- 1Department of Medical Pharmacology, Cerrahpasa Medical Faculty, Istanbul University-Cerrahpasa, Istanbul, Turkiye
- 2Department of Physiology, M. Islam Medical and Dental College, Gujranwala, Pakistan
- 3School of Biochemistry, Minhaj University Lahore, Lahore, Pakistan
- 4Institute of Molecular Biology and Biotechnology (IMBB), The University of Lahore, Lahore, Pakistan
- 5School of Pharmacy, Minhaj University Lahore, Lahore, Pakistan
- 6Centre for Environmental Studies, Ege University, Izmir, Turkiye
Introduction: Cadmium(Cd) an industrial poison present abundantly in the environment, causes human toxicity by an inflammatory process. Chronic exposure of cadmium can cause a number of molecular lesions that could be relevant to oncogenesis, through indirect or epigenetic mechanisms, potentially including abnormal activation of oncogenes and suppression of apoptosis by depletion of antioxidants. As induction of cyclooxygenase (COX)-2 is linked to inflammatory processes, use of luteolin, epiafzelechin, and albigenin alone or in different combinations may be used as anti-inflammatory therapeutic agents.
Methods: We, herein, performed in silico experiments to check the binding affinity of phytochemicals and their therapeutic effect against COX-2 in cadmium administered rats. Wistar albino rats were given phytochemicals in different combinations to check their anti-inflammatory activities against cadmium intoxication. The level of alanine aminotransferases (ALT), 4-hydroxynonenal (4HNE), 8-hydroxy-2-deoxyguanosine (8-OHdG), tumor necrosis factor-alpha (TNF-α), isoprostanes (IsoP-2α), COX-2, and malondialdehyde (MDA) were estimated with their respective ELISA and spectrophotometric methods.
Results: The generated results show that phytocompounds possessed good binding energy potential against COX-2, and common interactive behavior was observed in all docking studies. Moreover, the level of ALT, 4HNE, 8-OHdG, TNF-α, IsoP-2α, malondialdehyde, and COX-2 were significantly increased in rats with induced toxicity compared to the control group, whereas in combinational therapy of phytocompounds, the levels were significantly decreased in the group.
Discussion: Taken together, luteolin, epiafzelechin, and albigenin can be used as anti-inflammatory therapeutic agents for future novel drug design, and thus it may have therapeutic importance against cadmium toxicity.
1 Highlights
• AutoDock Vina program was used to perform molecular docking of luteolin, epiafzelechin, and albigenin with COX-2.
• Wistar albino rats were given phytochemicals to evaluate the anti-inflammatory effects against cadmium-induced insult.
• Luteolin, epiafzelechin, and albigenin can be used as anti-inflammatory agents for future novel drug designing.
2 Introduction
Chronic exposure to heavy metals like cadmium (Cd) can cause a number of molecular lesions that could be relevant to oncogenesis, through indirect or epigenetic mechanisms, potentially including abnormal activation of oncogenes and suppression of apoptosis (Waalkes, 2003). Cadmium intoxication results in the depletion of antioxidants such as glutathione, superoxide dismutase, and catalase that damage the lipid content of the cell membrane and induce lipid peroxidation and oxidative stress (Valko et al., 2005).
About 600 metric tons of Cd is synthesized in the United States annually, of which 150 metric tons is inhaled (Nordberg et al., 2007). One of the major factors involved in Cd toxicity is cigarette smoking, and there are high levels of Cd present in the blood of smokers (Richter et al., 2017). Other sources of Cd exposure include unhygienic food, water (that is present in sealed water pipes), drugs, and various dietary supplements (Abernethy et al., 2010). Cd, an industrial poison present abundantly in the environment because of slow processes of destruction and abrasion of rocks and soil, particularly due to fire in the forest and volcanic explosion, leads to an increase in environmental Cd (Everett and Frithsen, 2008). Animals and plants are highly exposed to environmental Cd, which is major cause of human Cd toxicity that destroys various biological organs, including the lungs, liver, kidney, bones, testes, and placenta (Bertin and Averbeck, 2006; Pari and Murugavel, 2007).
Bioinformatics is an exciting area that utilizes mathematical, statistical, and computational approaches for biological problems. The prediction of protein structures by NMR and X-ray crystallography techniques consumes time (Magana et al., 2014). In silico studies utilized various tools for model building of the targeted protein and molecular docking which are less time-consuming. The homology modelling approach was used to build a 3D structure of the targeted protein (Schmidt et al., 2014). The 3D structure of COX-2 is not reported in the Protein Data Bank. In this current study, in silico techniques are utilized to predict the 3D structure of COX-2 by homology modelling approaches to reveal the insight into COX-2 3D structure. The 3D structure of the targeted protein is fundamental for computational drug design.
Nature provides ample resources that are valuable to humankind. The selection of phytochemicals is based on their significance and novelty. The literature depicts the analyses of various phytochemicals; however, their solo and synergistic effects among the disease models need to be illustrated. Acacia senegal is found abundantly in Eastern Africa from Mozambique to South Africa and in tropical areas of Western and Central Africa (Odee et al., 2012). First, it was discovered in Australia, Egypt, the Virgin Islands, Puerto Rico, and South Asia. Acacia senegal is mostly cultivated in Nigeria, Pakistan, and India. It consists of various phytochemicals, playing a major role in food (flavor fixative, emulsifier, and stabilizer of dairy products), pharmaceutics, and therapeutics. Among its various phytocompounds, luteolin is a naturally occurring flavone available with significant biological properties (Muruganathan et al., 2022), limited to not only ameliorating breast cancer (Cook, 2018) but also attenuating sepsis-induced myocardial injury by enhancing autophagy in mice (Wu et al., 2020) and playing a significant hepatoprotective role (Balamurugan and Karthikeyan, 2012; Zhang et al., 2017).
Albizia lebbeck is commonly cultivated in Northern Australia, Africa and Asia regions and Acacia chundra is a perennial plant commonly found in the Asia region (Lampe et al., 2008). The two phytocompounds albigenin and epiafzelechin (present in Albizia lebbeck and Acacia chundra, respectively) exhibited therapeutic application in various inflammatory conditions (Lakshmi et al., 2018). Albigenin extract is derived from albigenic acid derived from the bark of Albizia lebbeck Benth (Barua and Raman, 1959). Albizia lebbeck (Family: Mimosaceae) possesses medicinal properties and has been conventionally used to treat several conditions, such as convulsions (Kasture et al., 2000) and anxiety, and to enhance the cognitive functions (Une et al., 2001).
