- 1School of Pharmacy, Shanghai University of Traditional Chinese Medicine, Shanghai, China
- 2Shuguang Hospital, Shanghai University of Traditional Chinese Medicine, Shanghai, China
Clusiaceae plants contain a wide range of biologically active metabolites that have gotten a lot of interest in recent decades. The chemical compositions of these plants have been demonstrated to have positive effects on a variety of ailments. The species has been studied for over 70 years, and many bioactive compounds with antioxidant, anti-proliferative, and anti-inflammatory properties have been identified, including xanthones, polycyclic polyprenylated acylphloroglucinols (PPAPs), benzophenones, and biphenyls. Prenylated side chains have been discovered in many of these bioactive substances. To date, there have been numerous studies on PPAPs and xanthones, while no comprehensive review article on biphenyls from Clusiaceae has been published. The unique chemical architectures and growing biological importance of biphenyl compounds have triggered a flurry of research and interest in their isolation, biological evaluation, and mechanistic studies. In particular, the FDA-approved drugs such as sonidegib, tazemetostat, daclatasvir, sacubitril and trifarotene are closely related to their biphenyl-containing moiety. In this review, we summarize the progress and development in the chemistry and biological activity of biphenyls in Clusiaceae, providing an in-depth discussion of their structural diversity and medicinal potential. We also present a preliminary discussion of the biological effects with or without prenyl groups on the biphenyls.
1 Introduction
Clusiaceae family is extensively used in traditional medicine for treating a number of diseases which include cancer, inflammation, and infection (Acuna et al., 2009). There are many species belonging to the Clusiaceae family, such as Clusia L., Garcinia L., Pentadesma Sabine and so on. Among them, Garcinia is a representative species comprising of about 400 recognized plants and is extensively dispersed throughout tropical and subtropical Asia, Africa, and America (2022). The chemical compositions of these plants contain a variety of valuable natural products with associated beneficial and healing properties such as anti-tumor (Fan et al., 2015), anti-obesity (Hasegawa, 2001; Kim et al., 2004), antibacterial (Negi et al., 2008; Auranwiwat et al., 2014), antioxidant (Farombi et al., 2013), antimalarial (Elfita et al., 2009), and so on (Grossman and Yang, 2020; Spontoni do Espirito Santo et al., 2020; Kalita et al., 2021). As a result, a considerable bunch of studies have been conducted to evaluate all types of phytochemical compositions from Clusiaceae, including polycyclic polyprenylated acylphloroglucinols (PPAPs) (Tian et al., 2016; Chen et al., 2020), xanthones (Nilar and Harrison, 2002; Rukachaisirikul et al., 2003b; Elfita et al., 2009), benzophenones (Elya et al., 2006), flavonoids (Nguyen et al., 2021), biphenyls (Auranwiwat et al., 2021) and others (Ly Dieu et al., 2012; Jiang et al., 2014).
Biphenyls are odorant and white crystals typically consisting of two adjacent benzene rings connected by a C1-C1’ bond (Kwong et al., 2017) (Figure 1). The biphenyl is normally used as an advantaged and functional moiety in the field of drug design and the process of medicinal advancement (Lu et al., 2015; Anusha et al., 2016; Tarade et al., 2017; Delgado et al., 2019). They have the potentials to be developed as prospecting therapeutic agents for a range of diseases (Chen et al., 2022), such as the dual inhibitors of acetylcholinesterase and butyrylcholinesterase (Wang et al., 2017) for Alzheimer’s disease (Inuzuka et al., 2022), the transient receptor potential vanilloid type 1 (TRPV1) antagonist (Oka et al., 2018), the human immunodeficiency virus -1 (HIV-1) nonnucleoside reverse transcriptase inhibitor (Sang et al., 2019). In addition, they could also be applied as auxiliary parts to enhance the biological properties (Ding et al., 2015) or act as chiroptical probes (Santoro et al., 2020). It is noted that some FDA-approved drugs, such as sonidegib, tazemetostat, daclatasvir, sacubitril, and trifarotene (Figure 2), have been developed in recent years with biphenyl as the core structure (Bhutani et al., 2021).
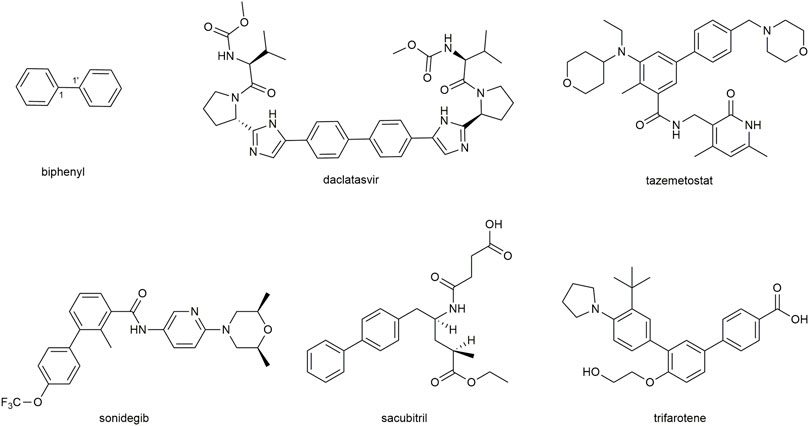
FIGURE 1. Basic structure of biphenyl and five FDA-approved drugs containing biphenyl structure from 2015-June 2020.
So far, Clusiaceae has yielded a variety of critical compounds with remarkable activity, such as α-mangostin (Gutierrez-Orozco and Failla, 2013), gambogic acid (Banik et al., 2018), oblongifolin C (Li et al., 2018) and so on. The majority of these key compounds belong to the structurally unique PPAPs and xanthones, which are the two principal metabolites (Chantarasriwong et al., 2010; Li et al., 2016; Xu et al., 2020). Biphenyls are secondary metabolites regularly yielded especially from individual species of the Garcinia genus (Chen et al., 2019). In addition, biphenyl can be classified as a phytoalexin that is produced in response to pathogen assault (Zhou et al., 2016) and it can be extracted from a wide range of plants with related bioactive ability (Teixeira et al., 2006; Yan et al., 2018; Ma et al., 2019; Yuan et al., 2019; Song et al., 2021). Because biphenyls have received little attention compared to PPAPs and xanthones in Clusiaceae, a comprehensive review of biphenyl chemicals in Clusiaceae is currently unavailable. Since 1974, a total of 69 new biphenyls (Tables 1–5) have been identified from Clusiaceae, all of which are structurally distinctive and possess various bioactive effects. It is obvious that a summary of the biphenyls in Clusiaceae would be beneficial for the utilization of these compounds. Therefore, we would like to sum up the development of biphenyls in the chemistry and biological activity from Clusiaceae, with the aim to provide a discussion of their structural diversity and medicinal potential.
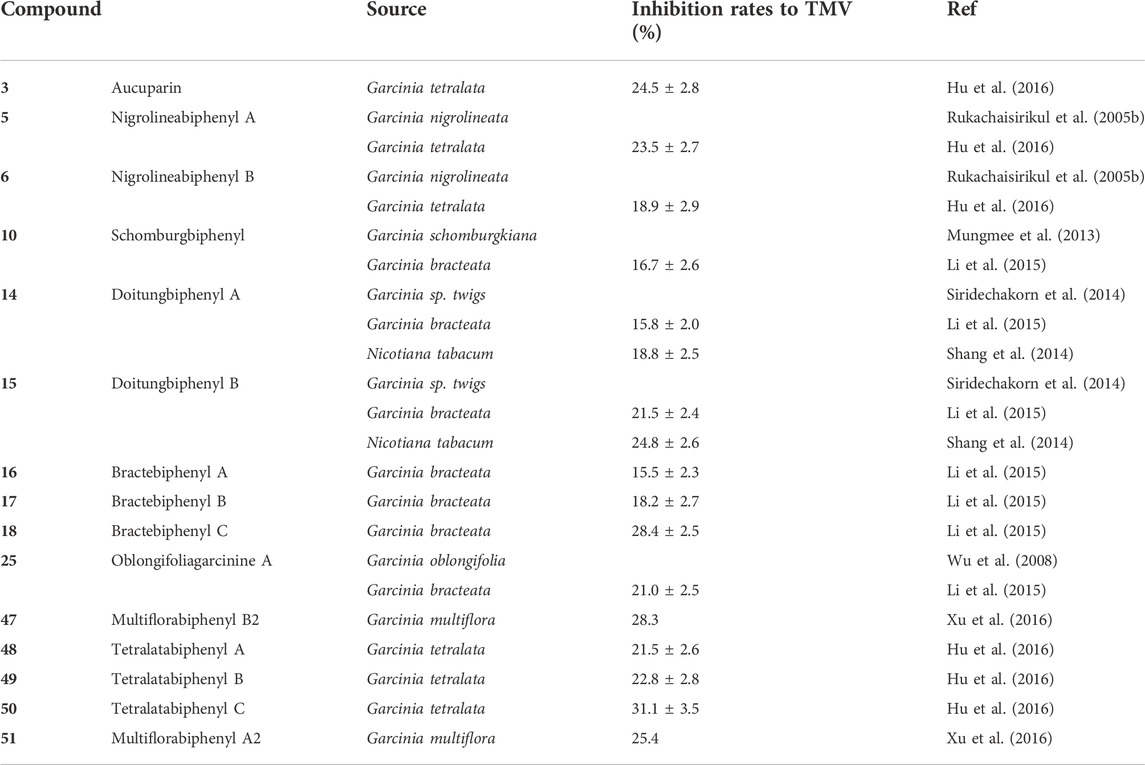
TABLE 3. Biphenyls from Clusiaceae and associated anti-tobacco mosaic virus (anti-TMV) activity at the concentration of 20 μM.
2 Diverse biphenyls from Clusiaceae and their bioactivity
Tables 1–5 outlines the biphenyls isolated from Clusiaceae and their bioactivities. As shown in Table 1, almost two-thirds of the compounds were examined for cytotoxicity, however, only a few of them were revealed with moderate bioactivities. Nine compounds (3, 5–6, 16–18, 29, 31 and 37) showed relatively good potency against the cells tested, with IC50 values below 10 μM. Tables 2, 3 list the substances that were tested for antibacterial and anti-tobacco mosaic virus (anti-TMV) activity. Compounds 3, 6, and 45 performed well in the anti-microbial test, with MIC values of roughly 20 μg/mL against corresponding bacteria. In terms of the capacity to inhibit tobacco mosaic virus, two-thirds of the compounds examined exhibited good activity, with inhibition rates greater than 20%, while the positive control ningnanmycin generally showed inhibition rates in the range of 30%–35% (Shang et al., 2014; Hu et al., 2016). Among them, compounds 18, 47 and 50 showed better inhibition rates, which were close to 30%. Tables 4, 5 present the results of a small number of compounds that were tested for anti-rotavirus and anti-HIV efficacy. It is obvious that all the substances under study have some potential when their therapeutic indices (TIs) or the selective indices (SIs) are compared to the corresponding positive controls. The TI value of the anti-rotavirus positive control Ribavirin is about 20 (Gao et al., 2016), and the SI value of the anti-syncytium positive control azidothymidine is larger than 2.73 (Chaturonrutsamee et al., 2018).
In the following section, the origins of the compounds, their structures, and an extensive description of their biological activities are presented based on the Tables. The isolated compounds are organized based on the structures and their bioactivities.
2.1 Biphenyls with associated cytotoxicities
A total of 39 compounds (Figure 3) were isolated and assayed for cytotoxicity from Clusiaceae. Three of them were from Clusia paralicola G. Mariz and the rest of the compounds were from Garcinia genus. The cytotoxicity of the substances is detailed below by categorizing them into three groups based on the number of hydroxyl groups and substitution sites on the structures.
2.1.1 Biphenyls with 2,3,4-trihydroxyl group
The biphenyl structures in this section contain three hydroxyl groups at the C2, C3, and C4 positions, and their cytotoxicities have been evaluated on several cancer cell lines.
A biphenyl was isolated from the methanol extract of twigs and leaves of Garcinia bancana MIQ. from Southern Thailand, which was determined as [1.1′-biphenyl]-2-(3-methyl-2-butenyl)-3-methoxy-4.4′,5,6-tetraol (1) (Figure 3) (Rukachaisirikul et al., 2005a). The source of biphenyl 1, Garcinia bancana, is a member of the Clusiaceae family that is native to Southeast Asian countries including Thailand, Malaysia and Indonesia (Whitmore, 1973; Rukachaisirikul et al., 2005a). The n-hexane extract of the Garcinia bancana leaves showed antioxidant activity according to 1,1-diphenyl-2-picrylhydrazyl (DPPH) and ferric reducing antioxidant power (FRAP) tests (Putri et al., 2018). In the research of Auranwiwat and co-workers, cytotoxicity against KB cells of 1 was tested, but 1 was inactive to both cells with IC50 > 50 µM (Table 1) (Auranwiwat et al., 2021).
Garcinia cylindrocarpa Kosterm is native to Indonesia’s Maluku Island, where it is known as “Kogbirat” and is used as a fever remedy in traditional Indonesian medicine (Sukandar et al., 2016a). Tip-pyang and others explored Garcinia cylindrocarpa for sustained research on bioactive compounds of Garcinia and attained cylindrobiphenyl B (2) (Figure 3). By utilizing the 3-(4,5-dimethylthiazol-2-yl)-2,5-diphenyl-2,3-dihydro-1H-tetrazol-3-ium bromide (MTT) colorimetric technique, biphenyl 2 showed no activity against KB, HeLa S3, MCF-7, HepG2, or HT-29 cancer cell lines. (Table 1) (Sukandar et al., 2018).
Aucuparin (3) (Figure 3), a constitutive component from the heartwood of Sorbus aucuparia (Erdtman et al., 1961), also could be extracted and separated from the Garcinia species (Chen et al., 2004; Mungmee et al., 2013; Hu et al., 2016). Although the structure of 3 is very simple, many studies have explored its biological properties such as antifungal (Cortez et al., 1998; Song et al., 2021), anti-TMV (Hu et al., 2016), anti-inflammatory, as it is a natural phytoalexin (Kokubun et al., 1995). Given that 3 has attracted a lot of attention and has been studied by many groups, some of its biological features including cytotoxicity, antibacterial activity and anti-inflammatory were summarized individually in the following sections.
