- 1Translational Medicine Research Centre, The First Affiliated Hospital of Shandong First Medical University and Shandong Provincial Qianfoshan Hospital, Jinan, China
- 2College of Radiology, Shandong First Medical University and Shandong Academy of Medical Sciences, Jinan, China
Aggregation-induced emission (AIE) molecules have garnered considerable interest since its first appearance in 2001. Recent studies on AIE materials in biological and medical areas have demonstrated that they show their promise as biomaterials for bioimaging and other biomedical applications. Benefiting from significant advantages of their high sensitivity, excellent photostability, and good biocompatibility, AIE-based materials provide dramatically improved analytical capacities for in vivo detection and demonstration of vital biological processes. Herein, we introduce the development history of AIE molecules and recent progress in areas of biotesting and bioimaging. Additionally, this review also offers an outlook for the potential applications of versatile AIE materials for tracing and treating pathological tissues, including overcoming challenges and feasible solutions.
Highlights
The history of the development of AIE molecules is narrated and discussed.
Different kinds of AIE structures are summarized.
The properties of sensing and imaging are exhibited as separate listings.
Challenges in designing and applications are considered and outlooked.
Introduction
Over the past several decades, the discovery and design of small organic molecules capable of fluorescence imaging have become a rapidly expanding area of research (Maity et al., 2015; Jia and Wu, 2020a; Zhang et al., 2020a; Younis et al., 2020; Zhu et al., 2020), particularly owing to their possible practical applications in environmental monitoring, tissue engineering (Dong et al., 2020; Song et al., 2020; Zeng et al., 2020), and medical examination and treatments (Hu et al., 2020a; Jia and Wu, 2020b; Yu et al., 2020). As a powerful tool for tracing, demonstrating, and analyzing biomolecules such as esters (Cai et al., 2020), carbohydrates (Qu et al., 2020), peptides (Gao et al., 2020a), enzymes (Dong et al., 2020a; Zhao et al., 2020a), and nucleotides (Zhang et al., 2020b), fluorescent biosensors provide deep insight into the complicated in vivo chemical, biological, and physiological processes (Wang et al., 2019) to explore authentic pathogenesis and precise diagnosis. Under these circumstances, the research and development of fluorescent biosensors with superior performance are significant.
To achieve this, suitable fluorescence molecules and materials for in vivo tracing and demonstration are key. The optical properties of most organic dyes usually depend on their large conjugate structure, which may be extremely weak owing to their poor water solubility as well as the fluorescence quenching in the aggregate state, also known as the vicious aggregation-caused quenching (ACQ) effect. This phenomenon could be explained as tedious conjugation with aromatic rings accumulated via strong π–π interactions. This ACQ effect of conventional organic fluorescence molecules makes the design of desirable fluorescent structures challenging. In this case, the development of molecules that can overcome the disadvantages of the ACQ effect is extremely urgent. Fortunately, fluorophores with aggregation-induced emission (AIE) property provide a clear path to achieve this since Tang (Luo et al., 2001) first discovered this dramatic phenomenon in 2001. As is shown in Figure 1, AIE fluorophores exhibit bright fluorescence in the aggregated state but very weak fluorescence in good solvent, making them ideal “turn-on” fluorescent probes for bioanalysis (Chen et al., 2020a; Huang et al., 2020a; Li et al., 2020a; Yang et al., 2020a; Zhang et al., 2020b; Korzec et al., 2020). With sufficient special and unique optical properties, including their simple molecular structure (Pratihar et al., 2020), high SNR (signal-to-noise ratio) (Xu et al., 2020a), high luminescence efficiency (Ma et al., 2020a; Fei et al., 2020; Leng et al., 2020), excellent photostability (Jiang et al., 2020a; Mondal et al., 2020a; Wei et al., 2020), and biocompatibility (Xu et al., 2020b; Zhu et al., 2020), AIE fluorescent probes have been widely used as fluorescent components of molecular probes and nanoprobes for biomedical applications in clinical examination and therapy as well as in biological sensing (Gao et al., 2020b; Zhao et al., 2020b; Xu et al., 2020c; Su et al., 2020) and in vivo theranostics.
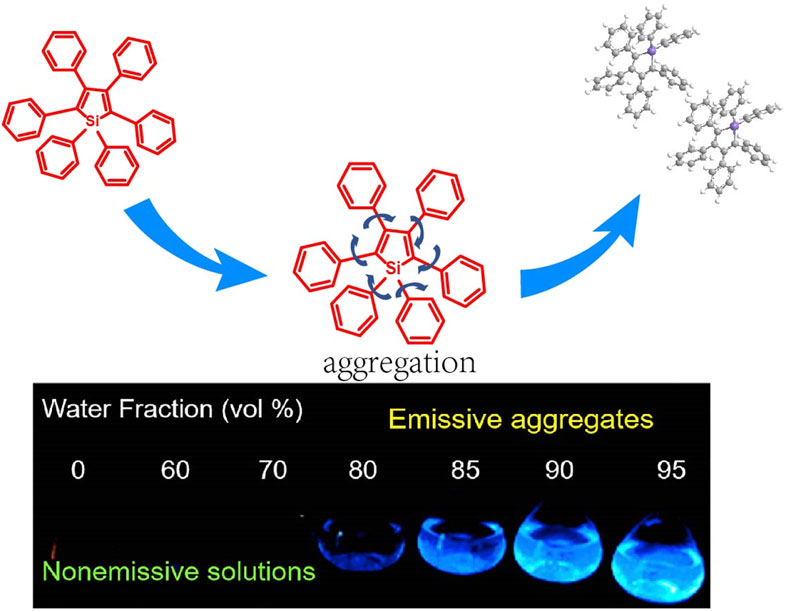
FIGURE 1. Aggregation-induced emission effect: fluorescence photographs of silole molecules in THF/water mixtures under UV light (Dong et al., 2020a).
Herein, we aim to analyze recent advances in AIE molecules and its applications in biotesting and bioimaging, as well as their impact on in medical theranostics. We studied categories of AIE molecules to comprehend the relationship between the luminophore structure and optical properties, the biotesting applications of AIE sensors with target quantitative analysis, and the bioimaging applications of visual demonstration. Finally, we share our outlook about the use of AIE molecules and materials for physiological application.
Categories of AIE materials
The restriction of intramolecular motion (RIM) is the basis of the AIE effect (Mei et al., 2014), which includes the restriction of intramolecular rotation (RIR) and the restriction of intramolecular vibration (RIV). Independent quantum chemical investigations have been conducted aiming for thorough understanding of the relationship between intramolecular motion and AIE properties. Suzuki et al. (2015) explored quenching pathways of three multiluminescent molecules and predicted a largely distorted structure near the minimum energy conical intersection (MECI). The MECI state is easy to achieve in solution but difficult to achieve in solid state and impossible to achieve in crystalline state (Figure 2A). As demonstrated in Figure 2B, the fluorescence quenching of AIEgens in solution must occur through an internal conversion process (S1→S0), whose efficiency is directly affected by the restrictions of intramolecular motions (Suzuki et al., 2020).
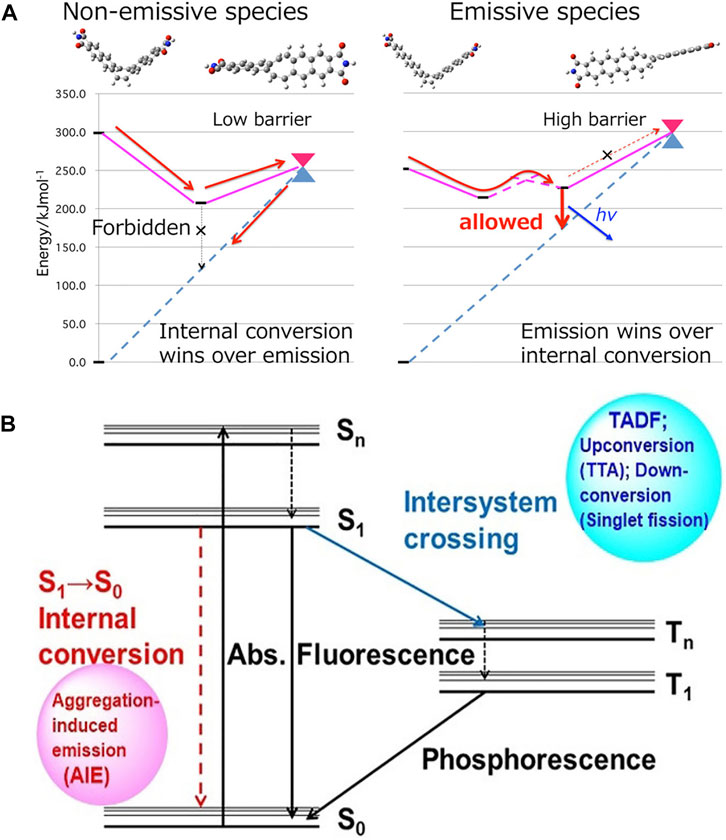
FIGURE 2. (A) Schematic illustration of the conical intersection accessibility in dilute solution (left) and aggregates (right) (Suzuki et al., 2015). (B) Jablonski diagram of an atypical organic fluorophore (Suzuki et al., 2020).
Since Tang first reported hexaphenylsilole (HPS) as the first AIEgen, numerous fluorogens with AIE properties such as tetraphenylethene (Pradhan et al., 2015; Zhao et al., 2020a; Huang et al., 2020b; Jiang et al., 2020b; Zhao L. et al., 2020b; Zhang et al., 2020c; Zhan et al., 2020), siloes (Zhang et al., 2020a), diphenyl sulfones (Zheng et al., 2020), and distyrylanthracene derivatives (Diao et al., 2020; Zhang et al., 2020e) have been studied for analysis and exhibition (Figure 3). Mechanically, most have a non-coplanar structure to reduce the energy barrier. Owing to their small size and low steric hindrance, AIEgens can be modified by cross-linking, esterification, grafting, and molecular and compositional modification to improve their capabilities.
The pattern of manifestation is also important for AIEgens to improve their performance. Synthetic AIE materials such as metal nanoparticles (Munirathnappa et al., 2020; Wu et al., 2020), nonconventional polymers (Dong et al., 2020b; Ma H. et al., 2020b; Yang et al., 2020b; Huang et al., 2020c; Zhang et al., 2020f; Xue et al., 2020), and nanodots (Dong et al., 2020a; Crucho et al., 2020) are generally free of aromatic building blocks and conjugated structures. These synthetic AIEgens generally display concentration-enhanced emission and aggregation-induced emission (AIE) characteristics. Given the presence of these systems, the clustering-triggered emission (CTE) mechanism could help explain the photophysical processes of these synthetic AIEgens (Hu et al., 2020b; Lu et al., 2020).
Application of biotesting
Chemosensor
Compared with the other detection methods, AIE-based fluorescent chemosensors show promise in the detection of ions (Liu et al., 2020; Wang Q. et al., 2020; Li et al., 2020; Wang et al., 2020; Guan et al., 2020; Zhou et al., 2020) and organic molecules (Yan et al., 2020; Yang et al., 2020; Zhao et al., 2020; Xu et al., 2020b; Feng et al., 2020; Rajalakshmi and Palanisami, 2020). According to the excited-state intramolecular proton-transfer (ESIPT) mechanism, the fluorescent properties of the fluorophore skeleton are highly dependent on the solvent environment due to the variation of the intramolecular hydrogen bond.
pH sensor
As one of the most important parameters in chemical, physiological, and biological processes, pH plays an indispensable role in the qualitative and quantitative analysis chemistry. In particular, subtle changes in intracellular pH associated with various cellular processes provide ample information on the studied organisms.
For the past few years, pH tracer based on fluorescence responses has been designed and developed. The commonly used fluorescent structures cover the emission region from visible to near-infrared (NIR), such as tetraphenylethene (TPE) (Chen et al., 2019), coumarin (Meimetis et al., 2014), naphthalimide (Ramasamy and Thambusamy, 2017), rhodamine (Mao et al., 2019), boron dipyrromethene (BODIPY) (Li et al., 2016), and cyanine (Cy) (Hou et al., 2017). To take advantage of the varied classifications of the fluorophores, it is easy to find out the common functional mechanism of these probes: structure transformation attributed to protonation and deprotonation in fluorescein, which makes them strong pH probe candidates.
To date, given the diversity of designing fluorescence molecules, the majority of the existing AIE pH probes were developed through the molecular engineering of electron donors, acceptors, spacers, and D–A conjugated groups (Liu S. et al., 2020). A silacyclopentadiene derivative was the first discovered AIEgens. This was combined with the rhodamine B structure to create an energy donor and acceptor (Wang X. et al., 2020a), which eliminate fluorescence quenching from the non-emissive character of the silole group in an aqueous solution. Complexation with hydrion results in the dredging elutriate test (DRET) procedure and leads to dramatic fluorescence enhancement. As shown in Figure 4, this mixed-mode probe responds to pH from 2.75 to 5, with a red shift of 220 nm.
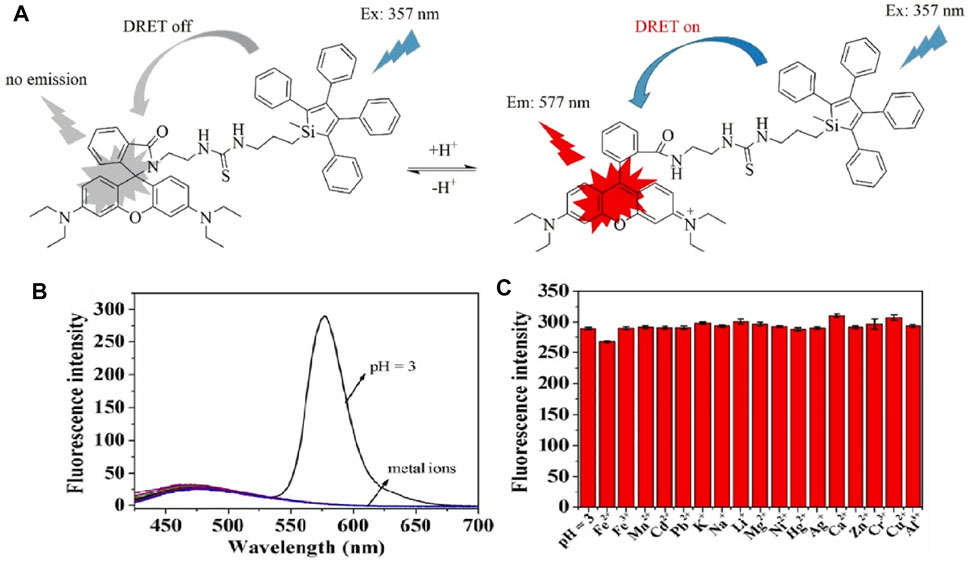
FIGURE 4. (A) Fluorescence properties of silole derivatives in mixed methanol and citrate buffer with adjustment of pH; (B) fluorescence responses of SRH to pH value and metal ions under an excitation of 357 nm. (C) Fluorescence intensity of SRH in mixed methanol and citrate buffer in the presence of marked metal ions.
