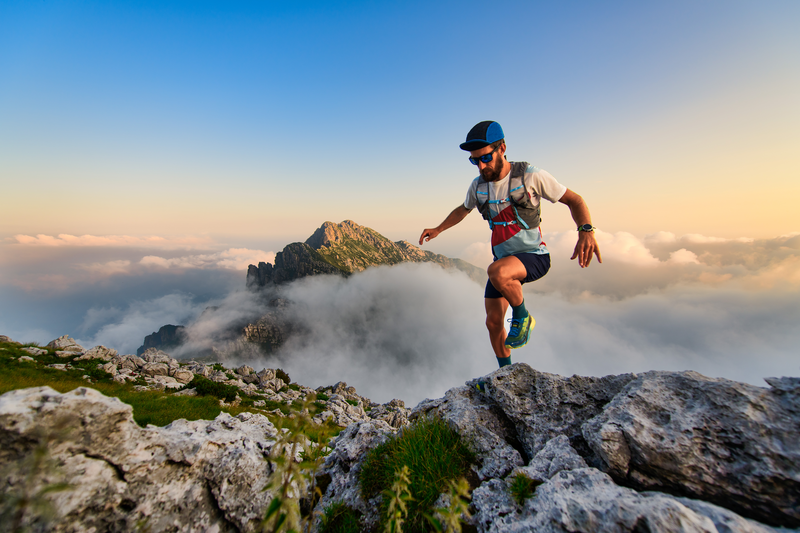
95% of researchers rate our articles as excellent or good
Learn more about the work of our research integrity team to safeguard the quality of each article we publish.
Find out more
ORIGINAL RESEARCH article
Front. Chem. , 22 July 2022
Sec. Green and Sustainable Chemistry
Volume 10 - 2022 | https://doi.org/10.3389/fchem.2022.966270
This article is part of the Research Topic Preparation of Functional Materials and Utilization of Renewable Resources in Green Solvents View all 12 articles
Functional use of biomass based on its structural properties is an efficient approach for the valuable utilization of biomass resources. In this work, carboxymethyl cellulose zirconium-based catalyst (Zr-CMC) was constructed by the coordination between the carboxylic groups in sodium carboxymethyl cellulose (CMC-Na) with transition metal Zr4+. The prepared catalyst was applied into the synthesis of furfuryl alcohol (FAL) by catalytic transfer hydrogenation of biomass-derived furfural (FF) using isopropanol as hydrogen donor. Both the preparation conditions and the reaction conditions of Zr-CMC catalyst were investigated and optimized. The results showed that Zr-CMC was efficient for the reaction with the FF conversion, FAL yield and selectivity reaching to 92.5%, 91.5 %, and 99.0%, respectively, under the mild conditions (90°C). Meanwhile, the Zr-CMC catalyst could be reused at least for five times without obvious decrease in efficiency, indicating the catalyst had excellent stability. With the advantages of sustainable raw materials, high efficiency, and excellent stability, the prepared catalyst is potential for application in the field of biomass conversion.
As an important carbon source on Earth, biomass is abundant, renewable and can produce fuel and high-value chemicals through transformation. It is expected to replace or partially replace traditional fossil resources and reduce the dependence on fossil resources for human. The high-value utilization of biomass resources can improve the economy and utilization efficiency of carbon resources in biomass refining process, and the production of high-value chemicals from biomass or its derivatives is an effective way to improve the utilization efficiency of biomass resources (Li et al., 2016a). Among the various platform compounds from biomass, furfural is a valuable chemical derived from pentosan by hydrolyzing dehydration and cyclization reaction. Furfural can be used to produce valuable chemicals such as furfuryl alcohol (FA), cyclopentanone (CLA), 2-methylfuran (2-MF) etcetera through hydrogenation (Wang et al., 2018a; Pirmoradi et al., 2020; Byun and Lee, 2021). Furfuryl alcohol is an important organic chemical raw material, mainly used in the production of furfural resin, furfuryl alcohol-ural resin, phenolic resin and so on. It is also used to prepare fruit acid, plasticizer, solvent and rocket fuel. In addition, in the fields of dye, synthetic fiber, rubber, pesticides and casting and other industrial sectors, furfuryl alcohol is also widely used (Wang et al., 2018b; Campisi et al., 2020; Lee et al., 2020; Qiu et al., 2020).