A lot of groups have probed its cytotoxic effects against diverse cell lines, and 3 showed different levels of cytotoxicity against P-388 (IC50 = 3.21 μg/mL (Chen et al., 2004)), HT-29 (IC50 = 5.39 μg/mL (Chen et al., 2004)), XF498, A549, SK-OV-3, SK-MEL-2, HCT15 (IC50 > 30 µM (Kim et al., 2009; Kim et al., 2016; Suh et al., 2017)), KB, HeLa S3, HepG2 and MCF-7 (IC50 > 100 µM (Sukandar et al., 2016b)) cells (Table 1).
For the synthesis of oxygenated biphenyls, the tandem Michael addition reaction followed by aromatization reaction was developed by Chittimalla group. They successfully applied this methodology in the synthesis of 3 (Scheme 1) (Chittimalla et al., 2015). At the beginning, cyclohexa-2,4-dienone (3a) and phenylboronic acid (3b) were used as the starting materials to make 3c, which underwent quantitative methylation by reacting with MeI and K2CO3 in acetonitrile to give trimethoxybiphenyl 3d. After selective demethylation, biphenyl 3 was accomplished in 82% yield.
Garcinia linii is an endemic evergreen tree that sprang up in Langyu land along with the Green island of Taiwan and contains xanthones, biphenyls, and other compounds. Chen et al. (2019). Chen group made significant contributions to the separation of biphenyls from the root of Garcinia linii. They analyzed and identified garcibiphenyl C (4) (Figure 3), by way of spectral analyses in 2006 (Chen et al., 2006). Biphenyl 4 was tested for its cytotoxic activity against P388, KB, Col-2, BCA-1, Lu-1, ASK, NALM-6, but it was found to be ineffective against these cells (Table 1) (Ito et al., 2013; Pailee et al., 2018). With respect to the synthesis of 4, the strategy reported by Schmidt group was featured with protecting-group free strategy. (Scheme 2). At the outset, bromoarene (4b) was produced from 2,6-dimethoxyphenol (4a) via the bromination of 4a (Lee et al., 2004). Then 4b was reacted with (4-hydroxyphenyl) boronic acid (4c) in the presence of Pd/C catalyst in water to give 4 in 52% yield. In addition, this research reported that it could get access to diverse biphenyls via Suzuki−Miyaura cross coupling (Schmidt and Riemer, 2014).
Garcinia nigrolineata Planch. Ex T. Anderson, known as kandis in the local, is widely distributed in Malaysia (Mohd Norfaizal et al., 2014). Many xanthones have been isolated (Rukachaisirikul et al., 2003a; Rukachaisirikul et al., 2003c) from this plant. On the other hand, only two biphenyls, nigrolineabiphenyls A-B (5–6) (Figure 3) were obtained from Garcinia nigrolineata by Rukachaisirikul in 2005 (Rukachaisirikul et al., 2005b). The cytotoxicities of 5-6 on a series of tumor cell lines were studied including SW620, BT474, HepG2, CHAGO, NB4, A549, NALM-6 and so on (Table 1) (Ito et al., 2013; Mungmee et al., 2013; Zhou et al., 2015). Biphenyls 5-6 showed weak to moderate inhibitory activity towards most of the cell lines mentioned above, while 6 displayed stronger cytotoxicity against SW620 cells with the IC50 values of 0.36 µM (Mungmee et al., 2013).
The first investigation to identify the phytochemical of Garcinia nuntasaenii Ngerns. and Suddee attained the isolation of garcinuntabiphenyls A-C (7–9) (Figure 3), which were evaluated for cytotoxicity (Chaturonrutsamee et al., 2018). Garcinia nuntasaenii is distributed in northeastern Thailand and commonly named “Chang-nga-ek”. It is a dioecious shrub growing with white flowers and green fruits. Its roots could be used for relieving muscle pain in Thai folk medicine (Ngernsaengsaruay and Suddee, 2016). The cytotoxic effects of biphenyls (7–9) against a panel of cultured mammalian cancer cell lines, including P-388, KB, HT-29, MCF-7, A549, ASK, were generally weak. Only the cytotoxicity of 8 to P-388 (IC50 = 37.87 µM) and KB (IC50 = 41.94 µM), as well as 9 to KB (IC50 = 39.04 µM) were detected and quantified (Table 1).
A new biphenyl, schomburgbiphenyl (10) (Figure 3), was obtained as a white solid from the wood of Garcinia schomburgkiana Pierre and determined as [1.1′-biphenyl]-2-(3-hydroxy-3-methylbutyl)-3-methoxy-4.4′,5-triol (Mungmee et al., 2013). Its source, Garcinia schomburgkiana, is a medium-sized tree widely scattered in Vietnam, Laos, Cambodia, and Thailand and called Ma-dan in Thailand. It has previously been reported to possess the activity of DNA polymerase Inhibitory, antioxidant and anti-diabetic properties (Le et al., 2016; Gonsap and Vongsak, 2019; Thummajitsakul et al., 2020). Biphenyl 10 was analyzed for its cytotoxic activity against five human cancer cell lines, but it was not active in the test (Table 1) (Mungmee et al., 2013). Another study carried out by Hu also tested its cytotoxicities using the other five kinds of tumor cell lines (NB4, A549, SHSY5Y, PC-3, MCF-7) in 2015. And it could be known that all IC50 values were above 10 µM from this research (Table 1) (Li et al., 2015).
Investigating the EtOAc extract of the stems of Garcinia schomburgkiana led to the fractionation and the elucidation of a new biphenyl derivative, schomburgbiphenyl B (11) (Figure 3), as colorless oil. The compound (11) was screened for the ability to repress the growth of several leukemia cell lines containing Jurkat, NALM-6, K562 and HPB-ALL. It was found that biphenyl 11 showed limited cytotoxicity against NALM-6 cells, with cell viability ranging from 73% to 80% at the concentration of 50 µM (Table 1) (Ito et al., 2013).
Two biphenyls, garciosines A-B (12–13) (Figure 3), were isolated, established, and confirmed from Garcinia speciose Wall by employing extensive spectroscopic methods and single crystal X-ray diffraction analysis (Pailee et al., 2018). Garcinia speciose, namely “PhaWaa” in Thailand, is an indigenous plant that can be used for the therapy of blood disorders, skin wounds, inflammation, laxative and so on (Sakunpak and Panichayupakaranant, 2012; Sangsuwon and Jiratchariyakul, 2015). Garciosines A-B (12–13) were examined with cytotoxic properties against a panel of cancer cell lines (P388, KB, Col-2, BCA-1, Lu-1, ASK, HeLa, S-3, MCF-7, HepG2, HT-29). The activity of 12–13 to restrain these tumor cells was not prominent. The IC50 value of 12 against KB cells was 89.05 ± 3.11 µM, and the IC50 value of 13 against P388 cells was 18.48 µM (Table 1) (Pailee et al., 2018; Sukandar et al., 2018).
The separation of doitungbiphenyls A (14) and B (15) (Figure 3) from the acetone extract of the twigs of Garcinia sp. was achieved for the first time by Laphookhieo and co-works. By virtue of 1D and 2D NMR spectroscopy as well as HR-EI-MS, they were elucidated to contain prenylated chains in the skeleton. Obviously, 15 is formed from the methylation of 14. Their bioactivities to suppress the reproduction of the tumor cells were tested by using KB and MCF-7 cell lines. However, the two compounds were inactive against the two cell lines, and 15 displayed weak inhibition against the MCF-7 cell line with an IC50 value of 102.52 μM (Table 1) (Siridechakorn et al., 2014).
2.1.2 Biphenyls with 2,3-dihydroxyl group
The structural similarity of the compounds described in this section is characterized by the presence of two adjacent hydroxyl substituents at the C2, C3 positions. Their cytotoxicities against diverse tumor cell lines were summarized in the following.
Mixed forests of Garcinia bracteata C. Y. Wu ex Y. H. Li are commonly distributed on limestone hills of Yunnan and Guangxi Province of China (Li et al., 2011). To search for new bioactive metabolites, Hu et al. examined the chemical components of the twigs from Garcinia bracteata. Consequently, three biphenyls, namely bractebiphenyls A-C (16–18) (Figure 3), were extracted and separated by using 70% aqueous acetone. Their structures were elucidated by spectroscopic methods, such as UV spectrum, IR spectrum and 1D and 2D NMR techniques. As for the experimental data of cytotoxic abilities, three compounds displayed moderate cytotoxicities against five cell lines (NB4, A549, SHSY5Y, PC-3, and MCF-7), with IC50 values typically below 10 µM. Among these compounds, biphenyl 18 was the most potential with high cytotoxicities against A549 and PC-3 cells (IC50 = 3.6 and 2.7 µM, respectively) (Table 1) (Li et al., 2015).
For the aim of searching for undiscovered natural products of Garcinia mckeaniana Craib, the constituents belonging to the flower and twig extracts of this plant were investigated by Auranwiwat and others and a biphenyl (19) was found (Figure 3) in 2021 (Auranwiwat et al., 2021). Garcinia mckeaniana, also called Xen mu, is a common plant that is widespread in the tropical secondary forests of Sapa town and Son La province of Vietnam in particular. So far, a few studies have been conducted on the phytochemistry and biological properties of Garcinia mckeaniana (Auranwiwat et al., 2016; Ha et al., 2021; Nguyen et al., 2021; Thi Thu et al., 2021). The biphenyl (19) was denominated as mckeaninabiphenyl and had been synthesized previously (Sato and Mikawa, 1960). This was the first time that it was reported as a natural phytochemical. Its structure is symmetrically featured as 4.4′-dihydroxy-2.2′-dimethoxybiphenyl, which is like biphenyls that were isolated from fungi formerly (Li et al., 2017; Zhu et al., 2019). Its cytotoxicity was tested, but it was inactive against KB cells with IC50 value > 50 µM in assays (Table 1).
Yuan group systematically discovered and structurally characterized Garmultines A-C (20–22) (Figure 3) from Garcinia multiflora Champion ex Bentham (Tian et al., 2017), which contains PPAPs with apoptosis-inducing or cytotoxic properties (Chien et al., 2008; Liu et al., 2010; Fan et al., 2015; Tian et al., 2016; Yang et al., 2020) and others (Wang et al., 2018). Compounds 21 and 22 are proved to be isomers. The cytotoxic capacities of the three novel natural biphenyls (20–22) were evaluated (Tian et al., 2017). Their cytotoxicities were tested on five human tumor cell lines (HeLa, MCF-7, A-549, MGC-803, and COLO-205), which turned out that these biphenyls (20–22) were moderate against the five cells with the IC50 values ranging from 12.4 to > 40 μg/mL (Table 1). It concluded that an additional prenyl chain at C9 (20) could make compound 20 more active in inhibiting tumor cells.
Two biphenyls, multiflorabiphenyls B1 (23) and multiflorabiphenyl D (24) (Figure 3) were obtained from the acetone-extracted leaves of Garcinia multifora through bioassay-directed fractionation. The cytotoxic abilities of compounds 23–24 against five human cancer cell lines (HeLa, SGC7901, TE1, HCT116, and Capan 2) were evaluated with IC50 values > 10 µM (Table 1) (Fu et al., 2015).
Garcinia oblongifolia Champ. ex Benth. is a medium-sized shrub mainly distributed in tropical areas of southern China and northern Vietnam and has been used to reduce the pains of burns and diminish inflammation (Liu et al., 2016). Xanthones, PPAPs and other bioactive components have been found in this plant (Hamed et al., 2006; Zhang et al., 2016). Four new biphenyls, oblongifoliagarcinines A–D (25–28) (Figure 3), were acquired from samples of Garcinia oblongifolia collected in Guangxi province of China and structurally determined on the basis of spectroscopic analysis. The cytotoxic effects of 25–28 were assessed against the tumor cell lines A549 and HL-60 (Table 1). It turned out that biphenyls 25–28 were inactive toward these two cancer cells in vitro (Wu et al., 2008). Whereas, compounds 25 and 26 showed weak influence on the growth of HeLa, MCF-7, A-549, MGC-803, and COLO-205 cells with IC50 values ranging from 18.4 to > 40 μg/mL (Tian et al., 2017).
The new biphenyl (29) (Figure 3) was obtained and confirmed as 3-methoxy-5-methoxycarbonyl-4-hydroxy-biphenyl after the extraction, separation and purification from the stems of Garcinia oligantha Merrill (Liu et al., 2015). Garcinia oligantha is a tall shrub mainly growing in the Guangdong and Hainan provinces of China and northern Vietnam (Li et al., 2011). The plant was usually explored for the bioactive xanthones possessing diverse activity such as cytotoxic property and suppressing convulsant behavior (Tang et al., 2016; Tang et al., 2019; Gong et al., 2020; Yang et al., 2021). The biphenyl (29) was measured for its cytotoxic effects against NB4, A549, SHSY5Y, PC-3 and MCF-7 tumor cells. The results showed 29 could modestly exert the repression toward tumor proliferation with IC50 values of 7.1, 6.2 and 4.8 µM against SHSY5Y, A549 and MCF-7 cells, respectively (Table 1) (Liu et al., 2015).
Schomburgbiphenyl A (30) (Figure 3) was also extracted as colorless oil from the stems of Garcinia schomburgkiana at the same time with Schomburgbiphenyl B (11). Like compound 11, 30 was accessed for the cytotoxicity against a series of leukemia cell lines containing Jurkat, NALM-6, K562 and HPB-ALL cells. It (30) was not very effective to repress the proliferation of these tumor cells in study (Table 1) (Ito et al., 2013).
2.1.3 Biphenyls with 2, 4-dihydroxyl group
Biphenyls with bis-hydroxyl substituted at the C2 and C4 positions and their cytotoxic testing results are outlined in this section.
