- 1Faculty of Chemical Engineering & Technology, Universiti Malaysia Perlis, Arau, Perlis, Malaysia
- 2Micro System Technology, Centre of Excellence (CoE), Universiti Malaysia Perlis (UniMAP), Arau, Perlis, Malaysia
- 3Institute of Nano Electronic Engineering, Universiti Malaysia Perlis, Kangar, Perlis, Malaysia
- 4Centre for Chemical Biology (CCB), Universiti Sains Malaysia, Bayan Lepas, Penang, Malaysia
- 5School of Biological Sciences, Universiti Sains Malaysia, Georgetown, Penang, Malaysia
- 6Department of Oral & Craniofacial Sciences, Faculty of Dentistry, University of Malaya, Kuala Lumpur, Malaysia
- 7Research & Development, Sree Balaji Medical College and Hospital (SBMCH)- BIHER, Chennai, Tamil Nadu, India
- 8Department of Biochemistry, Faculty of Medicine, Bioscience and Nursing, MAHSA University, Jenjarom, Selangor, Malaysia
- 9Department of Periodontics, Saveetha Dental College and Hospitals, Saveetha Institute of Medical and Technical Sciences, Chennai, India
- 10Department of Foundation, RCSI & UCD Malaysia Campus, Georgetown, Pulau Pinang, Malaysia
- 11Department of Microbiology, Yogi Vemana University, Kadapa, Andhra Pradesh, India
Nanoscale iron oxide-based nanostructures are among the most apparent metallic nanostructures, having great potential and attracting substantial interest due to their unique superparamagnetic properties. The green production of nanostructures has received abundant attention and been actively explored recently because of their various beneficial applications and properties across different fields. The biosynthesis of the nanostructure using green technology by the manipulation of a wide variety of plant materials has been the focus because it is biocompatible, non-toxic, and does not include any harmful substances. Biological methods using agro-wastes under green synthesis have been found to be simple, environmentally friendly, and cost-effective in generating iron oxide-based nanostructures instead of physical and chemical methods. Polysaccharides and biomolecules in agro-wastes could be utilized as stabilizers and reducing agents for the green production of nanostructured iron oxide towards a wide range of benefits. This review discusses the green production of iron oxide-based nanostructures through a simple and eco-friendly method and its potential applications in medical and sustainable agro-environments. This overview provides different ways to expand the usage of iron oxide nanomaterials in different sectors. Further, provided the options to select an appropriate plant towards the specific applications in agriculture and other sectors with the recommended future directions.
1 Introduction
The development of nanoscience and nanotechnology is evolving as a promptly developing field, using various applications in science, research, and technology for the purpose of manufacturing new nanoscale levels of macromolecular matter (Cortez-Jugo et al., 2021; Qindeel et al., 2021). Nanotechnology is quickly developing nanomaterials that can be applied in various commercial products, including bio-sensing paints, sunscreens, cosmetics, clothing, the food industry, and medical devices (Parhi et al., 2020; Epps and Abolhasani, 2021; Huguet-Casquero et al., 2021). A nanostructure can be defined as a system of intermediate size that is between a molecular structure and a microscopic image, at typical dimensions between 1 and 100 nm. A nanostructured material consists of crystallites of nanoscale size with different crystallographic orientations. This material consists of atoms or molecules that become the essential building blocks of materials arranged in nano-sized groups. The composition and building blocks in three-dimensional arrangements can affect the properties of the materials. All natural structures are built up from molecular precursors and substructures of nano-size (Gleiter, 2000).
Recently, nanotechnology has recognized great potential, and nanomaterials are extensively used in a wide variety of modern life—including in the modern agricultural sector—to transform various agricultural practices (Usman et al., 2020). Nanomaterials used in agricultural development are newer ideas that have been proven to recover soil quality, improve the degradation of pesticide residues, and encourage seed germination (Rui et al., 2016). Therefore, the consumption of cheap and abundant agricultural waste has become more pressing than ever. However, the production of such waste is expected to increase rapidly due to the tremendous growth of activities in the modern agricultural sector, which can cause adverse effects to the environment. Therefore, precision farming is the best alternative; it allows precise control at the nanometer scale and reduces production costs through the advancement of nanotechnology. Different types of nanoparticles/nanomaterials have been produced by employing varied approaches and these nanomaterials are with a single type or as hybrid (Ghosh et al., 2020; Chandraker et al., 2022; Kumar et al., 2022; Tamang et al., 2022). Among the generated nanomaterials, silver nanoparticle become one of the prominent metals due to its easier and simple generation from the precursor (Jalab et al., 2021). Silver nanoparticle has different potentials compared to iron oxide nanoparticles (IONP) and other nanoparticles. Similar to silver nanoparticle, IONP is also cheaper and easier to prepare, however, IONPs have totally different property such as, magnetism, which makes them with unique applications. With the magnetic properties, IONPs have been considered in several applications compared to gold, copper, silica, titanium, zinc and so on. With the same properties IONP made the special applications with other metals as a hybrid.
The green production of nanostructures using agro-wastes could minimize the usage of hazardous chemical reagents and reduce the cost of synthesis (Ahmed and Mustafa, 2020; Alkhalaf et al., 2020; Jain et al., 2021). Green methods have been followed widely due to their appealing advantages primarily as cheaper, easier to follow and suitable for larger scale preparation (Behravan et al., 2019; Vanlalveni et al., 2021; Chandraker et al., 2022). Plant and the associated materials are widely used for the production of metallic nanomaterials in different shapes. Because medicinal phytoextract contains alkaloids, phenolics, terpenoids, proteins, lipids, carbohydrates, and other nitrogen-containing chemicals, it provides a route that can be an efficient reductant to reduce precursor into the nanomaterial. The reduced nanomaterial produced by employing medicinal phytoextract as reductant compounds can then be used in biomedical applications because phytoextract is pure and non-toxic by nature. Naturally occurring polyphenols and biomolecules found in plant material wastes act as driving forces and play an active role in the formation of nanostructures with distinct sizes and shapes that are safe, greener, and environmentally friendly (Figure 1) (Herlekar et al., 2014; Lingamdinne et al., 2019; Sahu et al., 2021). Agro-waste is commonly known as agricultural wastes and is defined as the residues in the form of solids, liquids, or slurries that are produced from a wide variety of agriculture activities. These wastes are usually produced from the processing of raw agricultural products through farming activities, including vegetables, fruits, daily products, crops, and meats (Figure 2). Two types of constituents that can be found in agro-wastes are soluble constituents, such as organic acids, and insoluble constituents, such as lignin and cellulose. Furthermore, the major quantity of agro-wastes are comprised of food processing wastes, crop wastes, vegetable wastes, and animal wastes. The composition of the waste depends on the type of agricultural activity and the systems it uses. Moreover, agro-wastes provide an advantage, as the decaying part of plants can be used by farmers to advance the fertility of soil. Agro-wastes are the non-product outputs from the processing of agricultural products that are usually discarded to the environment. Disposal of these wastes generated from agricultural production activities may cause pollution to the environment. Furthermore, some research has proven that agricultural waste can be economically transformed into different nanostructure materials, such as nanowires, nanosensors, nanotubes, and nanofibers, which have gained much attention and are usable in many potential applications due to their unique optical properties, small size, and great surface-to-volume ratio (Mukhopadhyay, 2014).
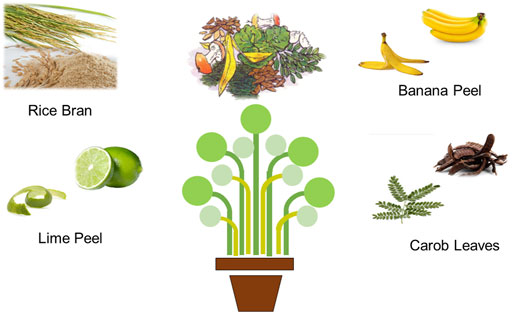
FIGURE 2. Visual observations of different agro-wastes, which can be used for the production of IONP.
Moreover, the quality of modern agricultural practices could be enhanced by nanotechnology to generate safer and better agricultural products. There are significant applications in the management of water quality from nanotechnology, such as the removal of heavy metals from pollution to ensure good water quality [5, 6]. Hence, reuse of agro-wastes has a high chance of developing the green production of iron oxide-based nanostructures in different forms, which have unique properties and composition due to their abundance, low cost, and ease of attainability.
2 Forms of iron oxide
2.1 Magnetite
Magnetite with the chemical formula Fe3O4 is a mineral iron ore, which is also known as one of the members of the oxide of iron. It is the most common magnetic mineral found in metamorphic, sedimentary, and igneous rocks with black or brownish- black color (Jamlos and MF, 2017). Magnetite is a particle that shows a difference from other iron oxides due to its structure, which has ferromagnetic properties. These properties can offer powerful magnetization of IONP (Smuleac et al., 2011). It also has a face-centered cubic structure on 30 O2− ions by the arrangement of cubic close-packed in a regular pattern. Furthermore, magnetite revealed four different crystalline polymorphs. It also presents both bivalent and trivalent iron ions at room temperature. Magnetite has a crystal structure that belongs to the inverse spinel formed by stacking plans as a polyhedral model (Wu et al., 2015).
