- 1School of Chemistry and Environmental Engineering, Henan Key Laboratory of Germplasm Innovation and Utilization of Eco-economic Woody Plant, Pingdingshan University, Pingdingshan, China
- 2School of Pharmacy, Henan University of Chinese Medicine, Zhengzhou, China
- 3College of Information and Electronic Engineering, Hunan City University, Yiyang, China
MXenes and MXene-based composite materials have potential applications in a wide range of areas due to their unique physical and chemical characteristics. At present, it is still a major challenge to develop a simple, safe, and efficient route to prepare MXenes without using fluorinated etchants. Herein, we design a facile method to prepare Ti3C2Tx MXene sheets by selectively etching Ti3AlC2 powders in an aqueous diluted H2SO4 solution with H2O2 as an oxidant. In a system of H2SO4 and H2O2, an aqueous H2SO4 solution with a concentration of 6 mol/L is a strongly acidic medium with no volatility, and 30% H2O2 acts as a strong green oxidizer without harmful by-products. The experimental process is safe and convenient to conduct in a beaker under a water bath of 40°C. The etching process can be completed in 1 h under the air atmosphere conditions. The experimental results confirmed that the etched Ti3AlC2 powders can be successfully separated into Ti3C2Tx nanosheets under ultrasound treatment without using any intercalation agent. The relevant etching mechanism is may be attributed to the synergy effect of H2SO4 and H2O2, which triggers sequential selective etching of Al layers from the Ti3AlC2 phase. It may provide a new green way to prepare MXene-based materials without using toxic HF or HF-containing etchants.
Introduction
Since a new family of two-dimensional (2D) materials was first discovered in 2011 (Naguib et al., 2011), increasing attention has been paid to these novel 2D transition metal carbides, carbonitrides, and nitrides (named MXenes), and currently, more than 40 MXene compositions (Naguib et al., 2021) have been synthesized by different efficient routes (Naguib and Gogotsi, 2015; Alhabeb et al., 2017; Anasori et al., 2017; Tao et al., 2017; Zhou et al., 2017; Gogotsi and Anasori, 2019; Fan et al., 2022). The MAX phases are layered ternary carbides and nitrides with a general formula Mn+1AXn, where M represents transition metals (such as Sc, Ti, Zr, Hf, V, Nb, Ta, Cr, Mo, etc.), A represents elements from the group 13 and 14 of the periodic table, and X is carbon and/or nitrogen (Naguib and Gogotsi, 2015). After the discovery of Ti3C2Tx MXene in 2011 (Naguib et al., 2011), many MXenes were synthesized by selectively etching different precursors in liquid mediums such as HF, HF-containing, or HF-forming etchants; thus, these etching processes unavoidably caused some surface functional groups such as −O, −F, or −OH, marked as Tx in a general formula Mn+1XnTx for MXenes (Naguib and Gogotsi, 2015; Anasori et al., 2017; Tao et al., 2017; Zhou et al., 2017).
More importantly, MXenes and MXene-based composite materials have potential applications in various fields such as energy storage (Lukatskaya et al., 2013; Ghidiu et al., 2014; Liang et al., 2015; Wang et al., 2015; Luo et al., 2020; VahidMohammadi et al., 2021), flexible electronics (Xu et al., 2021a; Xu et al., 2021b; Shi et al., 2022a; Xu et al., 2022), electromagnetic shielding (Shahzad et al., 2016; Iqbal et al., 2020), catalysis (Seh et al., 2016; Liu et al., 2020), and water treatment (Ren et al., 2015) due to their unique physical and chemical properties (Halim et al., 2014; Dillon et al., 2016; Hantanasirisakul et al., 2016). In order to promote their potential applications, numerous research efforts have been carried out to explore the emerging etching methods and the stable storage of MXene, especially the large-scale preparing methods (Naguib and Gogotsi, 2015; Alhabeb et al., 2017; Gogotsi and Anasori, 2019; Fan et al., 2022).
In general, MXene nanosheets were synthesized by selectively etching MAX phases, as well as subsequent intercalation and delamination. In the early stage, the typical etching methods were dominated by HF etching methods (Naguib et al., 2011) and in situ HF-forming etching methods (Ghidiu et al., 2014; Halim et al., 2014). For example, Gogotsi et al. designed that the synthesis of MXene can be scaled up in a small-batch wet chemical etching process, and 1.0 g of the Ti3AlC2 powders was slowly peeled off in a system of HF and HCl for 24 h at 35°C (Shuck et al., 2020). Since 2017, fluorine-free etching methods such as electrochemical etching methods (Pang et al., 2019), alkali etching methods (Li et al., 2017; Li et al., 2018), molten salt etching methods (Li et al., 2019), halogen etching methods (Jawaid et al., 2021; Shi et al., 2021), and other methods (Ghazaly et al., 2021) were developed to prepare fluorine-free MXenes with different characteristics. For example, the electrochemical etching method (Pang et al., 2019) and alkali etching method (Li et al., 2018) can avoid the use of fluorinated etchants, and at the same time, the HF toxicity to the human body, corrosiveness, and harm to the environment can be effectively avoided. In 2019, a new method for MAX phase etching at a high temperature of 550°C was developed by using Lewis acid molten salt as an etching agent (Li et al., 2019). This method avoids the use of HF, HF-containing, or HF-forming etchants, but the high temperature is a necessary condition. In 2021, Song et al. developed a controllable HCl-hydrothermal etching strategy for Mo2CTx MXenes based on DFT calculation (Wang et al., 2021). In this process, Mo2CTx MXenes were prepared through a hydrothermal etching process with concentrated HCl in an autoclave at 120 or 140°C for 5 days. However, the high concentration, high temperature, and long etching time also impede its widespread use. More recently, Xiao et al. delicately exploited a low-temperature photo-Fenton strategy to fabricate F-free Ti3C2 with 95% high purity, and this work would play an important role in the F-free fabrication of MXene and synthesis of cathodes with excellent performance for flexible lithium-sulfur batteries (Liang et al., 2022).