Among numerous studies, intracellular pH sensing is a characteristic work in which more attention has been paid to biomedical application. In Figure 5, Liu (Wang et al., 2018) designed a ratiometric probe with a TPE donor and a NIR hemicyanine acceptor for sensitive detection of pH changes in live cells. This strategy can be used to develop a variety of novel proportional fluorescent probes to accurately detect different analytes in the chemical reaction by introducing appropriate sensing ligands into the hemicyandiamide group to form the spironolactone switch.
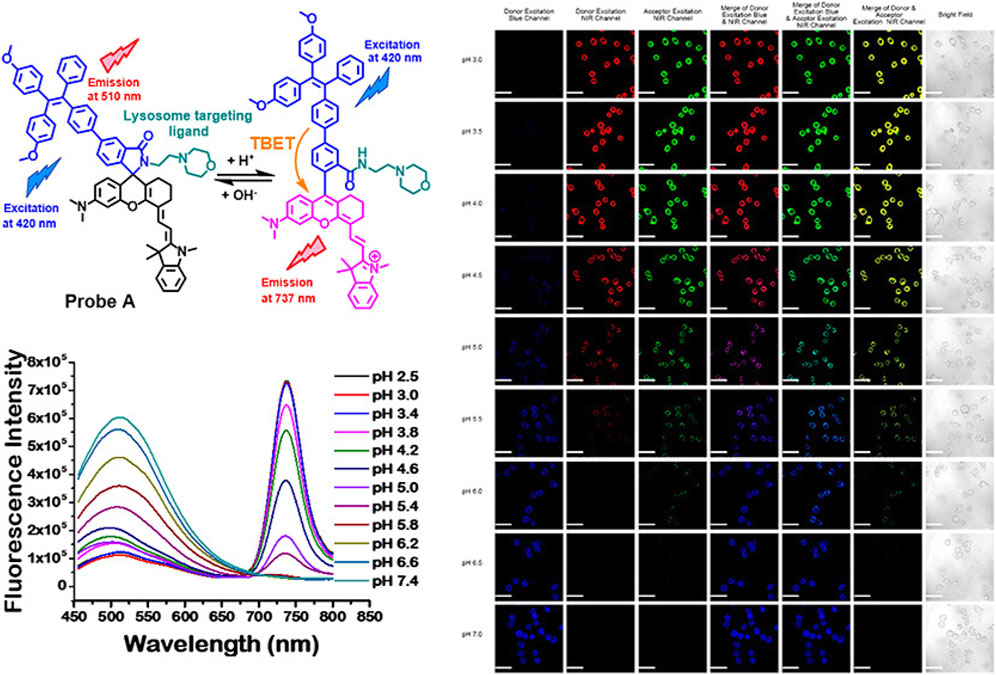
FIGURE 5. Chemical structure responses of ratiometric near-infrared fluorescent probes to pH changes; fluorescence spectra of 5 µM probe A in 10 mM citrate buffers with different pH values, containing 30% ethanol under an excitation of 420 nm; fluorescence images of HeLa cells incubated with 15 µM fluorescent probe A (Wang et al., 2018).
Ion sensor
Being the main form of existence of metals in environmental and living systems, ions can be further divided into cations and anions and possess important implications for all physiological processes. To figure out the quantification and thermal decomposition of ions, chemosensors with AIEgens for ion detection have been studied extensively (Yan L. et al., 2020; Zhai et al., 2020). The commonly used method is the probe that binds with metal ions through noncovalent interactions (mainly metal–ligand coordination), and this recognition process is in a reversible manner. In contrast, another strategy involves an irreversible chemical reaction as induced by a target metal ion that acts as either a reactant or a catalyst, leading to forming a new product after the reaction. Thus, the unique reaction-based indicators are also described as chemodosimeters.
Nitrogen heterocycles with lone pairs of electrons often exhibit aggregation-induced emissive properties. Based on this, Surajit (Mondal S. et al., 2020) designed and prepared triazole-based molecules with outstanding AIE effects (Figure 6). Formed by reacting ether-linked-triazole and pyridine with copper ions intramolecularly, the probe could recognize Cu2+ with a low limit of detection (LOD) in the nM range, demonstrating its high sensitivity, and can be explained by the mechanism of the photoinduced electron transfer (PET) process. Cytotoxicity tests confirmed the potential of the system as a novel cell imaging technique.
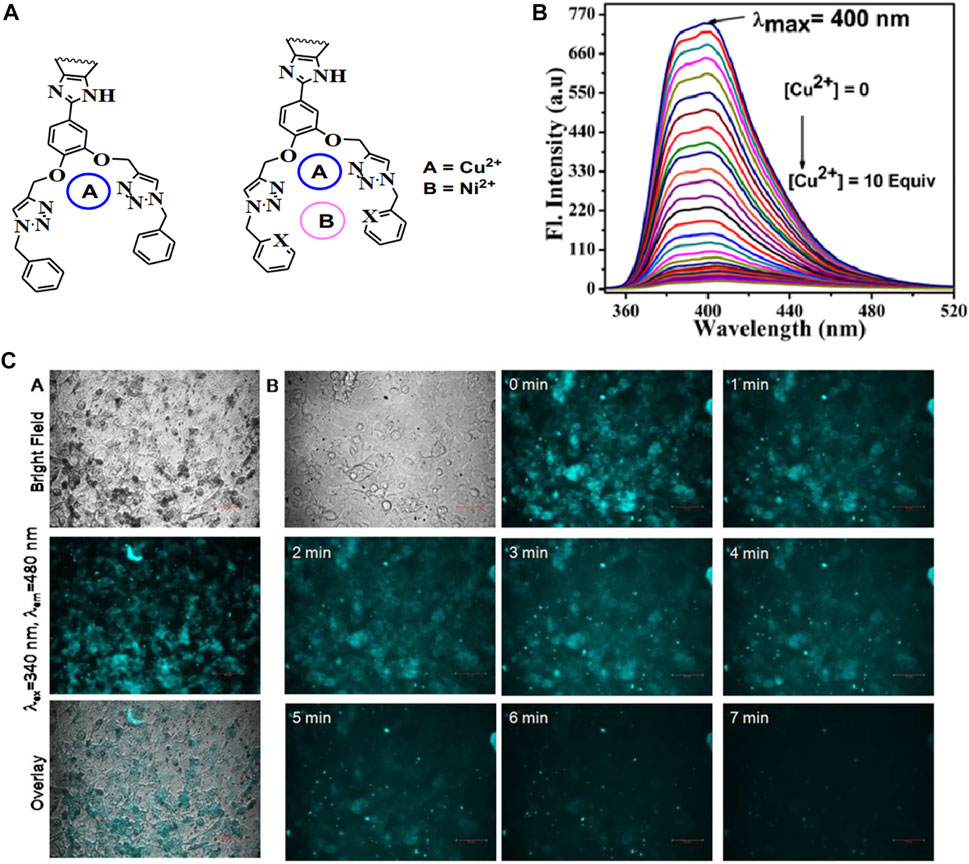
FIGURE 6. (A) Dual-cavities and probable binding mode of the sensors; (B) emission spectra of probe P3 with the incremental amount of Cu2+ in ACN; (C) fluorescent bio-images of C6 (rat glial cell) incubated with P3 and cell incubated with P3 along with Cu2+ with different time intervals (Mondal S. et al., 2020).
Without typical luminophores in its structure, the emission behavior of the ethylene succinamide salt PA24S regarding numerous physicochemical variable parameters as its acid–base properties, temperature, concentration, and anti-interference ability has been discussed by Qinghui Wang et al. (2020). As shown in Figure 7, it was found that the fluorescence emission functioned through aggregation and molecular clusters, which was verified by its spectroscopic properties, micromorphology, and structural characterization by NMR. The probe showed significant sensitivity to Cu2+ in an aqueous solution with effective fluorescence quenching, indicating that PA24S exhibited superior for Cu2+ detection ability and visual monitoring.
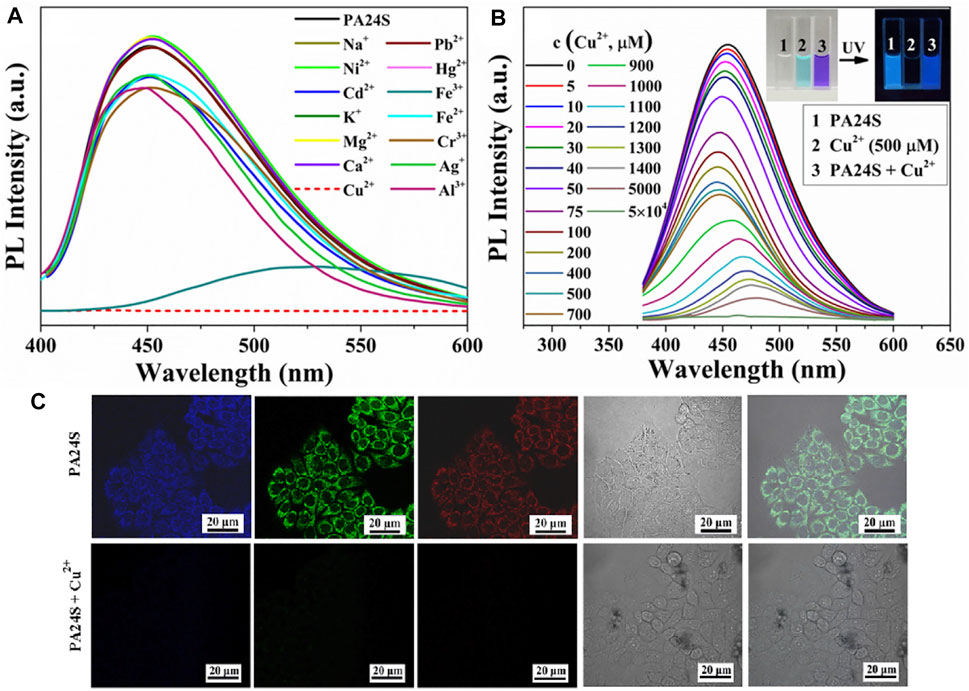
FIGURE 7. (A) Fluorescence emission spectra (λEx = 370 nm) of PA24S solution in the presence of different metal ions; (B) those of PA24S solution as a function of Cu2+ concentration; (C) images of CFM of HeLa cells cultured for 30 min in the presence of PA24S and Cu2+ under 405 nm, 488 nm, 561 nm, bright field, and their overlay (Wang Q. et al., 2020).
Associated with the regulation of biochemical signal transduction in the nervous system, potassium plays critical roles in biological processes including muscle contraction, cardiac motion, nerve conduction, and urinary functions. Fluorescent probes with crown ethers and other structures have been developed and attracted significant attention. Lu (Lu et al., 2017) designed a novel G-quadruplex structure-based K+ probe (Figure 8). Benefit from the TPE AIEgen, the probe exhibits high sensitivity with extended photostability, which facilitates the prolonged fluorescence observations of K+ in living cells.
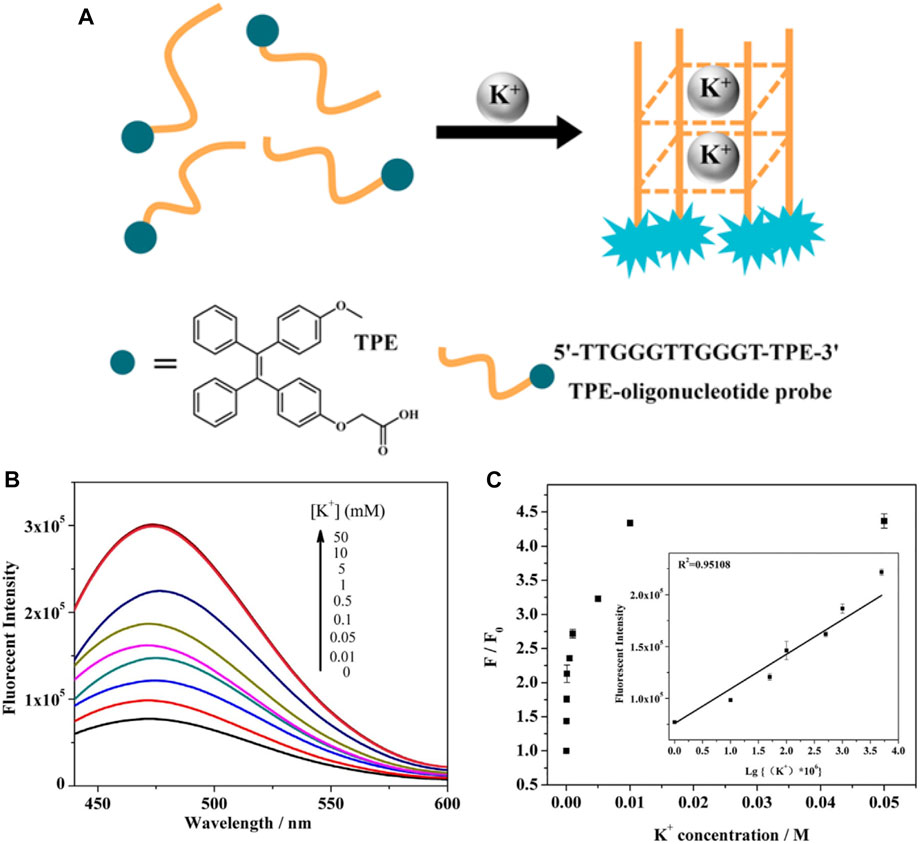
FIGURE 8. (A) Schematic illustration for TPE-oligonucleotide K+ probe; (B) fluorescence spectra for different concentrations of K+ based on the AIE effect of TPE-oligonucleotide probe. (C) Plot of fluorescence enhancement (F/F0) at 475 nm as a function of K+ concentration (Lu et al., 2017).
Nanoparticles with AIEgens hold tremendous potential as a tracer material, for they can penetrate biotic tissues with no damage. AIE-based fluorescence resonance energy transfer (FRET) nanoparticles (Nie et al., 2020a) were developed to recognize ClO− in living cells (Figure 9). Different from common FRET-based nanosensors, the NPs were prepared via facile co-assembly strategy in water combining amphiphilic tetraphenylethene with thienyl-diketopyrrolopyrrole. The fluorescent imaging of the probe with ClO− demonstrates how NPs can effectively pass through the cell membrane and safely recognize ClO− in living cells.