2.2 Maghemite
Maghemite, γ-Fe2O3 nanoparticle is a ferromagnetic mineral that has an identical lattice arrangement with magnetite, whereas the iron atoms are in an oxidation state of iron (III). It can be transformed to hematite, which is antiferromagnetic at higher temperatures. Maghemite has a crystal structure of spinel that is like magnetite and has the same electron diffraction configurations (Shokrollahi, 2017). The difference between both oxides is their vacancies in cation sublattice. It can give a high magnetic response and is easily magnetized while reacting to an external magnetic field. The lattice vacancies of maghemite are able to give a stronger magnetization than the other iron (III) oxides, such as hematite. Moreover, the outstanding magnetic properties and less cause of hazard to health have proved that maghemite has received great attention for its biomedical applications. Thus, maghemite has a favorable selection for the production of magnetic nanoparticles for biomedical applications because its structure didn’t cause any significant side effects to humans (Winsett et al., 2019).
2.3 Hematite
Hematite (α-Fe2O3) is an oxide that exists in nature as mineral; it is one of the most common polymorphs and among the most well-known of iron oxides that occur widely in soils and rocks. Hematite has weak ferromagnetic behavior at room temperature; meanwhile, it is paramagnetic when at above temperature of 956 K. It is extremely stable under environmental conditions, so it is easier to synthesize than other oxides. Its crystal structure shows both rhombohedral and corundum types. Furthermore, hematite has received great attention in numerous applications, such as magnetic storage media, gas sensors, and environmental treatments, as it is biodegradable, nontoxic, has low corrosion, and does not require high processing expenses (Wu et al., 2015).
3 Greener syntheses of nanomaterials
The reduction between the precursor ion and natural reducing agents—such as plants and microorganisms that consist of a wide variety of species of bacteria, algae, yeast, and fungi—is known as a green synthesis of the iron oxide-based nanostructure (Mahdavi et al., 2013; Saranya et al., 2017; Ikram et al., 2021). There is no use of chemical reagents or organic solvents in green production. This biological production of iron oxide-based nanostructures is proposed as an eco-friendly and cost-effective method, as the reducing agents used are bio-based (Wang et al., 2014). Examples of bio-based agents include plants and their extracts, carbohydrates, peptides, and proteins. A green chemistry approach that connects nanotechnology with plants is known as the plant-mediated production of metal-based nanostructures. Plants and their extracts seem to be the best options for biological reducing and capping agents that are suitable for the green production of nanostructured iron oxide (Parveen et al., 2016). Xing et al. (2014) demonstrated that there are probable mechanisms for the green production of iron oxide-based nanostructures: enzymatic and non-enzymatic reductions. Enzymatic reduction requires biological reducing agents, which are peptides, proteins, and carbohydrates. On the other hand, plants and microorganisms also undergo non-enzymatic reduction to synthesize iron oxide-based nanostructures. Enzymatic reduction is a slower process than non-enzymatic reduction. Non-enzymatic reduction is often a fast process that can be completed within a few minutes and has the ability to handle extreme parameters, such as high temperatures and pH (Ramanathan and Gopinath, 2017).
In the chemical method, surfactants, protective agents, and reducing agents are used to synthesize high-stability NPs and avoid particle agglomeration. There are disadvantages in the chemical methods for the synthesis of iron oxide-based nanostructures, including that the contamination of nanoparticles will occur when different chemicals are used during nanoparticle synthesis. Therefore, two ways of chemical reduction of iron oxide-based nanostructure discovered which uses a strong reducing agent and the weak one as a reducing agent in order to fix the complications.
On the other hand, green synthesis has numerous advantages, including that it is a biocompatible, cost-effective, and environmentally friendly method that eliminates the usage of toxic reagents [15, 16, 17]. It can also be known as a simple method that is able to generate highly stable and well-characterized iron oxide-based nanostructures. The size, structure, and morphologies of the iron oxide-based nanostructure are controlled by altering situations such as the temperature, pH, exposure time, mixing speed, and concentration of the substrate. Hence, green synthesis is an efficient method due to its ability to synthesize iron oxide-based nanostructures by using biological reducing and stabilizing agents.
3.1 Green production of nanostructured iron oxide using agro-wastes
The biological production of metal-based nanostructures is known as a green technology from the manipulation of various agro-wastes because it is biocompatible, non-toxic, and does not include any harmful substances (Lakshmnarayanan et al., 2021; Periakaruppan et al., 2021). The green production of nanostructured iron oxide uses agro-wastes without including any toxic chemical reagents, so this method can be said to be more appealing than physical and chemical methods (Prodan et al., 2013; Ansari et al., 2016). This type of synthesis has received great attention and has been actively explored recently. Organic compounds such as flavonoids, polyphenols, and vitamins are significant compounds that can be found in agro-wastes. The reduction of metal precursors can occur from the wide variety of functional groups present in the compounds. These compounds enable agro-wastes to act as reducing or stabilizing agents. Previous studies have shown that the application of food waste has the potential to be utilized for the green production of different NPs (Sharma et al., 2015). The compositions of agro-wastes not only can affect particle distribution but also can affect the size and shape of particles (Mohamad et al., 2013). A previous study reported that mango peel extract can be used for the production of gold NPs as a natural reducing agent. The synthesized gold NPs produced were monodispersed and proved that there was no involvement of biological toxicity in the normal kidney cells of African green monkeys and normal human fetal lung fibroblast cells (Yang et al., 2014). In addition, tangerine peel extract can be used in order to synthesize iron oxide-based nanostructures for the removal of cadmium in contaminated water. The report showed that different concentrations of tangerine peel influenced the size of the particles. The average size of the iron oxide-based nanostructure decreased as the concentration of the tangerine peel extract increased (Ehrampoush et al., 2015). Moreover, the fruit seed waste extract of Syzygium cumini as a reducing agent could reduce iron ions to crystalline iron oxide NPs. S. cumini seed has a lot of biomolecules and polyphenol compounds for the synthesis of iron oxide NPs. The synthesized nanostructured iron oxides are spherically shaped (Venkateswarlu et al., 2014). The seed extract of Hordeum vulgare has the potential to synthesize crystalline nanostructured iron oxide. The biomolecules present in this plant are citric or oxalic acids, which act as reducing gent to facilitate the conversion of iron ions to nanoparticles in aqueous solutions (Makarov et al., 2014). Citrus limetta (Mosambi peel) and Curcuma longa L. (Turmeric leaves) from agro-wastes in India were found to successfully synthesize nanostructured iron oxide. High polyphenol content was determined from both plant materials, which can be reduced by the metal ions to form complex. These agro-wastes can potentially be used for the biological synthesis of nanostructured iron oxide, which provides great potential for municipal wastewater treatment (Herlekar et al., 2015). A previous study reported that carob leaf extract could be used for the synthesis of nanostructured iron oxide as a natural reducing agent. The synthesized IONPs produced had an average size of 4–8 nm (Awwad et al., 2013). Plant extract of Clitoria ternatea (white Clitoria flower) is used to synthesize IONP with the presence of phenolic compounds as a natural reducing agent (Baby et al., 2017). The formation of bonding between phytochemicals in plant extracts and nanostructured iron oxide can produce stable metallic nanoparticles. Furthermore, Mimosa pudica root extract contains mimosine, which can act as a reducing agent of iron ions to produce highly stable and crystalline nanostructured iron oxide (Niraimathee et al., 2016a). Furthermore, in another study, Eucalyptus globulus leaf extract showed great potential for synthesizing high-density nanostructured iron oxide, since it has significant promise for antimicrobial activities against food-spoiling microorganisms and higher medicinal properties in these plants (Balamurugan et al., 2014). Moreover, a spherical shape and uniformly distributed nanostructured iron oxide with an average size of 33 nm can be produced using the leaf extract of Caricaya papaya (Latha and Gowri, 2014). The leaf extract of Cynometra ramiflora can be used as a reducing agent to reduce iron ions to crystalline nanostructured iron oxide. The nanoparticles have highly effective antimicrobial activity against S. epidermidis and E. coli (Groiss et al., 2017). Table 1 displays the list of agro-waste extracts used for the production of nanostructured iron oxide.
4 Iron oxide nanoparticles
Nanoparticles are particles with nanoscale measurement in the range of 1–100 nm that have received great attention because of their unique small size and great surface-to-volume ratio (Bao et al., 2009; Lee et al., 2018). Nanoparticle (NP) synthesis has great potential for procedures to manage both organic and inorganic toxic pollutants. Nanoscale IONPs are among the most apparent metallic nanoparticles; they have great potential and have attracted substantial interest due to their various specific magnetic behaviors and properties, such as high magnetic perceptivity and unique superparamagnetic traits. This type of nanoparticle is also less sensitive to oxidation and possesses strong ferrimagnetic behavior (Kandpal et al., 2014). Iron oxide is a common chemical compound that occurs abundantly in nature. Iron oxides are magnetic nanomaterials composed of iron and oxygen. Different magnetic properties, structures, and crystal structures are present in iron oxide. Furthermore, there are 16 iron oxides, hydroxides, and oxide-hydroxides. Iron oxide-based nanostructures involve three different phases: magnetite, maghemite, and hematite (Campos et al., 2015).
