- 1School of Chemical and Environmental Engineering, Shanghai Institute of Technology, Shanghai, China
- 2School of Chemistry and Chemical Engineering, Nantong University, Nantong, China
- 3Department of Hepatobiliary and Pancreatic Surgery, Affiliated Hospital of Nantong University, Nantong, China
Photoluminescence materials have been widely applied in biological imaging and sensing, anti-counterfeiting, light-emitting diodes, logic gates et al. The fabrication of luminescent materials with adjustable emission color by self-assembly of π-conjugated molecules has attracted particular attention. In this study, we designed and synthesized a thiophene-based α-cyanostyrene-derivative (TPPA), then investigate its self-assembly morphology and fluorescence emission under different organic solvents, different proportions of H2O/THF (DMSO) mixture and different pH conditions by UV, FL and SEM images. It was found that TPPA formed nanoparticles by self-assembly in organic solvent (THF or DMSO), accompanied by strong fluorescence emission. However, with the increase of water ratio, the fluorescence intensity decreased accompany with red shift, and the self-assembly morphology changed from nanoparticles to fibers. More interestingly, when pillar[5]arene (P5) was added to form host-guest complex with TPPA, white light emission could be successfully constructed when the ratio of TPPA to P5 was 1:20 and THF to water was 19:1.
Introduction
In recent years, highly efficient photoluminescent materials with tunable multicolor luminescence properties have been widely applied in biological imaging and sensing (Yang et al., 2013; Guo et al., 2020a; Zhao et al., 2021; Wang et al., 2022a), anti-counterfeiting (Yu et al., 2020; Yang et al., 2021), light-emitting diodes (Fung et al., 2016), molecular switches and logic gates (Erbas-Cakmak et al., 2018). At present, the main strategy for the construction of luminescent materials is physical mixing or covalently linking complementary chromophores to achieve appropriate color mixing balance (Park et al., 2009; Peng et al., 2021). Among all kinds of luminescent materials, white luminescent materials are particularly valued because they are key components in various display and lighting applications (Aizawa et al., 2014; Sun et al., 2018). Compared with physical mixing and covalent bonding, the luminescent materials based on self-assembly have the advantages of modularization, simple synthesis and adjustable properties, so they have a broad application prospect (Wang et al., 2019; Li L. et al., 2020; Zhang Y. et al., 2020; Cai et al., 2020; Liu M. et al., 2021). By applying a variety of external stimuli, such as solvent polarity, light exposure, mechanical/thermal stimulation and humidity, the emitted colors can be effectively regulated, which also provides an environmentally friendly method for organic modules to prepare luminescent materials in aqueous media (Zhu et al., 2013; Wei et al., 2016; Cheng et al., 2017; Fang et al., 2017). Since the modulation of π-conjugated dyes in terms of their optical properties depends largely on the way the molecules are arranged, the effective manipulation of their emission can be achieved by adjusting the morphology of the photoluminescent proto-components, which is also critical in preparing valuable materials (Ostroverkhova 2016; Kundu et al., 2021; Lu et al., 2021). Thiophene-based α-cyanostilbene derivatives are a typical class of π-conjugated molecules (Yun et al., 2012; Martínez-Abadía et al., 2018). In addition to their interesting electrical properties, these compounds also have significant optical properties, so they are considered to be a very suitable and general choice for the development of functional materials (Bhaumik and Banerjee., 2020; Wang X.-H. et al., 2021; Li et al., 2021).
Pillar[n]arenes (Ogoshi et al., 2008; Xiao et al., 2018; Duan et al., 2020; Wang et al., 2020; Wang et al., 2022b) are the fifth generation of macrocyclic hosts following crown ethers (An et al., 2021), cyclodextrins (Zhou et al., 2021), calixarenes (An et al., 2019; Guo et al., 2020b) and cucurbiturils (Yan et al., 2021). They are oligo-cyclic compounds obtained by methylene bridged p-methoxylbenzene (Zhang et al., 2019; Lou and Yang, 2021). Various functional groups can be easily modified to pillar[n]arenes by reacting with phenolic hydroxyl groups along the upper and lower edges of the pillar[n]arene-frameworks (Zhang R. et al., 2020; Guo et al., 2021; Schmidt and Esser, 2021). In addition, the adjustable cavity size of pillar[n]arenes also endow them with rich host-guest properties (Wang M. et al., 2021; Cai et al., 2021; Wang Y. et al., 2022), such as alkyl chain guests trend to complex with pillar[5]arene, while pyridinium guests trend to complex with pillar[6]arene (Guo et al., 2020c; Shen et al., 2020; Liu D. et al., 2021). In recent years, pillar[n]arenes have developed rapidly from synthesis (Ma et al., 2019), host-guest interaction (Li B. et al., 2020; Huang et al., 2020) to functional derivation (Wu et al., 2018), and have been successfully applied to gas separation, ion detection, drug release, tumor therapy, optical materials et al. (Cen et al., 2020; Liu X. et al., 2021; Xiao et al., 2022). In particular, the pillar[n]arene-based photoluminescent materials are of particular interest because of their multiple stimulus responsiveness and controllable optical properties (Sun et al., 2021). For example, Yang and co-workers fabricated a non-metallic white light-emitting fluorescent material based on pillar[5]arene-tripoxamide system, and found that supramolecular assembly plays a key role in the process of white light-emitting (Yang et al., 2020).
