocatalyst fluorescent 2-amino-4-pyrazolyl-6-aryldiazenyl-4H-chromene-3-carbonitriles 117 in good to excellent yields (Scheme 22B) (Korade et al., 2021)
Absorption maxima of the dyes are found in the range of 290–294 nm and can be ascribed to the n–π* transitions. In contrast to many azo compounds the dyes 117 fluoresce with large Stokes shifts (Δ
7 Miscellaneous
7.1 Metalcomplex dyes
Organoboron complexes have been known for quite some time (Michaelis, 1894; Michaelis and Richter, 1901), but only in the 21st century their fluorescent properties were recognized as favorable for application in OLEDs (Entwistle and Marder, 2002; Entwistle and Marder, 2004; Jäkle, 2010; Rao and Wang, 2011). For example, boron Schiff bases have indeed been employed in OLEDs (Vidyasagar et al., 2019) and as non-linear optical chromophores (Reyes et al., 2002; Lamère et al., 2006; Muñoz et al., 2008; Jiménez-Pérez et al., 2015a; Jiménez-Pérez et al., 2015b). In addition, they are used in bioimaging (António et al., 2019; Ibarra-Rodríguez et al., 2019; Russo et al., 2020).
Recently, a multicomponent synthesis of two boron Schiff bases 118a and 118b through the condensation reaction of 2-hydroxynaphthaldehyde with the corresponding amines and in situ generated diphenylborinic acid has been reported (Scheme 23A) (Corona-Lopez et al., 2021).
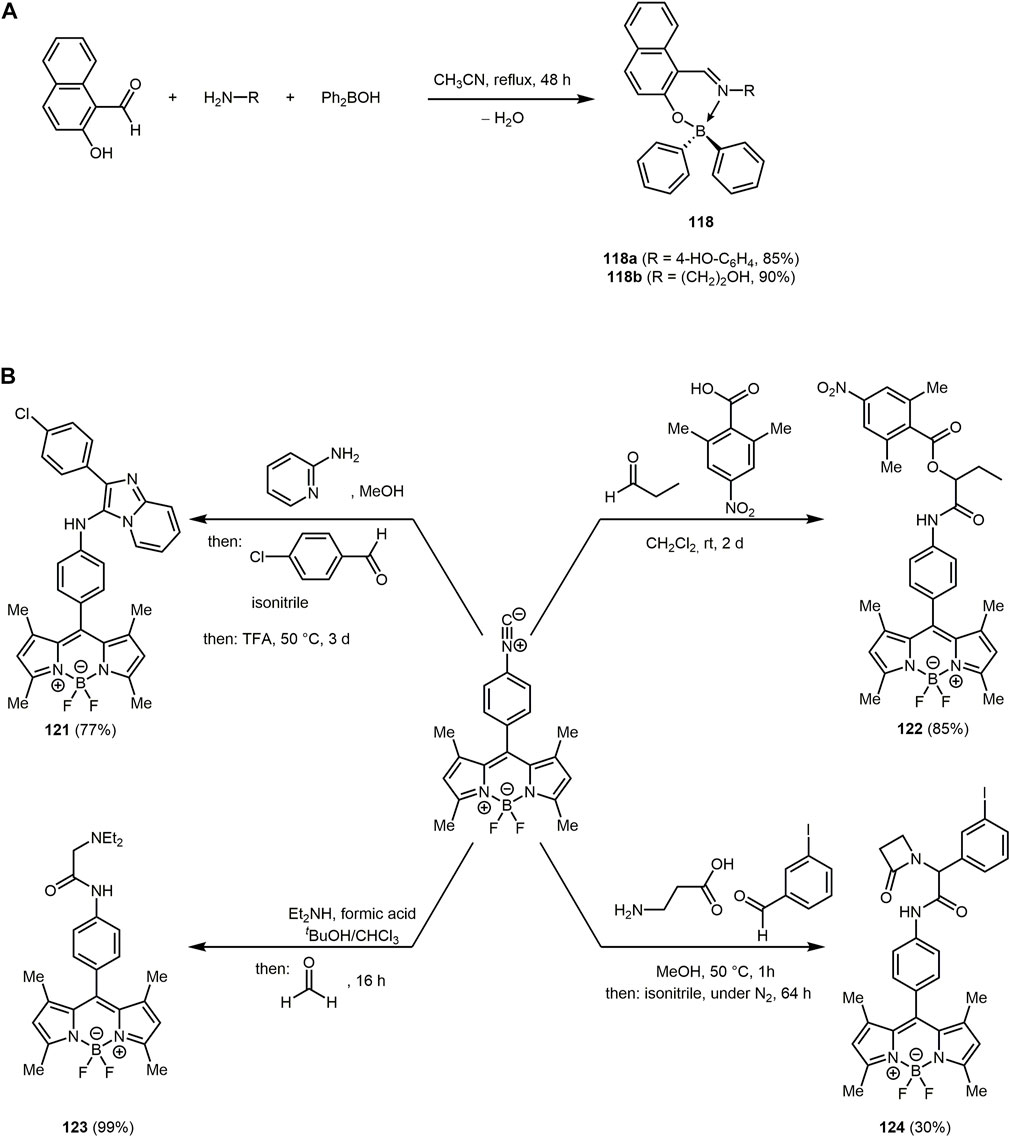
SCHEME 23. (A) One-pot synthesis of boron Schiff base dyes 118 (Corona-Lopez et al., 2021). (B) Derivatization of an isonitrile functionalized BODIPY dye via various MCR to synthesize compounds 121–124 (Vazquez-Romero et al., 2013).
The longest wavelength absorption maxima can be detected between 396 and 404 nm. The emission maxima lie between 474 and 525 nm with large Stokes shifts, but low quantum yields. In addition, boron complexes 118a and 118b can be classified as semiconductors based on the determined optical band gaps Eg between 2.57 and 2.78 eV for the n-
Further tetracoordinated boron atoms bearing Schiff bases 119 can be synthesized via a three-component condensation reaction starting from damnacanthal as a biogenic component in a remarkably rapid manner (Supplementary Material S43) (Garcia-Lopez et al., 2022).
The organoboron esters 119 are fluorescent and exhibit emission maxima at around 400 nm. Notably, the oscillator strength of the electronic transition can be influenced by the position of the substituent. Thus, the derivative with the nitro group substituent in 4-position displays a larger extinction coefficient. Similar to the organoboron complexes 118, the chromophores 119 also show low quantum yields around 0.01 and can also be classified as semiconductors due to the optical band gaps (Eg ≈ 252 eV). As a result of the damnacanthal utilized as the starting material, 119 possess biological activity and in particular 119b showed cytotoxicity activity against MDA-MB-231 breast cancer cells.
A fast and efficient approach to chromophores applicable also in biological fields such as boron hydrazone dyes 120, can be achieved by microwave-assisted domino multicomponent condensation reaction of diverse aryl aldehydes, benzoylhydrazide, or 4-nitrobenzoylhidrazine, and diphenyl boronic acid (Supplementary Material S44) (Molina-Paredes et al., 2019).
The UV/Vis spectra of boron complexes 120 exhibit either one or two absorption maxima and longest wavelength absorption bands can be detected in a range from 368 to 448 nm with molar extinction coefficients ε between 13,000 and 55,000 M−1 cm−1. The emission maxima appear between 420 and 520 nm with moderate Stokes shifts (Δ
Further organoboron complexes have low cytotoxicity are employed in the medical diagnostics, especially as pH indicators and cell markers (Baruah et al., 2006; Yang et al., 2013; Liu et al., 2015; Yang et al., 2016). In particular, 4,4-difluoro-4-bora-3a,4a-diaza-s-indacene (BODIPY) scaffold, which exhibits excellent photophysical properties, are commonly encountered in fluorescent probes (Loudet and Burgess, 2007; Ulrich et al., 2008; Boens et al., 2012; Kolemen and Akkaya, 2018). Emission and absorption typically below 600 nm as well as small Stokes shifts and high quantum yields are characteristic of fluorophores containing a BODIPY core (Gürses et al., 2016). Using a series of multicomponent reactions starting from isonitrile functionalized BODIPY dye allows for derivatization of this framework (Scheme 23B) (Vazquez-Romero et al., 2013). The isonitrile-BODIPY scaffold was previously synthesized starting from BODIPY aniline and subsequently functionalized by Groebcke-Bienaymé-Blackburn (121), Passerini (122), and Ugi reaction (123 and 124).
The emission and absorption of the fluorescent BODIPY dyes 123 lie in the typical range of BODIPY fluorophores and exhibit quantum yields of up to 0.61. The dyes 121 can be utilized as a fluorescent probe for in vivo imaging of phagocytosing macrophages.
Moreover, by varying the functional group of the BODIPY dye further substance libraries of BODIPY complexes can be obtained by MCR. The Passerini reaction of formyl-containing BODIPY derivatives with benzoic acid and t-butyl isocyanide yields highly substituted BODIPY dyes 125 and 126 (Supplementary Material S45) (Ramirez-Ornelas et al., 2016). The formyl-containing BODIPY complex are prepared via Liebeskind−Srogl cross-coupling or Vilsmeier reaction starting from Biellmann BODIPYs (Goud et al., 2006).
The photophysical properties of the BODIPY dyes are not affected by the ligations to the para position of the 8-phenyl group or to 2-position directly on the BODIPY core, but rather are influenced by the free motion of the 8-aryl. For example, boronic complexes containing an unhindered 8-phenyl (125a and 126a) show a low fluorescence response, due to free rotational motion of the ring. In contrast, high quantum yields (ΦF = 0.82) are detected for aryls with methylation at the ortho positions (126b), as mesityl groups prevent the aryl from rotating freely due to a higher rotational barrier. In addition, these complexes can be used to stain blood cells with very intense and stable signals at a very low exposure time.
Luminescence in metal complexes typically results from metal-to-ligand charge transfer in the excited state (Fredericks et al., 1979; Striplin and Crosby, 1994). Therefore, their photophysical properties are characterized by high Stokes shifts and long luminescence lifetimes, which are especially essential in the bioanalytical field (Ma et al., 2014; Albada and Metzler-Nolte, 2016). For instance, organotin compounds derived from Schiff base complexes can be applied as analytical luminescent chemosensors for the identification of metals (Vinayak and Nayek, 2019). In addition, since these complexes are capable of staining silk fibroin, they can potentially be used as scaffolds for tissue engineering (Lara-Ceron et al., 2017). Organotin complexes with n-butyl (127) and phenyl residues (128) can be prepared by microwave-assisted three-component condensation reaction of 2-hydroxy-1-naphthaldehyde, L-amino acids, and diorganotin oxides (Scheme 24A). Intrinsically fluorescent amino acids, such as tryptophan, tyrosine, and phenylalanine can be thereby implemented.
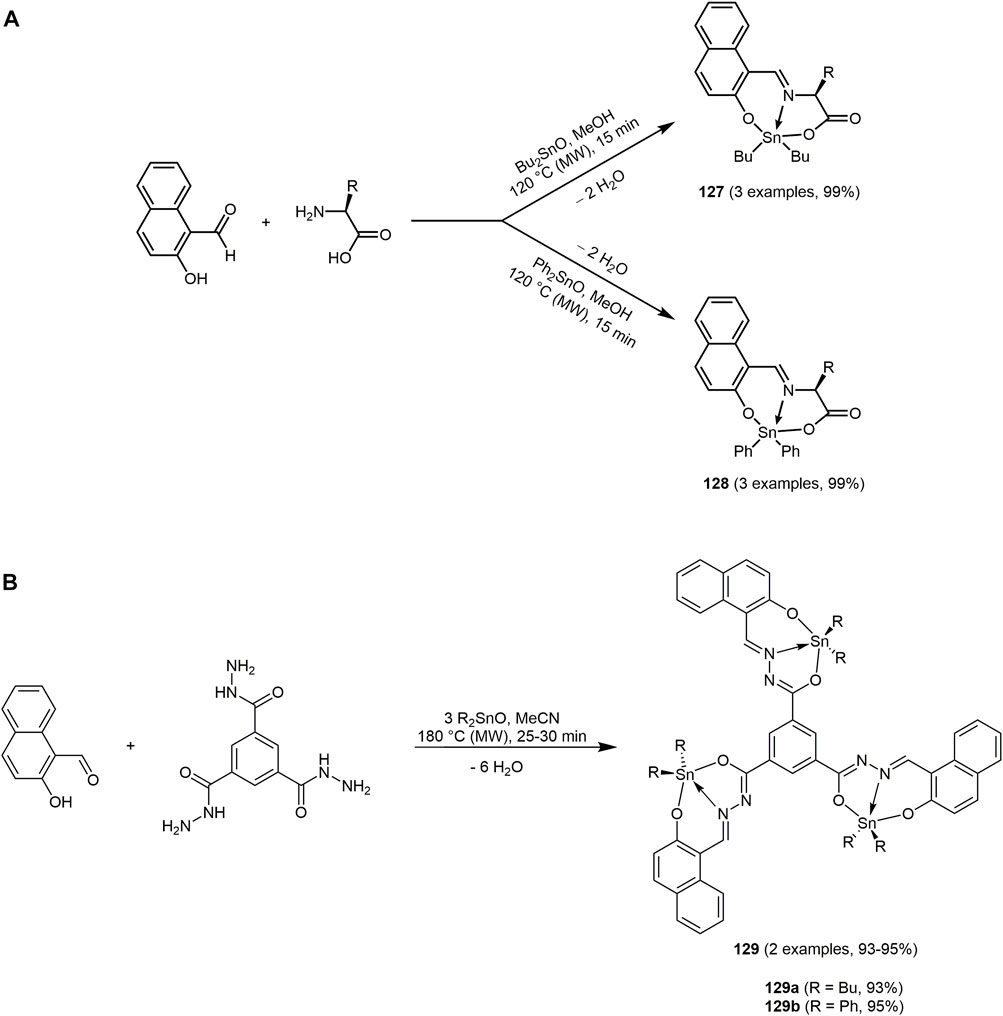
SCHEME 24. (A) One-pot condensation reaction to synthesized pentacoordinate and chiral organotin compounds 127 and 128 derived from amino acid based Schiff bases (Lara-Ceron et al., 2017). (B) Microwave-assisted pseudo seven-component condensation reaction to synthesize two fluorescent pentacoordinated organotin complexes 129 derived from Schiff bases with three central tin atoms (Canton-Diaz et al., 2021).
The UV/Vis and fluorescence spectra of compounds 127 and 128 display similar maxima. Two absorption maxima are observed at 420 nm, which can be assigned to n-π* transitions of the carboxylate and imine groups, and at 335 nm, which can be assigned to the π-π* intraligand charge transfer within the naphthyl segment. The absorption maximum of the π-π* transition also differs from the free ligands (λmax,abs = 303 nm). The bathochromic shift of the bands for the tin complexes can be attributed to azomethine-N→Sn coordination. The emission can be detected at a maximum of 465 nm fluorescence quantum yields ranging from 0.08 to 0.21.
By replacing the amine with just one primary amine group by a component with three reactive moieties, such as benzene-1,3,5-tricarbohydrazide, organotin compounds with a C3-symmetric Schiff base 129 with excellent yields can be generated (Scheme 24B) (Canton-Diaz et al., 2021).
The photophysical properties of the two organotin complexes with three central tin atoms are similar to those with one central tin atom in which [N-(2-oxido-1-naphthaldehyde)-4-hydroxybenzyhydrazidate] was employed (Jiménez-Pérez et al., 2015b). However, the extinction coefficient of 129a exhibits a larger value (ε = 68,400 M−1 cm−1) and thus a larger oscillator strength. Theoretical calculations attribute the S0→S1 excitation to natural transition orbitals. The tin atoms do not interact electronically, as both organotin complexes show quantum yields in chloroform of approximately 0.52, with lifetimes of about 3 ns. Both complexes show green emission in solution.
Sn(IV)-porphyrins have an affinity for oxygen donor ligands (Arnold and Blok, 2004). Therefore, these complexes are suitable for the construction of axially coordination bound multiporphyrin arrays (Redman et al., 2001; Prodi et al., 2002; Scandola et al., 2006; Shetti et al., 2012). In a one-step procedure meso-pyridyl Sn(IV)-porphyrin, meso-hydroxyphenyl-21,23-dithiaporphyrin, and Ru(II)-porphyrin react to give Sn(IV)-porphyrin-based oligomers 130 with yields ranging from 60% to 70% (Supplementary Material S46) (Dvivedi et al., 2014). Here, the Ru(II)-porphyrins are coordinated as peripheral ligands to the meso-pyridyl group(s) of the Sn(IV)-porphyrin.
The similar overlapping absorption bands of all three constituent monomers with slight shifts in their absorption maxima and the weak interaction of the porphyrin units in the oligomers observable in the electrochemical study indicate that the various porphyrin units of compound 130 act as decoupled supramolecular arrays. However, the steady state fluorescence study shows emission quenching in presence of the Ru(II)-porphyrin units, presumably caused by strong spin-orbit coupling.
7.2 Peptoidic dyes
Phthalocyanines are structurally related to porphyrins. This macrocyclic chromophore can be employed in Ugi 4CR in a scaffold approach to access sidechain modified metallophthalocyanines 131 and 132 (Scheme 25A) (Afshari et al., 2019). The electrochemical and optical properties as well as the solubility of phthalocyanines can be adjusted by rational tuning of the metal center as well as modification and functionalization of substrates. In the case of dye 131, where cobalt, copper, iron, nickel, and zinc ions are implemented as metal centers, the phthalocyanine acts as the carboxylic acid component in the Ugi reaction. For the synthesis of the cobalt complex 132, tetra-amino cobalt (II) phthalocyanine acts as the starting material, underling the substrate diversity of the synthetic route.
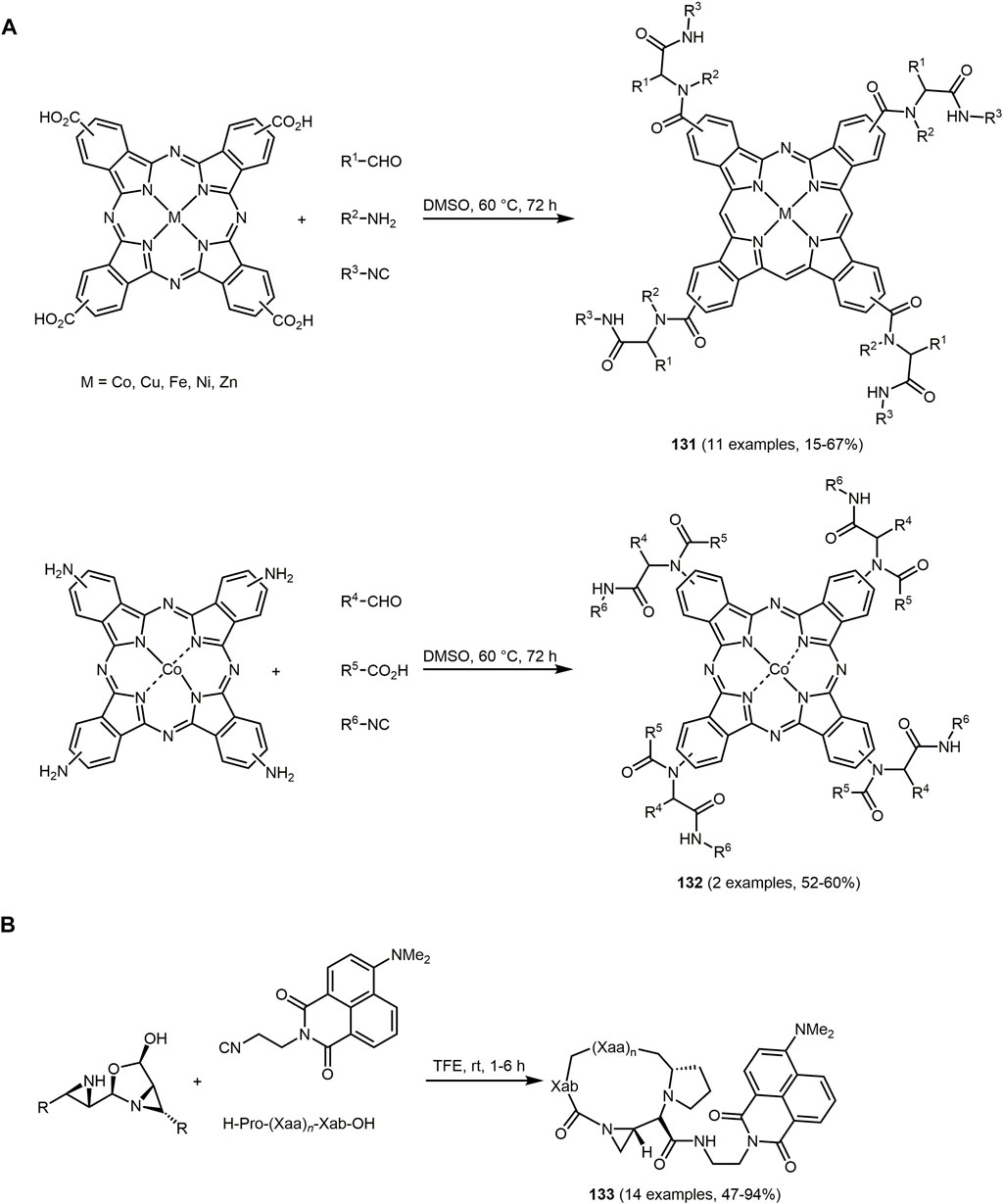
SCHEME 25. (A) One-pot catalyst-free Ugi 4CR for the synthesis carboxamide-modified-metallophthalocyanines 131 and 132 (Afshari et al., 2019). (B) One-pot synthesis of fluorophore decorated macrocyclic peptides 133 (Rotstein et al., 2011).
