- 1Faculdade de Ciências e Tecnologia, Campus de Gambelas, Universidade do Algarve, Faro, Portugal
- 2Centro de Ciências do Mar (CCMar), Universidade do Algarve, Faro, Portugal
- 3Instituto de Nanociencia y Materiales de Aragón (INMA), Consejo Superior de Investigaciones Científicas-Universidad de Zaragoza, Zaragoza, Spain
- 4CIBER de Bioingeniería, Biomateriales y Nanomedicina, Instituto de Salud Carlos III, Madrid, Spain
- 5South China University of Technology, Guangzhou, China
Editorial on the Research Topic
Emerging polyoxometalates with biological, biomedical, and healthapplications
The biomedical application of metals, including platinum (Pt), lithium (Li), tungstate (W), gold (Au) or vanadium (V), among others, has become an important and a rapidly growing branch of science (Bertinat et al., 2018; Yeo et al., 2018; Vosahlikova et al., 2020; Ścibior et al., 2020; Silva et al., 2021; Pena et al., 2022; Ochoa, 2022). Besides the well-characterized platinum drugs, bio-active metal-based complexes and clusters, such as gold compounds and polyoxometalates (POMs), as well as metal-based nanoparticles have shown demonstrable anti-cancer, anti-viral and anti-bacterial activities (Bertinat et al., 2018; Yeo et al., 2018; Soria-Carrera et al., 2020; Vosahlikova et al., 2020; Ścibior et al., 2020; Silva et al., 2021; Aureliano et al., 2022a; Pena et al., 2022; Ochoa, 2022; Soria-Carrera et al., 2022). The biological and biomedical application of POMs–in the form of cluster ions, hybrid materials, and POM-based nanoparticles - has tripled in the last decade (Pimpão et al., 2020). In fact, the wide range of POMs uses in medicine may be due to the modulation of several proteins such as aquoporins and P-type ATPases (Gumerova et al., 2018; Fraqueza et al., 2019) although many other biomolecular and biochemical processes are affected by POMs, as illustrated by the well-studied polyoxovanadates (POVs) (Bijelic et al., 2018; Bijelic et al., 2019; Čolović et al., 2020; Aureliano et al., 2021; Aureliano et al., 2022b). POMs against bacteria and in cancer therapy and diagnostics, their modes of action, protein targets and future perspectives were recently reviewed and highlighted (Bijelic et al., 2018; Gumerova et al., 2018; Bijelic et al., 2019; Fraqueza et al., 2019; Čolović et al., 2020; Aureliano et al., 2021; Aureliano et al., 2022b). The majority of the biomedical studies have addressed how POMs affect cancer and bacterial cell growth, not to mention their antiviral activity (Bijelic et al., 2018; Fraqueza et al., 2019; Guedes et al., 2020; Čolović et al., 2020; Aureliano et al., 2021; Aureliano, 2022; Aureliano et al., 2022b), however, much remains to be understood concerning the biochemical mechanism of action of these compounds (Bijelic et al., 2018; Bijelic et al., 2019; Pimpão et al., 2020; Čolović et al., 2020; Aureliano et al., 2021; Aureliano et al., 2022b). The isopolyoxovanadate decavanadate [V10O28]6−, {V10}, is perhaps the most widely studied POM in biology, showing several roles in key biochemical and cellular processes (Crans et al., 2004; Aureliano, 2009; Aureliano and Crans, 2009; Aureliano et al., 2013; Bijelic et al., 2018; Bijelic et al., 2019; Čolović et al., 2020; Aureliano et al., 2021; Sciortino et al., 2021; Aureliano et al., 2022b). Particularly interesting under the topic of POMs speciation (Gumerova and Rompel, 2020), was the observation that {V10} binding to G-actin inhibits its polymerization to F-actin while it prevents {V10} decomposition (Ramos et al., 2006). The V10/G-actin interaction might interfere with cytoskeleton dynamics and inducing cell morphology changes (Ramos et al., 2006; Sciortino et al., 2021).
Taken in consideration all these effects and properties, it was clear that the future bio-based applications of POMs is bright (Aureliano, 2022). That was why the Guest Editors decided to pursue at Frontiers the promotion and the development of a research topic about “Emerging Polyoxometalates with Biological, Biomedical, and Health Applications” (Figure 1).