In this study, we constructed a new white light emission material based on the co-assembly of pillar[5]arene and thiophene-based α-cyanostyrene-derivative (TPPA). TPPA was observed to form nanoparticles when self-assembled in organic solvent (THF or DMSO), accompanied with bright fluorescence emission. However, with the increase of water ratio, the fluorescence intensity decreased sharply accompany with red shift, and the morphology of the assemblies changed from nanoparticles to nanofibers (Scheme 1). Importantly, when pillar[5]arene (P5) was added to form host-guest complex with TPPA, white light emission could be successfully constructed in THF/H2O mixture (Scheme 1).
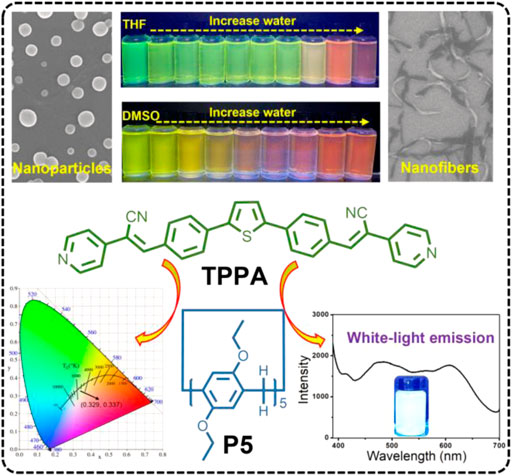
SCHEME 1. Chemical Structures of thiophene-based α-cyanostyrene-derivative (TPPA), and pillar[5]arene (P5) and Cartoon Representation of TPPA self-assembly in various solvents and further construction of pillar`[5]arene-based host-guest white-light emission system.
Experiment section
Synthesis of thiophene-based α-cyanostyrene-derivative (TPPA)
TBA (0.34 mmol), 4-pyridylacetonitrile (0.68 mmol) and piperidine (1.36 mmol) were dissolved in CH3CH2OH and stirred inside a Schlenk tube. The reaction mixture was heated to 85°C overnight under continuous stirring. Then, the obtained orange precipitate was washed several times with ethanol and hexane through centrifugation. An orange-colored solid was obtained with a 48% yield (Scheme 2).
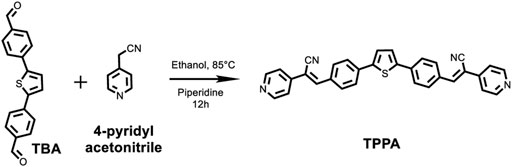
SCHEME 2. Synthetic route to (2Z,2′Z)-3,3′-(thiophene-2,5-diylbis (4,1-phenylene))-bis(2-(pyridin-4-yl)acrylonitrile) (TPPA).
TPPA: orange-colored solid, yield 48%. 1H NMR (Supplementary Figure S1) (400 MHz, Chloroform-d) δ 8.73–8.70 (m, 4H), 8.01 (d, J = 8.2 Hz, 4H), 7.78 (d, J = 8.1 Hz, 4H), 7.73 (s, 2H), 7.60 (d, 4H), 7.49 (s, 2H). 13C NMR (Supplementary Figure S2) (101 MHz, CDCl3) δ 150.54, 144.19, 143.66, 141.95, 136.97, 130.66, 126.00, 119.98, 117.06, 108.65. MS (ESI) (Supplementary Figure S3) Calcd. for C32H20N4SNa ([M + Na]+): 515.1, found: 515.1.
Synthesis of pillar[5]arene (P5)
Pillar[5]arene was prepared according previous report, in a typical process (Scheme 3), 1,4-diethoxybenzene (1.66 g, 1.0 mmol) and paraformaldehyde (0.30 g) were added to 60 ml ClCH2CH2Cl under vigorous stirring at room temperature. 1 ml BF3(Et2O) was added to the mixture and then reacted for 1 h. 50 ml H2O was added to stop the reaction, and pillar[5]arene was obtained by column chromatography (volume ratio: dichloromethane: petroleum ether = 1 : 1). White solid, 80%; 1H NMR (Supplementary Figure S4) (400 MHz, CDCl3) δ 6.72 (s, 10H, ArH), 3.83 (20H, OCH2-), 3.76 (s, 10H, ph-CH2-ph), 1.26 (t, J = 6.00 Hz, 30H, CH3).
Materials and methods
The TBA was prepared according to previous report. 4-pyridylacetonitrile, 1,4-diethoxybenzene and the reagents (ethanol, piperidine, ClCH2CH2Cl and so on) were commercially available (99%) and used as received. Further purification and drying of the solvents by standard methods were employed and distilled prior to use when necessary.
1H NMR and 13C NMR spectra were recorded on a Bruker AVIII-400 MHz spectrometer. All NMR used tetramethylsilane (TMS) as the internal standard. Bruker Micro-TOF spectrometer was used to investigate the High-resolution Mass (ESI) of the compounds. Fluorescence spectra were recorded on a Hitachi F-7000FluorescenceSpectrophotometer. Confocal images were acquired using an Olympus FLUOVIEWFV1000confocallaser scanning unit mounted on an IX81 fixed stage upright microscope. Scanning electron microscopy (SEM) investigations were carried out on a JEOL6390LVinstrument.