Recently, iron oxide-based nanostructures have been actively explored due to their various applications in wastewater treatment, cell separation, as contrast agents for magnetic resonance imaging (MRI), for drug delivery to a desired targeted cell, and for hyperthermia and cancer therapies (Irshad et al., 2017; Soetaert et al., 2020; Nguyen et al., 2021). The explorations are expected to further reform the practice of medicine and may have significant applications in all aspects of disease, diagnosis, prevention, and treatment. Speranza et al. (2010) revealed that the advantages are that iron oxide-based nanostructures can be injected into the human body without any significant side effects on health. On the other hand, nanomaterials have been used to produce NPs due to their many applications, which have been revealed by their physical, chemical, or biological characteristics (Zhao et al., 2014; Gaillet and Rouanet, 2015; Chung et al., 2016). Recently, iron oxide-based nanostructures have proven appealing for the elimination of heavy metal pollution because of their magnetic property, small size, and large surface area (Rasheed and Meera, 2016; BhartiJangwan et al., 2022). It has been proven that the synthesis of iron oxide-based nanostructures with high potential applications is in great demand (Ramimoghadam et al., 2014). NPs of magnetic iron oxides, including magnetite and maghemite, are known for their non-toxicity and biocompatibility due to the presence of iron ions that can be used in medicine (Ali et al., 2016). This type of nanoparticle is potentially of high concern to researchers in bio-applications, data storage, and catalysis. Furthermore, the most common biomedical application is magnetic resonance imaging, which enables researchers to improve bio-conjugated magnetic iron oxides concerning brain tumors with simultaneous monitoring (Ali et al., 2016). However, controlling size, shape, stability, and dispersibility could be a challenge for this application (Cantillon-Murphy et al., 2010). An appropriate surface coating, which is emerging with several adequate protection strategies, is significant for controlling the strength of IONPs (Wu et al., 2008). Iron oxide-based nanostructures have a huge surface-to-volume ratio and require high surface energies. Therefore, the surface energies that are able to reduce from the aggregation occur (Wu et al., 2015). IONPs have some disadvantages with naked iron oxide-based nanostructures; therefore, iron oxide-based nanostructures should be stabilized to prevent agglomeration, which keeps the particles from being oxidized and reduces the surface area. Thus, it is important to synthesize iron oxide-based nanostructures with nontoxic biocompatible protective layers to stabilize the magnetism and morphology of IONPs (Tran et al., 2011).
Several methods involved in the synthesis of iron oxide-based nanostructures have been considerably demonstrated (Batool et al., 2021). Physical methods require a very large quantity of energy for evaporation and condensation in a large tube furnace. Parameters in the tube furnace require a longer timeframe to complete the iron oxide NP synthesis (Ramanathan and Gopinath, 2017). In the chemical method, the strong reducing agents in the chemical synthesis of iron oxide-based nanostructures will generate larger sized NPs—though there still remains the challenge of managing the size variations (Rasheed and Meera, 2016). To fix these complications, two ways of chemically reducing iron oxide-based nanostructures have been developed, the first of which uses a strong reducing agent and the other of which uses a weak reducing agent. By using the reduction method, a small size and shape of stable iron oxide-based nanostructures are generated. Among the available methods, green synthesis has been found to be a simple, environmentally friendly, and cost-effective way to generate a stable IONP (Ahmed et al., 2016).
5 Potential applications of nanostructured iron oxide
The production of IONPs has elevated their importance in several fields, including agriculture, wastewater treatment, catalysts, drug treatment, and imaging (Schneider et al., 2022) (Figure 3).
5.1 In the agriculture sector
Agriculture has proven to have potential for various promising nanotechnology applications (Chhipa and Joshi, 2016). The use of nanotechnology in agriculture has increased due to the realization that the productivity of farms would not increase by using conventional techniques. Degradation of soil quality and increased use of fertilizer would lead to necessity of greater energy inputs to maintain the yield by using traditional agricultural practices. Hence, important attention is being paid to the use of nanotechnology in the agricultural sector, as it has the potential to transform food production and conventional agriculture practices. The required amount of farm inputs, such as fertilizers, could be determined by the development of nanomaterial mediated nanosensors that indicate the water or nutrient status of the farm’s products (Ditta et al., 2015). The application of nanobiosensors and other smart delivery systems could be used to attack crop microorganisms in the agricultural sector and offer a capable means of allocating fertilizers and pesticides. The application of nanotechnology in different fields, such as nanofarming and nanofood packaging, has emphasized the ecological balance of its properties (Mukhopadhyay, 2014). It is necessary to determine the optimum levels of IONP to be used with these applications before they are applied.
5.1.1 As a nano-fertilizer
The quality and food production could be enhanced by using fertilizers after the fertilizer responsive varieties and introduction of high yielding. Excess use of chemical fertilizers to increase crop production is not an appropriate option, as they can decrease soil fertility and disturb the soil mineral balance. It can cause air pollution, as residual minerals may leach down into the soil. Excessive use of chemical fertilizers in mineral cycles, soil structures, and food chains across the ecosystem will also lead to inherited changes in future generations of consumers. Therefore, the development of nanofertilizers would be a great option and a new idea for reducing the consumption of excessive fertilizers for crop fields. The application of nanofertilizers also plays a significant role in agriculture because they are able to provide better yields, efficiency in controlling nutrition deficiency, and the environmental boon to nature of reducing soil pollution (Rameshaiah et al., 2015). Furthermore, the yield and growth could be enhanced by nanofertilizers that can provide one or more nutrients to the plants, which eases the better performance of conventional fertilizers that would not supply crops with nutrients directly. Furthermore, the ability for high reactivity and greater absorbance, which is able to improve the growth of crops, has become one of the potential contributions of nanofertilizers. Research has proven the efficiency of nanostructured iron oxide as a fertilizer, which is better than conventional fertilizer, in attaining sustainable growth in agricultural applications with negligible environmental impact. Nanocompounds are able to be absorbed completely and rapidly by plants, as well as to fix nutrient shortages and growing needs with the potential contribution of nanofertilizers (Rui et al., 2016). Apart from this, the ratio of IONP usage to chemical fertilizer needs to be determined to make a better plant yield.
5.1.2 In high-performance of nanosensor
Nanosensors have been developed that have proven to be user friendly and able to control the level of soil nutrients. This technology can reduce the consumption of fertilizer and minimize pollution to the environment. It is also beneficial in precision agriculture, which can be used to detect weeds and pesticides (Chhipa and Joshi, 2016). Molecular interactions in biological media can be detected by magnetic nanosensors, which exhibit biocompatibility and high specificity. This causes variations in the spin-spin relaxation times of adjoining water molecules upon target-induced nanoassembly development. There are various molecular targets alternating from enzymatic activity, proteins, specific mRNA, and pathogens; those with a low sensitivity in the femtomole range (0.5–30 fmol) can be detected by these magnetic nanosensors (Perez et al., 2004). For high-performance analysis with nanosensors, the size of the IONP might play a crucial role and vary with different sensing surfaces and sensing systems. For applications in the agricultural sector, the IONP-mediated high-performance can be used to monitor the abundance of important biomarkers, which are responsible for fruit ripening, accumulation of heavy metals in rice grains, the occurrence the pollutant/detrimental compounds, and so on.
5.1.3 Photocatalysis
Photocatalysis involves a catalyst, which also employed with nanotechnology, that requires light and has been developed to increase the reactions involved. It is defined as a material that is utilized for transformation and regenerates its composition after each cycle of relations, which is accomplished by producing electron-hole pairs and absorbed light. Iron oxide is one type of metal oxides that is utilized for light absorption to induce a charge separation process for oxidizing organic substrates. Some research has shown that the anions and radicals generated from the photocatalytic activity of metal oxides could be used as photocatalysts that react with pollutants to reduce them to non-harmful byproducts, including in the agriculture sector. Photocatalytic nanomaterials have been used for the degradation of pollutants and heavy metals; these compounds are detrimental to agricultural products. Therefore, this type of catalyst has great potential for hydrogen production and environmental remediation associated with agriculture (Khan et al., 2015). It was attested that waste materials can be destroyed by this technique under catalysts with light irradiation (Khedr et al., 2009). Similar to the applications with photocatalysis, IONP is also highly associated with electrocatalysis and is well-versed with electrochemical reactions as an electrode interface, in which IONP is rolling to be an electron acceptor/donor and a catalyst. This application has been widely seen in dielectric sensing systems, where the involvement of the IONP accelerates the dipole moment. Dipole moment with IONP speeds up ionic movement and enhances the output signal or display in several folds. Due to the involvement of dipole moment in a wide range of applications including on the sensing surfaces, the involvement of IONP is highly appreciated (Saber et al., 2022). Further, the ionic/molecular vibrations with IONP regarding to the above applications have potential future benefits in different industrial sectors (Ali Noman et al., 2021; Gabelica et al., 2021). The involvement of photocatalysis with IONP is highly beneficial for the purpose disinfection in water and air matrices (Gao and Keller, 2021).
5.2 Types of nanomaterials
5.2.1 Nanowire
Nanowires can be defined as nanostructures that have a diameter or thickness less than or equal to 100 nm. A nanowire can have the properties of an insulator, a semiconductor, or a metal, depending on the composition of the materials. Some of these materials include carbon, silicon, germanium, and a wide variety of metals, such as copper, iron, and gold. These nanostructures hold great interest and potential for both scientific and technical communities due to their capability for greatly reduced device dimensions. Furthermore, nanowires can be utilized for many possible applications, such as electronic devices, optics, and the sensing of proteins and chemicals using semiconductor wires. This small nanostructure has the potential for important advances in computer science because it is a good conductor through which electrons easily pass. Moreover, artificial protein-coding DNA can be created using amino acid nanowires. This advanced technique could be used to facilitate the creation or production of proteins, which could potentially lead to advances in therapeutic applications. A report has shown that nitrilotriacetic acid can be used as a chelating agent to produce iron oxide semiconductor nanowires under an efficient synthesis technique to produce polymeric chains with heat treatment. These nanowires exhibited unique magnetic properties and showed great sensitivities to acetic acid gases and ethanol (Wang et al., 2008).