In addition, the intercalation and delamination strategies of MXenes are mainly divided into organic intercalator delamination, inorganic intercalator delamination, and mechanical delamination (Mashtalir et al., 2013; Sang et al., 2016). The principle of intercalation and delamination is based on weakening the interlayer force (such as hydrogen bonding and van der Waals forces) among MXene nanosheets (Naguib et al., 2011; Alhabeb et al., 2017).
Although many successful synthetic routes and delamination routes of MXenes have been reported in the literature (Naguib et al., 2011; Alhabeb et al., 2017; Gogotsi and Anasori, 2019; Naguib et al., 2021; Fan et al., 2022), there are still a number of disadvantages such as the corrosion of etching agent to experimental equipment, harmful impact of reagents on the environment, and severe operating conditions. Therefore, it is highly desirable to develop a simple, safe, and efficient protocol for the synthesis of MXenes, and it is still a major challenge.
Inspired by the synthesis of MXenes through a controllable HCl-hydrothermal etching (Wang et al., 2021), a selective etching of MAX phase (Ti3SiC2) by using a solution of HF with oxidant (such as H2O2) (Alhabeb et al., 2018) and highly reactive radicals (HO• and O2•−) weakening the Ti–Al bonds in the MAX phase (Liang et al., 2022), we design a facile method to produce Ti3C2Tx MXenes by etching Ti3AlC2 powders in a system of H2SO4 and H2O2. In this system, an aqueous dilute H2SO4 solution is not volatile nor toxic, and H2O2 is a green oxidant; thus, this experimental process is safe and convenient to conduct in a beaker. The experimental results confirmed that Ti3C2Tx nanosheets can be successfully obtained, and this method is safe, rapid, and efficient.
Experimental section
Materials
Ti3AlC2 powders (98 wt% purity, 200 mesh) were purchased from Shanghai Rohn Reagent Co., Ltd. Sulfuric acid (H2SO4, 18 mol/L), hydrogen peroxide (H2O2, 30%), and sodium hydroxide (NaOH, analytical grade) were purchased from the National Pharmaceutical Reagent Company. All chemicals were used without further purification. Deionized water (a resistance of 18 MΩ) made from a Milli-Q solvent system was used throughout all the experiments.
Preparation of Ti3C2Tx Sheets
As a typical MAX phase, Ti3AlC2 powders were selected to be etched in a mixture of H2SO4 and H2O2 in our experiment.
1) Al layer etching. Typically, 2.0 g of Ti3AlC2 powders and 50 ml of H2SO4 solution (6 mol/L) were added into a beaker (200 ml) under electromagnetic stirring conditions. Then, 20 ml of H2O2 (30%) was slowly dropped into the abovementioned dispersion within 30 min under electromagnetic stirring conditions. A temperature-controlled water pot was used to control the temperature of 40 °C for this etching. Next, the obtained dispersion was still stirred at 40 °C for 30 min to further etch the Al layers from the Ti3AlC2 phase.
2) Sediment cleaning. After etching Al layers, the obtained dispersion was centrifuged at 3,000 rpm for 10 min with a high-speed centrifuge (Neofuge1600R) to obtain the sediment and recycle supernatant (including the etched Al layers and H2SO4 solution), respectively. The obtained sediment was cleaned with deionized water several times until the pH of the dispersion was close to 7. In addition, a nanocomposite of Al2O3 and TiO2 can be obtained from the supernatant by adding an appropriate amount of NaOH solution.
3) Ultrasonic stripping. A small amount of the obtained sediment was taken out and placed in a beaker with deionized water. No intercalation agent was required in this process. An ultrasonic cleaner was used to separate the obtained sediment in the beaker for 20 min to prepare Ti3C2Tx sheets with appropriate ultrasonic power (KQ-300GDV, frequency 40 kHz, output power 300 W, 50% amplitude). After ultrasonic treatment, the obtained aqueous solution of MXenes was filtered through a mixed cellulose ester microporous membrane using a water-circulating multi-purpose vacuum pump (SHB-III). Finally, the obtained sample was dried in a vacuum at 60°C for 24 h for characterizations.
Characterizations
The microstructure of Ti3AlC2 powders and Ti3C2Tx sheets were detected by scanning electron microscopy (SEM, Hitachi S-4800) and transmission electron microscopy (TEM, JEM-2100). The X-ray diffractometer (XRD, Bruker D8 diffractometer), Fourier transform infrared spectroscopy (FTIR, Nicolet5700), and X-ray photoelectron spectroscopy (XPS, K-alpha1063) were used to analyze the etching process and the relevant etching mechanism of Ti3AlC2 powders.
Results and discussion
Structure analysis
Based on the synthetic strategies of HCl-hydrothermal etching (Wang et al., 2021), HF/H2O2 oxidant-assisted etching (Alhabeb et al., 2018), and photo-Fenton radicals weakening, (Liang et al., 2022) a facile, safe, and rapid strategy was designed to prepare Ti3C2Tx MXenes by selectively etching Ti3AlC2 powders in an aqueous H2SO4 solution with H2O2 as oxidant. The detailed preparation process (including etching, cleaning, and stripping) was introduced in the experimental section, and the schematic procedure is shown in Figure 1A. The mixture of Ti3AlC2 powders and aqueous H2SO4 solution is black (Figure 1B), while an aqueous solution of Ti3C2Tx sheets without cleaning and stripping has a purple/magenta color (Naguib et al., 2011), as shown in Figure 1C. After cleaning and stripping, the resulting aqueous solution of Ti3C2Tx nanosheets presents a shallow green color (Figure 1D). Both the aqueous solutions have clear Tyndall effects under natural light (Li et al., 2017), indicating the presence of Ti3C2Tx nanosheets in two aqueous solutions, as shown in Figures 1C,D. Moreover, the resulting aqueous solution of Ti3C2Tx nanosheets can be kept for 90 days under the low temperature of 1–4°C without any color change.