For the hydrogenation of furfural to furfuryl alcohol, homogeneous catalysts are usually used, but the homogeneous catalysts are not easy to be recovered in the reaction system (Pasini et al., 2014; Panagiotopoulou et al., 2015). Meanwhile, the hydrogenation of furfural to furfural is mainly dependent on copper chromate and expensive precious metal catalyst (Li et al., 2022). Therefore, finding cheap and efficient heterogeneous catalyst is the key for the hydrogenation of furfural to furfuryl alcohol. With the development of heterogeneous catalytic technology, a variety of mono-metal based catalysts such as Zr, Cu, Ru, Ir, Co, Fe, Pd, Pt, etc. (Pang et al., 2014; Gong et al., 2019; Li et al., 2020; Qiu et al., 2020; Sohrabi et al., 2021; Valdebenito et al., 2021) and polymetallic catalysts such as Pt/Ge (Merlo et al., 2011), Cu/Fe (Yan and Chen, 2014), Cu/Mg/Al, etc. (Chen et al., 2018) have been developed, and the catalytic hydrogenation of furfural to alcohols or esters using hydrogen as hydrogen source was also reported (Mariscal et al., 2016). Although the hydrogenation efficiency of carbonyl compounds is improved by using the precious metals and gaseous hydrogen, the high cost of precious metals and the potential safety issue of gaseous hydrogen hinder the large-scale application of many catalysts.
Meerwein-Ponndorf-Verley (MPV) reduction is a common reaction for the catalytic hydrogenation of biomass derived aldehydes or carbonyls with secondary alcohols as hydrogen donors (Luo et al., 2014). Using alcohols as hydrogen source can not only avoid safety risks caused by high-pressure hydrogen, but also improve the economy of the hydrogenation of carbonyl compounds with relatively mild reaction conditions. The secondary alcohols play the role of both hydrogen donor and the reaction solvent for the catalytic hydrogenation of carbonyl compounds to produce high-value chemicals (Wright and Palkovits, 2012). The preparation of furfuryl alcohol by MPV reduction of furfural catalyzed by different heterogeneous metal catalysts (Han et al., 2015; Li et al., 2022) has been reported. Metal ion-organic ligand hybrid MPV catalysts are commonly used for the MPV reduction of furfural. The Zr-based catalysts such as UiO-66 (Qiu et al., 2020), Zr-DM (Hao et al., 2021), Zr-HAs (Sha et al., 2017), Zr-RSL (Hao et al., 2019) and Zr/Fe (Gu et al., 2020) showed good catalytic activity in the catalytic hydrogenation reaction using alcohols as hydrogen donors. The selection of ligands is important for the construction of metal ion-organic ligand hybrid MPV catalysts. Besides the pure organic carboxylic acids, the natural macromolecules rich in organic carboxylic groups are also used in the construction of the MPV catalysts. In our previous work, the lignite derived humic acids and even lignite were used to construct Zr-containing MPV catalysts, and the prepared catalysts were proved to be highly efficient for the MPV reduction of various carbonyl compounds (Sha et al., 2017; Hao et al., 2019; Hao et al., 2021). Natural compounds rich in acidic carboxyl functional groups are natural functional molecules widely existing in nature (Luo et al., 2015). With the advantages of wide sources, low cost, renewable, etc., natural compounds are widely used in biological materials, catalytic materials, and other fields (Sahiner et al., 2015). Sodium carboxymethyl cellulose (CMC-Na) is a common anionic polymer compound derived from natural cellulose with abundant carboxylate groups in its structure. CMC-Na can be used as a ligand or carrier to construct a variety of metal catalysts. For example, carboxymethyl cellulose palladium (II) (CMC-PdII) catalyst was prepared by ion exchange between PdCl2 aqueous solution and CMC-Na aqueous solution to catalyze the Suzuki-Miyaura and Mizoroki-heck coupling reaction (Xiao et al., 2015). The Fe3O4/CMC composite material was prepared by coprecipitating method with iron salt and CMC-Na. The Ni(0)-CMC-Na catalyst was obtained using the CMC-Na aqueous solution and the precursor NiCl2·6H2O, and the catalyst showed high efficiency for the hydrogenation of a variety of functionalized olefins at room temperature and moderate hydrogen pressures (Harrad et al., 2011). Based on the reported results, it is reasonable to speculate that CMC-Na could be applied into the construction of metal ion-organic ligand MPV catalyst for the conversion of furfural. However, to the best of our knowledge, there has been no report on the above idea.
In this work, a novel Zr-CMC catalyst was constructed using CMC-Na and Zr precursor as raw material via the interaction between the carboxylate groups in CMC-Na and transition metal Zr4+. The prepared catalyst was used for the catalytic transfer hydrogenation of furfural to furfuryl alcohol using isopropanol as hydrogen donor. The effects of the preparation conditions and the reaction conditions on the performance of the catalyst were studied. The structure and the reusability of the catalyst were investigated. This work can provide potential route for the exploration of efficient, cheap and green catalysts for biomass conversion and the efficient utilization of CMC biomass resources.