5.2.2 Nanofiber
Nanofibers have emerged as fibers with a range of nanometer-sized for downstream applications. They exist as one-dimensional nanomaterials for a wide variety of research and have great potential for many advanced applications. The methods of production and the types of polymers used are factors that can affect the diameter of nanofibers. These materials are unique due to their ability to possess extremely great surface-area-to-volume ratios and appreciable mechanical strength (Kenry and Lim, 2017). Moreover, nanofibers can also be created from two types of polymers: natural and synthetic. Natural polymers are often identical to macromolecular substances present in the human body. Examples of natural polymers are cellulose, collagen, gelatin, silk, chitosan, and keratin. Meanwhile, many synthetic polymers are utilized to form nanofibers; they represent the largest class of biomaterials. These involved polyurethane, polycaprolactone, and polylactic acid. Presently, there are different systems for the production of nanofibers, including self-assembly, electrospinning, and phase separation. Among these, electrospinning has emerged as the most used and attractive technique for the production of polymeric biomaterials into nanofibers. This method has the capability to control the size and composition of ultrathin fibers with a simple and straightforward experimental setup along with the involvement of IONP. Furthermore, nanofibers are able to provide many possible applications that are used in drug delivery, tissue engineering, and cancer diagnosis (Vasita and Katti, 2006).
6 Biomedical applications
6.1 Drug delivery
The conception of drug delivery using magnetic nanoparticles was proposed as one of the current technologies that can give birth to a new era in cancer therapy. Magnetic nanostructures, such as iron oxide-based nanoparticles, act as drug delivery agents. They can be attached to a drug to be delivered at the cancer site under the effect of an external magnetic field (magnetic drug delivery; Figures 4, 5). The dosage of drugs required can be reduced, and side effects can be eliminated by attaching drugs to specific sites (Faraji et al., 2010). Mody et al. (2014) demonstrated that the surfaces of iron oxide-based nanostructures have magnetic cores with an external coating of inorganic metals or organic polymers, which can be functionalized to make them biocompatible through the attachment of numerous bioactive molecules. After that, a catheter is used to inject the delivery drug agent into the bloodstream to place the injection site near the target. In this context, the selection of an appropriate probe molecule to reach the target site is crucial.
6.2 Hyperthermia
The use of synthesized nanostructured iron oxide for magnetic hyperthermia has attracted considerable attention as an effective therapy for cancer treatment. The superparamagnetic iron oxide-based nanostructure can convert magnetic energy from an external high-frequency field to particles in heat form. The range of temperature used is normally 41–47°C for the heating of cells. The particles begin to vibrate and produce heat to destroy pathogenic microbes or cancer cells within the magnetic field. The heat generated can be used in an in vivo application to raise the tumor tissue’s temperature (Thoidingjam and Tiku, 2017). The mechanisms that can be utilized by heating are frictional heating prompted by the relationship between the iron oxide-based nanostructure and the medium and eddy current heating that occurs from an alternating pulsed magnetic field (Abenojar et al., 2016). Furthermore, tumor cells are more sensitive to a rise in temperature compared with healthy ones. The advancement in temperature optimization may enhance radiation therapy and chemotherapy (Giustini et al., 2010). Recently, numerous modern techniques have required hyperthermia, which has been developed for the heating treatment of cancers (Wu et al., 2015). Yanase et al. (1998) demonstrated that the application of magnetite cationic liposomal nanoparticles, which have positive surface charges, is efficient for hyperthermia treatment in cancer therapy. Moreover, there is a significant challenge to increasing the heating rates of iron oxide-based nanostructures that require reaching therapeutic temperatures for this application. Increasing heating rates can be an alternative approach to increasing the monodispersity of iron oxide-based nanostructures. As one study showed, the hyperthermia effect of superparamagnetic iron oxide-based nanostructures can be enhanced dramatically with a coating of gold. It is possible for the application of very low rate oscillating magnetic fields from the results revealed (Mohammad et al., 2010).
6.3 Magnetic resonance imaging
Tumors form in humans across a series of procedures that occur over an extended period. Accretion of genetic variations will lead to advanced alteration of a normal cell into a malicious cell during this process (Gupta et al., 2010). MRI is an imaging system used principally in medical applications to create high-quality, detailed pictures of the human body in radiology. A strong magnetic field, radio waves, and an electric field gradient are utilized by MRI scanners to generate images of parts of the body. This application does not involve ultrasound, X-rays, or CT scans (Hanson, 2009). Moreover, this system can enable us to see inside cartilage, joints, ligaments, and muscles to identify various sports injuries. MRI is also able to observe internal body structures and analyze several disorders, such as tumors. It is widely used in research to measure brain structure and function, among other things (Faraji et al., 2010). Various types of antibodies directed to various kinds of receptors can be used to couple on iron oxide-based nanostructures. After that, the tumor can bind with the specific sites of the particles. The targeted site can be delivered to an iron oxide-based nanostructure using an external magnetic field. Furthermore, cell tracking with a resolution that approaches the size of the cell is acceptable when the cell overloads the magnetic iron oxide-based nanostructure (Figure 6). The monitoring of cell therapy and the observation of biological processes can be provided from in vivo cell labeling in MRI (Wu et al., 2015).
6.4 Bioseparation
Bioseparation is one of the most important applications in the synthesis of IONP. Superparamagnetic colloids are ultimate for this technique due to the nature of their magnetization, which assists in the passage of biomaterials with a magnetic field. Basically, the separation of specific biological entities is often involved in this application in biomedical research. This application is usually involved in cell, bacteria, virus, enzyme, protein, gene, protein, and in vitro DNA separation. Magnetic bioseparation has various advantages, including the ability to retrieve or localize with a magnet more quickly than traditional separation techniques. Magnetic iron oxide-based nanostructures have various characteristics for this application, as they have a high surface area and a small size. They are also more cost-effective and faster than traditional column affinity chromatography. Bioseparation is generally enhanced by surface-functionalized magnetic iron oxide-based nanostructures with suitable intermediate efficiency and alteration of polymers and surfactants for introducing functional end groups (Wu et al., 2015). Li et al. (2011) investigated how carboxymethylated dextran-coated magnetic iron oxide-based nanostructures can be synthesized using the co-precipitation method for bioseparation application. The particles covered with antibodies provide the ability for the antigen to separate from the sample solution, as they have superparamagnetic properties.
7 Environmental applications
Recently, many researchers have been attracted to the environmental applications of magnetic iron oxide-based nanostructures. These nanoparticles have been proven to have great potential for the removal of both organic and inorganic pollutants. Regardless of some still unresolved uncertainties related to the application of iron oxide-based nanostructures, the synthesized iron oxide-based nanostructures are predictable as beneficial tools in the rectification of a wide variety of pollutants in water, soil, and air at both experimental and field levels. Moreover, iron oxide-based nanostructures exhibit great flexibility for in situ applications. Modified iron oxide-based nanostructures that support and catalyze nanoparticles have been synthesized to further improve the speed and efficacy of remediation nanoparticles (Faraji et al., 2010). Tran et al. (2011) demonstrated that an iron oxide-based nanostructure can serve as a talented adsorbent for the removal of toxic metals, such as lead and arsenic, in contaminated water. The applications of synthesized iron oxide-based nanostructures are further expanded for different fields, and they have been revealed [85,86].
8 Antimicrobial activity of synthesized iron oxide nanoparticles
Antimicrobial activity can be identified by various classes of microorganisms. It is also known as the minimum inhibitory concentration, which is the lowest concentration of an antimicrobial agent that prevents the growth of bacteria after overnight incubation [87]. Four small filter paper discs were inoculated with the plant extract, solvent, antibiotics, and the synthesized IONP and then placed on the prepared agar plates of indicator organisms. The plates were incubated at 35–37°C for 24 h. The zone of bacterial inhibition could be investigated on agar plates after the incubation period. The zone of clearance around each filter paper showed that the antimicrobial activity of the specimens occurred on the agar plates. There are some factors that might affect the interpretation and results of antimicrobial activity: the incubation conditions, the concentration of the filter disc, and the agar plate of the indicator organism (Ramanathan and Gopinath, 2017). A report revealed that erythromycin as an antimicrobial agent was analyzed with an iron oxide-based nanostructure, and its antimicrobial characteristics were investigated against the bacterial culture of S. pneumoniae [88]. For antimicrobial applications with IONP, the condition to be optimized for the desired ratio as it causes the major output [88,89]. The biomedical applications of iron oxide nanoparticles have been further expanded with antimicrobial potential against a wide range of microbes, anti-tumour and cancer therapies (Sangaiya and Jayaprakash, 2018; Table 2).