Similarly, epiafzelechin is a flavonoid found in nature and is reported to be extracted from the leaves of E. acoroides. The extract is a white crystalline powder with antioxidant properties that inhibit oxidative radicals’ scavenging activity. It has been reported to inhibit lipid peroxidation responsible for increasing DNA damage (Seccon et al., 2010). Recent studies showed its protective effects against bone loss due to ovariectomy in adult mice (Wong et al., 2017). In another study, epiafzelechin prevented SARS-CoV-2 replication or transcription by locking 3CLpro and RdRp viral proteins (Dey et al., 2021).
Hence, the current study examines the synergistic use of the phytocompound (luteolin, albigenin, and epiafzelechin) extract to evaluate and determine the effects when administered alone or in combination on the Cd-induced signaling cascade involved in inflammation and DNA damage. We also aim to investigate the new dimensions for novel drug designing by targeting COX-2 through in silico studies.
3 Materials and methods
3.1 Protein structure prediction
The amino acid sequence (604 residues) of COX-2 protein was obtained from UniProt with accession number P35355 in the FASTA format (https://www.uniprot.org/uniprot/P35355). To predict the protein model, BLASTp was used against the Protein Data Bank (PDB) to search for the most appropriate template with the query sequence. The template was selected based on sequence identity and similarity, query coverage, and E-value. Finally, the MODELLER v9.17 tool was used to build the protein model by using a couple of commands: align2d.py and get-model.py (Eswar et al., 2008). The structural assessment of the generated model was confirmed through multiple online tools like PROCHECK (Laskowski et al., 1993), ERRAT (Colovos and Yeates, 1993), VERIFY3D (Eisenberg et al., 1997), and ANOLEA (Melo and Feytmans, 1998). The selected three-dimensional (3D) model of COX-2 was further minimized using UCSF Chimera v1.12 (Meng et al., 2006) at 1,000 steepest and 1,000 conjugate gradient runs with AMBER force field parameters.
3.2 Phytochemical preparation and molecular docking
Based on the literature survey, three phytocompounds, luteolin, albigenin, and epiafzelechin, were retrieved from PubChem (Bolton et al., 2008). The selected phytocompounds were sketched in ChemDraw Ultra and retrieved in the PDB format. For the comparative study, a standard drug (clavazin) was selected. The energy minimization of selected three phytocompounds was minimized using UCSF Chimera. The docking binding pocket of the target protein was predicted using CASTp (Dundas et al., 2006) and 3DLigandSite before energy minimization (Wass et al., 2010). AutoDock Vina was utilized for molecular docking (Trott and Olson, 2010). Drug properties of selected phytocompounds were calculated. Mcule (Kiss et al., 2012) and ADMET profile were calculated using the admetSAR online server (Cheng et al., 2012). UCSF Chimera was used to analyze the results of molecular docking.
3.3 Ethical approval
The experiment was performed in animal house and biochemical analysis was conducted at the Institute of Molecular Biology and Biotechnology (IMBB) of the University of Lahore. The experiment was approved and performed according to the guidelines of the Ethical Research Committee of the University of Lahore.
3.4 Plant extract
The standardized extract of phytochemicals luteolin (Product ID: 72511), epiafzelechin (Product ID: FE139053), and albigenin (Product ID: FDB015558) was purchased separately from Sigma-Aldrich Corporation (St. Louis, MO, United States), Biosynth (United States), and Genome Canada, respectively, and later their different doses were prepared according to the study design.
3.5 Study designing
The Wister albino rats were divided randomly into nine groups. Group A was the control group without inducing hepatotoxicity with Cd exposure. Rats in group B were induced hepatotoxicity with exposure to Cd 1.5 mM/kg body wt/d (90 days). Rats in groups C, D, and E were induced hepatotoxicity with exposure to Cd and also treated with single therapy of luteolin, epiafzelechin, and albigenin (@500 mg/kg body wt.), respectively. A preliminary study was carried out to check the toxicity of oral dosage of selected phytochemicals (luteolin, epiafzelechin, and albigenin) in the rat model, and guidelines for the subacute toxicity were followed. A single dose of 500 mg/kg administered orally to albino rats was studied for acute toxicity. Independent doses of 100, 200, 400, and 500 mg/kg were administered orally for 28 consecutive days, and findings for each parameter were then compared with healthy ones. No significant change in weight of selected organs was reported compared to healthy ones. No major toxicity or organ damage was reported, and the respective dose was considered to be safe for the administration in animal models. Moreover, the results were verified with the help of RSM (Response Surface Methodology).
Group F was exposed to Cd to induce liver damage and then treated with the combination of luteolin + epiafzelechin. The combination of two phytochemicals luteolin + albigenin was fed to rats exposed to Cd in group G. Group H was induced liver damage with Cd exposure and was administered with the combination of two phytochemicals epiafzelechin + albigenin. Group I animals received the combination therapy of three phytochemicals luteolin + epiafzelechin + albigenin to investigate their effect on liver damage induced by Cd (Table 1).
3.6 Biochemical analysis
A volume of 5 ml of the blood sample was taken from the heart of the Wister albino rats during dissection, and serum was separated from the blood samples after centrifugation for 10 min at 4000 rpm.
3.6.1 Estimation of oxidative and inflammatory biomarkers
The samples were analyzed for the levels of alanine aminotransferase (ALT), malondialdehyde, cyclooxygenase-2 (COX-2), 8-hydroxy-2′-deoxyguanosine (8-OHdG), isoprostanes, 4-hydroxynonenal (4-HNE), and tumor necrosis factor-α (TNF-α) using commercially available ELISA kits (Abcam), following their respective protocols.
3.7 Statistical analysis
The data were subjected to analyses of variance using a CoStat computer package (Cohort Software, Berkley, California). The mean values were compared with the least significance difference test following the methods proposed by Snedecor and Cochran. Statistical analysis was performed using one-way analysis of variance (ANOVA) followed by Duncan’s multiple range test (DMR-test). Pearson’s correlation test was used to find the statistical relationship between two variables. Values are represented as mean ± SD, and p-values < 0.05 were considered significant.
4 Results
4.1 Protein structure analysis
The structure validation of COX-2 was confirmed using ERRAT and VERIFY3D. The predicted results showed that the generated model has good structural accuracy, and most of the residues were present in the allowed regions. The predicted model of the protein is shown in Figure 1.
4.2 Chemoinformatic analysis of selected phytochemicals
Drug-likeness properties were calculated using the Mcule online server (Table 2). There are three phytocompounds luteolin, albigenin, and epiafzelechin examined in this study (Figures 2 A–C). The chemoinformatic analysis showed that all the three phytocompounds possessed good biological properties. The molecular weight of all the three compounds was comparable with the standard value (<500 mg/mol). Moreover, the hydrogen bond acceptor/donor (HBA/D) was less than 10 and 5, respectively. However, the LogP value of albigenin was more significant than 5, while that of both luteolin and epiafzelechin was 2.28 and 1.84, respectively. The Lipinski Rule of Five testified against all three compounds revealed that albigenin showed RO5 violation, whereas luteolin and epiafzelechin obeyed the rule.