Results and disscussion
Impact of solvents
The obtained TPPA can be dissolved in most organic solvents and the corresponding solutions are stable as no precipitations were observed overnight. As shown in Supplementary Figure S5A, all the solution of TPPA displayed the characteristic band at about 420 nm with the similar intensity. Fluorescence emission spectra of TPPA showed a characteristic band at about 530 nm (Supplementary Figure S5B), and the intensity in large polarity solvent (CH3CN) is much lower than in smaller polarity solvent (Toluene). We further investigated the optical properties of TPPA in water/THF binary mixture, and the water content in the system gradually increased from 0% to 90%. A blue-shift was observed in UV-vis spectra with the increase of water content, and a dramatical change was found when the water content reached 80% (Figure 1A). On the other hand, a red-shift was found in fluorescence spectrum with the increase of water content. What’s more, the intensity of the peak decreased, and the fluorescence quantum yield decreased from 9.5% to 2.5% when the water increased from 0% to 90%, which indicated the formation of aggregates (Supplementary Table S1).
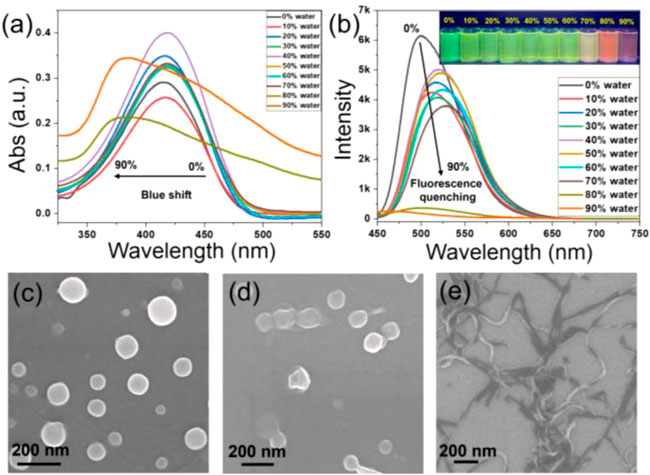
FIGURE 1. (A) absorption and (B) emission spectra of TPPA (0.4 mM, 4 ml) in 10 ml THF/water mixture with varying the water fraction from 0 to 90% with 10% of interval. (C) 0%, (D) 70%, and (E) 90% water content illustrating aggregation of spherical nanoparticles (0% W), semi-spherical oblong-shaped nanoparticles (70% W) to 1D-nanofibers (90% W).
The morphology of the TPPA in THF/H2O mixture was observed through SEM. SEM images clearly show that with the water fraction increased from 0% to 70% and finally to 90%, the morphology of the assemblies gradually changes from nanoparticles to oblong-shaped nanoparticles and finally to nanofibers. This indicates that water fraction plays a decisive role in the transformation of aggregate morphology. We further studied the fluorescence changes of TPPA in DMSO/H2O mixture to reveal the nature of fluorescence. Both UV and fluorescence spectra showed abrupt spectral when the water fraction reaches 40% (Supplementary Figure S6). At the same time, the aggregation curve of TPPA in DMSO/H2O also confirmed that TPPA aggregated when the water content is between 30% and 40%, which is significantly lower than the water content when TPPA aggregated in H2O/THF mixture, because the polarity of DMSO is greater than THF, indicating that the polarity of solvent plays a key role in the assembly behavior of TPPA.
Role of pH
The molecular structure, along with the polarity of the medium, plays a significant role in the formation of anisotropic nano-assembly. Because the pyridine N in TPPA can combine with H ion to change the polarity of the molecule, further affecting the optical properties and assembly behavior of the molecule in solution. The UV-vis spectra showed that the characteristic absorption peak have a significant red shift as pH decreased from 7 to 1, and the positions of the absorption peaks change dramatically when pH is between 3 and 4 (Figure 2A). Fluorescence spectra showed that the peak at 520 nm gradually decreased with the decrease of pH, but the peak at 600 nm gradually increased, indicating the formation of a new assembly morphology (Figure 2B). Furthermore, SEM images revealed a gradual disaggregation of the pristine nanofibers to nanoparticles with pH due to the protonation of the pyridinic nitrogen center upon the addition of acid (Figures 2C–H).
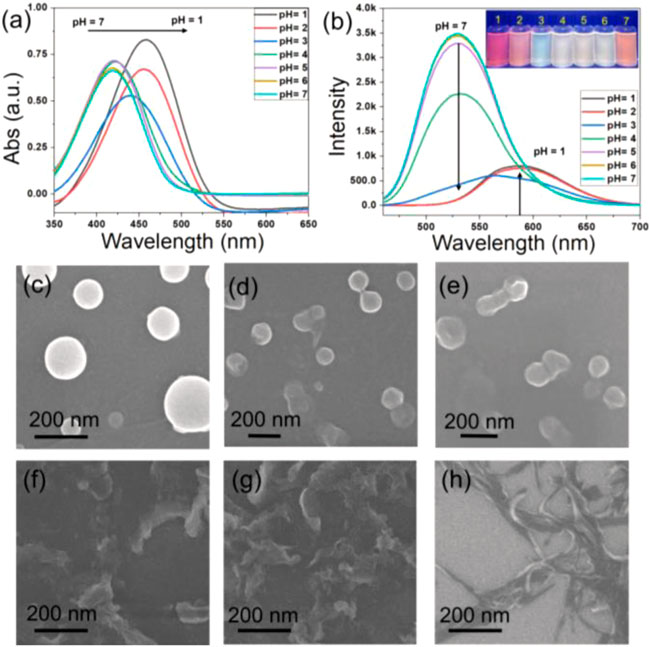
FIGURE 2. (A) absorption and (B) emission spectra of TPPA (0.4 mM, 4 ml) in 10 ml THF/water mixture (THF/H2O = 2:8) with different pH. SEM images of TPPA self-assembly in THF/water mixture (THF/H2O = 2:8) with different pH (C) pH = 1, (D) pH = 2, (E) pH = 3, (F) pH = 5, (G) pH = 6, (H) pH = 7.