The phthalocyanines containing carboxamide moieties 131 and 132 are characterized by high stability, diminished aggregation and increased of monomerization, resulting in good solubilty of the metallophthalocyanines in common solvents, especially in water.
Peptides perform a wide range of physiological functions in the human organism and possess biochemical properties that are valuable for drug development (Sánchez and Vázquez, 2017). Therefore, synthetic peptides have found numerous applications in the cellular arena and tagging them with fluorophores makes them suitable fluorescent imaging agents and activity-based probes (Blum et al., 2005; Loving and Imperiali, 2009; Sainlos et al., 2009; Baumes et al., 2010; Kwan et al., 2011). However, peptides have limited stability against proteolysis in vivo (Henninot et al., 2018; Lee et al., 2019). Cyclic peptides possess higher in vivo proteolytic stability and better cellular permeability (Matsuzaki et al., 1997; Gudmundsson et al., 1999; Fletcher et al., 2008). A possible synthetic route to fluorophore decorated macrocyclic peptides 133 is offered by the multicomponent reaction of peptides, aziridine aldehydes and isocyanides bearing a solvatochromic fluorophore (Scheme 25B) (Rotstein et al., 2011). The fluorescent peptide macrocycles 133 are fluorescent and their maximum emission incorporated di- and tripeptides lies in the range from 495 to 500 nm.
8 Conclusion and outlook
MCRs are a valuable tool for synthesizing functional organic chromophores with unique photophysical and electrochemical features. Two strategies, the scaffold and the chromophore concepts, have to provide the targeted structures, where chromophores are either ligated to a scaffold (which also can constitute a new chromophore) or where the chromophore, mostly linear and cyclic conjugated systems, is formed in a chromogenic fashion. Besides aiming for new chromophores, arrays of established chromophores, or providing systems for establishing systematic structure property relationships, in recent years diversity-oriented syntheses relying on green approaches involving environmentally friendly solvents, catalysts and purification processes have become increasingly important and intellectually challenging in the field of MCRs. Tailormade functional π-systems accessed by MCR not only provide fluorophores with AIE effects for extensive application possibilities as luminescent materials in optoelectronics (OLED, OFET, DSSC, OPV), but also reach by analytics applications beyond to life science and biomedical engineering. Furthermore, newly explored effects, such as AIE (aggregation-induced emission) or TADF (thermally activated delayed fluorescence), demand suitable tunable chromophores. Therefore, existing and still undiscovered MCRs will also provide synthetic solutions for tackling new actual and future scientific problems in chromophore research, which has become an evergreen in chemical sciences.
Author’s Note
Dedicated to the memory of Prof. Dr. Mikhail Krasavin (1975–2023).
Author contributions
LB wrote the first draft of the manuscript and was involved in reviewing and editing. TJJM conceptualized the topic, was involved in reviewing and editing and was responsible for funding acquisition.
Funding
The Fonds der Chemischen Industrie supported this work by ad personam funding for TJJM.
Acknowledgments
The authors cordially thank the Fonds der Chemischen Industrie for support.
Conflict of interest
The authors declare that the research was conducted in the absence of any commercial or financial relationships that could be construed as a potential conflict of interest.
Publisher’s note
All claims expressed in this article are solely those of the authors and do not necessarily represent those of their affiliated organizations, or those of the publisher, the editors and the reviewers. Any product that may be evaluated in this article, or claim that may be made by its manufacturer, is not guaranteed or endorsed by the publisher.
Supplementary material
The Supplementary Material for this article can be found online at: https://www.frontiersin.org/articles/10.3389/fchem.2023.1124209/full#supplementary-material
References
Abaee, M. S., Hadizadeh, A., Mojtahedi, M. M., and Halvagar, M. R. (2017). Exploring the scope of the Gewald reaction: Expansion to a four-component process. Tetrahedron Lett. 58, 1408–1412. doi:10.1016/j.tetlet.2017.02.071
Abdel-Mottaleb, M. S. A., Antonious, M. S., Ali, M. M. A., Ismail, L. F. M., El-Sayed, B. A., and Sherief, A. M. K. (1992). Photophysics and dynamics of coumarin laser dyes and their analytical implications. Proc. Indian Acad. Sci. 104, 185. doi:10.1007/BF02863363
Abraham, S., Mallia, V. A., Ratheesh, K. V., Tamaoki, N., and Das, S. (2006). Reversible thermal and Photochemical switching of liquid crystalline phases and luminescence in Diphenylbutadiene-based Mesogenic dimers. J. Am. Chem. Soc. 128, 7692–7698. doi:10.1021/ja061575k
Abu-Reziq, R., Wang, D., Post, M., and Alper, H. (2008). Separable catalysts in one-pot syntheses for greener chemistry. Chem. Mat. 20, 2544–2550. doi:10.1021/cm703208w
Affeldt, R. F., de Amorim Borges, A. C., Russowsky, D., and Severo Rodembusch, F. (2014). Synthesis and fluorescence properties of benzoxazole-1,4-dihydropyridine dyads achieved by a multicomponent reaction. New J. Chem. 38, 4607–4614. doi:10.1039/C4NJ00777H
Affeldt, R. F., Iglesias, R. S., Rodembusch, F. S., and Russowsky, D. (2012). Photophysical properties of a series of 4-aryl substituted 1,4-dihydropyridines. J. Phys. Org. Chem. 25, 769–777. doi:10.1002/poc.2916
Afshari, R., Ghasemi, V., Shaabani, S., Shaabani, A., Aladaghlo, Z., and Fakhari, A. R. (2019). Post-modification of phthalocyanines via isocyanide-based multicomponent reactions: Highly dispersible peptidomimetic metallophthalocyanines as potent photosensitizers. Dyes Pigments 166, 49–59. doi:10.1016/j.dyepig.2019.03.018
Al-Awadi, N. A., Ibrahim, M. R., Elnagdi, M. H., John, E., and Ibrahim, Y. A. (2012). Enaminones in a multicomponent synthesis of 4-aryldihydropyridines for potential applications in photoinduced intramolecular electron-transfer systems. Beilstein J. Org. Chem. 8, 441–447. doi:10.3762/bjoc.8.50
Albada, B., and Metzler-Nolte, N. (2016). Organometallic–peptide bioconjugates: Synthetic strategies and medicinal applications. Chem. Rev. 116, 11797–11839. doi:10.1021/acs.chemrev.6b00166
Ali, R., Razi, S. S., Srivastava, P., and Misra, A. (2015). Tetrasubstituted imidazole core containing ESIPT fluorescent chemodosimeter for selective detection of cyanide in different medium. Sens. Actuators B Chem. 221, 1236–1247. doi:10.1016/j.snb.2015.07.087
Altaf, A. A., Shahzad, A., Gul, Z., Rasool, N., Badshah, A., Lal, B., et al. (2015). A review on the medicinal importance of pyridine derivatives. Drug Des. Med. Chem. 1, 1–11. doi:10.11648/j.jddmc.20150101.11
Álvarez, R., Puebla, P., Díaz, J. F., Bento, A. C., García-Navas, R., de la Iglesia-Vicente, J., et al. (2013). Endowing indole-based tubulin inhibitors with an anchor for derivatization: Highly potent 3-substituted indolephenstatins and indoleisocombretastatins. J. Med. Chem. 56, 2813–2827. doi:10.1021/jm3015603
Amarnath, V., and Amarnath, K. (1995). Intermediates in the Paal-Knorr synthesis of furans. J. Org. Chem. 60, 301–307. doi:10.1021/jo00107a006
Amat, A., Rosi, F., Miliani, C., Sgamellotti, A., and Fantacci, S. (2011). Theoretical and experimental investigation on the spectroscopic properties of indigo dye. J. Mol. Struct. 993, 43–51. doi:10.1016/j.molstruc.2010.11.046
Ameri, T., Li, N., and Brabec, C. (2013). Highly efficient organic tandem solar cells: A follow up review. Energy Environ. Sci. 6, 2390–2413. doi:10.1039/C3EE40388B
António, J. P. M., Russo, R., Carvalho, C. P., Cal, P. M. S. D., and Gois, P. M. P. (2019). Boronic acids as building blocks for the construction of therapeutically useful bioconjugates. Chem. Soc. Rev. 48, 3513–3536. doi:10.1039/C9CS00184K
Anuradha, S. Patel, Patle, R., Parameswaran, P., Jain, A., and Shard, A. (2019). Design, computational studies, synthesis and biological evaluation of thiazole-based molecules as anticancer agents. Eur. J. Pharm. Sci. 134, 20–30. doi:10.1016/j.ejps.2019.04.005
Arjona-Esteban, A., Krumrain, J., Liess, A., Stolte, M., Huang, L., Schmidt, D., et al. (2015). Influence of solid-state packing of dipolar merocyanine dyes on transistor and solar cell performances. J. Am. Chem. Soc. 137, 13524–13534. doi:10.1021/jacs.5b06722
Armstrong, N. R., Wightman, R. M., and Gross, E. M. (2001). LIGHT-EMITTING electrochemical processes. Annu. Rev. Phys. Chem. 52, 391–422. doi:10.1146/annurev.physchem.52.1.391
Arnold, D. P., and Blok, J. (2004). The coordination chemistry of tin porphyrin complexes. Coord. Chem. Rev. 248, 299–319. doi:10.1016/j.ccr.2004.01.004
Arora, P., Arora, V., Lamba, H. S., and Wadhwa, D. (2012). Importance of heterocyclic chemistry: A review. Int. J. Pharm. Sci. Res. (9), 2947–2954. doi:10.13040/IJPSR.0975-8232.3
Arshad, A., Osman, H., Bagley, M. C., Lam, C. K., Mohamad, S., and Zahariluddin, A. S. M. (2011). Synthesis and antimicrobial properties of some new thiazolyl coumarin derivatives. Eur. J. Med. Chem. 46, 3788–3794. doi:10.1016/j.ejmech.2011.05.044
Asaadi, M. R., Behmadi, H., Massoudi, A., and Shaker, M. (2019). 4-Aryl-2, 6-di(pyren-1-yl)pyridines: A facile procedure for synthesis and studying of fluorescence properties. Eurasian Chem. Commun. 1, 268–275. doi:10.33945/sami/ecc.2019.3.4
Asif, M. (2017). A mini review: Biological significances of nitrogen hetero atom containing heterocyclic compounds. Int. J. Bioorg. Chem. 2, 146–152. doi:10.11648/J.IJBC.20170203.20
Ataee-Kachouei, T., Nasr-Esfahani, M., Mohammadpoor-Baltork, I., Mirkhani, V., Moghadam, M., Tangestaninejad, S., et al. (2019). Facile and green one-pot synthesis of fluorophore chromeno 4,3-b quinolin-6-one derivatives catalyzed by halloysite Nanoclay under solvent-free conditions. ChemistrySelect 4, 2301–2306. doi:10.1002/slct.201803707
Atkinson, R. E., and Speakman, P. R. H. (1971). Synthesis and emission characteristics of some fluorescent derivatives of 2-(2-quinolyl) thiophen. J. Chem. Soc. B, 20772081. doi:10.1039/J29710002077
Atwal, K. S., Rovnyak, G. C., O'Reilly, B. C., and Schwartz, J. (1989). Substituted 1,4-dihydropyrimidines. 3. Synthesis of selectively functionalized 2-hetero-1,4-dihydropyrimidines. J. Org. Chem. 54, 5898–5907. doi:10.1021/jo00286a020
Avenier, F., and Hollfelder, F. (2009). Combining medium effects and Cofactor catalysis: Metal-coordinated Synzymes Accelerate phosphate transfer by 108. Chem. Eur. J. 15, 12371–12380. doi:10.1002/chem.200802616
Balalaie, S., Bigdeli, M. A., Sheikhhosseini, E., Habibi, A., Moghadam, H. P., and Naderi, M. (2012). Efficient synthesis of novel coumarin-3-carboxamides (=2-Oxo-2H-1-benzopyran-3-carboxamides) containing lipophilic spacers. Helv. Chim. Acta 95, 528–535. doi:10.1002/hlca.201100238
Balme, G. (2004). Pyrrole syntheses by multicomponent coupling reactions. Angew. Chem. Int. Ed. 43, 6238–6241. doi:10.1002/anie.200461073
Balzani, A. C. V., and Venturi, M. (2003). Molecular devices and machines – a journey into the nano world. Weinheim: Wiley-VCH Verlag GmbH. doi:10.1002/3527601600.ch4
Balzani, V. (2001). Adv. Electron transf. Chem. Weinheim: Wiley-VCH Verlag GmbH. doi:10.1002/9783527618248.fmatter
Baradaran-Sirjani, Z., Roshani, M., Khashi, M., and Beyramabadi, S. A. (2018). Catalytic performance of Preyssler heteropolyacids and synthesis, experimental, theoretical characterizations, fluorescence properties of 1,8-dioxodecahydroacridine derivative. Indian J. Chem. B 57, 715–721.
Barrett, M. P., and Croft, S. L. (2012). Management of trypanosomiasis and leishmaniasis. Brit. Med. Bull. 104, 175–196. doi:10.1093/bmb/lds031
Bartkowiak, W., Zaleśny, R., Niewodniczański, W., and Leszczynski, J. (2001). Quantum chemical calculations of the first- and second-order Hyperpolarizabilities of molecules in solutions. J. Phys. Chem. A 105, 10702–10710. doi:10.1021/jp010682s
Baruah, M., Qin, W., Flors, C., Hofkens, J., Vallée, R. A. L., Beljonne, D., et al. (2006). Solvent and pH dependent fluorescent properties of a Dimethylaminostyryl Borondipyrromethene dye in solution. J. Phys. Chem. A 110, 5998–6009. doi:10.1021/jp054878u
Batalin, S. D., Golikova, M. A., Khrustaleva, A. A., Bakal, A. A., Ponomareva, T. S., and Goryacheva, I. Y. (2021). Substituted 2-(ortho-hydroxyaryl)cyclopenta b pyridines: Synthesis and fluorescent properties under neutral, acidic medium and solid state. ChemistrySelect 6, 11375–11380. doi:10.1002/slct.202103128
Battilocchio, C., Poce, G., Alfonso, S., Porretta, G. C., Consalvi, S., Sautebin, L., et al. (2013). A class of pyrrole derivatives endowed with analgesic/anti-inflammatory activity. Bioorgan. Med. Chem. 21, 3695–3701. doi:10.1016/j.bmc.2013.04.031
Baumes, J. M., Gassensmith, J. J., Giblin, J., Lee, J.-J., White, A. G., Culligan, W. J., et al. (2010). Storable, thermally activated, near-infrared chemiluminescent dyes and dye-stained microparticles for optical imaging. Nat. Chem. 2, 1025–1030. doi:10.1038/nchem.871
Bay, S., Makhloufi, G., Janiak, C., and Müller, T. J. J. (2014). The Ugi four-component reaction as a concise modular synthetic tool for photo-induced electron transfer donor-anthraquinone dyads. Beilstein J. Org. Chem. 10, 1006–1016. doi:10.3762/bjoc.10.100
Bay, S., and Müller, T. J. J. (2014). Phenothiazine-aromatic Hydrocarbon acceptor dyads as photo-induced electron transfer systems by Ugi four-component reaction. Z. Naturforsch. B 69, 541–553. doi:10.5560/znb.2014-4060
Bay, S., Villnow, T., Ryseck, G., Rai-Constapel, V., Gilch, P., and Müller, T. J. J. (2013). The Ugi four-component reaction route to photoinducible electron-transfer systems. ChemPlusChem 78, 137–141. doi:10.1002/cplu.201200279
Bell, J. D., Blount, J. F., Briscoe, O. V., and Freeman, H. C. (1968). The crystal structure of phenothiazine. Chem. Commun., 16561657. doi:10.1039/c19680001656
Bellina, F., Cauteruccio, S., and Rossi, R. (2007). Synthesis and biological activity of vicinal diaryl-substituted 1H-imidazoles. Tetrahedron 63, 4571–4624. doi:10.1016/j.tet.2007.02.075
Benkhaya, B., El Harfi, S., and El Harfi, A. (2017). Classifications, properties and applications of textile dyes: A review. Appl. J. Envir. Eng. Sci. 3. doi:10.48422/IMIST.PRSM/ajees-v3i3.9681
Benkhaya, S., M'Rabet, S., and El Harfi, A. (2020). Classifications, properties, recent synthesis and applications of azo dyes. Heliyon 6, e03271. doi:10.1016/j.heliyon.2020.e03271
Beyaert, R., Cuenda, A., Vanden Berghe, W., Plaisance, S., Lee, J. C., Haegeman, G., et al. (1996). The p38/RK mitogen-activated protein kinase pathway regulates interleukin-6 synthesis response to tumor necrosis factor. EMBO J. 15, 1914–1923. doi:10.1002/j.1460-2075.1996.tb00542.x
Bhat, U. G., Halasi, M., and Gartel, A. L. (2009). Thiazole antibiotics target FoxM1 and induce apoptosis in human cancer cells. PloS one 4, e5592. doi:10.1371/journal.pone.0005592
Bhowmik, B. B., and Ganguly, P. (2005). Photophysics of xanthene dyes in surfactant solution. Spectrochim. Acta A. 61, 1997–2003. doi:10.1016/j.saa.2004.07.031
Bienaymé, H., and Bouzid, K. (1998). A new heterocyclic multicomponent reaction for the combinatorial synthesis of fused 3-Aminoimidazoles. Angew. Chem. Int. Ed. 37, 2234–2237. doi:10.1002/(SICI)1521-3773(19980904)37:16<2234:AID-ANIE2234>3.0.CO;2-R
Bienaymé, H., Hulme, C., Oddon, G., and Schmitt, P. (2000). Maximizing synthetic efficiency: Multi-component transformations lead the way. Chem. Eur. J. 6, 3321–3329. doi:10.1002/1521-3765(20000915)6:18<3321:AID-CHEM3321>3.0.CO;2-A
Biesen, L., Krenzer, J., Nirmalananthan-Budau, N., Resch-Genger, U., and Müller, T. J. J. C. S. (2022). Asymmetrically bridged aroyl-S, N-ketene acetal-based multichromophores with aggregation-induced tunable emission. Chem. Sci. 13, 5374–5381. doi:10.1039/D2SC00415A
Biesen, L., May, L., Nirmalananthan-Budau, N., Hoffmann, K., Resch-Genger, U., and Müller, T. J. J. (2021). Communication of bichromophore emission upon aggregation – aroyl-S,N-ketene acetals as Multifunctional sensor merocyanines. Chem. Eur. J. 27, 13426–13434. doi:10.1002/chem.202102052
Biesen, L., and Müller, T. J. J. (2021). Multicomponent and one-pot syntheses of quinoxalines. Adv. Synth. Catal. 363, 980–1006. doi:10.1002/adsc.202001219
Biesen, L., Nirmalananthan-Budau, N., Hoffmann, K., Resch-Genger, U., and Müller, T. J. J. (2020). Solid-state emissive aroyl-S,N-ketene acetals with tunable aggregation-induced emission characteristics. Angew. Chem. Int. Ed. 59, 10037–10041. doi:10.1002/anie.201916396
Biswas, A. K., Das, A., and Ganguly, B. (2016). Can fused-pyrrole rings act as better π-spacer units than fused-thiophene in dye-sensitized solar cells? A computational study. New J. Chem. 40, 9304–9312. doi:10.1039/C6NJ02040B
Bizarro, A., Sousa, D., Lima, R. T., Musso, L., Cincinelli, R., Zuco, V., et al. (2018). Synthesis and evaluation of the tumor cell Growth inhibitory potential of new Putative HSP90 inhibitors. Molecules 23, 407. doi:10.3390/molecules23020407
Blackburn, C., Guan, B., Fleming, P., Shiosaki, K., and Tsai, S. (1998). Parallel synthesis of 3-aminoimidazo[1,2-a]pyridines and pyrazines by a new three-component condensation. Tetrahedron Lett. 39, 3635–3638. doi:10.1016/S0040-4039(98)00653-4
Blum, G., Mullins, S. R., Keren, K., Fonovič, M., Jedeszko, C., Rice, M. J., et al. (2005). Dynamic imaging of protease activity with fluorescently quenched activity-based probes. Nat. Chem. Biol. 1, 203–209. doi:10.1038/nchembio728
Boden, C., and Pattenden, G. (1994). Total synthesis of lissoclinamide 5, a cytotoxic cyclic peptide from the tunicate Lissoclinum patella. Tetrahedron Lett. 35, 8271–8274. doi:10.1016/0040-4039(94)88300-9
Boens, N., Leen, V., and Dehaen, W. (2012). Fluorescent indicators based on BODIPY. Fluoresc. Indic. based BODIPY 41, 1130–1172. doi:10.1039/C1CS15132K
Bridges, J. W., and Williams, R. T. (1968). The fluorescence of indoles and aniline derivatives. Biochem. J. 107, 225–237. doi:10.1042/bj1070225
Briehn, C. A., and Bäuerle, P. (2002). From solid-phase synthesis of pi-conjugated oligomers to combinatorial library construction and screening. Chem. Commun. 2, 1015–1023. doi:10.1039/b108846g
Brooker, L. G. S., Keyes, G. H., Sprague, R. H., VanDyke, R. H., VanLare, E., VanZandt, G., et al. (1951). Studies in the cyanine dye series. XI. 1 the merocyanines. J. Am. Chem. Soc. 73, 5326–5332. doi:10.1021/ja01155a095
Brown, R. C. D. (2005). Developments in furan syntheses. Angew. Chem. Int. Ed. 44, 850–852. doi:10.1002/anie.200461668
Bu, X. R., Li, H., Van Derveer, D., and Mintz, E. A. (1996). A novel approach to synthesis of tricyanovinylthiophene for heterocyclic imidazole nonlinear optical chromophores. Tetrahedron Lett. 37, 7331–7334. doi:10.1016/0040-4039(96)01638-3
Bünau, G. v., and Birks, J. B. (1970). Photophysics of aromatic molecules, 74. Wiley-Interscience, 1294–1295. doi:10.1002/bbpc.19700741223
Burange, A. S., Gadam, K. G., Tugaonkar, P. S., Thakur, S. D., Soni, R. K., Khan, R. R., et al. (2021). Green synthesis of xanthene and acridine-based heterocycles of pharmaceutical importance: A review. Environ. Chem. Lett. 19, 3283–3314. doi:10.1007/s10311-021-01223-w
Burchak, O. N., Mugherli, L., Ostuni, M., Lacapere, J. J., and Balakirev, M. Y. (2011). Combinatorial discovery of fluorescent pharmacophores by multicomponent reactions in droplet arrays. J. Am. Chem. Soc. 133, 10058–10061. doi:10.1021/ja204016e
Bürckstümmer, H., Kronenberg, N. M., Meerholz, K., and Würthner, F. (2010). Near-infrared absorbing merocyanine dyes for bulk heterojunction solar cells. Org. Lett. 12, 3666–3669. doi:10.1021/ol101492q
Cairo, C. W., Key, J. A., and Sadek, C. (2010). Fluorescent small-molecule probes of biochemistry at the plasma membrane. Curr. Opin. Chem. Biol. 14, 57–63. doi:10.1016/j.cbpa.2009.09.032
Canton-Diaz, A., Munoz-Flores, B. M., Berrones-Reyes, J., Moggio, I., Arias, E., Turlakov, G., et al. (2021). Organotin compounds bearing C-3-symmetric Schiff base: Microwave-assisted multicomponent synthesis and their photophysical properties. J. Organomet. Chem. 2021, 122111–122955. doi:10.1016/j.jorganchem.2021.122111
Chang, D. W., Lee, H. J., Kim, J. H., Park, S. Y., Park, S.-M., Dai, L., et al. (2011). Novel quinoxaline-based organic sensitizers for dye-sensitized solar cells. Org. Lett. 13, 3880–3883. doi:10.1021/ol2012378
Chang, L. C. W., von Frijtag Drabbe Künzel, J. K., Mulder-Krieger, T., Spanjersberg, R. F., Roerink, S. F., van den Hout, G., et al. (2005). A series of ligands displaying a remarkable Agonistic−Antagonistic profile at the Adenosine A1 receptor. J. Med. Chem. 48, 2045–2053. doi:10.1021/jm049597+
Chang, Y.-T., Hsu, S.-L., Chen, G.-Y., Su, M.-H., Singh, T. A., Diau, E. W.-G., et al. (2008). Intramolecular donor–acceptor Regioregular Poly(3-hexylthiophene)s presenting Octylphenanthrenyl-imidazole moieties exhibit enhanced charge transfer for heterojunction solar cell applications. Adv. Funct. Mat. 18, 2356–2365. doi:10.1002/adfm.200701150
Chang, Y.-T., Hsu, S.-L., Su, M.-H., and Wei, K.-H. (2009). Intramolecular donor–acceptor Regioregular Poly(hexylphenanthrenyl-imidazole thiophene) exhibits enhanced hole Mobility for heterojunction solar cell applications. Adv. Mat. 21, 2093–2097. doi:10.1002/adma.200802379
Chen, C.-T., Wagner, H., and Still, W. C. (1998). Fluorescent, sequence-selective peptide detection by synthetic small molecules. Science 279, 851–853. doi:10.1126/science.279.5352.851
Chen, J., Gao, P., Yu, F., Yang, Y., Zhu, S., and Zhai, H. (2012). Total synthesis of (±)-Merrilactone A. Angew. Chem. Int. Ed. 51, 5897–5899. doi:10.1002/anie.201200378
Chen, Y., Xu, C., Xu, B., Mao, Z., Li, J.-A., Yang, Z., et al. (2019). Chirality-activated mechanoluminescence from aggregation-induced emission enantiomers with high contrast mechanochromism and force-induced delayed fluorescence. Mat. Chem. Front. 3, 1800–1806. doi:10.1039/C9QM00312F
Chène, P. (2003). Inhibiting the p53–MDM2 interaction: An important target for cancer therapy. Nat. Rev. Cancer 3, 102–109. doi:10.1038/nrc991
Cieplik, J., Stolarczyk, M., Pluta, J., Gubrynowicz, O., Bryndal, I., Lis, T., et al. (2011). Synthesis and antibacterial properties of pyrimidine derivatives. Acta Pol. Pharm. 68, 57–65.
