- 1Research Center of Chemical Biology and Pharmaceutical Chemistry, School of Life Sciences and Medicine, Shandong University of Technology, Zibo, China
- 2School of Pharmaceutical Science and Technology, Tianjin University, Tianjin, China
Chalcones have a three-carbon α,β-unsaturated carbonyl system composed of two phenolic rings. Many chalcones have shown broad spectrum of biological activities with clinical potentials against various diseases. They are usually abundant in seeds, fruit skin, bark and flowers of most edible plants. Among them, chalcones bearing 2-hydroxy-3-methyl-3-butenyl (HMB) group have been reported several times in the past few decades due to their novel scaffolds and numerous interesting biological activities. In this paper, we reviewed the isolation of twelve natural chalcones and a natural chalcone-type compound bearing 2-hydroxy-3-methyl-3-butenyl group discovered so far, and reviewed their synthesis methods and biological activities reported in the literature. We anticipate that this review will inspire further research of natural chalcones.
1 Introduction
The existing chalcones mainly include natural products and synthetic compounds (Rudrapal et al., 2021; Zhang et al., 2021; Yuan et al., 2022), and have been shown to exhibit a variety of biological activities, such as anticancer (Konieczny et al., 2007), anti-inflammatory (Nowakowska, 2007), antibacterial (Nielsen et al., 2005), antiviral (Duran et al., 2021), antimalaria (Smit and N’Da, 2014), and so on. It is an important approach for preclinical drug development to find new scaffolds from natural products and screen out lead compounds with high activity and low toxicity through chemical synthesis and structure-activity relationship study (SAR) (Gomes et al., 2017; Duvauchelle et al., 2021; Jasim et al., 2021; Knockleby et al., 2021). Chalcones have been extensively studied, and many reviews have been published in a wide variety of journals (Zhuang et al., 2017; Qin et al., 2020; Salehi et al., 2021). However, to our knowledge, there is no review of natural chalones bearing HMB group so far. Since the 1990s, twelve natural chalcones (1–7, 9–13) and a natural chalcone-type compound (8, Angusticornin A) with HMB group on A-ring or B-ring have been isolated and reported successively (Baba et al., 1990; Hano et al., 1995; Pistelli et al., 1996; Stevens et al., 2000; ElSohly et al., 2001; Ngameni et al., 2004; Ngadjui et al., 2005; RenQi and Shi, 2008; Shaffer et al., 2016; Yang and Jiang, 2021) (Figure 1). And the HMB group in their structures have also been proved to be an essential functional group for some biological activities (Sugii et al., 2005; Park et al., 2015). This review provides a research progress of the isolation, chemical synthesis and biological activities of natural chalcones bearing HMB group, and the plant species and biological activities of these chalcones are illustrated in Table 1.
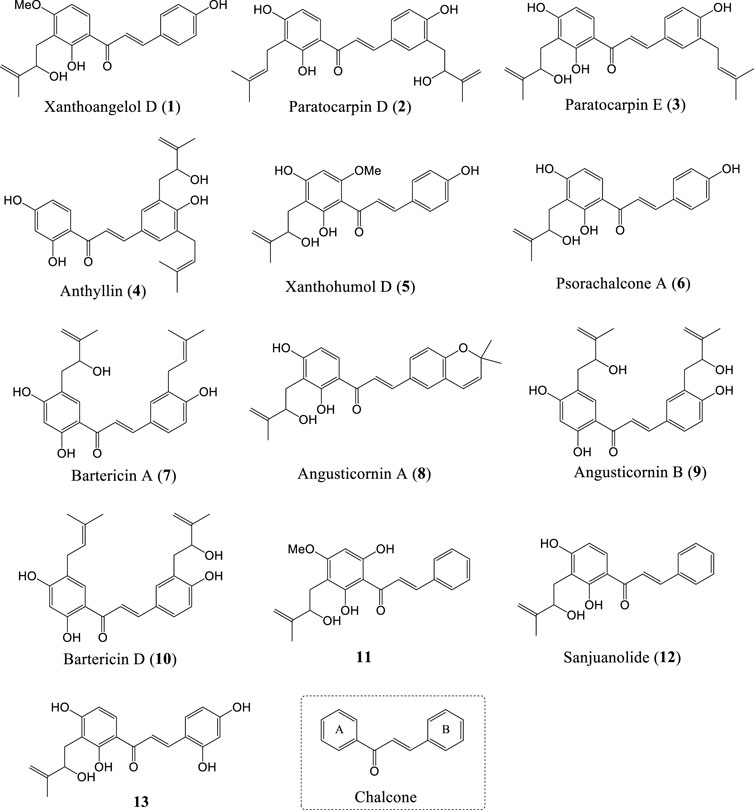
FIGURE 1. Structures of natural chalcones and a chalcone-type compound bearing HMB group (1-13) and chalcone.
2 Natural chalcones and a natural chalcone-type compound bearing HMB group
2.1 Xanthoangelol D (1)
2.1.1 Isolation and biological activities
Xanthoangelol D and five other chalcones were extracted from fresh roots of Angelica keiskei collected in Hachijyo Island (Japan) by using ethyl acetate (Baba et al., 1990). Subsequently, the results of Sugii et al. showed that Xanthoangelol D suppresses basal and tumor necrosis factor-α-induced endothelin-1 (ET-1) production, by inhibiting the activation of nuclear factor-kappa B (NF-κB), therefore, may be useful for the treatment of diseases involved NF-κB activation (Sugii et al., 2005). Kil et al. also isolated Xanthoangelol D from the aerial parts of Angelica keiskei Koidzumi together with twelve other chalcones, and Xanthoangelol D did not exhibit significant activity in the assay of promoter activity on heat shock protein 25 (hsp25, murine form of human hsp27) (Kil et al., 2015). Xanthoangelol D showed strong potein tyrosine phosphatase 1B (PTP1B) inhibitory effect with IC50 value of 3.97 ± 0.37 μg/ml (Li et al., 2015). Interestingly, the inhibitory effects of Xanthoangelol D (substitution of A-ring with the HMB group) on severe acute respiratory syndrome coronavirus (SARS-CoV) chymotrypsin-like protease activity produced 4-fold (IC50 = 26.6 ± 5.2 μM) higher potency than analogue that substitution of A-ring with the 3-methyl-2-butenyl group (Park et al., 2015). Furthermore, Xanthoangelol D did not exhibit anti-platelet-activities in vivo according to Ohkura et al. (Ohkura et al., 2016).
2.1.2 Chemical synthesis
Li et al. reported the first synthesis of Xanthoangelol D with Schenck ene reaction using tetraphenylporphin (TPP) as the photosensitizer followed by reduction with triphenylphosphine (Li et al., 2019), and the key intermediate 20 can be obtained through Claisen-Schmidt condensation, [1,3]-sigmatropic rearrangement and deprotection by using the method of Sugamoto et al. (Sugamoto et al., 2008; Sugamoto et al., 2011) (Scheme 1A). One year later, Sugamoto et al. synthesized Xanthoangelol D in 56% yield by using the photooxygenation of prenylated chalcone (20) in the presence of methylene blue in acetonitrile followed by reduction with trimethylphosphite (Sugamoto et al., 2020) (Scheme 1B). These two methods described above provide important reference for the construction of HMB group in chalcone derivatives.