Living cell imaging
Due to TPPA can self-assembly into fluorescent nanostructures, we wondered whether they could be applied in the field of biomedical detection. At first, the toxicity of TPPA to Hela cells was evaluated by 3-(4,5-dimethylthiazol-2-yl)-2,5-diphenyltetrazolium bromide (MTT) assay. After co-culture of Hela cells with TPPA at concentrations ranging from 5.0 to 80 μg/ML for 4 h, the viability of HeLa cells was basically unchanged, indicating that the TPPA-based nanostructure has good cellular compatibility and very low cytotoxicity. Subsequently, we used TPPA as a cell imaging reagent. After HeLa and HepG2 cells were treated with TPPA for 4 h, the distribution of TPPA in Hela and HepG2 cells was monitored by confocal laser scanning microscopy (CLSM). As shown in Figure 3, both TPPA-treated HeLa and HepG2 cells showed bright red fluorescence in the lysosomal of the cells, indicating that TPPA can be successfully used for live cell imaging.
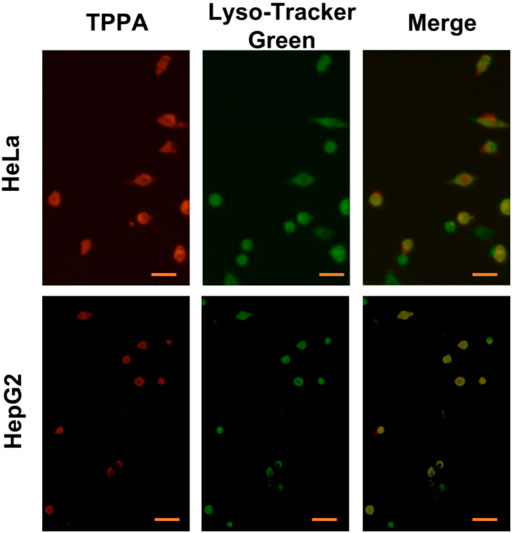
FIGURE 3. Confocal images of live HeLa and HepG2 cells after incubation with TPPA (5.00 × 10−4 M) for 4 h. Scale bar is 50 μm.
White-light emission
Over the past decade, white light emitting materials have attracted much attention due to their potential applications in display technology and fluorescence sensors. In this work, a simple and efficient way for constructing white light-emitting material through the assembly between methoxyl pillar[5]arene (P5) and TPPA. From 2D NMR (Supplementary Figure S9) and IR (Supplementary Figure S10) spectra, we found P5 could provide C−H···π acting force and rich electronic cavity while the pyridine groups of the TPPA serve as electron-deficient sites. First, we fixed the amount of TPPA (0.1 μmol) in H2O/THF mixture and gradually increased P5. We find that with the increase of P5, the peak at 500 nm decreases and the peak at 650 nm increases, while when P5/TPPA is greater than 20, the peak at 650 nm decreases and the peak at 600 nm increases, indicating that the system may present white emission when P5/TPPA is around 20 (Supplementary Figure S8). We then fixed P5/TPPA at 20:1 and changed the ratio of THF to water in the mixture. As shown in Figure 4A, the peak intensity decreased with the increase of water content from 30% to 80%, while the peak intensity increased when the water content larger than 90%. As shown in Figure 4B, the system 0.1 μmol TPPA and 2.0 μmol P5 in THF/H2O mixture (5% THF and 95% water) was perceived as white light emitting with color coordinates of (0.329, 0.337), and the fluorescence quantum yield was 2.05 ± 0.06. The coordinate is very close to the pure white point (0.333, 0.333). In this case, the luminescence covers the entire visible spectral region (400–700 nm), giving our system overall white light emission (Figure 4B). It should be pointed that the morphology of TPPA was transformed from nanofibers to microparticles after addition of P5 (Supplementary Figure S11), and these particles can also be used for living cell imaging (Supplementary Figure S12).
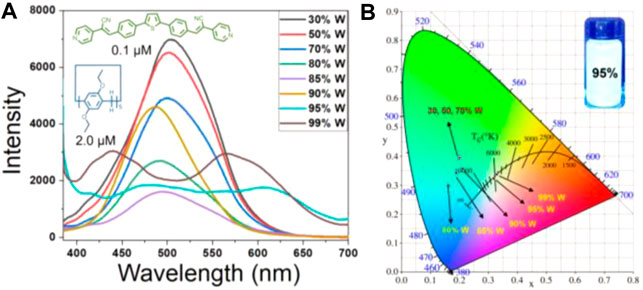
FIGURE 4. (A) Fluorescence spectra of 0.1 μmol TPPA and 2.0 μmol P5 in different ratio of THF/H2O mixture. (B) CIE chromaticity coordinates of TPPA&P5 according to the spectra recorded in (A). inset: luminescence image of TPPA&P5 under 365 nm UV light.