Corona-Lopez, M. M., Munoz-Flores, B. M., Chaari, M., Nunez, R., and Jimenez-Perez, V. M. (2021). Far-red and near-infrared boron schiff bases (BOSCHIBAs) dyes bearing anionic boron Clusters. Eur. Heart J. 2021, 2047–2054. doi:10.1002/ejic.202100144
Cruz-Monserrate, Z., Vervoort, H. C., Bai, R., Newman, D. J., Howell, S. B., Los, G., et al. (2003). Diazonamide A and a synthetic structural analog: Disruptive effects on Mitosis and cellular Microtubules and analysis of their interactions with Tubulin. Mol. Pharmacol. 63, 1273–1280. doi:10.1124/mol.63.6.1273
Csende, F., and Porkoláb, A. (2018). A review on antibacterial activity of some isoindole derivatives. Der Pharma. Chem. 10, 43–50.
Csuk, R., Heinold, A., Siewert, B., Schwarz, S., Barthel, A., Kluge, R., et al. (2012). Synthesis and biological evaluation of antitumor-active Arglabin derivatives. Arch. Pharm. Pharm. Med. Chem. 345, 215–222. doi:10.1002/ardp.201100065
Curtin, M. L., Florjancic, A. S., Cohen, J., Gu, W.-Z., Frost, D. J., Muchmore, S. W., et al. (2003). Novel and selective imidazole-containing biphenyl inhibitors of protein farnesyltransferase. Bioorgan. Med. Chem. Lett. 13, 1367–1371. doi:10.1016/S0960-894X(03)00096-9
D'Souza, D. M., and Müller, T. J. J. (2007). Multi-component syntheses of heterocycles by transition-metal catalysis. Chem. Soc. Rev. 36, 1095–1108. doi:10.1039/B608235C
D'Souza, D. M., Muschelknautz, C., Rominger, F., and Müller, T. J. J. (2010). Unusual solid-state luminescent push-pull indolones: A general one-pot three-component approach. Org. Lett. 12, 3364–3367. doi:10.1021/ol101165m
da Silveira Pinto, L. S., Couri, M. R. C., and de Souza, M. V. N. (2018). Multicomponent reactions in the synthesis of complex fused coumarin derivatives. Curr. Org. Chem. 15, 21–37. doi:10.2174/1570179414666170614124053
Dabiri, M., Arvin-Nezhad, H., Khavasi, H. R., and Bazgir, A. (2007). A novel and efficient synthesis of pyrimido[4,5-d]pyrimidine-2,4,7-trione and pyrido[2,3-d:6,5-d] dipyrimidine-2,4,6,8-tetrone derivatives. Tetrahedron 63, 1770–1774. doi:10.1016/j.tet.2006.12.043
Daidone, G., Maggio, B., and Schillaci, D. (1990). Salicylanilide and its heterocyclic analogues. A comparative study of their antimicrobial activity. Pharmazie 45, 441–442.
Das, S., Varghese, S., and Kumar, N. S. S. (2010). Butadiene-based photoresponsive soft materials. Langmuir 26, 1598–1609. doi:10.1021/la901962k
Davis, R., Mallia, V. A., and Das, S. (2003). Reversible Photochemical phase transition behavior of alkoxy-cyano-substituted Diphenylbutadiene liquid crystals. Chem. Mat. 15, 1057–1063. doi:10.1021/cm020710z
Davis, R., Mallia, V. A., Das, S., and Tamaoki, N. (2004). Butadienes as novel photochromes for color tuning of cholesteric glasses: Influence of microscopic molecular reorganization within the helical superstructure. Adv. Funct. Mat. 14, 743–748. doi:10.1002/adfm.200305190
Davis, R., Saleesh Kumar, N. S., Abraham, S., Suresh, C. H., Rath, N. P., Tamaoki, N., et al. (2008). Molecular packing and solid-state fluorescence of alkoxy-cyano substituted Diphenylbutadienes: Structure of the luminescent aggregates. J. Phys. Chem. C 112, 2137–2146. doi:10.1021/jp710352m
de Brito, A. C. F., Correa, R. S., Pinto, A. A., Matos, M. J. S., Tenorio, J. C., Taylor, J. G., et al. (2018). Synthesis, crystal structure, photophysical properties and theoretical studies of a novel bis(phenylisoxazolyl) benzene derivative. J. Mol. Struct. 1163, 197–204. doi:10.1016/j.molstruc.2018.03.009
De Esch, I. J. P., Vollinga, R. C., Goubitz, K., Schenk, H., Appelberg, U., Hacksell, U., et al. (1999). Characterization of the binding site of the histamine H3 receptor. 1. Various approaches to the synthesis of 2-(1H-Imidazol-4-yl)cyclopropylamine and Histaminergic activity of (1R,2R)- and (1S,2S)-2-(1H-Imidazol-4-yl)- cyclopropylamine. J. Med. Chem. 42, 1115–1122. doi:10.1021/jm9810912
de Moliner, F., Kielland, N., Lavilla, R., and Vendrell, M. (2017). Modern synthetic Avenues for the preparation of functional fluorophores. Angew. Chem. Int. Ed. 56, 3758–3769. doi:10.1002/anie.201609394
de Souza, V. P., Santos, F. S., Rodembusch, F. S., Braga, C. B., Ornelas, C., Pilli, R. A., et al. (2020). Hybrid 3,4-dihydropyrimidin-2-(thi)ones as dual-functional bioactive molecules: Fluorescent probes and cytotoxic agents to cancer cells. New J. Chem. 44, 12440–12451. doi:10.1039/D0NJ01368D
Debus, H. (1858). Ueber die Einwirkung des Ammoniaks auf Glyoxal. Liebigs Ann. Chem. 107, 199–208. doi:10.1002/jlac.18581070209
Deden, T., May, L., Reiss, G. J., and Müller, T. J. J. (2020). Rapid sequentially palladium catalyzed four-component synthesis of novel fluorescent biaryl-substituted isoxazoles. Catalysts 10, 1412. doi:10.3390/catal10121412
Denissen, M., Kraus, A., Reiss, G. J., and Müller, T. J. J. (2017). One-pot syntheses of blue-luminescent 4-aryl-1H-benzo[f]isoindole-1,3(2H)-diones by T3P® activation of 3-arylpropiolic acids. Beilstein J. Org. Chem. 13, 2340–2351. doi:10.3762/bjoc.13.231
Denissen, M., Nirmalananthan, N., Behnke, T., Hoffmann, K., Resch-Genger, U., and Müller, T. J. J. (2017). 3-Piperazinyl propenylidene indolone merocyanines: Consecutive three-component synthesis and electronic properties of solid-state luminophores with AIE properties. Mat. Chem. Front. 1, 2013–2026. doi:10.1039/c7qm00198c
Denißen, M., Hannen, R., Itskalov, D., Biesen, L., Nirmalananthan-Budau, N., Hoffmann, K., et al. (2020). One-pot synthesis of a white-light emissive bichromophore operated by aggregation-induced dual emission (AIDE) and partial energy transfer. Chem. Commun. 56, 7407–7410. doi:10.1039/D0CC03451G
Denmark, S. E., and Tymonko, S. A. (2005). Sequential cross-coupling of 1,4-Bissilylbutadienes: Synthesis of unsymmetrical 1,4-disubstituted 1,3-butadienes. J. Am. Chem. Soc. 127, 8004–8005. doi:10.1021/ja0518373
Diehl, H., and Markuszewski, R. (1989). Studies on fluorescein—VII: The fluorescence of fluorescein as a function of pH. Talanta 36, 416–418. doi:10.1016/0039-9140(89)80213-9
Dikshit, D. K., Kl, M., Kapil, R. S., Kamboj, V. P., and AnaNd, N. (1974). Antifertility agent. IX. synthesis of 2, 3, 4-Triphenylfurans & 2, 3, 4-Triphenyl-5-Methylfurans. Indian J. Chem. 12, 1144–1146.
Dikshit, D. K., Singh, S., Singh, M. M., and Kamboj, V. P. (1991). ChemInform abstract: Synthesis and biological activity of 2,3- and 3,4-Diarylfurans and 2,3, 4-Triaryl-2,5-dihydrofurans. ChemInform 22, 22. doi:10.1002/chin.199103156
Dini, D. (2005). Electrochemiluminescence from organic emitters. Chem. Mat. 17, 1933–1945. doi:10.1021/cm049567v
Dömling, A., and Ugi, I. (2000). Multicomponent reactions with isocyanides. Angew. Chem. Int. Ed. 39, 3168–3210. doi:10.1002/1521-3773(20000915)39:18<3168:AID-ANIE3168>3.0.CO;2-U
dos Santos, G. C., Bartolomeu, A. d. A., Ximenes, V. F., and da Silva-Filho, L. C. (2017). Facile synthesis and photophysical characterization of new quinoline dyes. J. Fluoresc. 27, 271–280. doi:10.1007/s10895-016-1954-5
Drießen, D., Biesen, L., and Müller, T. J. J. (2021). Sequentially catalyzed three-component Masuda–Suzuki–Sonogashira synthesis of fluorescent 2-Alkynyl-4-(7-azaindol-3-yl)pyrimidines: Three palladium-catalyzed processes in a one-pot fashion. Synlett 32, 491–496. doi:10.1055/s-0040-1707818
Dua, R., Shrivastava, S., Sonwane, S. K., and Srivastava, S. K. (2011). Pharmacological significance of synthetic heterocycles scaffold: A review. Adv. Biol. Res. 5, 120–144.
Duc, D. X. (2019). Recent progress in the synthesis of furan. Mini. Rev. Org. Chem. 16, 422–452. doi:10.2174/1570193X15666180608084557
Durani, N., Jain, R., Saeed, A., Dikshit, D. K., Durani, S., and Kapil, R. S. (1989). Structure-activity relationship of antiestrogens. A study using triarylbutenone, benzofuran, and triarylfuran analogs as models for triarylethylenes and triarylpropenones. J. Med. Chem. 32, 1700–1707. doi:10.1021/jm00128a006
Dvivedi, A., Pareek, Y., and Ravikanth, M. (2014). Sn-IV porphyrin scaffolds for axially bonded multiporphyrin arrays: Synthesis and structure Elucidation by NMR studies. Chem. Eur. J. 20, 4481–4490. doi:10.1002/chem.201304344
Edraki, N., Mehdipour, A. R., Khoshneviszadeh, M., and Miri, R. (2009). Dihydropyridines: Evaluation of their current and future pharmacological applications. Drug Discov. Today 14, 1058–1066. doi:10.1016/j.drudis.2009.08.004
Ekbote, A., Mobin, S. M., and Misra, R. (2020). Stimuli-responsive phenothiazine-based donor–acceptor isomers: AIE, mechanochromism and polymorphism. J. Mat. Chem. C 8, 3589–3602. doi:10.1039/C9TC05192A
El-Subbagh, H. I., Abu-Zaid, S. M., Mahran, M. A., Badria, F. A., and Al-Obaid, A. M. (2000). Synthesis and biological evaluation of Certain α,β-unsaturated ketones and their corresponding fused pyridines as antiviral and cytotoxic agents. J. Med. Chem. 43, 2915–2921. doi:10.1021/jm000038m
Elgemeie, G. H., Ahmed, K. A., Ahmed, E. A., Helal, M. H., and Masoud, D. M. (2015). Microwave assisted one-pot synthesis of pyrazoloquinazolinone derivatives and their application on printing of synthetic fabrics. Pigm. Resin Technol. 44, 165–171. doi:10.1108/prt-04-2014-0030
Elz, S., Kramer, K., Pertz, H. H., Detert, H., ter Laak, A. M., Kühne, R., et al. (2000). Histaprodifens: Synthesis, pharmacological in vitro evaluation, and molecular modeling of a new class of highly active and selective histamine H1-Receptor Agonists. J. Med. Chem. 43, 1071–1084. doi:10.1021/jm991056a
Entwistle, C. D., and Marder, T. B. (2004). Applications of three-Coordinate organoboron compounds and polymers in optoelectronics. Chem. Mat. 16, 4574–4585. doi:10.1021/cm0495717
Entwistle, C. D., and Marder, T. B. (2002)., 41. 2-L: CO, 2927–2931. doi:10.1002/1521-3773(20020816)41:16<2927:AID-ANIE2927>3.0Boron chemistry lights the way: Optical properties of molecular and polymeric systemsAngew. Chem. Int. Ed.