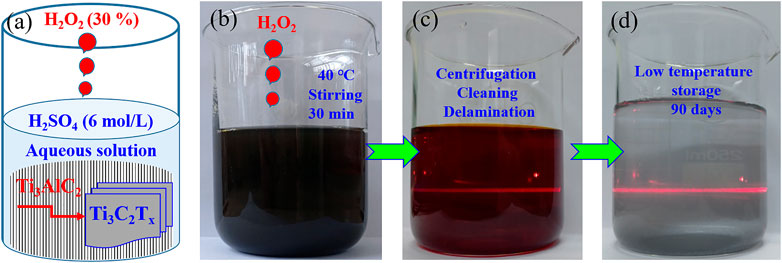
FIGURE 1. Ti3C2Tx nanosheets prepared by selective etching in a system of H2SO4 and H2O2. Schematic illustration of a preparation procedure in a beaker (A). Digital photographs of a mixture of Ti3AlC2 powders and aqueous H2SO4 solution (B), aqueous MXenes solution after Al layers etching (C), and aqueous MXenes solution after delamination (D).
In order to explore the etching effect, SEM images of the Ti3AlC2 powders and the obtained Ti3C2Tx sheets are carried out as contrast experiments. Figure 2 shows the microstructure of the Ti3AlC2 powders with different magnifications. It can be seen that the morphology and size of the Ti3AlC2 powders are very irregular (Figure 2B), and the individual particle has a compact layered structure, as shown in Figures 2C,D.
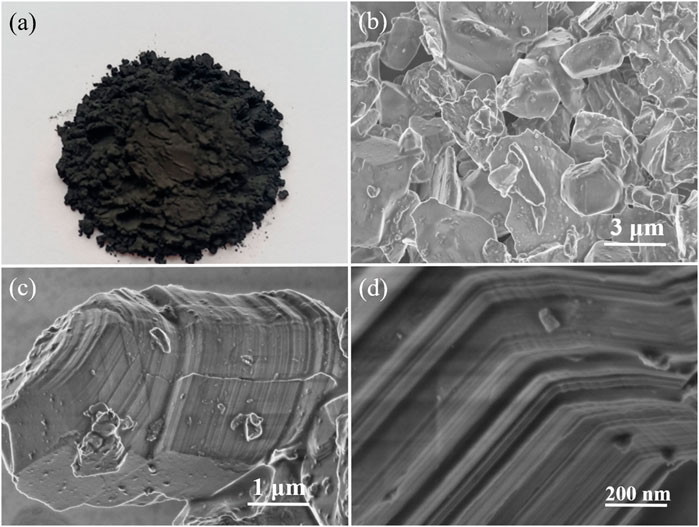
FIGURE 2. Digital photograph of the Ti3AlC2 powders (A), SEM images of the Ti3AlC2 powders with different magnifications (B–C), and magnified section SEM image of individual particle of Ti3AlC2 powders (D).
In contrast, SEM images in Figure 3 show the structural morphology of the obtained Ti3C2Tx sheets. The color of the obtained Ti3C2Tx MXene sheets is different from that of the Ti3AlC2 powders, namely, the colors change from black (Figure 2A) of the Ti3AlC2 powders to dark gray of the MXene sheets (Figure 3A). More importantly, the layered structure of the obtained Ti3C2Tx sheets is notably different from that of the Ti3AlC2 powders (Figures 2B–D), as clearly demonstrated in Figures 3B–D. Particularly, the structure of the obtained Ti3C2Tx sheets has some layered changes, and the lamellae have many hole defects, compared with that of the Ti3AlC2 powders; it is reasoned that when Al layers are etched away, a small amount of Ti layers is also etched out to leave holes and TiO2 nanoparticles on the surfaces due to the Ti vacancies triggering the oxidation process from Ti to TiO2, consistent with the results previous literature reported (Shi et al., 2022b; Huang et al., 2022; Jiang et al., 2022; Ma et al., 2022; Zhang et al., 2022).
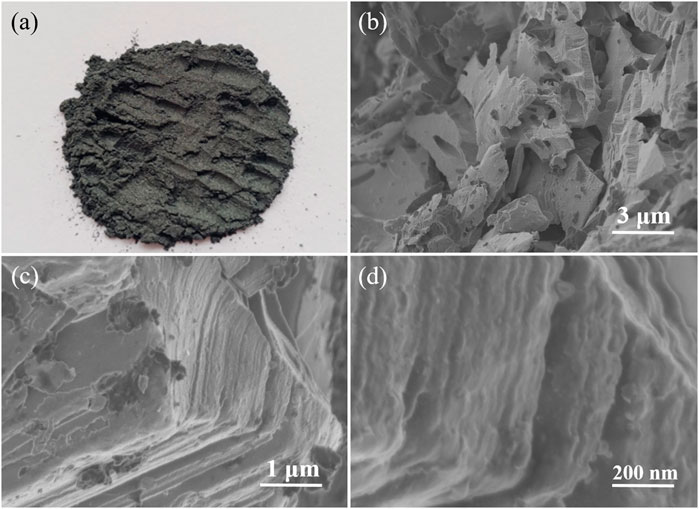
FIGURE 3. Digital photograph of Ti3C2Tx sheets (A), SEM images of Ti3C2Tx sheets obtained by etching Ti3AlC2 powders in the H2SO4/H2O2 solution with different magnifications (B–C), and magnified cross-section SEM image of Ti3C2Tx sheets (D).