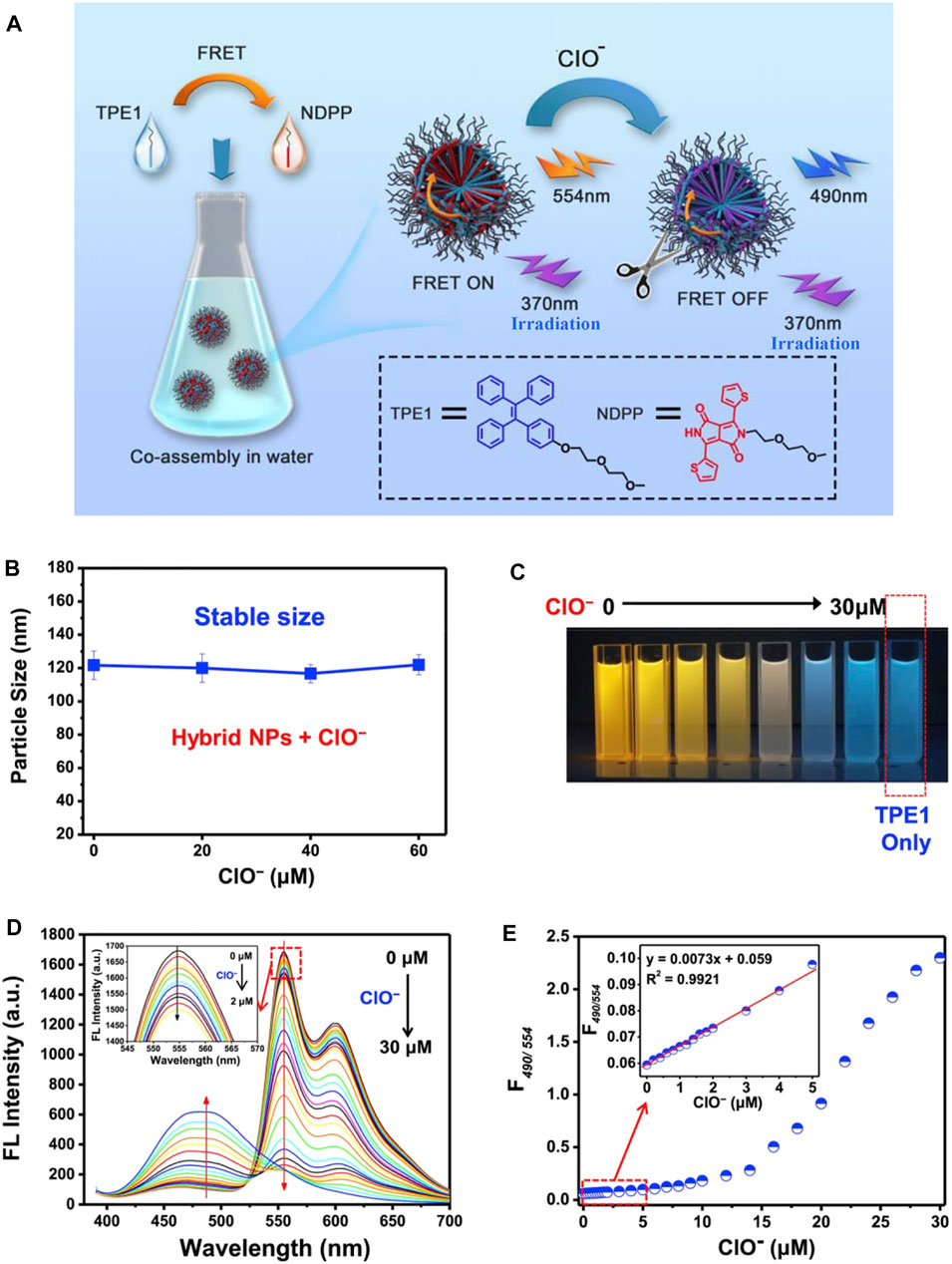
FIGURE 9. (A) Construction of a micellar FRET nanoprobe composed of a TPE/NDPP pair and the effect of ClO-; (B) changes in the DLS size of TPE1-NDPP NPs upon the addition of increasing amounts of ClO− in water; (C) photons of a solution with TPE1-NDPP NPs and different amounts of ClO− (0, 4, 8, 16, 20, 25, and 30 μM) under a UV lamp; (D) changes in the fluorescence spectra of the TPE1-NDPP NPs upon the addition of increasing amounts of ClO− (1–30 μM) in water. Inset: the addition of a low concentration of ClO− (0, 0.2, 0.4, 0.6, 0.8, 1.0, 1.2, 1.4, 1.6, 1.8, 2.0 μM); and (E) fluorescence intensity ratio (F490/554) changes of TPE1-NDPP NPs upon exposure to ClO− (Nie et al., 2020a).
Molecular sensor
In consideration of the different properties of objects including charge, hydrophobicity, bioactivity, chemical activity, etc., numerous sensors have been designed and developed for monitoring small molecules by rationally modulating recognition units and luminescent units (Yao et al., 2011). A distyrylanthracene-based molecule was developed with four carboxyl groups to improve the combining capacity toward amino acids (Jiang R. et al., 2020a). As shown in Figure 10, with structure similar to tetraphenylethene (TPE), this convenient and sensitive fluorescent probe with AIE character can distinguish protamine, heparin, and heparinase. The detecting mechanism was found to be aggregation caused by electrostatic attraction and enhanced the fluorescence of the system.
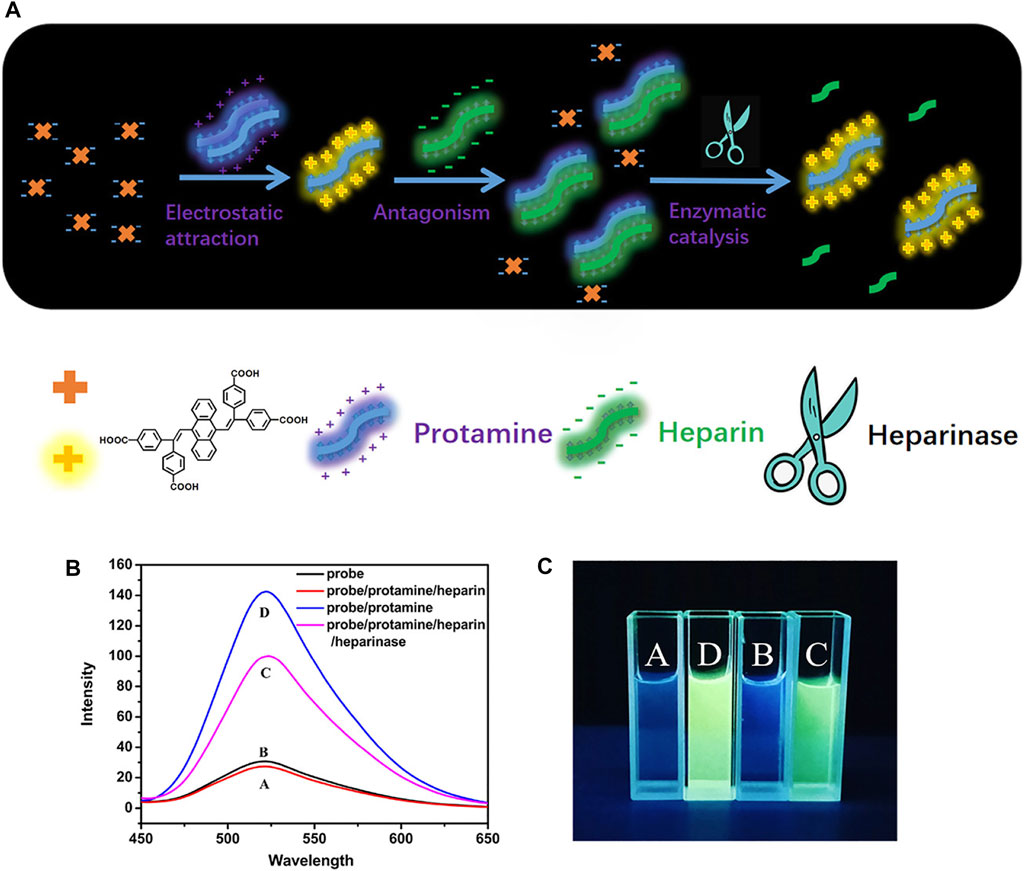
FIGURE 10. (A) Self-assembly of the copolymer (HSP-TPE) containing fluorescent TPE probe and sensing behavior of HSP-TPE aggregates toward picric acid (PA); (B) fluorescence spectra of DSA-4COOH, DSA-4COOH/PRO/HEP complex, DSA-4COOH/PRO/HEP/heparinase complex, and DSA-4COOH/PRO complex; and (C) photograph of digital photos of DSA-4COOH with protamine, heparin, and heparinase under irradiation at 365 nm.
Furthermore, the detection of other molecules, such as pesticide, toxin, and explosives (TNT, TNP, etc.), also has great implications for personal and social safety. TPE-grafted hyperbranched copolymers (Nabeel et al., 2020) were used to form a homogeneous solution in THF for the sensitive detection of picric acid (PA) (Figure 11). The aggregates showed great fluorescence enhancement in the THF/water mixture solution upon addition of TPE units to the polymers. This granted them high sensitivity to PA, with detection limits as low as 20 PPB. To a certain extent, it can create safer environments and reduce risk factors.
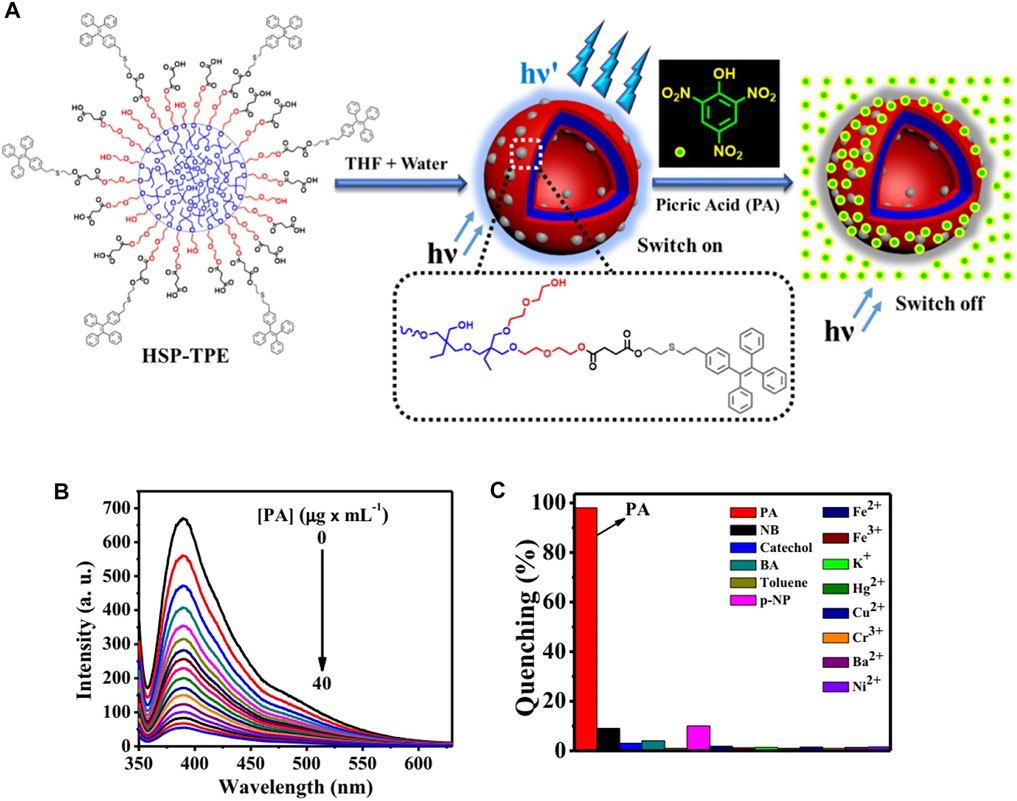
FIGURE 11. (A) Illustration of self-assembly of the copolymer (HSP-TPE) containing fluorescent TPE probe and sensing behavior of HSP-TPE aggregates toward picric acid (PA); (B) fluorescent spectra of (A) HSP-TPE in THF/water (3:7 v/v) mixture with varied amount of picric acid (PA) at an excitation wavelength of λ = 333 nm; (C) extent of fluorescence quenching efficiency of HSP-TPE by adding 10 equiv of picric acid (PA) and other molecules (Nabeel et al., 2020).
Biosensors
Detection of the biomolecules in the body fluid appears to be essential for disease diagnosis, monitoring, and management (Sargazi et al., 2022). Owing to their simple structure, low background noise, excellent photostability, and admirable biocompatibility, AIE molecules are beginning to establish themselves as significant tools in the areas of life science for biosensor (Zhang L. et al., 2020b; He et al., 2020) and comprehending bioprocesses (Wang et al., 2015; Gu et al., 2017; Gao F. et al., 2020). To elucidate the biological functions of fluorescent probes or drugs (Wang Z. et al., 2020), a comprehensive understanding of their intracellular division is of vital importance (Dong Y. et al., 2020; Tang et al., 2020). The most common pattern for detecting biological macromolecules is functionalizing with small hydrophilic groups such as amine groups, sulfonic acid groups, or hydrophilic peptide chains to greatly improve the water solubility of AIE molecules and then utilizing the electrostatic interaction, hydrophobic interaction, and receptor-assisted interactions between the water-soluble AIE molecules and biological macromolecules to turn on/off the fluorescence and achieve the detection (Xu et al., 2012).
As discussed above, AIE fluorophores (triphenylethene and tetraphenylethene) can be conjugated with biomolecules by condensation or click-reaction, in order to enhance their biocompatibility and bioactivity. Niu et al. (2020) chose quaternized tetraphenylethene salt as the AIE fluorescent module probe, which binds to single-stranded DNA by the electrostatic interaction. As shown in Figure 12, in the presence of DNA MTase, the methylation reaction-initiated DNA polymerization occurs with terminal deoxynucleotidyl transferase (TdT), which activated the fluorescence intensity through AIE. The assay was also effective for the detection of DNA MTase activity in human serum, demonstrating the inhibitory effect of 5-fluorouracil on Dam MTase.