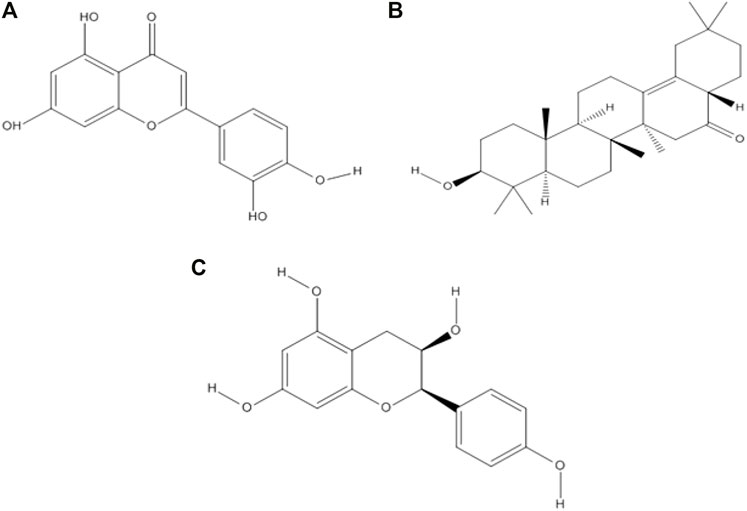
FIGURE 2. Two-dimensional structures of three selected phytocompounds. (A) Luteolin, (B) albigenin, and (C) epiafzelechin.
4.3 Pharmacokinetic analysis of selected compounds
The ADMET profile of selected phytocompounds was calculated by admetSAR (Table 3). Two parameters were selected for absorption: blood–brain barrier (BBB) penetration and human intestinal absorption (HIA). For BBB penetration, luteolin showed negative results, whereas albigenin and epiafzelechin exhibited positive results. Moreover, positive values (+0.9650, + 0.8704, and + 0.9857) were observed against intestinal absorption, which ensures that all three phytochemicals may potentially cross intestinal barriers through absorption. Moreover, all three compounds exhibited non-AMES and non-carcinogenic behavior. The overall results showed that all three compounds possessed good lead-like behavior based on these ADMET results and can be used for further analysis.
4.4 Molecular docking
4.4.1 Binding affinity (Kcal/mol)
The molecular docking study was utilized to identify binding pockets and affinities of these compounds against the target protein. Table 4 shows that all three phytochemicals were bound within the active binding sites of the target protein. Luteolin, albigenin, and epiafzelechin exhibited good binding energy values (−10.3, −9.6, and −7.8 kcal/mol, respectively) compared to a standard drug clavazin (−12.5 kcal/mol).
4.4.2 Binding interactions
Analyzing the docking complex confirmed the binding conformation positions of phytochemicals inside the binding pocket. In the luteolin–COX-2 docking complex, a couple of hydrogen bonds was observed at Phe-179 and His-176 residues having bond distances of 2.04 and 1.97 Å, respectively (Figure 3A). Figures 3B,C show the docking complexes of albigenin and epiafzelechin against COX-2, respectively. In albigenin and epiafzelechin docking complexes, a couple of hydrophobic pockets and hydrogen bonds was observed at particular residues with appropriate binding distances. The standard drug clavazin was also considered for docking analysis to check its binding profile against COX-2. Two hydrogen bonds were observed at Asn-344 and Asn-506 residues (Figure 3D).
4.5 In vivo analysis
4.5.1 Serum level of biochemical variables in Cd-treated rats
A significant improvement in the biochemical variables has been observed in rats receiving phytochemical therapy after Cd administration (Table 5). A significant reduction has been detected in rats receiving monotherapy of luteolin, epiafzelechin, and albigenin (groups C, D, and E) after stimulation with Cd. In contrast, the levels of biochemical variables were restored significantly (20.253 ± 4.08 IU/L, 6.26 ± 2.28 ng/L, 5.79 ± 2.19 pg/mL, 11.16 ± 1.66 ng/mL, 51.36 ± 5.377, 3.19 ± 1.187, and 3.36 ± 7.027, respectively) in rats receiving combination treatment of three plant extracts as compared to those of group B (131.35 ± 1.57 IU/L, 28.16 ± 1.89 ng/L, 20.19 ± 2.19 pg/mL, 97.17 ± 2.18 ng/mL, 151.16 ± 3.16 pg/mL, 6.38 ± 2.27 nmol/mL, and 6.19 ± 2.17 ng/mL, respectively). Moreover, the levels of ALT, HNE, 8-OHdG, TNF-α, IsoP-2α, MDA, and COX-2 were amended remarkably in rats receiving combinational therapy of phytocompounds extracted from plants (Tables 5, 6).
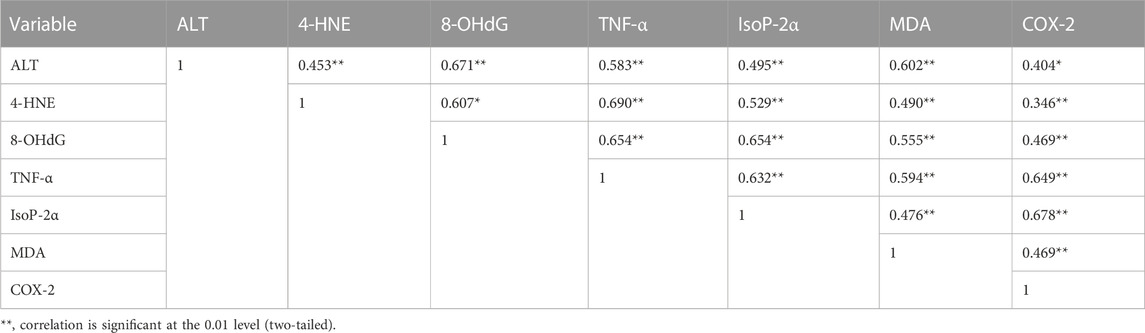
TABLE 6. Pearson’s correlation coefficients of different variables in liver tissues of rats stimulated with Cd.