Estevez, V., Villacampa, M., and Menendez, J. C. (2010). Multicomponent reactions for the synthesis of pyrroles. Chem. Soc. Rev. 39, 4402–4421. doi:10.1039/B917644F
Estévez, V., Villacampa, M., and Menéndez, J. C. (2014). Recent advances in the synthesis of pyrroles by multicomponent reactions. Chem. Soc. Rev. 43, 4633–4657. doi:10.1039/C3CS60015G
Evans, D. L., Minster, D. K., Jordis, U., Hecht, S. M., Mazzu, A. L., and Meyers, A. I. (1979). Nickel peroxide dehydrogenation of oxygen-sulfur-and nitrogen-containing heterocycles. J. Org. Chem. 44, 497–501. doi:10.1021/jo01318a005
Fasani, E., Fagnoni, M., Dondi, D., and Albini, A. (2006). Intramolecular electron transfer in the Photochemistry of some Nitrophenyldihydropyridines. J. Org. Chem. 71, 2037–2045. doi:10.1021/jo052463z
Fayed, E. A., Bayoumi, A. H., Saleh, A. S., Ezz Al-Arab, E. M., and Ammar, Y. A. (2021). In vivo and in vitro anti-inflammatory, antipyretic and ulcerogenic activities of pyridone and chromenopyridone derivatives, physicochemical and pharmacokinetic studies. Bioorg. Chem. 109, 104742. doi:10.1016/j.bioorg.2021.104742
Fei, D.-Q., Li, S.-G., Liu, C.-M., Wu, G., and Gao, K. (2007). Eremophilane-type sesquiterpene derivatives from the roots of ligularia lapathifolia. J. Nat. Prod. 70, 241–245. doi:10.1021/np060304k
Feng, X., Tong, B., Shen, J., Shi, J., Han, T., Chen, L., et al. (2010). Aggregation-induced emission enhancement of aryl-substituted pyrrole derivatives. J. Phys. Chem. B 114, 16731–16736. doi:10.1021/jp108254g
Festa, A. A., Storozhenko, O. A., Bella Ndoutoume, D. R., Varlamov, A. V., and Voskressensky, L. G. (2017). Sequential three-component reaction of homophthalonitrile, salicylaldehydes and nitromethane. Mendeleev Commun. 27, 451–453. doi:10.1016/j.mencom.2017.09.006
Festa, A. A., Storozhenko, O. A., Golantsov, N. E., Subramani, K., Novikov, R. A., Zaitseva, S. O., et al. (2019). Homophtalonitrile for multicomponent reactions: Syntheses and optical properties of o-cyanophenyl- or indol-3-yl-substituted chromeno 2,3-c isoquinolin-5-amines. Chemistryopen 8, 23–30. doi:10.1002/open.201800207
Fletcher, J. M., Morton, C. J., Zwar, R. A., Murray, S. S., O'Leary, P. D., and Hughes, R. A. (2008). Design of a conformationally defined and proteolytically stable circular mimetic of brain-derived neurotrophic factor. J. Biol. Chem. 283, 33375–33383. doi:10.1074/jbc.M802789200
Franz, A. W., Popa, L. N., Rominger, F., and Müller, T. J. J. (2009). First synthesis and electronic properties of diphenothiazine dumbbells bridged by heterocycles. Org. Biomol. Chem. 7, 469–475. doi:10.1039/B814850C
Fredericks, S. M., Luong, J. C., and Wrighton, M. S. (1979). Multiple emissions from rhenium (I) complexes: Intraligand and charge-transfer emission from substituted metal carbonyl cations. J. Am. Chem. Soc. 101, 7415–7417. doi:10.1021/ja00518a054
Fürstner, A. (2003). Chemistry and biology of roseophilin and the prodigiosin alkaloids: A survey of the last 2500 years. Angew. Chem. Int. Ed. 42, 3582–3603. doi:10.1002/anie.200300582
Fürstner, A., Szillat, H., Gabor, B., and Mynott, R. (1998). Platinum- and acid-catalyzed enyne metathesis reactions: Mechanistic studies and applications to the syntheses of streptorubin B and metacycloprodigiosin. J. Am. Chem. Soc. 120, 8305–8314. doi:10.1021/ja981183g
Ganellin, C. R., Fkyerat, A., Bang-Andersen, B., Athmani, S., Tertiuk, W., Garbarg, M., et al. (1996). A novel series of (Phenoxyalkyl)imidazoles as potent H3-Receptor histamine Antagonists. J. Med. Chem. 39, 3806–3813. doi:10.1021/jm960138l
Garcia-Lopez, M. C., Herrera-Espana, A. D., Estupinan-Jimenez, J. R., Gonzalez-Villasana, V., Caceres-Castillo, D., Bojorquez-Quintal, E., et al. (2022). New luminescent organoboron esters based on damnacanthal: One-pot multicomponent synthesis, optical behavior, cytotoxicity, and selectivity studies against MDA-MBA-231 breast cancer cells. New J. Chem. 46, 20138–20145. doi:10.1039/d2nj03959a
Geenen, S. R., Schumann, T., and Müller, T. J. J. (2020). Fluorescent donor-acceptor psoralen cruciforms by consecutive Suzuki-Suzuki and Sonogashira-Sonogashira one-pot syntheses. J. Org. Chem. 85, 9737–9750. doi:10.1021/acs.joc.0c01059
Gers-Panther, C. F., Fischer, H., Nordmann, J., Seiler, T., Behnke, T., Wuerth, C., et al. (2017). Four- and five-component syntheses and photophysical properties of emission solvatochromic 3-Aminovinylquinoxalines. J. Org. Chem. 82, 567–578. doi:10.1021/acs.joc.6b02581
Gewald, K., Schinke, E., and Bottcher, H. (1966). Heterocyclen aus CH-aciden Nitrilen, VIII. 2-Amino-thiophene aus methylenaktiven Nitrilen, Carbonylverbindungen und Schwefel. Chem. Ber. 99, 94–100. doi:10.1002/cber.19660990116
Ghashghaei, O., Caputo, S., Sintes, M., Reves, M., Kielland, N., Estarellas, C., et al. (2018). Multiple multicomponent reactions: Unexplored substrates, selective processes, and Versatile Chemotypes in biomedicine. Chem. Eur. J. 24, 14513–14521. doi:10.1002/chem.201802877
Gholami, A., Mokhtary, M., and Nikpassand, M. (2020). Choline chloride/Oxalic acid (ChCl/Oxa) catalyzed one-pot synthesis of novel azo and sulfonated pyrimido 4,5-b quinoline derivatives. Dyes Pigments 180, 108453. doi:10.1016/j.dyepig.2020.108453
Ghosh, J., Biswas, P., Drew, M. G. B., and Bandyopadhyay, C. (2015). A one-pot three-component reaction involving 2-aminochromone in aqueous micellar medium: A green synthesis of hexahydrochromeno[2,3- $$b$$ b ]quinolinedione. Mol. Divers. 19, 541–549. doi:10.1007/s11030-015-9573-7
Ghotekar, B. K., Jachak, M. N., and Toche, R. B. (2009). New one-step synthesis of pyrazolo 1,5-a pyrimidine and pyrazolo 1,5-a quinazoline derivatives via multicomponent reactions. J. Heterocycl. Chem. 46, 708–713. doi:10.1002/jhet.128
Giri, R., Goodell, J. R., Xing, C., Benoit, A., Kaur, H., Hiasa, H., et al. (2010). Synthesis and cancer cell cytotoxicity of substituted xanthenes. Bioorgan. Med. Chem. 18, 1456–1463. doi:10.1016/j.bmc.2010.01.018
Godugu, K., Gundala, T. R., Bodapati, R., Yadala, V. D. S., Loka, S. S., and Nallagondu, C. G. R. (2020). Synthesis, photophysical and electrochemical properties of donor-acceptor type hydrazinyl thiazolyl coumarins. New J. Chem. 44, 7007–7016. doi:10.1039/d0nj00082e
Gong, J., Han, J., Liu, Q., Ren, X., Wei, P., Yang, L., et al. (2019). An ideal platform of light-emitting materials from phenothiazine: Facile preparation, tunable red/NIR fluorescence, bent geometry-promoted AIE behaviour and selective lipid-droplet (LD) tracking ability. J. Mat. Chem. C 7, 4185–4190. doi:10.1039/C9TC00143C
Goodman, J., and Brus, L. E. (1978). Proton transfer and tautomerism in an excited state of methyl salicylate. J. Am. Chem. Soc. 100, 7472–7474. doi:10.1021/ja00492a005
Goud, T. V., Tutar, A., and Biellmann, J.-F. (2006). Synthesis of 8-heteroatom-substituted 4,4-difluoro-4-bora-3a,4a-diaza-s-indacene dyes (BODIPY). Tetrahedron 62, 5084–5091. doi:10.1016/j.tet.2006.03.036
Grando, S. R., Pessoa, C. M., Gallas, M. R., Costa, T. M. H., Rodembusch, F. S., and Benvenutti, E. V. (2009). Modulation of the ESIPT emission of benzothiazole type dye incorporated in silica-based hybrid materials. Langmuir 25, 13219–13223. doi:10.1021/la902242y
Grätzel, M. (2001). Photoelectrochemical cells. Photoelectrochem. cells 414, 338–344. doi:10.1038/35104607
Gregory, P. (2012). High-technology applications of organic colorants. New York: Springer Science & Business Media. doi:10.1007/978-1-4615-3822-6
Grimmett, M. R., Katritzky, A. R., and Rees, C. W. (1984). Comprehensive heterocyclic chemistry, 5. Oxford: Pergamon Press.
Groebke, K., Weber, L., and Mehlin, F. (1998). Synthesis of imidazo[1,2-a] annulated pyridines, pyrazines and pyrimidines by a novel three-component condensation. Synlett 1998, 661–663. doi:10.1055/s-1998-1721
Gudmundsson, O. S., Jois, S. D. S., Velde, D. G. V., Siahaan, T. J., Borchardt, R. T., and Wang, B. (1999). The effect of conformation on the membrane permeation of coumarinic acid- and phenylpropionic acid-based cyclic prodrugs of opioid peptides. Pept. Res. 53, 383–392. doi:10.1034/j.1399-3011.1999.00076.x
Gueiffier, A., Mavel, S., Lhassani, M., Elhakmaoui, A., Snoeck, R., Andrei, G., et al. (1998). Synthesis of imidazo[1,2-a]pyridines as antiviral agents. J. Med. Chem. 41, 5108–5112. doi:10.1021/jm981051y
Gündüzalp, A. B., Özsen, İ., Alyar, H., Alyar, S., and Özbek, N. (2016). Biologically active Schiff bases containing thiophene/furan ring and their copper(II) complexes: Synthesis, spectral, nonlinear optical and density functional studies. J. Mol. Struct. 1120, 259–266. doi:10.1016/j.molstruc.2016.05.002
Gürses, A., Açıkyıldız, M., Güneş, K., and Gürses, M. S. (2016). Dyes pigments. Cham: Springer International Publishing, 31–45. doi:10.1007/978-3-319-33892-7_3
Halasi, M., Zhao, H., Dahari, H., Bhat, U. G., Gonzalez, E. B., Lyubimo, A. V., et al. (2010). Thiazole antibiotics against breast cancer. Cell. Cycle 9, 1214–1217. doi:10.4161/cc.9.6.10955
Hamer, F. M. (1964). The chemistry of heterocyclic compounds, the cyanine dyes and related compounds. New York: Interscience Publishers. doi:10.1038/204213a0
Han, T., Feng, X., Tong, B., Shi, J., Chen, L., Zhi, J., et al. (2012). A novel “turn-on” fluorescent chemosensor for the selective detection of Al3+ based on aggregation-induced emission. Chem. Commun. 48, 416–418. doi:10.1039/C1CC15681K
Hantzsch, A. (1881). Condensationsprodukte aus Aldehydammoniak und ketonartigen Verbindungen. Ber. Dtsch. Chem. Ges. 14, 1637–1638. doi:10.1002/cber.18810140214
Hara, K., Sato, T., Katoh, R., Furube, A., Ohga, Y., Shinpo, A., et al. (2003). Molecular design of coumarin dyes for efficient dye-sensitized solar cells. J. Phys. Chem. B 107, 597–606. doi:10.1021/jp026963x
Harichandran, G., Parameswari, P., and Shanmugam, P. (2017). An efficient solvent free Amberlite IRA-400 Cl resin mediated multicomponent synthesis and photophysical properties of fluorescent 4H-chromene derivatives. Dyes Pigments 139, 541–548. doi:10.1016/j.dyepig.2016.12.026
Harichandran, G., Parameswari, P., and Shanmugam, P. (2018). Synthesis and photophysical properties of functionalized fluorescent 4H-chromenes and benzo a chromenophenazines as Fe3+ and Cu2+ ion sensor. Sens. Actuators B Chem. 272, 252–263. doi:10.1016/j.snb.2018.05.134
Hauck, M., Turdean, R., Memminger, K., Schönhaber, J., Rominger, F., and Müller, T. J. J. (2010). Luminescent, redox-active diphenothiazine dumbbells expanded by conjugated arenes and Heteroarenes. J. Org. Chem. 75, 8591–8603. doi:10.1021/jo101997t
Heller, A., and Williams, D. L. (1970). Intramolecular proton transfer reactions in excited fluorescent compounds. J. Phys. Chem. 74, 4473–4480. doi:10.1021/j100720a003
Henninot, A., Collins, J. C., and Nuss, J. M. (2018). Back future? Curr. State Pept. Drug Discov.J. Med. Chem. 61, 1382–1414. doi:10.1021/acs.jmedchem.7b00318
Hill, R. E., Himmeldirk, K., Kennedy, I. A., Pauloski, R. M., Sayer, B. G., Wolf, E., et al. (1996). The biogenetic anatomy of vitamin B6: A 13C NMR investigation of the biosynthesis of pyridoxol in Escherichia coli. J. Biol. Chem. 271, 30426–30435. doi:10.1074/jbc.271.48.30426
Hirano, T., Kikuchi, K., Urano, Y., Higuchi, T., and Nagano, T. (2000). Highly zinc-selective fluorescent sensor molecules suitable for biological applications. J. Am. Chem. Soc. 122, 12399–12400. doi:10.1021/ja002467f
Hong, G., Antaris, A. L., and Dai, H. (2017). Near-infrared fluorophores for biomedical imaging. Nat. Biomed. Eng. 1, 0010. doi:10.1038/s41551-016-0010
Hong, Y., Lam, J. W., and Tang, B. Z. (2011). Aggregation-induced emission. Chem. Soc. Rev. 40, 5361–5388. doi:10.1039/C1CS15113D
Hong, Y., Lam, J. W., and Tang, B. Z. (2009). Aggregation-induced emission: Phenomenon, mechanism and applications. Chem. Commun., 43324353–4353. doi:10.1039/B904665H
Hoppe, H., and Sariciftci, N. S. (2004). Organic solar cells: An overview. J. Mat. Res. 19, 1924–1945. doi:10.1557/JMR.2004.0252
Horiguchi, H., Tsurugi, H., Satoh, T., and Miura, M. (2008). Palladium/phosphite or phosphate catalyzed oxidative coupling of arylboronic acids with alkynes to produce 1,4-Diaryl-1,3-butadienes. Adv. Synth. Catal. 350, 509–514. doi:10.1002/adsc.200700533
Hu, H., Lin, C., Ao, M., Ji, Y., Tang, B., Zhou, X., et al. (2017). Synthesis and biological evaluation of 1-(2-(adamantane-1-yl)-1 H-indol-5-yl)-3-substituted urea/thiourea derivatives as anticancer agents. RSC Adv. 7, 51640–51651. doi:10.1039/C7RA08149A
Hu, R., Leung, N. L. C., and Tang, B. Z. (2014). AIE macromolecules: Syntheses, structures and functionalities. Chem. Soc. Rev. 43, 4494–4562. doi:10.1039/c4cs00044g
Huang, J., Canadien, V., Lam, G. Y., Steinberg, B. E., Dinauer, M. C., Magalhaes, M. A. O., et al. (2009). Activation of antibacterial autophagy by NADPH oxidases. P. Natl. A. Sci. India 106, 6226–6231. doi:10.1073/pnas.0811045106
Hussein, W., and Turan, G. (2018). Synthesis of new thiazole and thiazolyl derivatives of medicinal significant-a short review. MOJ Biorg. Org. Chem. 2, 52–55. doi:10.15406/mojboc.2018.02.0056
Ibarra-Rodríguez, M., Muñoz-Flores, B. M., Gómez-Treviño, A., Chan-Navarro, R., Berrones-Reyes, J. C., Chávez-Reyes, A., et al. (2019). Organoboron Schiff bases as cell-staining fluorescent probes: Synthesis, Chemio-photophysical characterization, DFT, and X-ray structures. Appl. Organomet. Chem. 33, e4718. doi:10.1002/aoc.4718
Ibrar, A., Tehseen, Y., Khan, I., Hameed, A., Saeed, A., Furtmann, N., et al. (2016). Coumarin-thiazole and -oxadiazole derivatives: Synthesis, bioactivity and docking studies for aldose/aldehyde reductase inhibitors. Bioorg. Chem. 68, 177–186. doi:10.1016/j.bioorg.2016.08.005
Igarashi, Y., Ogura, H., Furihata, K., Oku, N., Indananda, C., and Thamchaipenet, A. (2011). Maklamicin, an antibacterial polyketide from an endophytic micromonospora sp. J. Nat. Prod. 74, 670–674. doi:10.1021/np100727h
Irfan, A., Al-Sehemi, A. G., Chaudhry, A. R., and Muhammad, S. (2016). A first-principles study of the linear and nonlinear optical properties of isoxazole derivatives. J. Theor. Comp. Chem. 15, 1650060. doi:10.1142/s0219633616500607
Isaka, M., Srisanoh, U., Veeranondha, S., Choowong, W., and Lumyong, S. (2009). Cytotoxic eremophilane sesquiterpenoids from the saprobic fungus Berkleasmium nigroapicale BCC 8220. Tetrahedron 65, 8808–8815. doi:10.1016/j.tet.2009.08.077
Issaeva, N., Bozko, P., Enge, M., Protopopova, M., Verhoef, L. G. G. C., Masucci, M., et al. (2004). Small molecule RITA binds to p53, blocks p53–HDM-2 interaction and activates p53 function in tumors. Nat. Med. 10, 1321–1328. doi:10.1038/nm1146
Iyer, R. N., and Gopalachari, R. (1973). Studies in potential antifertility agents: VII. Synthesis of dialkylaminoethyl derivatives of 2,4,5-triphenylpyrroles & 2,4,5-triphenylfurans. Indian J. Chem. 11 (12), 1260–1262.
Jacques, E., Romain, M., Yassin, A., Bebiche, S., Harnois, M., Mohammed-Brahim, T., et al. (2014). An electron deficient dicyanovinylene-ladder-type pentaphenylene derivative for n-type organic field effect transistors. J. Mat. Chem. C 2, 3292–3302. doi:10.1039/C3TC31925C
Jahnke, A. C., Spulber, M., Neuburger, M., Palivan, C. G., and Wenger, O. S. (2014). Electronic coupling mediated by furan, thiophene, selenophene and tellurophene in a homologous series of organic mixed valence compounds. Chem. Commun. 50, 10883–10886. doi:10.1039/C4CC03806A
Jäkle, F. (2010). Advances in the synthesis of Organoborane polymers for optical, electronic, and sensory applications. Chem. Rev. 110, 3985–4022. doi:10.1021/cr100026f
Jamwal, A., Javed, A., and Bhardwaj, V. (2013). A review on pyrazole derivatives of pharmacological potential. Pharm. Bio. Sci. 3, 114–123.
Janiga, A., Glodkowska-Mrowka, E., Stoklosa, T., and Gryko, D. T. (2013). Synthesis and optical properties of tetraaryl-1,4-dihydropyrrolo[3,2-b]pyrroles. Asian J. Org. Chem. 2, 411–415. doi:10.1002/ajoc.201200201
Jennings, J. J., Bhatt, C. P., and Franz, A. K. (2016). Lanthanum(III)-Catalyzed three-component reaction of coumarin-3-carboxylates for the synthesis of indolylmalonamides and analysis of their photophysical properties. J. Org. Chem. 81, 6211–6222. doi:10.1021/acs.joc.6b00541
Jiang, P., and Guo, Z. (2004). Fluorescent detection of zinc in biological systems: Recent development on the design of chemosensors and biosensors. Coord. Chem. Rev. 248, 205–229. doi:10.1016/j.cct.2003.10.013
Jimenez, A. J., Fagnoni, M., Mella, M., and Albini, A. (2009). Photoinduced electron and energy transfer in aryldihydropyridines. J. Org. Chem. 74, 6615–6622. doi:10.1021/jo9010816
Jiménez-Pérez, V. M., García-López, M. C., Munoz-Flores, B. M., Chan-Navarro, R., Berrones-Reyes, J. C., Dias, H. R., et al. (2015). New application of fluorescent organotin compounds derived from schiff bases: Synthesis, X-ray structures, photophysical properties, cytotoxicity and fluorescent bioimaging. J. Mat. Chem. B 3, 5731–5745. doi:10.1039/c5tb00717h
Jiménez-Pérez, V. M., García-López, M. C., Munoz-Flores, B. M., Chan-Navarro, R., Berrones-Reyes, J. C., Dias, H. V. R., et al. (2015). New application of fluorescent organotin compounds derived from schiff bases: Synthesis, X-ray structures, photophysical properties, cytotoxicity and fluorescent bioimaging. J. Mat. Chem. B 3, 5731–5745. doi:10.1039/C5TB00717H
Jo, T. G., Lee, J. J., Nam, E., Bok, K. H., Lim, M. H., and Kim, C. (2016). A highly selective fluorescent sensor for the detection of Al 3+ and CN− in aqueous solution: Biological applications and DFT calculations. New J. Chem. 40, 8918–8927. doi:10.1039/C6NJ01544A
Jonas, R., Klockow, M., Lues, I., Prücher, H., Schliep, H. J., and Wurziger, H. (1993). Synthesis and biological activities of meribendan and related heterocyclic benzimidazolo-pyridazinones. Eur. J. Med. Chem. 28, 129–140. doi:10.1016/0223-5234(93)90005-Y
Jones, C. D., Winter, M. A., Hirsch, K. S., Stamm, N., Taylor, H. M., Holden, H. E., et al. (1990). Estrogen synthetase inhibitors. 2. Comparison of the in vitro aromatase inhibitory activity for a variety of nitrogen heterocycles substituted with diarylmethane or diarylmethanol groups. J. Med. Chem. 33, 416–429. doi:10.1021/jm00163a065
Jones, R. A., and Bean, G. P. (2013). The chemistry of pyrroles: Organic chemistry: A series of monographs, 34. New York: Academic Press.
Joshi, G., Nayyar, H., Alex, J. M., Vishwakarma, G. S., Mittal, S., and Kumar, R. (2016). Pyrimidine-fused derivatives: Synthetic strategies and medicinal attributes. Curr. Top. Med. Chem. 16, 3175–3210. doi:10.2174/1568026616666160506145046
Joshi, S., Bisht, A. S., and Juyal, D. (2017). Systematic scientific study of 1, 3-oxazole derivatives as a useful lead for pharmaceuticals: A review. Pharma Innov. 6, 109.
Jung, H. S., Ko, K. C., Kim, G.-H., Lee, A.-R., Na, Y.-C., Kang, C., et al. (2011). Coumarin-based thiol chemosensor: Synthesis, turn-on mechanism, and its biological application. Org. Lett. 13, 1498–1501. doi:10.1021/ol2001864
Kaim, L. E., Grimaud, L., and Schiltz, A. (2009). One-pot synthesis of oxazoles using isocyanide surrogates. Tetrahedron Lett. 50, 5235–5237. doi:10.1016/j.tetlet.2009.07.001
Kaiser, D. G., and Glenn, E. M. (1972). Correlation of plasma 4,5-bis (p-methoxyphenyl)-2-phenylpyrrole-3- acetonitrile levels with biological activity. J. Pharm. Sci. 61, 1908–1911. doi:10.1002/jps.2600611205
Kalluraya, B., Isloor, A. M., and Shenoy, S. (2001). Synthesis and biological activity of 6-substituted-3-[4-(3-substituted pyrazolidene) hydrazino-4-thiazolyl] coumarins. Indian J. heterocy. Chem. 11, 159–162.