Furfural (FF, 99%) (Furfural used in this work was freshly purchased and stored in the refrigerator (4°C). Before use, furfural was analyzed by GC to check its purity), furfuryl alcohol (FA, 98%) and ZrOCl2·8H2O (AR) were provided by J&K Scientific Ltd. Isopropanol (AR), ethanol (AR), decane (AR) and other chemicals were obtained from Beijing Institute of Chemical Reagent. The sodium carboxymethyl cellulose was purchased from Tianjin Guangfu Fine Chemical Research Institute.
Zr-based catalyst was prepared using sodium carboxymethyl cellulose (CMC-Na) and ZrOCl2·8H2O as the raw materials. In order to determine the appropriate ratio of sodium carboxymethyl cellulose and zirconium oxychloride octahydrate, a series of catalysts with different ratios were prepared, and their catalytic activity for hydrogenation of furfural was investigated under the same conditions, and then the appropriate ratio was selected. The mass ratios of sodium carboxymethyl cellulose and ZrOCl2·8H2O were 1:0.5, 1:1, 1:2, and 1:3, respectively.
Typical procedures were as follows (Figure 1): a certain amount of sodium carboxymethyl cellulose dissolved in 150 ml deionized water (solution A), heated and stirred in 60°C water bath to make it fully dissolved into transparent gel. At the same time, a certain amount of ZrOCl2·8H2O was proportionally weighed and dissolved in 50 ml deionized water (solution B). Pour the solution A into the separating funnel and slowly add it to the solution B, and stir the reaction system at room temperature for 5 h after dropping. The solution was then pumped and filtered or centrifuged (pH = 2–3), and washed with deionized water for at least seven times until pH neutralization. Rinse twice with anhydrous ethanol to remove the deionized water. Finally, the obtained solids were vacuum dried at 80°C for 12 h. The dried solids were ground thoroughly into powder form for subsequent use, denoted as Zr-CMC.
Scanning electron microscopy (SEM) measurements were performed on a HITACHI SU8220 scanning electron microscope operated at 20 kV. Transmission electron microscopy (TEM) images were obtained using a TEM JEOL-1011 with an accelerating voltage of 120 kV. X-ray diffraction (XRD) was carried out via an XD8 Advance-Bruker AXS X-ray diffractometer using Cu-Kα radiation (λ = 0.1543 nm) and Ni filter scanning at two per minute ranging from 5° to 90°. The tube voltage was 40 kV, and the current was 40 mA. Fourier transform-infrared spectra (FT-IR) were obtained using a PerkinElmer spectrometer. The thermogravimetric (TG) analysis of the catalyst was performed using a thermogravimetric analysis system (Diamond TG/DTA6300, PerkinElmer Instruments) under N2 atmosphere at the heating rate of 10°C min−1. The specific surface area was calculated by the BET method, and mesopore volume was derived from the adsorption isotherm according to the Barrett−Joyner−Halenda (BJH) model. All calculations were based on the adsorption isothermals.
All the hydrogenation of furfural to furfuryl alcohol in this experiment was carried out in a stainless steel reactor. In the lining of the reaction kettle (15 ml), accurate weighing of reactant furfural (1 mmol) and isopropanol (5 ml) as hydrogen source and reaction solvent, as well as a certain amount of catalyst and decane as internal standard, using atmospheric pressure operation, the reaction kettle is sealed and placed in an oil bath with constant temperature heating magnetic stirring. After the reaction, remove the reactor quickly from the oil bath and place it in the mixture of ice water for rapid cooling. After cooling, the reaction solution is separated from the solid catalyst by centrifugation. The residue of reaction product and substrate can be quantitatively analyzed by gas chromatograph (TECHCOMP GC7900). The specific conditions of gas chromatography were as follows: hydrogen flame ion detector (FID), nitrogen as carrier gas, inlet temperature of 40°C, detection temperature of 230°C, set the heating program for furfural column temperature program, using area internal standard method for quantitative analysis of the reactant furfural and target product furfuryl alcohol, using decane as internal standard.
In order to check the heterogeneity of the catalysts, the solid catalysts were removed from the reaction mixture after reaction for certain time, and the supernatant was allowed to react to see if the product yield could further increase with the absence of the solid catalysts. In the reusability experiments, the catalyst was separated by centrifugation, washed with fresh isopropanol for three times, and then reused for the next run without further treatments.
