- Chinese People’s Liberation Army Center for Disease Control and Prevention, Beijing, China
Metal-organic framework materials (MOFs) have been widely used in food contamination adsorption and detection due to their large specific surface area, specific pore structure and flexible post-modification. MOFs with specific pore size can be targeted for selective adsorption of some contaminants and can be used as pretreatment and pre-concentration steps to purify samples and enrich target analytes for food contamination detection to improve the detection efficiency. In addition, MOFs, as a new functional material, play an important role in developing new rapid detection methods that are simple, portable, inexpensive and with high sensitivity and accuracy. The aim of this paper is to summarize the latest and insightful research results on MOFs for the adsorption and detection of food contaminants. By summarizing Zn-based, Cu-based and Zr-based MOFs with low cost, easily available raw materials and convenient synthesis conditions, we describe their principles and discuss their applications in chemical and biological contaminant adsorption and sensing detection in terms of stability, adsorption capacity and sensitivity. Finally, we present the limitations and challenges of MOFs in food detection, hoping to provide some ideas for future development.
1 Introduction
Safety problems caused by food contamination have been paid more attention recently. According to the nature of different pollutants, food contaminants can be divided into biological, chemical, and physical contamination. Biological contamination mainly includes bacteria and bacterial toxins, fungi and fungal toxins, viruses, other microorganisms, and parasites and insects caused by pollution. Chemical contamination mainly includes pesticide and veterinary drug residues, toxic metals, and organisms (e.g., lead, arsenic, mercury, chromium, phenol), food additives (e.g., nitrite, potassium sorbate), prohibited additives (e.g., sodium formaldehyde sulfoxylate, melamine, plasticizers), harmful substances produced during food processing and storage (e.g., nitrosamines, acrylamide). Physical contamination mainly includes foreign substances and radioactive material contamination. These food risk factors in raw materials, processing, transportation, preparation, storage, and supply may produce harm at any time (Hitabatuma et al., 2022). In recent years, contamination incidents, including dioxin-contaminated eggs, milk powder contaminated with melamine, and food products carrying Salmonella, have been reported (Wang J et al., 2019). Therefore, detection and analysis of food contamination are critical in identifying and controlling risks to ensure food safety. Many methods have been developed to detect the presence of safety hazards in food using nanotechnology (Javier et al., 2016). Most of the methods used to assess food safety, such as high-performance liquid chromatography (HPLC), inductively coupled plasma mass spectrometry (ICP-MS), and gas chromatography-mass spectrometry (GC-MS), require great human labor, expensive equipment, long detection times, bulky equipment and the others, making them less applicable in rapid detection (Chinnapaiyan et al., 2022; Zahra et al., 2022). To overcome the limitations of conventional methods, there is an urgent need to develop new rapid detection methods that are easy to use, portable, less expensive, with high sensitivity and accuracy, such as fluorescence sensing, electrochemical sensing, surface-enhanced Raman scattering (SERS) and the others. It is also important to develop new materials with the required properties to enable their integration into sensor systems. Among various materials research, metal nanoparticles (NPs), graphene oxide (GO), carbon nanotubes (CNT), molecularly imprinted polymers (MIP), quantum dots (QDs) have all been extensively investigated. These materials are used in sensor construction as components in devices tailored to detect chemical or biological contaminants (Yang L et al., 2022). Metal-organic framework materials (MOFs) are increasingly being investigated as emerging functional materials in food detection (Hashemi et al., 2017). In addition, sample preconditioning is becoming increasingly important because the matrix of animal-derived food samples is complex and often requires purification and enrichment of residual compounds in sample before testing. Currently, the commonly used methods for food sample pretreatment are organic solvent dilution and extraction, which, however, could be time-consuming and could reduce the sensitivity of the method due to the effect of organic solvents on bioactive materials (Fang et al., 2019). Therefore, it is necessary to find an efficient, rapid, and biocompatible pretreatment method. In recent years, MOFs with more adsorption properties are considered ideal adsorbents (Tokalıoğlu et al., 2017) for sample purification, and it has been increasingly used in the adsorption and purification of hazardous substances (Li Z et al., 2019; Li et al., 2020b).
MOFs are a class of hybrid porous materials with infinite topological structures. At present, more than 20,000 kinds of MOFs have been reported (Jiao et al., 2019), and they have been used in fields including gas storage and release (Qin et al., 2022), catalysis (Jin et al., 2023), drug delivery (Weng et al., 2022), and contaminant removal (Ma et al., 2022a) due to their high porosity and tunable physical and chemical properties. MOFs have some special properties, such as subject-object interactions, hydrogen bonding between analyte and framework, chiral framework and analyte-specific signal response, manifesting their potential in the detection and analysis of food safety. Moreover, the application of MOFs in food is growing rapidly in recent years, with increasing number of papers being published each year, as shown in Figure 1. So far, MOFs have demonstrated their versatility, and some of them have been developed for pesticide and veterinary drug residue removal (Ma et al., 2022b), food packaging additives, food preservation, and food testing and monitoring (Li et al., 2017) for their high biocompatibility and non-reactivity with background substances (Shen et al., 2021). The unique adsorption properties of MOFs (e.g., high adsorption capacity and guest selectivity) allow them to be widely used in pre-treatment samples. The porous structure enables guest molecules to diffuse into their pores, and some special MOFs materials can be customized according to the morphology and size of the measured substance, which plays a role in concentrating the detector and eliminating impurity interference and in improving the accuracy, selectivity, and sensitivity of the detection method. Stability and recoverability are the most critical factors for the practical application of MOFs, especially in complex food matrices (Nong et al., 2020). One non-negligible drawback of MOFs is that their recoverability is lower than that of chromatographic columns (Wu et al., 2021), which can be improved by changing the ligand bonding properties, ligand conformation, and metal nodes. The stability of MOFs refers to their thermal, hydrolytic, and mechanical stability (Howarth et al., 2016). Thermal stability of MOFs is usually related to the strength of ligand bonds and number of linkers (Burtch et al., 2014). Increasing the metal-ligand binding strength using high-valent metal ions is an effective to improve thermal stability (Howarth et al., 2016). Currently, commonly used metal ions for the fabrication of chemically stable MOFs include aluminum, iron, zirconium, copper, and zinc (Jiao et al., 2019; Yuan et al., 2018). In addition, MOFs can be used as carriers to encapsulate, for example, proteins and enzymes to enhance biostability.
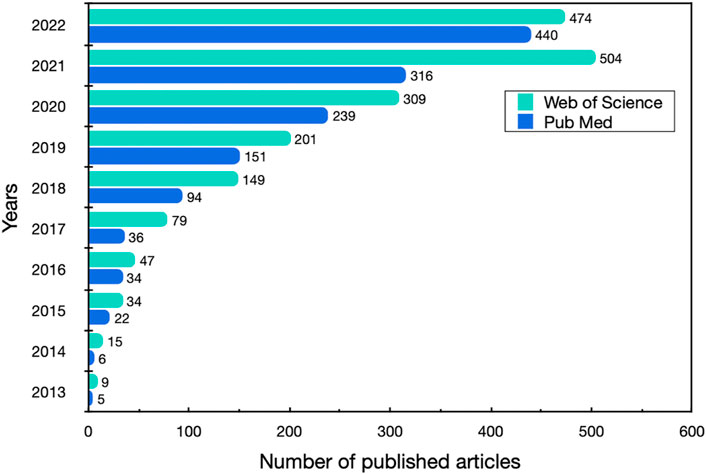
FIGURE 1. Number of published articles by MOFs in the field of food in the last decade. Data up to 17 December 2022 were obtained by searching the two databases for the keywords “metal organic framework and food.”
This paper reviewed the recent research progress in the adsorption and detection of food contamination from the perspective of different metal ion MOFs. Based on the preliminary literature research, the paper listed several common types of MOFs, including zinc-based, copper-based, and zirconium-based MOFs (Figure 2), which all share a common feature of easy synthesis and modification, and functionalization and are vital for their application in food detection.
2 Introduction of MOFs
2.1 Structural characteristics of MOFs
The concept of MOFs, also known as porous coordination polymers (PCPs), was first introduced by Yaghi and Li, (1995) in order to form a new crystalline material with a large surface area (typically in the range of 1,000 to 10,000 g/m2) by linking organic linking ligands to metal ions/clusters (secondary units SBUs). MOFs have a well-defined skeletal structure similar to that of zeolites. Unlike heterogeneous carbon materials, however, they have a very uniform pore distribution. In addition, the addition of functional groups (e.g., halogens, nitrogen, sulphur, carboxyl, cyano, nitro) to organic linkers, the selection of different metals depending on the application and the flexible adjustment of the electronic properties of the pore surface are difficult to achieve with commercially established materials such as zeolites and activated carbons (Fletcher et al., 2005). Rigid MOFs have relatively stable and robust porous frameworks with permanent porosity, similar to zeolites and other inorganic porous materials. Central metal ions/clusters play an important role in the stability of the backbone structure of MOFs. At the same time, a wide variety of organic ligands can produce MOFs with different pore sizes and pore structures (Nong et al., 2020) so that the dynamic interactions, sizes, and selectivity between pores and target guest molecules can be achieved by different organic ligands and functional groups (Deng et al., 2010). Flexible MOFs have a dynamic “soft” framework responding to external stimuli, for instance, pressure, temperature, and guest molecules, and such a sensitivity to external stimuli provides specific properties of MOFs and has been successfully combined with a variety of materials (biomolecules, carbon nanotubes, oxides, polymers, graphene, and quantum dots) to create materials with a variety of unique properties (Jiao et al., 2019).
MOF-5 [(ZnO4(BDC)3 (DMF)8C6H5Cl, DMF = dimethyl formamide], also named as IRMOF-1, was first synthesized by Li et al. (1999) as the most common of the IRMOFs series. MOF-5 uses terephthalic acid as an organic ligand coordination with Zn2+ to form a three-dimensional material in octahedral form, the specific surface area of MOF-5 is as high as 2,900 m2/g. The commonly studied ZIF-8 comprises transition metal ions Zn2+ coordinated with imidazole groups or imidazole derivatives. In the reaction, the N atom on the rigid imidazole ring loses its proton and coordinates with the transition metal ion. The combination of metal ions with imidazolyl or imidazole derivative ligands can produce different structures of ZIFs under different conditions, achieving a diverse and tunable structure. Guest@ZIFs, which are a kind of composite materials with ZIFs as the main body to load functional guest molecules, are widely studied because of their ability to enhance some properties of the main material, such as catalytic, electrochemical and fluorescent activity (Zhang X. X. et al., 2020). Although the stability of MOFs is improved by modification treatment, their pore volume and specific surface area are also reduced to some extent. Therefore, studies on the direct construction of synthetic MOFs with stability have been widely conducted. Cavka et al. (2008) synthesized UiO-66 (UiO stands for University of Oslo) consisting of Zr6 (μ3-O)4 (μ3-OH)4(CO2)12 metal ion cluster linked to phthalic acid. Metal ion clusters as nodes combining with organic ligands could greatly increase the porosity and specific surface area of the material, and UiO-66 also exhibits excellent stability. Due to their strong porosity and structural stability, Zr-MOFs have been intensively investigated in catalysis, molecular adsorption separation, drug delivery, fluorescence sensing, electrochemistry, and porous carriers (Bai et al., 2016).
The antimicrobial activity of MOFs may be caused by the release of metal ions or (and) through the disruption of cell membrane integrity through the active sites on material surface to degrade proteins and fatty acids on bacterial membrane. Synergistic effects (Sultana et al., 2022) can also be observed when metal ions and bioactive organic chains have antimicrobial activity. In addition, metal ions in MOFs can form coordination bond with cellulose carboxyl groups to promote the immobilization and distribution of MOFs on the surface of cellulose fibers, thereby enhancing the antimicrobial activity of biocomposites (Duan et al., 2018). Cu-based MOFs have open unsaturated Cu metal sites, and according to relevant literature, MOFs have obtained excellent antibacterial properties in the presence of Cu2+ (Gabriela et al., 2016). Gwon et al. (2020) conducted antibacterial tests using MOFs composed of the identical organic ligands of different metals, and the results showed that Cu-based MOFs have more potent antibacterial properties, and that the central metal of MOFs is more critical than the ligands in targeting the antibacterial effect.
2.2 Principle of MOFs for food contamination adsorption and detection
2.2.1 MOFs as adsorption material
The ability of MOFs as adsorbents can be attributed to their great structural potential, such as central metal ions, open metal sites, linkers, porosity, and functionalization/modification of MOFs. The main advantages of MOFs are their large specific surface area, huge porosity, and easy adjustment of pore size and shape from microporous to mesoporous scale, which can lead to high extraction efficiency (Bazargan et al., 2021). MOFs clearly have better adsorption capacity and selectivity than zeolites and other materials currently commercially available (Caroline et al., 2015). The ability of MOFs to act as adsorbent materials cannot be separated from the following points: 1) A large specific surface area and pore volume that allows them to have a high adsorption capacity, while the pore size is the basis for their selective adsorption of different sized objects. 2) The active sites of MOFs can be specific for the chemisorption of certain substances. 3) Better water stability. 4) Different metal ions and diverse organic ligands make MOFs structurally diverse and therefore have the potential for selective adsorption (Yang and Gates, 2019). Analytes are often present at trace or ultra-trace levels in the sample matrix, making it difficult to obtain accurate quantitative data for target analytes using instrumental analysis alone. The porous nature of MOFs allows adsorption and pre-concentration of analytes in complex sample matrices for purification and enrichment of target analytes, therefore further improving sensing sensitivity (Xuan et al., 2022). To achieve higher selectivity, researchers usually adjust the pore size of MOFs according to the size of specific food toxin molecules. Currently, solid phase extraction (SPE), micro solid phase extraction (μSPE), magnetic solid phase extraction (MSPE), solid phase microextraction (SPME), and stir bar sorptive extraction (SBSE) techniques have been used to extract target analytes from complex sample matrices, followed by instrumental assays (Wu et al., 2021).