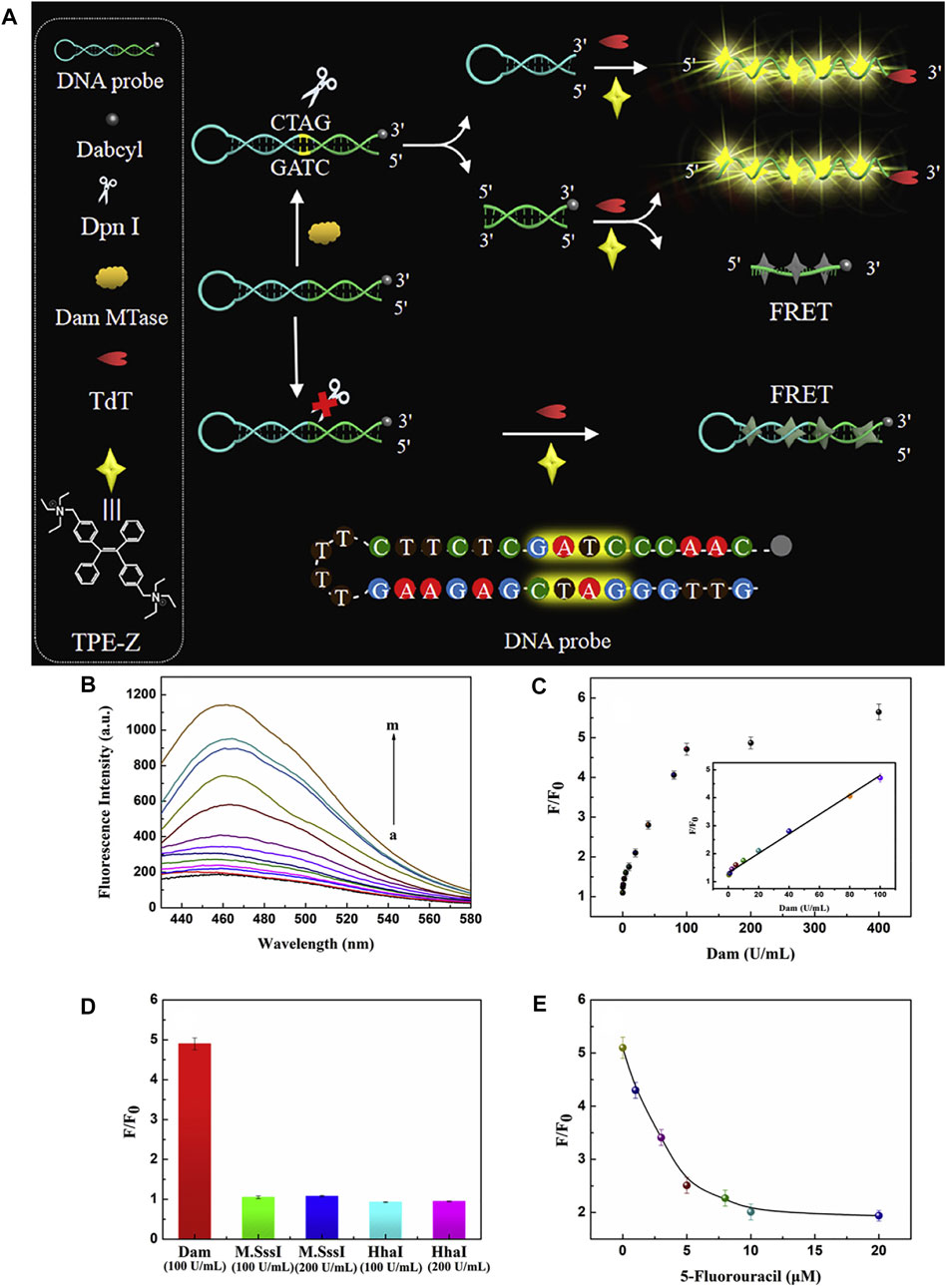
FIGURE 12. (A) Illustration of Dam MTase activity assay based on aggregation-induced emission and template-free DNA polymerization. (B) Fluorescence spectra of TPE-Z in response to Dam MTase in different concentrations (0, 0.1, 0.5, 1, 2.5, 5, 10, 20, 40, 80, 100, 200, 400 U/mL). (C) Corresponding fluorescence ratio at 462 nm. The inset represents the linear relationship between the signal and Dam MTase concentrations from 0.5 U/mL to 100 U/mL. (D) Selectivity study. (E) Inhibition assay for Dam MTase with different concentrations of 5-fluorouracil. The error bars were based on three repetitive experiments performed (Niu et al., 2020).
Since proteins are the key components of the organelle, the quantitative study thereof is important for understanding the basic principle of cellular systems and the related diseases (Olzscha et al., 2011; Burslem and Crews, 2017). It is essential to realize the progress of protein aggregation in biological samples before investigating effective therapies. Liu et al. (2022) developed a series of AIE probes based on 4-hydroxybenzylidene-imidazolinone (HBI) (Figure 13). The varying viscosity sensitivities of AIEgens were explored systematically to visualize protein aggregation in live cells, and other biological processes related to local viscosity changes were also investigated.
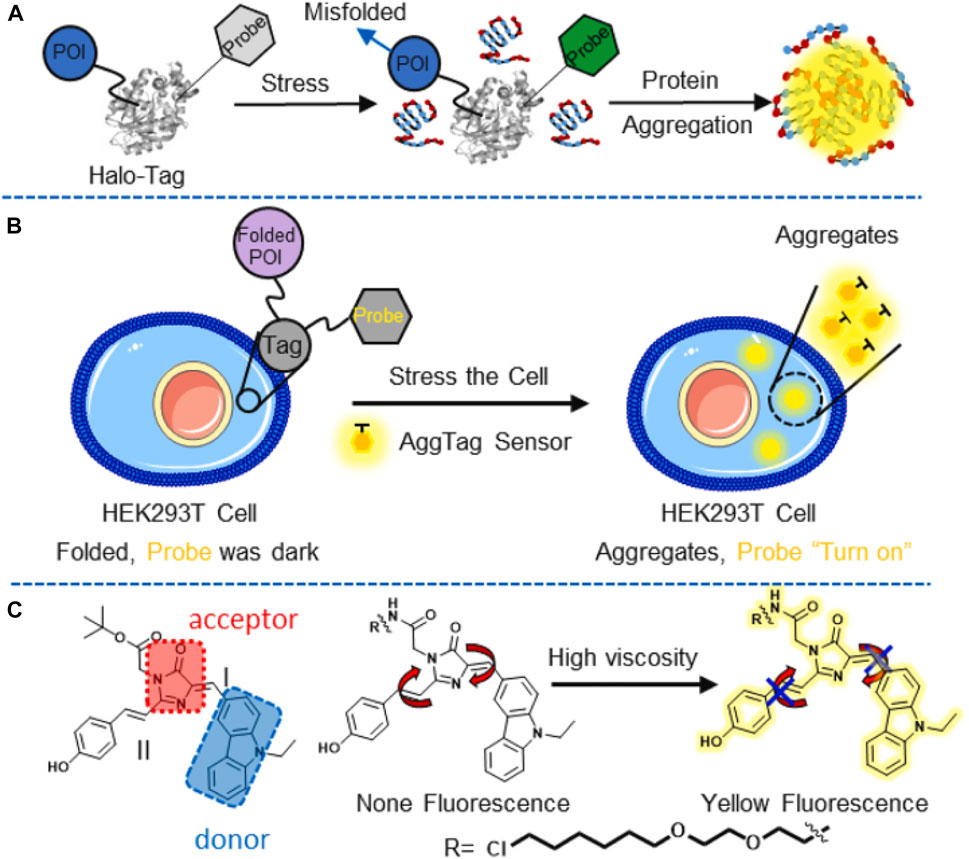
FIGURE 13. (A) Illustration of the Agg-Tag method; POI: protein-of-interest, Probe: Agg-Fluor probes; (B) schematic demonstration of the probe detection of aggregated proteins in HEK293T cells; and (C) fluorescence of the probe is turned on by rotation restriction at I and II region in highly viscosity microenvironment (Liu et al., 2022).
Application of bioimaging
To garner powerful tools for cancer diagnosis and medicinal therapy, fluorescent tracers and dyes have been generally fabricated by encapsulating AIEgens into lipid or BSA shells through a nanoprecipitation route, which allows further surface functionalization with specific recognition units (Wang X. et al., 2020b). The inherent ACQ effect of conventional organic luminophores has been a thorny problem to solve, while the AIE molecule offers more possibility for tracing and mapping special tissues. Because of their low cytotoxicity and high quantum yield, AIE tracers can achieve bioimaging in living systems (Nie et al., 2020b; Zhao W. et al., 2020; Wang X. et al., 2020c). In this case, the development of AIE tracers that enable the in vivo monitoring and long-term tracing biological processes with high resolution and sensitivity is of critical importance in both fundamental biological science and practical clinical applications.
Cell imaging
Among the molecular, organelle, and cellular level, organelles may represent suitable targets for the detection, imaging, and treatment of diseases. AIEgens modified with various targeting groups could serve as superior imaging agents for monitoring of the dynamics of various biological process-related organelles and enzymes (Gu et al., 2017). Receptor targeting is one of the general strategies for cell imaging due to the overexpression of specific receptors, which is strongly linked to the progression of disease (Ko et al., 2019). Abnormal intracellular lipid droplets (LDs) are important biomarkers of multifarious diseases and are associated with cancer, obesity, fatty liver, Alzheimer’s disease, and other diseases. Thus, the visual monitoring of LDs is of great significance. Yuxuan Wang et al. (2020) combined hydrophobic AIEgen tissue polypeptide antigen (TPA) with lecithins via the condensation of Schiff base for targeted aggregation on LDs in HeLa cells with high SNR. Induced by UV light (493 nm), the real-time tracing of LDs was achieved to explain cellular processes (Figure 14).
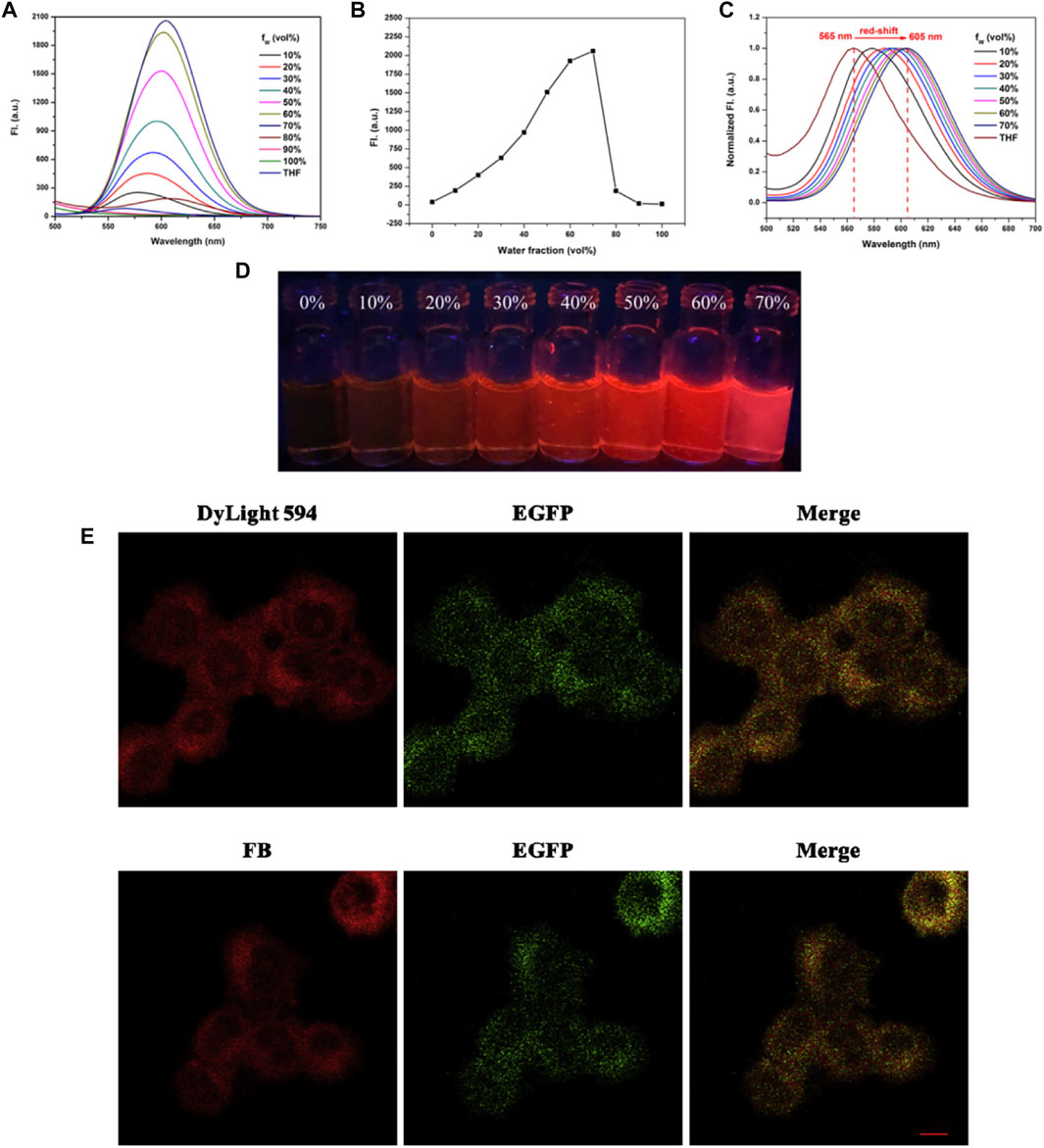
FIGURE 14. AIE characteristic study of the probe FB. (A) Fluorescence spectrum of the probe FB in THF-H2O solution. (B) Fluorescence intensity of the probe FB at 605 nm in various water fractions. (C) Normalized fluorescence spectrum of the probe FB in THF-H2O solution. (D) Photographs of the probe FB in THF-H2O solution under UV lamp (365 nm). (E) Cell staining images in the transfected PC-12 cells (Wang Y. et al., 2020).
In addition, 9,10-dithienylanthracene (DTA) derivatives with a rigid plane structure often exhibit desirable AIE behaviors and fluorescence efficiency in the near-infrared region. Wang developed a novel fluorescence tracer with a similar structure (Wang R. et al., 2020). Multiple hydrogen bond interactions between neighboring molecules limit their intramolecular rotation, allowing for significant fluorescence enhancement (Figure 15). The results confirmed that substituent groups had a significant effect on their molecular packing, morphology observation, and optical properties. Moreover, the DTA molecules reported could be used fluorochrome in the nucleolus and localized uniformly in HeLa cells.