5 Discussion
In the present study, we designed novel drugs of three phytochemicals through in-silico approaches. There has been considerable focus on phytocompounds as anti-inflammatory agents that could be used to cure or prevent diseases (Liu et al., 2013). The molecular docking analyses were performed on phytocompounds (luteolin, epiafzelechin, and albigenin) against COX-2 to identify the anti-inflammatory properties. The selected three phytocompounds showed high binding and anti-inflammatory activity against COX-2 (Table 4). Previous research ensured the significance of active sites consisting of amino acid residues (His-90, Val-117, Arg-120, Arg-121, Val-350, Leu-353, Ser-354, Tyr-355, Tyr-356, Leu-360, Phe-382, Tyr-385, Trp-387, Trp-388, Arg-513, Phe-519, Met-523, Val-524, Glu-527, Ala-528, Ser-530, Leu-531, and Leu-532) by X-ray crystallography (Orlando et al., 2015). Moreover, another structural analysis study of COX-2 depicts some key residues (Ala-185, Phe-186, Phe-187, Ala-188, Gln-189, His-190, Thr-192, His-193, Gln-194, Phe-196, Thr-198, Asn-368, Leu-370, Tyr-371, His-372, Trp-373, His-374, Leu-376, Leu-377, Val-433, Ser-437, Gln-440, Tyr-490, Leu-493, and Leu-494) involved in the binding pocket conformation (Amaravani et al., 2012). The comparative results showed that most of the residues were common, which ensured the significance of our docking results. These residues might be involved in inhibiting the inflammation process (Figure 7). Overexpression of COX-2 is linked to inflammation and many pathophysiological conditions like cancer and neuronal diseases (Choi et al., 2009; Amaravani et al., 2012). The in vivo results of the present study demonstrated the role of three phytochemicals in attenuating the inflammatory process along with significant antioxidant properties by activating various proteolytic enzymes that repair DNA and protein (Table 6). Our in silico results showed that three phytocompounds possessed good chemoinformatic and ADMET profiles (Table 3). The molecular properties of small compounds always depend on LogP values, including bioavailability and permeability of the membrane.
Long-range transport of cadmium pollution primarily occurs through air and water, eventually leading to topsoil deposition and accumulation in plants (e.g., tobacco). Tobacco smoke is the leading cause of Cd revelation (Satarug and Moore, 2004). The immediate effect of the Cd revelation is oxidative stress. The antioxidant glutathione (GSH) mitigates adverse physio-metabolic effects and defends against Cd stress (Jung et al., 2021). GSH reduction is also pretentious for the DNA duplication, repair, differentiation, propagation, and apoptotic mechanism (Chatterjee, 2013). DNA methylation and cell cycle developments are controlled by several cellular factors that can be activated or inactivated by Cd administration. DNA production and cell propagation rely on cumulative Cd concentrations (Yang et al., 2004). It has been found that the overexpression of the OLE1 gene is central to reducing oxidative stress induced by Cd, possibly through inhibiting lipid peroxidation and protecting the cytoplasmic membrane from damage in yeast (Ozturk et al., 2021). Exposure to toxic Cd levels triggers different RAS kinase pathways, expressively MAPK (Jonak et al., 2004). Cd persuades the overexpression of proto-oncogenes like c-fos, c-myc, and c-jun (Spruill et al., 2002). Cd revelation may lead to tumor growth and propagation through expression of these genes. The Myb-type transcription factor activates Cd-induced cyclin D and cyclin E in mammalian cells (Bertin and Averbeck, 2006). Cd increases the production of tumor necrosis factors (TNF-α), interleukin-10 (IL-10), and heme oxygenase-1(HO-1) (Weber et al., 2006; Lawal et al., 2015; Bonaventura et al., 2018).
Metallothioneins (MTs) are cysteine-rich, heavy metal-binding proteins that play a central role in essential trace element homeostasis and detoxification (Cobbett and Goldsbrough, 2002). Mammalian metallothionein can protect in contradiction of Cd injuries (López et al., 2003) as it makes complexes with free-Cd through cysteine deposition. Cd and oxidative stress triggered metallothionein production and its gene transcription along with specific heat shock proteins (HSPs) that influence the apoptosis pathways (Kusakabe et al., 2008). Metallothionein is a transporter protein; the Cd–MT complex enters into the blood and dysfunction the multiple organs of the body (Sabolić, 2006) through the activation of G-protein–metal coupled receptors present at the cell surface. It activates phospholipase C (PLC) (Gu et al., 2018), resulting in the formation of IP3 (inositol triphosphate) and DAG (diacylglycerol). At the same time, IP3 releases Ca2+ from the endoplasmic reticulum. Ca2+ activates calpain, which plays a significant role in apoptosis through the cleavage of BAX/BAK (Sobhan et al., 2013). BAX triggers cytochrome C release and apoptosis-inducing factor (AIF) from mitochondria to cytosol (Garrido et al., 2006). Calpain-1 activates caspase 9, which activates caspase-3 and induces apoptosis (Nakajima et al., 2014). Cell death results when an external signal activates a metabolic pathway (Compton, 1992). Physiological and pathological conditions trigger eukaryotic apoptosis, a different form of cell death (Arends and Wyllie, 1991).
The phenomenon of cell death is fundamental during multicellular growth to maintain homeostasis as it excludes undesirable cells (Vaux, 1993). Cd enters mitochondria either directly or through Ca2+, which results in increased levels of ROS through NADPH oxidase, which might be neutralized through the activation of the defense system by the cascade of reactions like SOD, CAT, and GSH (Smeets et al., 2009). Damaged epithelial cells formed the ROS with the activation of nicotinamide adenine dinucleotide phosphate (NADPH) oxidase (Fu et al., 2013). ROS produced in the mitochondrial cell through exogenous and endogenous signals promote prostaglandin production (Korbecki et al., 2013). Isoprostanes are free radical-catalyzed prostaglandin-like products of unsaturated fatty acids, such as arachidonic acid, eventually involved in inflammation and cardiovascular events (Halliwell and Lee, 2010; Bauer et al., 2014). In response to Cd, ROS can be formed by non-enzymatic and enzymatic reactions targeting the lung, liver, kidney, and testes following acute intoxication and causing nephrotoxicity, immunotoxicity, osteotoxicity, and tumors after prolonged exposure (Liu et al., 2009; Chmielowska-Bąk et al., 2014). Malondialdehyde (MDA) is the final product of lipid peroxidation and a relevant biomarker in clinical settings (Khoubnasab Jafari et al., 2015). MDA has a positive correlation with COX-2 (0.469**). Disorders in DNA occur due to the high MDA level, which is determined as 8-OHdG. Furthermore, the results of the present study showed that 8-OHdG has a positive correlation with MDA (0.555**). 8-OHdG is a stress biomarker and is a risk factor for cancer, diabetes, and atherosclerosis (Wu et al., 2004). Our study showed an 8-OHdG positive correlation with TNF-α (0.654**), IsoP-2α (0.654**), and COX-2 (0.469**). Owing to the disturbance in cells, I kappa B kinase (IKK) inhibits NF-kB, a nuclear factor pathway that takes part in inflammation. NF-kB is an inducible transcription factor for various procedures, like cell existence, differentiation, inflammation, and growth for genes, as shown in Figure 4.