9 Future perspectives
As discussed in this review, biological methods, especially for recycling agro-wastes, are found to be highly potential and reliable. Direct reduction of precursors under ambient conditions is classic and can be performed in low-resource laboratories. The capping of compounds from plants seems promising, and it can be scaled up in an easier way. For scaling-up purposes, for instance, with the production point in the cosmetics industry, determining the optimum conditions, such as temperature, reaction time, and usage of the right precursor, are mandatory. In addition, analyzing the primary compounds from plants is mandatory, and they bring a great increment in the final reduced product. Apart from the standard agro-wastes mediated by green synthesis, the implementation of methods generated for the production of IONP needs to be considered [6–8, 14]. Along with the methods discussed in this review, the involvement of other physical and chemical methods in greener ways might be associated. These directions will pave the way for making IONP in different nanostructures. In general, the production of nanoparticles under the routine classic method is spherical in shape, but the size may vary depending on the desired condition(s). However, with several current practical necessities, it demands different nanostructures, primarily for expanding surface areas. Surface expansion by modifying the nanostructure is greatly implemented in biomolecular capturing, particularly for bionanosensor development. The crucial part of generating different nanostructures is the right additional method. The popular methods include lithography, nanoprinting, exposure by laser, and ionic implantation (Figure 7).
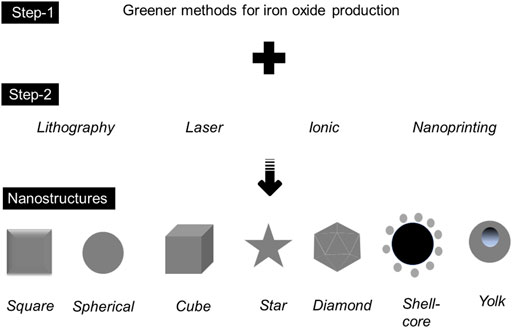
FIGURE 7. The production of different iron oxide-based nanostructures, including the involvement of other methods (step 2) along with greener production (step 1).
Compared to other organic nanoparticles, iron oxide nanoparticles have several practical advantages, such as readily forming oxides for the ultimate use in surface functionalization. Glutaraldehyde is the most common cross-linking reagent because it is easily accessible for linking two amines. On the IONP, glutaraldehyde can be functionalized through any amine coupling, such as 3-(aminopropyl)triethoxysilane. The use of glutaraldehyde as a multifunctional reagent can be reduced by drastic modification with an aminated probe during the immobilization process. Another cross-linking option for IONP with biomolecules is between amine and carboxyl on the substrate or receptor. The reaction between amine and carboxyl can be activated followed by stabilization with 1-Ethyl-3-(3-dimethylaminopropyl) carbodiimide and N-hydroxy succinimide-mediated coupling reactions. Both amine-carboxyl coupling and glutaraldehyde modifications on IONP are highly feasible. Iron oxides with nanomaterials have the potential to garner ideal surface functionalization, as oxides are reliable for making simple chemical reactions with linkers. Enhancement of oxides or making the oxide surface is achieved by treating the surface with potassium hydroxide. Iron nanomaterials for biomolecular immobilization are greatly utilizing the above functional groups, and the presence of different functional groups on the surface expands the applications. Further, iron oxides are highly in use with commercial cosmetic sectors and as ore, catalysts, pigment, and thermite. As revealed elsewhere, iron oxide nanoparticles are highly magnetic, which is not so with other nanomaterials. Current investigations brought several insights with the generation greener, heterostructured and hybrid materials, may help for further expansions (Chandraker et al., 2022; Sahu et al., 2022).
10 Conclusion
The review gleaned important aspects on the production of iron oxide nanoparticle and the associated downstream applications. This paper highlights the environmentally benign manufacture of iron oxide-based nanostructures using a straightforward process, as well as their uses in sustainable agriculture and medical fields. This overview offers numerous suggestions for increasing the use of iron oxide nanoparticles in various fields. Additionally, options were given to choose a suitable plant for the applications in agriculture and other sectors, along with suggested future developments along the green production. The green production of nanostructures has received abundant attention and has been actively explored recently because of their various beneficial applications and properties. The biological production of the nanostructure is known as green technology due to the manipulation of various plant materials and because it is biocompatible, non-toxic, and does not include any harmful substances. However, need to consider the presence of toxic or physiologically irrelevant compounds in some plant species. Based on our review, the green synthesis of iron oxide-based nanostructures under biological methods using plant extracts was found to be the preferable method due to its environmentally friendly, simple, non-toxic, and cost-effective manufacturing of iron oxide-based nanostructures. Polysaccharides and biomolecules in plant extracts are used as stabilizers and reducing agents for the green production of iron oxide-based nanostructures. The production of high-efficiency iron oxide-based nanostructures has attracted several researchers for the study of nanoparticles because of their small size and large surface-to-volume ratio. This dimensional appearance provides unique properties to nanoparticles. The size, shape and dimension of the produced nanomaterials may be affected by the level of compounds extracted from the plant source. The synthesis of iron oxide-based nanostructures by physical and chemical methods is unfavorable compared with biological methods because of the usage of chemical reagents, the extreme consumption of energy that requires equipment, and the longer time to complete nanoparticle synthesis. These factors lead to the formation of contaminated and hazardous nanostructured iron oxides. Moreover, this type of nanoparticle potentially invites great interest for researchers in bio-applications, data storage, and catalysis. Iron oxide-based nanostructures can act as antimicrobial agents, as their antimicrobial properties can be further explored in the biomedical industry. Furthermore, iron oxide-based nanostructures have created a demand for the elimination of pollutants in wastewater treatment. Hence, the synthesis of iron oxide-based nanostructures by an eco-friendly method using plant extracts as a reducing and capping agent has great potential for the production of large-scale iron oxide-based nanostructures. Considering other side, using green production with a large scale it is necessary to be optimized for the conditions to be used. In addition, the optimal condition may vary with the abundance of the capping and reducing agents.
Author contributions
Conceptualization, LP and SG; methodology, LP; software, SC; validation, LP, SG, SS, YC, and PV; formal analysis, LP, SG, SS, YC, and PV; investigation, LP, SG, SS, and YC; resources, RG; data curation, VL; writing—original draft preparation, LP and SG; writing—review and editing, LP, SG, SS, YC, PV, SC, RG, and VL; visualization, LP and SG; supervision, SG; project administration, LP and SG; funding acquisition, SG. All authors have read and agreed to the published version of the manuscript.
Funding
Funded by The Ministry of Higher Education, Malaysia under Fundamental Research Grant Scheme (FRGS/1/2019/TK10/UNIMAP/03/3).
Conflict of interest
The authors declare that the research was conducted in the absence of any commercial or financial relationships that could be construed as a potential conflict of interest.
Publisher’s note
All claims expressed in this article are solely those of the authors and do not necessarily represent those of their affiliated organizations, or those of the publisher, the editors and the reviewers. Any product that may be evaluated in this article, or claim that may be made by its manufacturer, is not guaranteed or endorsed by the publisher.
References
Abenojar, E. C., Wickramasinghe, S., Bas-Concepcion, J., and Samia, A. C. S. (2016). Structural effects on the magnetic hyperthermia properties of iron oxide nanoparticles. Prog. Nat. Sci. Mater. Int. 26, 440–448. doi:10.1016/J.PNSC.2016.09.004
Ahmed, R. H., and Mustafa, D. E. (2020). Green synthesis of silver nanoparticles mediated by traditionally used medicinal plants in Sudan. Int. Nano Lett. 10, 1–14. doi:10.1007/s40089-019-00291-9
Ahmed, S., Ahmad, M., Swami, B. L., and Ikram, S. (2016). Green synthesis of silver nanoparticles using Azadirachta indica aqueous leaf extract. J. Radiat. Res. Appl. Sci. 9, 1–7. doi:10.1016/J.JRRAS.2015.06.006
Ali, A., Zafar, H., Zia, M., Ul Haq, I., Phull, A. R., Ali, J. S., et al. (2016). Synthesis, characterization, applications, and challenges of iron oxide nanoparticles. Nanotechnol. Sci. Appl. 9, 49–67. doi:10.2147/NSA.S99986
Ali Noman, E., Al-Gheethi, A., Saphira Radin Mohamed, R. M., Talip, B. A., Hossain, M. S., Ali Hamood Altowayti, W., et al. (2021). Sustainable approaches for removal of cephalexin antibiotic from non-clinical environments: A critical review. J. Hazard. Mat. 417, 126040. doi:10.1016/j.jhazmat.2021.126040
Alkhalaf, M. I., Hussein, R. H., and Hamza, A. (2020). Green synthesis of silver nanoparticles by Nigella sativa extract alleviates diabetic neuropathy through anti-inflammatory and antioxidant effects. Saudi J. Biol. Sci. 27, 2410–2419. doi:10.1016/j.sjbs.2020.05.005
Alves, J. O., Tenório, J. A. S., Zhuo, C., and Levendis, Y. A. (2012). Characterization of nanomaterials produced from sugarcane bagasse. J. Mat. Res. Technol. 1, 31–34. doi:10.1016/s2238-7854(12)70007-8
Ansari, Z., Sarkar, K., Saha, A., Singha, A., and Sen, K. (2016). Enhanced anion sensing by γ-irradiated polyphenol capped iron oxide nanoparticles. J. Radioanal. Nucl. Chem. 308, 517–525. doi:10.1007/s10967-015-4473-y
Awwad, A. M., and Salem, N. M. (2012). A green and facile approach for synthesis of magnetite nanoparticles. Nanosci. Nanotechnol. 2, 208–213. doi:10.5923/j.nn.20120206.09
Awwad, A. M., Salem, N. M., and Abdeen, A. O. (2013). Green synthesis of silver nanoparticles using carob leaf extract and its antibacterial activity. Int. J. Industrial Chem. 4, 29. doi:10.1186/2228-5547-4-29
Baby, C., Prasad, A., Kanchana, A., Rajeswary, M., Sajitha, M., Praveen, K. H., et al. (2017). Biogenic synthesis of iron oxide nanoparticles using leaf extract of Clitoria ternatea. Int. J. Adv. Res. Sci. Eng. 06, 66–71.