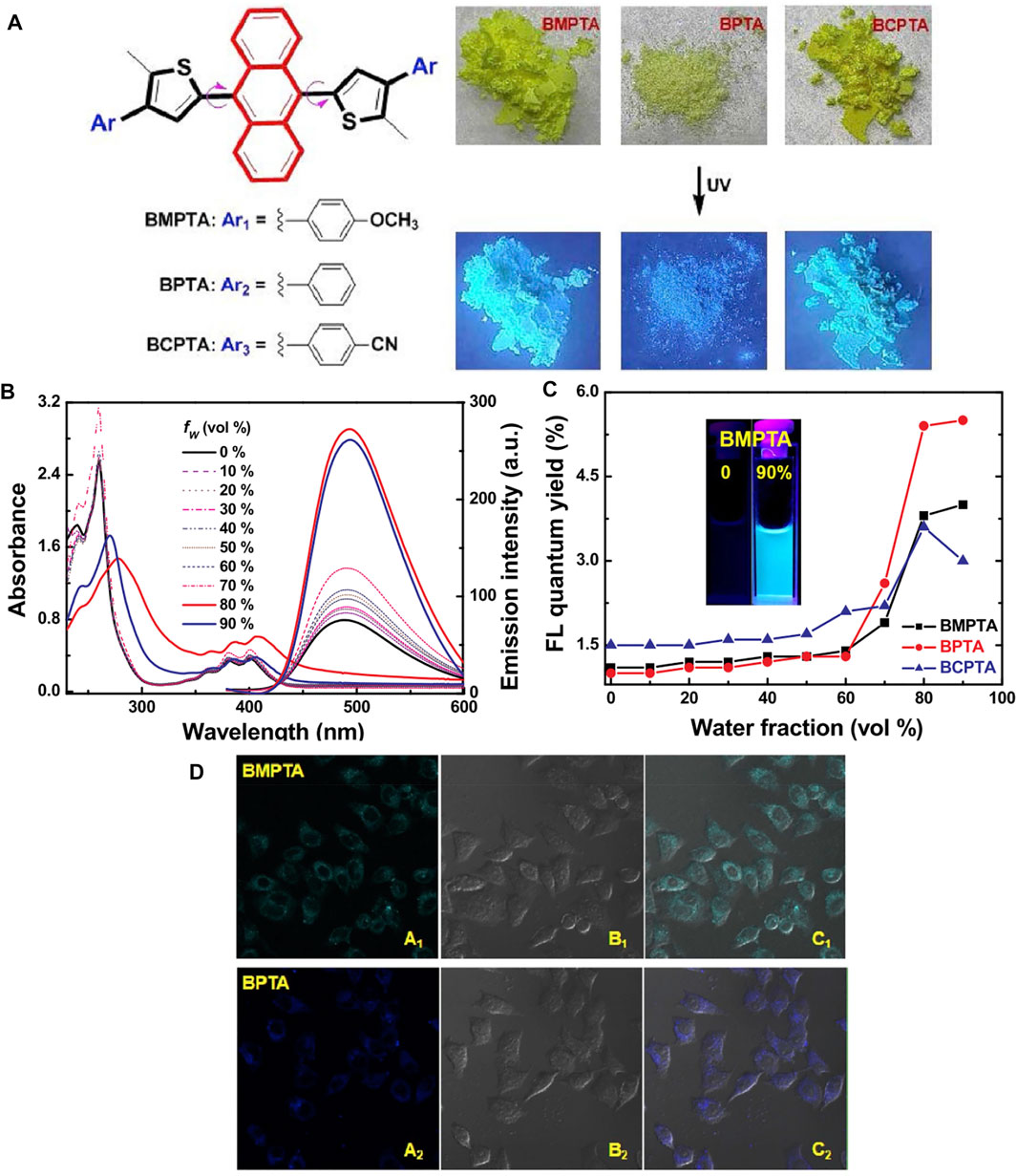
FIGURE 15. (A) Molecular structures of DTA derivatives and their photographic images under 365 nm UV light; (B) the absorption and fluorescence spectra of BMPTA in THF/H2O with different fractions; (C) their changes in absolute fluorescence quantum yield versus water fraction in THF; and (D) fluorescent confocal microscopy images of HeLa cells stained with 20 μM of BTA compounds for 30 min dark field images, bright field images, overlaid of dark and bright field images (Wang R. et al., 2020).
Amphipathic poly (PEGDA-co-TPE) copolymers were synthesized via a catalyst-free one-pot Passerini reaction (Jiang R. et al., 2020b) under fairly mild experimental conditions. In comparison with small fluorescence molecules, the macromolecular colorants have high luminous efficiency, large Stokes shift, and remarkable water dispersibility (with the hydrophilic group), demonstrating the great potential for mapping and tracing target cells in vivo (Figure 16).
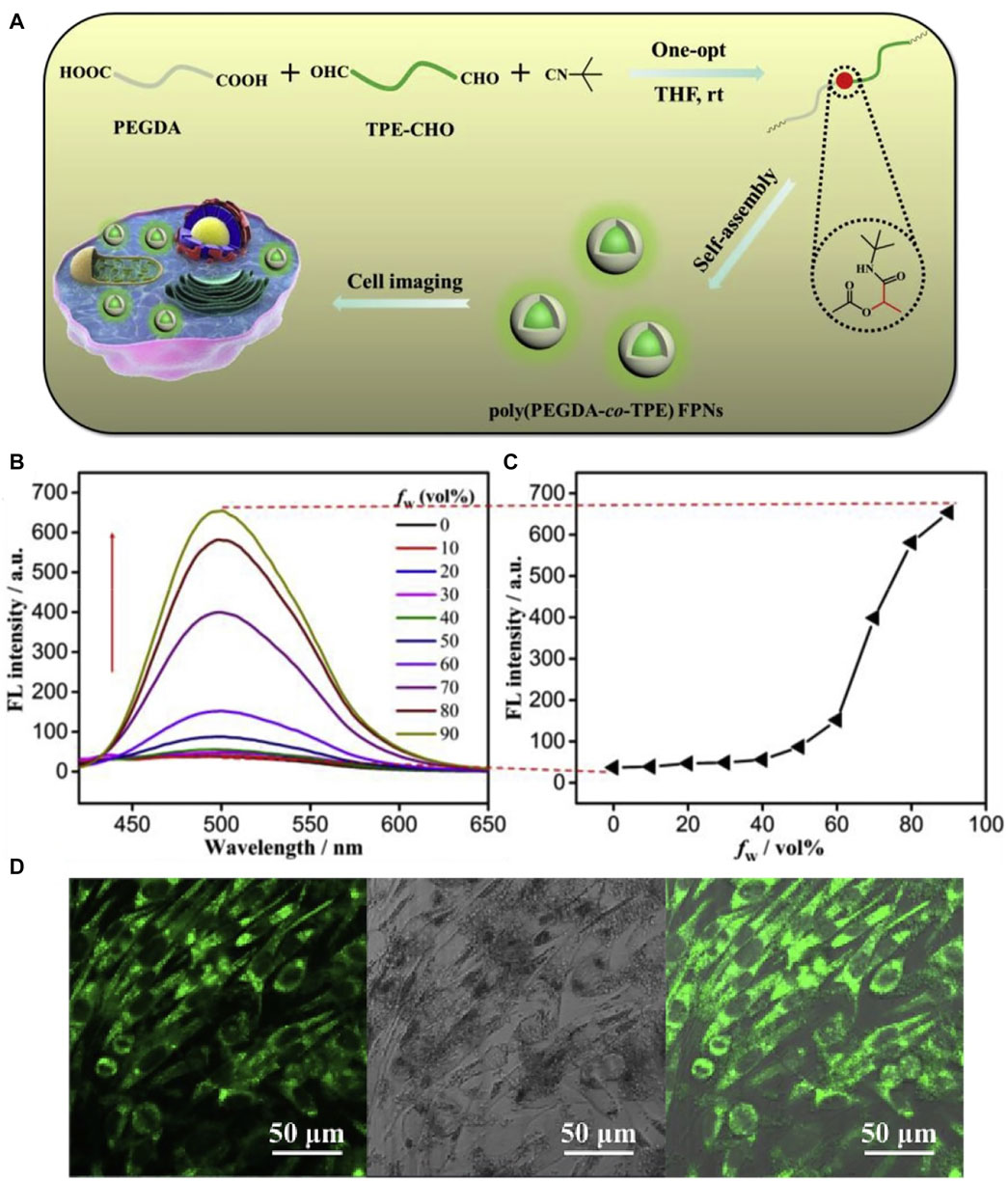
FIGURE 16. (A) Schematic showing the preparation of poly (PEGDA-co-TPE) FPNs for cell imaging; (B) the fluorescent spectra of poly (PEGDA-co-TPE) in DMF/water mixtures with different water fractions; (C) the relationship of fluorescent spectra of poly (PEGDA-co-TPE) versus water fraction in the mixed solution; and (D) CLSM images of L929 cells incubated with 40 μg ml−1 of poly (PEGDAco-TPE) FPNs (Jiang R. et al., 2020b).
Tissue imaging
Unlike cell imaging, tissue imaging by AIEgen offers two main benefits. The high luminescence efficiency and structural stability of AIE materials warranted improved in vitro tissue imaging; furthermore, with the assistance of fluorescence sensors, medical staff could define boundaries of pathological tissue and delineate tiny tumor nodules more precisely, remarkably improving the success rate of surgeries. As tumor resection surgery remains the preferred and most commonly used strategy, accurate intraoperative tumor resection is guided by optical imaging to address these severe problems taking advantage of its excellent sensitivity, real-time modality, super temporal resolution, and fine biosafety (Chen C. et al., 2020). This strategy was supported by the mapping performance of AIE probes, as a precise and effective means of tracing and treating pathological tissues.
Symmetric structure sometimes allows for the precise localization of tumor tissues. Qi et al. (2020) designed a novel dragonfly-shaped NIR molecule with the AIE structure of TPE (Figure 17). Through self-assembly, the nanoparticles possess exciting long-term tumor mapping capacity; the accumulation of different organs was also investigated, indicating that this molecule ensures the efficiency of in vivo tumor imaging.
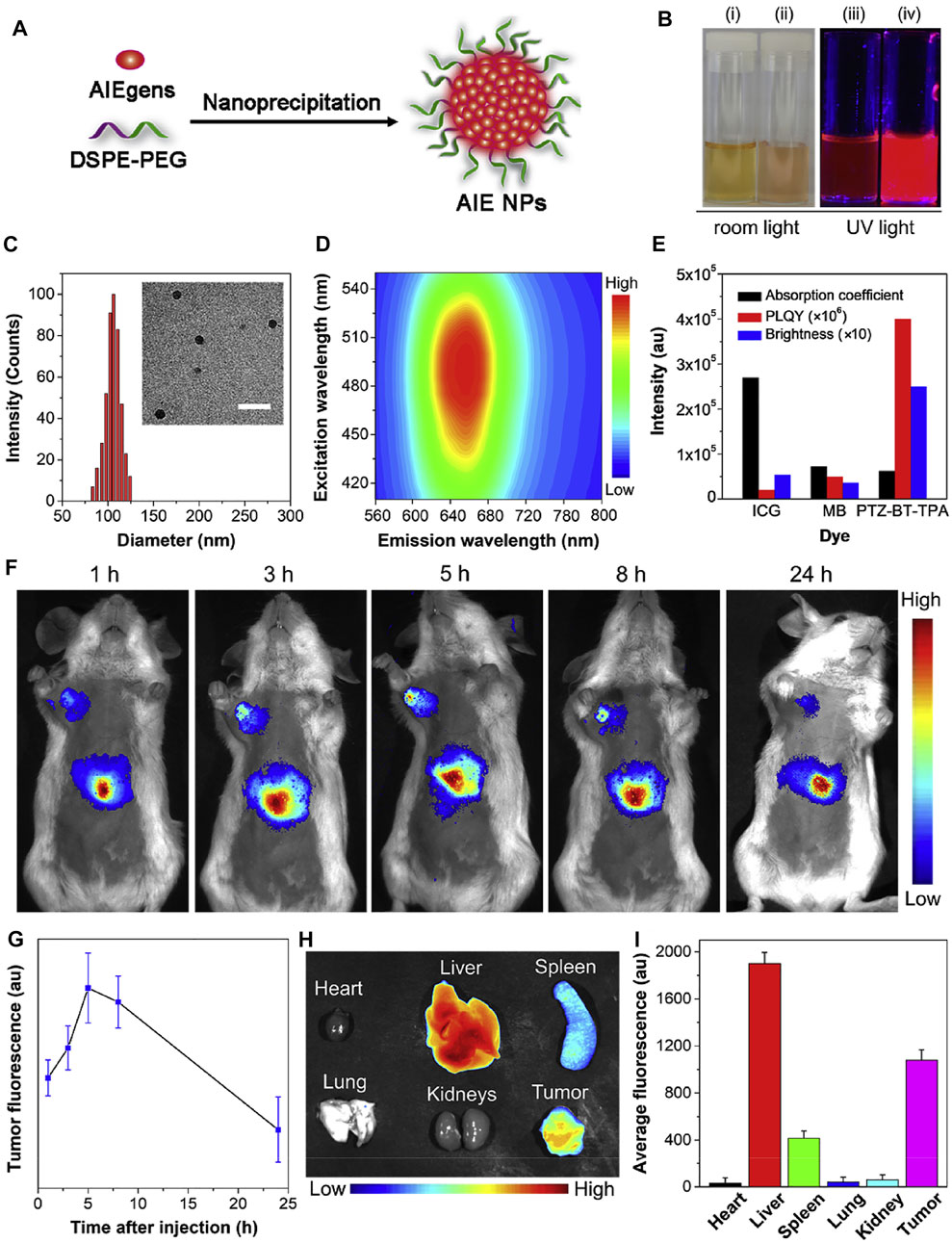
FIGURE 17. (A) Schematic illustration of the preparation of AIE-NPs through a nanoprecipitation method. (B) Photographs of PTZ-BT-TPA (i, iii) in THF and (ii, iv) as NPs under room and UV light (365 nm), respectively. (C) Representative DLS profile and TEM image of the AIE-NPs. Scale bar = 300 nm. (D) Photoluminescence excitation mapping of the AIE-NPs. (E) Comparison of the absorption coefficient, PLQY and brightness of ICG, MB and PTZ-BT-TPA in water. (F) In vivo fluorescence imaging of tumor-bearing mice and (G) the corresponding fluorescence intensity of tumor site at different time intervals after intravenous injection of the AIE-NPs. Data are presented as the means ± SD (n = 3). (H) Ex vivo fluorescence image and (I) fluorescence intensity of main organs (heart, liver, spleen, lung, and kidneys) and tumor after intravenous administration of AIE-NPs for 24 h.