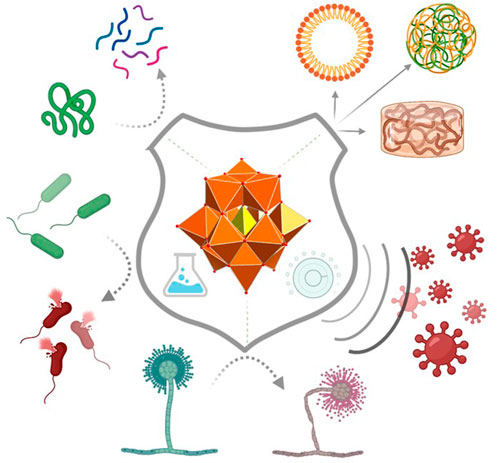
FIGURE 1. POMs are described with anticancer, antiviral, and antimicrobial activities as well as other biological or biomedical applications such as artificial protease activity (top-left) and self-assembly with biomacromolecules to make micelles, gels & other superstructures (top-right).
Thus, the present Research Topic (RT) aimed to highlight recent advances into in vitro and in vivo POMs, be they pure, hybrid, or POM-based nanoparticles with anticancer, antiviral, and antimicrobial activities as well as other biological or biomedical applications, such as in diabetes and neurological diseases (Treviño et al., 2019; Treviño and González-Vergara, 2019; Zhao et al., 2019; Atrián-Blasco et al., 2022). Moreover, studies on POMs as biosensors, the redox activity of POMs as sensors for biological factors or markers of specific illnesses were also welcome. Papers addressing biomolecular POMs targets; POMs as ion channels in lipid/cell membranes and transportation of POMs across cell membranes was also welcomed in this Research Topic. The RT had an excellent number of authors (27) that confirmed, immediately after invitation, their participation. However, among others several reasons, directly and/or indirectly COVID has had a major impact on the number of papers submitted, preventing to fulfill, at least in part, some objectives of the present RT.
The first paper published on the present RT Corona-Motolinia et al., by the research group of Professor Enrique González-Vergara from the Benemérita Universidad Autónoma de Puebla (Mexico) in collaboration with coworkers from the Universidad de Granada (Spain). Enrique González-Vergara is a well-known researcher in our scientific community for publishing, for example, several studies about the antidiabetic properties of {V10} compounds, particularly metformin-decavanadate (Sánchez-Lombardo et al., 2014; Treviño et al., 2016; Treviño et al., 2019; Treviño and González-Vergara, 2019). The hybrid metformin-decavanadate (Metf-V10) (Chatkon et al., 2013) has shown in vivo nontoxicological effects on liver and kidney, leading it to be considered as a better treatment for diabetes than metformin (Treviño et al., 2016). However, as suggested previously by Enrique González-Vergara and coworkers, Meft-V10 might be also a more effective treatment than metformin in cancer (Sánchez-Lara et al., 2018; Treviño et al., 2019). In fact, herein, the focus of the paper is the antineoplastic activity of another hybrid POVs containing {V10}. Among the findings, molecular docking studies with small RNA fragments support the hypothesis that decavanadate’s anticancer activity could be attributed to its interaction with small non-coding RNA molecules.
From the University of Aveiro (Portugal), a review about “Polyoxometalate Functionalized Sensors”, by Veríssimo et al., brings an interesting perspective about POM-based biosensing applications. The University of Aveiro has a tradition in the use of POMs for several different applications (Gamelas et al., 2012; Veríssimo et al., 2017; Veríssimo et al., 2018). In the present review, the authors emphasized that POMs could be used as sensors for detecting and determining molecules and biomolecules in different matrices, many of them with biochemical and clinical relevance, along with analytical figures of merit and main virtues and drawbacks of such devices. Special emphasis is given to the stability of POMs sensitive layers, detection limits, selectivity, and the pH working range.
The third paper of this RT by Long-Sheng Wang from the Hubei University of Technology, Wuhan (China) reports the antiviral activity of POMs. These authors are from a University with previous experience in the use of these derivative POMs against virus (Wei et al., 2020). Herein, it was referred that the covalent linkage between the iodobenzoyldiazenido components and POMs can enhance the molecular inhibitory efficiency of iodobenzohydrazides against coxsackievirus B3.