To further demonstrate the morphology of Ti3C2Tx sheets, TEM imaging was performed after the obtained Ti3C2Tx sheets were treated by ultrasonic stripping in deionized water for 20 min. As shown in Figure 4, the obtained Ti3C2Tx nanosheets are single-layer or multilayer sheets with folded structures, consistent with the reported literature (Huang et al., 2022; Ma et al., 2022). A slight amount of TiO2 nanoparticles on the MXene surface can be ascribed to the Ti vacancies triggering the oxidation process from Ti to TiO2, consistent with SEM images in Figures 3B–D. Thus, the Ti3AlC2 powders can be easily stripped into Ti3C2Tx nanosheets by a simple oxidative etching and subsequent ultrasonic exfoliation in our experiments.
In order to analyze the etching process, the XRD patterns and FTIR spectra of the Ti3AlC2 powders and the Ti3C2Tx sheets were used to monitor their structural changes. From the distribution of the XRD peaks in Figure 5A, the Ti3AlC2 powders and Ti3C2Tx sheets have characteristic peaks at different positions, such as (002), (004), (101), (104), (105), and so on (Naguib et al., 2011; Alhabeb et al., 2017; Li et al., 2017; Li et al., 2018; Li et al., 2019). In particular, the XRD (002) peak of the Ti3C2Tx sheets is shifted toward a lower angle, namely, the peaks change from 9.75° of Ti3AlC2 powders to 9.30° of Ti3C2Tx sheets, indicating a larger spacing caused by etching Al atoms. Moreover, a certain amount of Ti3AlC2 is probably still present in Ti3C2Tx. From the FTIR spectra in Figure 5B, some new peaks appear, and they are very different from that of the pristine Ti3AlC2 powders. It can be found that there are lots of surface functional groups (Gao et al., 2021) such as 3,430 cm−1 of -OH, 1,620 cm−1 assigned to C=O, 1,400 cm−1 to the hydrogen bond of O-H, 1,090 cm−1 of C-O-C vibration, 790 cm−1 of the Ti-O bond (Wang et al., 2017), 610 cm−1 of SO42- vibration (Lin, 2015), and 460 cm−1 of Ti-C vibration (Wang et al., 2017). Thus, these new peaks of the FTIR spectrum may be attributed to the oxidation of H2O2 and the intercalation of SO42- (Lin, 2015; Wang et al., 2017; Gao et al., 2021).
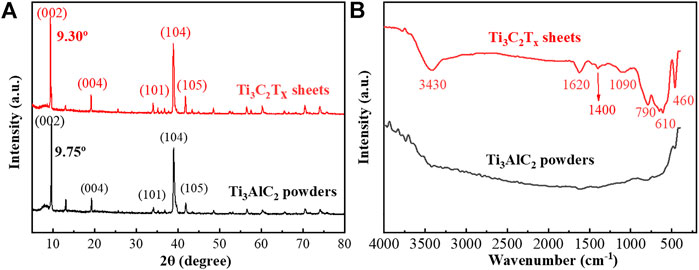
FIGURE 5. XRD patterns of Ti3AlC2 powders and Ti3C2Tx sheets (A), and FTIR spectra of Ti3AlC2 powders and Ti3C2Tx sheets (B).
In order to further explore the oxidation etching process, the XPS analysis of Ti3AlC2 powders and Ti3C2Tx sheets were performed to reveal their changes in chemical compositions. It can be found that the XPS survey spectra of Ti3C2Tx sheets present some new changes, such as a new peak of S 2p and a relatively elevated ratio of O 1s peak to C 1s peak (Figure 6A), compared with that of Ti3AlC2 powders.
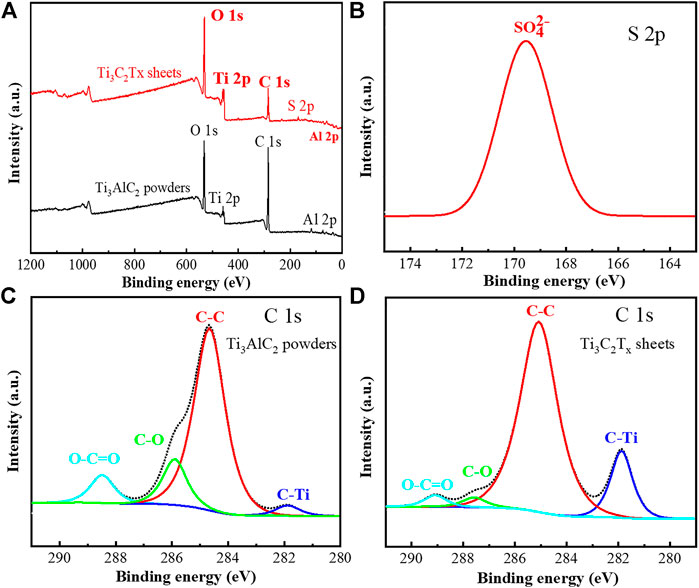
FIGURE 6. XPS survey spectra of Ti3AlC2 powders and Ti3C2Tx sheets (A), high-resolution S 2p XPS spectrum of Ti3C2Tx sheets (B), high-resolution C 1s XPS spectra of Ti3AlC2 powders and Ti3C2Tx sheets, respectively (C) and (D).
Moreover, the high-resolution S 2p XPS analysis (Figure 6B) confirms that the new peak (Park and Leitao, 2021) of S 2p is attributed to SO42-, consistent with the abovementioned FTIR analysis (Figure 5B). As shown in Figures 6C,D, the characteristic C 1s peaks of the Ti3AlC2 powders and Ti3C2Tx sheets also match those of previous work (Chen et al., 2020; Mathis et al., 2021), and the content ratio of C–Ti in the Ti3C2Tx sheets is higher than that of Ti3AlC2 powders, suggesting that most of Al atomic layers in Ti3C2Tx sheets have been selectively stripped.