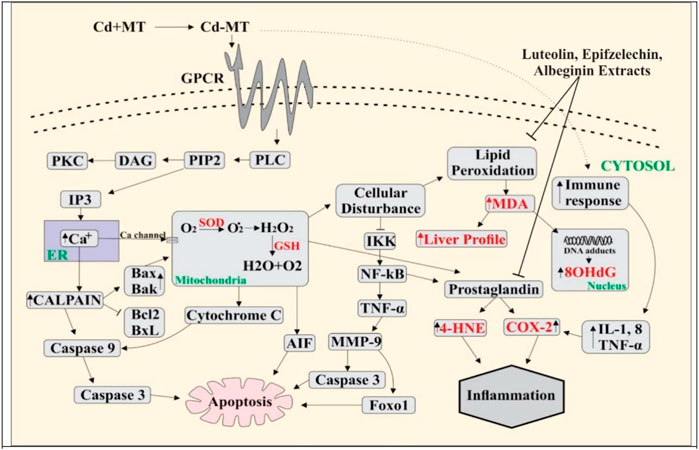
FIGURE 4. Cd enters blood by making a complex with metallothionein, and Cd binds with G-protein–metal coupled receptors on the cell surface, resulting in the activation of phospholipase C (PLC). Activated PLC results in PIP2 production that activates IP3, leading to the release of Ca2+ from ER. Ca2+ increases the level of calpain which further activates the caspases and as a result apoptosis occurs in the cell. Due to overloading, Cd enters into the mitochondria through the Ca2+ channels and ROS is produced in excess amount. This activates cytochrome C release and induces apoptosis through the caspases. Overproduction of ROS creates the disturbance in normal cell activity. Lipid peroxidation occurs when the level of MDA increases, DNA adducts are produced, and 8-OHdG levels are high in the nucleus. NF-kB is activated by the inhibition of IKK. NF-kB activates TNF-α, which produces MMP9, and apoptosis occurs through the activation of AKT and FOXO1. The PGE level increases by amplifying COX-2 and 4HNE. Immune response rises due to overloading Cd-activated inflammatory cytokines (IL-8, TNF, and IL-1), and the increase in the COX-2 level leads to inflammation.
In multiple systems, Cd activates NF-kB and TNF-α, further extending the inflammatory reaction. In the present study, TNF-α is positively correlated with IsoP-2α (0.632**) and MDA (0.594**). TNF-α increased the activation of matrix metalloproteinase (MMP), a protein involved in the degradation of the extracellular matrix of physiological and pathological processes (Kirschvink et al., 2005). MMP9 activates protein kinase B, which is involved in cell death, glucose metabolism, and cell proliferation (Endo et al., 2018; Ma et al., 2020). AKT is essential in activating caspase-3, which plays a role in cell death. MMP9 also activates Forkhead box protein O1 (FOXO1), which is critical for apoptosis. TNF-α increases cyclooxygenase 2 (COX-2) and 4-hydroxynonenal (4-HNE) levels, while COX-2 is a bifunctional enzyme that converts the arachidonic acid into prostaglandin and plays a vital role in inflammation. 4-HNE is vital for signal transduction, and it also plays a role in inflammation (Ayala et al., 2014; Hashim et al., 2022) and 4-HNE has a positive correlation with TNF-α (0.690**), IsoP-2α (0.529**), MDA (0.490**), and COX-2 (0.346**). The role of phytochemicals in Cd insult holds greater significance. A recent study showed that vitexin treatment reduced Cd-induced renal toxicity in rats (Umar Ijaz et al., 2021). Similarly, Hashim et al. (2022) found that Catharanthus roseus (CR) extract exhibited significant antioxidant activity against ROS-mediated DNA damage induced by Cd poisoning. The literature is in line with our findings that supports the use of bioactives for medicinal purposes.
Our findings provide evidence that the selected three phytocompounds ameliorated the toxic effects of Cd-induced toxicity by inhibiting inflammatory pathways. Hence, both in silico and in vivo data depicted the importance of these phytocompounds in novel drug design against inflammatory diseases.
Data availability statement
The original contributions presented in the study are included in the article/Supplementary Material; further inquiries can be directed to the corresponding author.
Ethics statement
The animal study was reviewed and approved by the Animal House and Biochemical Analysis Institute of Molecular Biology and Biotechnology (IMBB) of the University of Lahore.
Author contributions
Conception and design of the study: NT, MA, and AM. Acquisition of data, analysis, and interpretation of data: SW, AZ, and MJ. Drafting the article: AS, HS, and MO. Revising the article critically for important intellectual content: AS, AM, MA, and MO. Final approval of the version to be submitted: MO and AM. All authors contributed equally and have read and agreed to the published version of the manuscript.
Conflict of interest
The authors declare that the research was conducted in the absence of any commercial or financial relationships that could be construed as a potential conflict of interest.
Publisher’s note
All claims expressed in this article are solely those of the authors and do not necessarily represent those of their affiliated organizations, or those of the publisher, the editors, and the reviewers. Any product that may be evaluated in this article, or claim that may be made by its manufacturer, is not guaranteed or endorsed by the publisher.
References
Abernethy, D. R., Destefano, A. J., Cecil, T. L., Zaidi, K., and Williams, R. L. (2010). Metal impurities in food and drugs. Pharm. Res. 27, 750–755. doi:10.1007/s11095-010-0080-3
Amaravani, M., Prasad, N. K., and Ramakrishna, V. (2012). COX-2 structural analysis and docking studies with gallic acid structural analogues. SpringerPlus 1, 58. doi:10.1186/2193-1801-1-58
Arends, M. J., and Wyllie, A. H. (1991). “Apoptosis: Mechanisms and roles in pathology,” in International review of experimental pathology (Amsterdam, Netherlands: Elsevier).
Ayala, A., Muñoz, M. F., and Argüelles, S. (2014). Lipid peroxidation: Production, metabolism, and signaling mechanisms of malondialdehyde and 4-hydroxy-2-nonenal. Oxid. Med. Cell Longev. 2014, 1–31. doi:10.1155/2014/360438
Balamurugan, K., and Karthikeyan, J. (2012). Evaluation of luteolin in the prevention of N-nitrosodiethylamine-induced hepatocellular carcinoma using animal model system. Indian J. Clin. Biochem. IJCB 27, 157–163. doi:10.1007/s12291-011-0166-7
Barua, A. K., and Raman, S. P. (1959). The constitution of albigenic acid—A new triterpenoid sapogenin from albizzia lebbeck benth. Tetrahedron 7, 19–23. doi:10.1016/0040-4020(59)80047-8
Bauer, J., Ripperger, A., Frantz, S., Ergün, S., Schwedhelm, E., and Benndorf, R. A. (2014). Pathophysiology of isoprostanes in the cardiovascular system: Implications of isoprostane-mediated thromboxane A2 receptor activation. Br. J. Pharmacol. 171, 3115–3131. doi:10.1111/bph.12677
Bertin, G., and Averbeck, D. (2006). Cadmium: Cellular effects, modifications of biomolecules, modulation of DNA repair and genotoxic consequences (a review). Biochimie 88, 1549–1559. doi:10.1016/j.biochi.2006.10.001
Bolton, E. E., Wang, Y., Thiessen, P. A., and Bryant, S. H. (2008). “PubChem: Integrated platform of small molecules and biological activities,” in Annual reports in computational Chemistry (Amsterdam, Netherlands: Elsevier).