Three novel biphenyls clusiparalicolines A-C (31–33) (Figure 3) were obtained by the bioassay-guided fractionation from the roots of Clusia paralicola G. Mariz (Seo et al., 1999), which is a native species distributed in Brazil (POWO, 2022). These three biphenyls were tested for cytotoxicity against KB cells. The results showed that biphenyls 31–33 could inhibit the proliferation of KB cells modestly (Table 1). The report also assessed the DNA strand scission ability to evaluate their antineoplastic potential. Compounds 31 and 32 demonstrated considerable DNA strand scission action. The DNA relaxation rates were 77% and 65%, respectively, at the concentration of 2.5 g/mL (the rate of bleomycin at 0.025 g/mL was about 50%). Compound 33 was observed to be inactive. Looking into their structures, the ring generated by cyclization involving the prenyl and hydroxyl groups distinguishes compounds 31 and 32. And compound 33 lacks the catechol and geranyl moiety in comparison to compounds 31 and 32. As a result, it is possible that the 3,3-dimethylallyl group and the hydroxyl group of compound 31 have no effect on DNA strand scission activity, because there was no significant difference in DNA strand scission activity between compounds 31 and 32. Furthermore, based on previous work by Wall and Wani’s group (Huang et al., 1996), it is confirmed that the presence of the catechol and geranyl moiety in 31 and 32 may be connected with DNA strand-scission activity.
The first synthesis of biphenyl 31 was successfully finished in 2002 by Fukuyama’s team (Scheme 3). The design of two geranylated and prenylated phenols is needed for the synthesis of clusiparalicoline A (31) since it is not entirely symmetrical. Beginning with O-dimethylphloroglusinol 31a, the preparation of left part was undertaken. After the protection, bromination, C-alkylation, removal of the TBDMS and subsequent triflation, the left part of 31g with a triflate group was provided quantitatively. Starting with the commercially available 3,4-dihydroxybenzaldehyde 31h, the protection of the catechol group, Baeyer–Villiger oxidation of the aldehyde, and reduction were performed to afford compound 31i. Then, the generated phenolic group was protected as an allyl ether, and subsequent bromination, deallylation, palladium-catalyzed Stille reaction yielded compound 31l. Next, it was successful to convert the bromide 31l into a pinacol boronic ester 31m using the Suzuki-Miyaura protocol. Following that, Suzuki coupling between 31g and 31m was carried out smoothly, yielding the coupling product 31n in 90% yield. At last, following the deprotection of all MOM groups and separation by HPLC, the desired biphenyl 31 was synthesized (Takaoka et al., 2002).
Cylindrobiphenyl A (34) was found by Tip-pyang and others during the investigation of exploring Garcinia cylindrocarpa (Figure 3). Its (34) cytotoxicity against KB, HeLa S3, MCF-7, Hep G2, or HT-29 cancer cell lines was assayed by MTT in vitro. But it was inactive to all tested cells (Table 1) (Sukandar et al., 2018).
Garcibiphenyls A-B (35–36) (Figure 3) were separated and determined from Garcinia linii by Chen group in 2004 (Chen et al., 2004). Compounds 35 and 36 were assessed to figure out their cytotoxicities against two tumor cells. Their ED50 value against P-388 were 10.2 μg/mL, 6.63 μg/mL, respectively. And the ED50 values of 35–36 against HT-29 were 13.5 μg/mL, 12.7 μg/mL, respectively (Table 1) (Chen et al., 2004).
Garcibenzopyran (37) (Figure 3) was found and identified with 35–36 from Garcinia linii during the same investigation conducted by Chen group (Chen et al., 2004). The ED50 values against P-388 and HT-29 cell lines of 37 were 3.98 μg/mL and 6.90 μg/mL, respectively, which supported that 37 could be slightly beneficial for anti-cancer and anti-proliferation therapy (Table 1) (Chen et al., 2004).
Multiflorabiphenyl C (38) (Figure 3), an isomer of multiflorabiphenyl D (24), was obtained from the leaves of Garcinia multifora. The cytotoxic activity of compound 38 against five human cancer cell lines (HeLa, SGC7901, TE1, HCT116, and Capan 2) was evaluated, and the results showed that its IC50 values were all above 10 µM (Table 1) (Fu et al., 2015).
Garciosine C (39) (Figure 3) was another biphenyl isolated and established from Garcinia speciose by extensive spectroscopic methods in 2018 (Pailee et al., 2018). The cytotoxic ability of garciosine C (39) was examined on a panel of cancer cell lines (P388, KB, Col-2, BCA-1, Lu-1, ASK, HeLa, S-3, MCF-7, HepG2, HT-29). In fact, biphenyl 39 cannot inhibit tumor cells effectively. The IC50 value of 39 against P388 cell was 32.36 µM. In addition, it was inactive against the other cell lines (Table 1) (Pailee et al., 2018; Sukandar et al., 2018).
2.1.4 Structure-activity relationship of biphenyls with associated cytotoxicity
A brief analysis of the structure-activity relationship for the cytotoxic activity of these functionalized biphenyls was discussed in this section based on the reference data that is currently available. In addition to the analyses mentioned above, the following discussion could be useful for the research of the biphenyls according to Table 1 and Figure 3.
Compounds 5 and 6 (Figure 3) were both derived from the Garcinia nigrolineata (Rukachaisirikul et al., 2005b). And it could be known from the research of Hu group and Suttisri group that compound 6 had a higher inhibitory effect on tested PC-3, MCF-7 and SW620 cells than that of compound 5 (Table 1) (Mungmee et al., 2013; Zhou et al., 2015). It could be inferred that the methoxyl substitution on R5 (C9) had a better influence on the bioactivity compared to the hydroxyl substitution. From the structures and their cytotoxicity of compounds 7 and 8, 7 and 9 (Figure 3 and Table 1), it indicated that methoxylation on the R6 (C10) or the R4 (C5) positions may improve the cytotoxic effect. Although the cytotoxic effects of compounds 14 and 15 were not greatly outstanding, it suggested that compound 14 was slightly superior to compound 15 (Table 1) (Siridechakorn et al., 2014). Thus, it is obvious that a meta-hydroxyl substitution at the 3,3-dimethylallyl chain in their structures may be more advantageous for activity. The comparison of biphenyls 16 and 17 suggested that hydroxylation of the terminal carbon of the 3,3-dimethylallyl group did not have much effect on their activity (Li et al., 2015). Similarly, the experimental data for compounds 25 and 26 showed that the 3,3-dimethylallyl substitution at the C9 position did not significantly influence activity (Tian et al., 2017).
2.2 Biphenyls with associated antibacterial activity
A total of 11 biphenyls (Figure 3 and Figure 4) isolated from Clusiaceae were evaluated for antibacterial activity, four of which (1, 3, 6, 15) were listed in the cytotoxicity section above. Except for compound 43 (Figure 4), which was produced from Clusia burlemarxii Bittrich, another 10 compounds were isolated and extracted from Garcinia. They were also described below according to their structures.
2.2.1 Biphenyls with 2,3,4-trihydroxyl or 2,3,5-trihydroxyl group
In addition to the cytotoxicity, several 2,3,4-trihydroxy-substituted biphenyls were also subjected to antimicrobial evaluation. The antimicrobial activity of these biphenyls and another undescribed 2,3,5-trihydroxybiphenyl are summarized in this section.
The biphenyl (1) (Figure 3) exhibited weak antibacterial activity to Methicillin-resistant Staphylococcus aureus (MRSA) with MIC values of 64 μg/mL (Rukachaisirikul et al., 2005a) (Table 2). In terms of antibacterial activity (Table 2), a study indicated that biphenyl 3 (Figure 3) could suppress Gram-positive bacteria, which showed antimicrobial activity against Bacillus subtilis with MIC values of 3.12 μg/mL and against S. aureus with a MIC value of 12.5 μg/mL, respectively. It has no effect on Gram-negative bacteria including Pseudomonas aeruginosa and Escherichia coli (MIC ≥100 μg/ml). Meanwhile, penicillin-resistant S. aureus (PRSA) strains (MIC = 6.25 μg/mL) seemed to be more sensitive to 3 than penicillin-susceptible S. aureus (PSSA) strains (MIC = 12.5 μg/mL), which was advantageous for clinical drug development. The observation that anti-bactericidal activity of 3 functionalized in a dose-dependent manner was proved by investigating the kinetics of bactericidal activity against S. aureus at six concentrations of 3 (0.25 ×, 0.5 ×, 1 ×, 2 ×, 4 × and 8 × the MIC). It was surprising that there was no regrowth of bacteria after monitoring for up to 24 h (Cortez et al., 2002). The suppressive degree of 3 against Mycobacterium tuberculosis 90 ± 221387 was also tested to evaluate its antitubercular activity and it turned out that 3 played a weak role in antitubercular effect (Chen et al., 2006). With respect to biphenyl 6 (Figure 3), a study tested the antibacterial activity of 6 against three different strains of Helicobacter pylori (H. pylori) (Table 2). The results indicated 6 could restrain H. pylori in a gentle manner with MIC values of 226.3 µM, 56.5 µM, 226.3 µM against H. pylori ATCC 43504, H. pylori DMST 20165 and H. pylori HP40, respectively (Nontakham et al., 2014). On the other hand, compound 15 (Figure 3) was indicated to show anti-staphylococcal activity with MIC values at 50 μg/mL towards series of S. aureus strains (Table 2) (Zheng et al., 2021).
Two previously unknown biphenyls, garciesculenbiphenyls A-B (40–41) (Figure 4) were recently isolated and identified from Garcinia esculenta Y. H. Li, an endemic tree mainly distributed in Yunnan province of China (Zhu et al., 2014). Compounds 40 and 41 are two chemicals that are structurally related. Compound 41 could be converted to 40 after oxo-cyclization. These two compounds were tested for antibacterial activity against MSSA-Newman, two MRSA strains USA300 LAC and USA400 MW2, and VISA-Mu50 (Table 2). As it revealed, compound 40 presented weak anti-staphylococcal activity with MIC values at 50 μg/mL, whereas compound 41 did not show obvious anti-staphylococcal activity (Zheng et al., 2021).
2.2.2 Biphenyls with 2, 3-dihydroxyl group
The compound 42 (Figure 4) was isolated from Garcinia cowa Roxb. Garcinia cowa, also known as Cha muang in Thailand. It is a plant that can produce a variety of bioactive substances and is used as an antipyretic folk medicine (na Pattalung et al., 1994; Likhitwitayawuid et al., 1998; Mahabusarakam et al., 2005; Panthong et al., 2006) (+)-Garciniacowol (42), a dimeric dihydrobenzopyran with highly symmetrical structure, was found and identified from the stembarks of Garcinia cowa (Siridechakorn et al., 2012). The antibacterial property of 42 was analyzed (Table 2) and the results showed that 42 was inert against Gram-positive bacteria such as S. aureus-TISTR 1466 and MRSA-SK1. On the other hand, 42 had a mild effect on Gram-negative bacteria such as Escherichia coli TISTR 780 and Salmonella typhimurium TISTR 292 with MIC values at 128 g/mL for both.
2.2.3 Biphenyls with 2, 4-dihydroxyl group
2,2-dimethyl-3,5-dihydroxy-7-(4-hydroxyphenyl)chromane (43) (Figure 4) was extracted and determined from the trunk of Clusia burlemarxii Bittrich, a shrub distributed in Brazil. The research carried out the preliminary tests in vitro to determine the inhibitory effect of biphenyl 43 against a series of bacteria. The results of the initial analysis revealed that biphenyl 43 significantly inhibited all tested Gram-positive bacteria which was stronger against Micrococcus luteus (MIC = 25 μg/mL−1) and S. aureus (MIC = 50 μg/mL−1). However, it was weaker against Bacillus subtilis and Streptococcus mutans with MIC values at 100 μg/mL−1. Additionally, it had no effect on Gram-negative bacteria (Table 2) (Ribeiro et al., 2011).
Garcibiphenyls D-E (44–45) (Figure 4) were discovered at the same time as garcibiphenyl C (4) from Garcinia linii by Chen group in 2006 (Chen et al., 2006). Regarding to biphenyls 44 and 45, their anti-tubercular effects in vitro were evaluated against Mycobacterium tuberculosis 90 ± 221387. Both of them showed moderate activity with MIC values at 50.3 ± 4.2 μg/mL, 25.4 ± 3.1 μg/mL, respectively (Table 2) (Chen et al., 2006).
(S)-3-hydroxygarcibenzopyran (46) (Figure 4) was found and identified simultaneously with biphenyls 4, 44–45 from Garcinia linii (Chen et al., 2006). It is apparent that 46 is the derivative of 37 with an additional hydroxyl on the pyran ring. Although the antibacterial effect of biphenyl 46 was investigated, the testing result displayed that biphenyl 46 could not inhibit Mycobacterium tuberculosis 90 ± 221387 effectively (MIC >100 μg/mL) (Table 2) (Chen et al., 2006).
2.2.4 Structure-activity relationship of biphenyls with antibacterial activity
According to the study of Xu group in 2021, it could be known that biphenyls 15 (Figure 3), 40 (Figure 4) had comparable antibacterial capacities. Furthermore, both biphenyl 15 and 40 were more active than compound 41 (Figure 4) (Zheng et al., 2021). Hence, it could assume that the antibacterial activity may be stronger when the 3,3-dimethylallyl chain was positioned at para-position of the hydroxyl group (C1) or cyclized with the hydroxyl group rather than at the ortho-position of hydroxyl group (C5). Conclusions could also be obtained by comparing the activity of compounds 44 and 45, 44 and 46 (Figure 4; Table 2): 1) 3-hydroxymethyl-3-methyl-but-2-enyl substitution might be more beneficial for antibacterial activity than 2-hydroxy-3-methyl-but-3-enyl substitution; 2) the cyclization of 2-hydroxy-3-methyl-but-3-enyl may impair the antibacterial abilities of the compounds.
2.3 Biphenyls with associated anti-TMV activity
The ability of 15 different substances (Figure 3 and Figure 5) to inhibit the tobacco mosaic virus was examined. And Garcinia genus was the source of all compounds. They can be separated into the following two major groups based on their structural features.