Conclusion
In this paper, a new π-conjugated molecule thiophene-based α-cyanostyrene-derivative (TPPA) was designed and synthesized successfully. TPPA showed bright fluorescence when dissolving in different organic solvents, and the fluorescence intensity increased with the decrease of the solvent polarity. Further investigation of TPPA in THF(DMSO)/H2O mixture found that TPPA formed nanoparticles by self-assembly in organic solvent (THF or DMSO), accompanied by strong fluorescence emission. However, with the increase of water ratio, the fluorescence intensity decreased accompany with red shift, and the self-assembly morphology changed from nanoparticles to fibers. Importantly, when macrocyclic host pillar[5]arene (P5) was added to form host-guest complex with TPPA, white light emission could be successfully constructed. This work provided a useful strategy for construction of photoluminescent materials based on supramolecular self-assembly.
Data availability statement
The original contributions presented in the study are included in the article/Supplementary Material, further inquiries can be directed to the corresponding authors.
Author contributions
Single authors contributed to the present papers as follows: HZ prepared all the compounds; YW, SZ, and LL analyzed the data. YW and LL wrote the paper.
Funding
This work was supported by the National Natural Science Foundation of China (22007052), the Natural Science Foundation of Jiangsu Province (BK20190917), Science and Technology Project of Nantong City (JC2020055), China Postdoctoral Science Foundation (2020M680071).
Conflict of interest
The authors declare that the research was conducted in the absence of any commercial or financial relationships that could be construed as a potential conflict of interest.
Publisher’s note
All claims expressed in this article are solely those of the authors and do not necessarily represent those of their affiliated organizations, or those of the publisher, the editors and the reviewers. Any product that may be evaluated in this article, or claim that may be made by its manufacturer, is not guaranteed or endorsed by the publisher.
Supplementary material
The Supplementary Material for this article can be found online at: https://www.frontiersin.org/articles/10.3389/fchem.2022.980173/full#supplementary-material.
References
Aizawa, N., Pu, Y.-J., Watanabe, M., Chi, T., Ideta, K., Toyota, N., et al. (2014). Solution-processed multilayer small-molecule light-emitting devices with high-efficiency white-light emission. Nat. Commun. 5, 5756. doi:10.1038/ncomms6756
An, L., Wang, C., Han, L., Liu, J., Huang, T., Zheng, Y., et al. (2019). Structural Design, synthesis, and preliminary biological evaluation of novel dihomooxacalix[4]arene-based anti-tumor agents. Front. Chem. 7, 856. doi:10.3389/fchem.2019.00856
An, S., Xu, Q., Ni, Z., Hu, J., Peng, C., Zhai, L., et al. (2021). Construction of covalent organic frameworks with crown ether struts. Angew. Chem. Int. Ed. 60, 9959–9963. doi:10.1002/anie.202101163
Bhaumik, S. K., and Banerjee, S. (2020). Tunable multi-color luminescence from a self-assembled cyanostilbene and cucurbit[7]uril in aqueous media. Chem. Commun. 56, 655–658. doi:10.1039/c9cc09277c
Cai, Y., Wang, Y., Wang, C., Long, R., Cao, L., Chen, Y., et al. (2020). Hierarchical self-assembly of 3D amphiphilic discrete organoplatinum(II) metallacage in water. Chin. Chem. Lett. 31, 689–692. doi:10.1016/j.cclet.2019.08.036
Cai, Y., Yan, X., Wang, S., Zhu, Z., Cen, M., Ou, C., et al. (2021). Pillar[5]arene-Based 3D hybrid supramolecular polymer for green catalysis in water. Inorg. Chem. 60, 2883–2887. doi:10.1021/acs.inorgchem.0c03645
Cen, M., Ding, Y., Wang, J., Yuan, X., Lu, B., Wang, Y., et al. (2020). Cationic water-soluble pillar[5]arene-modified Cu2–xSe nanoparticles: Supramolecular trap for ATP and application in targeted photothermal therapy in the NIR-II window. ACS Macro Lett. 9, 1558–1562. doi:10.1021/acsmacrolett.0c00714
Cheng, Y., Wang, J., Qiu, Z., Zheng, X., Leung, N. L. C., Lam, L. W. Y., et al. (2017). Multiscale humidity visualization by environmentally sensitive fluorescent molecular rotors. Adv. Mat. 29, 1703900. doi:10.1002/adma.201703900