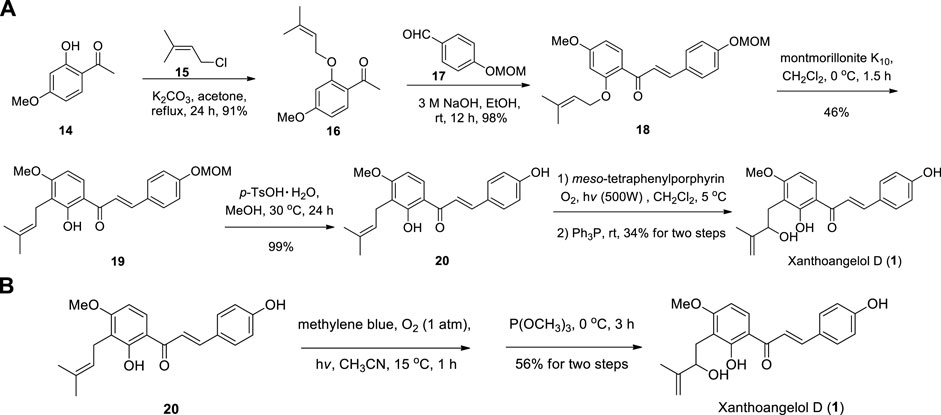
SCHEME 1. (A) Synthesis of Xanthoangelol D by Li et al.; (B) Synthesis of Xanthoangelol D by Sugamoto et al.
2.2 Paratocarpin D and E (2, 3)
2.2.1 Isolation and biological activities
In 1995, Paratocarpin D and E were isolated from the Indonesian moraceous plant (Bark of Paratocarpus venenosa Zoll) by Hano et al. (1995) for the first time. Liu et al. (2005) also reported the isolation of Paratocarpin E along with two other new chalcones from the roots of Hedysarum gmelinii (collected from Inner Mongolia, China), and it was the first time that Paratocarpin E has been isolated from Hedysarum genus (Liu et al., 2005). Gao et al. isolated Paratocarpin E from Euphorbia humifusa Wild., and Paratocarpin E showed significant cytotoxicity against five cancer cell lines (MCF-7, 786-O, 769-P, U-937 and HL-60) with IC50 values ranging from 19.6 to 28.6 μM. According to the report, Paratocarpin E typical apoptosis of MCF-7 cells by activating p38 and JNK and inhibiting Erk pathway, and affect apoptosis and autophagy by promotes the activation and nuclear translocation of NF-κB (Gao et al., 2016; Al-Emam et al., 2019). Paratocarpin D and E were evaluated for antiproliferative activity against five human cancer cell lines (HepG2, A549, Du145, BGC823, and HCT116) and in vitro anti-inflammatory activity by Liu et al. (2005) but only Paratocarpin E exhibited weak inhibitory effects (IC50 values in the range of 10.33–18.18 μM) on lipopolysaccharide-induced nitric oxide production in murine microglial BV-2 cells (Liu et al., 2018). Furthermore, the racemate Paratocarpin E obtained by chemical synthesis exhibited significant antibacterial activity (MIC = 6.25 μg/ml) against Bacillus subtilis strain (Li et al., 2019). In 2021, Ibraheem et al. isolated Paratocarpin D from baobab (Adansonia digitata L.) fruit pulp methanolic extract, however, no activity data of individual compounds were reported (Ibraheem et al., 2021).
2.2.2 Chemical synthesis
Li et al. reported the synthesis of Paratocarpin E using key intermediate 24 as the starting material, employing Schenck ene reaction, Claisen-Schmidt condensation and deprotection, respectively (Li et al., 2019) (Scheme 2). And the intermediate 24 can be prepared from 2,4-dihydroxyacetophenone (21) in two steps (Dong et al., 2007). However, the chemical synthesis of Paratocarpin D has not been reported yet.
2.3 Anthyllin (4)
In 1996, Anthyllin has been isolated from the aerial parts of Anthyllis hermanniae, along with six chalcone and isoflavonoid derivatives (Pistelli et al., 1996). Up to now, there have been no previous reports on biological activity or chemical synthesis of Anthyllin.
2.4 Xanthohumol D (5)
2.4.1 Isolation and biological activities
In 2000, Xanthohumol D was isolated from Humulus lupulus cv. “Galena” by Stevens et al. for the first time (Stevens et al., 2000; Bocquet et al., 2018; Zhou et al., 2021). Zhao et al. isolated Xanthohumol D from the ethyl acetate fraction of Humulus lupulus L., and Xanthohumol D significantly inhibited NO production at 5 μg/ml (completely suppressed the expression of inducible NO synthase induced by lipopolysaccharide/IFN-γ) (Zhao et al., 2003; Zhao et al., 2005). In 2004, Chadwick et al. isolated Xanthohumol D from spent Nugget hop pellets (Humulus lupulus L. cv. Nugget) by supercritical CO2 extraction Chadwick et al. (2004). Subsequently, Xanthohumol D was tested for induction of quinone reductase in Heap 1c1c7 murine hepatoma cells by Liu et al. (2005) and the CD value was 7.4 ± 0.7 μM. Chesnokova et al. also isolated Xanthohumol D from hops (Humulus lupulus) by using Soxhlet apparatus Chesnokova et al. (2009). Choi et al. isolated Xanthohumol D from ethanolic extract of hops (Humulus lupulus L.), and Xanthohumol D was used to determine the inhibition of quinone reductase-2 (IC50 = 110 ± 27 μM) (Choi et al., 2011; Cieśla and Moaddel, 2016; Wei et al., 2016; Chen et al., 2018). Tronina et al. (2013) assessed the ability of Xanthohumol D to scavenge 2,2′-diphenyl-1-picrylhydrazyl (DPPH) radicals (IC50 = 2.37 ± 0.40 μM). In addition, the antiproliferative activity of Xanthohumol D against MCF-7 (IC50 = 20.60 ± 0.22 μM), PC-3 (IC50 = 37.88 ± 13.90 μM) and HT-29 (IC50 = 78.33 ± 8.83 μM) human cancer cell lines were also determined. Yu et al. (2014) isolated Xanthohumol D and seven other chalcones from Humulus lupulus, and the quinone reductase induction activity results showed that Xanthohumol D has moderate activity (IR = 2.22 ± 0.05, viability = 0.45%) in using human Heap 1c1c7 cells at the concentration of 20 μM. Sangiovanni et al. (2019) isolated Xanthohumol D from hop extracts (Humulus lupulus L. cultivar Cascade), and reported the anti-inflammatory activity of the hop extracts (Xanthoangelol D and Xanthoangelol A as the main active components) in human gastric epithelial cells. Fu et al. (2020) evaluated the antibacterial activities of synthetic Xanthohumol D against two Gram positive bacteria (Staphylococcus aureus CMCC 26003, Bacillus subtilis CMCC(B) 63,501) and two Gram negative bacteria (Escherichia coli CMCC 44102, Pseudomonas aeruginosa CMCC (B) 10,104), and Xanthohumol D showed significant activity towards Bacillus subtilis (MIC = 12.5 μg/ml) but no obvious inhibitory activity to the other three strains (MIC > 200 μg/ml).