Finally, the RT is finished with a mini-review by Gil and Carbó from the Universidad de Zaragoza and Universitat Rovia i Virgili (Spain), respectively. The authors highlight the relevance of the combination of molecular modulations simulations with quantum mechanics/molecular mechanics methods and theoretical calculations on cluster models. These calculations are starting to shed light on the factors governing the activity and selectivity for the hydrolysis of peptide and phosphoester bonds catalyzed by POMs. The authors have previously experience on theoretical calculations in cluster models (Solé-Daura et al., 2020). Moreover, the phosphoester bond hydrolysis catalysed by molybdate anions as artificial phosphoesterases has been also studied computationally (Lanuza et al., 2021; Martins et al., 2021; Sánchez-González et al., 2021). POMs as artificial enzymes have been tested by the group of Parac-Vogt, using POMs as catalysts in the hydrolysis of peptide bonds (Absillis and Parac-Vogt, 2012). Further computational studies have focus on the characterization of the reaction mechanism and the rationalization of the observed selectivity (Jayasinghe-Arachchige et al., 2019; Ly et al., 2019). In sum, the authors of the present mini-review are confident that in the coming years the computational studies on the biological activity of POMs will be an emergent research topic.
Altogether, the present RT reflects emergent 21st century applications of POMs, namely anticancer, antidiabetic and antiviral activities besides the applications of POMs as sensors and the contribution of computational studies for the understanding the biological activities of POMs. Within this RT a total of 25 authors were involved, where the majority are young researchers, The future is bright for POMs!
Author contributions
All authors listed have made a substantial, direct, and intellectual contribution to the work and approved it for publication.
Acknowledgments
MA received Portuguese national funds from FCT—Foundation for Science and Technology through projects UIDB/04326/2020 and LA/P/0101/2020.
Conflict of interest
The authors declare that the research was conducted in the absence of any commercial or financial relationships that could be construed as a potential conflict of interest.
Publisher’s note
All claims expressed in this article are solely those of the authors and do not necessarily represent those of their affiliated organizations, or those of the publisher, the editors and the reviewers. Any product that may be evaluated in this article, or claim that may be made by its manufacturer, is not guaranteed or endorsed by the publisher.
References
Absillis, G., and Parac-Vogt, T. N. (2012). Peptide bond hydrolysis catalyzed by the wells-dawson Zr(α2-P2w17o61)2 polyoxometalate. Inorg. Chem. 51, 9902–9910. doi:10.1021/ic301364n
Atrián-Blasco, E., de Cremoux, L., Lin, X., Mitchell-Heggs, R., Sabater, L., Blanchard, S., et al. (2022). Keggin-type polyoxometalates as Cu(ii) chelators in the context of Alzheimer's disease. Chem. Commun. 58, 2367–2370. doi:10.1039/d1cc05792h
Aureliano, M., and Crans, D. C. (2009). Decavanadate (V10O286-) and oxovanadates: Oxometalates with many biological activities. J. Inorg. Biochem. 103 (4), 536–546. doi:10.1016/j.jinorgbio.2008.11.010
Aureliano, M. (2009). Decavanadate: a journey in a search of a role. Dalton Trans. 42, 9093–9100. doi:10.1039/b907581j
Aureliano, M., Fraqueza, G., and Ohlin, C. A. (2013). Ion pumps as biological targets for decavanadate. Dalton Trans. 42 (33), 11770. doi:10.1039/c3dt50462j
Aureliano, M., Gumerova, N. I., Sciortino, G., Garribba, E., McLauchlan, C. C., Rompel, A., et al. (2022). Polyoxidovanadates’ interactions with proteins: An overview. Coord. Chem. Rev. 454, 214344. doi:10.1016/j.ccr.2021.214344
Aureliano, M., Gumerova, N. I., Sciortino, G., Garribba, E., Rompel, A., Crans, D. C., et al. (2021). Polyoxovanadates with emerging biomedical activities. Coord. Chem. Rev. 447, 214143. doi:10.1016/j.ccr.2021.214143
Aureliano, M. (2022). The future is bright for polyoxometalates. Biochem. 2 (1), 8–26. doi:10.3390/biochem2010002
Aureliano, M., Marques-da-Silva, D., Serrano, A., Martins, J., Faleiro, L., Fonseca, C., et al. (2022b). “Chapter 7, Polyoxometalates with anticancer, antibacterial and antiviral activities,” in Polyoxometalates: Advances, Properties, and Applications. Editors L. R. Rubio, B. Artetxe, J. M. Gutiérrez-Zorrilla, and J. L. Vilas. 1nd Edn. (Singapore: Jenny Stanford Publishing).