2.3.1 Biphenyls with 2,3,4-trihydroxyl group
Despite with a simple structure, biphenyl 3 (Figure 3) has a wide range of activities. It could moderately suppress the tobacco mosaic virus with the inhibition rates of 24.5 ± 2.8% (Table 3) (Hu et al., 2016). Additionally, biphenyl 5 and 6 (Figure 3) derived from compound 3 presented modest anti-TMV properties. Their inhibition rates were 23.5 ± 2.7% and 18.9 ± 2.9% at the concentration of 20 μM, respectively (Table 3) (Hu et al., 2016). The anti-TMV ability of 10 (Figure 3) was also assessed at the concentration of 20 μM, which showed weak inhibitory capacity that its inhibition rate was 16.7 ± 2.6% (Table 3) (Li et al., 2015). With respect to the anti-TMV activity of biphenyls (14–15) (Figure 3), they were found to be modest against the tobacco mosaic virus with the inhibition rates ranging from 15.8 ± 2.0% to 24.8 ± 2.6% (Table 3) (Shang et al., 2014; Li et al., 2015).
One new biphenyl, multiflorabiphenyl B2 (47) (Figure 5), found in the twigs of Garcinia multiflora was analyzed and elucidated via spectral techniques. Assays were managed to evaluate its anti-TMV activity, which demonstrated that 47 was potential in anti-TMV with inhibition rates of 28.3%, which was similar to the inhibition rates of the positive control, ningnanmycin (a commercial product for plant disease in China, 33.5%) (Table 3) (Xu et al., 2016).
Three biphenyl derivatives, tetralatabiphenyls A–C (48–50) (Figure 5), were isolated and elucidated structurally for the first time from the ethanol extract of the twigs of Garcinia tetralata C. Y. Wu ex Y. H. Li. Garcinia tetralata is a plant peculiarly distributed in the south and southwest Yunnan province in China. Up to now, the phytochemical investigation of this plant was not paid much attention (Wang et al., 2008; Guo et al., 2011). To evaluate the bioactive characterizations of biphenyls 48–50, the activity to resist the tobacco mosaic virus was assayed. Tetralatabiphenyl C (50) showed the strongest activity among these biphenyls (48–50) with an inhibition rate of 31.1 ± 3.5% at the concentration of 20 μM, while the inhibition rates of 48 and 49 were 21.5 ± 2.6%, 22.8 ± 2.8% respectively (Table 3) (Hu et al., 2016).
2.3.2 Biphenyls with 2,3-dihydroxyl group
The anti-TMV inhibition rates of 16–18 (Figure 3) at the concentration of 20 µM were 15.5 ± 2.3%, 18.2 ± 2.7%, 28.4 ± 2.5% (Table 3), respectively, while 18 showed similar inhibition rates compared with ningnanmycin (30.2%). In addition, this study also tested the anti-TMV activity of biphenyl 25 (Figure 3) and it showed that 25 could inhibit tobacco mosaic virus moderately with the inhibition rate of 21.0 ± 2.5% at the concentration of 20 µM (Table 3) (Li et al., 2015). Multiflorabiphenyl A2 (51) (Figure 5) was another biphenyl isolated from the twigs of Garcinia multiflora. Like biphenyl 47 (Figure 5), it was subject to the tests for evaluating its anti-TMV activity. The results exhibited that it was a mild inhibitor for tobacco mosaic virus with inhibition rates of 25.4% (Table 3) (Xu et al., 2016).
2.3.3 Structure-activity relationship of biphenyls with anti-TMV activity
Contrary to the cytotoxicity, compound 5 had higher anti-tobacco mosaic virus activity than compound 6 (Figure 3; Table 3). It could be considered that the hydroxyl group at the R5 (C9) position may be helpful to increase the anti-TMV activity. Since compound 3 and compound 5 exhibited similar activity, it presumed that the adjacent double hydroxyl group at C9 and C10 had no impact on the compounds’ potential to inhibit the tobacco mosaic virus. The results of the anti-TMV activity tests for compounds 14 and 15 were also in conflict with the results of the cytotoxic activity tests. Since biphenyl 15 was shown to have a higher rate of inhibition than biphenyl 14 in both studies (Shang et al., 2014; Li et al., 2015), it can be deduced that methoxylation of the hydroxyl group at the meta-isopentenyl position in the structures was advantageous for enhancing the resistance to tobacco mosaic virus (Figure 3; Table 3). However, the tobacco mosaic virus inhibition rates of compounds 16 and 17 were also similar, in agreement with their cytotoxic test results. This suggested that the shift from 3,3-dimethylallyl group to 3-hydroxymethyl-3-methyl-but-2-enyl chain in their structures does not affect their activity levels (Figure 3; Table 3).
2.4 Biphenyls with associated anti-rotavirus activity
The biphenyls in the Clusiaceae family that have anti-rotavirus effect are all from the genus Garcinia. They all feature a basic structural backbone of 2,3,4-trihydroxyl substitution.
Garcinia lancilimba C. Y. Wu ex Y. H. Li is a small tree mainly distributed in humid dense forests of southern Yunnan province. A number of prenylated xanthones and biphenyls were isolated from this plant (Yang et al., 2007; Han et al., 2008; Gao et al., 2018). Garcilancibiphenyls A-C (52–54) (Figure 5), which are structurally characterized by the acetoxyprenyl group on a benzene ring, were identified via spectroscopic evidence from the extract of the air-dried and powdered stems of Garcinia lancilimba at room temperature. In the report, the anti-rotavirus activity (Table 4) of 52–54 was tested on MA104 cells in terms of CC50, EC50 and the TI (CC50/EC50). It was evident that 52 and 53 were potential for medicinal development against rotavirus with TI values above 10 (17.65, 14.56, 19.8 for 52, 53, Ribavirin, respectively) (Gao et al., 2018).
Multibiphenyls A-C (55–57) were three new biphenyls isolated from Garcinia multiflora Champion ex Bentham (Figure 5) (Gao et al., 2016). The distinct structures of these biphenyls are interestingly correlated. Apparently, 57 could be obtained from the deacetylation of 56, while 56 might be the product derived from the cyclization of 55. The potential of 55–57 to prevent the cytopathic effects caused by rotavirus was quantified in MA104 cells for the sake of assessing their bioactive significance and competence. The determination of antiviral activity was displayed as the values of CC50, EC50 and TI, together with ribavirin serving as a positive control. The results showed that all TI values were greater than 10, demonstrating the good anti-rotavirus potential of these biphenyls (55–57) (Table 4).
Two new biphenyls (58–59) (Figure 5) were yielded in a continuous search for more chemical components from Garcinia tetralata. Biphenyl 58 was determined as 2-isopropenyl-6-methoxy-7-hydroxy-(4-hydroxyphenyl)-dihydrobenzofuran, which can be known as the first biphenyl substituted with 2-isopropenyl dihydrobenzofuran. Another one (59) was recorded as [1.1′-biphenyl]-3-methoxy-4.4′,5-triol following the extensive analysis of spectroscopy techniques. The compounds (58–59) were screened to figure out their anti-rotavirus activity in MA104 cells. Both of them exhibited good anti-rotavirus potential with SI values were above 10 (Table 4) (Ji et al., 2017).
Except for compound 54, the other compounds tested for anti-rotavirus activity had TI or SI values greater than 10 (Table 4). Comparing the structures of biphenyls 54 and 55, it can be inferred that a double hydroxyl substitution next to the prenylated chain would favour the safety and efficacy of the compounds against rotavirus. While compound 55 can be converted to compound 56 via chain cyclization, this change has no influence on the activity (Figure 5).
2.5 Biphenyls with associated anti-HIV activity
A total of 7 biphenyls (Figure 3) were tested for their anti-HIV ability. They have been all mentioned above. Among them, six compounds had 2,3,4-trihydroxyl substituted structures.
2.5.1 Biphenyls with 2,3,4-trihydroxyl group
Probing the anti-HIV ability of 4 (Figure 3) was practiced by methods of syncytium inhibition assay and reverse transcriptase (RT) assay. Biphenyl 4 exhibited moderate activity in the syncytium inhibition assay. It could reduce 50% of syncytium formation at the concentration of 40.17 µM and its SI (IC50/EC50) was 1.88 in the model of 1A2 cells infected by ΔTat/RevMC99 virus (Table 5). However, its repressive effect on HIV-1 reverse transcriptase was not very good. Its inhibition rate was only 8.57% at the concentration of 200 μg/mL. Therefore, its IC50 was not determined in the anti-HIV-1 RT assay (Pailee et al., 2018).
As for the anti-HIV activity of the biphenyls (7–9) (Figure 3), they presented various efficacy in the anti-HIV-1 RT assay and the syncytium inhibition assay with the system of ΔTat/RevMC99 virus and 1A2 cell line. For anti-HIV-1 RT assay of 7-9, both 7 and 8 were mild to repress the HIV-1 reverse transcriptase with the inhibition rates of 39.54% and 49.36% at the concentration of 200 μg/mL respectively. On the other hand, 9 was very active with the inhibition rate reaching up to 91.15% at the concentration of 200 μg/mL and its IC50 value was 202.50 µM by contrast. In the respect of syncytium inhibition assay, all the three compounds were active to suppress the syncytium formation by ΔTat/RevMC99 virus in 1A2 cells, and similar SI scores were obtained at 1.68, 2.98, 3.48 for 7, 8, 9, respectively (Table 5).
The remaining two compounds with 2,3,4-trihydroxyl substitution that were measured for anti-HIV activity were biphenyls 12 and 13 (Figure 3). With respect to their (12–13) anti-HIV effects, the tests were also performed by utilizing syncytium inhibition assay and the RT assay. They all demonstrated activity in the syncytium inhibition assay proceeding with the ΔTat/RevMC99 virus and 1A2 cell line system, with SI values all above one and were 1.91, >4.76 respectively (Table 5). Biphenyl 13 displayed moderate activity, whilst biphenyls 12 was inactive in the HIV-1 RT assay on the contrary. The prescreened inhibition rates of 12–13 at the concentration of 200 μg/mL were −2.53% and 53.83% respectively, but their IC50 values were not determined (Pailee et al., 2018).
2.5.2 Biphenyls with 2,4-dihydroxyl group
Biphenyl 39 (Figure 3) was isolated and analyzed in the same study as compounds 12 and 13. Along with biphenyls 12 and 13, it was also subjected to both the syncytium inhibition assay and the HIV-1 RT assay. In fact, the result of the syncytium inhibition test for compound 39 was better than compound 12. Its (39) SI value was above one and was 7.51 in the anti-syncytium test (Table 5). However, it (39) was inactive in the HIV-1 RT assay as the inhibition rate at the concentration of 200 μg/ml was 7.84%. The IC50 value was not determined. (Pailee et al., 2018).
2.5.3 Structure-activity relationship of biphenyls with anti-HIV activity
It was clear from the aforementioned and Table 5 that the anti-HIV effects of the investigated biphenyls were generally similar. Compound 9 (Figure 3) demonstrated the best performance in the anti-HIV-1 RT assay. It was suspected that, in comparison to compound 7 (Figure 3), the methylation of hydroxyl at the R4(C5) site of biphenyl 9 favored to enhance inhibition ability.
2.6 Biphenyls with other bioactive abilities
2.6.1 Biphenyls with antioxidant activity
Through 2.2′-azinobis-(3-ethylbenzthiazoline-6-sulphonate) (ABTS) radical cation decolorization assay, the anti-oxidant property of biphenyls 20–22 (Figure 3) and biphenyls 25–26 (Figure 3) was evaluated. They all turned out to have good or moderate anti-oxidant possibilities, with IC50 values of 7.78 µM, 38.3 µM, 8.78 µM, 7.84 µM and 29.57 µM for 20–22, 25–26, respectively. Ascorbic acid was employed as a positive control, with an IC50 value of 7.70 µM. The compound 22 appears to be stronger than 21 in terms of anti-oxidant effect, which indicated the influence of the position of the methoxy group on the phenyl. Meanwhile, although the cytotoxicities of 25 and 26 were similar, it can be seen that the bioactive properties along with the anti-oxidant ability of 26 were certainly better than 25, which might be owing to the additional prenylated group on the phenyl of 26. (Tian et al., 2017).
2.6.2 Biphenyls with anti-inflammatory activity
Superoxide anion radical (O2−) is a predecessor of other reactive oxygen species (ROS) and could be generated by activated neutrophils in response to stimuli (Reichl et al., 2001; Selloum et al., 2001). The over-production of O2− is a major cause for the development of inflammation and the scavenging of excess O2− is helpful for anti-inflammatory (Kroes et al., 1992; Salvemini et al., 2001). Experiments were performed to evaluate the efficacy of 3 (Figure 3) to suppress O2− generation induced by chemotactic peptide N-Formyl-L-methionyl (Met)-L-leucyl (Leu)-l-phenylalanine (Phe) (fMLP). The results shown that 3 presented effective inhibitory activity (IC50 = 17.0 ± 2.8 μM) on O2− generation compared with positive control Ibuprofen (IC50 = 27.5 ± 3.5 μM) (Chen et al., 2009). Furthermore, the anti-neuroinflammatory activity and neuroprotective effect of 3 were probed through measuring nitric oxide (NO) inhibition in lipopolysaccharide (LPS)-stimulated murine microglia BV2 cell and determining the secretion of nerve growth factor (NGF) in C6 glial cells, respectively (Kim et al., 2016; Suh et al., 2017). It indicated that the inhibitory activity of 3 to reduce the production of NO was potent with IC50 values of 20.04 μM (IC50 values of positive control L-NMMA = 21.82 μM), and the potency of 3 in offering more NGF for neuroprotection was moderate with NGF secretion levels of 125.76 ± 4.52% (the values of positive control 6-Shogaol = 158.18 ± 6.56%). In 2021, Kim and Yoon group presented a plausible mechanism of how 3 inhibited idiopathic pulmonary fibrosis (IPF), a lung disease generating lung scarring, in a bleomycin (BLM)-induced lung fibrosis mouse model. The research found out that 3 could depress the genes of inflammation and decrease macrophage activation marker genes, along with raising the expression level of anti-fibrotic markers in vivo. More significantly, it revealed that 3 could hamper the production of inflammatory cytokine-induced by transforming growth factor-β (TGF-β) from macrophages and the collagen synthesis from fibroblasts. These results demonstrated that 3 could play a protective role in lung fibrosis via its anti-inflammatory ability and it might be a promising therapy for IPF (Lee et al., 2021). Meanwhile, biphenyl 15 (Figure 3) could slightly exert anti-inflammatory activity against cyclooxygenase-2 (COX-2), an enzyme increased may due to inflammatory events, with the IC50 value of 108.54 ± 0.42 μM (IC50 values of positive control celecoxib = 18.08 ± 0.12 μM) (Ma et al., 2019).