Balamurugan, M., Saravanan, S., and Soga, T. (2014). Synthesis of iron oxide nanoparticles by using Eucalyptus globulus plant extract. e-J. Surf. Sci. Nanotechnol. 12, 363–367. doi:10.1380/ejssnt.2014.363
Bao, J. F., He, P., Yu, X. J., Jiang, S. L., and Yan, Q. Y. (2009). Detection of Hg2+ using gold nanoparticle probes. Acta Chim. Sin. 67, 2139–2143.
Batool, F., Iqbal, M. S., Khan, S. U. D., Khan, J., Ahmed, B., and Qadir, M. I. (2021). Biologically synthesized iron nanoparticles (FeNPs) from Phoenix dactylifera have anti-bacterial activities. Sci. Rep. 11, 22132. doi:10.1038/s41598-021-01374-4
Behravan, M., Hossein Panahi, A., Naghizadeh, A., Ziaee, M., Mahdavi, R., and Mirzapour, A. (2019). Facile green synthesis of silver nanoparticles using Berberis vulgaris leaf and root aqueous extract and its antibacterial activity. Int. J. Biol. Macromol. 124, 148–154. doi:10.1016/j.ijbiomac.2018.11.101
BhartiJangwan, J. S., Kumar, S. S., Kumar, V., Kumar, A., and Kumar, D. (2022). A review on the capability of zinc oxide and iron oxides nanomaterials, as a water decontaminating agent: Adsorption and photocatalysis. Appl. Water Sci. 12, 46. doi:10.1007/s13201-021-01566-3
Campos, E. A., Stockler Pinto, D. V. B., Oliveira, J. I. S. de, Mattos, E. D. C., Dutra, R. D. C. L., Campos, E. A., et al. (2015). Synthesis, characterization and applications of iron oxide nanoparticles - a short review. J. Aerosp. Technol. Manag. 7, 267–276. doi:10.5028/jatm.v7i3.471
Cantillon-Murphy, P., Wald, L. L., Adalsteinsson, E., and Zahn, M. (2010). Simulating magnetic nanoparticle behavior in low-field MRI under transverse rotating fields and imposed fluid flow. J. Magn. Magn. Mat. 322, 2607–2617. doi:10.1016/j.jmmm.2010.03.029
Chandraker, S. K., Lal, M., Dhruve, P., Yadav, A. K., Singh, R. P., Varma, R. S., et al. (2022). Bioengineered and biocompatible silver nanoparticles from Thalictrum foliolosum DC and their biomedical applications. Clean. Techn. Environ. Policy. doi:10.1007/s10098-022-02329-7
Chhipa, H., and Joshi, P. (2016). Nanofertilisers, nanopesticides and nanosensors in agriculture. Cham: Springer, 247–282. doi:10.1007/978-3-319-39303-2_9
Chung, I. M., Park, I., Seung-Hyun, K., Thiruvengadam, M., and Rajakumar, G. (2016). Plant-mediated synthesis of silver nanoparticles: Their characteristic properties and therapeutic applications. Nanoscale Res. Lett. 11, 40–14. doi:10.1186/s11671-016-1257-4
Cortez-Jugo, C., Czuba-Wojnilowicz, E., Tan, A., and Caruso, F. (2021). A focus on “bio” in bio–nanoscience: The impact of biological factors on nanomaterial interactions. Adv. Healthc. Mat. 10, 2100574. doi:10.1002/adhm.202100574
Ditta, A., Arshad, M., and Ibrahim, M. (2015). “Nanoparticles in sustainable agricultural crop production: Applications and perspectives,” in Nanotechnology and plant sciences (Cham: Springer International Publishing), 55–75. doi:10.1007/978-3-319-14502-0_4
Ehrampoush, M. H., Miria, M., Salmani, M. H., and Mahvi, A. H. (2015). Cadmium removal from aqueous solution by green synthesis iron oxide nanoparticles with tangerine peel extract. J. Environ. Health Sci. Eng. 13, 84. doi:10.1186/s40201-015-0237-4
Epps, R. W., and Abolhasani, M. (2021). Modern nanoscience: Convergence of AI, robotics, and colloidal synthesis. Appl. Phys. Rev. 8, 041316. doi:10.1063/5.0061799
Faraji, M., Yamini, Y., and Rezaee, M. (2010). Magnetic nanoparticles: Synthesis, stabilization, functionalization, characterization, and applications. J. Iran. Chem. Soc. 7, 1–37. doi:10.1007/BF03245856
Gabelica, I., Ćurković, L., Mandić, V., Panžić, I., Ljubas, D., and Zadro, K. (2021). Rapid microwave-assisted synthesis of fe3o4/sio2/tio2 core-2-layer-shell nanocomposite for photocatalytic degradation of ciprofloxacin. Catalysts 11, 1136. doi:10.3390/catal11101136
Gaillet, S., and Rouanet, J.-M. (2015). Silver nanoparticles: Their potential toxic effects after oral exposure and underlying mechanisms – a review. Food Chem. Toxicol. 77, 58–63. doi:10.1016/j.fct.2014.12.019
Gao, Q., and Keller, A. A. (2021). Redesigning water disinfection using recyclable nanomaterials and metal ions: Evaluation with Escherichia coli. ACS Est. Water 1, 185–194. doi:10.1021/acsestwater.0c00066
Ghosh, M. K., Jain, K., Khan, S., Das, K., and Ghorai, T. K. (2020). New dual-functional and reusable bimetallic Y2ZnO4 nanocatalyst for organic transformation under microwave/green conditions. ACS Omega 5, 4973–4981. doi:10.1021/acsomega.9b03875
Giustini, A. J., Petryk, A. A., Cassim, S. M., Tate, J. A., Baker, I., and Hoopes, P. J. (2010). Magnetic nanoparticle hyperthermia in cancer treatment. Nano Life 1, 17–32. doi:10.1142/S1793984410000067
Gleiter, H. (2000). Nanostructured materials: Basic concepts and microstructure. Acta Mat. 48, 1–29. doi:10.1016/S1359-6454(99)00285-2
Groiss, S., Selvaraj, R., Varadavenkatesan, T., and Vinayagam, R. (2017). Structural characterization, antibacterial and catalytic effect of iron oxide nanoparticles synthesised using the leaf extract of Cynometra ramiflora. J. Mol. Struct. 1128, 572–578. doi:10.1016/j.molstruc.2016.09.031
Gupta, S. C., Kim, J. H., Prasad, S., and Aggarwal, B. B. (2010). Regulation of survival, proliferation, invasion, angiogenesis, and metastasis of tumor cells through modulation of inflammatory pathways by nutraceuticals. Cancer Metastasis Rev. 29, 405–434. doi:10.1007/s10555-010-9235-2
Hanson, L. G. (2009). Introduction to magnetic resonance imaging techniques. New Delhi: DTU Library.
Herlekar, M., Barve, S., and Kumar, R. (2015). “Biological synthesis of iron oxide nanoparticles using agro-wastes and feasibility for municipal wastewater treatment,” in Conference: 47th Indian Water Works Annual Convention, Kolkata, India, 30 Jan-01 Feb 2015.
Herlekar, M., Barve, S., and Kumar, R. (2014). Plant-mediated green synthesis of iron nanoparticles. J. Nanoparticles 2014, 1–9. doi:10.1155/2014/140614
Huguet-Casquero, A., Gainza, E., and Pedraz, J. L. (2021). Towards Green Nanoscience: From extraction to nanoformulation. Biotechnol. Adv. 46, 107657. doi:10.1016/j.biotechadv.2020.107657
Ikram, M., Javed, B., Hassan, S. W. U., Satti, S. H., Sarwer, A., Raja, N. I., et al. (2021). Therapeutic potential of biogenic titanium dioxide nanoparticles: A review on mechanistic approaches. Nanomedicine 16, 1429–1446. doi:10.2217/nnm-2021-0020
Irshad, R., Tahir, K., Li, B., Ahmad, A., Siddiqui, R., et al. (2017). Antibacterial activity of biochemically capped iron oxide nanoparticles: A view towards green chemistry. J. Photochem. Photobiol. B Biol. 170, 241–246. doi:10.1016/j.jphotobiol.2017.04.020
Jain, A. S., Pawar, P. S., Sarkar, A., Junnuthula, V., and Dyawanapelly, S. (2021). Bionanofactories for green synthesis of silver nanoparticles: Toward antimicrobial applications. Int. J. Mol. Sci. 22, 11993. doi:10.3390/ijms222111993
Jalab, J., Abdelwahed, W., Kitaz, A., and Al-Kayali, R. (2021). Green synthesis of silver nanoparticles using aqueous extract of Acacia cyanophylla and its antibacterial activity. Heliyon 7, e08033. doi:10.1016/j.heliyon.2021.e08033
Jamlos, Y. M. N., and MF, A. H. M. S. (2017). Synthesis of superparamagnetic hydroxyapatite core-shell nanostructure by a rapid sol-gel route. e-J. Surf. Sci. Nanotech. 15, 121–126. doi:10.1380/ejssnt.2017.121
Kandpal, N. D., Sah, N., Loshali, R., Joshi, R., and Prasad, J. (2014). Co-precipitation method of synthesis and characterization of iron oxide nanoparticles. J. Sci. Ind. Res. 73, 87–90.