AIE materials are more than excellent tool of detection and exhibition. In recent years, aggregation-induced emission photosensitizers (AIE-PSs) with strong photosensitization perform well in the efficient tumor photodynamic therapy. For the design of functional materials, load factor is regarded as an important parameter of graft, modification, and composition. Cheng et al. (2020) designed novel pH-responsive nanoparticles self-assembled by amphiphilic AIE molecule with a 100% loading efficiency. As shown in Figure 18, the fluorescence quantum yield of AIE-NPs reached 56.7%, better than the probes commonly used in clinic. As a result, the AIE-NPs exhibited an effect on extracorporeal photodynamic therapy in vitro under white light (50 mW cm−2). The experiment using tumor-bearing mice verified the high tumor accumulation, penetration, and therapeutic efficiency of AIE-NPs.
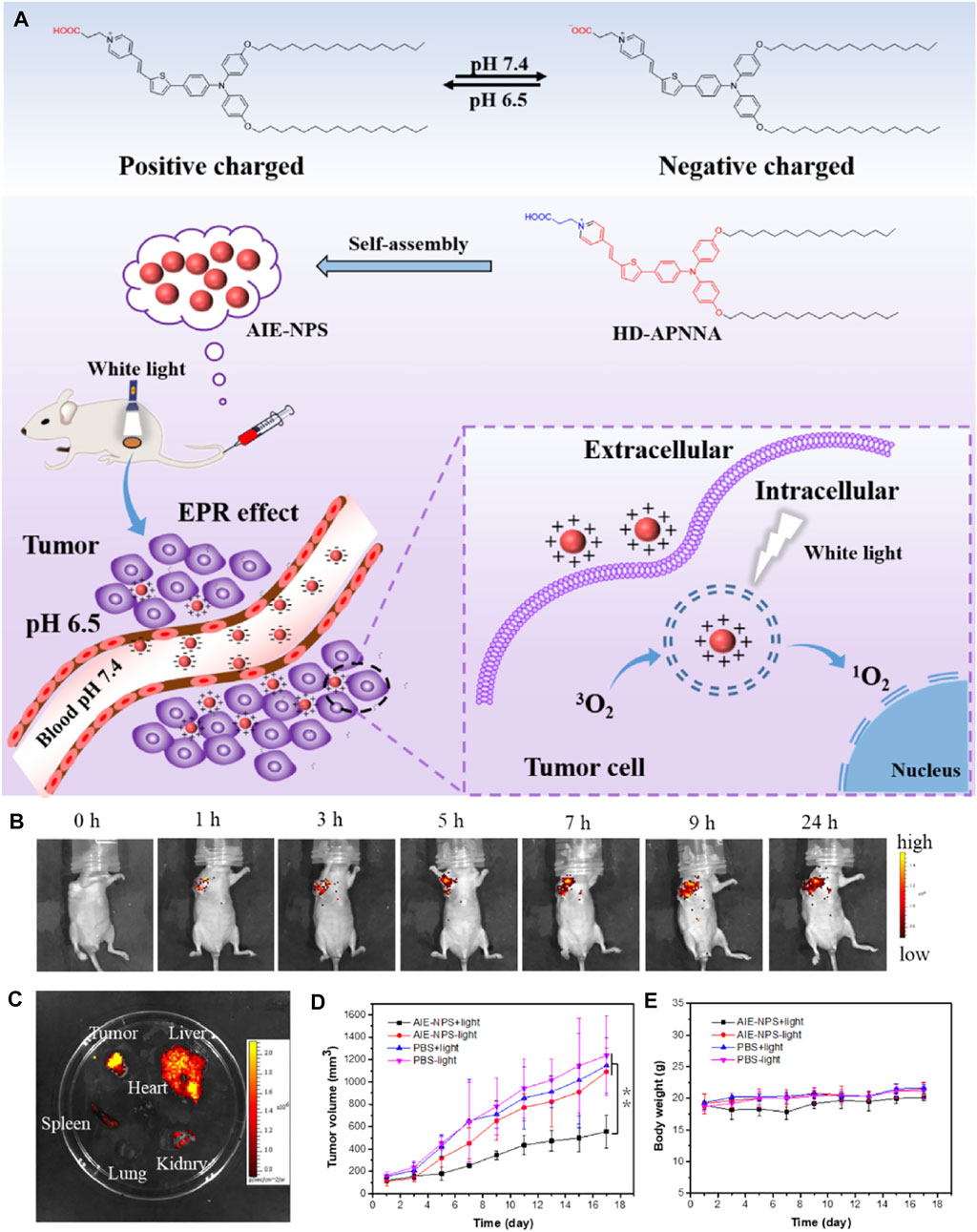
FIGURE 18. (A) Illumination of carrier-free AIE-NPs for tumor photodynamic therapy. (B) In vivo fluorescence images of HeLa tumor-bearing mice after intravenous injection with the AIE-NPs (1 mg/ml, 50 μL per mouse). (C) Ex vivo fluorescence images of organs and tumor at 9 h after intravenous injection of the AIE-NPs. (D) Volume growth curves of tumors at different time points posttreatment in different groups (n = 3, *p < 0.05, **p < 0.01. (E) Body weight measurement of the mice in each group (Cheng et al., 2020).
AIE molecules can monitor key factors during biological processes with high precision and satisfying reliability in real time in addition to acting as the delivery platform of drugs, similar to the image-guided therapeutic response. The therapeutic AIE-active materials are still in the earlier developmental stages but have the potential to create a new direction toward the study of bio-optical material, and much more work should be accomplished in this promising pursuit.
Conclusion and perspectives
In summary, we have detailed the recent research strategies of AIE molecules, which can potentially be used in biological testing and imaging applications. The recent synthesis of numerous AIE molecules has offered a great opportunity to study the photophysical properties of such compounds revealing the subtle mechanisms that led to this phenomenon.
Although tremendous development in the study of AIE molecules has been achieved, there are still plenty of opportunities and challenges remaining in this field. Achieving the following research goals will deepen our comprehension and help to facilitate the creation of innovative light-based biotechnologies: 1) broadening the scope of mechanisms for building novel AIE-based structures; 2) designing novel molecules with high light penetrability and high quantum yield; 3) combining of sensing and imaging strategies for the detection of target biomolecules and in vivo fluorescence imaging; and 4) designing and manufacturing of AIE biosensors with multi-model sensors and imaging capabilities (MRI, THz, photoacoustic imaging, etc.).
The development of novel AIE-based molecules/materials is highly desirable for many applications, e.g., biomolecular sensing, biological imaging, chemical sensing, as stimuli-responsive materials, and optoelectronic systems. The simplicity and promising advantages of AIE systems will encourage scientists to study new AIE materials, not only increasing the variety of molecules developed but also working toward biological and physiological applications for the accurate diagnosis and treatment of diseases.
Author contributions
JM received a PhD degree in the Department of Chemistry and Chemical Engineering at Southwest Petroleum University, Chengdu, China. He is a lecturer in Shandong First Medical University. His research interest lies in biosensors based on biomaterials and precise medical diagnosis. YG has a Master’s degree from Central South University, Changsha, China. She is a teaching assistant in Shandong First Medical University. Her research interests are bioengineering and multi-model imaging. DM has a PhD degree from Shandong University, Jinan, China. He is a lecturer in Shandong First Medical University. His research interests are polymeric biomaterials. WL has a Master’s degree from Fuzhou University, Fuzhou China. He is an experimentalist in Shandong First Medical University. His research interests are medical imaging processing and rebuilding. JQ has a PhD degree from Capital Medical University, Beijing, China. He is a professor in Shandong First Medical University. His research interest lies in medical functional imaging.
Funding
This work was supported by the Science and Technology funding from Jinan (No. 2020GXRC018); the Taishan Scholars Program of Shandong Province, China (No. ts201712065); and the academic promotion program of Shandong First Medical University, China (2019QL009).
Conflict of interest
The authors declare that the research was conducted in the absence of any commercial or financial relationships that could be construed as a potential conflict of interest.
Publisher’s note
All claims expressed in this article are solely those of the authors and do not necessarily represent those of their affiliated organizations, or those of the publisher, the editors, and the reviewers. Any product that may be evaluated in this article, or claim that may be made by its manufacturer, is not guaranteed or endorsed by the publisher.
References
Burslem, G. M., and Crews, C. M. (2017). Small-molecule modulation of protein homeostasis. Chem. Rev. 117 (17), 11269–11301. doi:10.1021/acs.chemrev.7b00077
Cai, L., Sun, X., He, W., Hu, R., Liu, B., and Shen, J. (2020). A solvatochromic AIE tetrahydro[5]helicene derivative as fluorescent probes for water in organic solvents and highly sensitive sensors for glyceryl monostearate. Talanta 206, 120214. doi:10.1016/j.talanta.2019.120214
Chen, C., Tang, Y., and Ding, D. (2020). Intramolecular motion-associated biomaterials for image-guided cancer surgery. Smart Mater. Med. 1, 24–31. doi:10.1016/j.smaim.2020.05.001
Chen, H., He, X., Yu, Y., Qian, Y., Shen, J., and Zhao, S. (2020). Execution of aggregation-induced emission as nano-sensors for hypochlorite detection and application for bioimaging in living cells and zebrafish. Talanta 214, 120842. doi:10.1016/j.talanta.2020.120842
Chen, W., Zhang, C., Han, X., Liu, S. H., Tan, Y., and Yin, J. (2019). Fluorophore-labeling tetraphenylethene dyes ranging from visible to near-infrared region: AIE behavior, performance in solid state, and bioimaging in living cells. J. Org. Chem. 84 (22), 14498–14507. doi:10.1021/acs.joc.9b01976
Cheng, G., Wang, H., Zhang, C., Hao, Y., Wang, T., Zhang, Y., et al. (2020). Multifunctional nano-photosensitizer: A carrier-free aggregation-induced emission nanoparticle with efficient photosensitization and pH-responsibility. Chem. Eng. J. 390, 124447. doi:10.1016/j.cej.2020.124447
Crucho, C. I. C., Avo, J., Nobuyasu, R., S, N. P., Fernandes, F., Lima, J. C., et al. (2020). Silica nanoparticles with thermally activated delayed fluorescence for live cell imaging. Mater. Sci. Eng. C 109, 110528. doi:10.1016/j.msec.2019.110528
Diao, L., Zhang, J., Wang, R., Liu, G., and Pu, S. (2020). Synthesis and properties of asymmetric 9, 10-dithienylanthracene derivatives with AIEE properties and their applications in cell imaging. J. Photochem. Photobiol. A Chem. 400, 112663. doi:10.1016/j.jphotochem.2020.112663
Dong, F., Lai, H., Liu, Y., Li, Q., Chen, H., Ji, S., et al. (2020). Highly selective isomer fluorescent probes for distinguishing homo-/cysteine from glutathione based on AIE. Talanta 206, 120177. doi:10.1016/j.talanta.2019.120177
Dong, J., Jiang, R., Huang, H., Chen, J., Tian, J., Deng, F., et al. (2020). Facile preparation of fluorescent nanodiamond based polymer nanoparticles via ring-opening polymerization and their biological imaging. Mater. Sci. Eng. C 106, 110297. doi:10.1016/j.msec.2019.110297
Dong, J., Jiang, R., Wan, W., Ma, H., Huang, H., Feng, Y., et al. (2020). Two birds one stone: Facile preparation of AIE-active fluorescent polymeric nanoparticles via self-catalyzed photo-mediated polymerization. Appl. Surf. Sci. 508, 144799. doi:10.1016/j.apsusc.2019.144799
Dong, Y., Chen, Z., Hou, M., Qi, L., Yan, C., Lu, X., et al. (2020). Mitochondria-targeted aggregation-induced emission active near infrared fluorescent probe for real-time imaging. Spectrochimica Acta Part A Mol. Biomol. Spectrosc. 224, 117456. doi:10.1016/j.saa.2019.117456
Fei, N., Wei, Q., Cao, L., Bai, Y., Ji, H., Peng, R., et al. (2020). A symmetric nonpolar blue AIEgen as nondoped fluorescent OLED emitter with low efficiency roll-off. Org. Electron. 78, 105574. doi:10.1016/j.orgel.2019.105574
Feng, A., Jiang, F., Huang, G., and Liu, P. (2020). Synthesis of the cationic fluorescent probes for the detection of anionic surfactants by electrostatic self-assembly. Spectrochimica Acta Part A Mol. Biomol. Spectrosc. 224, 117446. doi:10.1016/j.saa.2019.117446
Gao, F., Wu, J., Gao, H., Hu, X., Liu, L., Midgley, A. C., et al. (2020). Hypoxia-tropic nanozymes as oxygen generators for tumor-favoring theranostics. Biomaterials 230, 119635. doi:10.1016/j.biomaterials.2019.119635
Gao, L., Lin, X., and Chen, X. (2020). Ionic liquid decorated AIE luminogen for selective detection of HSA in biofluids and early disease screening. Talanta 212, 120763. doi:10.1016/j.talanta.2020.120763
Gu, X., Kwok, R. T. K., Lam, J. W. Y., and Tang, B. Z. (2017). AIEgens for biological process monitoring and disease theranostics. Biomaterials 146, 115–135. doi:10.1016/j.biomaterials.2017.09.004
Guan, P., Yang, B., and Liu, B. (2020). Fabricating a fluorescence resonance energy transfer system with AIE molecular for sensitive detection of Cu(II) ions. Spectrochimica Acta Part A Mol. Biomol. Spectrosc. 225, 117604. doi:10.1016/j.saa.2019.117604
He, Z., Jiang, R., Long, W., Huang, H., Liu, M., Feng, Y., et al. (2020). Red aggregation-induced emission luminogen and Gd(3+) codoped mesoporous silica nanoparticles as dual-mode probes for fluorescent and magnetic resonance imaging. J. Colloid Interface Sci. 567, 136–144. doi:10.1016/j.jcis.2020.02.009
Hou, J.-R., Jin, D., Chen, B., Si, L.-L., Jin, Y.-H., Chen, L.-G., et al. (2017). Two near-infrared highly sensitive cyanine fluorescent probes for pH monitoring. Chin. Chem. Lett. 28 (8), 1681–1687. doi:10.1016/j.cclet.2017.03.037
Hu, J., Han, T., Liu, Y., Zhang, X., Duan, Y., Li, Z., et al. (2020). Strategy for optical data encryption and decryption using a D-A type stimuli-responsive AIE material. Spectrochimica Acta Part A Mol. Biomol. Spectrosc. 239, 118486. doi:10.1016/j.saa.2020.118486
Hu, P., Wang, D., Zhang, Y., Cai, Z., Ye, T., Tong, L., et al. (2020). Apoptosis-triggered decline in hippocampal microglia mediates adolescent intermittent alcohol exposure-induced depression-like behaviors in mice. Neuropharmacology 170, 108054. doi:10.1016/j.neuropharm.2020.108054
Huang, J., Qin, H., Liang, H., and Lu, J. (2020). An AIE polymer prepared via aldehyde-hydrazine step polymerization and the application in Cu2+ and S2− detection. Polymer 202, 122663. doi:10.1016/j.polymer.2020.122663
Huang, Y., Huang, J., Wang, Y., Ma, F., Ji, J., and Lei, J. (2020). Progressive aggregation-induced emission strategy for imaging of aluminum ions in cellular microenvironment. Talanta 211, 120699. doi:10.1016/j.talanta.2019.120699
Huang, Z.-J., Mou, H.-R., Xie, J.-P., Li, F., Gong, C.-B., Tang, Q., et al. (2020). AIE-active electrochromic materials based on tetraphenylethylene cored benzoates with high optical contrast and coloration efficiency. Sol. Energy Mater. Sol. Cells 206, 110293. doi:10.1016/j.solmat.2019.110293