2.2.2 MOFs as sensor
Highly active sensors composed of nanocomposites typically have high adsorption capacity, large surface area, inter-crystalline mesoporosity and low electron transfer resistance (Prathap et al., 2018). MOFs-based sensors have been widely developed. According to the different principles of action, they can be divided into fluorescent sensors, electrochemical sensors, mechanical sensors, and so on (Lei et al., 2014). Fluorescent and electrochemical sensors are currently the most studied sensors (Stassen et al., 2017; Wang X et al., 2019). Fluorescence sensors utilize the optical properties of material and convert changes into analytical signals (Marimuthu et al., 2022). MOFs-based fluorescent sensors have sensing targets, including cations, anions, small organic molecules, gases, biomarkers. In the current research, luminescent metal-organic frameworks (LMOFs) were developed according to the following approaches: 1) Luminescent molecules as ligands for MOFs for fluorescence sensing; 2) Lanthanide elements with unique fluorescence characteristics as metal ions of MOFs; 3) Doping of various functional elements into MOFs for fluorescence sensing. Some MOFs have luminescent behaviors due to their structural properties such as metal-to-ligand charge transfer (MLCT), ligand-to-metal charge transfer (LMCT), adsorption emission (Allendorf et al., 2009).
Metal ions are often used as signal tags in electrochemical assay systems because signals generated by chemical valence changes can be electrochemically detected, allowing some MOFs to exhibit excellent conductivity and high charge mobility. Many MOFs are involved in redox reactions. For efficient electrochemical sensors, two main points need to be satisfied: 1) high specificity of the signal tag and 2) high sensitivity and stability of the modified electrodes. MOFs and their composites can be designed as signal tags for electrochemical sensing because of their special structure, and water-stable MOFs are usually seen as long-term stable sensors (Yang Z et al., 2022). In addition, MOFs are ideal materials for surface modification of electrodes, and by combining MOFs with the kinetics of analytes or high-loading capacity materials, multiple properties can be integrated to improve the sensitivity of MOFs-based electrochemical sensors (Kreno et al., 2012).
3 Application of MOFs in food contamination adsorption and detection
3.1 Application of Zn-based MOFs in food contamination adsorption and detection
Zn-based MOFs usually consist of metallic ZnO or ZnO octahedral clusters as metal nodes, which can be combined with different organic ligands to form MOFs with different pore sizes. For example, the IRMOF series consists of ZnO octahedral clusters with various organodicarboxylate adapters forming different three-dimensional stereo networks with pore sizes ranging from IRMOF 1 to IRMOF 16, with a range of 12.8–28.8 Å (Bahrani et al., 2019). Another classical group of zinc-based MOFs is the ZIF series, which exhibit high thermal stability (up to 550°C in N2) and stability in organic solvents due to the strong bond between the central metal ion and the nitrogen atom in the ligand. ZIF-8, composed of zinc ions and 2-methylimidazole, has been extensively studied due to its unique properties such as large endopores and specific surface area (11.6 Å and 1,947 m2/g) (Bazargan et al., 2021).
3.1.1 Zn-based MOFs as adsorption materials for food contamination
3.1.1.1 Adsorption of chemical contamination by Zn-based MOFs
Many of the analytes in food analysis pretreatments are large molecules. While most MOFs typically have relatively small pores, the small pore windows of some MOFs make it unlikely for analytes to reach the interior of the pores. However, MOFs still show the ability to extract these analytes, which can be attributed to external surface adsorption. ZIF-8 has a sodium feldspar-type Zn (mim)2 framework and a large cage-like (∼12.5 Å) highly hydrophobic pore system connected by small windows (Huang et al., 2006) (∼3.3 Å), which have high porosity (micropore volume: ∼0.636 cm3/g), large surface area (BET surface area: ∼1,630 m2/g), and excellent stability in organic solvents, water and highly alkaline aqueous solutions (Rahul et al., 2008). ZIF-8 shows excellent advantages in food sample detection pretreatment (Ge and Lee, 2011). Yang et al. (2013) reported the use of ZIF-8 as a column-packing sorbent for online solid phase extraction (SPE) combined with high-performance liquid chromatography (HPLC) for the detection of oxytetracycline (OTC), tetracycline (TC) and chlortetracycline (CTC) in water and milk samples. OTC, TC, and CTC are commonly found in foods of animal origin because they are broad-spectrum antibiotics used as drugs for the treatment of livestock and as additives in animal feed. Excellent analytical performance was achieved with the help of ZIF-8, with recoveries of tetracyclines in milk and water samples ranging from 70.3% to 107.4%. However, tetracyclines molecular size is larger than the ZIF-8 pore size and cannot diffuse into the pores of ZIF-8. The observed extraction performance may result from analyte adsorption on the external crystal surface of ZIF-8. ZIF-8 is strongly thermally stable, maintaining high structural stability at a temperature up to 550°C. ZIF-8 has a more abundant and direct synthesis method than other MOFs or adsorbent materials. However, it is poorly dispersed in water and tends to agglomerate, which would lead to reduced adsorption capacity (Mo et al., 2022). Therefore, ZIF-8 can be loaded on different carriers by several modifications to improve its dispersibility. Both malachite green (MG) and rhodamine B (RhB) are harmful dyes that are highly teratogenic and carcinogenic, and have side effects when absorbed by humans (Sriram et al., 2022). Abdi and Abedini, 2020 synthesized a novel polymeric nanocomposite PES/ZIF-8/ZIF-67 microspheres based on ZIFs for the removal of MG from colored wastewater, which show an excellent adsorption capacity of 613.2 mg/g of MG. Mahmoodi et al. (2020) similarly designed and synthesized a ZIF-8-based composite material and prepared ZIF8@CS/PVA-ENF nanofibers by encapsulating ZIF-8 crystals on chitosan/polyvinyl alcohol electrospun nanofibers (CS/PVA-ENF), exhibiting higher adsorption performance on MG with adsorption capacity of 1,000 mg/g and excellent chemical stability (Figure 3).
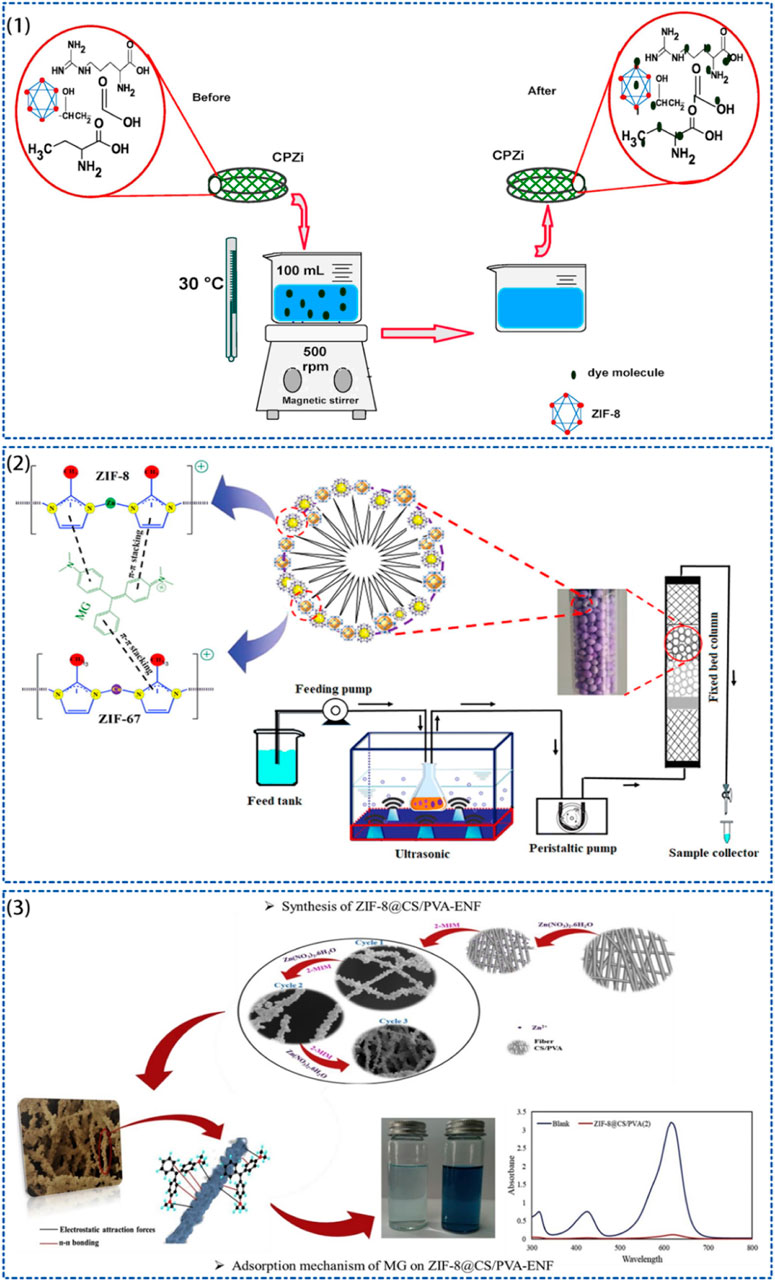
FIGURE 3. Schematic diagram of the process of MG adsorption by ZIF-8-based composites. (1) ZIF-8/CS/PVA composite membrane adsorbed MG with the maximum adsorption amount of 482.48 mg/g (Khajavian et al., 2020). (2) PES/ZIF-8/ZIF-67 microspheres adsorbed MG with the maximum adsorption amount of 613.2 mg/g (Abdi and Abedini, 2020). (3) ZIF-8@CS/PVA-ENF nanofibers adsorbed MG with the maximum adsorption amount was 1,000 mg/g (Mahmoodi et al., 2020).
3.1.1.2 Adsorption of biological contamination by Zn-based MOFs
Mycotoxins are secondary metabolites produced by fungal growth in food and feed, which are highly toxic and carcinogenic, resistant to high temperatures, chemically stable, and difficult to remove during general food processing. Han et al. (2020) synthesized a new Zn-based composite MOFs (Fe3O4@AMP&ZnCl2@McAbs) for multi-target adsorb deoxynivalenol (DON) (also called vomitoxin), zearalenone (ZEA), and T-2 and HT-2 toxins produced by Fusarium oxysporum. The results showed that the maximum adsorption amounts of Fe3O4@AMP&ZnCl2@McAbs per 100 mg composite were DON 688.26 ng, ZEN 864.98 ng, T-2 1,272.06 ng, HT-2 1,529.74 ng, respectively, and the recovery of the four mycotoxins decreased with the increase of the number of cycles. However, the decrease was insignificant, indicating the composites’ stability to some extent. The strong chemical and thermal stability of aflatoxin B1 (AFB1) make it more difficult to be removed from practical applications. Using reactive oxygen species with high reactivity is considered as an effective means to eliminate AFB1. Recently, Zhang X et al. (2022) developed a porous carbon material based on Fe-ZIF-8 with multiple active sites providing catalytic activity. The material can generate a large amount of highly reactive oxygen species to destroy or inactivate terminal furan ring and methoxy double bond of AFB1. Meanwhile, the large specific surface area and multi-stage porous structure of Fe-ZIF-8-PC achieve high exposure of active sites and fast mass transfer rate of reactants, enabling an excellent adsorption performance to AFB1.
3.1.2 Zn-based MOFs as sensors for food contamination detection
3.1.2.1 Detection of chemical contamination by Zn-based MOFs
Luminescent metal-organic frameworks (LMOFs) have received increasing attention in sensing (William et al., 2017). Zn-based MOFs are commonly designed as fluorescent sensors for food detection applications to detect food quality and safety (Magri et al., 2021). Pang et al. (2022) created a novel fluorescent sensor with a zinc-based porphyrin metal-organic framework (Zn-TCPP-MOF) for the ultrasensitive and quantitative detection of bisphenol A (BPA). Zn-TCPP-MOF exhibits a luminescent band in the wavelength range of 500–800 nm, probably due to ligand-to-metal charge transfer (LMCT). Meanwhile, the fluorescence spectrum of Zn-TCPP-MOF was blue-shifted compared with that of TCPP, mainly because the formation of coordination bonds between zinc ions and TCPP reduced the free electrons of porphyrin molecules, thereby increasing the lowest excited state energy level. The experiment showed that the detection limit of Zn-TCPP-MOF for BPA was 0.906 nM (0.002 mg/L), about 5 times the detection limit of GB 31604.10–2016 of the national standard for food safety in China. ZIF-8 has recently shown unique advantages in improving the suitability of enzymes. In Figure 4, Guo et al. (2021) prepared a zeolitic imidazole framework (AuNCs/β-Gal/GOx@ZIF-8) containing bovine serum albumin (BSA)-immobilized gold nanoclusters (AuNCs), β-galactosidase (β-Gal) and glucose oxidase (GOx) for detected lactose to improve the quenching rate of fluorescence AuNCs. Since AuNCs are only 0.8 nm in size and have BSA on their surface, BSA-immobilized AuNCs can be co-encapsulated with β-Gal and GOx in ZIF-8 at room temperature. When lactose is in the system, lactose is first decomposed into galactose and glucose by β-Gal, and glucose is decomposed into gluconic acid and hydrogen peroxide (H2O2) by GOx in the presence of O2 in the air. The reaction between H2O2 and Fe2+ generates hydroxyl radicals, leading to the fluorescence quenching of AuNCs. Based on the above principle, the detection of lactose content in lactose-free milk had a detection limit of 41.67 μM at a signal-to-noise ratio of 3, which was much lower than the threshold value of lactose-free milk samples (5 g/L, 15 mM). As the long-term storage stability of enzymes is crucial for the utility of the sensor, the authors stored AuNCs/β-Gal/GOx at ZIF-8 and the free enzyme system (AuNCs/β-Gal/GOx) for 3 days, and found that the relative activity was 76.83% and 5.30% of the initial total activity, respectively, indicating that the ZIF-8 framework material established a tremendous digestive enzyme tolerance. Meanwhile, the quenching plastic rate of AuNCs/β-Gal/GOx@ZIF-8 fluorescence by lactose was increased to 3.4 times that of the free enzyme system.