Kalyani, N. T., and Dhoble, S. (2012). Organic light emitting diodes: Energy saving lighting technology—A review. Renew. Sust. Energy Rev. 16, 2696–2723. doi:10.1016/j.rser.2012.02.021
Kamada, K., Ueda, M., Nagao, H., Tawa, K., Sugino, T., Shmizu, Y., et al. (2000). Molecular design for organic nonlinear Optics: Polarizability and Hyperpolarizabilities of furan Homologues investigated by ab initio molecular orbital method. J. Phys. Chem. A 104, 4723–4734. doi:10.1021/jp993806y
Kang, K., Park, S., Kim, Y. S., Lee, S., and Back, K. (2009). Biosynthesis and biotechnological production of serotonin derivatives. Appl. Microbiol. Biot. 83, 27–34. doi:10.1007/s00253-009-1956-1
Karrouchi, K., Radi, S., Ramli, Y., Taoufik, J., Mabkhot, Y. N., Al-aizari, F. A., et al. (2018). Synthesis and pharmacological activities of pyrazole derivatives: A review. Molecules 23, 134. doi:10.3390/molecules23010134
Kasha, M. (1986). Proton-transfer spectroscopy. Perturbation of the tautomerization potential. J. Chem. Soc. B 82, 2379–2392. doi:10.1039/F29868202379
Kashyap, S. J., Garg, V. K., Sharma, P. K., Kumar, N., Dudhe, R., and Gupta, J. K. (2012). Thiazoles: Having diverse biological activities. Med. Chem. Res. 21, 2123–2132. doi:10.1007/s00044-011-9685-2
Kaur Bhatia, R. (2017). Isoindole derivatives: Propitious anticancer structural motifs. Curr. Top. Med. Chem. 17, 189–207. doi:10.2174/1568026616666160530154100
Kaur, M., Singh, M., Chadha, N., and Silakari, O. (2016). Oxindole: A chemical prism carrying plethora of therapeutic benefits. Eur. J. Med. Chem. 123, 858–894. doi:10.1016/j.ejmech.2016.08.011
Kaushik, N. K., Kaushik, N., Attri, P., Kumar, N., Kim, C. H., Verma, A. K., et al. (2013). Biomedical importance of indoles. Molecules 186620, 6620–6662. doi:10.3390/molecules18066620
Keay, B. A., and Dibble, P. W. (1996). Furans and their benzo derivatives: Applications. CHEC-II 2, 395–436.
Kercher, T., Rao, C., Bencsik, J. R., and Josey, J. A. (2007). Diversification of the three-component coupling of 2-Aminoheterocycles, aldehydes, and isonitriles: Efficient Parallel synthesis of a diverse and Druglike library of imidazo- and Tetrahydroimidazo[1,2-a] heterocycles. J. Comb. Chem. 9, 1177–1187. doi:10.1021/cc0700290
Kerru, N., Gummidi, L., Maddila, S., and Jonnalagadda, S. B. (2021). A review of recent advances in the green synthesis of azole-and pyran-based fused heterocycles using MCRs and sustainable catalysts. Curr. Org. Chem. 25, 4–39. doi:10.2174/1385272824999201020204620
Khabnadideh, S., Rezaei, Z., Khalafi-Nezhad, A., Bahrinajafi, R., Mohamadi, R., and Farrokhroz, A. A. (2003). Synthesis of N-Alkylated derivatives of imidazole as antibacterial agents. Bioorgan. Med. Chem. Lett. 13, 2863–2865. doi:10.1016/S0960-894X(03)00591-2
Khajuria, R., Dham, S., and Kapoor, K. K. (2016). Active methylenes in the synthesis of a pyrrole motif: An imperative structural unit of pharmaceuticals, natural products and optoelectronic materials. RSC Adv. 6, 37039–37066. doi:10.1039/C6RA03411J
Khalafi-Nezhad, A., Soltani Rad, M. N., Mohabatkar, H., Asrari, Z., and Hemmateenejad, B. (2005). Design, synthesis, antibacterial and QSAR studies of benzimidazole and imidazole chloroaryloxyalkyl derivatives. Bioorgan. Med. Chem. 13, 1931–1938. doi:10.1016/j.bmc.2005.01.014
Khan, A. T., Basha, R. S., and Lal, M. (2012). Bromodimethylsulfonium bromide (BDMS) catalyzed synthesis of imidazo 1,2-a pyridine derivatives and their fluorescence properties. Tetrahedron Lett. 53, 2211–2217. doi:10.1016/j.tetlet.2012.02.078
Khan, M. N., Pal, S., Karamthulla, S., and Choudhury, L. H. (2014). Multicomponent reactions for facile access to coumarin-fused dihydroquinolines and quinolines: Synthesis and photophysical studies. New J. Chem. 38, 4722–4729. doi:10.1039/c4nj00630e
Khan, M. N., Pal, S., Parvin, T., and Choudhury, L. H. (2012). A simple and efficient method for the facile access of highly functionalized pyridines and their fluorescence property studies. RSC Adv. 2, 12305–12314. doi:10.1039/c2ra21385k
Khan, S. A., and Asiri, A. M. (2015). Physicochemical and Critical Micelle concentration (CMC) of cationic (CATB) and anionic (SDS) surfactants with environmentally benign blue emitting TTQC dye. J. Fluoresc. 25, 1595–1599. doi:10.1007/s10895-015-1621-2
KhanYusufzai, S., Osman, H., Khan, M. S., Mohamad, S., Sulaiman, O., Parumasivam, T., et al. (2017). Design, characterization, in vitro antibacterial, antitubercular evaluation and structure–activity relationships of new hydrazinyl thiazolyl coumarin derivatives. Med. Chem. Res. 22, 1139–1148. doi:10.1007/s00044-017-1820-2
Khurana, J. M., Chaudhary, A., Lumb, A., and Nand, B. (2012). Efficient one-pot syntheses of dibenzo a,i xanthene-diones and evaluation of their antioxidant activity. Can. J. Chem. 90, 739–746. doi:10.1139/v2012-033
Kidwai, M., Saxena, S., Rahman Khan, M. K., and Thukral, S. S. (2005). Aqua mediated synthesis of substituted 2-amino-4H-chromenes and in vitro study as antibacterial agents. Bioorgan. Med. Chem. Lett. 15, 4295–4298. doi:10.1016/j.bmcl.2005.06.041
Kim, E., and Park, S. B. (2009). Chemistry as a prism: A review of light-emitting materials having tunable emission wavelengths. Chem. Asian J. 4, 1646–1658. doi:10.1002/asia.200900102
Kim, H. N., Swamy, K. M. K., and Yoon, J. (2011). Study on various fluorescein derivatives as pH sensors. Tetrahedron Lett. 52, 2340–2343. doi:10.1016/j.tetlet.2011.02.076
Kitani, S., Miyamoto, K. T., Takamatsu, S., Herawati, E., Iguchi, H., Nishitomi, K., et al. (2011). Avenolide, a Streptomyces hormone controlling antibiotic production in Streptomyces avermitilis. P. Natl. A. Sci. India 108, 16410–16415. doi:10.1073/pnas.1113908108
Kitson, R. R. A., Millemaggi, A., and Taylor, R. J. K. (2009). The Renaissance of α-Methylene-γ-butyrolactones: New synthetic approaches. Angew. Chem. Int. Ed. 48, 9426–9451. doi:10.1002/anie.200903108
Klein, C. L., Conrad, J. M., and Morris, S. A. (1985). Structure of N-phenylphenothiazine, C18H13NS. Acta Crystallogr. C 41, 1202–1204. doi:10.1107/s0108270185007144
Kloeters, L. N., May, L., Tengen, B., May, L., and Müller, T. J. J. (2022). Fluorescent phenothiazine-triazine donor-acceptor conjugates by facile consecutive nucleophilic displacement – color tuning by substitution and protochromicity. Dyes Pigments 206, 110564. doi:10.1016/j.dyepig.2022.110564
Klukas, F., Grunwald, A., Menschel, F., and Müller, T. J. J. (2014). Rapid pseudo five-component synthesis of intensively blue luminescent 2,5-di(hetero)arylfurans via a Sonogashira-Glaser cyclization sequence. Beilstein J. Org. Chem. 10, 672–679. doi:10.3762/bjoc.10.60
Knight, C. G., and Stephens, T. (1989). Xanthene-dye-labelled phosphatidylethanolamines as probes of interfacial pH. Studies in phospholipid vesicles. Biochem. J. 258, 683–687. doi:10.1042/bj2580683
Kochanowska-Karamyan, A. J., and Hamann, M. T. (2010). Marine indole alkaloids: Potential new drug leads for the Control of Depression and Anxiety. Chem. Rev. 110, 4489–4497. doi:10.1021/cr900211p
Kolemen, S., and Akkaya, E. U. (2018). Reaction-based BODIPY probes for selective bio-imaging. Coord. Chem. Rev. 354, 121–134. doi:10.1016/j.ccr.2017.06.021
Koner, R. R., Sinha, S., Kumar, S., Nandi, C. K., and Ghosh, S. (2012). 2-Aminopyridine derivative as fluorescence 'On-Off' molecular switch for selective detection of Fe3+/Hg2+. Tetrahedron Lett. 53, 2302–2307. doi:10.1016/j.tetlet.2012.02.094
Korade, S. N., Mhaldar, P. M., Kulkarni, P. P., Rashinkar, G. S., and Pore, D. M. (2021). Meglumine catalyzed one pot synthesis of new fluorescent 2-amino-4-pyrazolyl-6-aryldiazenyl-4H-chromene-3-carbonitriles. Synth. Commun. 51, 2336–2348. doi:10.1080/00397911.2021.1934875
Kozhevnikov, V. N., Kozhevnikov, D. N., Nikitina, T. V., Rusinov, V. L., Chupakhin, O. N., Zabel, M., et al. (2003). A Versatile strategy for the synthesis of functionalized 2,2‘-Bi- and 2,2‘:6‘,2‘ ‘-Terpyridines via their 1,2,4-triazine analogues. J. Org. Chem. 68, 2882–2888. doi:10.1021/jo0267955
Kraft, A., Grimsdale, A. C., and Holmes, A. B. (1998). Electroluminescent conjugated polymers—seeing polymers in a new light. Angew. Chem. Int. Ed. 37, 402–428. doi:10.1002/(SICI1521-3773(19980302)37:4<402:AID-ANIE402>3.0).CO;2–9
Kronenberg, N. M., Deppisch, M., Würthner, F., Lademann, H. W. A., Deing, K., and Meerholz, K. (2008). Bulk heterojunction organic solar cells based on merocyanine colorants. Chem. Commun., 64896491–6491. doi:10.1039/B813341G
Kulhánek, J., Bureš, F., Pytela, O., Mikysek, T., and Ludvík, J. (2011). Imidazole as a donor/acceptor Unit in charge-transfer chromophores with extended π-linkers. Chem. Asian J. 6, 1604–1612. doi:10.1002/asia.201100097
Kulinich, A. V., and Ishchenko, A. A. (2009). Merocyanine dyes: Synthesis, structure, properties and applications. Russ. Chem. Rev. 78, 141–164. doi:10.1070/RC2009v078n02ABEH003900
Kulinich, A. V., and Ishchenko, A. A. (2009). Merocyanine dyes: Synthesis, structure, properties and applications. Russ. Chem. Rev. 78, 141–164. doi:10.1070/RC2009v078n02ABEH003900
Kulkarni, A. P., Tonzola, C. J., Babel, A., and Jenekhe, S. A. (2004). Electron transport materials for organic light-emitting diodes. Chem. Mat. 16, 4556–4573. doi:10.1021/cm049473l
Kulkarni, A. P., Wu, P.-T., Kwon, T. W., and Jenekhe, S. A. (2005). Phenothiazine-phenylquinoline Donor−Acceptor molecules: Effects of structural isomerism on charge transfer Photophysics and electroluminescence. J. Phys. Chem. B 109, 19584–19594. doi:10.1021/jp0529772
Kumar, D., and Kumar Jain, S. (2016). A comprehensive review of N-heterocycles as cytotoxic agents. Curr. Med. Chem. 23, 4338–4394. doi:10.2174/0929867323666160809093930
Kumar, M., Kumawat, L. K., Bhatt, P., Jha, A., Agarwal, S., Sharma, A., et al. (2017). Optical and electrochemical dual channel sensing of Cu2+ using functionalized furo 2,3-d pyrimidines-2,4 1H,3H -diones. Spectrochim. Acta A. 181, 73–81. doi:10.1016/j.saa.2017.03.034
Kumbhare, R. M., Kosurkar, U. B., Janaki Ramaiah, M., Dadmal, T. L., Pushpavalli, S. N. C. V. L., and Pal-Bhadra, M. (2012). Synthesis and biological evaluation of novel triazoles and isoxazoles linked 2-phenyl benzothiazole as potential anticancer agents. Bioorgan. Med. Chem. Lett. 22, 5424–5427. doi:10.1016/j.bmcl.2012.07.041
Kundu, A., Pathak, S., and Pramanik, A. (2013). Synthesis and fluorescence properties of isatin-based spiro compounds: Switch off chemosensing of copper(II) ions. Asian J. Org. Chem. 2, 869–876. doi:10.1002/ajoc.201300153
Kwan, D. H., Chen, H.-M., Ratananikom, K., Hancock, S. M., Watanabe, Y., Kongsaeree, P. T., et al. (2011). Self-immobilizing Fluorogenic imaging agents of enzyme activity. Angew. Chem. Int. Ed. 50, 300–303. doi:10.1002/anie.201005705
Kymissis, I. (2008). Organic field effect transistors: Theory, fabrication and characterization. Springer Science & Business Media. doi:10.1007/978-0-387-92134-1
Lagoja, I. M. (2005). Pyrimidine as constituent of natural biologically active compounds. Chem. Biodivers. 2, 1–50. doi:10.1002/cbdv.200490173
Lai, R. Y., Fabrizio, E. F., Lu, L., Jenekhe, S. A., Bard, A. J., and Synthesis, Synthesis (2001). Synthesis, cyclic voltammetric studies, and electrogenerated chemiluminescence of a new DonorAcceptor Molecule: 3,7-[Bis[4-phenyl-2-quinolyl]]-10-methylphenothiazine. J. Am. Chem. Soc. 123, 9112–9118. doi:10.1021/ja0102235
Lamère, J. F., Lacroix, P. G., Farfán, N., Rivera, J. M., Santillan, R., and Nakatani, K. (2006). Synthesis, characterization and nonlinear optical (NLO) properties of a push–pull bisboronate chromophore with a potential electric field induced NLO switch. J. Mat. Chem. 16, 2913–2920. doi:10.1039/B606359D
Lara-Ceron, J. A., Jimenez-Perez, V. M., Molina-Paredes, A. A., Dias, H. V. R., Chavez-Reyes, A., Paudel, H. R., et al. (2017). Luminescent silk fibroin with organotin compounds from amino acid schiff bases - microwave-assisted synthesis, Chemo-optical characterization, cytotoxicity, and Confocal microscopy. Eur. J. Inorg. Chem. 2017, 2818–2827. doi:10.1002/ejic.201700187
Laursen, J. B., and Nielsen, J. (2004). Phenazine natural products: Biosynthesis, synthetic analogues, and biological activity. Chem. Rev. 104, 1663–1686. doi:10.1021/cr020473j
Le, H. T. M., El-Hamdi, N. S., and Miljanić, O. Š. (2015). Benzobisimidazole cruciform fluorophores. J. Org. Chem. 80, 5210–5217. doi:10.1021/acs.joc.5b00616
Lee, A. C.-L., Harris, J. L., Khanna, K. K., and Hong, J.-H. (2019). A comprehensive review on current advances in peptide drug development and design. Int. J. Mol. Sci. 20, 2383. doi:10.3390/ijms20102383
Lenze, M. R., Kronenberg, N. M., Würthner, F., and Meerholz, K. (2015). In-situ modification of PEDOT:PSS work function using alkyl alcohols as secondary processing solvents and their impact on merocyanine based bulk heterojunction solar cells. Org. Electron. 21, 171–176. doi:10.1016/j.orgel.2015.03.008
Levi, L., and Müller, T. J. J. (2016). Multicomponent syntheses of fluorophores initiated by metal catalysis. Eur. J. Org. Chem. 2016, 2902–2918. doi:10.1002/ejoc.201600409
Levi, L., and Müller, T. J. J. (2016). Multicomponent syntheses of functional chromophores. Chem. Soc. Rev. 45, 2825–2846. doi:10.1039/c5cs00805k
Li, Z. R. (2015). Organic light-emitting materials and devices. Boca Raton, USA: CRC Press. doi:10.1201/b18540
Lin, N.-H., Wang, L., Cohen, J., Gu, W.-Z., Frost, D., Zhang, H., et al. (2003). Synthesis and biological evaluation of 4-[3-biphenyl-2-yl-1-hydroxy-1-(3-methyl-3H-imidazol-4-yl)-prop-2-ynyl]-1-yl-benzonitrile as novel farnesyltransferase inhibitor. Bioorgan. Med. Chem. Lett. 13, 1293–1296. doi:10.1016/S0960-894X(03)00122-7
Lin, Y., Fan, H., Li, Y., and Zhan, X. (2012). Thiazole-based organic semiconductors for organic electronics. Adv. Mat. 24, 3087–3106. doi:10.1002/adma.201200721
Lin, Y., Li, Y., and Zhan, X. (2012). Small molecule semiconductors for high-efficiency organic photovoltaics. Chem. Soc. Rev. 41, 4245–4272. doi:10.1039/C2CS15313K
Lindquist, N., Fenical, W., Van Duyne, G. D., and Clardy, J. (1991). Isolation and structure determination of diazonamides A and B, unusual cytotoxic metabolites from the marine ascidian Diazona chinensis. J. Am. Chem. Soc. 113, 2303–2304. doi:10.1021/ja00006a060
Liu, C.-Y., and Luh, T.-Y. (2002). Combining furan annulation, Heck reaction, and Sonogashira coupling for the synthesis of oligoaryls. Org. Lett. 4, 4305–4307. doi:10.1021/ol026941t
Liu, J.-Q., Zhang, M., Zhang, C.-F., Qi, H.-Y., Bashall, A., Bligh, S. W. A., et al. (2008). Cytotoxic sesquiterpenes from Ligularia platyglossa. Phytochemistry 69, 2231–2236. doi:10.1016/j.phytochem.2008.05.018
Liu, X., Chen, B., Li, X., Zhang, L., Xu, Y., Liu, Z., et al. (2015). Self-assembly of BODIPY based pH-sensitive near-infrared polymeric micelles for drug controlled delivery and fluorescence imaging applications. Nanoscale 7, 16399–16416. doi:10.1039/C5NR04655F
Loudet, A., and Burgess, K. (2007). BODIPY dyes and their derivatives: Syntheses and spectroscopic properties. Chem. Rev. 107, 4891–4932. doi:10.1021/cr078381n
Loving, G., and Imperiali, B. (2009). Thiol-reactive derivatives of the solvatochromic 4-N,N-Dimethylamino-1,8-naphthalimide fluorophore: A highly sensitive toolset for the detection of biomolecular interactions. Bioconjugate Chem. 20, 2133–2141. doi:10.1021/bc900319z
Lu, W., Zhang, L.-H., Ye, X.-S., Su, J., and Yu, Z. (2006). Molecular receptors for monosaccharides: di(pyridyl)naphthyridine and di(quinolyl)naphthyridine. Tetrahedron 62, 1806–1816. doi:10.1016/j.tet.2005.11.048
Luca, L. D. (2006). Naturally occurring and synthetic imidazoles: Their chemistry and their biological activities. Curr. Med. Chem. 13, 1–23. doi:10.2174/092986706775197971
Ma, D.-L., Chan, D. S.-H., and Leung, C.-H. (2014). Group 9 Organometallic compounds for therapeutic and bioanalytical applications. Accounts Chem. Res. 47, 3614–3631. doi:10.1021/ar500310z
Ma, S., Zhang, J., Liu, Y., Qian, J., Xu, B., and Tian, W. (2017). Direct Observation of the symmetrical and asymmetrical protonation states in molecular crystals. J. Phys. Chem. Lett. 8, 3068–3072. doi:10.1021/acs.jpclett.7b01454
Machado, V. G., Stock, R. I., and Reichardt, C. (2014). Pyridinium N-phenolate betaine dyes. Chem. Rev. 114, 10429–10475. doi:10.1021/cr5001157
Maderna, A., Doroski, M., Subramanyam, C., Porte, A., Leverett, C. A., Vetelino, B. C., et al. (2014). Discovery of cytotoxic dolastatin 10 analogues with N-terminal modifications. J. Med. Chem. 57, 10527–10543. doi:10.1021/jm501649k
Mahmoodi, N. O., and Ghanbari Pirbasti, F. (2016). Overview on the recently developed thiazolyl heterocycles as useful therapeutic agents. Elem. 191, 811–843. doi:10.1080/10426507.2015.1119143
Mal, K., Naskar, B., Mondal, A., Goswami, S., Prodhan, C., Chaudhuri, K., et al. (2018). Dihydroindeno 1,2-b pyrroles: New Al3+ selective off-on chemosensors for bio-imaging in living HepG2 cells. Org. Biomol. Chem. 16, 5920–5931. doi:10.1039/c8ob01411f
Mangal, M., and Jangid, V. (2016). Synthesis and antimicrobial studies of indolyl pyrimidines. Heterocycl. Lett. 6, 795–803.