2.4.2 Chemical synthesis
Fu et al. reported the first synthesis of Xanthohumol D commenced from the Schenck ene reaction of intermediate 34, which introduced the HMB groups. Then 35 was carried out in using catalytic amounts of p-TsOH leading to the target product Xanthohumol D (Fu et al., 2020) (Scheme 3A). The key intermediate 34 was prepared from 28 by using the method of Khupse et al. (Khupse and Erhardt, 2007). Sugamoto et al. (2020) also synthesized Xanthohumol D by using the same procedure employed for the synthesis of Xanthoangelol D from prenylated chalcone (36) (Scheme 3B).
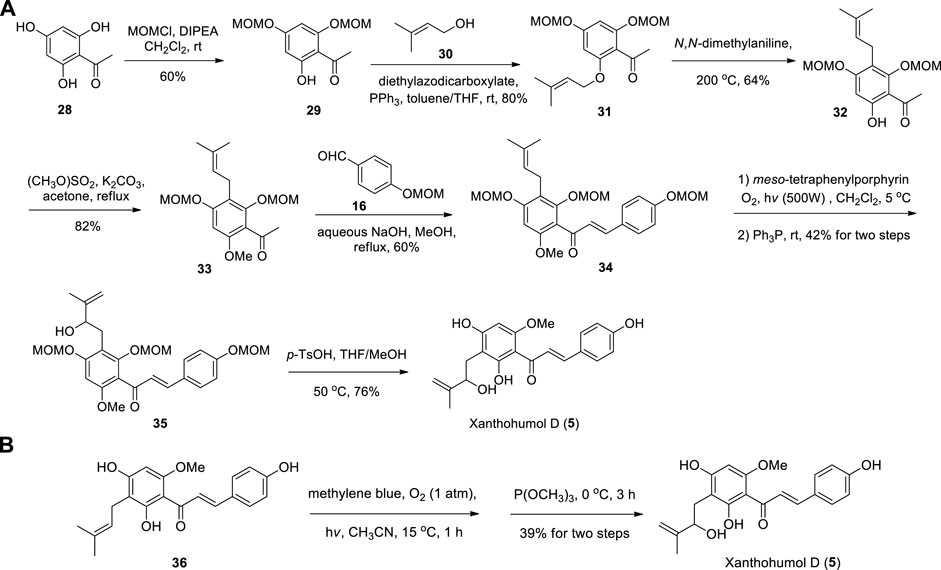
SCHEME 3. (A) Synthesis of Xanthohumol D by Fu et al.; (B) Synthesis of Xanthohumol D by Sugamoto et al.
2.5 Psorachalcone A (6)
2.5.1 Isolation and biological activities
In 2001, ElSohly et al. isolated 2′,4′,4,2″-tetrahydroxy-3′-[3″-methylbut-3″-enyl]-chalcone (6) from an ethanol extract of the leaves of Maclura tinctoria (L.) Gaud, but did not give it a Latin name. Chalcone 6 showed inhibitory activity against Candida albicans (IC50 = 15 μg/ml) and Cryptococcus neoformans (IC50 = 7 μg/ml) (ElSohly et al., 2001; Nowakowska, 2007). Li et al. (2002) evaluated the fatty acid synthase inhibitory activity of chalcone 6, and chalcone 6 exhibited marginal activity with IC50 of 46 μg/ml. Compound 6 was also isolated from the seeds of Psoralea corylifolia, and did not showed significant antibacterial activities against two pathogenic bacteria Staphylococcus aureus and S. epidermidis (MIC > 0.147 mM) (Yin et al., 2004). Ngadjui et al. (2005) isolated chalcone 6 from the twigs of Dorstenia angusticornis and Dorstenia barteri var. subtriangularis. Until 2005, Yu et al. isolated chalcone 6 and named it as Psorachalcone A (Yu et al., 2005; Xu et al., 2012). Zhai et al. evaluated the antiproliferative effects of synthetic Psorachalcone A against five cancer cells (PC-3, A375, PANC-1, A549 and MDA-MB-231), but no obvious inhibitory activity was observed (IC50 > 25 μM) (Zhai et al., 2019). Li et al. (2019) evaluated the antibacterial activities of synthetic Psorachalcone A against two Gram positive bacteria and two Gram negative bacteria, and Psorachalcone A showed slight activity towards Gram-positive bacteria (Staphylococcus aureus, MIC = 50 μg/ml; Bacillus subtilis, MIC = 25 μg/ml) but no obvious activity to Gram-negative bacteria (Escherichia coli and Pseudomonas aeruginosa, MIC > 200 μg/ml). In 2021, Psorachalcone A was isolated from the fruits of Morus nigra Linn., and did not exhibit obvious effect of inhibiting 3-phosphoglycerate dehydrogenase (Wang et al., 2021).
2.5.2 Chemical synthesis
The first synthesis of Psorachalcone A and its new analogues were achieved from 2,4-dihydroxyacetophenone (21) through six steps by Zhai et al. (2019). Methoxymethyl (MOM) was used to protect the C4′-hydroxy group of 21 selectively. And MOM-protected 37 was prenylated with bromide 22 to afford 38, which was further reacted with 39 to afford 40 by Claisen-Schmidt condensation. Then 40 was subjected to [1,3]-sigmatropic rearrangement, Schenck ene reaction and deprotection to obtain Psorachalcone A (Scheme 4A). Sugamoto et al. (2020) synthesized Psorachalcone A by using the same procedure employed for the synthesis of Xanthoangelol D from prenylated chalcone (43) (Scheme 4B).
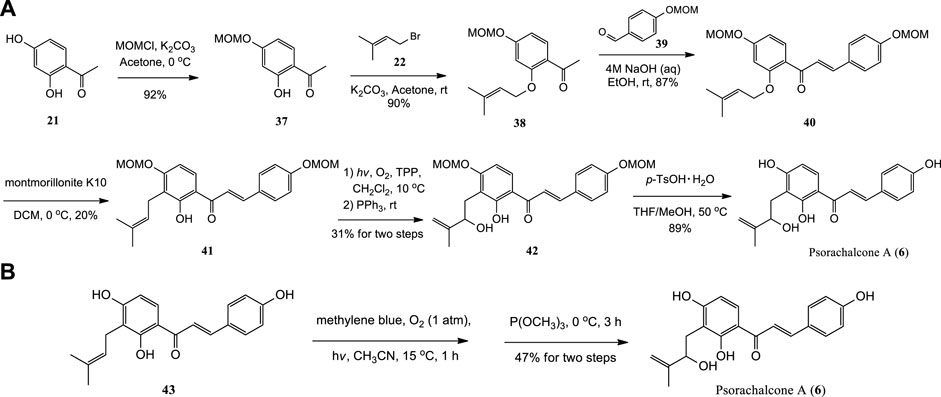
SCHEME 4. (A) Synthesis of Psorachalcone A by Zhai et al.; (B) Synthesis of Psorachalcone A by Sugamoto et al.