The high-resolution Ti 2p XPS spectra of Ti3AlC2 powders and Ti3C2Tx sheets show three characteristic peaks, corresponding to the Ti-C 2p3/2, Ti-C 2p1/2, and Ti-O orbitals, respectively (Figures 7A,B), consistent with the current literature reports (Li et al., 2019; Chen et al., 2020; Mathis et al., 2021). Moreover, based on the analysis of XPS data, the content of Ti increases from 5.50 At% of Ti3AlC2 powders to 10.50 At% of Ti3C2Tx sheets.
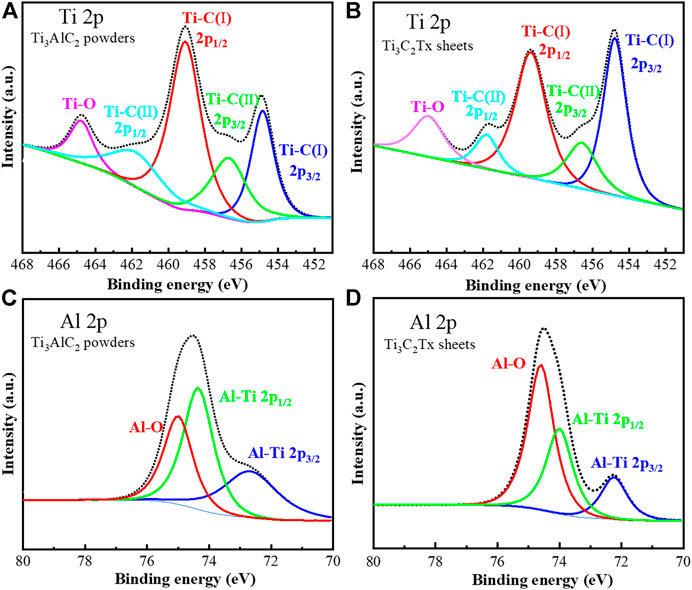
FIGURE 7. High-resolution XPS spectra of Ti 2p (A) and (B) and Al 2p (C) and (D) of Ti3AlC2 powders and Ti3C2Tx sheets, respectively.
On the other hand, the high-resolution Al 2p XPS spectra of Ti3AlC2 powders and Ti3C2Tx sheets show three characteristic peaks (Chen et al., 2020; Mathis et al., 2021) corresponding to the Al-Ti 2p3/2, Al-Ti 2p1/2, and Al-O orbitals, respectively (Figures 7C,D). In particular, the content ratio of Al-Ti 2p3/2 and Al-Ti 2p1/2 in the Ti3C2Tx sheets is significantly lower than that of Ti3AlC2 powders; in contrast, the content ratio of Al-O is higher. Based on the XPS analysis, the content of Al decreases from 6.50 At% of Ti3AlC2 powders to 1.50 At% of Ti3C2Tx sheets. Thus, from the abovementioned content changes of Ti and Al on the XPS analysis before and after corrosion, it can be reasoned that most of the Al layers can be etched out; as a result, the atomic percentage of Al decreases, and the atomic percentage of Ti increases in the Ti3C2Tx sheets.
Mechanism analysis
To analyze the selective etching process, some important factors should be considered as follows:
1) In the system of H2SO4 and H2O2, the aqueous solution H2SO4 of 6 mol/L is a strongly acidic medium with no volatility and 30% H2O2 acts as a strong green oxidizer without harmful by-products.
2) As the temperature is an important factor for the chemical reaction, to speed up the etching process, the temperature of the obtained mixture is heated by a temperature-controlled water pot. In our experiment, if the temperature is too high, it will accelerate the decomposition of H2O2, and 40°C is chosen as the appropriate temperature. Moreover, no notable reaction occurs when the Ti3AlC2 powders are only mixed with an H2SO4 solution of 6 mol/L, and it is reasoned that the oxidant of H2O2 plays a major role in the etching process. Particularly, the content of H2O2 is very crucial for the etching process; if the oxidant of H2O2 is excessive, there will be some TiO2 nanoparticles on the surface and interspace of MXene sheets because Ti can be oxidized to TiO2 by excessive H2O2 (Huang et al., 2022; Ma et al., 2022). Otherwise, a certain amount of Ti3AlC2 is still present in Ti3C2Tx without corrosion.
3) In the etching process, the microstructure change of intermediate sheets should be analyzed in detail. Seen from SEM images in Figures 8A,B, the obtained intermediate sheets show a loose, layered, and porous structure compared with Ti3AlC2 powders. This structure can be clearly demonstrated by the magnified parts of the selected area of the intermediate sheets (Figure 8B). Moreover, Figures 8C,D further reveal that the intermediate sheets are exfoliated along different directions, and lots of traces after stripping are left on the surface such as crack structures along longitudinal/transverse direction and TiO2 nanoparticles on the surface (marked in the red circle of Figure 8D). Thus, the obtained Ti3C2Tx nanosheets with clear layered and folded structures can be easily prepared after the intermediate sheets are stripped apart under ultrasonic treatment in deionized water (Figures 8E,F).
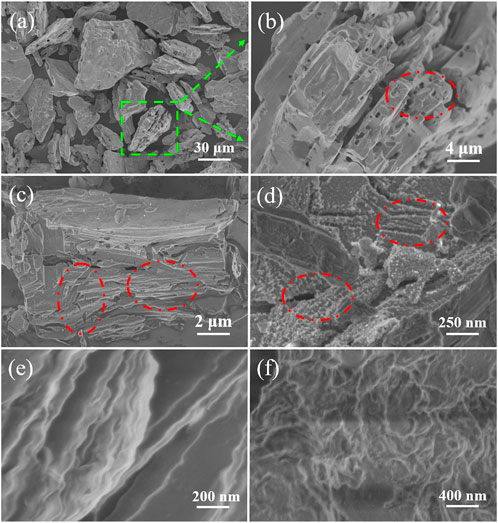
FIGURE 8. SEM images of intermediate sheets obtained by etching Ti3AlC2 powders in the H2SO4/H2O2 solution with different magnifications (A–D), and SEM images of Ti3C2Tx nanosheets with different magnifications (E–F).