Manjunatha, B., Bodke, Y. D., Mounesh, , Nagaraja, O., and Navaneethgowda, P. V. (2022). Coumarin-pyridone conjugate as a fluorescent tag for LFPs visualization and electrochemical sensor for nitrite detection. New J. Chem. 46, 5393–5404. doi:10.1039/d1nj04751e
Marcinkowska, M., Kołaczkowski, M., Kamiński, K., Bucki, A., Pawłowski, M., Siwek, A., et al. (2016). Design, synthesis, and biological evaluation of fluorinated imidazo [1, 2-a] pyridine derivatives with potential antipsychotic activity. Eur. J. Med. Chem. 124, 456–467. doi:10.1016/j.ejmech.2016.08.059
Martin, M. M., and Lindqvist, L. (1975). The pH dependence of fluorescein fluorescence. J. Lumin. 10, 381–390. doi:10.1016/0022-2313(75)90003-4
Martins, L. M., Vieira, S. d. F., Baldacim, G. B., Bregadiolli, B. A., Caraschi, J. C., Batagin-Neto, A., et al. (2018). Improved synthesis of tetraaryl-1,4-dihydropyrrolo[3,2-b]pyrroles a promising dye for organic electronic devices: An experimental and theoretical approach. Dyes Pigments 148, 81–90. doi:10.1016/j.dyepig.2017.08.056
Massarano, T., Mazir, A., Lavi, R., and Byk, G. (2020). Solid-phase multicomponent synthesis of 3-substituted isoindolinones generates new cell-penetrating probes as drug carriers. ChemMedChem 15, 833–838. doi:10.1002/cmdc.201900656
Mataga, N., and Tsuno, S. (1957). Hydrogen bonding effect on the fluorescence of some nitrogen heterocycles. IHydrogen bonding effect on the fluorescence of some nitrogen heterocycles. I 30, 368–374. doi:10.1246/bcsj.30.368
Matsuzaki, K., Yoneyama, S., Fujii, N., Miyajima, K., Yamada, K.-i., Kirino, Y., et al. (1997). Membrane Permeabilization mechanisms of a cyclic antimicrobial peptide, Tachyplesin I, and its linear analog. Biochemistry-US 36, 9799–9806. doi:10.1021/bi970588v
May, L., and Müller, T. J. J. (2020). Widely electronically tunable 2,6-disubstituted dithieno 1,4 thiazines-electron-rich fluorophores up to intense NIR emission. Chem. Eur. J. 26, 12978–12986. doi:10.1002/chem.202000859
Mayer, L., Kohlbecher, R., and Müller, T. J. J. (2020). Concatenating Suzuki arylation and Buchwald-Hartwig amination by A sequentially Pd-catalyzed one-pot process-consecutive three-component synthesis of C,N-Diarylated heterocycles. Chem. Eur. J. 26, 15130–15134. doi:10.1002/chem.202003837
Mayer, L., and Müller, T. J. J. (2021). 3,10-Diaryl phenothiazines - one-pot synthesis and conformational tuning of ground and excited state electronics. Eur. J. Org. Chem. 2021, 3516–3527. doi:10.1002/ejoc.202100659
Mazumdar, P., Maity, S., Shyamal, M., Das, D., Sahoo, G. P., and Misra, A. (2016). Proton triggered emission and selective sensing of picric acid by the fluorescent aggregates of 6,7-dimethyl-2,3-bis-(2-pyridyl)-quinoxaline. Phys. Chem. Chem. Phys. 18, 7055–7067. doi:10.1039/c5cp05827a
Meerakrishna, R. S., Periyaraja, S., and Shanmugam, P. (2016). Copper-catalyzed multicomponent synthesis of fluorescent 2-phenyl-1 ' H-spiro fluorene-9,4-naphtho 2 ',3-h quinoline -7 ',12 '-dione derivatives. Eur. J. Org. Chem. 2016, 4516–4525. doi:10.1002/ejoc.201600647
Mei, J., Leung, N. L. C., Kwok, R. T. K., Lam, J. W. Y., and Tang, B. Z. (2015). Aggregation-induced emission: Together we shine, united we Soar!Aggregation-induced emission: Together we shine. Chem. Rev.united We soar! 115, 11718–11940. doi:10.1021/acs.chemrev.5b00263
Mekheimer, R. A., Abdelhameed, A. M., Mohamed, S. M., and Sadek, K. U. (2010). Green, three component highly efficient synthesis of 2-amino-5,6,7,8-tetrahydro-4-H-chromen-3-carbonitriles in water at ambient temperature. Green Chem. Lett. Rev. 3, 161–163. doi:10.1080/17518251003619176
Memminger, K., Oeser, T., and Müller, T. J. J. (2008). Phenothiazinophanes: Synthesis, structure, and intramolecular electronic communication. Org. Lett. 10, 2797–2800. doi:10.1021/ol800920d
Merkt, F. K., Hoewedes, S. P., Gers-Panther, C. F., Gruber, I., Janiak, C., and Müller, T. J. J. (2018). Three-component activation/alkynylation/cyclocondensation (AACC) synthesis of enhanced emission solvatochromic 3-Ethynylquinoxalines. Chem. Eur. J. 24, 8114–8125. doi:10.1002/chem.201800079
Merkt, F. K., and Müller, T. J. J. (2018). Solid state and aggregation induced emissive chromophores by multi-component syntheses. Isr. J. Chem. 58, 889–900. doi:10.1002/ijch.201800058
Merkul, E., and Müller, T. J. J. (2006). A new consecutive three-component oxazole synthesis by an amidation–coupling–cycloisomerization (ACCI) sequence. Chem. Commun., 48174819–4819. doi:10.1039/B610839C
Meshram, H. M., Prasad, B. R. V., and Aravind Kumar, D. (2010). A green approach for efficient synthesis of N-substituted pyrroles in ionic liquid under microwave irradiation. Tetrahedron Lett. 51, 3477–3480. doi:10.1016/j.tetlet.2010.03.036
Meyer, T., Krug, R., and Müller, T. J. J. (2021). Three-component Suzuki–Knoevenagel synthesis of merocyanine libraries and correlation analyses of their oxidation potentials and optical band gaps. Molecules 26, 5149. doi:10.3390/molecules26175149
Meyer, T., and Müller, T. J. J. (2020). Consecutive three-component synthesis of donor-substituted merocyanines by a one-pot Suzuki–Knoevenagel condensation sequence. Org. Mat. 02, 064–070. doi:10.1055/s-0040-1702148
Michaelis, A. (1894). Untersuchungen über aromatische Borverbindungen. Ber 27, 244–262. doi:10.1002/cber.18940270150
Mishra, A., Behera, R. K., Behera, P. K., Mishra, B. K., and Behera, G. B. (2000). Cyanines during the 1990s: A review. Chem. Rev. 100, 1973–2012. doi:10.1021/cr990402t
Mishra, A., Fischer, M. K. R., and Bäuerle, P. (2009). Metal-free organic dyes for dye-sensitized solar cells: From structure: Property relationships to design Rules. Angew. Chem. Int. Ed. 48, 2474–2499. doi:10.1002/anie.200804709
Mishra, S., Pandey, A., and Manvati, S. (2020). Coumarin: An emerging antiviral agent. Heliyon 6, e03217. doi:10.1016/j.heliyon.2020.e03217
Mitschke, U., and Bäuerle, P. (2000). The electroluminescence of organic materials. J. Mat. Chem. 10, 1471–1507. doi:10.1039/A908713C
Miura, T., Carmieli, R., and Wasielewski, M. R. (2010). Time-resolved EPR studies of charge Recombination and triplet-state formation within Donor−Bridge−Acceptor molecules having Wire-like Oligofluorene bridges. J. Phys. Chem. A 114, 5769–5778. doi:10.1021/jp101523n
Molina-Paredes, A. A., Jimenez-Perez, V. M., Lara-Ceron, J. A., Moggio, I., Arias, E., Santillan, R., et al. (2019). Fluorescent boron Schiff bases dyes for staining silk fibroin: Green synthesis, structural characterization, DFT, and photophysical properties. Appl. Organomet. Chem. 33, e4609. doi:10.1002/aoc.4609
Molnar, M., Lončarić, M., and Kovač, M. (2020). Green chemistry approaches to the synthesis of coumarin derivatives. Curr. Org. Chem. 24, 4–43. doi:10.2174/1385272824666200120144305
Mondal, A., Naskar, B., Goswami, S., Prodhan, C., Chaudhuri, K., and Mukhopadhyay, C. (2018). I-2 catalyzed access of spiro indoline-3,4 '-pyridine appended amine dyad: New ON-OFF chemosensors for Cu2+ and imaging in living cells. Org. Biomol. Chem. 16, 302–315. doi:10.1039/c7ob02651j
Montagner, C., Souza, S. M. d., Groposo, C., Monache, F. D., Smânia, E. F. A., and Jr, A. S. (2008). Antifungal activity of coumarins. Z. Naturforsch. C 63, 21–28. doi:10.1515/znc-2008-1-205
Mortensen, D. S., Rodriguez, A. L., Carlson, K. E., Sun, J., Katzenellenbogen, B. S., and Katzenellenbogen, J. A. (2001). Synthesis and biological evaluation of a novel series of furans: Ligands selective for Estrogen receptor α. J. Med. Chem. 44, 3838–3848. doi:10.1021/jm010211u
Müllen, K., and Scherf, U. (2006). Organic light emitting devices: Synthesis, properties and applications. John Wiley & Sons. doi:10.1002/3527607986
Müller, T. J. J. (2016). Aggregation-induced emission: Materials and applications, 1226. American Chemical Society, 85–112. doi:10.1021/bk-2016-1226.ch006
Müller, T. J. J., and D'Souza, D. M. (2008). Diversity-oriented syntheses of functional pi-systems by multicomponent and domino reactions. Pure Appl. Chem. 80, 609–620. doi:10.1351/pac200880030609
Müller, T. J. J. (2007). Functional organic materials syntheses, strategies and applications. Weinheim: Wiley VCH. doi:10.1002/9783527610266
Müller, T. J. J. (2014). Science of synthesis: Multicomponent reactions Vol. 1: General discussion and reactions involving a carbonyl compound as electrophilic component. Georg Thieme Verlag. doi:10.1055/b-003-125816
Muñoz, B. M., Santillan, R., Rodríguez, M., Méndez, J. M., Romero, M., Farfán, N., et al. (2008). Synthesis, crystal structure and non-linear optical properties of boronates derivatives of salicylideniminophenols. J. Organomet. Chem. 693, 1321–1334. doi:10.1016/j.jorganchem.2008.01.032
Murata, T., Morita, Y., Yakiyama, Y., Fukui, K., Yamochi, H., Saito, G., et al. (2007). Hydrogen-bond interaction in organic Conductors: Redox activation, molecular recognition, structural Regulation, and proton transfer in Donor−Acceptor charge-transfer complexes of TTF-imidazole. J. Am. Chem. Soc. 129, 10837–10846. doi:10.1021/ja072607m
Muschelknautz, C., Mayer, B., Rominger, F., and Müller, T. J. J. (2013). Consecutive three-component synthesis of film luminescent indolone merocyanines with L-amino acid ester donors. Chem. Heterocycl. Compd. 49, 860–871. doi:10.1007/s10593-013-1320-3
Muschelknautz, C., Visse, R., Nordmann, J., and Müller, T. J. J. (2014). One-pot three-component synthesis and photophysical characteristics of novel triene merocyanines. Beilstein J. Org. Chem. 10, 599–612. doi:10.3762/bjoc.10.51
Nagham Mahmood Aljamali, R. M. U. M. Dr. (2021). Spectral investigation and Microbial studying of pyridine-heterocyclic compounds, Synthesis. Eur. J. Mol. Clin. Med. 7, 4444–4453.
Nandi, L. G., Nicoleti, C. R., Bellettini, I. C., and Machado, V. G. (2014). Optical chemosensor for the detection of cyanide in water based on ethyl(hydroxyethyl)cellulose functionalized with Brooker’s merocyanine. Anal. Chem. 86, 4653–4656. doi:10.1021/ac501233x
Naskar, B., Das, K., Mondal, R. R., Maiti, D. K., Requena, A., Cerón-Carrasco, J. P., et al. (2018). A new fluorescence turn-on chemosensor for nanomolar detection of Al 3+ constructed from a pyridine–pyrazole system. New J. Chem. 42, 2933–2941. doi:10.1039/C7NJ03955G
Nazeri, M. T., Farhid, H., Javanbakht, S., Shaabani, A., and Notash, B. (2020). Highly efficient Chemoselective synthesis of pyrrolo 2,3-c pyrazole bearing oxindole via sequential condensation-Michael addition-intramolecular cyclization reactions. Synlett 31, 965–971. doi:10.1055/s-0039-1690887
Ngabaza, T., Johnson, M. M., Moeno, S., and Patel, M. (2017). Identification of 5,6,8-Trihydroxy-7-methoxy-2-(4-methoxyphenyl)-4H-chromen-4-one with antimicrobial activity from Dodonaea viscosa var. angustifolia. angustifolia 112, 48–53. doi:10.1016/j.sajb.2017.05.024
Nieves-Neira, W., Rivera, M. I., Kohlhagen, G., Hursey, M. L., Pourquier, P., Sausville, E. A., et al. (1999). DNA protein cross-Links produced by NSC 652287, a novel thiophene derivative active against human Renal cancer cells. Mol. Pharmacol. 56, 478–484. doi:10.1124/mol.56.3.478
Nikpassand, M., Fekri, L. Z., and Pourahmad, A. (2018). One-pot Synthesis of new azo-linked 4H-benzo[d] [1,3]oxazine-2,4-diones from carbon dioxide using CuO@RHA/MCM-41 nanocomposite in green media. J. CO2 Util. 27, 320–325. doi:10.1016/j.jcou.2018.08.011
Nikpassand, M. (2020). NiFe2O4@SiO2@glucose amine nanoparticle catalyzed reaction of azo-linked thiosalicylic acid with CO2: Access to azo-linked benzo[d]oxathiine-2,4-diones. Dyes Pigments 173, 107936. doi:10.1016/j.dyepig.2019.107936
Nikpassand, M., and Pirdelzendeh, D. (2016). Green synthesis of novel azo-linked 2-phenyl benzimidazoles using ionic liquid [BDBDMIm]Br. Dyes Pigments 130, 314–318. doi:10.1016/j.dyepig.2016.03.038
Nilsson, D., Kugler, T., Svensson, P.-O., and Berggren, M. (2002). An all-organic sensor–transistor based on a novel electrochemical transducer concept printed electrochemical sensors on paper. Sens. Actuators B Chem. 86, 193–197. doi:10.1016/S0925-4005(02)00170-3
Noctor, G., Queval, G., and Gakière, B. (2006). NAD(P) synthesis and pyridine nucleotide cycling in plants and their potential importance in stress conditions. J. Exp. Bot. 57, 1603–1620. doi:10.1093/jxb/erj202
Ochs, M., Mayer, B., and Müller, T. J. J. (2019). Unimolecular exciplexes by Ugi four-component reaction. Front. Chem. 7, 717. doi:10.3389/fchem.2019.00717
Okazaki, M., Takeda, Y., Data, P., Pander, P., Higginbotham, H., Monkman, A. P., et al. (2017). Thermally activated delayed fluorescent phenothiazine–dibenzo[a,j]phenazine–phenothiazine triads exhibiting tricolor-changing mechanochromic luminescence. Chem. Sci. 8, 2677–2686. doi:10.1039/C6SC04863C
Oliveira, G. H. C., Ramos, L. M., de Paiva, R. K. C., Passos, S. T. A., Simoes, M. M., Machado, F., et al. (2021). Synthetic enzyme-catalyzed multicomponent React. Isoxazol-5(4H)-one Syntheses, their Prop. Biol. Appl. why should one study mechanisms? Org. Biomol. Chem. 19, 1514–1531. doi:10.1039/d0ob02114h
Oskuie, E. F., Azizi, S., Ghasemi, Z., Pirouzmand, M., Kojanag, B. N., and Soleymani, J. (2020). Zn/MCM-41-catalyzed unsymmetrical Hantzsch reaction and the evaluation of optical properties and anti-cancer activities of the polyhydroquinoline products. Monatsh. Chem. 151, 243–249. doi:10.1007/s00706-020-02549-x
Osman, H., Arshad, A., Lam, C. K., and Bagley, M. C. (2012). Microwave-assisted synthesis and antioxidant properties of hydrazinyl thiazolyl coumarin derivatives. Chem. Cent. J. 6, 32. doi:10.1186/1752-153X-6-32
Pairas, G. N., Perperopoulou, F., Tsoungas, P. G., and Varvounis, G. (2017). The isoxazole ring and its N-oxide: A privileged core structure in neuropsychiatric therapeutics. ChemMedChem 12, 408–419. doi:10.1002/cmdc.201700023
Palmer, D. C., and Venkatraman, S. (2003). Oxazoles: Synthesis, reactions, and spectroscopy, 1. New Jersey: John Wiley & Sons–390. doi:10.1002/0471428035.ch1
Papadopoulos, J., Gerlach, T., Reiss, G. J., Mayer, B., and Müller, T. J. J. (2022). Consecutive multicomponent coupling-addition synthesis and chromophore characteristics of cyclohexene-embedded merocyanines and cyanines. Photochem 2, 672–693. doi:10.3390/photochem2030044
Paprocki, D., Koszelewski, D., Madej, A., Brodzka, A., Walde, P., and Ostaszewski, R. (2020). Evaluation of Biodegradable glucose based surfactants as a promoting medium for the synthesis of Peptidomimetics with the coumarin scaffold. ChemistrySelect 5, 9607–9614. doi:10.1002/slct.202002266
Parisi, O. I., Morelli, C., Scrivano, L., Sinicropi, M. S., Cesario, M. G., Candamano, S., et al. (2015). Controlled release of sunitinib in targeted cancer therapy: Smart magnetically responsive hydrogels as restricted access materials. RSC Adv. 5, 65308–65315. doi:10.1039/C5RA12229E
Park, J.-S., Chae, H., Chung, H. K., and Lee, S. (2011). Thin film encapsulation for flexible AM-OLED: A review. Semicond. Sci. Technol. 26, 034001. doi:10.1088/0268-1242/26/3/034001
Park, S., Kwon, O.-H., Kim, S., Park, S., Choi, M.-G., Cha, M., et al. (2005). Imidazole-based excited-state intramolecular proton-transfer materials: Synthesis and Amplified Spontaneous emission from a large single crystal. J. Am. Chem. Soc. 127, 10070–10074. doi:10.1021/ja0508727
Patel, P. N., Patel, N. C., and Desai, D. H. (2022). Synthesis of novel disperse dyes with dihydropyrimidinone scaffold: Development of multicomponent Protocol. Russ. J. Org. Chem. 58, 536–540. doi:10.1134/s1070428022040108
Paul, S., and Lee, Y. R. (2016). Eco-friendly construction of highly functionalized chromenopyridinones by an organocatalyzed solid-state melt reaction and their optical properties. Green Chem. 18, 1488–1494. doi:10.1039/c5gc02658j
Peddibhotla, S. (2009). 3-Substituted-3-hydroxy-2-oxindole, an emerging new scaffold for drug discovery with potential anti-cancer and other biological activities. Curr. Bioact. Compd. 5, 20–38. doi:10.2174/157340709787580900
Pei, K., Wu, Y., Wu, W., Zhang, Q., Chen, B., Tian, H., et al. (2012). Constructing organic D–A–π-A-Featured sensitizers with a quinoxaline Unit for high-efficiency solar cells: The effect of an auxiliary acceptor on the absorption and the energy level Alignment. Chem. Eur. J. 18, 8190–8200. doi:10.1002/chem.201103542
Peng, T., Murase, T., Goto, Y., Kobori, A., and Nakatani, K. (2005). A new ligand binding to G–G mismatch having improved thermal and alkaline stability. Bioorgan. Med. Chem. Lett. 15, 259–262. doi:10.1016/j.bmcl.2004.11.003
Pestellini, V., Giannotti, D., Giolitti, A., Fantò, N., Riviera, L., and Bellotti, M. G. (1987). New benzofuran-imidazoles as antimycotic agents. I. Synthesis and characterization. Chemioterapia 6, 269–271.