2.6 Bartericin A and D (7, 10)
2.6.1 Isolation and biological activities
Bartericin A and Bartericin D were isolated from the twigs of Dorstenia barteri var. subtriangularis successively, along with several other diprenylated chalcones (Ngameni et al., 2004; Ngadjui et al., 2005). (±)-Bartericin A obtained by the synthetic method was used to evaluate its antibacterial activity, and it showed moderate inhibitory activity against two Gram positive bacteria (Staphylococcus aureus and Bacillus subtilis, MIC = 25 μg/ml) (Fu et al., 2020).
2.6.2 Chemical synthesis
Fu et al. completed the first chemical synthesis of Bartericin A from the key intermediate 45 (Fu et al., 2020), and the preparation of 45 referred to the report of Dong et al. (Tan et al., 1999; Dong et al., 2007), which is similar to the synthetic steps of Paratocarpin E (Scheme 5). In addition, the chemical synthesis of Bartericin D has not been reported until now.
2.7 Angusticornin A and B (8, 9)
2.7.1 Isolation and biological activities
Angusticornin A and B were first isolated from the twigs of Dorstenia angusticornis and Dorstenia barteri var. subtriangularis (Ngadjui et al., 2005; Simo et al., 2005). According to the report by Kuete et al., Angusticornin B didn′t exhibit obvious antimicrobial activity against a serials of Gram-negative multidrug-resistant bacteria, but increased significantly against Escherichia coli AG100A (MIC values, 64 vs. 16 mg/L) in the presence of the efflux pump inhibitor phenylalanine arginine β-naphthylamide (20 mg/L) (Kuete et al., 2011). Angusticornin A and B obtained by the synthetic method were used to evaluate their antibacterial activities, and only Angusticornin A showed moderate inhibitory activity against Bacillus subtilis (MIC = 25 μg/ml) (Li et al., 2019; Fu et al., 2020).
2.7.2 Chemical synthesis
Angusticornin A was synthesized from methyl ketone 38 and aldehyde 48 (Damodar et al., 2017) through Claisen-Schmidt condensation, [1,3]-sigmatropic rearrangement, Schenck ene reaction and deprotection (Li et al., 2019) (Scheme 6A). Angusticornin B was prepared from natural product Stipulin (52) by Schenck ene reaction (Scheme 6B) Fu et al. (2020).
2.8 2′,6′-dihydroxy-3′-(2-hydroxy-3-methyl-3-butenyl)-4′-methoxychalcone (11)
2.8.1 Isolation and biological activities
Ren et al. isolated chalcone 11 from the whole plant of Anaphalis lactea (RenQi and Shi, 2008). The synthetic chalcone 11 was evaluated the antibacterial activities against two Gram positive bacteria (Staphylococcus aureus CMCC 26003, Bacillus subtilis CMCC(B) 63,501) and two Gram negative bacteria (Escherichia coli CMCC 44102, Pseudomonas aeruginosa CMCC(B) 10,104), but no obvious activity was observed in the four test strains (MIC > 200 μg/ml) (Fu et al., 2020).
2.8.2 Chemical synthesis
According to Fu et al., methoxymethylation of compound 53 (Grayfer et al., 2016), and then subjected to Claisen-Schmidt condensation, Schenck ene reaction, and demethoxymethylation of 56 to obtain chalcone 11 (Fu et al., 2020) (Scheme 7).
2.9 Sanjuanolide (12)
2.9.1 Isolation and biological activities
In 2016, Sanjuanolide was isolated from Dalea frutescens by Shaffer et al., and it exhibited slightly greater cytotoxic activities against PC-3 (IC50 = 11 ± 4 μM) and DU 145 (IC50 = 7 ± 3 μM) prostate cancer cell lines (Shaffer et al., 2016). Sanjuanolide was also isolated from the leaves and stem bark of Artocarpus integer in 2021 (Duong et al., 2021). Zhai et al. (2019) reported the anti-cancer activities of the synthetic Sanjuanolide against PC-3, A375, PANC-1, A549 and MDA-MB-231 cell lines, and Sanjuanolide showed moderate inhibitory activity against PC-3 (IC50 = 17.5 μM) and A375 (IC50 = 13.1 μM) cells. According to the report of Fang et al., (R)-Sanjuanolide efficiently inhibited the lipopolysaccharides-induced expression of tumor necrosis factor alpha and interleukin-6 (IC50 = 1.1 μM), but (S)-Sanjuanolide didn′t showed significant anti-inflammatory effect. Furthermore, (R)-Sanjuanolide effectively inhibited the mRNA expression of several inflammatory cytokines after the lipopolysaccharides challenge in vitro (Fang et al., 2019).
2.9.2 Chemical synthesis
Zhai et al. (2019) completed the total synthesis of (±)-Sanjuanolide from commercially available materials in seven steps (12% overall yield) (Scheme 8A), along with its seven new analogues. At the same time, Fang et al. completed the total synthesis of (±)-Sanjuanolide (Scheme 8B) in 15 steps with overall yield of 3.8%. In addition, (S)-Sanjuanolide and (R)-Sanjuanolide were also prepared in 17 steps with overall yields of 7.3% and 4.2%, respectively (Fang et al., 2019) (Scheme 8C).