Table 1 showed the comparison of the activity of the catalysts prepared in different proportions. The results showed that with the increase of ZrOCl2·8H2O content, the conversion of furfural, the yield of furfuryl alcohol and the selectivity of the reaction increased gradually. When the mass ratio of CMC-Na to ZrOCl2·8H2O was 1:2, the activity of the prepared catalyst reached the highest, possibly because the carboxylic acid groups in CMC-Na and Zr4+ were completely combined at this ratio. With the further increase of the mass of ZrOCl2·8H2O, the catalytic performance decreases, indicating that there is no excess carboxylic acid group in CMC-Na for the coordination of Zr4+. It may be due to that when the mass ratio of CMC-Na to ZrOCl2.8H2O is 1:3, the acidity of the solution increased, which may affect the coordination reaction between zirconium precursor and carboxylic acid in CMC-Na, leading to the low content of Zr in the catalyst. Besides, the precipitation amount obtained under this condition decreases. Therefore, from viewpoint of catalyst activity and the utilization efficiency of Zr precursor and CMC-Na, the best mass ratio of CMC-Na and ZrOCl2·8H2O in the preparation process is 1:2.
TABLE 1. Performances of Zr-CMC catalysts prepared under different mass ratios of CMC-Na to ZrOCl2·8H2O.
In order to better understand the structure and morphology characteristics of the prepared Zr-CMC catalyst, SEM, TEM, XRD, FTIR, TG, and BET were used to characterize it. Meanwhile, CMC-Na was also characterized by XRD, FTIR, and TG, and compared with the prepared Zr-CMC catalyst in structure. Firstly, the Zr content in the optimal catalyst was analyzed by ICP-OES, and the result showed that the Zr content in Zr-CMC (1:2) catalyst was around 20.5 wt%. As given in Figure 2, SEM and TEM showed that the Zr-CMC catalyst was composed of particles with no uniform shapes (Figures 2A,B). N2 adsorption/desorption test showed that the Zr-CMC catalyst prepared was nonporous (Figure 2C), and the BET surface area was around 3 m2/g. The low specific surface area may be caused by the dense structures formed by CMC-Na with Zr precursor and how to improve the surface area is under studying in our group. A strong X-ray diffraction peak was observed for the pure CNC-Na at about 21°, while a weak bulge peak was observed for the Zr-CMC catalyst at 20–30°, indicating that the prepared catalyst had no specific X-ray diffraction peak and had an amorphous structure (Figure 2D). The FTIR spectra of CMC-Na and the Zr-CMC catalyst were compared in Figure 2E. The FTIR spectra of CMC-Na showed that there were asymmetric and symmetric stretching vibration absorption peaks of the carboxyl functional groups at 1,591 and 1,456 cm−1, respectively. In the FTIR spectra of Zr-CMC catalyst, the asymmetric and symmetric stretching vibration absorption peaks of the carboxyl functional groups were at 1,579 and 1,420 cm−1, respectively. Compared with CMC-Na, the difference between the asymmetric and symmetric stretching vibration absorption peaks of the carboxyl functional groups in Zr-CMC catalyst changes from 135 cm−1 to 159 cm−1, and a new vibration absorption peak appears at 1,456 cm−1. The above results indicate that zirconium is successfully combined with carboxyl functional groups (Song et al., 2015a). As shown in the figure (Figure 2F) for TG analysis, the prepared Zr-CMC catalyst begins to decompose at 300°C, while the reaction temperature is below 100°C. Therefore, the Zr-CMC catalyst is stable under the reaction temperature (Peng et al., 2012).
FIGURE 2. Characterization of the as-prepared Zr-CMC catalyst by SEM (A), TEM (B), N2 adsorption-desorption (C), XRD pattern (D), FTIR spectra (E) and TG analysis (F).
The important parameters influencing the performances of the catalysts were investigated, including the catalyst dosage, reaction temperature, and reaction time. Figure 2 showed the performances of the Zr-CMC catalyst under different conditions. As could be seen from Figure 3A, the conversion of furfural and the yield of furfuryl alcohol increased continually with the increasing of the catalyst dosage from 0.05 to 0.2 g. Under the catalyst dosage of 0.2 g, the conversion, yield and selectivity were 87.9%, 96.3 %, and 90.9%, respectively. However, the furfural conversion, furfuryl alcohol yield and reaction selectivity did not increase further when the amount of catalyst was increased to 0.25 g, which may be because the excessive amount of catalyst affected the mass transfer of catalyst in the reaction system. The above results indicate that 0.2 g dosage may be the appropriate catalyst dosage under the current reaction conditions, which can be used for further experimental studies. The effect of reaction temperature was shown in Figure 3B, and it was obvious that the reaction temperature had a significant influence on the performance of the Zr-CMC catalyst. Before 90°C, the furfural conversion and furfural alcohol yield increased significantly with the increase of temperature, reaching 90.9 % and 87.9%, respectively, under 90°C. However, as the temperature continued to rise, although the conversion continued to increase at a slower rate, the yield and selectivity declined slightly, presumably due to the formation of some by-products, which could be proved by the deep color of the reaction system under 100°C. Therefore, subsequent investigations were conducted at 90°C as the suitable temperature under the present reaction conditions. Under the above reaction conditions, the time-profile of the reaction was studied, as shown in Figure 3C. The conversion of furfural and the yield of furfuryl alcohol increased significantly when the reaction time increased from 0.5 to 3 h. As the reaction time was extended to 6 h, the conversion and selectivity of the reactant increased slightly, which may be due to that the extension of the reaction time led to the formation of the condensation by-products of alcohol and aldehyde. This could be proved by the presence of some weak and unknown peaks during GC detect.