Moreover, the Al2O3 and TiO2 nanocomposite (Figure 9A) can be obtained from the supernatant by adding a certain amount of NaOH solution, vacuum filtration, and drying. The precipitate of Al2O3 and TiO2 can be precipitated by only adding an alkaline solution, which suggests that Al3+ ions accompanied by a small amount of TiO2 are in the supernatant. As shown in Figure 9B, the XRD patterns of this nanocomposite are analyzed to prove the existence of Al2O3 and TiO2 nanoparticles, consistent with those reported in the literature (Han et al., 2012; Abazari et al., 2014; Ali et al., 2018).
In summary, the relevant etching mechanism is may be attributed to the synergy effect of H2SO4 and H2O2, which triggers Al layers to be etched sequentially from the MAX phase. It is speculated that the reactive oxygen species (such as HO• and O2•-) radicals can be produced because H2O2 is heated in the course of our experiment, very similar to the advanced oxidation process of the photo-Fenton reaction (Liang et al., 2022). Thus, the radicals can weaken the Ti–Al bonds and attack the defect sites of the external surfaces of MAX phases (Liu et al., 2020; Wei et al., 2021), and synchronously, Al layers are etched into Al3+ ions in the aqueous H2SO4 solution of 6 mol/L, and there is no accumulation of Al(OH)3 in this strongly acidic solution to retard this etching process. In addition, a lot of bubbles (such as O2 and H2) are produced to promote this etching process. Therefore, the synergy effect of H2SO4 and H2O2 triggers sequential selective etching of Al layers from the Ti3AlC2 phase.
On the other hand, abundant oxygen-containing functional groups are attached to the intermediate sheets, and Ti can be oxidized to TiO2 by excessive H2O2. Interestingly, the SO42- ions can be also inserted into the obtained Ti3C2Tx nanosheets and TiO2 nanoparticles can be in situ loaded on the surface of the obtained MXene nanosheets. The structure and morphology of the obtained MXene nanosheets can be further optimized by selecting the appropriate process such as the reaction rate and content of H2O2.
Conclusion
In conclusion, we develop a simple, safe, and efficient method to prepare MXene (Ti3C2Tx) nanosheets by selective etching Ti3AlC2 powders in a system of H2SO4/H2O2 and subsequent ultrasonic stripping. The obtained Ti3C2Tx nanosheets can be confirmed from the characterization analyses of SEM, TEM, XRD, FTIR, and XPS. Lots of oxygen-containing functional groups (−O, −OH) are supported on the MXene surface, the SO42- ions are inserted into the layers of the MXene sheets, and even TiO2 nanoparticles can be in situ loaded due to the oxidation of excessive H2O2. In the system of H2SO4/H2O2, the aqueous H2SO4 solution of 6 mol/L is a strongly acidic medium, and 30% H2O2 acts as a strong green oxidizer. The relevant etching mechanism may be attributed to the synergy effect of H2SO4 and H2O2, which triggers Al layers to be etched sequentially from the MAX phase. Our group is further exploring the etching mechanism. This work can provide a new way to develop HF-free and large-scale synthesis of 2D layered MXenes for many practical applications.
Data availability statement
The original contributions presented in the study are included in the article/Supplementary Material; further inquiries can be directed to the corresponding authors.
Author contributions
ZT and SB are responsible for designing and completing experiments. HT and KC are responsible for the preparation and characterization of materials. QP and YW are responsible for the literature search and review. QZ put forward constructive suggestions on the revision of the manuscript.
Funding
This work was supported by the Program for the High-Level Talents Start-up Fund (PXY-BSQD2016010) and the National Cultivation Fund (PXY-PYJJ2017001) of Pingdingshan University, the Natural Science Foundation of China (11904092), and the Hunan Provincial Natural Science Foundation (2019JJ50028).
Conflict of interest
The authors declare that the research was conducted in the absence of any commercial or financial relationships that could be construed as a potential conflict of interest.
Publisher’s note
All claims expressed in this article are solely those of the authors and do not necessarily represent those of their affiliated organizations, or those of the publisher, the editors, and the reviewers. Any product that may be evaluated in this article, or claim that may be made by its manufacturer, is not guaranteed or endorsed by the publisher.