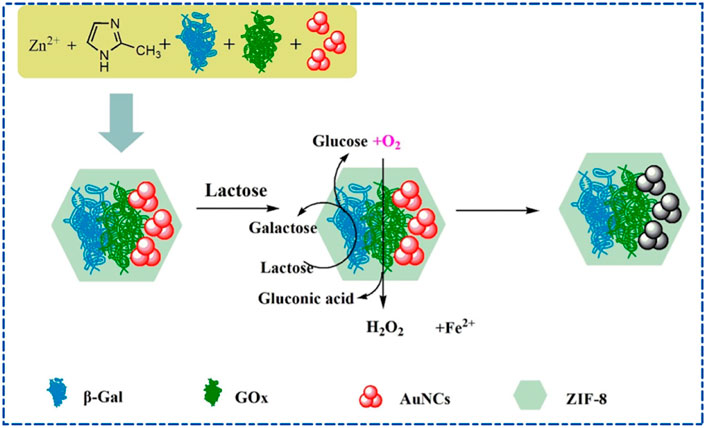
FIGURE 4. Synthesis method of AuNCs/β-Gal/GOx@ZIF-8 and measurement principle of lactose sensor (Guo et al., 2021).
3.1.2.2 Detection of biogenic contamination by Zn-based MOFs
In a previous study (Zhong et al., 2019), the authors prepared a sandwich-like electrochemical immunosensor using CdS@ZIF-8 as a novel signal probe for the ultrasensitive detection of E. coli O157:H7. In this system, cadmium sulfide quantum dots (CdS QDs) were encapsulated in ZIF-8 to construct a CdS@ZIF-8 complex with a core-shell structure. To specifically recognize O157:H7 cells, CdS@ZIF-8 particles introduced amino groups on the surface by wrapping them with polyethyleneimine (PEI) to enhance the interaction with antibodies. The biosensor had a linear range of 10–108 CFU/mL for O157:H7 detection and a detection limit of 3 CFU/mL (S/N = 3). The sensitivity of the proposed method was 16 times higher than that of the immunosensor using only CdS quantum dots as signal tags. The detection efficiency of the CdS@ZIF-8@PEI sensor was tested by spiking O157:H7 in milk. The results showed recoveries in the range of 94.3%–104.8%, indicating that the biosensor can be used to detect E. coli O157:H7 in actual samples.
Tables 1, 2 summarizes the latest research results on Zn-based MOFs for the adsorption and detection of food contaminants. With the mature development of Zn-based MOFs materials, the potential to select this material as a primary material for development is also highlighted. As ZIF-8 has a wide range of modification conditions, there is a preference for the modification and processing based on this material. From some of the studies summarized above, it can be found that Zn-based MOFs materials are excellent materials for the adsorption and detection of food contaminants due to their low LOD, high adsorption capacity, high recovery value and high detection accuracy.
3.2 Application of Cu-based MOFs in food contamination adsorption and detection
Copper is a variable valence metal in transition metals, which is relatively present in large quantities and cheaper than other transition metals. Copper ions have Lewis acidity and strong electrophilic capacity. Generally, Cu-based MOFs have a large specific surface area, diverse structure, and unsaturated coordination metal centers, which greatly enhance their application in electrochemistry (Chen X et al., 2022). One of the most well-characterized and widely studied MOF materials to date is HKUST-1 (HKUST is an acronym for the Hong Kong University of Science and Technology, also known as MOF-199, CuBTC), a Cu-based MOF. It is composed of Cu ions and organic ligand benzenetricarboxylate, which has been extensively studied due to its relative ease of synthesis, excellent thermal stability and good moisture stability (Kim et al., 2012).
3.2.1 Cu-based MOFs as adsorption materials for food contamination
3.2.1.1 Adsorption of chemical contamination by Cu-based MOFs
Food packaging and cooking processes can introduce heavy metals into food (Wu et al., 2021), and heavy metals such as arsenic (As), mercury (Hg), lead (Pb), and cadmium (Cd) can damage human health even at trace level. Song et al. (2021) designed an adsorbent for the detection of trace amounts of total mercury in rice using the strong adsorption capacity of MOFs. Copper hydroxyphosphate (CHP) was used as a source of metal ions for the in situ synthesis of DMP-Cu at room temperature, and the modification of 2,5-dimercapto-1,3,4-thiadiazole (DMTZ) was found to increase the stability and effectiveness of the complex MOFs. The pH of the solution is one of the most critical factors affecting the adsorption performance. Mercury exists as Hg2+ when the pH is less than three and as Hg(OH)2 when the pH is more significant than 6.47. Both forms of mercury exist simultaneously in the pH range of 3–6.47 (Chandra and Kim, 2011). Therefore, the authors designed experiments to test the adsorption of the composite MOFs materials on mercury at pH 2–8, respectively. It has been found that the adsorption amount appeared to increase and then decrease. This was due to the presence of H+ and OH− ions interfering with the adsorption process, and further comparison showed that the adsorption of mercury by the modified DMP-Cu increased significantly, with maximum adsorption of 249.5 mg/g at a pH value of 4. The LOD was 0.0125 ng/mL by the detection of actual samples of rice, which is well below the detection limit of Hg2+ concentration in the standard. In addition, the recoveries of Hg in rice samples ranged from 98.8% to 109%, proving the feasibility of the method.
3.2.1.2 Adsorption of biological contaminants by Cu-based MOFs
At present, few MOFs are directly used as adsorbents for biological contaminants, possibly due to a weaker interaction between MOFs materials and biological contaminants. Carbon nanomaterials are more frequently reported due to a stronger p-π interactions with biogenic contaminants. It is well known that MOFs can be used as precursors to prepare porous carbon materials by simple carbonization. Some porous carbon materials derived from MOFs not only retain some excellent original properties of MOFs, but can also promote the adsorption capacity of biological contaminants. Recently, Ma et al. (2021) prepared a series of porous carbon materials (C-Cu-BTC) based on Cu-BTC for the adsorption of AFB1 from vegetable oil. The C-Cu-BTC exhibited excellent adsorption performance of AFB1 due to its enough active sites, diverse pore structure, and large specific surface area. It was found that more than 90% of AFB1 in vegetable oil could be removed within 30 min. The adsorption of AFB1 in water by the material reached 16.6667 mg/g through kinetic simulation, and the result was much higher than other reported materials.
3.2.2 Cu-based MOFs as sensors for food contamination detection
3.2.2.1 Detection of chemical contamination by Cu-based MOFs
Nanoscale MOFs are promising for research in electrochemical sensing. 2D MOFs exhibiting a highly ordered, pore-like arrangement at the two-dimensional scale have a larger specific surface area to facilitate the immobilization of enzymes, signal molecules, or catalysts, and can be used to achieve signal amplification (Qin et al., 2018). This signal amplification strategy was recently achieved by Ma J et al. (2022) using a combination of 2D MOFs (Cu-TCPP MOFs) and G-quadruplex and DNA nanomotors. In this report, a self-cleaning electrochemical sensor with high sensitivity and selectivity was presented for the cyclic detection of Pb2+ ions. This sensor detected Pb2+ in the linear range between 5 nM and 5 μM with a detection limit of 1.7 nM, and it can selectively detect Pb2+ in the presence of other metal ions. Electrochemical sensors are divided into label-free and labeled technologies. The labeled technologies use enzymes, metal nanoparticles, small molecule oxidation reducers, and metal ions as signal tags to greatly amplify the electrochemical signal. However, there are some drawbacks, for instance, the need for complex pre-enrichment steps (Zhang et al., 2009) and the vulnerability to the activity and stability of the signal tag (Liu et al., 2016). To this end, Zhang X X et al. (2020) developed a signal label-free electrochemical sensor based on Cu-MOFs material for determining Hg2+ in dairy products. The sensing assay was based on the specific recognition between Hg2+ and T-rich DNA strands to form a stable T-Hg2+-T coordination chemistry, which effectively eliminates the interference of the sample matrix and substantially improves the accuracy of the assay results. The presence of a large amount of Cu2+ in the probe eliminated the need for a complex metal pre-enrichment process. During the experiment, it was found that there was no significant effect on the Hg2+ signal response when interfering ions were introduced.
3.2.2.2 Detection of biogenic contamination by Cu-based MOFs
Wang S et al. (2017) developed a simple, sensitive, and selective colorimetric assay for S. aureus based on Cu-MOF. Cu-MOF nanoparticles have been shown to possess peroxidase-like activity and can catalyze the yellow color development reaction of 3.3′,5.5′-tetramethylbenzidine (TMB) in the presence of H2O2. Furthermore, the presence of abundant amine groups on the surface of Cu-MOF nanoparticles allows for a simple modification of the S. aureus aptamer, therefore ensuring the selective recognition of S. aureus by Cu-MOF nanoparticles. By combining the Cu-MOF-catalyzed chromogenic reaction with aptamer recognition and magnetic separation, the detection limit for S. aureus detection was 20 CFU/mL. Recently, Sun et al. (2021) also designed an electrochemical biosensor for S. aureus detection using Cu-MOF, which reduced the detection limit of S. aureus to 5.2 CFU/mL (Figure 5).
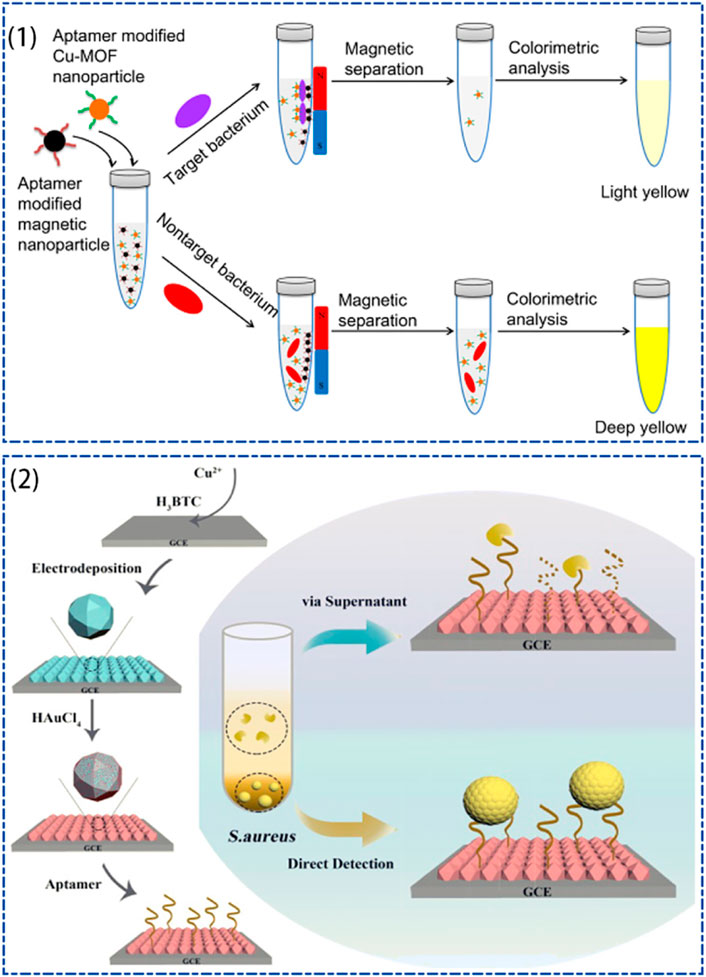
FIGURE 5. Schematic diagram of Cu-MOF-based for the detection of S. aureus, (1) schematic diagram of aptamer-modified Cu-MOF for colorimetric detection of S. aureus (Wang S et al., 2017), (2) schematic diagram of DNA/AuNPs/Cu-MOFs electrochemical sensing platform preparation and S. aureus detection (Sun et al., 2021).
The potential value of Cu-based MOFs for the adsorption and detection of food contaminants has yet to be fully explored. From the data we have collected (Table 3), there are relatively few studies on Cu-based MOFs as adsorbent materials for food contamination. Cu-based MOFs are mostly electrochemically sensed when used as sensors, probably due to the redox properties of Cu ions being used primarily for electrochemical sensing (Table 4). Furthermore, according to a related report (Liang et al., 2022), the antibacterial properties of Cu ions should not be overlooked and need to be further developed and utilized in food packaging and additives.