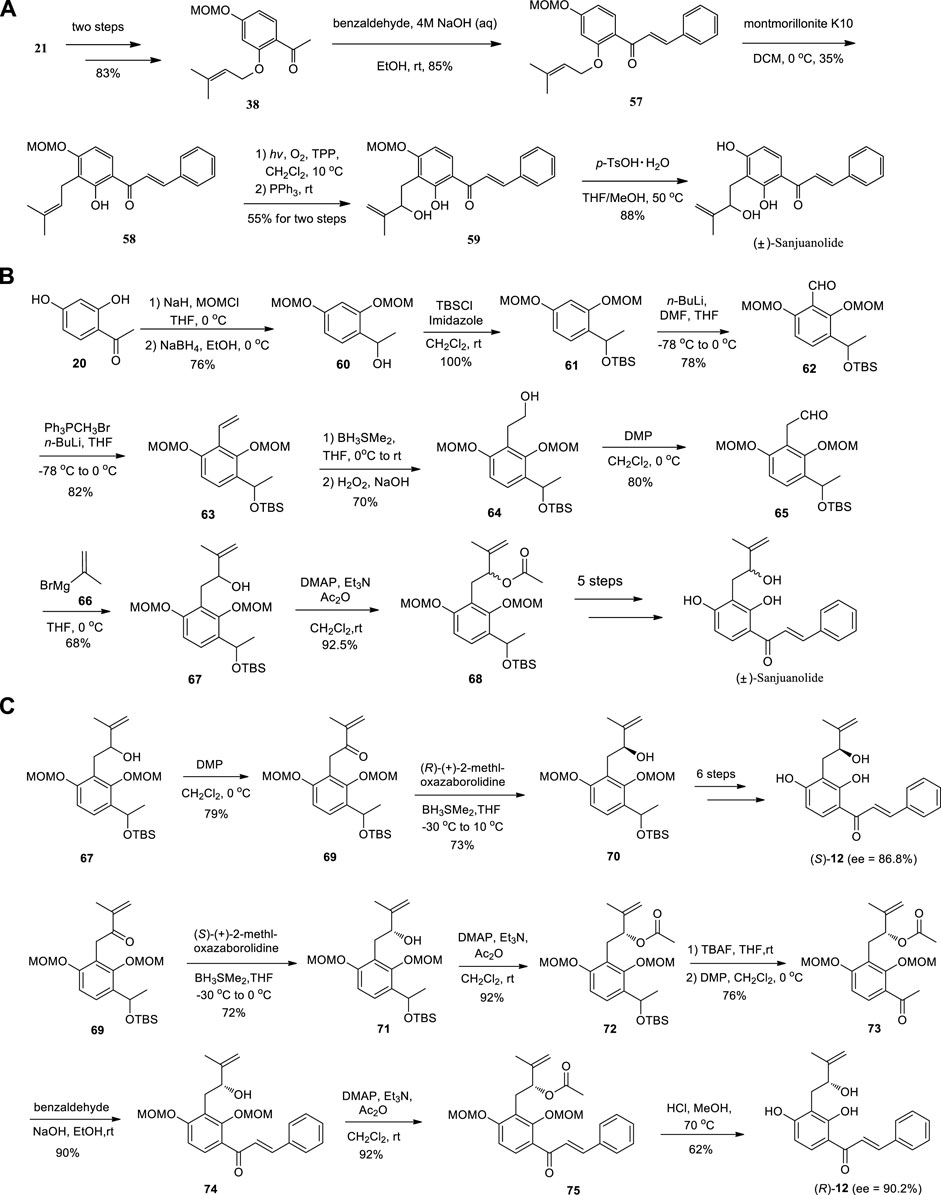
SCHEME 8. (A) Synthesis of (±)-Sanjuanolide by Zhai et al.; (B) Synthesis of (±)-Sanjuanolide by Fang et al.; (C) Synthesis of (S)-Sanjuanolide and (R)-Sanjuanolide by Fang et al.
2.10 2,2′,4,4′-tetrahydroxy-3-(2″-hydroxy-3″-methylbutyl-3″-alkenyl)chalcone (13)
Chalcone 13 was isolated from a Morus alba leaf by Yang et al., and it has a high free radical scavenging capacity while exhibits an IC50 of 21.6 μM against DPPH radicals (Yang and Jiang, 2021). In addition, no chemical synthesis of chalcone 13 has been reported so far.
3 Conclusion and outlook
Chalcone scaffolds, which is considered as the key bioactive precursors of plant flavonoids, have attracted more and more attention in medicinal chemistry and pharmacology. Herein, the isolation, chemical synthesis and biological activities of twelve natural chalcones and a chalcone-type compound bearing HMB group are reviewed. Although only a few dozen isolated or synthesized chalcones with HMB group have been reported, their various biological activities have aroused extensive interest of academic researchers, and it is believed that more and more natural or synthetic chalcones with HMB group will be presented in the further study. Furthermore, natural flavonoids with HMB group, which showed exciting biological activities, have also been reported by researchers in the past several decades, such as Ephedroidin and Dinklagin C (Lee et al., 1998; Pistelli et al., 1998; Xu et al., 2015; Owor et al., 2020). Moreover, further research on chalcones with HMB group might have much potential for drug discovery, especially as an adjuvant for a combination strategy between antibiotics and chalcones. And further studies on SAR, pharmacokinetics and toxicology are still needed, since the chemistry and biological importance of these biologically active compounds have not been systematically explored. Therefore, rational chemical derivatization of the natural chalcones and flavonoids bearing HMB group is necessary for further investigation of SAR, which play a key role in the screening of novel lead compounds.
Author contributions
JZ and BS: wrote the original draft. FS: conceived the topic and approved the final draft. All authors read and gave approval to the final draft.
Funding
This work was supported by the Natural Science Foundation of Shandong Province (No. ZR2021MB093), (No. ZR2021QH177) and (No. ZR2016HQ04).
Conflict of interest
The authors declare that the research was conducted in the absence of any commercial or financial relationships that could be construed as a potential conflict of interest.
Publisher’s note
All claims expressed in this article are solely those of the authors and do not necessarily represent those of their affiliated organizations, or those of the publisher, the editors and the reviewers. Any product that may be evaluated in this article, or claim that may be made by its manufacturer, is not guaranteed or endorsed by the publisher.
References
Al-Emam, A., Al-Shraim, M., Eid, R. A., Jamil, A.-M., Moustafa, M. F., and Radad, K. (2019). Cytotoxicity of Euphorbia peplus extract on MCF7 breast cancer cells. Folia Biol. (Praha). 67 (3), 127–139. doi:10.3409/fb_67-3.13
Baba, K., Nakata, K., Taniguchi, M., Kido, T., and Kozawa, M. (1990). Chalcones from Angelica keiskei. Phytochemistry 29 (12), 3907–3910. doi:10.1016/0031-9422(90)85357-L
Bocquet, L., Sahpaz, S., Hilbert, J. L., Rambaud, C., and Rivière, C. (2018). Humulus lupulus L., a very popular beer ingredient and medicinal plant: Overview of its phytochemistry, its bioactivity, and its biotechnology. Phytochem. Rev. 17, 1047–1090. doi:10.1007/s11101-018-9584-y
Chadwick, L. R., Nikolic, D., Burdette, J. E., Overk, C. R., Bolton, J. L., Breemen, R. B., et al. (2004). Estrogens and congeners from spent hops (Humulus lupulus). J. Nat. Prod. 67 (12), 2024–2032. doi:10.1021/np049783i
Chen, G., Huang, B. X., and Guo, M. (2018). Current advances in screening for bioactive components from medicinal plants by affinity ultrafiltration mass spectrometry. Phytochem. Anal. 29 (4), 375–386. doi:10.1002/pca.2769
Chesnokova, A. N., Lutskii, V. I., and Gorshkov, A. G. (2009). Prenylated chalcones from Humulus lupulus. Chem. Nat. Compd. 45 (5), 712–714. doi:10.1007/s10600-009-9424-9
Choi, Y., Jermihov, K., Nam, S.-J., Sturdy, M., Maloney, K., Qiu, X., et al. (2011). Screening natural products for inhibitors of quinone reductase-2 using ultrafiltration LC-MS. Anal. Chem. 83 (3), 1048–1052. doi:10.1021/ac1028424
Cieśla, Ł., and Moaddel, R. (2016). Comparison of analytical techniques for the identification of bioactive compounds from natural products. Nat. Prod. Rep. 33, 1131–1145. doi:10.1039/c6np00016a
Damodar, K., Kim, J.-K., and Jun, J.-G. (2017). Efficient, collective synthesis and nitric oxide inhibitory activity of rubrolides E, F, R, S and their derivatives. Tetrahedron Lett. 58 (1), 50–53. doi:10.1016/j.tetlet.2016.11.096
Dong, X., Fan, Y., Yu, L., and Hu, Y. (2007). Synthesis of four natural prenylflavonoids and their estrogen-like activities. Arch. Pharm. Weinh. 340 (7), 372–376. doi:10.1002/ardp.200700057