Duan, Q., Wang, F., Zhang, H., and Lu, K. (2020). pH-responsive host-guest complexations between a water-soluble pillar[6]arene dodecyl-ammonium chloride and aromatic sulfonic acids. Front. Chem. 8, 588201. doi:10.3389/fchem.2020.588201
Erbas-Cakmak, S., Kolemen, S., Sedgwick, A. C., Gunnlaugsson, T., James, T. D., Yoon, J., et al. (2018). Molecular logic gates: The past, present and future. Chem. Soc. Rev. 47, 2228–2248. doi:10.1039/C7CS00491E
Fang, Q., Li, J., Li, S., Duan, R., Wang, S., Yi, Y., et al. (2017). Thermally populated “bright” states for wide-range and high temperature sensing in air. Chem. Commun. 53, 5702–5705. doi:10.1039/C7CC00551B
Fung, M.-K., Li, Y.-Q., and Liao, L.-S. (2016). Tandem organic light-emitting diodes. Adv. Mat. 28, 10381–10408. doi:10.1002/adma.201601737
Guo, H., Yan, X., Lu, B., Wang, J., Yuan, X., Han, Y., et al. (2020a). Pillar[5]arene-based supramolecular assemblies with two-step sequential fluorescence enhancement for mitochondria-targeted cell imaging. J. Mat. Chem. C 8, 15622–15625. doi:10.1039/D0TC04343E
Guo, H., Ye, J., Zhang, Z., Wang, Y., Yuan, X., Ou, C., et al. (2020c). Pillar[5]arene-Based [2]Rotaxane: Synthesis, characterization, and application in a coupling reaction. Inorg. Chem. 59, 11915–11919. doi:10.1021/acs.inorgchem.0c01752
Guo, H., Zhang, R., Han, Y., Wang, J., and Yan, C. (2020b). A p-tert-Tutyldihomooxacalix[4]arene based soft gel for sustained drug release in water.. Front. Chem. 8, 33. doi:10.3389/fchem.2020.00033
Guo, S., Huang, Q., Chen, Y., Wei, J., Zheng, J., Wang, L., et al. (2021). Synthesis and bioactivity of guanidinium-functionalized pillar[5]arene as a biofilm disruptor. Angew. Chem. Int. Ed. 60, 618–623. doi:10.1002/anie.202013975
Huang, B., Wang, P., Ouyang, Y., Pang, S., Liu, S., Hong, C., et al. (2020). Pillar[5]arene-Based switched supramolecular photosensitizer for self-amplified and pH-activated photodynamic therapy. ACS Appl. Mat. Interfaces 12, 41038–41046. doi:10.1021/acsami.0c10372
Kundu, S., Chowdhury, A., Nandi, S., Bhattacharyya, K., and Patra, A. (2021). Deciphering the evolution of supramolecular nanofibers in solution and solid-state: A combined microscopic and spectroscopic approach. Chem. Sci. 12, 5874–5882. doi:10.1039/d0sc07050e
Li, B., Cui, L., and Li, C. (2020b). Macrocycle Co-crystals showing vapochromism to haloalkanes. Angew. Chem. Int. Ed. 59, 22012–22016. doi:10.1002/anie.202010802
Li, J., Tao, L., Wang, Y., Yao, Y., and Guo, Q. (2021). Heptazine-based π-conjugated materials for light-emitting. Front. Chem. 9, 717569. doi:10.3389/fchem.2021.717569
Li, L., Tuo, W., Zhu, Q., Sepehrpour, H., Yao, Y., Yan, C., et al. (2020a). Resorcinarene induced assembly of carotene and lutein into hierarchical superstructures. J. Am. Chem. Soc. 142, 20583–20587. doi:10.1021/jacs.0c10901
Liu, D., Du, J., Qi, S., Li, M., Wang, J., Liu, M., et al. (2021b). Supramolecular nanoparticles constructed from pillar[5]arene-based host–guest complexation with enhanced aggregation-induced emission for imaging-guided drug delivery. Mat. Chem. Front. 5, 1418–1427. doi:10.1039/D0QM00974A
Liu, M., Creemer, C. N., Reardon, T. J., and Parquette, J. R. (2021a). Light-driven dissipative self-assembly of a peptide hydrogel. Chem. Commun. 57, 13776–13779. doi:10.1039/D1CC04971B
Liu, X., Liu, J., Meng, C., Zhu, P., Liu, X., Qian, J., et al. (2021c). Pillar[6]arene-Based supramolecular nanocatalysts for synergistically enhanced chemodynamic therapy by the intracellular cascade reaction. ACS Appl. Mat. Interfaces 13, 53574–53585. doi:10.1021/acsami.1c15203
Lou, X.-Y., and Yang, Y.-W. (2021). Pyridine-conjugated pillar[5]arene: From molecular crystals of blue luminescence to red-emissive coordination nanocrystals. J. Am. Chem. Soc. 143, 11976–11981. doi:10.1021/jacs.1c07006
Lu, B., Zhang, Z., Jin, D., Yuan, X., Wang, J., Ding, Y., et al. (2021). A–DA’D–A fused-ring small molecule-based nanoparticles for combined photothermal and photodynamic therapy of cancer. Chem. Commun. 57, 12020–12023. doi:10.1039/D1CC04629B
Ma, X.-Q., Wang, Y., Wei, T.-B., Qi, L.-H., Jiang, X.-M., Ding, J. D., et al. (2019). A novel AIE chemosensor based on quinoline functionalized Pillar[5]arene for highly selective and sensitive sequential detection of toxic Hg2+ and CN−. Dyes Pigm. 164, 279–286. doi:10.1016/j.dyepig.2019.01.049
Martínez-Abadía, M., Giménez, R., and Ros, M. B. (2018). Self-assembled α-cyanostilbenes for advanced functional materials. Adv. Mat. 30, 1704161. doi:10.1002/adma.201704161