3.3 Application of Zr-based MOFs in food contamination adsorption and detection
Zr-based MOFs generally have better mechanical stability because they have high connectivity of Zr group clusters and strong Zr-O coordination bonds in the framework (Kong and Li, 2021). Zr-based MOFs mainly refer to carboxylic acid Zr-based MOFs, which exhibit better water stability than other reported MOFs. The main feature of Zr-MOFs is the high valence state of the Zr(IV) cation, which gives a strong affinity between Zr(IV) and carboxylic acid O (Xia et al., 2021). UiO-66 was first synthesized by Cavka et al. (2008), and this MOF consists of a Zr4+ node provided by Zr6O4(OH)4 and a 1,4-benzenedicarboxylic acid ligand. Its thermal, mechanical and hydrolytic stability all up to 450°C is mainly attributed to the stable coordination bond between Zr4+ and the carboxylate ligand.
3.3.1 Zr-based MOFs as adsorption materials for food contamination
3.3.1.1 Adsorption of chemical contamination by Zr-based MOFs
Zr-MOFs have high chemical, thermal and mechanical stability relative to other materials and are widely used in practical applications. The stability of Zr-MOFs can be attributed to the oxygenophilic nature of the Zr6 clusters and Coulombic gravitational force between these Zr cationic clusters and the anionic ligands (Ahn et al., 2016). UiO-66 as Zr-based MOF has been widely investigated by researchers for its excellent properties, high chemical and thermal stability, and diverse modification modifications (e.g., -NH2 (Tang et al., 2021), -NO2 (Wang et al., 2020), -OH (Sun et al., 2020)). Wang et al. (2022) developed a MOF-ELISA assay for a rapid detection of sulfadimethoxine (SM2) in milk (Figure 6). The saturation adsorption of SM2 by UiO-66-NH2 was found to be 139.64 mg/g at 298K, which was much higher than that of the other two MOFs materials, and reached 178.80 mg/g when the temperature was increased to 318 K. A close correlation was observed between MOF-ELISA and LC-MS/MS (R2>0.99). Adsorption kinetic study revealed that the adsorption kinetic model of MOFs was more consistent with pseudo-second-order adsorption kinetics, indicating that the rate-limiting step of the adsorption process was chemisorption (Chen et al., 2020). There were two patterns for MOFs to adsorb SM2. The adsorption of SM2 in MOFs could be affected by both the internal diffusion of the crystal particles and the interaction of the material boundary layer with SM2 (Wu et al., 2019). The slope of the pre-sorption curve larger than that of the mid-and late-sorption curve indicated that MOFs had sufficient adsorption sites in the early stage of adsorption. When the adsorption entered the second stage, the active sites of MOFs were gradually occupied, and the adsorption rate started to decrease (the slope of the adsorption curve was smaller than that of the first stage). When the adsorption equilibrium was reached, the adsorption capacity no longer varied with the adsorption time, indicating that the internal pore structure had a significant effect on the adsorption of SM2.
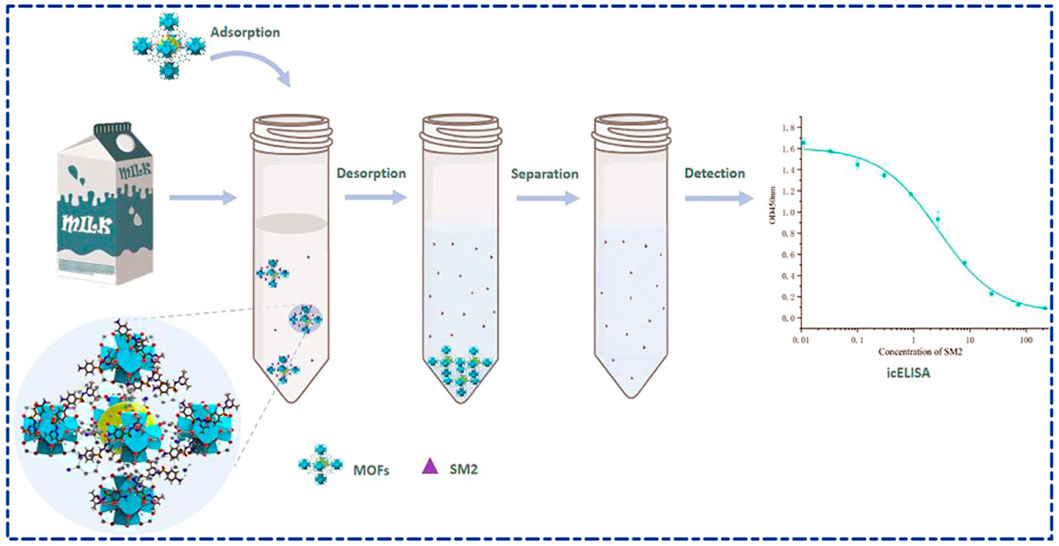
FIGURE 6. Schematic diagram of ELISA detection of SM2 adsorbed by MOFs in milk (Wang et al., 2022).
In addition to the detection of veterinary drug residues in food, Zr-based MOFs also have a great adsorption ability for heavy metal contaminants. Hu et al. (2022a) proposed a composite MOFs material (CS-UiO-66-NH2) using chitosan (CS) modified UiO-66-NH2, which solved the problem of agglomeration of UiO-66-NH2 in practical applications and increased its contact area with heavy metal ions, achieving a stronger adsorption capacity. The UiO-66-NH2 attached to the main chain of CS by covalent bonding did not reduce the active adsorption sites but noticeably improved the dispersibility and preserved the morphology of the MOFs as much as possible. The adsorption performance of Pb(II) showed that the maximum adsorption capacity reached 555.56 mg/g. After five cycles, the adsorption capacity still reached 74.8% of the first adsorption capacity, indicating that the composite MOFs material had an excellent reusability. In another study, Hu et al. (2022b) modified UiO-66-NH2 with polyethyleneimine (PEI), and the prepared composite PEI@UiO-66-NH2 exhibited even better adsorption performance for Pb(II) (Figure 7).
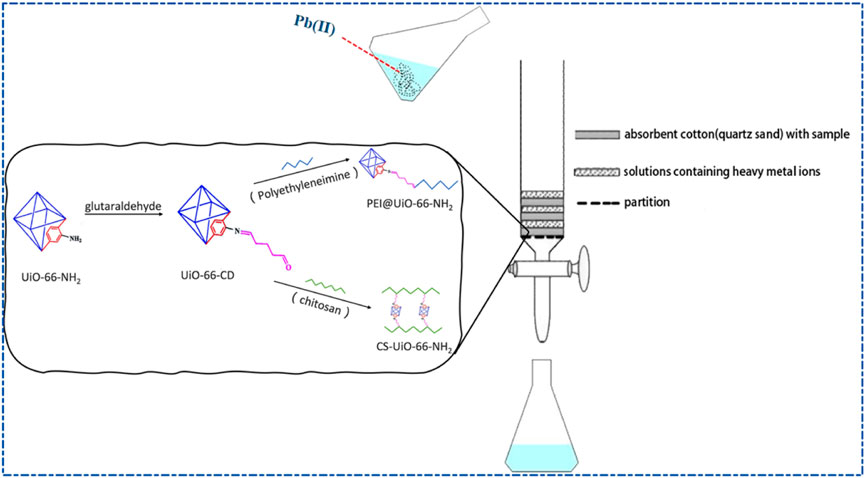
FIGURE 7. Schematic diagram of the preparation process and removal of Pb(II) from wastewater for CS-UiO-66-NH2 and PEI@UiO-66-NH2.
3.3.1.2 Adsorption of biological contamination by Zr-based MOFs
Most conventional MOFs are difficult to be biologically active as a chemical material. Recently, Wei et al. (2022) used the graded porous properties of MOFs to synthesize PCN-222 with peroxidase-like activity, which derives its biological activity from Fe-TCPP (TCPP = tetrakis (4-carboxyphenyl) porphyrin), and the peroxidase-like activity can be further enhanced by loading Pd nanoparticles onto PCN-222. The composite material was used for the adsorption and removal of AFB1 from edible oil, with an adsorption and removal rate of 96.52% by testing. Deoxynivalenol (DON) is difficult to enter the pore interior of MOFs because of large spatial site resistance of molecules. Cheng et al. (2021) designed and developed a stable adsorbent material BUT-16 based on cage-like Zr(IV)-MOFs with high performance, and found that DON molecules could interact with Zr6 clusters in BUT-16 to form a large number of hydrogen bonds. However, BUT-17, which has the same spatial configuration, has a tiny difference between the ligands used to build MOFs (ligand H4CPTTA for building BUT-17 has a benzene ring at its center, and ligand H4BCPIA for building BUT-16 has a pyridine ring at its center). As a result, the O atom in the adsorbed DON molecule can only form a hydrogen bond with the H atom on the ligand in BUT-17, therefore showing a tremendous difference in the adsorption. Meanwhile, such a slight difference in the ligands made the angle of the planar rings in the ligands different and allowed the DON molecules to enter the Zr6 cluster in BUT-16 more easily. By testing in water samples, BUT-16 demonstrated a high adsorption capacity of 46 mg/g for DON molecules.
3.3.2 Zr-based MOFs as sensors for food contamination detection
3.3.2.1 Detection of chemical contamination by Zr-based MOFs
Based on molecular imprinting technique, Amiripour et al. (2021) prepared a fluorescent sensor (MIP/Zr-MOF) consisting of molecularly imprinted polymers (MIPs) wrapped with Zr-based MOFs for detecting chloramphenicol (CAP) in milk and honey. Zr-LMOFs have specific binding sites for CAP recognition and therefore a high selectivity and sensitivity to CPA when encapsulated by MIPs. For the detection of CAP in real milk and honey samples, the linear range was 0.16–161.56 μg/L, and the LOD was 0.013 μg/L. Meanwhile, the recoveries measured by this method were in the range of 96%–105%, which generally in accordance with the recoveries by LC-MS/MS (95%–105%). This indicated that the functionalized LMOFs can be well applied to the analysis of CAP residues in food safety assessment. In order to detect organophosphorus pesticide residues, Gao et al. (2021) designed a novel non-enzymatic electrochemical sensor with terephthalic acid as the ligand and Zr as the metal ion in combination with electroreduced graphene oxide (rGO). The Zr-OH group in the Zr-BDC-rGO composite showed a high affinity for phosphate groups, enabling it to selectively recognize methyl parathion (MP) and have a specific adsorption capacity. The sensor was tested to have a wide linear range from 0.001–3.0 μg/mL, and the detection limit for MP was as low as 0.5 ng/mL.
3.3.2.2 Detection of Zr-based MOFs against biogenic contamination
Li Z et al. (2019) developed a novel water-stable luminescent metal-organic framework material, Zr-LMOFs (Zr-CAU-24). Due to the intense blue fluorescence of Zr-CAU-24, the sensitive and rapid detection of AFB1 in water without antibodies or biomolecular modifications was achieved. Using this porous nanomaterial, the detection limit of AFB1 in walnut and almond beverages was as low as 19.97 ppb (64 nM) with a high recovery (91%–108%). The structural stability of the Zr6 ion cluster in water plays a crucial role in mycotoxin detection. Li Z et al. (2021) recently reported a composite sponge material based on Zr-LMOFs, which was loaded on a melamine (MF) sponge using in situ hydrothermal synthesis. Such a bifunctional hybrid sponge not only retained the great mechanical processing properties of MF sponge, but also had a high tolerance to large deformation. At the same time, the pore structure of the preserved Zr-LMOFs crystals allowed the guest molecules to enter well inside the cavity, enabling the detection of AFB1 with a relatively high sensitivity (LOD = 1.6 ppb) and a wide linear range of 0.1–50 μM. It was further found that the analyte-sensor interaction played a crucial role in the efficient quenching. Moreover, AFB1 was rapidly enriched in the cavity of Zr-LMOF, producing stronger analyte-sensor interaction and higher-order π-π interaction, which could accelerate the electron transport and generate a greater quenching effect for sensitive detection. Therefore, based on this property, it is feasible to develop hybrid materials based on Zr-LMOF for rapid detection and removal of toxic and hazardous substances.
The Zr6 clusters of Zr-based MOFs give them high chemical, thermal and mechanical stability compared to other materials. As can be seen from Tables 5, 6, research for chemical and biological contamination applications is prevalent. High sensitivity and a wide linear detection range are achieved by compounding with different functional materials. At the same time, continuously optimized materials have improved the adsorption capacity for contaminants. In particular, the use of bioactive substances to modify MOFs makes them well suited for the adsorption and detection of biological contamination.