Bonaventura, P., Lamboux, A., Albarède, F., and Miossec, P. (2018). Differential effects of TNF-α and IL-1β on the control of metal metabolism and cadmium-induced cell death in chronic inflammation. PLoS One 13, e0196285. doi:10.1371/journal.pone.0196285
Chatterjee, A. (2013). Reduced glutathione: A radioprotector or a modulator of DNA-repair activity? Nutrients 5, 525–542. doi:10.3390/nu5020525
Cheng, F., Li, W., Zhou, Y., Shen, J., Wu, Z., Liu, G., et al. (2012). admetSAR: A comprehensive source and free tool for assessment of chemical ADMET properties. J. Chem. Inf. Model. 52, 3099–3105. doi:10.1021/ci300367a
Chmielowska-Bąk, J., Gzyl, J., Rucińska-Sobkowiak, R., Arasimowicz-Jelonek, M., and Deckert, J. (2014). The new insights into cadmium sensing. Front. Plant Sci. 5, 245. doi:10.3389/fpls.2014.00245
Choi, S. H., Aid, S., and Bosetti, F. (2009). The distinct roles of cyclooxygenase-1 and -2 in neuroinflammation: Implications for translational research. Trends Pharmacol. Sci. 30, 174–181. doi:10.1016/j.tips.2009.01.002
Cobbett, C., and Goldsbrough, P. (2002). Phytochelatins and metallothioneins: Roles in heavy metal detoxification and homeostasis. Annu. Rev. Plant Biol. 53, 159–182. doi:10.1146/annurev.arplant.53.100301.135154
Colovos, C., and Yeates, T. O. (1993). Verification of protein structures: Patterns of nonbonded atomic interactions. Protein Sci. 2, 1511–1519. a publication of the Protein Society. doi:10.1002/pro.5560020916
Compton, M. M. (1992). A biochemical hallmark of apoptosis: Internucleosomal degradation of the genome. Cancer Metastasis Rev. 11, 105–119. doi:10.1007/bf00048058
Cook, M. T. (2018). Mechanism of metastasis suppression by luteolin in breast cancer. Breast Cancer (Dove Med. Press) 10, 89–100. doi:10.2147/BCTT.S144202
Dey, D., Dey, N., Ghosh, S., Chandrasekaran, N., Mukherjee, A., and Thomas, J. (2021). Potential combination therapy using twenty phytochemicals from twenty plants to prevent SARS- CoV-2 infection: An in silico Approach. Virusdisease 32, 108–116. doi:10.1007/s13337-021-00658-7
Dundas, J., Ouyang, Z., Tseng, J., Binkowski, A., Turpaz, Y., and Liang, J. (2006). CASTp: Computed atlas of surface topography of proteins with structural and topographical mapping of functionally annotated residues. Nucleic Acids Res. 34, W116–W118. doi:10.1093/nar/gkl282
Eisenberg, D., Lüthy, R., and Bowie, J. U. (1997). “[20] VERIFY3D: Assessment of protein models with three-dimensional profiles,” in Methods in enzymology (Amsterdam, Netherlands: Elsevier).
Endo, H., Owada, S., Inagaki, Y., Shida, Y., and Tatemichi, M. (2018). Glucose starvation induces LKB1-AMPK-mediated MMP-9 expression in cancer cells. Sci. Rep. 8, 10122. doi:10.1038/s41598-018-28074-w
Eswar, N., Eramian, D., Webb, B., Shen, M.-Y., and Sali, A. (2008). “Protein structure modeling with MODELLER,” in Methods in molecular Biology (New Jersey, United States: Humana Press).
Everett, C. J., and Frithsen, I. L. (2008). Association of urinary cadmium and myocardial infarction. Environ. Res. 106, 284–286. doi:10.1016/j.envres.2007.10.009
Fu, P., Mohan, V., Mansoor, S., Tiruppathi, C., Sadikot, R. T., and Natarajan, V. (2013). Role of nicotinamide adenine dinucleotide phosphate-reduced oxidase proteins in Pseudomonas aeruginosa-induced lung inflammation and permeability. Am. J. Respir. Cell Mol. Biol. 48, 477–488. doi:10.1165/rcmb.2012-0242oc
Garrido, C., Galluzzi, L., Brunet, M., Puig, P. E., Didelot, C., and Kroemer, G. (2006). Mechanisms of cytochrome c release from mitochondria. Cell Death Differ. 13, 1423–1433. doi:10.1038/sj.cdd.4401950
Gu, J., Dai, S., Liu, Y., Liu, H., Zhang, Y., Ji, X., et al. (2018). Activation of Ca(2+)-sensing receptor as a protective pathway to reduce Cadmium-induced cytotoxicity in renal proximal tubular cells. Sci. Rep. 8, 1092. doi:10.1038/s41598-018-19327-9
Halliwell, B., and Lee, C. Y. (2010). Using isoprostanes as biomarkers of oxidative stress: Some rarely considered issues. Antioxid. Redox Signal 13, 145–156. doi:10.1089/ars.2009.2934
Hashim, M., Arif, H., Tabassum, B., Arif, A., Rehman, A. A., Rehman, S., et al. (2022). Protective effect of Catharanthus roseus extract on cadmium-induced toxicity in albino rats: A putative mechanism of detoxification. Metabolites 12, 1059. doi:10.3390/metabo12111059
Jonak, C., Nakagami, H., and Hirt, H. (2004). Heavy metal stress. Activation of distinct mitogen-activated protein kinase pathways by copper and cadmium. Plant Physiol. 136, 3276–3283. doi:10.1104/pp.104.045724
Jung, H.-I., Lee, T.-G., Lee, J., Chae, M.-J., Lee, E.-J., Kim, M.-S., et al. (2021). Foliar-applied glutathione mitigates cadmium-induced oxidative stress by modulating antioxidant-scavenging, redox-regulating, and hormone-balancing systems in Brassica napus. Front. Plant Sci. 12, 700413. doi:10.3389/fpls.2021.700413
Kasture, V. S., Chopde, C. T., and Deshmukh, V. K. (2000). Anticonvulsive activity of Albizzia lebbeck, Hibiscus rosa sinesis and Butea monosperma in experimental animals. J. Ethnopharmacol. 71, 65–75. doi:10.1016/s0378-8741(99)00192-0
Khoubnasab Jafari, M., Ansarin, K., and Jouyban, A. (2015). Comments on "use of malondialdehyde as a biomarker for assesing oxidative stress in different disease pathologies: A review. Iran. J. Public Health 44, 714–715.