FIGURE 3. The influences of reaction parameters on the catalysts (A) Effect of the Zr-CMC catalyst dosage. (B) Effect of the reaction temperature. (C) Effect of the reaction time. Reaction conditions: Furfural (1 mmol), isopropanol (5 ml). (A) 90°C, 3 h (B) Catalyst 0.2 g, 3 h (C) Catalyst 0.2 g, 90°C.
After studying the reaction condition, the performance of the prepared Zr-CMC catalyst was compared with other common Zr-based catalysts in the literatures (Table 2). The results showed that the activity of the prepared catalyst was comparable with many other catalysts under similar reaction conditions. The performance of the Zr-CMC catalyst was even superior to some catalyst in aspects of selectivity and TOF. Besides the high activity, the Zr-CMC catalyst possessed the advantages of biomass-derived and renewable raw ligands during preparation, making the Zr-CMC catalyst promising for potential application.
TABLE 2. Comparison of the prepared Zr-CMC catalyst with other Zr-based catalysts in literatures for the conversion of furfural into furfuryl alcohol using isopropanol as hydrogen donora.
Reusability and heterogeneity were important for the solid heterogeneous catalyst. The reusability of Zr-CMC catalyst showed that the conversion, selectivity and product yield of the catalyst did not decrease significantly after repeated use for 5 times, indicating that the catalyst has good stability and can be reused (Figure 4A). The heterogeneity of Zr-CMC catalyst was also studied. The results showed that the reaction stopped after the catalyst was removed from the system, and no overflow of the active site of the catalyst was detected in the reaction solution, indicating that the catalyst was heterogeneous in the catalytic process (Figure 4B).
FIGURE 4. Reusability (A) and heterogeneity (B) of the prepared catalyst. Reaction conditions: Furfural 1 mmol, isopropanol 5 ml, Zr-CMC 0.2 g, reaction temperature 90°C, and reaction time 1 h (0.5 h for heterogeneity).
In summary, a route of using the sodium carboxymethyl cellulose (CMC-Na) from cellulose derivatives to construct Zr-CMC catalyst was identified. The results of SEM and TEM showed that the catalyst was irregularly granular. TG results showed that the Zr-CMC catalyst has good thermal stability at the reaction temperature. FT-IR showed that Zr4+ was successfully coordinated with carboxyl group in CMC-Na. The XRD results showed that the prepared catalyst was amorphous. The reaction results showed that when the mass ratio of CMC-Na to ZrOCl2·8H2O was 1: 2, the reaction activity of the obtained catalyst was the highest. Under the condition of furfural 1 mmol, reaction temperature 90°C, catalyst 0.2 g, reaction time 5 h, the conversion of furfural, the selectivity and yield of furfuryl alcohol could be reached to 92.5%, 91.5 %, and 99.0%, respectively. The heterogeneity experiment showed that the catalyst was heterogeneous during the reaction, and the activity did not change significantly when the catalyst was recycled 5 times. On the whole, the catalyst could be reused and had good stability. The proposed route in this work may find its potential applications in the field of biomass utilization with the advantages of high efficiency of the catalysts, broad applicability, and simple preparing processes.
The original contributions presented in the study are included in the article/supplementary material, further inquiries can be directed to the corresponding author.
The laboratory work, analysis of data and writing was carried out predominantly by JH. HZ and NL helped in the design and review of data and interpretation. YZ and TZ did supplementary experiment. QL, KZ, and RH provided experimental design ideas. All authors contributed to the final writing of the paper.
This study was supported by the National Nature Science Foundation of China (21968021, 21868020, 21868021), Grassland Talents Engineering of Inner Mongolia, CAS “Light of West China” Program, Local Science and Technology Development Fund Projects Guided by the Central Government (2020ZY0010), the Inner Mongolia Nature Science Foundation (2019MS02025), the Inner Mongolia Major Science and Technology Project (2021ZD0020), the Science and Research Projects of Inner Mongolia University of Technology (IMUT) (ZY202004 and BS2021018), and the Startup Fund for New Teachers of Inner Mongolia University of Technology (IMUT).
The authors declare that the research was conducted in the absence of any commercial or financial relationships that could be construed as a potential conflict of interest.