2.6.3 Biphenyls with antimalarial activity, anti-diabetic activity, neurotrophic activity, or anti-virulence activity
Except for cytotoxicity and antibacterial, biphenyl 1 (Figure 3) showed weak antimalarial activity against Plasmodium falciparum with the IC50 values of 39.4 µM against TM4/8.2, and 43.1 µM against K1CB1, respectively (Auranwiwat et al., 2021).
Furthermore, the anti-diabetic activity of biphenyl 4 (Figure 3) was assessed by testing the inhibition against α-glucosidase in parallel with the inducibility of glucose consumption by 3T3-L1 cells. It could be known that the α-glucosidase inhibitory activity of 4 was not determined. However, it can induce glucose consumption without causing toxic effects with IC50 values of 34.7 µM. Metformin was used as positive control with an IC50 value of 45.4 µM (Phukhatmuen et al., 2020).
A study examined the impact of biphenyl 31 (Figure 3) on neurite outgrowth in rat cortical neurons in a primary culture. It discovered that 1 μM of biphenyl 31 could stimulate neurite outgrowth. At this concentration, its activity could be comparable to the basic fibroblast growth factor (bFGF, 40 ng/mL). However, when 10 μM of 31 was administered to neural cells, all neurons were killed during 6 days culture period. The morphology and viability of cortical neurons at a lower dosage of 0.1 μM were not affected by 31. It was suggested that biphenyl 31 with a biaryl moiety containing alkenyl groups, would have some beneficial effects on the growth and survival of neurons (Takaoka et al., 2002).
5,6,6′-trihydroxy-[1.1′-biphenyl]-3.3’dicarboxylic acid (60) (Figure 6) is a new natural product from the ethyl acetate extract of Mesua ferrea L. flower. The purpose of the research was to identify chemical inhibitors for the protein secretion system of Salmonella enterica, which could be thought to be responsible for the pathogenicity of the bacteria. However, biphenyl 60 lacked sufficient activity to exert inhibitory effects (Zhang et al., 2019).
2.7 Biphenyls with biological activity not investigated
2.7.1 Biphenyls with 2,3,4-trihydroxyl group
Garcinia kola Heckel, a plant that is native throughout West and West Central Africa, has been intensively studied for many years due to its edible seeds, usually known as bitter kola or male kola, and medicinal potential in the treatment of a variety of infectious disorders (Hussain et al., 1982; Iwu et al., 2002; Emmanuel et al., 2022). In 1994, Niwa group isolated and identified a 6-aryl-1,2-benzopyran derivative named garcipyran (61) from Garcinia Kola. (Figure 6) (Niwa et al., 1994a).
Two distinct biphenyls, in terms of garcifurans A-B (62–63) (Figure 6), from the methanol extract of Garcinia Kola roots collected in Nigeria were obtained by Niwa in 1994 (Niwa et al., 1994b). 62 and 63 were found to be the natural biphenyls claimed to possess a 5-arylbenzofuran nucleus for the first time. In order to validate the structure assignment of 63, the total synthesis of 63 was accomplished by Kelly group in 1997 (Scheme 4). The benzofuran 63d was prepared through condensation between 5-bromo-2-hydroxybenzaldehyde (63a) and bromo-malonic ester, followed by hydrolysis, and subsequent decarboxylation according to the literature (Kurdukar and Subba Rao, 1963). Next, arylstannanes 63g-63h were gained by lithiation and quenching with Bu3SnCl or Me3SnCl after the protection of 63e with CCl2Ph2. Afterward, the palladium-catalyzed coupling reaction between 63 g/h and 63d smoothly led to the precursors 63i in 18% yield and 44% yield respectively. Heating 63i under reflux in AcOH/H2O to deprotect the protecting group achieved 63 in 73% yield (Kelly et al., 1997).
2.7.2 Biphenyls with 2,4-dihydroxyl group
Calophymembranside A (64) (Figure 6), a novel biphenyl C-glycoside, was identified via chemical analysis of the traditional Chinese medicine Calophyllum membranaceum Gardn. et Champ. The structure of 64 has been determined to be 3,5-dihydroxybiphenyl-4-C-β-glucopyranoside. It is the only biphenyl C-glycoside described in this article (Zou et al., 2005). Furthermore, in 2006, chromatographic treatment of the dichloromethane extract of Clusia melchiorii Gleason trunk resulted in the discovery of a novel biphenyl, 2,2-dimethyl-5-hydroxi-7-phenylchromene (65) (Figure 6) (Teixeira et al., 2006). To the best of our knowledge, Garcinia mangostana L (mangosteen) is a tropical tree used as medicine in Southeast Asia over the past decades and has been well-known because of its fruit, which contains a great deal of polyphenolic xanthones exhibiting various biological effects in vitro (Obolskiy et al., 2009). The novel geranylated biphenyl derivative 3-hydroxy-4-geranyl-5-methoxybiphenyl (66) (Figure 6) was separated and elucidated from extracts of root bark, stem bark and the latex collected from the green fruits of Garcinia mangostana by Dharmaratne and co-workers (Dharmaratne et al., 2005). Meanwhile, multiflorabiphenyl A1 (67) (Figure 6) was found in the stem barks of Garcinia multiflora in 2013 (Jing et al., 2013). And 3.4′,5-trihydroxy-4-(3-hydroxy-3-methylbutyl) biphenyl (68) (Figure 6) and 3,5-dihydroxy-4-(3-hydroxy-3-methylbutyl) biphenyl (69) (Figure 6) were extracted and analyzed from Pentaphafangium Sofomonse Warb (Cotterill et al., 1974).
2.7.3 Others
After the separation of clusiaparalycolines A-C (30–31) (Figure 3), clusiaparalycoline D (70) (Figure 6) was isolated and elucidated during a re-examination of Clusia paralycola roots in 2002 (Delle Monache et al., 2002).
3 Conclusion and remarks
This review summarized the research progress of the biphenyls from Clusiaceae. The chemical structure and biological activity of 70 biphenyls were discussed in this review. Among these biphenyls, 52 compounds are found to present prenylated segment or mutative structures. Since the discovery of biphenyl 68–69 in Pentaphafangium Sofomonse, a total of 24 other Garcinia plants have been identified as sources of various biphenyls, in which Garcinia multiflora and Garcinia linii are the top two plants with the most biphenyls ascertained. The discovery of compounds 68–69 can be traced back to 1974 (Niwa et al., 1994a). And compound 3, which was obtained from other species (Erdtman et al., 1961), could be extracted and generated from different plants of Garcinia.
Biphenyls 1–70 have been subjected to extensive bioactive studies, primarily involving cytotoxicity, antibacterial and antivirus activity. Compound 6, a derivative of chemical 3 with one additional hydroxyl and methoxyl substitution, showed especially potential in repressing the proliferation of P388 cell line with IC50 values of 0.36 μM (Mungmee et al., 2013). Therefore, its mechanism of action deserves to be further studied. The biphenyls 5, 16–18, 29, 31 and 37 also displayed promising cytotoxic effects, with IC50 values below 10 μM (Table 1). With regard to the bioactive qualities of other biphenyls, compound 3 is effective at inhibiting Bacillus subtilis with MIC values of 3.12 μg/mL (Cortez et al., 2002). Both compounds 44 and 45 were tested for antimicrobial activity. The MIC values for 45 were nearly half of those for 44 (Table 2). The results implied that the structural change from a 2-hydroxy-3-methyl-but-3-enyl side chain to a 3-hydroxymethyl-3-methyl-but-2-enyl side chain in 44 would have a positive influence on their antibacterial activity. Furthermore, the structure-activity relationship of compounds 16–18 based on the anti-tobacco mosaic virus activities (Table 3) indicated that the cyclization of the prenylated chain to form a six-membered heterocyclic ring might enhance the anti-TMV abilities. And biphenyl 50 is the most promising compound to resist tobacco mosaic virus with the inhibition rates of 31.1 ± 3.5% (Hu et al., 2016). Moreover, the compounds also performed well in antiviral test, with compound 52 exhibiting great potential against rotavirus with TI values of 17.65 (Gao et al., 2018).
Most of the biphenyls collected in this review have free hydroxyl groups, which may undergo metabolic deactivation by forming sulfates or glucuronides. This could be one of the reasons that some of the biphenyls in this review have moderate or no bioactivity. For example, compound 12 had no cytotoxicity against the P-388 cell line, whereas compound 13 with a protected C2 hydroxyl group had moderate cytotoxicity. Similarly, compound 37 with a tetrahydropyran ring had better cytotoxicity against P-388 and HT-29 compared to compound 36 with same core structure. To avoid the metabolic deactivation, the free hydroxyl group on the phenyl may be replaced by its bio-isosterism in the drug design. In addition, biphenyls are known for atropisomerism and have been exploited in drug discovery. It should be noted that compounds 42 and 60 with bulky groups next to C6 and C7 may have this structural feature.
Although biphenyl is a kind of compound with simple structure, it is worth to be studied because of its easy modification and diverse bioactivity. Several biphenyls from Clusiaceae do possess outstanding activity in multiple fields, such as compounds 6, 50 and 52. Evaluation of the action mechanism of these promising biphenyls should be noted and endeavored in the future.
Author contributions
All authors listed have made a substantial, direct, and intellectual contribution to the work and approved it for publication.
Funding
We are grateful to the National Natural Science Foundation of China (81973438, 82174025), the NSFC-Joint Foundation of Yunnan Province (U1902213), Fok Ying-Tong Education Foundation (161039), the Guangdong Province Key Area R&D Program of China (2020B1111110003) for financial support.
Conflict of interest
The authors declare that the research was conducted in the absence of any commercial or financial relationships that could be construed as a potential conflict of interest.
The reviewer DZ declared a past co-authorship with the author HX to the handling editor.
Publisher’s note
All claims expressed in this article are solely those of the authors and do not necessarily represent those of their affiliated organizations, or those of the publisher, the editors and the reviewers. Any product that may be evaluated in this article, or claim that may be made by its manufacturer, is not guaranteed or endorsed by the publisher.