Kirschvink, N., Vincke, G., Fiévez, L., Onclinx, C., Wirth, D., Belleflamme, M., et al. (2005). Repeated cadmium nebulizations induce pulmonary MMP-2 and MMP-9 production and enphysema in rats. Toxicology 211, 36–48. doi:10.1016/j.tox.2005.02.012
Kiss, R., Sandor, M., and Szalai, F. A. (2012). http://Mcule.com: a public web service for drug discovery. J. Cheminformatics 4. doi:10.1186/1758-2946-4-S1-P17
Korbecki, J., Baranowska-Bosiacka, I., Gutowska, I., and Chlubek, D. (2013). The effect of reactive oxygen species on the synthesis of prostanoids from arachidonic acid. J. Physiol. Pharmacol. 64, 409–421.
Kusakabe, T., Nakajima, K., Nakazato, K., Suzuki, K., Takada, H., Satoh, T., et al. (2008). Changes of heavy metal, metallothionein and heat shock proteins in Sertoli cells induced by cadmium exposure. Toxicol Vitro 22, 1469–1475. doi:10.1016/j.tiv.2008.04.021
Lakshmi, T., Sri Renukadevi, B., Senthilkumar, S., Haribalan, P., Parameshwari, R., Vijayaraghavan, R., et al. (2018). Seed and bark extracts of Acacia catechu protects liver from acetaminophen induced hepatotoxicity by modulating oxidative stress, antioxidant enzymes and liver function enzymes in Wistar rat model. Biomed. Pharmacother. 108, 838–844. doi:10.1016/j.biopha.2018.08.077
Lampe, B. J., Park, S. K., Robins, T., Mukherjee, B., Litonjua, A. A., Amarasiriwardena, C., et al. (2008). Association between 24-hour urinary cadmium and pulmonary function among community-exposed men: The VA normative aging study. Environ. Health Perspect. 116, 1226–1230. doi:10.1289/ehp.11265
Laskowski, R. A., Macarthur, M. W., Moss, D. S., and Thornton, J. M. (1993). Procheck: A program to check the stereochemical quality of protein structures. J. Appl. Crystallogr. 26, 283–291. doi:10.1107/s0021889892009944
Lawal, A. O., Marnewick, J. L., and Ellis, E. M. (2015). Heme oxygenase-1 attenuates cadmium-induced mitochondrial-caspase 3- dependent apoptosis in human hepatoma cell line. BMC Pharmacol. Toxicol. 16, 41. doi:10.1186/s40360-015-0040-y
Liu, J., Qu, W., and Kadiiska, M. B. (2009). Role of oxidative stress in cadmium toxicity and carcinogenesis. Toxicol. Appl. Pharmacol. 238, 209–214. doi:10.1016/j.taap.2009.01.029
Liu, Z., Fang, H., Reagan, K., Xu, X., Mendrick, D. L., Slikker, W., et al. (2013). In silico drug repositioning – what we need to know. Drug Discov. Today 18, 110–115. doi:10.1016/j.drudis.2012.08.005
López, E., Figueroa, S., Oset-Gasque, M. J., and González, M. P. (2003). Apoptosis and necrosis: Two distinct events induced by cadmium in cortical neurons in culture. Br. J. Pharmacol. 138, 901–911. doi:10.1038/sj.bjp.0705111
Ma, J. H., Qin, L., and Li, X. (2020). Role of STAT3 signaling pathway in breast cancer. Cell Commun. Signal 18, 33. doi:10.1186/s12964-020-0527-z
Magana, A. J., Taleyarkhan, M., Alvarado, D. R., Kane, M., Springer, J., and Clase, K. (2014). A survey of scholarly literature describing the field of bioinformatics education and bioinformatics educational research. CBE life Sci. Educ. 13, 607–623. doi:10.1187/cbe.13-10-0193
Melo, F., and Feytmans, E. (1998). Assessing protein structures with a non-local atomic interaction energy. J. Mol. Biol. 277, 1141–1152. doi:10.1006/jmbi.1998.1665
Meng, E. C., Pettersen, E. F., Couch, G. S., Huang, C. C., and Ferrin, T. E. (2006). Tools for integrated sequence-structure analysis with UCSF Chimera. BMC Bioinforma. 7, 339. doi:10.1186/1471-2105-7-339
Muruganathan, N., Dhanapal, A. R., Baskar, V., Muthuramalingam, P., Selvaraj, D., Aara, H., et al. (2022). Recent updates on source, biosynthesis, and therapeutic potential of natural flavonoid luteolin: A review. Metabolites 12, 1145. doi:10.3390/metabo12111145
Nakajima, E., Hammond, K. B., Shearer, T. R., and Azuma, M. (2014). Activation of the mitochondrial caspase pathway and subsequent calpain activation in monkey RPE cells cultured under zinc depletion. Eye (Lond) 28, 85–92. doi:10.1038/eye.2013.239
Nordberg, G. F., Nogawa, K., Nordberg, M., and Friberg, L. T. (2007). “Cadmium,” in Handbook on the toxicology of metals (Amsterdam, Netherlands: Elsevier).