Petronijevic, J., Jankovic, N., and Bugarcic, Z. (2018). Synthesis of quinoxaline-based compounds and their antitumor and antiviral potentials. Mini. Rev. Org. Chem. 15, 220–226. doi:10.2174/1570193X14666171201143357
Pettit, G. R., Knight, J. C., Herald, D. L., Davenport, R., Pettit, R. K., Tucker, B. E., et al. (2002). Isolation of Labradorins 1 and 2 from Pseudomonas syringae pv. coronafaciens. J. Nat. Prod. 65, 1793–1797. doi:10.1021/np020173x
Poondra, R. R., Nallamelli, R. V., Meda, C. L. T., Srinivas, B., Grover, A., Muttabathula, J., et al. (2013). Discovery of novel 1, 4-dihydropyridine-based PDE4 inhibitors. Bioorg. Med. Chem. Lett. 23, 1104–1109. doi:10.1016/j.bmcl.2012.11.121
Prodi, A., Indelli, M. T., Kleverlaan, C. J., Alessio, E., and Scandola, F. (2002). Energy transfer pathways in pyridylporphyrin metal adducts and side-to-face arrays. Coord. Chem. Rev. 229, 51–58. doi:10.1016/S0010-8545(02)00107-8
Puthumana, S. S. E., and Damodaran, B. (2018). Multicomponent-reaction- (MCR-) assisted synthesis of a coumarin-based deep blue emitter for OLEDs and related applications. ChemistrySelect 3, 2951–2957. doi:10.1002/slct.201702989
Qu, B., Chen, Z., Liu, Y., Cao, H., Xu, S., Cao, S., et al. (2006). Orange and red emitting OLEDs based on phenothiazine polymers. J. Phys. D. Appl. Phys. 39, 2680–2683. doi:10.1088/0022-3727/39/13/007
Que, E. L., Domaille, D. W., and Chang, C. J. (2008). Metals in Neurobiology: Probing their chemistry and Biology with molecular imaging. Chem. Rev. 108, 1517–1549. doi:10.1021/cr078203u
Radzisewski, B. (1882). Ueber Glyoxalin und seine Homologe. Ber. Dtsch. Chem. Ges. 15, 2706–2708. doi:10.1002/cber.188201502245
Raingeaud, J., Gupta, S., Rogers, J. S., Dickens, M., Han, J., Ulevitch, R. J., et al. (1995). Pro-inflammatory Cytokines and environmental stress cause p38 mitogen-activated protein kinase activation by dual Phosphorylation on tyrosine and Threonine. J. Biol. Chem. 270, 7420–7426. doi:10.1074/jbc.270.13.7420
Ramirez-Ornelas, D. E., Alvarado-Martinez, E., Banuelos, J., Lopez Arbeloa, I., Arbeloa, T., Mora-Montes, H. M., et al. (2016). FormylBODIPYs: Privileged building blocks for multicomponent reactions. The case of the Passerini reaction. J. Org. Chem. 81, 2888–2898. doi:10.1021/acs.joc.5b02893
Rao, Y.-L., and Wang, S. (2011). Four-coordinate organoboron compounds with a π-conjugated chelate ligand for optoelectronic applications. Inorg. Chem. 50, 12263–12274. doi:10.1021/ic200658v
Raschip, I. E., Fifere, N., Varganici, C.-D., and Dinu, M. V. (2020). Development of antioxidant and antimicrobial xanthan-based cryogels with tuned porous morphology and controlled swelling features. Int. J. Biol. Macromol. 156, 608–620. doi:10.1016/j.ijbiomac.2020.04.086
Rawat, P., and Verma, S. M. (2016). Design and synthesis of chroman derivatives with dual anti-breast cancer and antiepileptic activities. Drug Des. Devel Ther. 10, 2779–2788. doi:10.2147/DDDT.S111266
Rawtani, D., and Agrawal, Y. K. (2012). Multifarious applications of halloysite nanotubes: A review. Rev. Adv. Mat. Sci. 30, 282–295.
Reddy, T. R. K., Mutter, R., Heal, W., Guo, K., Gillet, V. J., Pratt, S., et al. (2006). Library design, synthesis, and screening: Pyridine dicarbonitriles as potential prion disease therapeutics. J. Med. Chem. 49, 607–615. doi:10.1021/jm050610f
Redman, J. E., Feeder, N., Teat, S. J., and Sanders, J. K. M. (2001). Rh(III) porphyrins as building blocks for porphyrin coordination arrays: From dimers to Heterometallic Undecamers. Inorg. Chem. 40, 2486–2499. doi:10.1021/ic001038f
Reyes, H., Muñoz, B. M., Farfán, N., Santillan, R., Rojas-Lima, S., Lacroix, P. G., et al. (2002). Synthesis, crystal structures, and quadratic nonlinear optical properties in a series of push–pull boronate derivatives. J. Mat. Chem. 12, 2898–2903. doi:10.1039/B205308J
Richter, M. M. (2004). Electrochemiluminescence (ECL). Chem. Rev. 104, 3003–3036. doi:10.1021/cr020373d
Riva, R., Moni, L., and Müller, T. J. J. (2016). Multicomponent strategies for the diversity-oriented synthesis of blue emissive heterocyclic chromophores. Targets heterocyc. Syst. 20, 85–112. doi:10.17374/targets.2017.20.85
Rodembusch, F. S., Leusin, F. P., Campo, L. F., and Stefani, V. (2007). Excited state intramolecular proton transfer in amino 2-(2′-hydroxyphenyl)benzazole derivatives: Effects of the solvent and the amino group position. J. Lum. 126, 728–734. doi:10.1016/j.jlumin.2006.11.007
Roethle, P. A., and Trauner, D. (2008). The chemistry of marine furanocembranoids, pseudopteranes, gersolanes, and related natural products. Nat. Prod. Rep. 25, 298–317. doi:10.1039/B705660P
Rotstein, B. H., Mourtada, R., Kelley, S. O., and Yudin, A. K. (2011). Solvatochromic reagents for multicomponent reactions and their utility in the development of cell-Permeable macrocyclic peptide vectors. Chem. Eur. J. 17, 12257–12261. doi:10.1002/chem.201102096
Russo, R., Padanha, R., Fernandes, F., Veiros, L. F., Corzana, F., and Gois, P. M. P. (2020). Engineering boron hot spots for the site-selective installation of iminoboronates on peptide chains. Chem. Eur. J. 26, 15226–15231. doi:10.1002/chem.202002675
Sacoman Torquato da Silva, B. H., Bregadiolli, B. A., de Oliveira Graeff, C. F., and da Silva-Filho, L. C. (2017). NbCl5-Promoted synthesis of fluorescein dye derivatives: Spectroscopic and Spectrometric characterization and their application in dye-sensitized solar cells. ChemPlusChem 82, 261–269. doi:10.1002/cplu.201600530
Safak, C., and Simsek, R. (2006). Fused 1,4-dihydropyridines as potential calcium modulatory compounds. Mini. Rev. Med. Chem. 6, 747–755. doi:10.2174/138955706777698606
Safari, J., and Zarnegar, Z. (2015). An environmentally friendly approach to the green synthesis of azo dyes in the presence of magnetic solid acid catalysts. RSC Adv. 5, 17738–17745. doi:10.1039/C4RA13562H
Sailer, M., Franz, A. W., and Müller, T. J. J. (2008). Synthesis and electronic properties of Monodisperse oligophenothiazines. Chem. Eur. J. 14, 2602–2614. doi:10.1002/chem.200701341
Saini, M. S., Kumar, A., Dwivedi, J., and Singh, R. (2013). A review: Biological significances of heterocyclic compounds. Int. J. Pharm. Sci. Res. 4, 66–77.
Sainlos, M., Iskenderian, W. S., and Imperiali, B. (2009). A general screening strategy for peptide-based Fluorogenic ligands: Probes for Dynamic studies of PDZ Domain-mediated interactions. J. Am. Chem. Soc. 131, 6680–6682. doi:10.1021/ja900371q
Sánchez, A., and Vázquez, A. (2017). Bioactive peptides: A review. FQS 1, 29–46. doi:10.1093/fqs/fyx006
Santos, J., Mintz, E. A., Zehnder, O., Bosshard, C., Bu, X. R., and Günter, P. (2001). New class of imidazoles incorporated with thiophenevinyl conjugation pathway for robust nonlinear optical chromophores. Tetrahedron Lett. 42, 805–808. doi:10.1016/S0040-4039(00)02143-2
Saraswat, P., Jeyabalan, G., Hassan, M. Z., Rahman, M. U., and Nyola, N. K. (2016). Review of synthesis and various biological activities of spiro heterocyclic compounds comprising oxindole and pyrrolidine moities. Synth. Commun. 46, 1643–1664. doi:10.1080/00397911.2016.1211704
Sasaki, Y., Araki, Y., Ito, O., and Alam, M. M. (2007). Generation of long-lived radical ions through enhanced photoinduced electron transfer processes between [60]fullerene and phenothiazine derivatives. Photochem. Photobio. Sci. 6, 560–565. doi:10.1039/B617229F
Sato, K., Kawasaki, A., Karuo, Y., Tarui, A., Kawai, K., and Omote, M. (2020). Synthesis of new fluorescent molecules having an aggregation-induced emission property derived from 4-fluoroisoxazoles. Beilstein J. Org. Chem. 16, 1411–1417. doi:10.3762/bjoc.16.117
Scandola, F., Chiorboli, C., Prodi, A., Iengo, E., and Alessio, E. (2006). Photophysical properties of metal-mediated assemblies of porphyrins. Coord. Chem. Rev. 250, 1471–1496. doi:10.1016/j.ccr.2006.01.019
Schachinger, V., Britten, M. B., Dimmeler, S., and Zeiher, A. M. (2001). NADH/NADPH oxidase p22 phox gene polymorphism is associated with improved coronary endothelial vasodilator function. Eur. Heart J. 22, 96–101. doi:10.1053/euhj.2000.2123
Schaffroth, M., Lindner, B. D., Vasilenko, V., Rominger, F., and Bunz, U. H. F. (2013). Alkynylated diazadioxaacenes: Syntheses and properties. J. Org. Chem. 78, 3142–3150. doi:10.1021/jo400092r
Sedighian, H., Mamaghani, M. B., Notash, B., and Bazgir, A. (2021). Iodide-catalyzed selenium-assisted sequential multicomponent synthesis of a luminescence benzo-oxazino-isoindole framework. J. Org. Chem. 86, 2244–2253. doi:10.1021/acs.joc.0c02391
Seitz, M., and Reiser, O. (2005). Synthetic approaches towards structurally diverse γ-butyrolactone natural-product-like compounds. Curr. Opin. Chem. Biol. 9, 285–292. doi:10.1016/j.cbpa.2005.03.005
Sellamuthu, S., Singh, M., Kumar, A., and Singh, S. K. (2017). Type-II NADH dehydrogenase (NDH-2): A promising therapeutic target for antitubercular and antibacterial drug discovery. Expert Opin. Ther. Target. 21, 559–570. doi:10.1080/14728222.2017.1327577
Selvam, T. P., James, C. R., Dniandev, P. V., and Valzita, S. K. (2015). A mini review of pyrimidine and fused pyrimidine marketed drugs. J. Res. Pharm. Pract. 2.
Şener, İ., Karcı, F., Ertan, N., and Kılıç, E. (2006). Synthesis and investigations of the absorption spectra of hetarylazo disperse dyes derived from 2,4-quinolinediol. Dyes Pigments 70, 143–148. doi:10.1016/j.dyepig.2005.05.003
Seo, K. D., Song, H. M., Lee, M. J., Pastore, M., Anselmi, C., De Angelis, F., et al. (2011). Coumarin dyes containing low-band-gap chromophores for dye-sensitised solar cells. Dyes Pigments 90, 304–310. doi:10.1016/j.dyepig.2011.01.009
Shabir, G., Saeed, A., and Ali Channar, P. (2018). A review on the recent trends in synthetic strategies and applications of xanthene dyes. Mini. Rev. Org. Chem. 15, 166–197. doi:10.2174/1570193X14666170518130008
Shaikh, S. K. J., Sannaikar, M. S., Kumbar, M. N., Bayannavar, P. K., Kamble, R. R., Inamdar, S. R., et al. (2018). Microwave-expedited green synthesis, photophysical, computational studies of coumarin-3-yl-thiazol-3-yl-1,2,4-triazolin-3-ones and their anticancer activity. ChemistrySelect 3, 4448–4462. doi:10.1002/slct.201702596
Sharma, P. C., Bansal, K. K., Sharma, A., Sharma, D., and Deep, A. (2020). Thiazole-containing compounds as therapeutic targets for cancer therapy. Eur. J. Med. Chem. 188, 112016. doi:10.1016/j.ejmech.2019.112016
Sharma, V., and Kumar, V. (2014). Indolizine: A biologically active moiety. Med. Chem. Res. 23, 3593–3606. doi:10.1007/s00044-014-0940-1
Shetti, V. S., Pareek, Y., and Ravikanth, M. (2012). Sn(IV) porphyrin scaffold for multiporphyrin arrays. Coord. Chem. Rev. 256, 2816–2842. doi:10.1016/j.ccr.2012.09.013
Shi, X., Wang, H., Han, T., Feng, X., Tong, B., Shi, J., et al. (2012). A highly sensitive, single selective, real-time and “turn-on” fluorescent sensor for Al 3+ detection in aqueous media. J. Mat. Chem. 22, 19296–19302. doi:10.1039/C2JM33393G
Shibata, K., Satoh, T., and Miura, M. (2005). Palladium-catalyzed intermolecular three-component coupling of aryl iodides, alkynes, and Alkenes to produce 1,3-Butadiene derivatives. Org. Lett. 7, 1781–1783. doi:10.1021/ol0503743
Shiomi, K., Ui, H., Suzuki, H., Hatano, H., Nagamitsu, T., Takano, D., et al. (2005). A γ-lactone form nafuredin, nafuredin-γ, also inhibits helminth complex I. J. Antibiot. 58, 50–55. doi:10.1038/ja.2005.5
Shukla, P., Deswal, D., Pandit, M., Latha, N., Mahajan, D., Srivastava, T., et al. (2022). Exploration of novel TOSMIC tethered imidazo 1,2-a pyridine compounds for the development of potential antifungal drug candidate. Drug. Dev. Res. 83, 525–543. doi:10.1002/ddr.21883
Sigel, A., and Sigel, H. (1998). Metal ions in biological systems, volume 35: Iron transport and storage microorganisms, plants, and animals. Met.-Based Drugs 5, 262. doi:10.1155/MBD.1998.262a
Silva, S. J. G. J. F. M., and Pinto, A. C. (2001). The chemistry of isatins: A review from 1975 to 1999. J. Braz. Chem. Soc. 12, 273–324. doi:10.1590/S0103-50532001000300002
Silvestri, R., Artico, M., La Regina, G., Di Pasquali, A., De Martino, G., D'Auria, F. D., et al. (2004). Imidazole analogues of Fluoxetine, a novel class of anti-Candida agents. J. Med. Chem. 47, 3924–3926. doi:10.1021/jm049856v
Simon, S., and Petrášek, J. (2011). Why plants need more than one type of auxin. Plant Sci. 180, 454–460. doi:10.1016/j.plantsci.2010.12.007
Sindhu, J., Singh, H., and Khurana, J. M. (2015). Efficient synthesis of spiro[diindenopyridine-indoline]triones catalyzed by PEG-OSO3H-H2O and [NMP]H2PO4. Synth. Commun. 45, 202–210. doi:10.1080/00397911.2014.906616
Singh, H., Sindhu, J., Khurana, J. M., Sharma, C., and Aneja, K. R. (2013). A facile Eco-friendly one-pot five-component synthesis of novel 1,2,3-triazole-linked pentasubstituted 1,4-dihydropyridines and their biological and photophysical studies. Aust. J. Chem. 66, 1088–1096. doi:10.1071/ch13217
Singh, H., Sindhu, J., Khurana, J. M., Sharma, C., and Aneja, K. R. (2014). Ultrasound promoted one pot synthesis of novel fluorescent triazolyl spirocyclic oxindoles using DBU based task specific ionic liquids and their antimicrobial activity. Eur. J. Med. Chem. 77, 145–154. doi:10.1016/j.ejmech.2014.03.016
Sjöback, R., Nygren, J., and Kubista, M. (1995). Absorption and fluorescence properties of fluorescein. Spectrochim. Acta A 51, L7–L21. doi:10.1016/0584-8539(95)01421-p
Skonieczny, K., Ciuciu, A. I., Nichols, E. M., Hugues, V., Blanchard-Desce, M., Flamigni, L., et al. (2012). Bright, emission tunable fluorescent dyes based on imidazole and π-expanded imidazole. J. Mat. Chem. 22, 20649–20664. doi:10.1039/C2JM33891B
Smyth, T., Ramachandran, V. N., and Smyth, W. F. (2009). A study of the antimicrobial activity of selected naturally occurring and synthetic coumarins. Int. J. Antimicrob. Ag. 33, 421–426. doi:10.1016/j.ijantimicag.2008.10.022
Sokolova, E. A., Festa, A. A., Golantsov, N. E., Lukonina, N. S., Ioffe, I. N., Varlamov, A. V., et al. (2019). Highly fluorescent pyrido 2,3-b indolizine-10-carbonitriles through pseudo three-component reactions of N-(Cyanomethyl)pyridinium salts. Eur. J. Org. Chem. 2019, 6770–6775. doi:10.1002/ejoc.201900995
Soleymani, M., and Chegeni, M. (2019). The chemistry and applications of the quinoxaline compounds. Curr. Org. Chem. 23, 1789–1827. doi:10.2174/1385272823666190926094348
Somasundaram, S., Kamaraj, E., Hwang, S. J., and Park, S. (2018). Structural, photophysical, and theoretical studies of imidazole-based excited-state intramolecular proton transfer molecules. Spectrochim. Acta A 191, 325–335. doi:10.1016/j.saa.2017.10.023
Song, A., Wu, T., Chen, S., Zhang, M., and Shen, T. (1998). Syntheses and photophysical properties of amphiphilic dyads of fluorescein and carbazole linked with a flexible or semi-rigid bridge. Dyes Pigments 39, 371–382. doi:10.1016/S0143-7208(98)00022-9
Soni, R., Durgapal, S. D., Soman, S. S., and Georrge, J. J. (2019). Design, synthesis and anti-diabetic activity of chromen-2-one derivatives. Arab. J. Chem. 12, 701–708. doi:10.1016/j.arabjc.2016.11.011
Soumya, T. V., Thasnim, P., and Bahulayan, D. (2014). Step-economic and cost effective synthesis of coumarin based blue emitting fluorescent dyes. Tetrahedron Lett. 55, 4643–4647. doi:10.1016/j.tetlet.2014.06.071
Speck, K., and Magauer, T. (2013). The chemistry of isoindole natural products. Beilstein J. Org. Chem. 9, 2048–2078. doi:10.3762/bjoc.9.243
Stähelin, M., Burland, D. M., Ebert, M., Miller, R. D., Smith, B. A., Twieg, R. J., et al. (1992). Re-evaluation of the thermal stability of optically nonlinear polymeric guest-host systems. Appl. Phys. Lett. 61, 1626–1628. doi:10.1063/1.108497
Starrett, J. E., Montzka, T. A., Crosswell, A. R., and Cavanagh, R. L. (1989). Synthesis and biological activity of 3-substituted imidazo [1, 2-a] pyridines as antiulcer agents. J. Med. Chem. 32, 2204–2210. doi:10.1021/jm00129a028
Stasyuk, A. J., Banasiewicz, M., Cyrański, M. K., and Gryko, D. T. (2012). Imidazo[1,2-a]pyridines Susceptible to excited state intramolecular proton transfer: One-pot synthesis via an Ortoleva–King reaction. J. Org. Chem. 77, 5552–5558. doi:10.1021/jo300643w
Stephan, M., Stute, B., von Lieres, E., and Müller, T. J. J. (2022). Consecutive three-component synthesis of phenothiazine-based merocyanines – Bayesian optimization, electronic properties, and DSSC characteristics. Eur. J. Org. Chem. 2022, e202200163. doi:10.1002/ejoc.202200163
Striplin, D. R., and Crosby, G. A. (1994). Nature of the emitting 3MLCT manifold of rhenium(I) (diimine) (CO) 3Cl complexes. Chem. Phys. Lett. 221, 426–430. doi:10.1016/0009-2614(94)00282-7
Su, Y.-W., Lan, S.-C., and Wei, K.-H. (2012). Organic photovoltaics. Mat. Today 15, 554–562. doi:10.1016/S1369-7021(13)70013-0
Sueki, S., Takei, R., Zaitsu, Y., Abe, J., Fukuda, A., Seto, K., et al. (2014). Synthesis of 1,4-dihydropyridines and their fluorescence properties. Eur. J. Org. Chem. 2014, 5281–5301. doi:10.1002/ejoc.201402426
Suero, M. G., De la Campa, R., Torre-Fernandez, L., Garcia-Granda, S., and Florez, J. (2012). Enantioselective multicomponent synthesis of fused 6-5 bicyclic 2-butenolides by a Cascade Heterobicyclisation process. Chem. Eur. J. 18, 7287–7295. doi:10.1002/chem.201102288