References
Acuna, U. M., Jancovski, N., and Kennelly, E. J. (2009). Polyisoprenylated benzophenones from Clusiaceae: Potential drugs and lead compounds. Curr. Top. Med. Chem. (Sharjah, United Arab. Emir. 9 (16), 1560–1580. doi:10.2174/156802609789909830
Anusha, S., Mohan, C. D., Ananda, H., Baburajeev, C. P., Rangappa, S., Mathai, J., et al. (2016). Adamantyl-tethered-biphenylic compounds induce apoptosis in cancer cells by targeting Bcl homologs. Bioorg. Med. Chem. Lett. 26 (3), 1056–1060. doi:10.1016/j.bmcl.2015.12.026
Auranwiwat, C., Laphookhieo, S., Rattanajak, R., Kamchonwongpaisan, S., Pyne, S. G., and Ritthiwigrom, T. (2016). Antimalarial polyoxygenated and prenylated xanthones from the leaves and branches of Garcinia mckeaniana. Tetrahedron 72 (43), 6837–6842. doi:10.1016/j.tet.2016.09.018
Auranwiwat, C., Limtharakul, T., Pyne, S. G., Rattanajak, R., and Kamchonwongpaisan, S. (2021). A new xanthone and a biphenyl from the flower and twig extracts of Garcinia mckeaniana. Nat. Prod. Res. 35 (20), 3404–3409. doi:10.1080/14786419.2019.1700505
Auranwiwat, C., Trisuwan, K., Saiai, A., Pyne, S. G., and Ritthiwigrom, T. (2014). Antibacterial tetraoxygenated xanthones from the immature fruits of Garcinia cowa. Fitoterapia 98, 179–183. doi:10.1016/j.fitote.2014.08.003
Banik, K., Harsha, C., Bordoloi, D., Lalduhsaki Sailo, B., Sethi, G., Leong, H. C., et al. (2018). Therapeutic potential of gambogic acid, a caged xanthone, to target cancer. Cancer Lett. (N. Y., N. Y. U. S.) 416, 75–86. doi:10.1016/j.canlet.2017.12.014
Bhutani, P., Joshi, G., Raja, N., Bachhav, N., Rajanna, P. K., Bhutani, H., et al. (2021). U.S. FDA approved drugs from 2015-june 2020: A perspective. J. Med. Chem. 64 (5), 2339–2381. doi:10.1021/acs.jmedchem.0c01786
Chantarasriwong, O., Batova, A., Chavasiri, W., and Theodorakis, E. A. (2010). Chemistry and Biology of the caged Garcinia xanthones. Chem. Eur. J. 16 (33), 9944–9962. doi:10.1002/chem.201000741
Chaturonrutsamee, S., Kuhakarn, C., Surawatanawong, P., Prabpai, S., Kongsaeree, P., Jaipetch, T., et al. (2018). Polycyclic polyprenylated acylphloroglucinols and biphenyl derivatives from the roots of Garcinia nuntasaenii Ngerns. & Suddee. Phytochemistry 146, 63–74. doi:10.1016/j.phytochem.2017.12.001
Chen, J.-J., Chen, I.-S., and Duh, C.-Y. (2004). Cytotoxic xanthones and biphenyls from the root of Garcinia linii. Planta Med. 70 (12), 1195–1200. doi:10.1055/s-2004-835851
Chen, J.-J., Luo, Y.-T., Liao, C.-H., Chen, I.-S., and Liaw, C.-C. (2009). A new dibenzofuran and further constituents from the stems of pourthiaea lucida with inhibitory activity on superoxide generation by neutrophils. Chem. Biodivers. 6 (5), 774–778. doi:10.1002/cbdv.200800118
Chen, J.-J., Peng, C.-F., Huang, H.-Y., and Chen, I.-S. (2006). Benzopyrans, biphenyls and xanthones from the root ofGarcinia liniiand their activity againstMycobacterium tuberculosis. Planta Med. 72 (5), 473–477. doi:10.1055/s-2005-916253
Chen, R., Yuan, D., and Ma, J. (2022). Advances of biphenyl small-molecule inhibitors targeting PD-1/PD-L1 interaction in cancer immunotherapy. Future Med. Chem. 14 (2), 97–113. doi:10.4155/fmc-2021-0256
Chen, T.-H., Tsai, M.-J., Fu, Y.-S., and Weng, C.-F. (2019). The exploration of natural compounds for anti-diabetes from distinctive species Garcinia linii with comprehensive review of the Garcinia family. Biomolecules 9 (11), 641. doi:10.3390/biom9110641
Chen, Y., Xue, Q., Teng, H., Qin, R., Liu, H., Xu, J., et al. (2020). Acylphloroglucinol derivatives with a tricyclo-[4.4.1.1(1, 4)] dodecane skeleton from Garcinia bracteata fruits. J. Org. Chem. 85 (10), 6620–6625. doi:10.1021/acs.joc.0c00637
Chien, S.-C., Chyu, C.-F., Chang, I. S., Chiu, H.-L., and Kuo, Y.-H. (2008). A novel polyprenylated phloroglucinol, Garcinialone, from the roots of Garcinia multiflora. Tetrahedron Lett. 49 (36), 5276–5278. doi:10.1016/j.tetlet.2008.06.102
Chittimalla, S. K., Kuppusamy, R., and Akavaram, N. (2015). Palladium-catalyzed regioselective synthesis of oxygenated biphenyls. Synlett 26 (5), 613–618. doi:10.1055/s-0034-1379987
Cortez, D. A. G., Abreu Filho, B. A., Nakamura, C. V., Dias Filho, B. P., Marston, A., and Hostettmann, K. (2002). Antibacterial activity of a biphenyl and xanthones from kielmeyera coriacea. Pharm. Biol. (Lisse, Neth.) 40 (7), 485–489. doi:10.1076/phbi.40.7.485.14687
Cortez, D. A. G., Young, M. C. M., Marston, A., Wolfender, J. L., and Hostettmann, K. (1998). Xanthones, triterpenes and a biphenyl from kielmeyera coriacea. Phytochemistry 47 (7), 1367–1374. doi:10.1016/S0031-9422(97)00731-0
Cotterill, P. J., Owen, P. J., and Scheinmann, F. (1974). Extractives from guttiferae. Part XXVII. Structure and synthesis of new biphenyls from Pentaphalangium solomonse warb. J. Chem. Soc. Perkin 1 (21), 2423. doi:10.1039/P19740002423
Delgado, J. L., Lentz, S. R. C., Kulkarni, C. A., Chheda, P. R., Held, H. A., Hiasa, H., et al. (2019). Probing structural requirements for human topoisomerase I inhibition by a novel N1-Biphenyl fluoroquinolone. Eur. J. Med. Chem. 172, 109–130. doi:10.1016/j.ejmech.2019.03.040
Delle Monache, F., Delle Monache, G., Botta, B., and Gacs-Baitz, E. (2002). A biphenyl, a dihydrophenanthrene and a xanthone from Clusia paralycola. Heterocycles 56 (1-2), 589–597. doi:10.3987/com-01-s(k)67
Dharmaratne, H. R. W., Piyasena, K. G. N. P., and Tennakoon, S. B. (2005). A geranylated biphenyl derivative from Garcinia mangostana. Nat. Prod. Res. 19 (3), 239–243. doi:10.1080/14786410410001710582
Ding, Y., Mao, L., Xu, D., Xie, H., Yang, L., Xu, H., et al. (2015). C-aryl glucoside SGLT2 inhibitors containing a biphenyl motif as potential anti-diabetic agents. Bioorg. Med. Chem. Lett. 25 (14), 2744–2748. doi:10.1016/j.bmcl.2015.05.040
Elfita, E., Muharni, M., Latief, M., Darwati, D., Widiyantoro, A., Supriyatna, S., et al. (2009). Antiplasmodial and other constituents from four Indonesian Garcinia spp. Phytochemistry 70 (7), 907–912. doi:10.1016/j.phytochem.2009.04.024
Elya, B., He, H. P., Kosela, S., Hanafi, M., and Hao, X. J. (2006). A new benzophenone from the stem bark of Garcinia benthami. Nat. Prod. Res. 20 (12), 1059–1062. doi:10.1080/14786410500462512
Emmanuel, O., Uche, M. E., Dike, E. D., Etumnu, L. R., Ugbogu, O. C., and Ugbogu, E. A. (2022). A review on Garcinia kola Heckel: Traditional uses, phytochemistry, pharmacological activities, and toxicology. Biomarkers 27 (2), 101–117. doi:10.1080/1354750x.2021.2016974
Erdtman, H., Eriksson, G., and Norin, T. (1961). Phenolic biphenyl derivatives from the heartwood of Sorbus aucuparia (L). Acta Chem. Scand. 15, 1796. doi:10.3891/acta.chem.scand.15-1796
Fan, Y.-M., Yi, P., Li, Y., Yan, C., Huang, T., Gu, W., et al. (2015). Two unusual polycyclic polyprenylated acylphloroglucinols, including a pair of enantiomers from Garcinia multiflora. Org. Lett. 17 (9), 2066–2069. doi:10.1021/acs.orglett.5b00588
Farombi, E. O., Adedara, I. A., Oyenihi, A. B., Ekakitie, E., and Kehinde, S. (2013). Hepatic, testicular and spermatozoa antioxidant status in rats chronically treated with Garcinia kola seed. J. Ethnopharmacol. 146 (2), 536–542. doi:10.1016/j.jep.2013.01.018
Fu, W., Wu, M., Zhu, L., Lao, Y., Wang, L., Tan, H., et al. (2015). Prenylated benzoylphloroglucinols and biphenyl derivatives from the leaves of Garcinia multiflora Champ. RSC Adv. 5 (95), 78259–78267. doi:10.1039/c5ra09213b
Gao, X.-M., Cui, D., Wang, S.-S., Huang, W.-Z., Li, G.-P., Zeng, G.-Z., et al. (2018). New biphenyls from Garcinia lancilimba. Phytochem. Lett. 27, 219–222. doi:10.1016/j.phytol.2018.07.027
Gao, X.-M., Ji, B.-K., Li, Y.-K., Ye, Y.-Q., Jiang, Z.-Y., Yang, H.-Y., et al. (2016). New biphenyls from Garcinia multiflora. J. Braz. Chem. Soc. 27 (1), 10–14. doi:10.5935/0103-5053.20150235
Gong, G., Chen, H., Kam, H., Chan, G., Tang, Y.-X., Wu, M., et al. (2020). In vivo screening of xanthones from Garcinia oligantha identified oliganthin H as a novel natural inhibitor of convulsions. J. Nat. Prod. 83 (12), 3706–3716. doi:10.1021/acs.jnatprod.0c00963
Gonsap, K., and Vongsak, B. (2019). In vitro alpha-glucosidase and alpha-amylase enzyme inhibitory effects and free radical scavenging activity of Garcinia schomburgkiana extracts. Planta Med. 85 (18), 1578–1579. doi:10.1055/s-0039-3400424
Grossman, R. B., and Yang, X.-W. (2020). Structural revision of garcinielliptin oxide and garcinielliptone E. J. Nat. Prod. 83 (6), 2041–2044. doi:10.1021/acs.jnatprod.0c00306
Guo, Y.-E., Wang, L.-L., Li, Z.-L., Niu, S.-L., Liu, X.-Q., Hua, H.-M., et al. (2011). Triterpenes and xanthones from the stem bark of Garcinia tetralata. J. Asian Nat. Prod. Res. 13 (5), 440–443. doi:10.1080/10286020.2011.568414
Gutierrez-Orozco, F., and Failla, M. L. (2013). Biological activities and bioavailability of mangosteen xanthones: A critical review of the current evidence. Nutrients 5, 3163–3183. doi:10.3390/nu5083163
Ha, N. T. T., Tuyen, N. V., Tra, N. T., Anh, L. T. T., Son, N. T., Litaudon, M., et al. (2021). Garcimckeans A-C, three new xanthones from the stems of Garcinia mckeaniana, and their cytotoxic activity. Nat. Prod. Res. 5, 1–8. doi:10.1080/14786419.2021.1950717
Hamed, W., Brajeul, S., Mahuteau-Betzer, F., Thoison, O., Mons, S., Delpech, B., et al. (2006). Oblongifolins A-D, polyprenylated benzoylphloroglucinol derivatives from Garcinia oblongifolia. J. Nat. Prod. 69 (5), 774–777. doi:10.1021/np050543s
Han, Q.-B., Tian, H.-L., Yang, N.-Y., Qiao, C.-F., Song, J.-Z., Chang, D. C., et al. (2008). Polyprenylated xanthones from Garcinia lancilimba showing apoptotic effects against HeLa-C3 cells. Chem. Biodivers. 5 (12), 2710–2717. doi:10.1002/cbdv.200890225
Hasegawa, N. (2001). Garcinia extract inhibits lipid droplet accumulation without affecting adipose conversion in 3T3-L1 cells. Phytother. Res. 15 (2), 172–173. doi:10.1002/ptr.689
Hu, Q.-F., Wang, Y.-D., Zhu, D.-L., Yu, Z.-H., Zhan, J.-B., Xing, H.-H., et al. (2016). Three new biphenyls from the twigs of Garcinia tetralata and their anti-tobacco mosaic virus activity. J. Asian Nat. Prod. Res. 18 (12), 1115–1121. doi:10.1080/10286020.2016.1193490
Huang, L., Fullas, F., McGivney, R. J., Brown, D. M., Wani, M. C., Wall, M. E., et al. (1996). A new prenylated flavonol from the root of petalostemon purpureus. J. Nat. Prod. 59 (3), 290–292. doi:10.1021/np960068l
Hussain, R. A., Owegby, A. G., Parimoo, P., and Waterman, P. G. (1982). Kolanone, a novel polyisoprenylated benzophenone with antimicrobial properties from the fruit of Garcinia kola. Planta Med. 44 (2), 78–81. doi:10.1055/s-2007-971406
Inuzuka, H., Liu, J., Wei, W., and Rezaeian, A.-H. (2022). PROTACs technology for treatment of Alzheimer's disease: Advances and perspectives. Acta Mat. Med. 1 (1), 24–41. doi:10.15212/amm-2021-0001
Ito, C., Matsui, T., Noda, E., Ruangrungsi, N., and Itoigawa, M. (2013). Biphenyl derivatives from Garcinia schomburgkiana and the cytotoxicity of the isolated compounds. Nat. Prod. Commun. 8 (9), 1934578X1300800–1267. doi:10.1177/1934578x1300800921
Iwu, M. M., Diop, A. D., Meserole, L., and Okunji, C. O. (2002). Garcinia kola: A new look at an old adaptogenic agent. Adv. Phytomed. 1, 191–199. doi:10.1016/S1572-557X(02)80026-5
Ji, B.-K., Gao, X.-M., Cui, D., Wang, S.-S., Huang, W.-Z., Li, Y.-K., et al. (2017). Two new biphenyls from the stems of Garcinia tetralata. Nat. Prod. Res. 31 (13), 1544–1550. doi:10.1080/14786419.2017.1283492
Jiang, G., Du, F., and Fang, G. (2014). Two new proanthocyanidins from the leaves of Garcinia multiflora. Nat. Prod. Res. 28 (7), 449–453. doi:10.1080/14786419.2013.873431
Jing, W.-Y., Jiang, C., Ji, F., Hua, H.-M., and Li, Z.-L. (2013). Chemical constituents from the stem barks of Garcinia multiflora. J. Asian Nat. Prod. Res. 15 (11), 1152–1157. doi:10.1080/10286020.2013.819854
Kalita, A., Das, M., Baro, M. R., and Das, B. (2021). Exploring the role of aquaporins (AQPs) in LPS induced systemic inflammation and the ameliorative effect of Garcinia in male wistar rat. Inflammopharmacology 29 (3), 801–823. doi:10.1007/s10787-021-00832-9
Kelly, T. R., Szabados, A., and Lee, Y.-J. (1997). Total synthesis of garcifuran B. J. Org. Chem. 62 (2), 428–429. doi:10.1021/jo9615662
Kim, C. S., Subedi, L., Kwon, O. K., Kim, S. Y., Yeo, E.-J., Choi, S. U., et al. (2016). Isolation of bioactive biphenyl compounds from the twigs of chaenomeles sinensis. Bioorg. Med. Chem. Lett. 26 (2), 351–354. doi:10.1016/j.bmcl.2015.12.004
Kim, K. H., Choi, S. U., Ha, S. K., Kim, S. Y., and Lee, K. R. (2009). Biphenyls from berberis koreana. J. Nat. Prod. 72 (11), 2061–2064. doi:10.1021/np900460j
Kim, M. S., Kim, J. K., Kwon, D. Y., and Park, R. (2004). Anti-adipogenic effects of Garcinia extract on the lipid droplet accumulation and the expression of transcription factor. BioFactors 22 (1-4), 193–196. doi:10.1002/biof.5520220140
Kokubun, T., Harborne, J. B., Eagles, J., and Waterman, P. G. (1995). Antifungal biphenyl compounds are the phytoalexins of the sapwood of Sorbus aucuparia. Phytochemistry 40 (1), 57–59. doi:10.1016/0031-9422(95)00307-s
Kroes, B. H., Van den Berg, A. J. J., Quarles van Ufford, H. C., Van Dijk, H., and Labadie, R. P. (1992). Anti-inflammatory activity of gallic acid. Planta Med. 58 (6), 499–504. doi:10.1055/s-2006-961535
Kurdukar, R., and Subba Rao, N. V. (1963). Search for physiologically active compounds. Proc. Indian Acad. Sci. 58 (6), 336–342. doi:10.1007/BF03046395
Kwong, H. C., Kumar, C. S. C., Mah, S. H., Chia, T. S., Quah, C. K., Loh, Z. H., et al. (2017). Novel biphenyl ester derivatives as tyrosinase inhibitors: Synthesis, crystallographic, spectral analysis and molecular docking studies. PLoS One 12 (2), e0170117. doi:10.1371/journal.pone.0170117
Le, D. H., Nishimura, K., Takenaka, Y., Mizushina, Y., and Tanahashi, T. (2016). Polyprenylated benzoylphloroglucinols with DNA polymerase inhibitory activity from the fruits of Garcinia schomburgkiana. J. Nat. Prod. 79 (7), 1798–1807. doi:10.1021/acs.jnatprod.6b00255
Lee, H., Kim, D., Lee, H.-K., Qiu, W., Oh, N.-K., Zin, W.-C., et al. (2004). Discotic liquid crystalline materials for potential nonlinear optical applications: Synthesis and liquid crystalline behavior of 1, 3, 5-triphenyl-2, 4, 6-triazine derivatives containing achiral and chiral alkyl chains at the periphery. Tetrahedron Lett. 45 (5), 1019–1022. doi:10.1016/j.tetlet.2003.11.085
Lee, S. Y., Park, S.-Y., Lee, G.-E., Kim, H., Kwon, J.-H., Kim, M. J., et al. (2021). Aucuparin suppresses bleomycin-induced pulmonary fibrosis via anti-inflammatory activity. J. Med. Food 24 (2), 151–160. doi:10.1089/jmf.2020.4861
Li, H. W., Li, J., and Stevens, P. F. (2011). “Guttiferae,” in Flora of China. Editor E. C. o. FOC (Beijing and Saint Louis: Science Press and Missouri Botanical Garden Press).