Jia, J., and Wu, L. (2020). Reversible fluorescent switching properties of pyrene-substituted acylhydrazone derivatives toward mechanical force and acid vapor with aggregation-induced emission. J. Photochem. Photobiol. A Chem. 399, 112640. doi:10.1016/j.jphotochem.2020.112640
Jia, J., and Wu, L. (2020). Stimuli-responsive fluorescence switching: Aggregation-induced emission (AIE), protonation effect and reversible mechanofluochromism of tetraphenylethene hydrazone-based dyes. Org. Electron. 76, 105466. doi:10.1016/j.orgel.2019.105466
Jiang, R., Chen, J., Huang, H., Liu, L., Mao, L., Liu, M., et al. (2020b). Fluorescent copolymers with aggregation-induced emission feature from a novel catalyst-free three-component tandem polymerization. Dyes Pigments 172, 107868. doi:10.1016/j.dyepig.2019.107868
Jiang, R., Zhao, S., Chen, L., Zhao, M., Qi, W., Fu, W., et al. (2020a). Fluorescence detection of protamine, heparin and heparinase II based on a novel AIE molecule with four carboxyl. Int. J. Biol. Macromol. 156, 1153–1159. doi:10.1016/j.ijbiomac.2019.11.150
Jiang, S., Qiu, J., Chen, S., Guo, H., and Yang, F. (2020b). Double-detecting fluorescent sensor for ATP based on Cu(2+) and Zn(2+) response of hydrazono-bis-tetraphenylethylene. Spectrochimica Acta Part A Mol. Biomol. Spectrosc. 227, 117568. doi:10.1016/j.saa.2019.117568
Jiang, S., Qiu, J., Lin, B., Guo, H., and Yang, F. (2020a). First fluorescent sensor for curcumin in aqueous media based on acylhydrazone-bridged bis-tetraphenylethylene. Spectrochimica Acta Part A Mol. Biomol. Spectrosc. 229, 117916. doi:10.1016/j.saa.2019.117916
Ko, Y. J., Kim, W. J., Kim, K., and Kwon, I. C. (2019). Advances in the strategies for designing receptor-targeted molecular imaging probes for cancer research. J. Control. Release 305, 1–17. doi:10.1016/j.jconrel.2019.04.030
Korzec, M., Malarz, K., Mrozek-Wilczkiewicz, A., Rzycka-Korzec, R., Schab-Balcerzak, E., and Polanski, J. (2020). Live cell imaging by 3-imino-(2-phenol)-1, 8-naphthalimides: The effect of ex vivo hydrolysis. Spectrochimica Acta Part A Mol. Biomol. Spectrosc. 238, 118442. doi:10.1016/j.saa.2020.118442
Leng, P., Sun, S., Guo, R., Zhang, Q., Liu, W., Lv, X., et al. (2020). Modifying the AIE-TADF chromophore with host-substituents to achieve high efficiency and low roll-off non-doped OLEDs. Org. Electron. 78, 105602. doi:10.1016/j.orgel.2019.105602
Li, M., Du, F., Xue, P., Tan, X., Liu, S., Zhou, Y., et al. (2020). An AIE fluorescent probe with a naphthalimide derivative and its application for detection of hypochlorite and imaging inside living cells. Spectrochimica Acta Part A Mol. Biomol. Spectrosc. 227, 117760. doi:10.1016/j.saa.2019.117760
Li, X., Lai, Z., Gu, J., Liu, W., Gao, J., and Wang, Q. (2020). Sequential determination of cerium (IV) ion and ascorbic acid via a novel organic framework: A subtle interplay between intramolecular charge transfer (ICT) and aggregated-induced-emission (AIE). J. Mol. Liq. 304, 112705. doi:10.1016/j.molliq.2020.112705
Li, Z., Li, L.-J., Sun, T., Liu, L., and Xie, Z. (2016). Benzimidazole-BODIPY as optical and fluorometric pH sensor. Dyes Pigments 128, 165–169. doi:10.1016/j.dyepig.2016.01.029
Liu, C., Zhang, K., Sun, Q., and Li, W. (2020). Bile acid-terpyridine conjugates: Steroidal skeleton controlled AIE effect and metal-tunable fluorescence and supramolecular assembly properties. Tetrahedron 76 (26), 131283. doi:10.1016/j.tet.2020.131283
Liu, L., Jin, W., Huang, Y., Dai, J., Zheng, X., Liu, Y., et al. (2022). Detecting the insoluble protein aggregates in live cells using an AIE derivative of fluorescent protein chromophore. Sensors Actuators B Chem. 353, 131098. doi:10.1016/j.snb.2021.131098
Liu, S., Li, Y., Kwok, R. T. K., Lam, J. W. Y., and Tang, B. Z. (2020). Structural and process controls of AIEgens for NIR-II theranostics. Chem. Sci. 12 (10), 3427–3436. doi:10.1039/d0sc02911d
Lu, D., He, L., Wang, Y., Xiong, M., Hu, M., Liang, H., et al. (2017). Tetraphenylethene derivative modified DNA oligonucleotide for in situ potassium ion detection and imaging in living cells. Talanta 167, 550–556. doi:10.1016/j.talanta.2017.02.064
Lu, Q., Jiang, G., Li, F., Lin, L., Wang, C. K., Fan, J., et al. (2020). Substitution effect on luminescent property of thermally activated delayed fluorescence molecule with aggregation induced emission: A QM/MM study. Spectrochimica Acta Part A Mol. Biomol. Spectrosc. 229, 117964. doi:10.1016/j.saa.2019.117964
Luo, J., Xie, Z., Lam, J. W., Cheng, L., Chen, H., Qiu, C., et al. (2001). Aggregation-induced emission of 1-methyl-1, 2, 3, 4, 5-pentaphenylsilole. Chem. Commun. 18, 1740–1741. doi:10.1039/b105159h
Ma, H., Zhang, A., Zhang, X., Zhao, H., Cui, Z., Fu, P., et al. (2020). Novel platform for visualization monitoring of hydrolytic degradation of bio-degradable polymers based on aggregation-induced emission (AIE) technique. Sensors Actuators B Chem. 304, 127342. doi:10.1016/j.snb.2019.127342
Ma, J., Xiao, Y., Zhang, C., Zhang, M., Wang, Q., Zheng, W., et al. (2020). Preparation a novel pyrene-based AIE-active ratiometric turn-on fluorescent probe for highly selective and sensitive detection of Hg2+. Mater. Sci. Eng. B 259, 114582. doi:10.1016/j.mseb.2020.114582
Maity, A., Ali, F., Agarwalla, H., Anothumakkool, B., and Das, A. (2015). Tuning of multiple luminescence outputs and white-light emission from a single gelator molecule through an ESIPT coupled AIEE process. Chem. Commun. 51 (11), 2130–2133. doi:10.1039/c4cc09211b
Mao, G. J., Liang, Z. Z., Gao, G. Q., Wang, Y. Y., Guo, X. Y., Su, L., et al. (2019). A photostable Si-rhodamine-based near-infrared fluorescent probe for monitoring lysosomal pH during heat stroke. Anal. Chim. Acta X. 1092, 117–125. doi:10.1016/j.aca.2019.09.053
Mei, J., Hong, Y., Lam, J. W., Qin, A., Tang, Y., and Tang, B. Z. (2014). Aggregation-induced emission: The whole is more brilliant than the parts. Adv. Mat. 26 (31), 5429–5479. doi:10.1002/adma.201401356
Meimetis, L. G., Carlson, J. C., Giedt, R. J., Kohler, R. H., and Weissleder, R. (2014). Ultrafluorogenic coumarin-tetrazine probes for real-time biological imaging. Angew. Chem. Int. Ed. Engl. 53 (29), 7661–7664. doi:10.1002/ange.201403890
Mondal, P., Biswas, S., Jana, D., Das, B., Ghorai, U. K., Ghorai, B. K., et al. (2020). Aggregation induction of tetraphenylethylene AIEgen and its supramolecular aggregates toward light-emitting diodes. J. Solid State Chem. 282, 121122. doi:10.1016/j.jssc.2019.121122
Mondal, S., Kumari, C., Hira, S. K., and Dey, S. (2020). Dual core clickate fluorophores for selective recognition of Cu2+ and Ni2+ along with live cell imaging. Inorganica Chim. Acta 509, 119655. doi:10.1016/j.ica.2020.119655
Munirathnappa, A. K., Maurya, S. K., Kumar, K., Navada, K. K., Kulal, A., and Sundaram, N. G. (2020). Scheelite like NaTb(WO4)2 nanoparticles: Green fluorescence and in vitro cell imaging applications. Mater. Sci. Eng. C 106, 110182. doi:10.1016/j.msec.2019.110182
Nabeel, F., Rasheed, T., Mahmood, M. F., and Khan, S. U.-D. (2020). Hyperbranched copolymer based photoluminescent vesicular probe conjugated with tetraphenylethene: Synthesis, aggregation-induced emission and explosive detection. J. Mol. Liq. 308, 113034. doi:10.1016/j.molliq.2020.113034
Nie, K., Dong, B., Shi, H., Chao, L., Long, M., Xu, H., et al. (2020a). Facile construction of AIE-based FRET nanoprobe for ratiometric imaging of hypochlorite in live cells. J. Luminescence 220, 117018. doi:10.1016/j.jlumin.2019.117018
Nie, K., Yuan, Y., Peng, X., Song, J., and Qu, J. (2020b). A diketopyrrolopyrrole-based hybrid organic nanoprobe for ratiometric imaging of endogenous hypochlorite in live cells. Sensors Actuators B Chem. 307, 127632. doi:10.1016/j.snb.2019.127632
Niu, S., Bi, C., and Song, W. (2020). Detection of DNA methyltransferase activity using template-free DNA polymerization amplification based on aggregation-induced emission. Anal. Biochem. 590, 113532. doi:10.1016/j.ab.2019.113532
Olzscha, H., Schermann, S. M., Woerner, A. C., Pinkert, S., Hecht, M. H., Tartaglia, G. G., et al. (2011). Amyloid-like aggregates sequester numerous metastable proteins with essential cellular functions. Cell. 144 (1), 67–78. doi:10.1016/j.cell.2010.11.050
Pradhan, N., Jana, D., Ghorai, B. K., and Jana, N. R. (2015). Detection and monitoring of amyloid fibrillation using a fluorescence "Switch-On" probe. ACS Appl. Mat. Interfaces 7 (46), 25813–25820. doi:10.1021/acsami.5b07751
Pratihar, S., Bhattacharyya, A., and Prasad, E. (2020). Achieving ACQ-AIE modulation using isostructural organic fluorophores. J. Photochem. Photobiol. A Chem. 396, 112458. doi:10.1016/j.jphotochem.2020.112458
Qi, J., Duan, X., Liu, W., Li, Y., Cai, Y., Lam, J. W. Y., et al. (2020). Dragonfly-shaped near-infrared AIEgen with optimal fluorescence brightness for precise image-guided cancer surgery. Biomaterials 248, 120036. doi:10.1016/j.biomaterials.2020.120036
Qu, F., Yang, Q., Wang, B., and You, J. (2020). Aggregation-induced emission of copper nanoclusters triggered by synergistic effect of dual metal ions and the application in the detection of H2O2 and related biomolecules. Talanta 207, 120289. doi:10.1016/j.talanta.2019.120289
Rajalakshmi, A. V., and Palanisami, N. (2020). Y-shaped ferrocene/non-ferrocene conjugated quinoxalines for colorimetric and fluorimetric detection of picric acid. Spectrochimica Acta Part A Mol. Biomol. Spectrosc. 228, 117812. doi:10.1016/j.saa.2019.117812
Ramasamy, K., and Thambusamy, S. (2017). Dual emission and pH based naphthalimide derivative fluorescent sensor for the detection of Bi3+. Sensors Actuators B Chem. 247, 632–640. doi:10.1016/j.snb.2017.03.043
Sargazi, S., Fatima, I., Hassan Kiani, M., Mohammadzadeh, V., Arshad, R., Bilal, M., et al. (2022). Fluorescent-based nanosensors for selective detection of a wide range of biological macromolecules: A comprehensive review. Int. J. Biol. Macromol. 206, 115–147. doi:10.1016/j.ijbiomac.2022.02.137
Song, Y. K., Lee, T. H., Lee, K. C., Choi, M. H., Kim, J. C., Lee, S.-H., et al. (2020). Coating that self-reports cracking and healing using microcapsules loaded with a single AIE fluorophore. Appl. Surf. Sci. 511, 145556. doi:10.1016/j.apsusc.2020.145556
Su, Y., Lv, C., Zhang, Y., Liu, S., Xie, Z., and Zheng, M. (2020). Fluorescent nanoparticles with ultralow chromophore loading for long-term tumor-targeted imaging. Acta Biomater. 111, 398–405. doi:10.1016/j.actbio.2020.05.013
Suzuki, S., Maeda, S., and Morokuma, K. (2015). Exploration of quenching pathways of multiluminescent acenes using the GRRM method with the SF-TDDFT method. J. Phys. Chem. A 119 (47), 11479–11487. doi:10.1021/acs.jpca.5b07682
Suzuki, S., Sasaki, S., Sairi, A. S., Iwai, R., Tang, B. Z., and Konishi, G. I. (2020). Principles of aggregation-induced emission: Design of deactivation pathways for advanced AIEgens and applications. Angew. Chem. Int. Ed. Engl. 59 (25), 9940–9951. doi:10.1002/ange.202000940
Tang, Y., Kang, A., Yang, X., Hu, L., Tang, Y., Li, S., et al. (2020). A robust OFF-ON fluorescent biosensor for detection and clearance of bacterial endotoxin by specific peptide based aggregation induced emission. Sensors Actuators B Chem. 304, 127300. doi:10.1016/j.snb.2019.127300
Wang, H., Zhao, E., Lam, J. W. Y., and Tang, B. Z. (2015). AIE luminogens: Emission brightened by aggregation. Mater. Today 18 (7), 365–377. doi:10.1016/j.mattod.2015.03.004