Bertinat, R., Westermeier, F., Gatica, R., and Nualart, F. (2018). Sodium tungstate: Is it a safe option for a chronic disease setting, such as diabetes? J. Cell. Physiol. 234 (1), 51–60. doi:10.1002/jcp.26913
Bijelic, A., Aureliano, M., and Rompel, A. (2019). Polyoxometalates as potential next-generation metallodrugs in the combat against cancer. Angew. Chem. Int. Ed. 58 (10), 2980–2999. doi:10.1002/anie.201803868
Bijelic, A., Aureliano, M., and Rompel, A. (2018). The antibacterial activity of polyoxometalates: structures, antibiotic effects and future perspectives. Chem. Commun. 54 (10), 1153–1169. doi:10.1039/c7cc07549a
Chatkon, A., Chatterjee, P. B., Sedgwick, M. A., Haller, K. J., and Crans, D. C. (2013). Counterion affects interaction with interfaces: The antidiabetic drugs metformin and decavanadate. Eur. J. Inorg. Chem. 2013 (10–11), 1859–1868. doi:10.1002/ejic.201201345
Čolović, M. B., Lacković, M., Lalatović, J., Mougharbel, A. S., Kortz, U., Krstić, D. Z., et al. (2020). Polyoxometalates in biomedicine: Update and overview. Curr. Med. Chem. 27 (3), 362–379. doi:10.2174/0929867326666190827153532
Crans, D. C., Smee, J. J., Gaidamauskas, E., and Yang, L. (2004). The Chemistry and biochemistry of vanadium and the biological activities exerted by vanadium compounds. Chem. Rev. 104 (2), 849–902. doi:10.1021/cr020607t
Fraqueza, G., Fuentes, J., Krivosudský, L., Dutta, S., Mal, S. S., Roller, A., et al. (2019). Inhibition of Na+/K+- and Ca2+-ATPase activities by phosphotetradecavanadate. J. Inorg. Biochem. 197, 110700. doi:10.1016/j.jinorgbio.2019.110700
Gamelas, J. A. F., Evtyugina, M. G., Portugal, I., and Evtuguin, D. V. (2012). New polyoxometalate-functionalized cellulosic fibre/silica hybrids for environmental applications. RSC Adv. 2, 831–839. doi:10.1039/c1ra00371b
Guedes, G., Wang, S., Santos, H. A., and Sousa, F. L. (2020). Polyoxometalate composites in cancer therapy and diagnostics. Eur. J. Inorg. Chem. 2020 (22), 2121–2132. doi:10.1002/ejic.202000066
Gumerova, N., Krivosudský, L., Fraqueza, G., Breibeck, J., Al-Sayed, E., Tanuhadi, E., et al. (2018). The P-type ATPase inhibiting potential of polyoxotungstates. Metallomics 10 (2), 287–295. doi:10.1039/c7mt00279c
Gumerova, N. I., and Rompel, A. (2020). Polyoxometalates in solution: speciation under spotlight. Chem. Soc. Rev. 49 (21), 7568–7601. doi:10.1039/d0cs00392a
Jayasinghe-Arachchige, V. M., Hu, Q., Sharma, G., Paul, T. J., Lundberg, M., Quiñonero, D., et al. (2019). J. Comput. Chem. 40, 51–61.
Lanuza, J., Sánchez-González, A., Bandeira, N. A. G., Lopez, X., and Gil, A. (2021). Inorg. Chem. 60, 11177.
Ly, H. G. T., Mihaylov, T. T., Proost, P., Pierloot, K., Harvey, J. M., Parac−Vogt, T. N., et al. (2019). Chemical mimics of aspartate-directed proteases: Predictive and strictly specific hydrolysis of a globular protein at Asp−X sequence promoted by polyoxometalate complexes rationalized by a combined experimental and theoretical approach. Chem. Eur. J. 25, 14370–14381. doi:10.1002/chem.201902675
Martins, F. F., Sánchez-González, A., Lanuza, J., Miras, H. N., Lopez, X., Bandeira, N. A. G., et al. (2021). Cover feature: probing the catalytically active species in POM‐catalysed DNA‐model hydrolysis. Chem. Eur. J. 27, 8977.