Duong, T.-H., Nguyen, H. T., Nguyen, C. H., Tran, N.-M.-A., Danova, A., Tran, T.-M.-D., et al. (2021). Identification of highly potent α-glucosidase inhibitors from Artocarpus integer and molecular docking studies. Chem. Biodivers. 18 (12), e2100499. doi:10.1002/cbdv.202100499
Duran, N., Polat, M. F., Aktas, D. A., Alagoz, M. A., Ay, E., Cimen, F., et al. (2021). New chalcone derivatives as effective against SARS-CoV-2 agent. Int. J. Clin. Pract. 75 (12), e14846. doi:10.1111/ijcp.14846
Duvauchelle, V., Majdi, C., Bénimélis, D., Dunyach-Remy, C., Meffre, P., and Benfodda, Z. (2021). Synthesis, structure elucidation, antibacterial activities, and synergistic effects of novel juglone and naphthazarin derivatives against clinical methicillin-resistant Staphylococcus aureus strains. Front. Chem. 9, 773981. doi:10.3389/fchem.2021.773981
ElSohly, H. N., Joshi, A. S., Nimrod, A. C., Walker, L. A., and Clark, A. M. (2001). Antifungal chalcones from Maclura tinctoria. Planta Med. 67 (1), 87–89. doi:10.1055/s-2001-10621
Fang, B., Xiao, Z., Qiu, Y., Shu, S., Chen, X., Chen, X., et al. (2019). Synthesis and anti-inflammatory evaluation of (R)-(S)-and (±)-Sanjuanolide isolated from Dalea frutescens. J. Nat. Prod. 82 (4), 748–755. doi:10.1021/acs.jnatprod.8b00596
Fu, L., Sun, B., Zhai, J., Li, Y., Liu, X., Song, R., et al. (2020). Synthesis and antibacterial activity study of natural 5'-hydroxyisoprenyl chalcones. Chin. J. Org. Chem. 40 (1), 201–208. doi:10.6023/cjoc201907027
Gao, S., Sun, D., Wang, G., Zhang, J., Jiang, Y., Li, G., et al. (2016). Growth inhibitory effect of Paratocarpin E, a prenylated chalcone isolated from Euphorbia humifusa Wild., by induction of autophagy and apoptosis in human breast cancer cells. Bioorg. Chem. 69, 121–128. doi:10.1016/j.bioorg.2016.10.005
Gomes, M. N., Muratov, E. N., Pereira, M., Peixoto, J. C., Rosseto, L. P., Cravo, P. V. L., et al. (2017). Chalcone derivatives: Promising starting points for drug design. Molecules 22 (8), 1210. doi:10.3390/molecules22081210
Grayfer, T. D., Grellier, P., Mouray, E., Dodd, R. H., Dubois, J., and Cariou, K. (2016). Mallotojaponins B and C: Total synthesis, antiparasitic evaluation, and preliminary SAR studies. Org. Lett. 18 (4), 708–711. doi:10.1021/acs.orglett.5b03676
Hano, Y., Itoh, N., Hanaoka, A., Itoh, Y., and Nomura, T. (1995). Paratocarpins A-E, five new isoprenoid-substituted chalcones from Paratocarpus venenose Zoll. Heterocycles 41 (1), 191–198. doi:10.3987/COM-94-6938
Ibraheem, S., Idris, Y. M. A., Mustafa, S., Kabeir, B., Abas, F., Maulidiani, M., et al. (2021). Phytochemical profile and biological activities of Sudanese baobab (Adansonia digitata L.) fruit pulp extract. Int. Food Res. J. 28 (1), 31–43. doi:10.47836/ifrj.28.1.03
Jasim, H. A., Nahar, L., Jasim, M. A., Moore, S. A., Ritchie, K. J., and Sarker, S. D. (2021). Chalcones: Synthetic chemistry follows where nature leads. Biomolecules 11 (8), 1203. doi:10.3390/biom11081203
Khupse, R. S., and Erhardt, P. W. (2007). Total synthesis of Xanthohumol. J. Nat. Prod. 70 (9), 1507–1509. doi:10.1021/np070158y
Kil, Y.-S., Choi, S.-K., Lee, Y.-S., Jafari, M., and Seo, E.-K. (2015). Chalcones from Angelica keiskei: Evaluation of their heat shock protein inducing activities. J. Nat. Prod. 78 (10), 2481–2487. doi:10.1021/acs.jnatprod.5b00633
Knockleby, J., Djigo, A. D., Lindamulage, I. K., Karthikeyan, C., Trivedi, P., and Lee, H. (2021). Lead optimization of novel quinolone chalcone compounds by a structure–activity relationship (SAR) study to increase efficacy and metabolic stability. Sci. Rep. 11, 21576. doi:10.1038/s41598-021-01058-z
Konieczny, M. T., Konieczny, W., Sabisz, M., Skladanowski, A., Wakieć, R., Augustynowicz-Kopeć, E., et al. (2007). Synthesis of isomeric, oxathiolone fused chalcones, and comparison of their activity toward various microorganisms and human cancer cells line. Chem. Pharm. Bull. 55 (5), 817–820. doi:10.1248/cpb.55.817
Kuete, V., Alibert-Franco, S., Eyong, K. O., Ngameni, B., Folefoc, G. N., Nguemeving, J. R., et al. (2011). Antibacterial activity of some natural products against bacteria expressing amultidrug-resistant phenotype. Int. J. Antimicrob. Agents 37 (2), 156–161. doi:10.1016/j.ijantimicag.2010.10.020
Lee, S.-J., Wood, A. R., Maier, C. G.-A., Dixon, R. A., and Mabry, T. J. (1998). Prenylated flavonoids from Maclura pomifera. Phytochemistry 49 (8), 2573–2577. doi:10.1016/S0031-9422(98)00380-X
Li, J.-L., Gao, L.-X., Meng, F.-W., Tang, C.-L., Zhang, R.-.J., Li, J.-Y., et al. (2015). PTP1B inhibitors from stems of Angelica keiskei (ashitaba). Bioorg. Med. Chem. Lett. 25 (10), 2028–2032. doi:10.1016/j.bmcl.2015.04.003
Li, X.-C., Joshi, A. S., ElSohly, H. N., Khan, S. I., Jacob, M. R., Zhang, Z., et al. (2002). Fatty acid synthase inhibitors from plants: Isolation, structure elucidation, and SAR studies. J. Nat. Prod. 65 (12), 1909–1914. doi:10.1021/np020289t
Li, Y., Sun, B., Zhai, J., Fu, L., Zhang, S., Zhang, J., et al. (2019). Synthesis and antibacterial activity of four natural chalcones and their derivatives. Tetrahedron Lett. 60 (43), 151165. doi:10.1016/j.tetlet.2019.151165
Liu, G., Eggler, A. L., Dietz, B. M., Mesecar, A. D., Bolton, J. L., Pezzuto, J. M., et al. (2005). Screening method for the discovery of potential cancer chemoprevention agents based on mass spectrometric detection of alkylated Keap1. Anal. Chem. 77 (19), 6407–6414. doi:10.1021/ac050892r