Kenry, , and Lim, C. T. (2017). Nanofiber technology: Current status and emerging developments. Prog. Polym. Sci. 70, 1–17. doi:10.1016/J.PROGPOLYMSCI.2017.03.002
Khan, M. M., Adil, S. F., and Al-Mayouf, A. (2015). Metal oxides as photocatalysts. J. Saudi Chem. Soc. 19, 462–464. doi:10.1016/J.JSCS.2015.04.003
Khedr, M. H., Abdel Halim, K. S., and Soliman, N. K. (2009). Synthesis and photocatalytic activity of nano-sized iron oxides. Mat. Lett. 63, 598–601. doi:10.1016/J.MATLET.2008.11.050
Kumar, S., Sikdar, S., Kumar, M., and Kumar, T. (2022). Materials Science for Energy Technologies Investigation of photocatalytic and DNA interaction of novel heterostructured GO/Bi 2 O 3/ZnO nanocomposite. Mat. Sci. Energy Technol. 5, 324–333. doi:10.1016/j.mset.2022.07.002
Lakshmnarayanan, S., Shereen, M. F., Niraimathi, K. L., Brindha, P., and Arumugam, A. (2021). One-pot green synthesis of iron oxide nanoparticles from Bauhinia tomentosa: Characterization and application towards synthesis of 1, 3 diolein. Sci. Rep. 11, 8643. doi:10.1038/s41598-021-87960-y
Latha, N., and Gowri, M. (2014). Bio synthesis and characterisation of Fe 3 o 4 nanoparticles using Caricaya papaya leaves extract. Int. J. Sci. Res. 3, 1551–1556.
Lee, J. H., Cho, H. Y., Choi, H. K., Lee, J. Y., and Choi, J. W. (2018). Application of gold nanoparticle to plasmonic biosensors. Int. J. Mol. Sci. 19, 2021. doi:10.3390/ijms19072021
Li, J., Zhou, Y., Li, M., Xia, N., Huang, Q., Do, H., et al. (2011). Carboxymethylated dextran-coated magnetic iron oxide nanoparticles for regenerable bioseparation. J. Nanosci. Nanotechnol. 11, 10187–10192. doi:10.1166/jnn.2011.5002
Lingamdinne, L. P., Koduru, J. R., and Karri, R. R. (2019). A comprehensive review of applications of magnetic graphene oxide based nanocomposites for sustainable water purification. J. Environ. Manage. 231, 622–634. doi:10.1016/j.jenvman.2018.10.063
Madheswaran, B. (2014). National conference on green engineering and technologies for sustainable future-2014 ocimum sanctum leaf extract mediated green synthesis of iron oxide nanoparticles: Spectroscopic and microscopic studies Fig.1 visual observations of A -leaf extract, B -. JCHPS Spec. Issue 4, 201–204.
Mahdavi, M., Namvar, F., Ahmad, M., and Mohamad, R. (2013). Green biosynthesis and characterization of magnetic iron oxide (Fe3O4) nanoparticles using seaweed (sargassum muticum) aqueous extract. Molecules 18, 5954–5964. doi:10.3390/molecules18055954
Makarov, V. V., Makarova, S. S., Love, A. J., Sinitsyna, O. V., Dudnik, A. O., Yaminsky, I. V., et al. (2014). Biosynthesis of stable iron oxide nanoparticles in aqueous extracts of Hordeum vulgare and Rumex acetosa plants. Langmuir 30, 5982–5988. doi:10.1021/la5011924
Mody, V. V., Cox, A., Shah, S., Singh, A., Bevins, W., and Parihar, H. (2014). Magnetic nanoparticle drug delivery systems for targeting tumor. Appl. Nanosci. 4, 385–392. doi:10.1007/s13204-013-0216-y
Mohamad, N. A. N., Arham, N. A., Jai, J., and Hadi, A. (2013). Plant extract as reducing agent in synthesis of metallic nanoparticles: A review. Adv. Mat. Res. 832, 350–355. doi:10.4028/www.scientific.net/amr.832.350
Mohammad, F., Balaji, G., Weber, A., Uppu, R. M., and Kumar, C. S. S. R. (2010). Influence of gold nanoshell on hyperthermia of superparamagnetic iron oxide nanoparticles. J. Phys. Chem. C 114, 19194–19201. doi:10.1021/jp105807r
Mukhopadhyay, S. S. (2014). Nanotechnology in agriculture: Prospects and constraints. Nanotechnol. Sci. Appl. 7, 63–71. doi:10.2147/NSA.S39409
Nguyen, T. T., Huynh, K. A., Padungthon, S., Pranudta, A., Amonpattaratkit, P., Tran, L. B., et al. (2021). Synthesis of natural flowerlike iron-alum oxide with special interaction of Fe-Si-Al oxides as an effective catalyst for heterogeneous Fenton process. J. Environ. Chem. Eng. 9, 105732. doi:10.1016/j.jece.2021.105732
Niraimathee, V. A., Subha, V., Ernest Ravindran, R. S., and Renganathan, S. (2016a). Green synthesis of iron oxide nanoparticles from Mimosa pudica root extract. Int. J. Environ. Sustain. Dev. 15, 227. doi:10.1504/IJESD.2016.077370
Parhi, B., Bharatiya, D., and Swain, S. K. (2020). Application of quercetin flavonoid based hybrid nanocomposites: A review. Saudi Pharm. J. 28, 1719–1732. doi:10.1016/j.jsps.2020.10.017
Parveen, K., Banse, V., and Ledwani, L. (2016). Green synthesis of nanoparticles: Their advantages and disadvantages. AIP Conf. Proc. 1724, 020048. doi:10.1063/1.4945168
Patra, J. K., and Baek, K.-H. (2017). Green biosynthesis of magnetic iron oxide (Fe3O4) nanoparticles using the aqueous extracts of food processing wastes under photo-catalyzed condition and investigation of their antimicrobial and antioxidant activity. J. Photochem. Photobiol. B Biol. 173, 291–300. doi:10.1016/j.jphotobiol.2017.05.045
Perez, J. M., Josephson, L., and Weissleder, R. (2004). Use of magnetic nanoparticles as nanosensors to probe for molecular interactions. ChemBioChem 5, 261–264. doi:10.1002/cbic.200300730
Periakaruppan, R., Li, J., Mei, H., Yu, Y., Hu, S., Chen, X., et al. (2021). Agro-waste mediated biopolymer for production of biogenic nano iron oxide with superparamagnetic power and antioxidant strength. J. Clean. Prod. 311, 127512. doi:10.1016/j.jclepro.2021.127512
Prasad, A. S. (2016). Iron oxide nanoparticles synthesized by controlled bio-precipitation using leaf extract of Garlic Vine (Mansoa alliacea). Mat. Sci. Semicond. process. 53, 79–83. doi:10.1016/J.MSSP.2016.06.009
Prodan, A. M., Iconaru, S. L., Ciobanu, C. S., Chifiriuc, M. C., Stoicea, M., and Predoi, D. (2013). Iron oxide magnetic nanoparticles: Characterization and toxicity evaluation by in vitro and in vivo assays. J. Nanomater. 2013, 1–10. doi:10.1155/2013/587021
Qindeel, M., Barani, M., Rahdar, A., Arshad, R., and Cucchiarini, M. (2021). Nanomaterials for the diagnosis and treatment of urinary tract infections. Nanomaterials 11, 546. doi:10.3390/nano11020546
Ramanathan, S., and Gopinath, S. C. B. (2017). Potentials in synthesizing nanostructured silver particles. Microsyst. Technol. 23, 4345–4357. doi:10.1007/s00542-017-3382-0
Rameshaiah, G. N., Pallavi, J., and Shabnam, S. (2015). Nano fertilizers and nano sensors – An attempt for developing smart agriculture. Int. J. Eng. Res. Gen. Sci. 3, 314–320.