Ogoshi, T., Kanai, S., Fujinami, S., Yamagishi, T.-a., and Nakamoto, Y. (2008). para-Bridged symmetrical pillar[5]arenes: Their lewis acid catalyzed synthesis and host–guest property. J. Am. Chem. Soc. 130, 5022–5023. doi:10.1021/ja711260m
Ostroverkhova, O. (2016). Organic optoelectronic materials: Mechanisms and applications. Chem. Rev. 116, 13279–13412. doi:10.1021/acs.chemrev.6b00127
Park, S., Kwon, J. K., Kim, S. H., Seo, J., Chung, K., Park, S.-Y., et al. (2009). A white-light-emitting molecule: Frustrated energy transfer between constituent emitting centers. J. Am. Chem. Soc. 131, 14043–14049. doi:10.1021/ja902533f
Peng, C., Liang, W., Ji, J., Fan, C., Kanagaraj, K., Wu, W., et al. (2021). Pyrene-tiaraed pillar[5]arene: Strong intramolecular excimer emission applicable for photo-writing. Chin. Chem. Lett. 32, 345–348. doi:10.1016/j.cclet.2020.03.079
Schmidt, M., and Esser, B. (2021). Cavity-promotion by pillar[5]arenes expedites organic photoredox-catalysed reductive dehalogenations. Chem. Commun. 57, 9582–9585. doi:10.1039/D1CC03221F
Shen, L., Zhao, Y., Dai, D., Yang, Y.-W., Wu, B., and Yang, X.-J. (2020). Stabilization of Grignard reagents by a pillar[5]arene host–Schlenk equilibria and Grignard reactions. Chem. Commun. 56, 1381–1384. doi:10.1039/C9CC08728A
Sun, S., Geng, M., Huang, L., Chen, Y., Cen, M., Lu, D., et al. (2018). A new amphiphilic pillar[5]arene: Synthesis and controllable self-assembly in water and application in white-light-emitting systems. Chem. Commun. 54, 13006–13009. doi:10.1039/C8CC07658H
Sun, X.-W., Wang, Z.-H., Li, Y.-J., Zhang, Y.-F., Zhang, Y.-M., Yao, H., et al. (2021). Tri-pillar[5]arene-Based multifunctional stimuli-responsive supramolecular polymer network with conductivity, aggregation induced emission, thermochromism, fluorescence sensing, and separation properties. Macromolecules 54, 373–383. doi:10.1021/acs.macromol.0c01972
Wang, J., Cen, M., Wang, J., Wang, D., Ding, Y., Zhu, G., et al. (2022a). Water-soluble pillar[4]arene[1]quinone: Synthesis, host-guest property and application in the fluorescence turn-on sensing of ethylenediamine in aqueous solution, organic solvent and air. Chin. Chem. Lett. 33, 1475–1478. doi:10.1016/j.cclet.2021.08.044
Wang, J., Wang, D., Cen, M., Jing, D., Bei, J., Huang, Y., et al. (2022b). GOx-assisted synthesis of pillar[5]arene based supramolecular polymeric nanoparticles for targeted/synergistic chemo-chemodynamic cancer therapy. J. Nanobiotechnology 20, 33. doi:10.1186/s12951-021-01237-0
Wang, M., Li, Q., Li, E., Liu, J., Zhou, J., and Huang, F. (2021b). Vapochromic behaviors of A solid-state supramolecular polymer based on exo-wall complexation of perethylated pillar[5]arene with 1, 2, 4, 5-tetracyanobenzene. Angew. Chem. Int. Ed. 60, 8115–8120. doi:10.1002/anie.202013701
Wang, P., Wang, R., and Xia, D. (2020). pH-Induced transition between single-chain macrocyclic amphiphile and [c2]Daisy chain-based bola-type Amphiphile and the related self-assembly behavior in water. Front. Chem. 7, 894. doi:10.3389/fchem.2019.00894
Wang, X.-H., Lou, X.-Y., Lu, T., Wang, C., Tang, J., Liu, F., et al. (2021a). Supramolecular engineering of efficient artificial light-harvesting systems from cyanovinylene chromophores and pillar[5]arene based polymer hosts. ACS Appl. Mat. Interfaces 13, 4593–4604. doi:10.1021/acsami.0c21651
Wang, Y., Cai, Y., Cao, L., Cen, M., Chen, Y., Zhang, R., et al. (2019). An amphiphilic metallaclip with enhanced fluorescence emission in water: Synthesis and controllable self-assembly into multi-dimensional micro-structures. Chem. Commun. 55, 10132–10134. doi:10.1039/C9CC04809J
Wang, Y., Wang, D., Wang, J., Wang, C., Wang, J., Ding, Y., et al. (2022c). Pillar[5]arene-derived covalent organic materials with pre-encoded molecular recognition for targeted and synergistic cancer photo- and chemotherapy. Chem. Commun. 58, 1689–1692. doi:10.1039/D1CC07072J
Wei, C., Gao, M., Hu, F., Yao, J., and Zhao, Y. S. (2016). Excimer emission in self-assembled organic spherical microstructures: An effective approach to wavelength switchable microlasers. Adv. Opt. Mat. 4, 1009–1014. doi:10.1002/adom.201600048