Wang, J., Xia, S., Bi, J., Fang, M., Mazi, W., Zhang, Y., et al. (2018). Ratiometric near-infrared fluorescent probes based on through-bond energy transfer and pi-conjugation modulation between tetraphenylethene and hemicyanine moieties for sensitive detection of pH changes in live cells. Bioconjug. Chem. 29 (4), 1406–1418. doi:10.1021/acs.bioconjchem.8b00111
Wang, Q., Li, B., Cao, H., Jiang, X., and Kong, X. Z. (2020). Aliphatic amide salt, a new type of luminogen: Characterization, emission and biological applications. Chem. Eng. J. 388, 124182. doi:10.1016/j.cej.2020.124182
Wang, R., Diao, L., Zhang, J., Chen, Z., and Pu, S. (2020). Aggregation-induced emission compounds based on 9, 10-dithienylanthracene and their applications in cell imaging. Dyes Pigments 175, 108112. doi:10.1016/j.dyepig.2019.108112
Wang, W. W., Wang, Y., Wu, W. N., Zhao, X. L., Xu, Z. Q., Xu, Z. H., et al. (2020). Pyrrole-quinazoline derivative as an easily accessible turn-off optical chemosensor for Cu2+ and resultant Cu2+ complex as a turn-on sensor for pyrophosphate in almost neat aqueous solution. Spectrochimica Acta Part A Mol. Biomol. Spectrosc. 226, 117592. doi:10.1016/j.saa.2019.117592
Wang, X., Ding, G., Duan, Y., Zhu, Y., Zhu, G., Wang, M., et al. (2020). A novel triphenylamine-based bis-Schiff bases fluorophores with AIE-Activity as the hydrazine fluorescence turn-off probes and cell imaging in live cells. Talanta 217, 121029. doi:10.1016/j.talanta.2020.121029
Wang, X., Huo, X., Yang, R., Li, Z., Sun, Y., Qu, L., et al. (2020). A novel fluorescence probe based on specific recognition of GABAA receptor for imaging cell membrane. Talanta 219, 121317. doi:10.1016/j.talanta.2020.121317
Wang, X., Zhang, L., Li, Q., and Gao, Y. (2020). A novel dark resonance energy transfer-based fluorescent probe with large Stokes shift for the detection of pH and its imaging application. Dyes Pigments 181, 108614. doi:10.1016/j.dyepig.2020.108614
Wang, Y., Qiu, Y., Sun, A., Xiong, Y., Tan, H., Shi, Y., et al. (2020). Dual-functional AIE fluorescent probes for imaging beta-amyloid plaques and lipid droplets. Anal. Chim. Acta X. 1133, 109–118. doi:10.1016/j.aca.2020.07.073
Wang, Y., Zhang, Y., Wang, J., and Liang, X. J. (2019). Aggregation-induced emission (AIE) fluorophores as imaging tools to trace the biological fate of nano-based drug delivery systems. Adv. Drug Deliv. Rev. 143, 161–176. doi:10.1016/j.addr.2018.12.004
Wang, Z., Zhang, Y., Yin, J., Li, M., Luo, H., Yang, Y., et al. (2020). An easily available camphor-derived ratiometric fluorescent probe with AIE feature for sequential Ga3+ and ATP sensing in a near-perfect aqueous media and its bio-imaging in living cells and mice. Sensors Actuators B Chem. 320, 128249. doi:10.1016/j.snb.2020.128249
Wei, M., Zhong, H., Zhou, J., Liu, W., Xi, W., Xu, P., et al. (2020). A phosphorescence “turn-on” probe for the detection and imaging of Al3+ based on aggregation-induced emission. Talanta 219, 121298. doi:10.1016/j.talanta.2020.121298
Wu, X., Wu, P., Gu, M., and Xue, J. (2020). Ratiometric fluorescent probe based on AuNCs induced AIE for quantification and visual sensing of glucose. Anal. Chim. Acta X. 1104, 140–146. doi:10.1016/j.aca.2020.01.004
Xu, C., Guan, R., Cao, D., Liu, K., Chen, Q., Ding, Y., et al. (2020). Bioinspired non-aromatic compounds emitters displaying aggregation independent emission and recoverable photo-bleaching. Talanta 206, 120232. doi:10.1016/j.talanta.2019.120232
Xu, L., Sun, L., Zeng, F., and Wu, S. (2020). Activatable fluorescent probe based on aggregation-induced emission for detecting hypoxia-related pathological conditions. Anal. Chim. Acta 1125, 152–161. doi:10.1016/j.aca.2020.05.046
Xu, X., Li, J., Li, Q., Huang, J., Dong, Y., Hong, Y., et al. (2012). A strategy for dramatically enhancing the selectivity of molecules showing aggregation-induced emission towards biomacromolecules with the aid of graphene oxide. Chem. Eur. J. 18 (23), 7278–7286. doi:10.1002/chem.201103638
Xu, Z., Liu, Y., Qian, C., Wu, L., Wu, Z., Zhang, J., et al. (2020). Tuning the morphology of melamine-induced tetraphenylethene self-assemblies for melamine detecting. Org. Electron. 76, 105476. doi:10.1016/j.orgel.2019.105476
Xu, Z., Liu, Y., Wang, R., Zhang, J., Cheng, J., Chen, S., et al. (2020). AIEE based “turn-on” fluorescent sensor for Al3+ ions and induced tetraphenylethene self-assemblies. Org. Electron. 85, 105820. doi:10.1016/j.orgel.2020.105820
Xue, J., Yang, L., Du, Y., Ren, Y., Ren, X., Ma, H., et al. (2020). Electrochemiluminescence sensing platform based on functionalized poly-(styrene-co-maleicanhydride) nanocrystals and iron doped hydroxyapatite for CYFRA 21-1 immunoassay. Sensors Actuators B Chem. 321, 128454. doi:10.1016/j.snb.2020.128454
Yan, C., Qin, W., Li, Z., Zhou, Y., Cui, Y., and Liang, G. (2020). Quantitative and rapid detection of explosives using an efficient luminogen with aggregation-induced emission characteristics. Sensors Actuators B Chem. 302, 127201. doi:10.1016/j.snb.2019.127201
Yan, L., Xie, Y., Li, J., and Zhu, W. (2020). A red fluorogen: AIEE characteristic, photoluminescence mechanism and its application as chemosensor for ClO. Spectrochimica Acta Part A Mol. Biomol. Spectrosc. 228, 117794. doi:10.1016/j.saa.2019.117794
Yang, Q. Y., Zhang, Y. M., Ma, X. Q., Dong, H. Q., Zhang, Y. F., Guan, W. L., et al. (2020). A pillar[5]arene-based fluorescent sensor for sensitive detection of L-Met through a dual-site collaborative mechanism. Spectrochimica Acta Part A Mol. Biomol. Spectrosc. 240, 118569. doi:10.1016/j.saa.2020.118569
Yang, W., Yang, Y., Zhan, L., Zheng, K., Chen, Z., Zeng, X., et al. (2020). Polymorphism-dependent thermally activated delayed fluorescence materials with diverse three dimensional supramolecular frameworks. Chem. Eng. J. 390, 124626. doi:10.1016/j.cej.2020.124626
Yao, Z. J., Su, G., and Jin, G. X. (2011). Versatile reactivity of half-sandwich Ir and Rh complexes toward carboranylamidinates and their derivatives: Synthesis, structure, and catalytic activity for norbornene polymerization. Chem. Eur. J. 17 (47), 13298–13307. doi:10.1002/chem.201101638
Younis, O., Orabi, E. A., Kamal, A. M., Sayed, M., Hassanien, R., Davis, R. L., et al. (2020). Aggregation-induced emission with white, green, or blue luminescence from biologically-active indole derivatives. Opt. Mater. 100, 109713. doi:10.1016/j.optmat.2020.109713
Yu, Q., Chen, S., Han, C., Guo, H., and Yang, F. (2020). High solid fluorescence of novel tetraphenylethene-porphyrin. J. Luminescence 220, 117017. doi:10.1016/j.jlumin.2019.117017
Zeng, X., Liang, J., Wang, C., Yu, Z., Zhao, X., Lu, H., et al. (2020). Gelation process visualized by synchronous fluorescence enhancement of polyhydroxy benzoylhydrazone-based organogel. J. Luminescence 224, 117259. doi:10.1016/j.jlumin.2020.117259
Zhai, B., Hu, Z., Peng, C., Liu, B., Li, W., and Gao, C. (2020). Rational design of a colorimetric and fluorescence turn-on chemosensor with benzothiazolium moiety for cyanide detection in aqueous solution. Spectrochimica Acta Part A Mol. Biomol. Spectrosc. 224, 117409. doi:10.1016/j.saa.2019.117409
Zhan, Y., Yang, Z., Tan, J., Qiu, Z., Mao, Y., He, J., et al. (2020). Synthesis, aggregation-induced emission (AIE) and electroluminescence of carbazole-benzoyl substituted tetraphenylethylene derivatives. Dyes Pigments 173, 107898. doi:10.1016/j.dyepig.2019.107898
Zhang, H., Tao, F., Xu, Z., Cui, Y., Yu, W. W., and Zhang, D. (2020). Data encryption-decryption based on crystal-induced emission enhancement (CIEE) properties of barbituric acid derivatives. Dyes Pigments 180, 108408. doi:10.1016/j.dyepig.2020.108408
Zhang, L., Gao, J., Qi, A., and Gao, Y. (2020). A novel DRET and FRET combined fluorescent molecule and its applications in sensing and bioimaging. Sensors Actuators B Chem. 320, 128457. doi:10.1016/j.snb.2020.128457
Zhang, L., Zhou, S., Min, S., Yu, X., Hou, G., Tong, Q., et al. (2020). Water-soluble near-infrared fluorescent probes enhanced by ionic co-assembly of a four-armed amphiphile with SDBS: Toward application in cell imaging. Dyes Pigments 181, 108541. doi:10.1016/j.dyepig.2020.108541
Zhang, M., Wang, Y., Sun, X., Bai, J., Peng, Y., Ning, B., et al. (2020). Ultrasensitive competitive detection of patulin toxin by using strand displacement amplification and DNA G-quadruplex with aggregation-induced emission. Anal. Chim. Acta X. 1106, 161–167. doi:10.1016/j.aca.2020.01.064
Zhang, R., Gao, H., Yu, J., Liu, C., Tao, Y., and Cheng, X. (2020). AIE active TPE mesogens with p6mm columnar and Im3m cubic mesophases and white light emission property. J. Mol. Liq. 298, 112079. doi:10.1016/j.molliq.2019.112079
Zhang, S., Huang, Y., Kong, L., Zhang, X., and Yang, J. (2020). Aggregation-induced emission-active tetraphenylethylene derivatives containing arylimidazole unit for reversible mechanofluorochromism and selective detection of picric acid. Dyes Pigments 181, 108574. doi:10.1016/j.dyepig.2020.108574
Zhang, X., Zhen, S., Zhang, L., Chai, J., Zou, L., Xin, X., et al. (2020). The electrosynthesis of highly photofunctional porous polymer PTCPE and the effect of BFEE on its electrochemical polymerization and fluorescence property. Polymer 202 (5), 122731. doi:10.1016/j.polymer.2020.122731
Zhao, L., Liu, Y., Xie, S., Ran, P., Wei, J., Liu, Q., et al. (2020). Janus micromotors for motion-capture-ratiometric fluorescence detection of circulating tumor cells. Chem. Eng. J. 382, 123041. doi:10.1016/j.cej.2019.123041
Zhao, L., Zhang, Z., Liu, Y., Wei, J., Liu, Q., Ran, P., et al. (2020). Fibrous strips decorated with cleavable aggregation-induced emission probes for visual detection of Hg2+. J. Hazard. Mat. 385, 121556. doi:10.1016/j.jhazmat.2019.121556
Zhao, R., Zhang, M., Liu, Y., Zhang, X., Duan, Y., and Han, T. (2020). Fabricating D-A type AIE luminogen into film sensor for turn-on detection of methanol vapour. Sensors Actuators B Chem. 319, 128323. doi:10.1016/j.snb.2020.128323
Zhao, W., Ma, Y., Ye, J., and Jin, J. (2020). A closed bipolar electrochemiluminescence sensing platform based on quantum dots: A practical solution for biochemical analysis and detection. Sensors Actuators B Chem. 311, 127930. doi:10.1016/j.snb.2020.127930
Zheng, S., Liu, T., Song, Z., He, Z., Yang, Z., Wang, H., et al. (2020). Acetenyl bridged diphenyl sulfones: A gate way to AIE/MCL/TADF multifunctional white-light emission molecule. Dyes Pigments 176, 108204. doi:10.1016/j.dyepig.2020.108204
Zhou, G., Zhang, X., and Ni, X. L. (2020). Tuning the amphiphilicity of terpyridine-based fluorescent probe in water: Assembly and disassembly-controlled H g(2+) sensing, removal, and adsorption of H2S. J. Hazard. Mat. 384, 121474. doi:10.1016/j.jhazmat.2019.121474
Keywords: AIE (aggregation-induced emission), fluorescence, biotesting, bioimaging, material
Citation: Ma J, Gu Y, Ma D, Lu W and Qiu J (2022) Insights into AIE materials: A focus on biomedical applications of fluorescence. Front. Chem. 10:985578. doi: 10.3389/fchem.2022.985578
Received: 04 July 2022; Accepted: 05 August 2022;
Published: 15 September 2022.
Edited by:
Zheng Han, Shanghai Academy of Agricultural Sciences, ChinaReviewed by:
Yi Qu, Shanghai University of Engineering Sciences, ChinaLin Yuan, Hunan University, China
Copyright © 2022 Ma, Gu, Ma, Lu and Qiu. This is an open-access article distributed under the terms of the Creative Commons Attribution License (CC BY). The use, distribution or reproduction in other forums is permitted, provided the original author(s) and the copyright owner(s) are credited and that the original publication in this journal is cited, in accordance with accepted academic practice. No use, distribution or reproduction is permitted which does not comply with these terms.
*Correspondence: Jianfeng Qiu, jfqiu100@gmail.com