Ramimoghadam, D., Bagheri, S., and Hamid, S. B. A. (2014). Progress in electrochemical synthesis of magnetic iron oxide nanoparticles. J. Magn. Magn. Mat. 368, 207–229. doi:10.1016/j.jmmm.2014.05.015
Rasheed, R., and Meera, V. (2016). Synthesis of iron oxide nanoparticles coated sand by biological method and chemical method. Procedia Technol. 24, 210–216. doi:10.1016/j.protcy.2016.05.029
Rui, M., Ma, C., Hao, Y., Guo, J., Rui, Y., Tang, X., et al. (2016). Iron oxide nanoparticles as a potential iron fertilizer for peanut (Arachis hypogaea). Front. Plant Sci. 7, 815. doi:10.3389/fpls.2016.00815
Saber, O., Kotb, H. M., Osama, M., and Khater, H. A. (2022). An effective photocatalytic degradation of industrial pollutants through converting titanium oxide to magnetic nanotubes and hollow nanorods by kirkendall effect. Nanomaterials 12, 440. doi:10.3390/nano12030440
Sahu, J. N., Karri, R. R., and Jayakumar, N. S. (2021). Improvement in phenol adsorption capacity on eco-friendly biosorbent derived from waste Palm-oil shells using optimized parametric modelling of isotherms and kinetics by differential evolution. Ind. Crops Prod. 164, 113333. doi:10.1016/j.indcrop.2021.113333
Sahu, S. K., Sikdar, S., Ghosh, M. K., and Ghorai, T. K. (2022). Investigation of photocatalytic and DNA interaction of novel heterostructured GO/Bi2O3/ZnO nanocomposite. Mat. Sci. Energy Technol. 5, 324–333. doi:10.1016/j.mset.2022.07.002
Sangaiya, P., and Jayaprakash, R. (2018). A review on iron oxide nanoparticles and their biomedical applications. J. Supercond. Nov. Magn. 31, 3397–3413. doi:10.1007/s10948-018-4841-2
Saranya, S., Vijayarani, K., and Pavithra, S. (2017). Green synthesis of iron nanoparticles using aqueous extract of musa ornata flower sheath against pathogenic bacteria. Indian J. Pharm. Sci. 79, 688–694. doi:10.4172/pharmaceutical-sciences.1000280
Schneider, M. G. M., Martín, M. J., Otarola, J., Vakarelska, E., Simeonov, V., Lassalle, V., et al. (2022). Biomedical applications of iron oxide nanoparticles: Current insights progress and perspectives. Pharmaceutics 14, 204. doi:10.3390/pharmaceutics14010204
Sharma, D., Kanchi, S., and Bisetty, K. (2015). Biogenic synthesis of nanoparticles: A review. Arab. J. Chem. 12, 3576–3600. doi:10.1016/J.ARABJC.2015.11.002
Shokrollahi, H. (2017). A review of the magnetic properties, synthesis methods and applications of maghemite. J. Magn. Magn. Mat. 426, 74–81. doi:10.1016/j.jmmm.2016.11.033
Smuleac, V., Varma, R., Sikdar, S., and Bhattacharyya, D. (2011). Green synthesis of Fe and Fe/Pd bimetallic nanoparticles in membranes for reductive degradation of chlorinated organics. J. Memb. Sci. 379, 131–137. doi:10.1016/j.memsci.2011.05.054
Soetaert, F., Korangath, P., Serantes, D., Fiering, S., and Ivkov, R. (2020). Cancer therapy with iron oxide nanoparticles: Agents of thermal and immune therapies. Adv. Drug Deliv. Rev. 163–164, 65–83. doi:10.1016/j.addr.2020.06.025
Speranza, G., Minati, L., Torrengo, S., Rossi, B., Migliaresi, C., Maniglio, D., et al. (2010). XPS characterization of iron oxide and gold nanoparticles for tumor care. Adv. Sci. Technol. 76, 165–170. doi:10.4028/www.scientific.net/AST.76.165
Tamang, A. M., Singh, N., Chandraker, S. K., and Ghosh, M. K. (2022). Solvent impregnated resin a potential alternative material for separation dyes, metal and phenolic compounds: A review. Curr. Res. Green Sustain. Chem. 5, 100232. doi:10.1016/j.crgsc.2021.100232
Thakur, S., and Karak, N. (2014). One-step approach to prepare magnetic iron oxide/reduced graphene oxide nanohybrid for efficient organic and inorganic pollutants removal. Mat. Chem. Phys. 144, 425–432. doi:10.1016/J.MATCHEMPHYS.2014.01.015
Thoidingjam, S., and Tiku, A. B. (2017). New developments in breast cancer therapy: Role of iron oxide nanoparticles. Adv. Nat. Sci. Nanosci. Nanotechnol. 8, 023002. doi:10.1088/2043-6254/aa5e33
Tran, D. L., Le, V. H., Pham, H. L., Hoang, T. M. N., Nguyen, T. Q., Luong, T. T., et al. (2011). Biomedical and environmental applications of magnetic nanoparticles. Adv. Nat. Sci. Nanosci. Nanotechnol. 1, 045013. doi:10.1088/2043-6262/1/4/045013
Usman, M., Farooq, M., Wakeel, A., Nawaz, A., Cheema, S. A., Rehman, H. ur, et al. (2020). Nanotechnology in agriculture: Current status, challenges and future opportunities. Sci. Total Environ. 721, 137778. doi:10.1016/j.scitotenv.2020.137778
Vaanamudan, A., Sarkar, M., Sadhu, M., and Pamidimukkala, P. S. (2019). Clusters of superparamagnetic iron oxide nanoparticles capped with palm shell extract and modified with chitosan: Catalytic applications for degradation of cationic and anionic dyes and their binary mixtures. J. Environ. Chem. Eng. 7, 103244. doi:10.1016/j.jece.2019.103244
Vanlalveni, C., Lallianrawna, S., Biswas, A., Selvaraj, M., Changmai, B., and Rokhum, S. L. (2021). Green synthesis of silver nanoparticles using plant extracts and their antimicrobial activities: A review of recent literature. RSC Adv. 11, 2804–2837. doi:10.1039/d0ra09941d
Vasita, R., and Katti, D. S. (2006). Nanofibers and their applications in tissue engineering. Int. J. Nanomedicine 1, 15–30. doi:10.2147/nano.2006.1.1.15
Venkateswarlu, S., Natesh Kumar, B., Prasad, C. H., Venkateswarlu, P., and Jyothi, N. V. V. (2014). Bio-inspired green synthesis of Fe3O4 spherical magnetic nanoparticles using Syzygium cumini seed extract. Phys. B Condens. Matter 449, 67–71. doi:10.1016/J.PHYSB.2014.04.031
Venkateswarlu, S., Rao, Y. S., Balaji, T., Prathima, B., and Jyothi, N. (2013). Biogenic synthesis of Fe3O4 magnetic nanoparticles using plantain peel extract. Mat. Lett. 100, 241–244. doi:10.1016/j.matlet.2013.03.018
Wang, G., Gou, X., Horvat, J., and Park, J. (2008). Facile synthesis and characterization of iron oxide semiconductor nanowires for gas sensing application. J. Phys. Chem. C 112, 15220–15225. doi:10.1021/jp803869e
Wang, T., Lin, J., Chen, Z., Megharaj, M., and Naidu, R. (2014). Green synthesized iron nanoparticles by green tea and eucalyptus leaves extracts used for removal of nitrate in aqueous solution. J. Clean. Prod. 83, 413–419. doi:10.1016/j.jclepro.2014.07.006
Winsett, J., Moilanen, A., Paudel, K., Kamali, S., Ding, K., Cribb, W., et al. (2019). Quantitative determination of magnetite and maghemite in iron oxide nanoparticles using Mössbauer spectroscopy. SN Appl. Sci. 1, 1636. doi:10.1007/s42452-019-1699-2
Wu, W., He, Q., and Jiang, C. (2008). Magnetic iron oxide nanoparticles: Synthesis and surface functionalization strategies. Nanoscale Res. Lett. 3, 397–415. doi:10.1007/s11671-008-9174-9
Wu, W., Wu, Z., Yu, T., Jiang, C., and Kim, W.-S. (2015). Recent progress on magnetic iron oxide nanoparticles: Synthesis, surface functional strategies and biomedical applications. Sci. Technol. Adv. Mat. 16, 023501. doi:10.1088/1468-6996/16/2/023501
Xing, M., Ge, L., Wang, M., Li, Q., Li, X., and Ouyang, J. (2014). Nanosilver particles in medical applications: Synthesis, performance, and toxicity. Int. J. Nanomedicine 9, 2399–2407. doi:10.2147/IJN.S55015
Yanase, M., Shinkai, M., Honda, H., Wakabayashi, T., Yoshida, J., and Kobayashi, T. (1998). Intracellular hyperthermia for cancer using magnetite cationic liposomes: An in vivo study. Jpn. J. Cancer Res. 89, 463–470. doi:10.1111/j.1349-7006.1998.tb00586.x
Yang, N., WeiHong, L., and Hao, L. (2014). Biosynthesis of Au nanoparticles using agricultural waste mango peel extract and its in vitro cytotoxic effect on two normal cells. Mat. Lett. 134, 67–70. doi:10.1016/J.MATLET.2014.07.025
Keywords: biosynthesis, metallic nanostructure, plant waste, nanoparticle, biomass
Citation: Poh Yan L, Gopinath SCB, Subramaniam S, Chen Y, Velusamy P, Chinni SV, Gobinath R and Lebaka VR (2022) Greener synthesis of nanostructured iron oxide for medical and sustainable agro-environmental benefits. Front. Chem. 10:984218. doi: 10.3389/fchem.2022.984218
Received: 01 July 2022; Accepted: 23 August 2022;
Published: 20 September 2022.
Edited by:
Janardhan Reddy Koduru, Kwangwoon University, South KoreaReviewed by:
Mithun Kumar Ghosh, Govt College Hatta, IndiaRama Rao Karri, University of Technology Brunei, Brunei
Copyright © 2022 Poh Yan, Gopinath, Subramaniam, Chen, Velusamy, Chinni, Gobinath and Lebaka. This is an open-access article distributed under the terms of the Creative Commons Attribution License (CC BY). The use, distribution or reproduction in other forums is permitted, provided the original author(s) and the copyright owner(s) are credited and that the original publication in this journal is cited, in accordance with accepted academic practice. No use, distribution or reproduction is permitted which does not comply with these terms.
*Correspondence: Subash C. B. Gopinath, c3ViYXNoQHVuaW1hcC5lZHUubXk=