Li, H., Zhang, H., Fu, W., Tan, H., and Xu, H. (2016). Progress on polycyclic polyprenylated acylphloroglucinols from Garcinia species in China. World Chin. Med. 11 (7), 1195–1201. doi:10.3969/j.issn.1673-7202.2016.07.009
Li, X.-B., Chen, G.-Y., Liu, R.-J., Zheng, C.-J., Song, X.-M., and Han, C.-R. (2017). A new biphenyl derivative from the mangrove endophytic fungus phomopsis longicolla HL-2232. Nat. Prod. Res. 31 (19), 2264–2267. doi:10.1080/14786419.2017.1300799
Li, Y., Wang, Z., Wu, X., Yang, Y., Qin, Y., Xia, C., et al. (2015). Biphenyl derivatives from the twigs of Garcinia bracteata and their biological activities. Phytochem. Lett. 11, 24–27. doi:10.1016/j.phytol.2014.11.003
Li, Y., Xi, Z., Chen, X., Cai, S., Liang, C., Wang, Z., et al. (2018). Natural compound oblongifolin C confers gemcitabine resistance in pancreatic cancer by downregulating src/MAPK/ERK pathways. Cell Death Dis. 9 (5), 538–613. doi:10.1038/s41419-018-0574-1
Likhitwitayawuid, K., Phadungcharoen, T., and Krungkrai, J. (1998). Antimalarial xanthones from Garcinia cowa. Planta Med. 64 (1), 70–72. doi:10.1055/s-2006-957370
Liu, B., Zhang, X., Bussmann, R. W., Hart, R. H., Li, P., Bai, Y., et al. (2016). Garcinia in southern China: Ethnobotany, management, and niche modeling. Econ. Bot. 70 (4), 416–430. doi:10.1007/s12231-016-9360-0
Liu, G., Li, L., Wang, H., Yang, J., Lou, J., Hu, Q., et al. (2015). A new biphenyl from Garcinia oligantha and its cytotoxicity. Asian J. Chem. 27 (7), 2731–2732. doi:10.14233/ajchem.2015.17830
Liu, X., Yu, T., Gao, X.-M., Zhou, Y., Qiao, C.-F., Peng, Y., et al. (2010). Apoptotic effects of polyprenylated benzoylphloroglucinol derivatives from the twigs of Garcinia multiflora. J. Nat. Prod. 73 (8), 1355–1359. doi:10.1021/np100156w
Lu, W., Li, P., Shan, Y., Su, P., Wang, J., Shi, Y., et al. (2015). Discovery of biphenyl-based VEGFR-2 inhibitors. Part 3: Design, synthesis and 3D-QSAR studies. Bioorg. Med. Chem. 23 (5), 1044–1054. doi:10.1016/j.bmc.2015.01.006
Ly Dieu, H., Hansen, P. E., Duus, F., Hung Dinh, P., and Nguyen, L.-H. D. (2012). Oliveridepsidones A-D, antioxidant depsidones from Garcinia oliveri. Magn. Reson. Chem. 50 (3), 242–245. doi:10.1002/mrc.2862
Ma, Q.-G., Wei, R.-R., and Sang, Z.-P. (2019). Biphenyl derivatives from the aerial parts of oenanthe javanica and their COX-2 inhibitory activities. Chem. Biodivers. 16 (1), e1800480. doi:10.1002/cbdv.201800480
Mahabusarakam, W., Chairerk, P., and Taylor, W. C. (2005). Xanthones from Garcinia cowa Roxb. latex. Phytochemistry 66 (10), 1148–1153. doi:10.1016/j.phytochem.2005.02.025
Mohd Norfaizal, G., Masrom, H., Azuan, A., and Omar, Y. (2014). Flora diversity of sungai teris, krau wildlife reserve, pahang, Malaysia. J. Wildl. Parks 28, 81–91.
Mungmee, C., Sitthigool, S., Buakeaw, A., and Suttisri, R. (2013). A new biphenyl and other constituents from the wood of Garcinia schomburgkiana. Nat. Prod. Res. 27 (21), 1949–1955. doi:10.1080/14786419.2013.796469
Negi, P. S., Jayaprakasha, G. K., and Jena, B. S. (2008). Antibacterial activity of the extracts from the fruit rinds of Garcinia cowa and Garcinia pedunculata against food borne pathogens and spoilage bacteria. LWT - Food Sci. Technol. 41 (10), 1857–1861. doi:10.1016/j.lwt.2008.02.009
Ngernsaengsaruay, C., and Suddee, S. (2016). Garcinia nuntasaenii (Clusiaceae), a new species from Thailand. Thai For. Bull. 44, 134–139. doi:10.20531/tfb.2016.44.2.09
Nguyen, T. T. H., Nguyen, V. T., Van Cuong, P., Nguyen Thanh, T., Le Thi, T. A., Mai Huong, D. T., et al. (2021). A new flavonoid from the leaves of Garcinia mckeaniana Craib and α-glucosidase and acetylcholinesterase inhibitory activities. Nat. Prod. Res. 36, 5074–5080. doi:10.1080/14786419.2021.1916019
Nilar , , and Harrison, L. J. (2002). Xanthones from the heartwood of Garcinia mangostana. Phytochemistry 60 (5), 541–548. doi:10.1016/s0031-9422(02)00142-5
Niwa, M., Ito, J., Terashima, K., and Aqil, M. (1994a). Garcipyran, a novel 6-aryl-1, 2-benzopyran derivative from Garcinia kola. Heterocycles 38 (8), 1927. doi:10.3987/com-94-6773
Niwa, M., Terashima, K., Ito, J., and Aqil, M. (1994b). Two novel arylbenzofurans, garcifuran-A and garcifuran-B from Garcinia kola. Heterocycles 38 (5), 1071. doi:10.3987/com-93-6667
Nontakham, J., Charoenram, N., Upamai, W., Taweechotipatr, M., and Suksamrarn, S. (2014). Anti-Helicobacter pylori xanthones of Garcinia fusca. Arch. Pharm. Res. 37 (8), 972–977. doi:10.1007/s12272-013-0266-4
Obolskiy, D., Pischel, I., Siriwatanametanon, N., and Heinrich, M. (2009). Garcinia mangostana L.: A phytochemical and pharmacological review. Phytother. Res. 23 (8), 1047–1065. doi:10.1002/ptr.2730
Oka, H., Yonezawa, K., Kamikawa, A., Ikegai, K., Asai, N., Shirakami, S., et al. (2018). Design, synthesis, and biological evaluation of novel biphenyl-4-carboxamide derivatives as orally available TRPV1 antagonists. Bioorg. Med. Chem. 26 (12), 3716–3726. doi:10.1016/j.bmc.2018.06.001
Pailee, P., Kuhakarn, C., Sangsuwan, C., Hongthong, S., Piyachaturawat, P., Suksen, K., et al. (2018). Anti-HIV and cytotoxic biphenyls, benzophenones and xanthones from stems, leaves and twigs of Garcinia speciosa. Phytochemistry 147, 68–79. doi:10.1016/j.phytochem.2017.12.013
Panthong, K., Pongcharoen, W., Phongpaichit, S., and Taylor, W. C. (2006). Tetraoxygenated xanthones from the fruits of Garcinia cowa. Phytochem. (Elsevier) 67 (10), 999–1004. doi:10.1016/j.phytochem.2006.02.027
Pattalung, P., Thongtheeraparp, W., Wiriyachitra, P., and Taylor, W. C. (1994). Xanthones of Garcinia cowa. Planta Med. 60 (4), 365–368. doi:10.1055/s-2006-959502
Phukhatmuen, P., Raksat, A., Laphookhieo, S., Charoensup, R., Duangyod, T., and Maneerat, W. (2020). Bioassay-guided isolation and identification of antidiabetic compounds from Garcinia cowa leaf extract. Heliyon 6 (4), e03625. doi:10.1016/j.heliyon.2020.e03625
POWO (2022). Plants of the world online. Facilitated by the Royal Botanic Gardens, Kew. [Online] Available: http://www.plantsoftheworldonline.org/(Accessed June 06, 2022).
Putri, D. K., Elya, B., and Puspitasari, N. (2018). Fractionation of the n-hexane extract of Garcinia bancana MIQ. (Manggis hutan) leaves and its antioxidant activity based on 1, 1-diphenyl-2-picrylhydrazyl and ferric reducing antioxidant power assays. Int. J. App. Pharm. 10 (1), 407–411. doi:10.22159/ijap.2018.v10s1.90
Reichl, S., Vocks, A., Petkovic, M., Schiller, J., and Arnhold, J. (2001). The photoprotein pholasin as a luminescence substrate for detection of superoxide anion radicals and myeloperoxidase activity in stimulated neutrophils. Free Radic. Res. 35 (6), 723–733. doi:10.1080/10715760100301231
Ribeiro, P. R., Ferraz, C. G., Guedes, M. L. S., Martins, D., and Cruz, F. G. (2011). A new biphenyl and antimicrobial activity of extracts and compounds from Clusia burlemarxii. Fitoterapia 82 (8), 1237–1240. doi:10.1016/j.fitote.2011.08.012
Rukachaisirikul, V., Kamkaew, M., Sukavisit, D., Phongpaichit, S., Sawangchote, P., and Taylor, W. C. (2003a). Antibacterial xanthones from the leaves of Garcinia nigrolineata. J. Nat. Prod. 66 (12), 1531–1535. doi:10.1021/np0303254
Rukachaisirikul, V., Naklue, W., Sukpondma, Y., and Phongpaichit, S. (2005a). An antibacterial biphenyl derivative from Garcinia bancana MIQ. Chem. Pharm. Bull. 53 (3), 342–343. doi:10.1248/cpb.53.342
Rukachaisirikul, V., Painuphong, P., Sukpondma, Y., Koysomboon, S., Sawangchote, P., and Taylor, W. C. (2003b). Caged-triprenylated and -tetraprenylated xanthones from the latex of Garcinia scortechinii. J. Nat. Prod. 66 (7), 933–938. doi:10.1021/np030080v
Rukachaisirikul, V., Ritthiwigrom, T., Pinsa, A., Sawangchote, P., and Taylor, W. C. (2003c). Xanthones from the stem bark of Garcinia nigrolineata. Phytochem. (Elsevier) 64 (6), 1149–1156. doi:10.1016/s0031-9422(03)00502-8
Rukachaisirikul, V., Tadpetch, K., Watthanaphanit, A., Saengsanae, N., and Phongpaichit, S. (2005b). Benzopyran, biphenyl, and tetraoxygenated xanthone derivatives from the twigs of Garcinia nigrolineata. J. Nat. Prod. 68 (8), 1218–1221. doi:10.1021/np058050a
Sakunpak, A., and Panichayupakaranant, P. (2012). Antibacterial activity of Thai edible plants against gastrointestinal pathogenic bacteria and isolation of a new broad spectrum antibacterial polyisoprenylated benzophenone, chamuangone. Food Chem. x. 130 (4), 826–831. doi:10.1016/j.foodchem.2011.07.088
Salvemini, D., Mazzon, E., Dugo, L., Riley, D. P., Serraino, I., Caputi, A. P., et al. (2001). Pharmacological manipulation of the inflammatory cascade by the superoxide dismutase mimetic, M40403. Br. J. Pharmacol. 132 (4), 815–827. doi:10.1038/sj.bjp.0703841
Sang, Y., Han, S., Han, S., Pannecouque, C., De Clercq, E., Zhuang, C., et al. (2019). Follow on-based optimization of the biphenyl-DAPYs as HIV-1 nonnucleoside reverse transcriptase inhibitors against the wild-type and mutant strains. Bioorg. Chem. 89, 102974. doi:10.1016/j.bioorg.2019.102974
Sangsuwon, C., and Jiratchariyakul, W. (2015). Antiproliferative effect of lung cancer cell lines and antioxidant of macluraxanthone from Garcinia speciosa Wall. Procedia - Soc. Behav. Sci. 197, 1422–1427. doi:10.1016/j.sbspro.2015.07.089
Santoro, E., Vergura, S., Scafato, P., Belviso, S., Masi, M., Evidente, A., et al. (2020). Absolute configuration assignment to chiral natural products by biphenyl chiroptical probes: The case of the phytotoxins colletochlorin A and agropyrenol. J. Nat. Prod. 83 (4), 1061–1068. doi:10.1021/acs.jnatprod.9b01068
Sato, K., and Mikawa, H. (1960). Chlorination of guaiacol relating to bleaching mechanism of pulp. Bull. Chem. Soc. Jpn. 33, 1736–1737. doi:10.1246/bcsj.33.1736
Schmidt, B., and Riemer, M. (2014). Suzuki-miyaura coupling of halophenols and phenol boronic acids: Systematic investigation of positional isomer effects and conclusions for the synthesis of phytoalexins from pyrinae. J. Org. Chem. 79 (9), 4104–4118. doi:10.1021/jo500675a
Selloum, L., Reichl, S., Mueller, M., Sebihi, L., and Arnhold, J. (2001). Effects of flavonols on the generation of superoxide anion radicals by xanthine oxidase and stimulated neutrophils. Arch. Biochem. Biophys. 395 (1), 49–56. doi:10.1006/abbi.2001.2562