All claims expressed in this article are solely those of the authors and do not necessarily represent those of their affiliated organizations, or those of the publisher, the editors and the reviewers. Any product that may be evaluated in this article, or claim that may be made by its manufacturer, is not guaranteed or endorsed by the publisher.
Byun, M. Y., and Lee, M. S. (2021). Pt supported on hierarchical porous carbon for furfural hydrogenation. J. Ind. Eng. Chem. 104, 406–415. doi:10.1016/j.jiec.2021.08.038
Campisi, S., Chan-Thaw, C. E., Chinchilla, L. E., Chutia, A., Botton, G. A., Mohammed, K. M. H., et al. (2020). Dual-site-mediated hydrogenation catalysis on Pd/NiO: Selective biomass transformation and maintenance of catalytic activity at low Pd loading. ACS Catal. 10, 5483–5492. doi:10.1021/acscatal.0c00414
Chen, H., Ruan, H. H., Lu, X. L., Fu, J., Langrish, T., Lu, X. Y., et al. (2018). Efficient catalytic transfer hydrogenation of furfural to furfuryl alcohol in near-critical isopropanol over Cu/MgO-Al2O3 catalyst. Mol. Catal. 445, 94–101. doi:10.1016/j.mcat.2017.11.011
Gong, W., Lin, Y., Chen, C., Al-Mamun, M., Lu, H. S., Wang, G., et al. (2019). Nitrogen‐doped carbon nanotube confined Co–nx sites for selective hydrogenation of biomass‐derived compounds. Adv. Mat. 31, 1808341. doi:10.1002/adma.201808341
Gu, J., Zhang, J., Wang, Y., Li, D., Huang, H., Yuan, H., et al. (2020). Efficient transfer hydrogenation of biomass derived furfural and levulinic acid via magnetic zirconium nanoparticles: Experimental and kinetic study. Ind. Crops Prod. 145, 112133. doi:10.1016/j.indcrop.2020.112133
Han, H. F., Zhang, S. F., Guo, Z. Q., Tong, H. B., and Wei, X. H. (2015). Three asymmetric guanidinato metal complexes: Synthesis, crystal structures and their use as pre-catalysts in the Meerwein–Ponndorf–Verley reduction. Polyhedron 99, 71–76. doi:10.1016/j.poly.2015.06.026
Hao, J. X., Han, L. M., Liu, Q. S., Li, N., He, R., Zhi, K., et al. (2021). A general route of using lignite depolymerized derivatives for catalyst construction: Insights into the effects of the derivative structures and solvents. ACS Omega 6, 14926–14937. doi:10.1021/acsomega.1c00766
Hao, J. X., Zhou, H. C., Liu, Q. S., Yu, X., Ma, X., et al. (2019). Facile use of lignite as robust organic ligands to construct Zr-based catalysts for the conversion of biomass derived carbonyl platforms into alcohols. Fuel 239, 1304–1314. doi:10.1016/j.fuel.2018.11.129
Harrad, M. A., Valerga, P., Puerta, M. C., Houssini, I., Ali, M. A., Firdoussi, L. E., et al. (2011). Ni(0)-CMC-Na nickel colloids in sodium carboxymethyl-cellulose: Catalytic evaluation in hydrogenation reactions. Molecules 16, 367–372. doi:10.3390/molecules16010367
Iglesias, J., Melero, J., Morales, G., Moreno, J., Segura, Y., Paniagua, M., et al. (2015). Zr-SBA-15 lewis acid catalyst: Activity in meerwein ponndorf verley reduction. Catalysts 5, 1911–1927. doi:10.3390/catal5041911
Lee, J., Woo, J., Nguyen-Huy, C., Lee, M. S., Joo, S. H., An, K., et al. (2020). Highly dispersed Pd catalysts supported on various carbons for furfural hydrogenation. Catal. Today 350, 71–79. doi:10.1016/j.cattod.2019.06.032
Lestari, W. W., Suharbiansah, R. S. R., Larasati, L., Rahmawati, F., Arrozi, U. S. F., Durini, S., et al. (2022). A zirconium(IV)-based metal-organic framework modified with ruthenium and palladium nanoparticles: Synthesis and catalytic performance for selective hydrogenation of furfural to furfuryl alcohol. Chem. Pap. doi:10.1007/s11696-022-02193-1
Li, F., Jiang, S., Huang, J., Wang, Y., Lu, S., Li, C., et al. (2020). Catalytic transfer hydrogenation of furfural to furfuryl alcohol over a magnetic Fe3O4@C catalyst. New J. Chem. 44 (2), 478–486. doi:10.1039/c9nj04698d
Li, H., He, J., Riisager, A., Saravanamurugan, S., Song, B. A., Yang, S., et al. (2016). Acid–base bifunctional zirconium N-alkyltriphosphate nanohybrid for hydrogen transfer of biomassderived carboxides. ACS Catal. 6, 7722–7727. doi:10.1021/acscatal.6b02431