Wu, G.-Y., Wang, X.-Q., Chen, L.-J., Hu, Y.-X., Yin, G.-Q., Xu, L., et al. (2018). Supramolecular polymer cross-linked by discrete tris-[2]pseudorotaxane metallacycles and its redox-responsive behavior. Inorg. Chem. 57, 15414–15420. doi:10.1021/acs.inorgchem.8b02712
Xiao, T., Qian, H., Shen, Y., Wei, C., Ren, D., Zhang, L., et al. (2022). A tunable artificial light-harvesting system based on host-guest interaction exhibiting ultrahigh antenna effect and narrowed emission band. Mat. Today Chem. 24, 100833. doi:10.1016/j.mtchem.2022.100833
Xiao, T., Xu, L., Zhong, W., Zhou, T., Sun, X.-Q., Hu, X.-Y., et al. (2018). Advanced functional materials constructed from pillar[n]arenes. Isr. J. Chem. 58, 1219–1229. doi:10.1002/ijch.201800026
Yan, K., Wang, L., Zhou, H., Hua, Z., Xu, P., Xu, H., et al. (2021). Cucurbituril-mediated AIE: An unconventional indicator displacement assay for ketamine detection. Dyes Pigm. 197, 109875. doi:10.1016/j.dyepig.2021.109875
Yang, H-L., Li, Z.-H., Liu, P.-P., Sun, X.-W., Wang, Z.-H., Yao, H., et al. (2020). Metal-free white light-emitting fluorescent material based on simple pillar[5]arene-tripodal amide system and theoretical insights on its assembly and fluorescent properties. Langmuir 36, 13469–13476. doi:10.1021/acs.langmuir.0c02120
Yang, H., Li, M., Zhao, W., Guo, Z., and Zhu, W.-H. (2021). Photoresponsive aggregation-induced emission polymer film for anti-counterfeiting. Chin. Chem. Lett. 32, 3882–3885. doi:10.1016/j.cclet.2021.05.053
Yang, S. K., Shi, X., Park, S., Ha, T., and Zimmerman, S. C. (2013). A dendritic single-molecule fluorescent probe that is monovalent, photostable and minimally blinking. Nat. Chem. 5, 692–697. doi:10.1038/nchem.1706
Yu, X., Wu, L., Yang, D., Cao, M., Fan, X., Lin, H., et al. (2020). Hydrochromic CsPbBr3 nanocrystals for anti-counterfeiting. Angew. Chem. Int. Ed. 59, 14527–14532. doi:10.1002/anie.202005120
Yun, S. W., Kim, J. H., Shin, S., Yang, H., An, B.-K., Yang, L., et al. (2012). High-performance n-type organic semiconductors: Incorporating specific electron-withdrawing motifs to achieve tight molecular stacking and optimized energy levels. Adv. Mat. 24, 911–915. doi:10.1002/adma.201103978
Zhang, R., Wang, C., Long, R., Chen, T., Yan, C., and Yao, Y. (2019). Pillar[5]arene based [1]rotaxane systems with redox-responsive host-guest property: Design, synthesis and the key role of chain Length. Front. Chem. 7, 508. doi:10.3389/fchem.2019.00508
Zhang, R., Yan, X., Guo, H., Hu, L., Yan, C., Wang, Y., et al. (2020b). Supramolecular polymer networks based on pillar[5]arene: Synthesis, characterization and application in the fenton reaction. Chem. Commun. 56, 948–951. doi:10.1039/C9CC09155F
Zhang, Y., Shi, L., Zhang, Z., Zhao, Q., Zhang, Y., and Yao, Y. (2020a). A discrete platinum(II) amphiphile: Construction, characterization, and controllable self-assembly in different solvents. Inorg. Chem. 59, 7924–7927. doi:10.1021/acs.inorgchem.0c01164
Zhao, W., Yu, X., Peng, S., Luo, Y., Li, J., and Lu, L. (2021). Construction of nanomaterials as contrast agents or probes for glioma imaging. J. Nanobiotechnology 19, 125. doi:10.1186/s12951-021-00866-9
Zhou, W.-L., Chen, Y., Lin, W., and Liu, Y. (2021). Luminescent lanthanide–macrocycle supramolecular assembly. Chem. Commun. 57, 11443–11456. doi:10.1039/D1CC04672A
Keywords: self-assembly, pillar[5]arene, thiophene, π-Conjugated molecule, white-light emission
Citation: Zhong H, Li L, Zhu S and Wang Y (2022) Controllable self-assembly of thiophene-based π-conjugated molecule and further construction of pillar[5]arene-based host-guest white-light emission system. Front. Chem. 10:980173. doi: 10.3389/fchem.2022.980173
Received: 28 June 2022; Accepted: 09 August 2022;
Published: 02 September 2022.
Edited by:
Ruibo Zhao, Imperial College London, United KingdomCopyright © 2022 Zhong, Li, Zhu and Wang. This is an open-access article distributed under the terms of the Creative Commons Attribution License (CC BY). The use, distribution or reproduction in other forums is permitted, provided the original author(s) and the copyright owner(s) are credited and that the original publication in this journal is cited, in accordance with accepted academic practice. No use, distribution or reproduction is permitted which does not comply with these terms.
*Correspondence: Liang Li, lilianglcx@sit.edu.cn; Shajun Zhu, thetranquilheart@sohu.com; Yang Wang, Ywang85@ntu.edu.cn