References
Abazari, R., Mahjoub, A. R., and Sanati, S. (2014). A facile and efficient preparation of anatase titania nanoparticles in micelle nanoreactors: Morphology, structure, and their high photocatalytic activity under UV light illumination. RSC Adv. 4, 56406–56414. doi:10.1039/c4ra10018b
Alhabeb, M., Maleski, K., Anasori, B., Lelyukh, P., Clark, L., Sin, S., et al. (2017). Guidelines for synthesis and processing of two-dimensional Titanium carbide (Ti3C2Tx MXene). Chem. Mat. 29, 7633–7644. doi:10.1021/acs.chemmater.7b02847
Alhabeb, M., Maleski, K., Mathis, T. S., Sarycheva, A., Hatter, C. B., Uzun, S., et al. (2018). Selective etching of Silicon from Ti3SiC2 (MAX) to obtain 2D Titanium carbide (MXene). Angew. Chem. Int. Ed. Engl. 57, 5542–5546. doi:10.1002/ange.201802232
Ali, A. S., Mohammed, A. J., and Saud, H. R. (2018). Hydrothermal synthesis of TiO2/Al2O3 nanocomposite and its application as improved sonocatalyst. Int. J. Eng. Technol. 7, 22–25. doi:10.14419/ijet.v7i4.37.23607
Anasori, B., Lukatskaya, M. R., and Gogotsi, Y. (2017). 2D metal carbides and nitrides (MXenes) for energy storage. Nat. Rev. Mat. 2, 16098. doi:10.1038/natrevmats.2016.98
Chen, W. Y., Jiang, X., Lai, S-N., Peroulis, D., and Stanciu, L. (2020). Nanohybrids of a MXene and transition metal dichalcogenide for selective detection of volatile organic compounds. Nat. Commun. 11, 1302. doi:10.1038/s41467-020-15092-4
Dillon, A. D., Ghidiu, M. J., Krick, A. L., Griggs, J., May, S. J., Gogotsi, Y., et al. (2016). Highly conductive optical quality solution-processed films of 2D Titanium carbide. Adv. Funct. Mat. 26, 4162–4168. doi:10.1002/adfm.201600357
Fan, Y., Li, L., Zhang, Y., Zhang, X., Geng, D., Hu, W., et al. (2022). Recent advances in growth of Transition metal carbides and nitrides (MXenes) crystals. Adv. Funct. Mat. 32, 2111357. doi:10.1002/adfm.202111357
Gao, X., Wang, B., Wang, K., Xu, S., Liu, S., Liu, X., et al. (2021). Design of Ti3C2Tx/TiO2/PANI multi-layer composites for excellent electromagnetic wave absorption performance. J. Colloid Interface Sci. 583, 510–521. doi:10.1016/j.jcis.2020.09.094
Ghazaly, A. E., Ahmed, H., Rezk, A. R., Halim, J., Persson, Per O. Å., Yeo, L. Y., et al. (2021). Ultrafast, one-step, salt-solution-based acoustic synthesis of Ti3C2 MXene. ACS Nano 15, 4287–4293. doi:10.1021/acsnano.0c07242
Ghidiu, M., Lukatskaya, M. R., Zhao, M. Q., Gogotsi, Y., and Barsoum, M. W. (2014). Conductive two-dimensional Titanium carbide’clay’ with high volumetric capacitance. Nature 516, 78–81. doi:10.1038/nature13970
Gogotsi, Y., and Anasori, B. (2019). The rise of MXenes. ACS Nano 13, 8491–8494. doi:10.1021/acsnano.9b06394
Halim, J., Lukatskaya, M. R., Cook, K. M., Lu, J., Smith, C. R., Naslund, L. A., et al. (2014). Transparent conductive two-dimensional Titanium carbide epitaxial thin films. Chem. Mat. 26, 2374–2381. doi:10.1021/cm500641a
Han, C., Luque, R., and Dionysiou, D. D. (2012). Facile preparation of controllable size monodisperse anatase titania nanoparticles. Chem. Commun. 48, 1860–1862. doi:10.1039/c1cc16050h
Hantanasirisakul, K., Zhao, M. Q., Urbankowski, P., Halim, J., Anasori, B., Kota, S., et al. (2016). Fabrication of Ti3C2Tx MXene transparent thin films with tunable optoelectronic properties. Adv. Electron. Mat. 2, 1600050. doi:10.1002/aelm.201600050
Huang, Y., Lu, Q., Wu, D., Jiang, Y., Liu, Z., Chen, B., et al. (2022). Flexible MXene films for batteries and beyond. Carbon Energy 4, 598–620. doi:10.1002/cey2.200
Iqbal, A., Shahzad, F., Hantanasirisakul, K., Kim, M-K., Kwon, J., Hong, J., et al. (2020). Anomalous absorption of electromagnetic waves by 2D transition metal carbonitride Ti3CNTx (MXene). Science 369, 446–450. doi:10.1126/science.aba7977
Jawaid, A., Hassan, A., Neher, G., Nepal, D., Pachter, R., Kennedy, W. J., et al. (2021). Halogen etch of Ti3AlC2 MAX phase for MXene fabrication. ACS Nano 15, 2771–2777. doi:10.1021/acsnano.0c08630
Jiang, J., Bai, S., Zou, J., Liu, S., Hsu, J-P., Li, N., et al. (2022). Improving stability of MXenes. Nano Res. 15, 6551–6567. doi:10.1007/s12274-022-4312-8
Li, G., Tan, L., Zhang, Y., Wu, B., and Li, L. (2017). Highly efficiently delaminated single-layered MXene nanosheets with large lateral size. Langmuir 33, 9000–9006. doi:10.1021/acs.langmuir.7b01339
Li, M., Lu, J., Luo, K., Li, Y., Chang, K., Chen, K., et al. (2019). Element replacement approach by reaction with Lewis acidic molten salts to synthesize nanolaminated MAX phases and MXenes. J. Am. Chem. Soc. 141, 4730–4737. doi:10.1021/jacs.9b00574
Li, T., Yao, L., Liu, Q., Gu, J., Luo, R., Li, J., et al. (2018). Fluorine-free synthesis of high-purity Ti3C2Tx (T= OH, O) via alkali treatment. Angew. Chem. Int. Ed. 57, 6115–6119. doi:10.1002/anie.201800887