Odee, D. W., Telford, A., Wilson, J., Gaye, A., and Cavers, S. (2012). Plio-pleistocene history and phylogeography of Acacia Senegal in dry woodlands and savannahs of sub-saharan tropical Africa: Evidence of early colonisation and recent range expansion. Heredity 109, 372–382. doi:10.1038/hdy.2012.52
Orlando, B. J., Lucido, M. J., and Malkowski, M. G. (2015). The structure of ibuprofen bound to cyclooxygenase-2. J. Struct. Biol. 189, 62–66. doi:10.1016/j.jsb.2014.11.005
Ozturk, M., Metin, M., Altay, V., De Filippis, L., Ünal, B. T., Khursheed, A., et al. (2021). Molecular Biology of cadmium toxicity in Saccharomyces cerevisiae. Biol. Trace Elem. Res. 199, 4832–4846. doi:10.1007/s12011-021-02584-7
Pari, L., and Murugavel, P. (2007). Diallyl tetrasulfide improves cadmium induced alterations of acetylcholinesterase, ATPases and oxidative stress in brain of rats. Toxicology 234, 44–50. doi:10.1016/j.tox.2007.01.021
Richter, P., Faroon, O., and Pappas, R. S. (2017). Cadmium and cadmium/zinc ratios and tobacco-related morbidities. Int. J. Environ. Res. public health 14, 1154. doi:10.3390/ijerph14101154
Sabolić, I. (2006). Common mechanisms in nephropathy induced by toxic metals. Nephron Physiol. 104, p107–p114. doi:10.1159/000095539
Satarug, S., and Moore, M. R. (2004). Adverse health effects of chronic exposure to low-level cadmium in foodstuffs and cigarette smoke. Environ. Health Perspect. 112, 1099–1103. doi:10.1289/ehp.6751
Schmidt, T., Bergner, A., and Schwede, T. (2014). Modelling three-dimensional protein structures for applications in drug design. Drug Discov. Today 19, 890–897. doi:10.1016/j.drudis.2013.10.027
Seccon, A., Rosa, D. W., Freitas, R. A., Biavatti, M. W., and Creczynski-Pasa, T. B. (2010). Antioxidant activity and low cytotoxicity of extracts and isolated compounds from Araucaria angustifolia dead bark. Redox Rep. 15, 234–242. doi:10.1179/135100010x12826446921789
Smeets, K., Opdenakker, K., Remans, T., Van Sanden, S., Van Belleghem, F., Semane, B., et al. (2009). Oxidative stress-related responses at transcriptional and enzymatic levels after exposure to Cd or Cu in a multipollution context. J. Plant Physiol. 166, 1982–1992. doi:10.1016/j.jplph.2009.06.014
Sobhan, P. K., Seervi, M., Deb, L., Varghese, S., Soman, A., Joseph, J., et al. (2013). Calpain and reactive oxygen species targets Bax for mitochondrial permeabilisation and caspase activation in zerumbone induced apoptosis. PLoS One 8, e59350. doi:10.1371/journal.pone.0059350
Spruill, M. D., Song, B., Whong, W. Z., and Ong, T. (2002). Proto-oncogene amplification and overexpression in cadmium-induced cell transformation. J. Toxicol. Environ. Health A 65, 2131–2144. doi:10.1080/00984100290071379
Trott, O., and Olson, A. J. (2010). AutoDock Vina: Improving the speed and accuracy of docking with a new scoring function, efficient optimization, and multithreading. J. Comput. Chem. 31, 455–461. doi:10.1002/jcc.21334
Umar Ijaz, M., Batool, M., Batool, A., Al-Ghanimd, K. A., Zafar, S., Ashraf, A., et al. (2021). Protective effects of vitexin on cadmium-induced renal toxicity in rats. Saudi J. Biol. Sci. 28, 5860–5864. doi:10.1016/j.sjbs.2021.06.040
Une, H. D., Sarveiya, V. P., Pal, S. C., Kasture, V. S., and Kasture, S. B. (2001). Nootropic and anxiolytic activity of saponins of Albizzia lebbeck leaves. Pharmacol. Biochem. Behav. 69, 439–444. doi:10.1016/s0091-3057(01)00516-0
Valko, M., Morris, H., and Cronin, M. (2005). Metals, toxicity and oxidative stress. Curr. Med. Chem. 12, 1161–1208. doi:10.2174/0929867053764635
Vaux, D. L. (1993). Toward an understanding of the molecular mechanisms of physiological cell death. Proc. Natl. Acad. Sci. U. S. A. 90, 786–789. doi:10.1073/pnas.90.3.786
Waalkes, M. (2003). Cadmium carcinogenesis. Mutat. Research/Fundamental Mol. Mech. Mutagen. 533, 107–120. doi:10.1016/j.mrfmmm.2003.07.011
Wass, M. N., Kelley, L. A., and Sternberg, M. J. E. (2010). 3DLigandSite: Predicting ligand-binding sites using similar structures. Nucleic Acids Res. 38, W469–W473. doi:10.1093/nar/gkq406
Weber, M., Trampczynska, A., and Clemens, S. (2006). Comparative transcriptome analysis of toxic metal responses in Arabidopsis thaliana and the Cd2+-hypertolerant facultative metallophyte Arabidopsis halleri. Plant, Cell Environ. 29, 950–963. doi:10.1111/j.1365-3040.2005.01479.x
Wong, K. C., Cao, S., Dong, X., Law, M. C., Chan, T. H., and Wong, M. S. (2017). (- epiafzelechin protects against ovariectomy-induced bone loss in adult mice and modulate osteoblastic and osteoclastic functions in vitro. Nutrients 9, 530. doi:10.3390/nu9050530
Wu, B., Song, H., Fan, M., You, F., Zhang, L., Luo, J., et al. (2020). Luteolin attenuates sepsis-induced myocardial injury by enhancing autophagy in mice. Int. J. Mol. Med. 45, 1477–1487. doi:10.3892/ijmm.2020.4536
Wu, L. L., Chiou, C. C., Chang, P. Y., and Wu, J. T. (2004). Urinary 8-OHdG: A marker of oxidative stress to DNA and a risk factor for cancer, atherosclerosis and diabetics. Clin. Chim. Acta 339, 1–9. doi:10.1016/j.cccn.2003.09.010
Yang, P.-M., Chiu, S.-J., Lin, K.-A., and Lin, L.-Y. (2004). Effect of cadmium on cell cycle progression in Chinese hamster ovary cells. Chemico-Biological Interact. 149, 125–136. doi:10.1016/j.cbi.2004.08.001
Keywords: docking studies, antioxidant, COX-2 inhibitor, anti-inflammatory, AutoDock Vina
Citation: Shahzadi A, Tariq N, Sonmez H, Waquar S, Zahid A, Javed MA, Ashraf MY, Malik A and Ozturk M (2023) Potential effect of luteolin, epiafzelechin, and albigenin on rats under cadmium-induced inflammatory insult: In silico and in vivo approach. Front. Chem. 11:1036478. doi: 10.3389/fchem.2023.1036478
Received: 04 September 2022; Accepted: 19 January 2023;
Published: 01 March 2023.
Edited by:
James Adams, Independent Researcher, Benicia, CA, United StatesReviewed by:
Meenakshi Rana, Uttarakhand Open University, IndiaSubhash Chandra, Kumaun University, India
Copyright © 2023 Shahzadi, Tariq, Sonmez, Waquar, Zahid, Javed, Ashraf, Malik and Ozturk. This is an open-access article distributed under the terms of the Creative Commons Attribution License (CC BY). The use, distribution or reproduction in other forums is permitted, provided the original author(s) and the copyright owner(s) are credited and that the original publication in this journal is cited, in accordance with accepted academic practice. No use, distribution or reproduction is permitted which does not comply with these terms.
*Correspondence: Arif Malik, YXJpZnVhZkB5YWhvby5jb20=