3.4 Application of other MOF materials in food contamination adsorption and detection
In addition to the several metal-based MOFs mentioned above, many other MOFs materials have been investigated for the adsorption and detection of food contamination. Among them, Co-based MOFs are fast developing and have been gradually applied to food detection. Zhang X et al. (2022) found that Co-based MOFs exhibited good stability under alkaline conditions and could be utilized as bio-inspired nanoenzymes, which in turn led to the successful development of a portable detector that could be used to detect acetamiprid residues. Liu et al. (2019) developed an ultra-sensitive and highly selective Co-based MOF and nitrogen-rich COFs for the detection of the antibiotic ampicillin (AMP) using the electrochemical sensing platform Co-MOF@COFs, which has great potential for food safety applications. Fu et al. (2022) developed an Al-based MOF probe that uses the fluorescence signal and ratio of the scattered intensity signal for efficient and sensitive detection of ciprofloxacin (CIP). Since NH2-MIL-53 is a nanoscale particle, it has both fluorescence and second-order scattering signals. The intrinsic fluorescence signal of CIP overlaps with NH2-MIL-53, which enhances the background signal and is not conducive to detection. Therefore, the authors modified it with 8-hydroxy-2-quinolinecarboxaldehyde (HQCA), and the modified HQCA-MIL-53 showed almost no fluorescence properties compared to NH2-MIL-53, but still exhibited scattering properties. Ghiasi et al. (2022) prepared an aptamer functionalized material based on magnetic CoFe2O4@SiO2@MIL-101(Cr)-NH2 for magnetic solid phase adsorption (MSPE) of pesticide residue acetamiprid from fruit juice. The adsorbent utilized the synergistic effects of the high specific surface area of MIL-101(Cr), magnetic properties of CoFe2O4, and specific molecular recognition of the aptamer, and showed a strong performance in the extraction of aminopyralid with a maximum adsorption capacity of 3.5 mg/g. In recent years, cyclodextrin metal-organic frameworks (CD-MOFs) based on cyclodextrin (CD) have been widely investigated not only for their typical edible, regenerative, and biodegradable properties, but also for their large surface area, controllable pore size, and high adsorption properties on gases. Majd et al. (2022) successfully prepared a cross-linked polypropionitrile/γ-cyclodextrin-metal-organic framework (PAN/γ-CD-MOF) electrospun nanofibers based on CD-MOFs, which were used as an efficient adsorbent for extracting multiple herbicides from cereal samples prior to HPLC-UV analysis. This method was effectively applied for trace analysis of herbicides in wheat, rice, and barley samples. In addition, CD-MOFs were also used for sensing assays. Recently, Chen Y et al. (2022) proposed a novel sensor for determinating luteolin (3′, 4′, 5, 7-tetrahydroxyflavonoids, LU) by preparing a glassy carbon electrode (MoO3-PEDOT/CD-MOF/GCE) using γ-CD-MOF, MoO3 and a flexible conducting polymer poly (3,4-ethylenedioxythiophene) (PEDOT). The in situ growth of PEDOT on MoO3 nanorods effectively improves the stability, dispersion, and electrical conduction efficiency of PEDOT. Meanwhile, the cavities in CD-MOFs can absorb a large amount of LU and increase the local concentration of LU on the electrode surface, which further amplifies the current signal. Under the optimal conditions, the sensor exhibits a wide linear range of 0.4 nM–1800 nM and a low detection limit of 0.1 nM (S/N = 3).
4 Conclusion
In summary, MOFs have shown great potential for the adsorption and detection of food contaminants. This paper highlights recent insightful research results on the adsorption and detection of food contaminants by Zn-based, Cu-based and Zr-based MOFs, describes how they work and discusses their application in the adsorption and sensing detection of chemical and biological contaminants in terms of stability, adsorption capacity and sensitivity. Despite the many advances that have been made, there are still many challenges in their practical application. Firstly, although MOFs materials can be synthesized with different pore sizes depending on the length of the organic ligand and the size of the metal ionophore, most MOFs studied so far are more suitable for the adsorption of small molecules (e.g. heavy metals and other chemical contaminants) and have bulk rejection of large molecules, so precise control of pore size and volume for specific applications (including adsorption performance and sensing selectivity) is still to be development. Secondly, the functional versatility, structural flexibility and large specific surface area of MOF materials are their greatest advantages as adsorbent materials, compared to commercially available materials such as zeolites and activated carbon. However, some MOF materials still lack stability in aqueous solutions, which is an urgent issue in the development and utilization of MOFs. The stability of MOFs can be improved by compounding with different stabilizing materials. Furthermore, an important limitation of MOFs is the use of non-renewable raw materials for their synthesis, but this problem has recently been solved by the manufacture and use of γ-cyclodextrin-based MOFs. Currently, γ-cyclodextrin-MOFs are being developed as a new green and edible MOF and will be considered for future research in food packaging and food additives. Finally, some of the already commercialized MOFs (e.g. ZIF-8, UiO-66, HKUST-1) can be developed for specific applications depending on the metal ion. Zn-based and Zr-based MOFs materials have been widely employed in the detection of chemical contamination because of their stable skeleton structure as well as in fluorescence detection and analysis. Due to the redox reaction of Cu2+/Cu, Cu-based MOFs are electrochemically active, and the large number of electroactive sites in Cu-MOFs facilitates signal amplification, so they are used more in electrochemical sensors. Meanwhile, Cu ions have been studied more in food preservation due to their broad-spectrum antibacterial activity, such as loading mesoporous HKUST-1 on chitosan quaternary ammonium salt films to achieve good ethylene adsorption and antibacterial capacity (Nian et al., 2022). It can be seen that MOFs are a promising research material that can play a valuable role in food contamination adsorption and detection through targeted modifications and functionalization, thus enhancing food safety for rapid and accurate risk assessment.
Author contributions
QW and XG designed the article. LlW, LjW, and LB searched for primary publications and screened and appraised prior data. XG and QW extracted the data and wrote the manuscript. LlW and LB edited the manuscript. All authors read the manuscript and participated in preparing the final version of the manuscript. All authors read and approved the final manuscript.
Funding
This work was supported by the National Natural Science Foundation of China (No. 81973003), the Military Logistics Science and Technology Program Youth Incubation Project (13QNP161) and the Army Logistics Research Plan of China (AEP14C001).
Conflict of Interest
The authors declare that the research was conducted in the absence of any commercial or financial relationships that could be construed as a potential conflict of interest.
Publisher’s note
All claims expressed in this article are solely those of the authors and do not necessarily represent those of their affiliated organizations, or those of the publisher, the editors and the reviewers. Any product that may be evaluated in this article, or claim that may be made by its manufacturer, is not guaranteed or endorsed by the publisher.
References
Abdi, J., and Abedini, H. (2020). MOF-based polymeric nanocomposite beads as an efficient adsorbent for wastewater treatment in batch and continuous systems: Modelling and experiment. Chem. Eng. J. 400, 125862. doi:10.1016/j.cej.2020.125862
Ahn, S., Thornburg, N. E., Li, Z., Wang, T. C., Gallington, L. C., Chapman, K. W., et al. (2016). Stable Metal-Organic Framework-Supported Niobium Catalysts. Inorg. Chem. 55 (22), 11954–11961. doi:10.1021/acs.inorgchem.6b02103
Allendorf, M. D., Bauer, C. A., Bhakta, R. K., and Houk, R. J. T. (2009). Luminescent metal-organic frameworks. Chem. Soc. Rev. 38 (5), 1330–1352. doi:10.1039/B802352M
Amiripour, F., Ghasemi, S., and Azizi, S. N. (2021). Design of turn-on luminescent sensor based on nanostructured molecularly imprinted polymer-coated zirconium metal-organic framework for selective detection of chloramphenicol residues in milk and honey. Food Chem 347, 129034. doi:10.1016/j.foodchem.2021.129034
Awad, F. S., Bakry, A. M., Ibrahim, A. A., Lin, A., and El-Shall, M. S. (2021). Thiol- and Amine-Incorporated UIO-66-NH2 as an Efficient Adsorbent for the Removal of Mercury(II) and Phosphate Ions from Aqueous Solutions. Ind. Eng. Chem. Res. 60 (34), 12675–12688. doi:10.1021/acs.iecr.1c01892
Bai, Y., Dou, Y., Xie, L.H., Rutledge, W., Li, J.R., and Zhou, H.C. (2016). Zr-based metal-organic frameworks: Design, synthesis, structure, and applications. Chem. Soc. Rev. 45 (8), 2327–2367. doi:10.1039/C5CS00837A
Bahrani, S., Hashemi, S. A., Mousavi, S. M., and Azhdari, R. (2019). Zinc-based metal–organic frameworks as nontoxic and biodegradable platforms for biomedical applications: review study. Drug Metab. Rev. 51 (3), 356–377. doi:10.1080/03602532.2019.1632887
Bazargan, M., Ghaemi, F., Amiri, A., and Mirzaei, M. (2021). Metal–organic framework-based sorbents in analytical sample preparation. Coordin. Chem. Rev. 445, 214107. doi:10.1016/j.ccr.2021.214107
Bhardwaj, N., Bhardwaj, S. K., Mehta, J., Nayak, M. K., and Deep, A. (2016). Bacteriophage conjugated IRMOF-3 as a novel opto-sensor for S. arlettae. New J. Chem. 40 (9), 8068–8073. doi:10.1039/c6nj00899b
Burtch, N. C., Jasuja, H., and Walton, K. S. (2014). Water Stability and Adsorption in Metal-Organic Frameworks. Chem. Rev. 114 (20), 10575–10612. doi:10.1021/cr5002589
Caroline, S., Pieter, S., Shyam, B., Karen, L., and Pascal, V. (2015). Comparison of different solid adsorbents for the removal of mobile pesticides from aqueous solutions. Adsorption 21 (3), 243–254. doi:10.1007/s10450-015-9666-8
Cavka, J., Jakobsen, S., Olsbye, U., Guillou, N., Lamberti, C., Bordiga, S., and Lillerud, K. (2008). A new zirconium inorganic building brick forming metal organic frameworks with exceptional stability. J. Am. Chem. Soc. 130 (42), 13850–13851. doi:10.1021/ja8057953
Chandra, V., and Kim, K. S. (2011). Highly selective adsorption of Hg2+ by a polypyrrole–reduced graphene oxide composite. Chem. Commun(Camb). 47 (13), 3942–3944. doi:10.1039/C1CC00005E
Chen, P., He, X., Pang, M., Dong, X., Zhao, S., and Zhang, W. (2020). Iodine Capture Using Zr-Based Metal-Organic Frameworks (Zr-MOFs): Adsorption Performance and Mechanism. ACS Appl. Mater. Inter. 12 (18), 20429–20439. doi:10.1021/acsami.0c02129
Chen, X., Li, J., Li, J., Zhang, L., Zhao, P., Wang, C., et al. (2022). Determination of luteolin in Chrysanthemum tea with a ultra-sensitive electrochemical sensor based on MoO3/poly(3, 4-ethylene dioxythiophene)/gama-cyclodextrin metal-organic framework composites. Food Chem 397, 133723. doi:10.1016/j.foodchem.2022.133723
Chen, Y., Zhang, Y., Huang, Q., Lin, X., Zeb, A., Wu, Y., et al. (2022). Recent Advances in Cu-Based Metal–Organic Frameworks and Their Derivatives for Battery Applications. ACS Appl. Energy Mater. 5 (6), 7842–7873. doi:10.1021/acsaem.2c01405
Cheng, J., Wang, B., Lv, J., Wang, R., Du, Q., Liu, J., et al. (2021). Remarkable Uptake of Deoxynivalenol in Stable Metal-Organic Frameworks. ACS Appl. Mater. Inter. 13 (48), 58019–58026. doi:10.1021/acsami.1c19501