Li, Y., Lv, G., Wang, Y., Deng, T., Wang, Y., Hou, X., et al. (2016). Synthesis of 2, 5-hexanedione from biomass resources using a highly efficient biphasic system. ChemistrySelect 1 (6), 1252–1255. doi:10.1002/slct.201600280
Li, Z. F., Shen, Y., Zhang, Q., and Hu, T. L. (2022). Budget MOF-derived catalyst to realize full conversion from furfural to furfuryl alcohol. Mol. Catal. 518, 112092. doi:10.1016/j.mcat.2021.112092
Liu, C., Xu, G. Z., Hu, A. Y., Xie, Y. D., and Wang, H. J. (2019). Porous zirconium hydroxyphosphonoacetate: Catalyst for conversion of furfural into furfuryl alcohol. Chemistryselect 4 (27), 8000–8006. doi:10.1002/slct.201901612
Luo, H. Y., Consoli, D. F., Gunther, W. R., and Román-Leshkov, Y. (2014). Investigation of the reaction kinetics of isolated Lewis acid sites in Beta zeolites for the Meerwein–Ponndorf–Verley reduction of methyl levulinate to γ-valerolactone. J. Catal. 320, 198–207. doi:10.1016/j.jcat.2014.10.010
Luo, J., Zhang, N., Lai, J., Liu, R., and Liu, X. (2015). Tannic acid functionalized graphene hydrogel for entrapping gold nanoparticles with high catalytic performance toward dye reduction. J. Hazard. Mat. 300, 615–623. doi:10.1016/j.jhazmat.2015.07.079
Mariscal, R. M.-T. P., Ojeda, M., Sadaba, I., and Lopez Granados, M. (2016). Furfural: A renewable and versatile platform molecule for the synthesis of chemicals and fuels. Energy Environ. Sci. 9, 1144–1189. doi:10.1039/c5ee02666k
Merlo, A. B., Vetere, V., Ramallo-López, J. M., Requejo, F. G., and Casella, M. L. (2011). Liquid-phase furfural hydrogenation employing silica-supported PtSn and PtGe catalysts prepared using surface organometallic chemistry on metals techniques. Reac. Kinet. Mech. Cat. 104 (2), 467–482. doi:10.1007/s11144-011-0374-4
Panagiotopoulou, P., Martin, N., and Vlachos, D. G. (2015). Liquid-Phase catalytic transfer hydrogenation of furfural over homogeneous lewis acid-Ru/C catalysts. ChemSusChem 8 (12), 2046–2054. doi:10.1002/cssc.201500212
Pang, S. H., Love, N. E., and Medlin, J. W. (2014). Synergistic effects of alloying and thiolate modification in furfural hydrogenation over Cu-based catalysts. J. Phys. Chem. Lett. 5, 4110–4114. doi:10.1021/jz502153q
Pasini, T., Lolli, A., Albonetti, S., Cavani, F., and Mella, M. (2014). Methanol as a clean and efficient H-transfer reactant for carbonyl reduction: Scope, limitations, and reaction mechanism. J. Catal. 317, 206–219. doi:10.1016/j.jcat.2014.06.023
Peng, L., Zhang, J. L., Li, J. S., Han, B. X., Xue, Z. M., Yang, G. Y., et al. (2012). Surfactant-directed assembly of mesoporous metal-organic framework nanoplates in ionic liquids. Chem. Commun. 48, 8688. doi:10.1039/c2cc34416e
Pirmoradi, M., Gulotty, R. J., and Kastner, J. R. (2020). Continuous hydroxyketone production from furfural using Pd-TiO2 supported on activated carbon. Catal. Sci. Technol. 10, 7002–7015. doi:10.1039/d0cy01556c
Qiu, M., Guo, T. M., Xi, R., Li, D. N., and Qi, X. H. (2020). Highly efficient catalytic transfer hydrogenation of biomass-derived furfural to furfuryl alcohol using UiO-66 without metal catalysts. Appl. Catal. A General 602, 117719. doi:10.1016/j.apcata.2020.117719
Sahiner, N., Sagbas, S., and Aktas, N. (2015). Single step natural poly(tannic acid) particle preparation as multitalented biomaterial. Mater. Sci. Eng. C 49, 824–834. doi:10.1016/j.msec.2015.01.076
Sha, Y. F., Xiao, Z. H., Zhou, H. C., Yang, K., Song, Y., Li, N., et al. (2017). Direct use of humic acid mixtures to construct efficient Zr-containing catalysts for Meerwein–Ponndorf–Verley reactions. Green Chem. 19 (20), 4829–4837. doi:10.1039/c7gc01925d
Sohrabi, S., Abasabadi, R. K., Khodadadi, A. A., Mortazavi, Y., and Hoseinzadeh, A. (2021). In-situ one-step deposition of highly dispersed palladium nanoparticles into zirconium metal-organic framework for selective hydrogenation of furfural. Mol. Catal. 514, 111859. doi:10.1016/j.mcat.2021.111859