Liu, Y., Chen, H.-B., Qiao, L., and Zhao, Y.-Y. (2005). Two new isoprenyl chalcones from Hedysarum gmelinii. J. Asian Nat. Prod. Res. 7 (5), 723–727. doi:10.1080/1028602042000324925
Liu, Y., Zhang, J., Wen, R., Tu, G.-Z., Chen, H.-B., Liang, H., et al. (2018). Anti-inflammatory and antiproliferative prenylated chalcones from Hedysarum gmelinii. J. Asian Nat. Prod. Res. 20 (11), 1009–1018. doi:10.1080/10286020.2018.1450390
Ngadjui, B. T., Watchueng, J., Keumedjio, F., Ngameni, B., Simo, I. K., and Abegaz, B. M. (2005). Prenylated chalcones, flavone and other constituents of the twigs of Dorstenia angusticornis and Dorstenia barteri var. Phytochemistry 66 (6), 687–692. doi:10.1016/j.phytochem.2004.10.016
Ngameni, B., Ngadjui, B. T., Folefoc, G. N., Watchueng, J., and Abegaz, B. M. (2004). Diprenylated chalcones and other constituents from theTwigs of Dorstenia barteri var. Phytochemistry 65 (4), 427–432. doi:10.1016/j.phytochem.2003.10.021
Nielsen, S. F., Larsen, M., Boesen, T., Schønning, K., and Kromann, H. (2005). Cationic chalcone antibiotics. Design, synthesis, and mechanism of action. J. Med. Chem. 48 (7), 2667–2677. doi:10.1021/jm049424k
Nowakowska, Z. (2007). A review of anti-infective and anti-inflammatory chalcones. Eur. J. Med. Chem. 42 (2), 125–137. doi:10.1016/j.ejmech.2006.09.019
Ohkura, N., Ohnishi, K., Taniguchi, M., Nakayama, A., Usuba, Y., Fujita, M., et al. (2016). Anti-platelet effects of chalcones from Angelica keiskei Koidzumi (ashitaba) in vivo. Pharmazie 71 (11), 651–654. doi:10.1691/ph.2016.6678
Owor, R. O., Bedane, K. G., Zühlke, S., Derese, S., Ong′amo, G. O., Ndakala, A., et al. (2020). Anti-inflammatory flavanones and flavones from Tephrosia linearis. J. Nat. Prod. 83 (4), 996–1004. doi:10.1021/acs.jnatprod.9b00922
Park, J.-Y., Ko, J.-A., Kim, D. W., Kim, Y. M., Kwon, H.-J., Jeong, H. J., et al. (2015). Chalcones isolated from Angelica keiskei inhibit cysteine proteases of SARS-CoV. J. Enzyme Inhib. Med. Chem. 31 (1), 23–30. doi:10.3109/14756366.2014.1003215
Pistelli, L., Bertoli, A., Giachi, I., and Manunta, A. (1998). Flavonoids from Genista ephedroides. J. Nat. Prod. 61 (11), 1404–1406. doi:10.1021/np980112s
Pistelli, L., Spera, K., Flamini, G., Mele, S., and Morelli, I. (1996). Isoflavonoids and chalcones from Anthyllis hermanniae. Phytochemistry 42 (5), 1455–1458. doi:10.1016/0031-9422(96)00128-8
Qin, H.-L., Zhang, Z.-W., Lekkala, R., Alsulami, H., and Rakesh, K. P. (2020). Chalcone hybrids as privileged scaffolds in antimalarial drug discovery: A key review. Eur. J. Med. Chem. 193, 112215. doi:10.1016/j.ejmech.2020.112215
Ren, Z.-Y., Qi, H.-Y., and Shi, Y.-P. (2008). Phytochemical investigation of Anaphalis lactea. Planta Med. 74 (8), 859–863. doi:10.1055/s-2008-1074547
Rudrapal, M., Khan, J., Dukhyil, A. A. B., Alarousy, R. M. I. I., Attah, E, I., Sharma, T., et al. (2021). Chalcone scaffolds, bioprecursors of flavonoids: Chemistry, bioactivities, and pharmacokinetics. Molecules 26 (23), 7177. doi:10.3390/molecules26237177
Salehi, B., Quispe, C., Chamkhi, I., Omari, N. E., Balahbib, A., Sharifi-Rad, J., et al. (2021). Pharmacological properties of chalcones: A review of preclinical including molecular mechanisms and clinical evidence. Front. Pharmacol. 11, 592654. doi:10.3389/fphar.2020.592654
Sangiovanni, E., Fumagalli, M., Santagostini, L., Forino, M., Piazza, S., Colombo, E., et al. (2019). A bio-guided assessment of the anti-inflammatory activity of hop extracts (Humulus lupulus L. Cv. Cascade) in human gastric epithelial cells. J. Funct. Foods 57, 95–102. doi:10.1016/j.jff.2019.03.041
Shaffer, C. V., Cai, S., Peng, J., Robles, A. J., Hartley, R. M., Powell, D. R., et al. (2016). Texas native plants yield compounds with cytotoxic activities against prostate cancer cells. J. Nat. Prod. 79 (3), 531–540. doi:10.1021/acs.jnatprod.5b00908
Simo, I. K., Ngadjui, B. T., and Abegaz, B. M. (2005). Prenylated flavonoids and other constituents from the twigs of Dorstenia angusticornis. Biochem. Syst. Ecol. 33 (12), 1283–1287. doi:10.1016/j.bse.2005.07.015
Smit, F. J., and N′Da, D. D. (2014). Synthesis, in vitro antimalarial activity and cytotoxicity of novel 4-Aminoquinolinyl-chalcone amides. Bioorg. Med. Chem. 22 (3), 1128–1138. doi:10.1016/j.bmc.2013.12.032
Stevens, J. F., Taylor, A. W., Nickerson, G. B., Ivancic, M., Henning, J., Haunold, A., et al. (2000). Prenylflavonoid variation in Humulus lupulus: Distribution and taxonomic significance of xanthogalenol and 4'-O-methylxanthohumol. Phytochemistry 53 (7), 759–775. doi:10.1016/S0031-9422(00)00005-4
Sugamoto, K., Kurogi, C., Matsusita, Y.-I., and Matsui, T. (2008). Synthesis of 4-hydroxyderricin and related derivatives. Tetrahedron Lett. 49 (47), 6639–6641. doi:10.1016/j.tetlet.2008.09.015
Sugamoto, K., Matsusita, Y.-I., Matsui, K., Kurogi, C., and Matsui, T. (2011). Synthesis and antibacterial activity of chalcones bearing prenyl or geranyl groups from Angelica keiskei. Tetrahedron 67 (29), 5346–5359. doi:10.1016/j.tet.2011.04.104
Sugamoto, K., Yoshifuji, T., Soejima, S., and Honda, Y. (2020). Synthesis of chalcones bearing 2-Hydroperoxy-3-methyl-3-butenyl or 2-Hydroxy-3-methyl-3-butenyl group from prenylated chalcones. Synth. Commun. 50 (10), 1523–1527. doi:10.1080/00397911.2020.1745242