Sujatha, K., and Vedula, R. R. (2018). Novel one-pot expeditious synthesis of 2,4-disubstituted thiazoles through a three-component reaction under solvent free conditions. Synth. Commun. 48, 302–308. doi:10.1080/00397911.2017.1399422
Sun, X., Liu, Y., Xu, X., Yang, C., Yu, G., Chen, S., et al. (2005). Novel Electroactive and photoactive molecular materials based on conjugated Donor−Acceptor structures for optoelectronic device applications. J. Phys. Chem. B 109, 10786–10792. doi:10.1021/jp0509515
Sun, X., Wang, Y.-W., and Peng, Y. (2012). A selective and Ratiometric Bifunctional fluorescent probe for Al3+ ion and proton. Org. Lett. 14, 3420–3423. doi:10.1021/ol301390g
Taek Han, Y., Jung, J.-W., and Kim, N.-J. (2017). Recent advances in the synthesis of biologically active Cinnoline, Phthalazine and quinoxaline derivatives. Curr. Org. Chem. 21, 1265–1291. doi:10.2174/1385272821666170221150901
Tasior, M., Gajewski, P., Vakuliuk, O., and Gryko, D. T. (2021). Novel donor-acceptor systems bearing an isoxazol-5-one core. Arkivoc 2021, 17–24. doi:10.24820/ark.5550190.p011.223
Taylor, A. P., Robinson, R. P., Fobian, Y. M., Blakemore, D. C., Jones, L. H., and Fadeyi, O. (2016). Modern advances in heterocyclic chemistry in drug discovery. Org. Biomol. Chem. 14, 6611–6637. doi:10.1039/C6OB00936K
Thakur, A., Singla, R., and Jaitak, V. (2015). Coumarins as anticancer agents: A review on synthetic strategies, mechanism of action and sar studies. Eur. J. Med. Chem. 101, 476–495. doi:10.1016/j.ejmech.2015.07.010
Thuita, J. K., Karanja, S. M., Wenzler, T., Mdachi, R. E., Ngotho, J. M., Kagira, J. M., et al. (2008). Efficacy of the diamidine DB75 and its prodrug DB289, against murine models of human African trypanosomiasis. Acta Trop. 108, 6–10. doi:10.1016/j.actatropica.2008.07.006
Tong, S., Zhao, S., He, Q., Wang, Q., Wang, M.-X., and Zhu, J. (2017). Fluorophores for excited-state intramolecular proton transfer by an Yttrium triflate catalyzed reaction of isocyanides with thiocarboxylic acids. Angew. Chem. Int. Ed. 56, 6599–6603. doi:10.1002/anie.201702488
Tong, Y., Lin, N.-H., Wang, L., Hasvold, L., Wang, W., Leonard, N., et al. (2003). Discovery of potent imidazole and cyanophenyl containing farnesyltransferase inhibitors with improved oral bioavailability. Bioorgan. Med. Chem. Lett. 13, 1571–1574. doi:10.1016/S0960-894X(03)00195-1
Torsi, L., Magliulo, M., Manoli, K., and Palazzo, G. (2013). Organic field-effect transistor sensors: A tutorial review. Chem. Soc. Rev. 42, 8612–8628. doi:10.1039/C3CS60127G
Tsuji, H., Sato, K., Sato, Y., and Nakamura, E. (2009). Benzo [b] phosphole sulfides. Highly electron-transporting and thermally stable molecular materials for organic semiconductor devices. J. Mat. Chem. 19, 3364–3366. doi:10.1039/B906197E
Tsuji, H., Sato, K., Sato, Y., and Nakamura, E. (2010). Benzophosphole oxide and sulfide for thermally stable Cathode buffer Layers in organic Thin-film photovoltaic devices. Chem. Asian J. 5, 1294–1297. doi:10.1002/asia.201000093
Tu, S., Wu, S., Han, Z., and Hao, W. (2009). An efficient microwave-assisted synthesis of pyrido[2,3-d]pyrimidine derivatives. Chin. J. Chem. 27, 1148–1152. doi:10.1002/cjoc.200990192
Uchida, M., Takamatsu, S., Arima, S., Miyamoto, K. T., Kitani, S., Nihira, T., et al. (2011). Total synthesis and absolute configuration of avenolide, extracellular factor in Streptomyces avermitilis. J. Antibiot. 64, 781–787. doi:10.1038/ja.2011.90
Ukawa, T. I. K., Kuriki, H., and Nohara, A. (1985). Synthesis of the metabolites and Degradation products of 2-Amino-7-isopropyl-5-oxo-5H-[1] benzopyrano [2, 3-b] pyridine-3-carboxylic acid (Amoxanox). Chem. Pharm. Bull. 33, 4432–4437. doi:10.1248/cpb.33.4432
Ulrich, G., Ziessel, R., and Harriman, A. (2008). The chemistry of fluorescent Bodipy dyes: Versatility Unsurpassed. Angew. Chem. Int. Ed. 47, 1184–1201. doi:10.1002/anie.200702070
Urselmann, D., Deilhof, K., Mayer, B., and Müller, T. J. J. (2016). Thiophene-forming one-pot synthesis of three thienyl-bridged oligophenothiazines and their electronic properties. Beilstein J. Org. Chem. 12, 2055–2064. doi:10.3762/bjoc.12.194
Usta, H., Risko, C., Wang, Z., Huang, H., Deliomeroglu, M. K., Zhukhovitskiy, A., et al. (2009). Design, synthesis, and characterization of ladder-type molecules and polymers. Air-stable, solution-Processable n-channel and Ambipolar semiconductors for Thin-film transistors via experiment and theory. J. Am. Chem. Soc. 131, 5586–5608. doi:10.1021/ja809555c
Valinsky, J. E., Easton, T. G., and Reich, E. (1978). Merocyanine 540 as a fluorescent probe of membranes: Selective staining of leukemic and immature hemopoietic cells. Cell. 13, 487–499. doi:10.1016/0092-8674(78)90322-7
Vasylyev, M. V., Maayan, G., Hovav, Y., Haimov, A., and Neumann, R. (2006). Palladium nanoparticles stabilized by alkylated polyethyleneimine as aqueous biphasic catalysts for the chemoselective stereocontrolled hydrogenation of alkenes. Org. Lett. 8, 5445–5448. doi:10.1021/ol062052k
Vazquez-Romero, A., Kielland, N., Arevalo, M. J., Preciado, S., Mellanby, R. J., Feng, Y., et al. (2013). Multicomponent reactions for de Novo synthesis of BODIPY probes: In vivo imaging of phagocytic macrophages. J. Am. Chem. Soc. 135, 16018–16021. doi:10.1021/ja408093p
Venugopala, K. N., Khedr, M. A., Attimarad, M., Padmashali, B., Kulkarni, R. S., Venugopala, R., et al. (2017). Review on chemistry of natural and synthetic indolizines with their chemical and pharmacological properties. J. Basic Clin. Pharm. 8.
Verma, T., Sinha, M., and Bansal, N. (2020). Heterocyclic compounds bearing triazine scaffold and their biological significance: A review. Anti-Cancer Agents Med. Chemistry- Anti-Cancer Agents) 20, 4–28. doi:10.2174/1871520619666191028111351
Videnov, G., Kaiser, D., Kempter, C., and Jung, G. (1996). Synthesis of naturally occurring, conformationally restricted oxazole- and thiazole-containing di- and tripeptide Mimetics. Angew. Chem. Int. Ed. 35, 1503–1506. doi:10.1002/anie.199615031
Vidyasagar, C. C., Muñoz Flores, B. M., Jiménez-Pérez, V. M., and Gurubasavaraj, P. M. (2019). Recent advances in boron-based schiff base derivatives for organic light-emitting diodes. Mat. Today Chem. 11, 133–155. doi:10.1016/j.mtchem.2018.09.010
Vinayak, R., and Nayek, H. P. (2019). Selective sensing of a Cu(ii) ion by organotin anchored keto-enamine ligands. New J. Chem. 43, 16050–16057. doi:10.1039/C9NJ03204E
Vitório, F., Pereira, T. M., Castro, R. N., Guedes, G. P., Graebin, C. S., and Kümmerle, A. E. (2015). Synthesis and mechanism of novel fluorescent coumarin–dihydropyrimidinone dyads obtained by the Biginelli multicomponent reaction. New J. Chem. 39, 2323–2332. doi:10.1039/C4NJ02155J
Wachenfeldt, H. v., Röse, P., Paulsen, F., Loganathan, N., and Strand, D. (2013). Catalytic three-component domino reaction for the preparation of trisubstituted oxazoles. Chem. Eur. J. 19, 7982–7988. doi:10.1002/chem.201300019
Wagenknecht, H.-A. (2008). Fluorescent DNA base modifications and Substitutes: Multiple fluorophore labeling and the DETEQ concept. Ann. Ny. Acad. Sci. 1130, 122–130. doi:10.1196/annals.1430.001
Walker, B., Kim, C., and Nguyen, T.-Q. (2011). Small molecule solution-Processed bulk heterojunction solar cells. Chem. Mat. 23, 470–482. doi:10.1021/cm102189g
Wang, S., Zhao, L., Xu, Z., Wu, C., and Cheng, S. (2002). Novel nonlinearity–transparency–thermal stability trade-off of imidazole chromophores for nonlinear optical application. Mat. Lett. 56, 1035–1038. doi:10.1016/S0167-577X(02)00671-7
Wang, X.-C., Wang, L.-L., Ouyang, X.-W., Ma, S.-P., Liu, J.-H., and Hu, L.-H. (2009). Sesquiterpenes and dimers thereof from chloranthus fortunei. HCA 92, 313–320. doi:10.1002/hlca.200800262
Wang, Z.-S., Cui, Y., Hara, K., Dan-oh, Y., Kasada, C., and Shinpo, A. (2007). A high-light-Harvesting-efficiency coumarin dye for stable dye-sensitized solar cells. Adv. Mat. 19, 1138–1141. doi:10.1002/adma.200601020
Weber, L., Illgen, K., and Almstetter, M. (1999). Discovery of new multi component reactions with combinatorial methods. Synlett 1999, 366–374. doi:10.1055/s-1999-2612
Wenzler, T., Boykin, D. W., Ismail, M. A., Hall, J. E., Tidwell, R. R., and Brun, R. (2009). New treatment option for second-stage african sleeping sickness: In vitro and in vivo efficacy of aza analogs of DB289. Antimicrob. Agents Chemother. 53, 4185–4192. doi:10.1128/AAC.00225-09
Wilbert, F., and Müller, T. J. J. (2022). Consecutive multicomponent syntheses of N-substituted 3-arylallylidene indolones – solid-state emitters and photoisomerization. Dyes Pigments 198, 109938. doi:10.1016/j.dyepig.2021.109938
Williamson, P., Mattocks, K., and Schlegel, R. A. (1983). Merocyanine 540, a fluorescent probe sensitive to lipid packing. BBA-Biomembranes 732, 387–393. doi:10.1016/0005-2736(83)90055-X
Winters, B. H., Mandelberg, H. I., and Mohr, W. B. (1974). Photochemical products in coumarin laser dyes. Appl. Phys. Lett. 25, 723–725. doi:10.1063/1.1655376
Woody, K. B., Henry, E. M., Jagtap, S., and Collard, D. M. (2011). Synthesis and characterization of Poly(5,8-quinoxaline ethynylene)s. Macromolecules 44, 9118–9124. doi:10.1021/ma201347z
Wu, B., Santra, M., and Yoshikai, N. (2014). A highly modular one-pot multicomponent approach to functionalized benzo b phosphole derivatives. Angew. Chem. Int. Ed. 53, 7543–7546. doi:10.1002/anie.201404019
Wu, H., Lin, W., Wan, Y., Xin, H.-q., Shi, D.-q., Shi, Y.-h., et al. (2010). Silica gel-catalyzed one-pot syntheses in water and fluorescence properties studies of 5-Amino-2-aryl-3H-chromeno 4,3,2-de 1,6 naphthyridine-4-carbonitriles and 5-Amino-2-aryl-3H-quinolino 4,3,2-de 1,6 naphthyridine-4-carbonitriles. J. Comb. Chem. 12, 31–34. doi:10.1021/cc9001179
Wu, Q.-X., Yang, A.-M., and Shi, Y.-P. (2005). Sesquiterpenoids from Ligularia virgaurea spp. oligocephala. Tetrahedron 61, 10529–10535. doi:10.1016/j.tet.2005.08.058
Wu, Y., and Zhu, W. (2013). Organic sensitizers from D–π–A to D–A–π–A: Effect of the internal electron-withdrawing units on molecular absorption, energy levels and photovoltaic performances. Chem. Soc. Rev. 42, 2039–2058. doi:10.1039/C2CS35346F
Wurthner, F. (1999). DMF in acetic anhydride: A useful reagent for multiple-component syntheses of merocyanine dyes. Synthesis 1999, 21032113–2113. doi:10.1055/s-1999-3635
Würthner, F., and Meerholz, K. (2010). Systems chemistry approach in organic photovoltaics. Antimicrob. Agents Chem. 16, 9366–9373. doi:10.1002/chem.201001153
Wurthner, F., Sens, R., Etzbach, K. H., and Seybold, G. (1999). Design, synthesis, and evaluation of a dye library: Glass-forming and solid-state luminescent merocyanines for functional materials. Angew. Chem. Int. Ed. 38, 1649–1652. doi:10.1002/(sici)1521-3773(19990601)38:11<1649:aid-anie1649>3.0.co;2-h
Xie, Y., Pan, Y., Xiao, F., Lei, Y., Zhou, Y., Liu, M., et al. (2021). Synthesis, crystal structures and solid-state acidochromism of multiaryl-substituted pyridine derivatives with aggregation-induced emission property. Dyes Pigm 188, 109217. doi:10.1016/j.dyepig.2021.109217
Xu, D., Hao, J., Gao, H., Wang, Y., Wang, Y., Liu, X., et al. (2018). Twisted donor–acceptor cruciform fluorophores exhibiting strong solid emission, efficient aggregation-induced emission and high contrast mechanofluorochromism. Dyes Pigments 150, 293–300. doi:10.1016/j.dyepig.2017.12.031
Yadav, R., Darakshan, , Bhaumick, P., Choudhury, L. H., and Parvin, T. (2022). Synthesis of pentacyclic pyran fused pyrazolo benzo h quinolines by multicomponent reaction and their photophysical studies. ChemistrySelect 7. doi:10.1002/slct.202104124
Yam, V. W. (2010). WOLEDs and organic photovoltaics: Recent advances and applications, 1. Berlin, Heidelberg, Berlin: Springer. doi:10.1007/978-3-642-14935-1
Yamashita, M., Hirano, K., Satoh, T., and Miura, M. (2011). Synthesis of 1,4-Diarylbuta-1,3-dienes through palladium-catalyzed decarboxylative coupling of unsaturated carboxylic acids. Adv. Synth. Cat. 353, 631–636. doi:10.1002/adsc.201000897
Yan, C.-G., Cai, X.-M., Wang, Q.-F., Wang, T.-Y., Zheng, M. J. O., and Chemistry, B. (2007). Microwave-assisted four-component, one-pot condensation reaction: An efficient synthesis of annulated pyridines. Org. Biomol. Chem. 5, 945–951. doi:10.1039/b617256c
Yang, K., Chen, Z. X., Zhou, Y. J., Chen, Q., Yu, S. W., Luo, S. H., et al. (2022). Simple inorganic base promoted polycyclic construction using mucohalic acid as a C-3 synthon: Synthesis and AIE probe application of benzo 4,5 imidazo 1,2-a pyridines. Org. Chem. Front. 9, 1127–1136. doi:10.1039/d1qo01753e
Yang, L., Liu, Y., Zhou, X., Wu, Y., Ma, C., Liu, W., et al. (2016). Asymmetric anthracene-fused BODIPY dye with large Stokes shift: Synthesis, photophysical properties and bioimaging. Dyes Pigments 126, 232–238. doi:10.1016/j.dyepig.2015.11.028
Yang, Y., Zhao, Q., Feng, W., and Li, F. (2013). Luminescent chemodosimeters for bioimaging. Chem. Rev. 113, 192–270. doi:10.1021/cr2004103
Yang, Y., Zou, J., Rong, H., Qian, G. D., Wang, Z. Y., and Wang, M. Q. (2007). Influence of various coumarin dyes on the laser performance of laser dyes co-doped into ORMOSILs. Appl. Phys. B 86, 309–313. doi:10.1007/s00340-006-2462-0
Yao, C.-S., Lu, K., Song, B., Liu, B., Li, T.-J., and Yu, C.-X. (2014). An efficient synthesis and fluorescent properties of pyrazole 3,4-b thieno 2,3-e pyridine derivatives. J. Heterocycl. Chem. 51, 1807–1810. doi:10.1002/jhet.1888
Yao, J., Dou, W., Qin, W., and Liu, W. (2009). A new coumarin-based chemosensor for Fe3+ in water. Inorg. Chem. Commun. 12, 116–118. doi:10.1016/j.inoche.2008.11.012
Young, G. P. (1984). Calcium channel blockers in emergency medicine. Ann. Emerg. Med. 13, 712–722. doi:10.1016/s0196-0644(84)80734-9
Zakerhamidi, M. S., and Sorkhabi, S. G. (2015). Solvent effects on the molecular resonance structures and photo-physical properties of a group of oxazine dyes. J. Lumin. 157, 220–228. doi:10.1016/j.jlumin.2014.08.062
Zayed, M. E. M., and Kumar, P. (2017). Spectroscopic physicochemical and photophysical investigation of biologically active 2-oxo-quinoline-3-carbonitrile derivative. J. Fluoresc. 27, 853–860. doi:10.1007/s10895-016-2021-y
Zeni, G., and Larock, R. C. (2004). Synthesis of heterocycles via palladium π-Olefin and π-alkyne chemistry. Chem. Rev. 104, 2285–2310. doi:10.1021/cr020085h
Zhang, G., Sun, J., Xue, P., Zhang, Z., Gong, P., Peng, J., et al. (2015). Phenothiazine modified triphenylacrylonitrile derivates: AIE and mechanochromism tuned by molecular conformation. J. Mat. Chem. C 3, 2925–2932. doi:10.1039/C4TC02925A
Zhang, M.-Z., Chen, Q., and Yang, G.-F. (2015). A review on recent developments of indole-containing antiviral agents. Eur. J. Med. Chem. 89, 421–441. doi:10.1016/j.ejmech.2014.10.065
Zhang, X., and Larock, R. C. (2003). Synthesis of highly substituted 1,3-dienes and 1,3,5-Trienes by the palladium-catalyzed coupling of vinylic halides, internal alkynes, and Organoboranes. Org. Lett. 5, 2993–2996. doi:10.1021/ol0348349
Zheng, B., and Huo, L. (2021). Recent advances of furan and its derivatives based semiconductor materials for organic photovoltaics. Small Methods 5, 2100493. doi:10.1002/smtd.202100493
Zhu, J., Wang, Q., and Wang, M. (2014). Multicomponent reactions in organic synthesis. John Wiley & Sons.
Zhu, Q., Huang, L., Chen, Z., Zheng, S., Lv, L., Zhu, Z., et al. (2013). A new series of C-6 unsubstituted tetrahydropyrimidines: Convenient one-pot Chemoselective synthesis, aggregation-induced and size-independent emission characteristics. Chem. Eur. J. 19, 1268–1280. doi:10.1002/chem.201203012
Ziarani, G. M., Mohammadsaeed, S., Badiei, A., and Ghasemi, J. B. (2022). Multicomponent synthesis and investigations fluorescence activity of chromenone-pyrazole compounds. J. Fluoresc. 32, 347–357. doi:10.1007/s10895-021-02835-9
Zonouzi, A., Hosseinzadeh, F., Karimi, N., Mirzazadeh, R., and Ng, S. W. (2013). Novel approaches for the synthesis of a library of fluorescent Chromenopyrimidine derivatives. ACS Comb. Sci. 15, 240–246. doi:10.1021/co300141j
Keywords: absorption, chromophores, diversity-oriented synthesis, donor-acceptor systems, fluorescence, multicomponent reactions, one-pot synthesis
Citation: Brandner L and Müller TJJ (2023) Multicomponent synthesis of chromophores – The one-pot approach to functional π-systems. Front. Chem. 11:1124209. doi: 10.3389/fchem.2023.1124209
Received: 14 December 2022; Accepted: 26 January 2023;
Published: 17 March 2023.
Edited by:
Fabiano Severo Rodembusch, Universidade Federal do Rio Grande do Sul—Instituto de Química, BrazilReviewed by:
Felipe Lange Coelho, Universidade Federal de Goiás, BrazilRicardo Ferreira Affeldt, Federal University of Santa Catarina, Brazil
Copyright © 2023 Brandner and Müller. This is an open-access article distributed under the terms of the Creative Commons Attribution License (CC BY). The use, distribution or reproduction in other forums is permitted, provided the original author(s) and the copyright owner(s) are credited and that the original publication in this journal is cited, in accordance with accepted academic practice. No use, distribution or reproduction is permitted which does not comply with these terms.
*Correspondence: Thomas J. J. Müller, ThomasJJ.Mueller@hhu.de; Larissa Brandner, Larissa.Brandner@hhu.de