Chinnapaiyan, S., Umamaheswari, R., Chen, S., Liu, T., De Oliveira Filho, J., and Chang, Y. (2022). Fabrication of thulium metal–organic frameworks based smartphone sensor towards arsenical feed additive drug detection: Applicable in food safety analysis. Electrochim. Acta. 401, 139487. doi:10.1016/j.electacta.2021.139487
Dai, G., and Ye, K. (2019). Editorial introduction to special issue on “Biomaterials for cell manufacturing and tissue biofabrication. Acta Biomater 95, 1–2. doi:10.1016/j.actbio.2019.08.006
Deng, H., Doonan, C., Furukawa, H., Ferreira, R., Towne, J., Knobler, C., et al. (2010). Multiple functional groups of varying ratios in metal-organic frameworks. Science 327 (5967), 846–850. doi:10.1126/science.1181761
Ding, L., Cao, Y., Li, H., Wang, F., Guo, D. Y., Yang, W., et al. (2022). A ratiometric fluorescence-scattering sensor for rapid, sensitive and selective detection of doxycycline in animal foodstuffs. Food Chem 373, 131669. doi:10.1016/j.foodchem.2021.131669
Dong, J., Zhang, D., Li, C., Bai, T., Jin, H., and Suo, Z. (2022). A sensitive electrochemical sensor based on PtNPs@Cu-MOF signal probe and DNA walker signal amplification for Pb2+ detection. Bioelectrochemistry 146, 108134. doi:10.1016/j.bioelechem.2022.108134
Duan, C., Meng, J., Wang, X., Meng, X., Sun, X., Xu, Y., er, al., et al. (2018). Synthesis of novel cellulose- based antibacterial composites of Ag nanoparticles@ metal-organic frameworks@ carboxymethylated fibers. Carbohydr. Polym. 193, 82–88. doi:10.1016/j.carbpol.2018.03.089
Duan, N., Yang, W., Wu, S., Zou, Y., and Wang, Z. (2020). A Visual and Sensitive Detection of Escherichia coli Based on Aptamer and Peroxidase-like Mimics of Copper-Metal Organic Framework Nanoparticles. Food Anal. Method. 13 (7), 1433–1441. doi:10.1007/s12161-020-01765-9
Fang, B., Hu, S., Wang, C., Yuan, M., Huang, Z., Xing, K., et al. (2019). Lateral flow immunoassays combining enrichment and colorimetry-fluorescence quantitative detection of sulfamethazine in milk based on trifunctional magnetic nanobeads. Food Cont 98, 268–273. doi:10.1016/j.foodcont.2018.11.039
Fletcher, A. J., Thomas, K. M., and Rosseinsky, M. J. (2005). Flexibility in metal-organic framework materials: Impact on sorption properties. J. Solid State Chem. 178 (8), 2491–2510. doi:10.1016/j.jssc.2005.05.019
Fu, B., Chen, J., Cao, Y., Li, H., Gao, F., Guo, D. Y., et al. (2022). Post-modified metal-organic framework as ratiometric fluorescence-scattering probe for trace ciprofloxacin residue based on competitive coordination. Sensor. Actuat. B Chem. 369, 132261. doi:10.1016/j.snb.2022.132261
Gabriela, W., Bartosz, M., Barbara, G., and Przemysław, D. (2016). Metal-organic frameworks: Mechanisms of antibacterial action and potential applications. Drug Discov. Today. 21 (6), 1009–1018. doi:10.1016/j.drudis.2016.04.009
Gao, N., Tan, R., Cai, Z., Zhao, H., Chang, G., and He, Y. (2021). A novel electrochemical sensor via Zr-based metal organic framework–graphene for pesticide detection. J. Mater. Sci. 56 (34), 19060–19074. doi:10.1007/s10853-021-06436-6
Ge, D., and Lee, H. (2011). Water stability of zeolite imidazolate framework 8 and application to porous membrane-protected micro-solid-phase extraction of polycyclic aromatic hydrocarbons from environmental water samples. J. Chromatogr. A. 1218 (47), 8490–8495. doi:10.1016/j.chroma.2011.09.077
Ghiasi, A., Malekpour, A., and Mahpishanian, S. (2022). Aptamer functionalized magnetic metal-organic framework MIL-101(Cr)-NH2 for specific extraction of acetamiprid from fruit juice and water samples. Food Chem 382, 132218. doi:10.1016/j.foodchem.2022.132218
Guo, M., Chi, J., Zhang, C., Wang, M., Liang, H., Hou, J., et al. (2021). A simple and sensitive sensor for lactose based on cascade reactions in Au nanoclusters and enzymes co-encapsulated metal-organic frameworks. Food Chem 339, 127863. doi:10.1016/j.foodchem.2020.127863
Gupta, A., Bhardwaj, S. K., Sharma, A. L., Kim, K.H., and Deep, A. (2019). Development of an advanced electrochemical biosensing platform for E. coli using hybrid metal-organic framework/polyaniline composite. Environ. Res. 171, 395–402. doi:10.1016/j.envres.2019.01.049
Gwon, K., Han, I., Lee, S., Kim, Y., and Lee, D. (2020). Novel Metal-Organic Framework-Based Photocrosslinked Hydrogel System for Efficient Antibacterial Applications. ACS Appl. Mater. Inter. 12 (18), 20234–20242. doi:10.1021/acsami.0c03187
Han, Q., Sun, Y., Ding, K., Chen, X., and Han, T. (2020). Preparation of multitarget immunomagnetic beads based on metal–organic frameworks and their application in food samples. J. Chromatogra. B. 1158, 122341. doi:10.1016/j.jchromb.2020.122341
Hashemi, B., Zohrabi, P., Raza, N., and Kim, K.H. (2017). Metal-organic frameworks as advanced sorbents for the extraction and determination of pollutants from environmental, biological, and food media. TrAC Trend. Anal. Chem. 97, 65–82. doi:10.1016/j.trac.2017.08.015
Hatamluyi, B., Rezayi, M., Beheshti, H. R., and Boroushaki, M. T. (2020). Ultra-sensitive molecularly imprinted electrochemical sensor for patulin detection based on a novel assembling strategy using Au@Cu-MOF/N-GQDs. Sensor. Actuat. B Chem. 318, 128219. doi:10.1016/j.snb.2020.128219
He, K., Li, Z., Wang, L., Fu, Y., Quan, H., Li, Y., et al. (2019). A Water-Stable Luminescent Metal-Organic Framework for Rapid and Visible Sensing of Organophosphorus Pesticides. ACS Appl. Mater. Inter. 11 (29), 26250–26260. doi:10.1021/acsami.9b06151
Hitabatuma, A., Wang, P., Su, X., and Ma, M. (2022). Metal-Organic Frameworks-Based Sensors for Food Safety. Foods 11 (3), 382. doi:10.3390/foods11030382
Hou, J., Jia, P., Yang, K., Bu, T., Zhao, S., Li, L., et al. (2022). Fluorescence and Colorimetric Dual-Mode Ratiometric Sensor Based on Zr-Tetraphenylporphyrin Tetrasulfonic Acid Hydrate Metal-Organic Frameworks for Visual Detection of Copper Ions. ACS Appl. Mater. Inter. 14 (11), 13848–13857. doi:10.1021/acsami.1c23199
Howarth, A. J., Liu, Y., Li, P., Li, Z., Wang, T. C., Hupp, J. T., et al. (2016). Chemical, thermal and mechanical stabilities of metal–organic frameworks. Nat. Rev. Mater. 1 (3), 15018. doi:10.1038/natrevmats.2015.18
Hu, H., Chen, F., Zhang, Z., Liu, D., Liang, Y., and Chen, Z. (2022). Heterometallic Metal-Organic Framework Based on [Cu4I4] and [Hf6O8] Clusters for Adsorption of Iodine. Front. Chem. 10, 864131. doi:10.3389/fchem.2022.864131
Hu, S., Huang, T., Zhang, N., Lei, Y., and Wang, Y. (2022a). Chitosan-assisted MOFs dispersion via covalent bonding interaction toward highly efficient removal of heavy metal ions from wastewater. Carbohydr. Polym. 277, 118809. doi:10.1016/j.carbpol.2021.118809
Hu, S., Huang, T., Zhang, N., Lei, Y., and Wang, Y. (2022b). Enhanced removal of lead ions and methyl orange from wastewater using polyethyleneimine grafted UiO-66-NH2 nanoparticles. Sep. Purif. Technol. 297, 121470. doi:10.1016/j.seppur.2022.121470
Hu, S., Ouyang, W., Guo, L., Lin, Z., Jiang, X., Qiu, B., et al. (2017). Facile synthesis of Fe3O4/g-C3N4/HKUST-1 composites as a novel biosensor platform for ochratoxin A. Biosens. Bioelectron. 92, 718–723. doi:10.1016/j.bios.2016.10.006
Hu, Y., Huang, Z., Liao, J., and Li, G. (2013). Chemical bonding approach for fabrication of hybrid magnetic metal-organic framework-5: High efficient adsorbents for magnetic enrichment of trace analytes. Anal. Chem. 85 (14), 6885–6893. doi:10.1021/ac4011364
Hu, Z., Lustig, W. P., Zhang, J., Zheng, C., Wang, H., Teat, S. J., et al. (2015). Effective Detection of Mycotoxins by a Highly Luminescent Metal-Organic Framework. J. Am. Chem. Soc. 137 (51), 16209–16215. doi:10.1021/jacs.5b10308
Huang, X., Lin, Y., Zhang, J., and Chen, X. (2006). Ligand-directed strategy for zeolite-type metal-organic frameworks: Zinc(II) imidazolates with unusual zeolitic topologies. Angew. Chem. Int. Ed. Engl. 45 (10), 1557–1559. doi:10.1002/anie.200503778
Huang, J., Ou, C., Lv, F., Cao, Y., Tang, H., Zhou, Y., et al. (2017). Determination of aliphatic amines in food by on-fiber derivatization solid-phase microextraction with a novel zeolitic imidazolate framework 8-coated stainless steel fiber. Talanta 165, 326–331. doi:10.1016/j.talanta.2016.12.075
Huang, Z., Liu, S., Xu, J., Yin, L., Sun, F., Zhou, N., et al. (2018). Fabrication of 8-aminocaprylic acid doped UIO-66 as sensitive solid-phase microextraction fiber for nitrosamines. Talanta 178, 629–635. doi:10.1016/j.talanta.2017.09.090
Jahangiri, F., Zare, H. R., and Shekari, Z. (2022). A Non-label Electrochemical Aptasensor Based on Cu Metal-Organic Framework to Measure Aflatoxin B1 in Wheat Flour. Food Anal. Method. 15 (1), 192–202. doi:10.1007/s12161-021-02109-x
Jalili, R., Irani-nezhad, M. H., Khataee, A., and Joo, S. W. (2021). A ratiometric fluorescent probe based on carbon dots and gold nanocluster encapsulated metal–organic framework for detection of cephalexin residues in milk. Spectrochim. Acta. A Mol. Biomol. Spectrosc. 262, 120089. doi:10.1016/j.saa.2021.120089
Javier, G.S., Bárbara, S.R., Javier, H.B., and Rodriguez-Delgado, M. A. (2016). Nanomaterials as sorbents for food sample analysis. TrAC Trend. Anal. Chem. 85, 203–220. doi:10.1016/j.trac.2016.09.009
Jia, Y., Zhou, G., Wang, X., Zhang, Y., Li, Z., Liu, P., et al. (2020). A metal-organic framework/aptamer system as a fluorescent biosensor for determination of aflatoxin B1 in food samples. Talanta 219, 121342. doi:10.1016/j.talanta.2020.121342
Jiang, X., Rao, J., Li, S., Lei, T., Bai, H., et al. (2021). Magnetic metal-organic framework (Fe3O4@ZIF-8) core-shell composite for the efficient removal of Pb(II) and Cu(II) from water. J. Environ. Chem. Eng. 9 (5), 105959. doi:10.1016/j.jece.2021.105959
Jiao, L., RuSeow, J. Y., ScottSkinner, W., Wang, Z. U., and Jiang, H. L. (2019). Metal-organic frameworks: Structures and functional applications. Mater. Today 27, 43–68. doi:10.1016/j.mattod.2018.10.038
Jin, J. C., Wang, J., Guo, J., Yan, M. H., Wang, J., Devyani, S., et al. (2023). A 3D rare cubane-like tetramer Cu(II)-based MOF with 4-fold dia topology as an efficient photocatalyst for dye degradation. Colloid. Surface A. 656, 130475. doi:10.1016/j.colsurfa.2022.130475
Khajavian, M., Salehi, E., and Vatanpour, V. (2020). Chitosan/polyvinyl alcohol thin membrane adsorbents modified with zeolitic imidazolate framework (ZIF-8) nanostructures: Batch adsorption and optimization. Sep. Purif. Technol. 241, 116759. doi:10.1016/j.seppur.2020.116759
Kim, J., Cho, H., Y., and Ahn, W., S. (2012). Synthesis and Adsorption/Catalytic Properties of the Metal Organic Framework CuBTC. Catal. Surv. Asia. 16, 106–119. doi:10.1007/s10563-012-9135-2
Kong, X. J., and Li, J. R. (2021). An Overview of Metal–Organic Frameworks for Green Chemical Engineering. Engineering 7 (8), 1115–1139. doi:10.1016/j.eng.2021.07.001
Kreno, L. E., Leong, K., Farha, O. K., Allendorf, M., Van Duyne, R. P., and Hupp, J. T. (2012). Metal–Organic Framework Materials as Chemical Sensors. Chem. Rev. 112 (2), 1105–1125. doi:10.1021/cr200324t
Lan, H., Pan, D., Sun, Y., Guo, Y., and Wu, Z. (2016). Thin metal organic frameworks coatings by cathodic electrodeposition for solid-phase microextraction and analysis of trace exogenous estrogens in milk. Anal. Chim. Acta. 937, 53–60. doi:10.1016/j.aca.2016.07.041
Lei, J., Qian, R., Ling, P., Cui, L., and Ju, H. (2014). Design and sensing applications of metal–organic framework composites. TrAC Trend. Anal. Chem. 58, 71–78. doi:10.1016/j.trac.2014.02.012
Li, H., Eddaoudi, M., O’Keeffe, M., and Yaghi, O. M. (1999). Design and synthesis of an exceptionally stable and highly porous metal-organic framework. Nature 402 (6759), 276–279. doi:10.1038/46248
Li, X., Gao, X., Gai, P., Liu, X., and Li, F. (2020a). Degradable metal-organic framework/methylene blue composites-based homogeneous electrochemical strategy for pesticide assay. Sensor. Actuat. B Chem. 323, 128701. doi:10.1016/j.snb.2020.128701
Li, X., Ma, W., Li, H., Bai, Y., and Liu, H. (2019). Metal-organic frameworks as advanced sorbents in sample preparation for small organic analytes. Coord. Chem. Rev. 397, 1–13. doi:10.1016/j.ccr.2019.06.014