Sugii, M., Ohkita, M., Taniguchi, M., Baba, K., Kawai, Y., Tahara, C., et al. (2005). Xanthoangelol D isolated from the roots of Angelica keiskei inhibits endothelin-1 production through the suppression of nuclear factor-κb. Biol. Pharm. Bull. 28 (4), 607–610. doi:10.1248/bpb.28.607
Tan, W., Li, W., Huang, C., Li, Y., and Xing, Y. (1999). Facile syntheses of 3′, 4′-methylenedioxy- 2″, 2″-dimethylpyrano-[5″, 6″:7, 8]-flavoneand (±)-Ponganone III, two pyranoflavanoids. Synth. Commun. 29 (19), 3369–3377. doi:10.1080/00397919908085964
Tronina, T., Bartmańska, A., Filip-Psurska, B., Wietrzyk, J., Popłoński, J., and Huszcza, E. (2013). Fungal metabolites of Xanthohumol with potent antiproliferative activity on human cancer cell lines in vitro. Bioorg. Med. Chem. 21 (7), 2001–2006. doi:10.1016/j.bmc.2013.01.026
Wang, Y., Zheng, M., Jiang, Q., Xu, Y., Zhou, X., Zhang, N., et al. (2021). Chemical components of the fruits of Morus nigra Linn. Methyl caffeate as a potential anticancer agent by targeting 3-phosphoglycerate dehydrogenase. J. Agric. Food Chem. 69 (42), 12433–12444. doi:10.1021/acs.jafc.1c03215
Wei, H., Zhang, X., Tian, X., and Wu, G. (2016). Pharmaceutical applications of affinity-ultrafiltration mass spectrometry: Recent advances and future prospects. J. Pharm. Biomed. Anal. 131, 444–453. doi:10.1016/j.jpba.2016.09.021
Xu, M.-J., Wu, B., Ding, T., Chu, J.-H., Li, C.-Y., Zhang, J., et al. (2012). Simultaneous characterization of prenylated flavonoids and isoflavonoids in Psoralea corylifolia L. By liquid chromatography with diode-array detection and quadrupole time-of-flight mass spectrometry. Rapid Commun. Mass Spectrom. 26 (19), 2343–2358. doi:10.1002/rcm.6361
Xu, W.-H., Al-Rehaily, A. J., Yousaf, M., Ahmad, M. S., Khan, S., and Khan, I. A. (2015). Two new flavonoids from Retama raetam. Helv. Chim. Acta 98 (4), 561–568. doi:10.1002/hlca.201400315
Yang, B., and Jiang, Y. (2021). Chalcone compound and preparation method thereof. Pub. No.US 2021/0331995 A1. Date: Oct. 28, 2021.
Yin, S., Fan, C.-Q., Wang, Y., Dong, L., and Yue, J.-M. (2004). Antibacterial prenylflavone derivatives from Psoralea corylifolia, and their structure-activity relationship study. Bioorg. Med. Chem. 12 (16), 4387–4392. doi:10.1016/j.bmc.2004.06.014
Yu, L., Chen, Y., Liu, J., Lu, Y., and Gui, S. (2005). Chalcones from the seeds of Psoralea corylifolia. ChemInform 79 (7), 1173–1177. doi:10.1002/chin.200548207
Yu, L., Zhang, F., Hu, Z., Ding, H., Tang, H., Ma, Z., et al. (2014). Novel prenylated bichalcone and chalcone from Humulus lupulus and their quinone reductase induction activities. Fitoterapia 93, 115–120. doi:10.1016/j.fitote.2013.12.019
Yuan, L., Cai, Y., Zhang, L., Liu, S., Li, P., and Li, X. (2022). Promoting apoptosis, a promising way to treat breast cancer with natural products: A comprehensive review. Front. Pharmacol. 12, 801662. doi:10.3389/fphar.2021.801662
Zhai, J., Fu, L., Li, Y., Zhao, R., Wang, R., Deng, H., et al. (2019). Synthesis and biological activities evaluation of Sanjuanolide and its analogues. Bioorg. Med. Chem. Lett. 29 (2), 326–328. doi:10.1016/j.bmcl.2018.11.020
Zhang, C., Yue, H., Sun, P., Hua, L., Liang, S., Ou, Y., et al. (2021). Discovery of chalcone analogues as novel NLRP3 inflammasome inhibitors with potent anti-inflammation activities. Eur. J. Med. Chem. 219, 113417. doi:10.1016/j.ejmech.2021.113417
Zhao, F., Nozawa, H., Daikonnya, A., Kondo, K., and Kitanaka, S. (2003). Inhibitors of nitric oxide production from hops (Humulus lupulus L.). Biol. Pharm. Bull. 26 (1), 61–65. doi:10.1248/bpb.26.61
Zhao, F., Watanabe, Y., Nozawa, H., Daikonnya, A., Kondo, K., and Kitanaka, S. (2005). Prenylflavonoids and phloroglucinol derivatives from hops (Humulus lupulus). J. Nat. Prod. 68 (1), 43–49. doi:10.1021/np0499113
Zhou, K., Yang, S., and Li, S.-M. (2021). Naturally occurring prenylated chalcones from plants: Structural diversity, distribution, activities and biosynthesis. Nat. Prod. Rep. 38 (12), 2236–2260. doi:10.1039/d0np00083c
Keywords: chalcone, 2-hydroxy-3-methyl-3-butenyl, isolation, synthesis, biological activity
Citation: Zhai J, Sun B and Sang F (2022) Progress of isolation, chemical synthesis and biological activities of natural chalcones bearing 2-hydroxy-3-methyl-3-butenyl group. Front. Chem. 10:964089. doi: 10.3389/fchem.2022.964089
Received: 08 June 2022; Accepted: 20 July 2022;
Published: 15 August 2022.
Edited by:
Raghu Raj, DAV College Amritsar, IndiaReviewed by:
Vera L. M. Silva, University of Aveiro, PortugalSumit Kumar, University of Cape Town, South Africa
Copyright © 2022 Zhai, Sun and Sang. This is an open-access article distributed under the terms of the Creative Commons Attribution License (CC BY). The use, distribution or reproduction in other forums is permitted, provided the original author(s) and the copyright owner(s) are credited and that the original publication in this journal is cited, in accordance with accepted academic practice. No use, distribution or reproduction is permitted which does not comply with these terms.
*Correspondence: Feng Sang, ZmVuZ3NhbmdAc2R1dC5lZHUuY24=
†These authors have contributed equally to this work