Seo, E.-K., Huang, L., Wall, M. E., Wani, M. C., Navarro, H., Mukherjee, R., et al. (1999). New biphenyl compounds with DNA strand-scission activity from Clusia paralicola. J. Nat. Prod. 62 (11), 1484–1487. doi:10.1021/np9900775
Shang, S.-Z., Xu, W.-X., Lei, P., Zhao, W., Tang, J.-G., Miao, M.-M., et al. (2014). Biphenyls from nicotiana tabacum and their anti-tobacco mosaic virus. Fitoterapia 99, 35–39. doi:10.1016/j.fitote.2014.08.013
Siridechakorn, I., Maneerat, W., Sripisut, T., Ritthiwigrom, T., Cheenpracha, S., and Laphookhieo, S. (2014). Biphenyl and xanthone derivatives from the twigs of a Garcinia sp. (Clusiaceae). Phytochem. Lett. 8, 77–80. doi:10.1016/j.phytol.2014.02.004
Siridechakorn, I., Phakhodee, W., Ritthiwigrom, T., Promgool, T., Deachathai, S., Cheenpracha, S., et al. (2012). Antibacterial dihydrobenzopyran and xanthone derivatives from Garcinia cowa stem barks. Fitoterapia 83 (8), 1430–1434. doi:10.1016/j.fitote.2012.08.006
Song, C., Wang, X., Yang, J., Kuang, Y., Wang, Y., Yang, S., et al. (2021). Antifungal biphenyl derivatives from Sorbus pohuashanensis leaves infected by alternaria tenuissi and their effect against crop pathogens. Chem. Biodivers. 18 (5), e2100079. doi:10.1002/cbdv.202100079
Spontoni do Espirito Santo, B. L., Santana, L. F., Kato, W. H., de Araujo, F. d. O., Bogo, D., Freitas, K. d. C., et al. (2020). Medicinal potential of Garcinia species and their compounds. Molecules 25 (19), 4513. doi:10.3390/molecules25194513
Suh, W. S., Park, K. J., Kim, D. H., Subedi, L., Kim, S. Y., Choi, S. U., et al. (2017). A biphenyl derivative from the twigs of chaenomeles speciosa. Bioorg. Chem. 72, 156–160. doi:10.1016/j.bioorg.2017.04.003
Sukandar, E. R., Ersam, T., Fatmawati, S., Siripong, P., Aree, T., and Tip-pyang, S. (2016a). Cylindroxanthones A-C, three new xanthones and their cytotoxicity from the stem bark of Garcinia cylindrocarpa. Fitoterapia 108, 62–65. doi:10.1016/j.fitote.2015.11.017
Sukandar, E. R., Kaennakam, S., Rassamee, K., Siripong, P., Fatmawati, S., Ersam, T., et al. (2018). Xanthones and biphenyls from the stems of Garcinia cylindrocarpa and their cytotoxicity. Fitoterapia 130, 112–117. doi:10.1016/j.fitote.2018.08.019
Sukandar, E. R., Siripong, P., Khumkratok, S., and Tip-pyang, S. (2016b). New depsidones and xanthone from the roots of Garcinia schomburgkiana. Fitoterapia 111, 73–77. doi:10.1016/j.fitote.2016.04.012
Takaoka, S., Nakade, K., and Fukuyama, Y. (2002). The first total synthesis and neurotrophic activity of clusiparalicoline A, a prenylated and geranylated biaryl from Clusia paralicola. Tetrahedron Lett. 43 (39), 6919–6923. doi:10.1016/S0040-4039(02)01630-1
Tang, Y.-X., Fu, W.-W., Wu, R., Tan, H.-S., Shen, Z.-W., and Xu, H.-X. (2016). Bioassay-guided isolation of prenylated xanthone derivatives from the leaves of Garcinia oligantha. J. Nat. Prod. 79 (7), 1752–1761. doi:10.1021/acs.jnatprod.6b00137
Tang, Y.-X., Fu, W.-W., Xi, Z.-C., Yang, J.-L., Zheng, C.-W., Lu, Y., et al. (2019). Xanthone derivatives from the leaves of Garcinia oligantha. Eur. J. Med. Chem. 181, 111536. doi:10.1016/j.ejmech.2019.07.039
Tarade, D., Ma, D., Pignanelli, C., Mansour, F., Simard, D., van den Berg, S., et al. (2017). Structurally simplified biphenyl combretastatin A4 derivatives retain in vitro anti-cancer activity dependent on mitotic arrest. PLoS One 12 (3), e0171806. doi:10.1371/journal.pone.0171806
Teixeira, J. S. R., Moreira, L. d. M., Guedes, M. L. d. S., and Cruz, F. G. (2006). A new biphenyl from Clusia melchiorii and a new tocotrienol from C. Obdeltifolia. J. Braz. Chem. Soc. 17 (4), 812–815. doi:10.1590/s0103-50532006000400027
Thi Thu, H. N., Minh, Q. P., Van, C. P., Van, T. N., Van, K. P., Thanh, T. N., et al. (2021). Cytotoxic and α-glucosidase inhibitory xanthones from Garcinia mckeaniana leaves and molecular docking study. Chem. Biodivers. 18 (11), e2100396. doi:10.1002/cbdv.202100396
Thummajitsakul, S., Samaikam, S., Tacha, S., and Silprasit, K. (2020). Study on FTIR spectroscopy, total phenolic content, antioxidant activity and anti-amylase activity of extracts and different tea forms of Garcinia schomburgkiana leaves. LWT-Food Sci. Technol. 134, 110005. doi:10.1016/j.lwt.2020.110005
Tian, D.-S., Gu, W., Wang, L.-P., Huang, L.-J., Rao, Q., Huang, T., et al. (2017). Cytotoxic and anti-oxidant biphenyl derivatives from the leaves and twigs of Garcinia multiflora. Phytochem. Lett. 19, 132–135. doi:10.1016/j.phytol.2016.12.021
Tian, D. S., Yi, P., Xia, L., Xiao, X., Fan, Y. M., Gu, W., et al. (2016). Garmultins A-G, biogenetically related polycyclic acylphloroglucinols from Garcinia multiflora. Org. Lett. 18 (22), 5904–5907. doi:10.1021/acs.orglett.6b03004
Wang, D.-m., Feng, B., Fu, H., Liu, A.-l., Wang, L., Du, G.-h., et al. (2017). Design, synthesis, and biological evaluation of a new series of biphenyl/bibenzyl derivatives functioning as dual inhibitors of acetylcholinesterase and butyrylcholinesterase. Molecules 22 (1), 172. doi:10.3390/molecules22010172
Wang, L.-L., Li, Z.-L., Hua, H.-M., and Liu, X.-Q. (2008). Chemical constituents from barks of Garcinia tetralata. China J. Chin. materia medica 33 (20), 2350–2352.
Wang, Z.-Q., Li, X.-Y., Hu, D.-B., and Long, C.-L. (2018). Cytotoxic garcimultiflorones K-Q, lavandulyl benzophenones from Garcinia multiflora branches. Phytochemistry 152, 82–90. doi:10.1016/j.phytochem.2018.04.019
Whitmore, M. A. (1973). Tree flora of malaya. Malaysia: Forest Department Ministry of Primary Industries: Longman.
Wu, X., Ke, C.-Q., Yang, Y.-P., and Ye, Y. (2008). New biphenyl constituents from Garcinia oblongifolia. Helv. Chim. Acta 91 (5), 938–943. doi:10.1002/hlca.200890099
Xu, J., Jin, S., Gan, F., Xiong, H., Mei, Z., Chen, Y., et al. (2020). Polycyclic polyprenylated acylphloroglucinols from Garcinia xanthochymus fruits exhibit antitumor effects through inhibition of the STAT3 signaling pathway. Food Funct. 11 (12), 10568–10579. doi:10.1039/d0fo02535f
Xu, X., Shi, J., Li, L., Zhu, D., Yu, Z., Yu, W., et al. (2016). Biphenyls from the twigs of Garcinia multiflora and their anti-tobacco mosaic virus activities. Rec. Nat. Prod. 10 (5), 566–571.
Yan, X.-T., An, Z., Tang, D., Peng, G.-R., Cao, C.-Y., Xu, Y.-Z., et al. (2018). Hyperelatosides A-E, biphenyl ether glycosides from Hypericum elatoides, with neurotrophic activity. RSC Adv. 8 (47), 26646–26655. doi:10.1039/c8ra05322g
Yang, H., Tian, D., Zeng, Y., Huang, L., Gu, W., Hao, X., et al. (2020). Phenolic derivatives from Garcinia multiflora Champion ex Bentham and their chemotaxonomic significance. Biochem. Syst. Ecol. 88, 103981. doi:10.1016/j.bse.2019.103981
Yang, J.-L., Fu, W.-W., Xiang, Q., Wu, R., Tang, Y.-X., Zheng, C.-W., et al. (2021). Cytotoxic 7-methoxylated caged xanthones from the twigs of Garcinia oligantha. Chin. J. Chem. 39 (10), 2898–2910. doi:10.1002/cjoc.202100362
Yang, N.-Y., Han, Q.-B., Cao, X.-W., Qiao, C.-F., Song, J.-Z., Chen, S.-L., et al. (2007). Two new xanthones isolated from the stem bark of Garcinia lancilimba. Chem. Pharm. Bull. 55 (6), 950–952. doi:10.1248/cpb.55.950
Yuan, Y., Subedi, L., Lim, D., Jung, J.-K., Kim, S. Y., and Seo, S.-Y. (2019). Synthesis and anti-neuroinflammatory activity of N-heterocyclic analogs based on natural biphenyl-neolignan honokiol. Bioorg. Med. Chem. Lett. 29 (2), 329–333. doi:10.1016/j.bmcl.2018.11.014
Zhang, H., Dan, Z., Ding, Z.-J., Lao, Y.-Z., Tan, H.-S., and Xu, H.-X. (2016). UPLC-PDA-QTOFMS-guided isolation of prenylated xanthones and benzoylphloroglucinols from the leaves of Garcinia oblongifolia and their migration-inhibitory activity. Sci. Rep. 6, 35789. doi:10.1038/srep35789
Zhang, X., Gao, R., Liu, Y., Cong, Y., Zhang, D., Zhang, Y., et al. (2019). Anti-virulence activities of biflavonoids from <i>Mesua ferrea</i> L. flower. Drug Discov. Ther. 13 (4), 222–227. doi:10.5582/ddt.2019.01053
Zheng, D., Zhang, H., Jiang, J.-M., Chen, Y.-Y., Wan, S.-J., Lin, Z.-X., et al. (2021). Prenylated xanthones and biphenyls from Garcinia esculenta with antistaphylococcal activity. Nat. Prod. Res. 35 (13), 2137–2144. doi:10.1080/14786419.2019.1663511
Zhou, L., Yang, J., Yang, G., Kang, C., Xiao, W., Lv, C., et al. (2016). Biphenyl phytoalexin in Sorbus pohuashanensis suspension cell induced by yeast extract. Molecules 21 (9), 1180. doi:10.3390/molecules21091180
Zhou, M., Zhou, K., Lou, J., Wang, Y.-D., Dong, W., Li, G.-P., et al. (2015). New biphenyl derivatives from the leaves of nicotiana tabacum and their cytotoxic activity. Phytochem. Lett. 14, 226–229. doi:10.1016/j.phytol.2015.10.025
Zhu, J. X., Ding, L., and He, S. (2019). Discovery of a new biphenyl derivative by epigenetic manipulation of marine-derived fungus Aspergillus versicolor. Nat. Prod. Res. 33 (8), 1191–1195. doi:10.1080/14786419.2018.1465423
Zhu, L.-L., Fu, W.-W., Watanabe, S., Shao, Y.-N., Tan, H.-S., Zhang, H., et al. (2014). Xanthine oxidase inhibitors from Garcinia esculenta twigs. Planta Med. 80 (18), 1721–1726. doi:10.1055/s-0034-1383193
Keywords: Garcinia genus, biphenyl, prenyl, cytotoxicity, antibacterial activity
Citation: Wang Y, Huang Q, Zhang L, Zheng C and Xu H (2022) Biphenyls in Clusiaceae: Isolation, structure diversity, synthesis and bioactivity. Front. Chem. 10:987009. doi: 10.3389/fchem.2022.987009
Received: 05 July 2022; Accepted: 14 November 2022;
Published: 01 December 2022.
Edited by:
Guillermo R. Labadie, National University of Rosario, ArgentinaReviewed by:
Yan-Hui Fu, Hainan Normal University, ChinaDan Zheng, Shanghai Jiao Tong University, China
Copyright © 2022 Wang, Huang, Zhang, Zheng and Xu. This is an open-access article distributed under the terms of the Creative Commons Attribution License (CC BY). The use, distribution or reproduction in other forums is permitted, provided the original author(s) and the copyright owner(s) are credited and that the original publication in this journal is cited, in accordance with accepted academic practice. No use, distribution or reproduction is permitted which does not comply with these terms.
*Correspondence: Changwu Zheng, emhlbmdjd0BzaHV0Y20uZWR1LmNu; Hongxi Xu, eHVob25neGk4OEBnbWFpbC5jb20=
†These authors have contributed equally to this work and share first authorship