Li, X., Ma, W., Li, H., Zhang, Q., and Liu, H. (2020b). Sulfur-functionalized metal-organic frameworks: Synthesis and applications as advanced adsorbents. Coord. Chem. Rev. 408, 213191. doi:10.1016/j.ccr.2020.213191
Li, X., Wang, X., Ma, W., Ai, W., Bai, Y., Ding, L., et al. (2017). Fast analysis of glycosides based on HKUST-1-coated monolith solid-phase microextraction and direct analysis in real-time mass spectrometry. J.Sep.Sci. 40 (7), 1589–1596. doi:10.1002/jssc.201601115
Li, Y., Wang, C., Zeng, X., Sun, X., Zhao, C., Fu, H., et al. (2022). Seignette salt induced defects in Zr-MOFs for boosted Pb(Ⅱ) adsorption: Universal strategy and mechanism insight. Chem. Eng. J. 442, 136276. doi:10.1016/j.cej.2022.136276
Li, Y., Wang, J., Huang, Z., Qian, C., Tian, Y., and Duan, Y. (2021). An Eu-doped Zr-metal-organic framework for simultaneous detection and removal of antibiotic tetracycline. J. Environ. Chem. Eng. 9 (5), 106012. doi:10.1016/j.jece.2021.106012
Li, Z., Xu, X., Fu, Y., Guo, Y., Zhang, Q., Zhang, Q., et al. (2019). A water-stable luminescent metal–organic framework for effective detection of aflatoxin B1 in walnut and almond beverages. RSC Adv 9 (2), 620–625. doi:10.1039/c8ra07804a
Li, Z., Xu, X., Quan, H., Zhang, J., Zhang, Q., Fu, Y., et al. (2021). Adsorptive and responsive hybrid sponge of melamine foam and metal organic frameworks for rapid collection/removal and detection of mycotoxins. Chem. Eng. J. 410, 128268. doi:10.1016/j.cej.2020.128268
Liang, Y., He, J., Huang, Z., Li, H., Zhang, Y., Wang, H., et al. (2020). An amino-functionalized zirconium-based metal-organic framework of type UiO-66-NH2 covered with a molecularly imprinted polymer as a sorbent for the extraction of aflatoxins AFB1, AFB2, AFG1 and AFG2 from grain. Mikrochim. Acta. 187 (1), 32. doi:10.1007/s00604-019-3959-7
Liang, Y., Yao, Y., Liu, Y., Li, Y., Xu, C., Fu, L., et al. (2022). Curcumin-loaded HKUST-1@ carboxymethyl starch-based composites with moisture-responsive release properties and synergistic antibacterial effect for perishable fruits. Int. J. Biol. Macromol. 214, 181–191. doi:10.1016/j.ijbiomac.2022.06.022
Liu, T., Hu, R., Zhang, X., Zhang, K., Liu, Y., Zhang, X., et al. (2016). Metal–Organic Framework Nanomaterials as Novel Signal Probes for Electron Transfer Mediated Ultrasensitive Electrochemical Immunoassay. Anal. Chem. 88 (24), 12516–12523. doi:10.1021/acs.analchem.6b04191
Liu, X., Hu, M., Wang, M., Song, Y., Zhou, N., He, L., et al. (2019). Novel nanoarchitecture of Co-MOF-on-TPN-COF hybrid: Ultralowly sensitive bioplatform of electrochemical aptasensor toward ampicillin. Biosens. Bioelectron. 123, 59–68. doi:10.1016/j.bios.2018.09.089
Liu, X., Zhang, T., Li, X., Ai, S., and Zhou, S. (2022). Non-enzymatic electrochemical sensor based on an AuNPs/Cu-N-C composite for efficient nitrite sensing in sausage samples. New J. Chem. 46 (21), 10415–10421. doi:10.1039/D2NJ01640K
Liu, Y., Gao, Z., Wu, R., Wang, Z., Chen, X., and Chan, T. (2017). Magnetic porous carbon derived from a bimetallic metal-organic framework for magnetic solid-phase extraction of organochlorine pesticides from drinking and environmental water samples. J. Chromatogr. A. 1479, 55–61. doi:10.1016/j.chroma.2016.12.014
Ma, F., Cai, X., Mao, J., Yu, L., and Li, P. (2021). Adsorptive removal of aflatoxin B1 from vegetable oils via novel adsorbents derived from a metal-organic framework. J. Hazard. Mater. 412, 125170. doi:10.1016/j.jhazmat.2021.125170
Ma, J., Bai, W., and Zheng, J. (2022). A novel self-cleaning electrochemical biosensor integrating copper porphyrin-derived metal-organic framework nanofilms, G-quadruplex, and DNA nanomotors for achieving cyclic detection of lead ions. Biosens. Bioelectron. 197, 113801. doi:10.1016/j.bios.2021.113801
Ma, P., Xu, W., Teng, Z., Luo, Y., Gong, C., and Wang, Q. (2022). An Integrated Food Freshness Sensor Array System Augmented by a Metal-Organic Framework Mixed-Matrix Membrane and Deep Learning. ACS Sens 7 (7), 1847–1854. doi:10.1021/acssensors.2c00255
Ma, W., Yang, B., Li, J., and Li, X. (2022a). Amino-functional metal–organic framework as a general applicable adsorbent for simultaneous enrichment of nine neonicotinoids. Chem. Eng. J. 434, 134629. doi:10.1016/j.cej.2022.134629
Ma, W., Li, J., Li, X., and Liu, H. (2022b). Enrichment of diamide insecticides from environmental water samples using metal-organic frameworks as adsorbents for determination by liquid chromatography tandem mass spectrometry. J. Hazard. Mater. 422, 126839. doi:10.1016/j.jhazmat.2021.126839
Magri, A., Petriccione, M., and Guti errez, T. J. (2021). Metal-organic frameworks for food applications: A review. Food Chem 354, 129533. doi:10.1016/j.foodchem.2021.129533
Mahmoodi, N. M., Oveisi, M., Taghizadeh, A., and Taghizadeh, M. (2020). Synthesis of pearl necklace-like ZIF-8@chitosan/PVA nanofiber with synergistic effect for recycling aqueous dye removal. Carbohydr. Polym. 227, 115364. doi:10.1016/j.carbpol.2019.115364
Majd, M., Nojavan, S., and Maghsoudi, M. (2022). Preparation of electrospun polyacrylonitrile/ϒ-cyclodextrin metal–organic framework nanofibers for extraction of multi-classes herbicides from cereal samples before HPLC-UV analysis. Food Chem 393, 133350. doi:10.1016/j.foodchem.2022.133350
Marimuthu, M., Arumugam, S. S., Jiao, T., Sabarinathan, D., Li, H., and Chen, Q. (2022). Metal organic framework based sensors for the detection of food contaminants. TrAC Trend. Anal. Chem. 154, 116642. doi:10.1016/j.trac.2022.116642
Mo, Z., Tai, D., Zhang, H., and Shahab, A. (2022). A comprehensive review on the adsorption of heavy metals by zeolite imidazole framework (ZIF-8) based nanocomposite in water. Chem. Eng. J. 443, 136320. doi:10.1016/j.cej.2022.136320
Nian, L., Wang, M., Wang, F., Xie, Y., Sun, X., Cheng, S., et al. (2022). Multifunctional material Cer@MHKUST-1 with efficient preservation capability. Chem. Eng. J. 433, 133267. doi:10.1016/j.cej.2021.133267
Nong, W., Liu, X., Wang, Q., Wu, J., Guan, Y., et al. (2020). Metal-organic Framework-based Materials: Synthesis, Stability and Applications in Food Safety and Preservation. ES Food and Agroforestry 1, 11–40. doi:10.30919/esfaf0001
Pang, Y., Cao, Y., Han, J., Xia, Y., He, Z., Sun, L., et al. (2022). A novel fluorescence sensor based on Zn porphyrin MOFs for the detection of bisphenol A with highly selectivity and sensitivity. Food Cont 132, 108551. doi:10.1016/j.foodcont.2021.108551
Pirot, S. M., and Omer, K. M. (2022). Designing of robust and sensitive assay via encapsulation of highly emissive and stable blue copper nanocluster into zeolitic imidazole framework (ZIF-8) with quantitative detection of tetracycline. J. Anal. Sci. Technol. 13 (1), 22. doi:10.1186/s40543-022-00333-6
Prathap, M., Kaur, B., and Srivastava, R. (2018). Electrochemical Sensor Platforms Based on Nanostructured Metal Oxides, and Zeolite-Based Materials. Chem. Rec. 19 (5), 883–907. doi:10.1002/tcr.201800068
Qiao, X., Ma, X., Ma, X., Yue, T., and Sheng, Q. (2021). A label-free aptasensor for ochratoxin a detection with signal amplification strategies on ultrathin micron-sized 2D MOF sheets. Sensor. Actuat. B Chem. 334, 129682. doi:10.1016/j.snb.2021.129682
Qin, L., Li, Y., Liang, F., Li, L., Lan, Y., Li, Z., et al. (2022). A microporous 2D cobalt-based MOF with pyridyl sites and open metal sites for selective adsorption of CO2. Micropor. Mesopor. Mat 341, 112098. doi:10.1016/j.micromeso.2022.112098
Qin, L., Wang, X., Liu, Y., and Wei, H. (2018). 2D-Metal-Organic-Framework-Nanozyme Sensor Arrays for Probing Phosphates and Their Enzymatic Hydrolysis. Anal. Chem. 90 (16), 9983–9989. doi:10.1021/acs.analchem.8b02428
Rahul, B., Anh, P., Wang, B., Carolyn, K., Hiroyasu, F., Michael, O., and Yaghi, O. M. (2008). High-throughput synthesis of zeolitic imidazolate frameworks and application to CO2 capture. Scienc 319 (5865), 939–943. doi:10.1126/science.1152516
Rasuli, L., Dehghani, M. H., Alimohammadi, M., Yaghmaeian, K., Rastkari, N., and Salari, M. (2021). Mesoporous metal organic frameworks functionalized with the amino acids as advanced sorbents for the removal of bacterial endotoxins from water: Optimization, regression and kinetic models. J. Mol. Liq. 339, 116801. doi:10.1016/j.molliq.2021.116801
Razavi, S. A. A., Masoomi, M. Y., and Morsali, A. (2017). Double Solvent Sensing Method for Improving Sensitivity and Accuracy of Hg(II) Detection Based on Different Signal Transduction of a Tetrazine-Functionalized Pillared Metal-Organic Framework. Inorg. Chem. 56 (16), 9646–9652. doi:10.1021/acs.inorgchem.7b01155
Shen, M., Liu, D., and Ding, T. (2021). Cyclodextrin-metal-organic frameworks (CD-MOFs): Main aspects and perspectives in food applications. Curr. Opin. Food Sci. 41, 8–15. doi:10.1016/j.cofs.2021.02.008
Song, C., Zhang, Y., Li, X., Ouyang, G., Cui, J., Zhang, L., et al. (2021). Morphology-maintaining synthesis of copper hydroxy phosphate@metal–organic framework composite for extraction and determination of trace mercury in rice. Food Chem 343, 128508. doi:10.1016/j.foodchem.2020.128508
Sriram, G., Bendre, A., Mariappan, E., Altalhi, T., Kigga, M., Ching, Y., et al. (2022). Recent trends in the application of metal-organic frameworks (MOFs) for the removal of toxic dyes and their removal mechanism-a review. Sustainable Materials and Technologies 31, e00378. doi:10.1016/j.susmat.2021.e00378
Stassen, I., Burtch, N., Talin, A., Falcaro, P., Allendorf, M., and Ameloot, R. (2017). An updated roadmap for the integration of metal-organic frameworks with electronic devices and chemical sensors. Chem. Soc. Rev. 46 (11), 3185–3241. doi:10.1039/c7cs00122c
Su, H., Lin, Y., Wang, Z., Wong, Y., Chen, X., and Chan, T. (2016). Magnetic metal-organic framework-titanium dioxide nanocomposite as adsorbent in the magnetic solid-phase extraction of fungicides from environmental water samples. J. Chromatogr. A. 1466, 21–28. doi:10.1016/j.chroma.2016.08.066
Su, Y., Wang, S., Zhang, N., Cui, P., Gao, Y., and Bao, T. (2020). Zr-MOF modified cotton fiber for pipette tip solid-phase extraction of four phenoxy herbicides in complex samples. Ecotoxicol. Environ. Saf. 201, 110764. doi:10.1016/j.ecoenv.2020.110764
Sultana, A., Kathuria, A., and Gaikwad, K. K. (2022). Metal–organic frameworks for active food packaging. A review. Environ. Chem. Lett. 20 (2), 1479–1495. doi:10.1007/s10311-022-01387-z
Sun, Y., Chen, M., Liu, H., Zhu, Y., Wang, D., and Yan, M. (2020). Adsorptive removal of dye and antibiotic from water with functionalized zirconium-based metal organic framework and graphene oxide composite nanomaterial Uio-66-(OH)2/GO. Appl. Surf. Sci. 525, 146614. doi:10.1016/j.apsusc.2020.146614
Sun, Z., Peng, Y., Wang, M., Lin, Y., Jalalah, M., Alsareii, S. A., et al. (2021). Electrochemical Deposition of Cu Metal–Organic Framework Films for the Dual Analysis of Pathogens. Anal. Chem. 93 (25), 8994–9001. doi:10.1021/acs.analchem.1c01763
Tang, J., Chen, Y., Zhao, M., Wang, S., and Zhang, L. (2021). Phenylthiosemicarbazide-functionalized UiO-66-NH2 as highly efficient adsorbent for the selective removal of lead from aqueous solutions. J. Hazard. Mater. 413, 125278. doi:10.1016/j.jhazmat.2021.125278
Tao, C., Chen, B., Liu, X., Zhou, L., Zhu, X., Cao, J., et al. (2017). A highly luminescent entangled metal–organic framework based on pyridine-substituted tetraphenylethene for efficient pesticide detection. Chem. Commun(Camb). 53 (72), 9975–9978. doi:10.1039/C7CC05031C
Tokalıoğlu, S., Yavuz, E., Demir, S., and Patat, S. (2017). Zirconium-based highly porous metal-organic framework (MOF-545) as an efficient adsorbent for vortex assisted-solid phase extraction of lead from cereal, beverage and water samples. Food Chem 237, 707–715. doi:10.1016/j.foodchem.2017.06.005