Ochoa, E. L. M. (2022). Lithium as a neuroprotective agent for bipolar disorder: an overview. Cell. Mol. Neurobiol. 42 (1), 85–97. doi:10.1007/s10571-021-01129-9
Pena, J., Peñ, Q., Wang, A., Zaremba, O., Shi, Y., Scheeren, H. W., et al. (2022). Metallodrugs in cancer nanomedicine. Chem. Soc. Rev. 51, 2544–2582. doi:10.1039/d1cs00468a
Pimpão, C., da Silva, I. V., Mósca, A. F., Pinho, J. O., Gaspar, M. M., Gumerova, N. I., et al. (2020). The aquaporin-3-inhibiting potential of polyoxotungstates. Int. J. Mol. Sci. 21 (7), 2467. doi:10.3390/ijms21072467
Ramos, S., Manuel, M., Tiago, T., Duarte, R., Martins, J., Gutiérrez-Merino, C., et al. (2006). Decavanadate interactions with actin: Inhibition of G-actin polymerization and stabilization of decameric vanadate. J. Inorg. Biochem. 100 (11), 1734–1743. doi:10.1016/j.jinorgbio.2006.06.007
Sánchez-González, A., Bandeira, N. A. G., Ortiz de Luzuriaga, I., Martins, F. F., Elleuchi, S., Jarraya, K., et al. (2021). New insights on the interaction of phenanthroline based ligands and metal complexes and polyoxometalates with duplex DNA and G-quadruplexes. Molecules 26, 4737. doi:10.3390/molecules26164737
Sánchez-Lara, E., Treviño, S., Sánchez-Gaytán, B. L., Sánchez-Mora, E., Eugenia Castro, M., Meléndez-Bustamante, F. J., et al. (2018). Decavanadate salts of cytosine and metformin: a combined experimental-theoretical study of potential metallodrugs against diabetes and cancer. Front. Chem. 6, 402. doi:10.3389/fchem.2018.00402
Sánchez-Lombardo, I., Sánchez-Lara, E., Pérez-Benítez, A., Mendoza, Á., Bernès, S., González-Vergara, E., et al. (2014). Synthesis of metforminium(2+) decavanadates – crystal structures and solid-state characterization. Eur. J. Inorg. Chem. 2014 (27), 4581–4588. doi:10.1002/ejic.201402277
Ścibior, A., Ł, Pietrzyk, Plewa, Z., and Skiba, A. (2020). Vanadium: risks and possible benefits in the light of a comprehensive overview of its pharmacotoxicological mechanisms and multi-applications with a summary of further research trends. J. Trace Elem. Med. Biol. 61, 126508. doi:10.1016/j.jtemb.2020.126508
Sciortino, G., Aureliano, M., and Garribba, E. (2021). Rationalizing the decavanadate(V) and oxidovanadium(IV) binding to G-actin and the competition with decaniobate(V) and ATP. Inorg. Chem. 60 (1), 334–344. doi:10.1021/acs.inorgchem.0c02971
Silva, M. J. S. A., Gois, P. M. P., and Gasser, G. (2021). Unveiling the potential of transition metal complexes for medicine: Translational in situ activation of metal-based drugs from bench to in vivo applications. Chembiochem 22 (10), 1740–1742. doi:10.1002/cbic.202100015
Solé-Daura, A., Rodríguez-Fortea, A., Poblet, J. M., Robinson, D., Hirst, J. D., Carbó, J. J., et al. (2020). Origin of selectivity in protein hydrolysis by Zr(IV)-Containing metal oxides as artificial proteases. ACS Catal. 10, 13455–13467. doi:10.1021/acscatal.0c02848
Soria-Carrera, H., Atrián-Blasco, E., de la Fuente, J. M., Mitchell, S. G. S. G., and Martín-Rapún, R. (2022). Polyoxometalate–polypeptide nanoassemblies as peroxidase surrogates with antibiofilm properties. Nanoscale 14 (16), 5999–6006. doi:10.1039/d1nr08223j
Soria-Carrera, H., Franco-Castillo, I., Romero, P., Martín, S., de la Fuente, J. M., Mitchell, S. G., et al. (2020). On-POM ring-opening polymerisation of N-Carboxyanhydrides. Angew. Chem. Int. Ed. 60, 3449–3453.