Song, J. L., Wu, L. Q., Zhou, B. W., Zhou, H. C., Fan, H. L., Yang, Y. Y., et al. (2015). A new porous Zr-containing catalyst with a phenate group: An efficient catalyst for the catalytic transfer hydrogenation of ethyl levulinate to γ-valerolactone. Green Chem. 17, 1626–1632. doi:10.1039/c4gc02104e
Song, J. L., Zhou, B. W., Zhou, H. C., Wu, L. Q., Meng, Q. L., Liu, Z. M., et al. (2015). Porous zirconiumphytic acid hybrid: A highly efficient catalyst for meerwein-ponndorf-verley reductions. Angew. Chem. Int. Ed. Engl. 54, 9531–9535. doi:10.1002/ange.201504001
Valdebenito, G., Parra-Melipan, S., Lopez, V., Aranda, B., García, E., Vega, A., et al. (2021). Selective hydrogenation of furfural to furfuryl alcohol catalysed by ruthenium complexes containing phosphorus-nitrogen ligands. Appl. Organomet. Chem. 35 (11), 6382. doi:10.1002/aoc.6382
Wang, W., Villa, A., Kübel, C., Hahn, H., and Wang, D. (2018). Tailoring the 3D structure of Pd nanocatalysts supported on mesoporous carbon for furfural hydrogenation. ChemNanoMat 4, 1125–1132. doi:10.1002/cnma.201800308
Wang, Y. T., Prinsen, P., Triantafyllidis, K. S., Karakoulia, S. A., Trikalitis, P. N., Yepez, A., et al. (2018). Comparative study of supported monometallic catalysts in the liquid-phase hydrogenation of furfural: Batch versus continuous flow. ACS Sustain. Chem. Eng. 6, 9831–9844. doi:10.1021/acssuschemeng.8b00984
Wright, W. R., and Palkovits, R. (2012). Development of heterogeneous catalysts for the conversion of levulinic acid to gamma-valerolactone. ChemSusChem 5 (9), 1657–1667. doi:10.1002/cssc.201200111
Xiao, J. L., Lu, Z. X., and Li, Y. Q. (2015). Carboxymethylcellulose-supported palladium nanoparticles generated in situ from palladium(II) carboxymethylcellulose as an efficient and reusable catalyst for ligand- and base-free Heck–Matsuda and Suzuki–Miyaura couplings. Appl. Organomet. Chem. 29 (9), 646–652. doi:10.1002/aoc.3346
Yan, K., and Chen, A. (2014). Selective hydrogenation of furfural and levulinic acid to biofuels on the ecofriendly Cu–Fe catalyst. Fuel 115, 101–108. doi:10.1016/j.fuel.2013.06.042
Keywords: sodium carboxymethyl cellulose, furfural, hydrogenation, catalyst, biomass
Citation: Hao J, Zhang Y, Zhang T, Zhou H, Liu Q, Zhi K, Li N and He R (2022) A novel and highly efficient Zr-containing catalyst supported by biomass-derived sodium carboxymethyl cellulose for hydrogenation of furfural. Front. Chem. 10:966270. doi: 10.3389/fchem.2022.966270
Received: 10 June 2022; Accepted: 30 June 2022;
Published: 22 July 2022.
Edited by:
Jinliang Song, Guangdong University of Technology, ChinaReviewed by:
Chao Xie, South-Central University for Nationalities, ChinaCopyright © 2022 Hao, Zhang, Zhang, Zhou, Liu, Zhi, Li and He. This is an open-access article distributed under the terms of the Creative Commons Attribution License (CC BY). The use, distribution or reproduction in other forums is permitted, provided the original author(s) and the copyright owner(s) are credited and that the original publication in this journal is cited, in accordance with accepted academic practice. No use, distribution or reproduction is permitted which does not comply with these terms.
*Correspondence: Jianxiu Hao, aGp4MjAyMEBpbXV0LmVkdS5jbg==
Disclaimer: All claims expressed in this article are solely those of the authors and do not necessarily represent those of their affiliated organizations, or those of the publisher, the editors and the reviewers. Any product that may be evaluated in this article or claim that may be made by its manufacturer is not guaranteed or endorsed by the publisher.
Research integrity at Frontiers
Learn more about the work of our research integrity team to safeguard the quality of each article we publish.