Wang, B., Lv, X., Feng, D., Xie, L., Zhang, J., Li, M., et al. (2016). Highly Stable Zr(IV)-Based Metal-Organic Frameworks for the Detection and Removal of Antibiotics and Organic Explosives in Water. J. Am. Chem. Soc. 138 (19), 6204–6216. doi:10.1021/jacs.6b01663
Wang, C., Lee, Y. J., Hsu, J. P., and Lee, D. (2020). Phosphate or arsenate modified UiO-66-NO2: Amorphous mesoporous matrix. J. Taiwan Inst. Chem. Eng. 108, 129–133. doi:10.1016/j.jtice.2020.01.010
Wang, J., Li, Y., Lv, Z., Xie, Y., Shu, J., Alsaedi, A., et al. (2019). Exploration of the adsorption performance and mechanism of zeolitic imidazolate framework-8@graphene oxide for Pb(II) and 1-naphthylamine from aqueous solution. J. Colloid. Interface Sci. 542, 410–420. doi:10.1016/j.jcis.2019.02.039
Wang, J., Liu, X., and Wei, Y. (2018). Magnetic solid-phase extraction based on magnetic zeolitic imazolate framework-8 coupled with high performance liquid chromatography for the determination of polymer additives in drinks and foods packed with plastic. Food Chem 256, 358–366. doi:10.1016/j.foodchem.2018.02.136
Wang, P., Xie, L., Joseph, E. A., Li, J., Su, X., and Zhou, H. (2019). Metal-Organic Frameworks for Food Safety. Chem. Rev. 119 (18), 10638–10690. doi:10.1021/acs.chemrev.9b00257
Wang, S., Deng, W., Yang, L., Tan, Y., Xie, Q., and Yao, S. (2017). Copper-Based Metal–Organic Framework Nanoparticles with Peroxidase-Like Activity for Sensitive Colorimetric Detection of Staphylococcus aureus. ACS Appl. Mater. Inter. 9 (29), 24440–24445. doi:10.1021/acsami.7b07307
Wang, S., Wang, Z., Zhang, L., Xu, Y., Xiong, J., Zhang, H., et al. (2022). Adsorption and convenient ELISA detection of sulfamethazine in milk based on MOFs pretreatment. Food Chem 374, 131712. doi:10.1016/j.foodchem.2021.131712
Wang, X., Feng, D., Tang, J., Zhao, Y.D., Li, J., Yang, J., et al. (2019). A water-stable zinc(II)–organic framework as a multiresponsive luminescent sensor for toxic heavy metal cations, oxyanions and organochlorine pesticides in aqueous solution. Dalton Trans 48 (44), 16776–16785. doi:10.1039/C9DT03195B
Wang, Y., Chen, H., Tang, J., Ye, G., Ge, H., and Hu, X. (2015). Preparation of magnetic metal organic frameworks adsorbent modified with mercapto groups for the extraction and analysis of lead in food samples by flame atomic absorption spectrometry. Food Chem 181, 191–197. doi:10.1016/j.foodchem.2015.02.080
Wang, Y., Wang, L., Huang, W., Zhang, T., Hu, X., Perman, J. A., et al. (2017). A metal–organic framework and conducting polymer based electrochemical sensor for high performance cadmium ion detection. J. Mater. Chem. A. 5 (18), 8385–8393. doi:10.1039/C7TA01066D
Wei, J., Wu, X., Wu, C., Hou, F., Wu, L., and Huang, H. (2022). Metal-organic frameworks with peroxidase-like activity for efficient removal of aflatoxin B1. Food Chem 378, 132037. doi:10.1016/j.foodchem.2021.132037
Weng, C., Li, G., Zhang, D., Duan, Z., Chen, K., Zhang, J., et al. (2022). Nanoscale Porphyrin Metal-Organic Frameworks Deliver siRNA for Alleviating Early Pulmonary Fibrosis in Acute Lung Injury. Front. Bioeng. Biotechnol. 10, 939312. doi:10.3389/fbioe.2022.939312
Wen, X., Huang, Q., Nie, D., Zhao, X., Cao, H., Wu, W., et al. (2021). A Multifunctional N-Doped Cu–MOFs (N–Cu–MOF) Nanomaterial-Driven Electrochemical Aptasensor for Sensitive Detection of Deoxynivalenol. Molecules 26 (8), 2243. doi:10.3390/molecules26082243
William, P. L., Soumya, M., Nathan, R., Aamod, D., Li, J., and Sujit, K. G. (2017). Metal-organic frameworks: Functional luminescent and photonic materials for sensing applications. Chem. Soc. Rev. 46 (11), 3242–3285. doi:10.1039/c6cs00930a
Wu, C., Chou, L., Long, L., Si, X., Lo, W., Tsung, C., et al. (2019). Structural Control of Uniform MOF-74 Microcrystals for the Study of Adsorption Kinetics. ACS Appl. Mater. Inter. 11 (39), 35820–35826. doi:10.1021/acsami.9b13965
Wu, K., Wu, C., Fang, M., Ding, B., Liu, P., Zhou, M., et al. (2021). Application of metal–organic framework for the adsorption and detection of food contamination. TrAC Trend. Anal. Chem. 143, 116384. doi:10.1016/j.trac.2021.116384
Xia, J., Gao, Y., and Yu, G. Y. (2021). Tetracycline removal from aqueous solution using zirconium-based metal-organic frameworks (Zr-MOFs) with different pore size and topology: Adsorption isotherm, kinetic and mechanism studies. J. Colloid. Interface Sci. 590, 495–505. doi:10.1016/j.jcis.2021.01.046
Xiao, L., Xu, R., Yuan, Q., and Wang, F. (2017). Highly sensitive electrochemical sensor for chloramphenicol based on MOF derived exfoliated porous carbon. Talanta 167, 39–43. doi:10.1016/j.talanta.2017.01.078
Xu, X., Guo, Y., Wang, X., Li, W., Qi, P., Wang, Z., et al. (2018). Sensitive detection of pesticides by a highly luminescent metal-organic framework. Sensor. Actuat. B Chem. 260, 339–345. doi:10.1016/j.snb.2018.01.075
Xuan, X., Wang, M., Manickam, S., Boczkaj, G., Yoon, J. Y., and Sun, X. (2022). Metal-Organic Frameworks-Based Sensors for the Detection of Toxins in Food: A Critical Mini-Review on the Applications and Mechanisms. Front. Bioeng. Biotechnol. 10, 906374. doi:10.3389/fbioe.2022.906374
Yaghi, O. M., and Li, H. (1995). Hydrothermal Synthesis of a Metal-Organic Framework Containing Large Rectangular Channels. J. Am. Chem. Soc. 117 (41), 10401–10402. doi:10.1021/ja00146a033
Yan, Z., Wu, M., Hu, B., Yao, M., Zhang, L., et al. (2018). Electrospun UiO-66/polyacrylonitrile nanofibers as efficient sorbent for pipette tip solid phase extraction of phytohormones in vegetable samples. J. Chromatogr. A. 1542, 19–27. doi:10.1016/j.chroma.2018.02.030
Yang, D., and Gates, B. C. (2019). Catalysis by Metal Organic Frameworks: Perspective and Suggestions for Future Research. ACS Catal 9 (3), 1779–1798. doi:10.1021/acscatal.8b04515
Yang, L., Wang, J., Lv, H., Ji, X.M., Liu, J.M., and Wang, S. (2022). Hollow-Structured Microporous Organic Networks Adsorbents Enabled Specific and Sensitive Identification and Determination of Aflatoxins. Toxins 14 (2), 137. doi:10.3390/toxins14020137
Yang, Q., Zhou, L., Wu, Y., Zhang, K., Cao, Y., Zhou, Y., et al. (2018). A two dimensional metal-organic framework nanosheets-based fluorescence resonance energy transfer aptasensor with circular strand-replacement DNA polymerization target-triggered amplification strategy for homogenous detection of antibiotics. Anal. Chim. Acta. 1020, 1–8. doi:10.1016/j.aca.2018.02.058
Yang, S., Liu, W., Li, G., Bu, R., Li, P., and Gao, E. (2020). A pH-Sensing Fluorescent Metal–Organic Framework: PH-Triggered Fluorescence Transition and Detection of Mycotoxin. Inorg. Chem. 59 (20), 15421–15429. doi:10.1021/acs.inorgchem.0c02419
Yang, X., Yang, C., and Yan, X. (2013). Zeolite imidazolate framework-8 as sorbent for on-line solid-phase extraction coupled with high-performance liquid chromatography for the determination of tetracyclines in water and milk samples. J. Chromatogr. A. 1304, 28–33. doi:10.1016/j.chroma.2013.06.064
Yang, Z., Zhang, W., Yin, Y., Fang, W., and Xue, H. (2022). Metal-organic framework-based sensors for the detection of toxins and foodborne pathogens. Food Cont 133, 108684. doi:10.1016/j.foodcont.2021.108684
Yao, J., Xie, M., and Li, Y. (2021). Dual-emissive bimetallic organic framework hybrids with Eu(III) and Zr(IV) for ratiometric fluorescence sensing of acrylamide in fried and baked foods. Micropor. Mesopor. Mat. 317, 110831. doi:10.1016/j.micromeso.2020.110831
Yu, Y., Huang, G., Luo, X., Lin, W., Han, Y., Huang, J., et al. (2022). Carbon dots@Cu metal–organic frameworks hybrids for ratiometric fluorescent determination of pesticide thiophanate-methyl. Mikrochim. Acta. 189 (9), 325–9. doi:10.1007/s00604-022-05438-8
Yuan, S., Feng, L., Wang, K., Pang, J., Bosch, M., Lollar, C., et al. (2018). Stable Metal-Organic Frameworks: Design, Synthesis, and Applications. Adv. Mater. 30 (37), 1704303. doi:10.1002/adma.201704303
Zahra, K., Negin, D., Mohammad, T. S., and Khalil, A. (2022). Metal organic frameworks as advanced functional materials for aptasensor design. Spectrochimi. Acta A Mol. Biomol. Spectrosc. 276, 121251. doi:10.1016/j.saa.2022.121251
Zeng, Y., Camarada, M. B., Lu, X., Tang, K., Li, W., Qiu, D., et al. (2022). Detection and electrocatalytic mechanism of zearalenone using nanohybrid sensor based on copper-based metal-organic framework/magnetic Fe3O4-graphene oxide modified electrode. Food Chem 370, 131024. doi:10.1016/j.foodchem.2021.131024
Zhang, J., Tan, Y., and Song, W.J. (2020). Zeolitic imidazolate frameworks for use in electrochemical and optical chemical sensing and biosensing: A review. Mikrochim. Acta. 187 (4), 234. doi:10.1007/s00604-020-4173-3
Zhang, S., Yang, Q., Yang, X., Wang, W., Li, Z., Zhang, L., et al. (2017). A zeolitic imidazolate framework based nanoporous carbon as a novel fiber coating for solid-phase microextraction of pyrethroid pesticides. Talanta 166, 46–53. doi:10.1016/j.talanta.2017.01.042
Zhang, X., Geng, P., Liu, H., Teng, Y., Liu, Y., Wang, Q., et al. (2009). Development of an electrochemical immunoassay for rapid detection of E. coli using anodic stripping voltammetry based on Cu@Au nanoparticles as antibody labels. Biosens. Bioelectron. 24 (7), 2155–2159. doi:10.1016/j.bios.2008.11.019
Zhang, X., Huang, X., Wang, Z., Zhang, Y., Huang, X., Li, Z., et al. (2022). Bioinspired nanozyme enabling glucometer readout for portable monitoring of pesticide under resource-scarce environments. Chem. Eng. J. 429, 132243. doi:10.1016/j.cej.2021.132243
Zhang, X. X., Zhu, M., Jiang, Y., Wang, X., Guo, Z., Shi, J., et al. (2020). Simple electrochemical sensing for mercury ions in dairy product using optimal Cu2+-based metal-organic frameworks as signal reporting. J. Hazard. Mater. 400, 123222. doi:10.1016/j.jhazmat.2020.123222
Zhang, Y., Li, B., Ma, H., Zhang, L., and Zheng, Y. (2016). Rapid and facile ratiometric detection of an anthrax biomarker by regulating energy transfer process in bio-metal-organic framework. Biosens. Bioelectron. 85, 287–293. doi:10.1016/j.bios.2016.05.020
Zhang, Y., Sun, Y., Man, Y., Yuan, H., Zhao, R., Xiang, G., et al. (2022). Highly efficient adsorption and catalytic degradation of aflatoxin B1 by a novel porous carbon material derived from Fe-doped ZIF-8. Chem. Eng. J. 440, 135723. doi:10.1016/j.cej.2022.135723
Zhong, M., Yang, L., Yang, H., Cheng, C., Deng, W., Tan, Y., et al. (2019). An electrochemical immunobiosensor for ultrasensitive detection of Escherichia coli O157:H7 using CdS quantum dots-encapsulated metal-organic frameworks as signal-amplifying tags. Biosens. Bioelectron. 126, 493–500. doi:10.1016/j.bios.2018.11.001
Zhou, J., Liu, J., Pan, P., Li, T., Yang, Z., Wei, J., et al. (2022). Electrochemical determination of levofloxacin with a Cu-metal-organic framework derivative electrode. J. Mater. Sci. Mater. El. 33 (13), 9941–9950. doi:10.1007/s10854-022-07985-5
Keywords: food contamination, adsorption material, sensor, metal-organic frameworks (MOFs), Zn-based MOFs, Cu-based MOFs, Zr-based MOFs
Citation: Guo X, Wang L, Wang L, Huang Q, Bu L and Wang Q (2023) Metal-organic frameworks for food contaminant adsorption and detection. Front. Chem. 11:1116524. doi: 10.3389/fchem.2023.1116524
Received: 05 December 2022; Accepted: 02 January 2023;
Published: 18 January 2023.
Edited by:
Long Wu, Hainan University, ChinaReviewed by:
Qinhe Pan, Hainan University, ChinaXianjiang Li, National Institute of Metrology, China
Jianqiang Liu, Guangdong Medical University, China
Copyright © 2023 Guo, Wang, Wang, Huang, Bu and Wang. This is an open-access article distributed under the terms of the Creative Commons Attribution License (CC BY). The use, distribution or reproduction in other forums is permitted, provided the original author(s) and the copyright owner(s) are credited and that the original publication in this journal is cited, in accordance with accepted academic practice. No use, distribution or reproduction is permitted which does not comply with these terms.
*Correspondence: Qiang Wang, wang76qiang@163.com
†These authors have contributed equally to this work