Treviño, S., and González-Vergara, E. (2019). Metformin-decavanadate treatment ameliorates hyperglycemia and redox balance of the liver and muscle in a rat model of alloxan-induced diabetes. New J. Chem. 43 (45), 17850–17862. doi:10.1039/c9nj02460c
Treviño, S., Velázquez-Vázquez, D., Sánchez-Lara, E., Diaz-Fonseca, A., Flores-Hernandez, J. Á., Pérez-Benítez, A., et al. (2016). Metforminium decavanadate as a potential metallopharmaceutical drug for the treatment of diabetes mellitus. Oxid. Med. Cell. Longev. 2016, 1–14. doi:10.1155/2016/6058705
Treviño, S., Díaz, A., Sánchez-Lara, E., Sanchez-Gaytan, B. L., Perez-Aguilar, J. M., González-Vergara, E., et al. (2019). Vanadium in biological action: chemical, pharmacological aspects, and metabolic implications in diabetes mellitus. Biol. Trace Elem. Res. 188, 68–98. doi:10.1007/s12011-018-1540-6
Veríssimo, M. I. S., Gamelas, J. A. F., Evtuguin, D. V., and Gomes, M. T. S. R. (2017). Determination of 5-hydroxymethylfurfural in honey, using headspace-solid-phase microextraction coupled with a polyoxometalate- coated piezoelectric quartz crystal. Food Chem. 220, 420–426. doi:10.1016/j.foodchem.2016.09.204
Veríssimo, M. I. S., Gamelas, J. A. F., Simões, M. M. Q., Evtuguin, D. V., and Gomes, M. T. S. R. (2018). Quantifying acetaldehyde in cider using a Mn(III)-substituted polyoxotungstate coated acoustic wave sensor. Sensors Actuators B Chem. 255, 2608–2613. doi:10.1016/j.snb.2017.09.068
Vosahlikova, M., Roubalova, L., Cechova, K., Kaufman, J., Musil, S., Miksik, I., et al. (2020). Na+/K+-ATPase and lipid peroxidation in forebrain cortex and hippocampus of sleep-deprived rats treated with therapeutic lithium concentration for different periods of time. Prog. Neuropsychopharmacol. Biol. Psychiatry 102, 109953. doi:10.1016/j.pnpbp.2020.109953
Wei, Y., Wang, H., Xi, C., Li, N., Li, D., Yao, C., et al. (2020). Antiviral effects of novel 2-benzoxyl-phenylpyridine derivatives. Molecules 25, 1409. doi:10.3390/molecules25061409
Yeo, C. I., Ooi, K. K., and Tiekink, E. R. T. (2018). Gold-based medicine: a paradigm shift in anti-cancer therapy? Molecules 23 (6), 1410. doi:10.3390/molecules23061410
Keywords: polyoxometalates, antibacterial, antiviral, antidiabetic, biosensors, molecular modulations simulations
Citation: Aureliano M, Mitchell SG and Yin P (2022) Editorial: Emerging polyoxometalates with biological, biomedical, and health applications. Front. Chem. 10:977317. doi: 10.3389/fchem.2022.977317
Received: 24 June 2022; Accepted: 28 June 2022;
Published: 09 August 2022.
Edited and reviewed by:
Vadim G Kessler, Swedish University of Agricultural Sciences, SwedenCopyright © 2022 Aureliano, Mitchell and Yin. This is an open-access article distributed under the terms of the Creative Commons Attribution License (CC BY). The use, distribution or reproduction in other forums is permitted, provided the original author(s) and the copyright owner(s) are credited and that the original publication in this journal is cited, in accordance with accepted academic practice. No use, distribution or reproduction is permitted which does not comply with these terms.
*Correspondence: Manuel Aureliano, maalves@ualg.pt; Scott G. Mitchell, scott.mitchell@csic.es; Panchao Yin, yinpc@scut.edu.cn