Liang, L., Niu, L., Wu, T., Zhou, D., and Xiao, Z. (2022). Fluorine-free fabrication of MXene via photo-Fenton approach for advanced lithium-sulfur batteries. ACS Nano 16, 7971–7981. doi:10.1021/acsnano.2c00779
Liang, X., Garsuch, A., and Nazar, L. F. (2015). Sulfur cathodes based on conductive MXene nanosheets for high-performance lithium-sulfur batteries. Angew. Chem. Int. Ed. Engl. 54, 3979–3983. doi:10.1002/ange.201410174
Lin, X. H. (2015). Impact of the spatial distribution of sulfate species on the activities of SO42−/TiO2 photocatalysts for the degradation of organic pollutants in reverse osmosis concentrate. Appl. Catal. B Environ. 170, 263–272. doi:10.1016/j.apcatb.2015.02.001
Liu, A., Liang, X., Ren, X., Guan, W., Gao, M., Yang, Y., et al. (2020). Recent progress in MXene-based materials: Potential high-performance electrocatalysts. Adv. Funct. Mat. 30, 2003437. doi:10.1002/adfm.202003437
Lukatskaya, M. R., Mashtalir, O., Ren, C. E., Dall’Agnese, Y., Rozier, P., Taberna, P. L., et al. (2013). Cation intercalation and high volumetric capacitance of two-dimensional Titanium carbide. Science 341, 1502–1505. doi:10.1126/science.1241488
Luo, J., Matios, E., Wang, H., Tao, X., and Li, W. (2020). Interfacial structure design of MXene-based nanomaterials for electrochemical energy storage and conversion. InfoMat 2, 1057–1076. doi:10.1002/inf2.12118
Ma, Y., Cheng, Y., Wang, J., Fu, S., Zhou, M., Yang, Y., et al. (2022). Flexible and highly-sensitive pressure sensor based on controllably oxidized MXene. InfoMat 4, e12328. doi:10.1002/inf2.12328
Mashtalir, O., Naguib, M., Mochalin, V. N., Dall’Agnese, Y., Heon, M., Barsoum, M. W., et al. (2013). Intercalation and delamination of layered carbides and carbonitrides. Nat. Commun. 4, 1716. doi:10.1038/ncomms2664
Mathis, T. S., Maleski, K., Goad, A., Sarycheva, A., Anayee, M., Foucher, A. C., et al. (2021). Modified MAX phase synthesis for environmentally stable and highly conductive Ti3C2 MXene. ACS Nano 15, 6420–6429. doi:10.1021/acsnano.0c08357
Naguib, M., Barsoum, M. W., and Gogotsi, Y. (2021). Ten years of progress in the synthesis and development of MXenes. Adv. Mat. 2021, 2103393. doi:10.1002/adma.202103393
Naguib, M., and Gogotsi, Y. (2015). Synthesis of two-dimensional materials by selective extraction. Acc. Chem. Res. 48, 128–135. doi:10.1021/ar500346b
Naguib, M., Kurtoglu, M., Presser, V., Lu, J., Niu, J., Heon, M., et al. (2011). Two-dimensional nanocrystals produced by exfoliation of Ti3AlC2. Adv. Mat. 23, 4248–4253. doi:10.1002/adma.201102306
Pang, S-Y., Wong, Y-T., Yuan, S., Liu, Y., Tsang, M-K., Yang, Z., et al. (2019). Universal strategy for HF-free facile and rapid synthesis of two-dimensional MXenes as multifunctional energy materials. J. Am. Chem. Soc. 141, 9610–9616. doi:10.1021/jacs.9b02578
Park, K. W., and Leitao, E. M. (2021). The link to polysulfides and their applications. Chem. Commun. 57, 3190–3202. doi:10.1039/d1cc00505g
Ren, C. E., Hatzell, K. B., Alhabeb, M., Ling, Z., Mahmoud, K. A., and Gogotsi, Y. (2015). Charge- and size-selective ion sieving through Ti3C2Tx MXene membranes. J. Phys. Chem. Lett. 6, 4026–4031. doi:10.1021/acs.jpclett.5b01895
Sang, X., Xie, Y., Lin, M. W., Alhabeb, M., Van Aken, K. L., Gogotsi, Y., et al. (2016). Atomic defects in monolayer Titanium carbide (Ti3C2Tx ) MXene. ACS Nano 10, 9193–9200. doi:10.1021/acsnano.6b05240
Seh, Z. W., Fredrickson, K. D., Anasori, B., Kibsgaard, J., Strickler, A. L., Lukatskaya, M. R., et al. (2016). Two-dimensional Molybdenum carbide (MXene) as an efficient electrocatalyst for hydrogen evolution. ACS Energy Lett. 1, 589–594. doi:10.1021/acsenergylett.6b00247
Shahzad, F., Alhabeb, M., Hatter, C. B., Anasori, B., Man Hong, S., Koo, C. M., et al. (2016). Electromagnetic interference shielding with 2D transition metal carbides (MXenes). Science 353, 1137–1140. doi:10.1126/science.aag2421
Shi, H., Zhang, P., Liu, Z., Park, S. W., Lohe, M. R., Wu, Y., et al. (2021). Ambient-stable two-dimensional Titanium carbide (MXene) enabled by Iodine etching. Angew. Chem. Int. Ed. Engl. 60, 8771–8775. doi:10.1002/ange.202015627
Shi, L-N., Cui, L-T., Ji, Y-R., Xie, Y., Zhu, Y-R., and Yi, T-F. (2022). Towards high-performance electrocatalysts: Activity optimization strategy of 2D MXenes-based nanomaterials for water-splitting. Coord. Chem. Rev. 469, 214668. doi:10.1016/j.ccr.2022.214668
Shi, Y., Zhou, D., Wu, T., and Xiao, Z. (2022). Deciphering the Sb4O5Cl2-MXene hybrid as a potential anode material for advanced potassium-ion batteries. ACS Appl. Mat. Interfaces 14, 29905–29915. doi:10.1021/acsami.2c06948
Shuck, C. E., Sarycheva, A., Anayee, M., Levitt, A., Zhu, Y., Uzun, S., et al. (2020). Scalable synthesis of Ti3C2Tx MXene. Adv. Eng. Mat. 2020, 1901241. doi:10.1002/adem.201901241
Tao, Q., Dahlqvist, M., Lu, J., Kota, S., Meshkian, R., Halim, J., et al. (2017). Two-dimensional Mo1.33C MXene with divacancy ordering prepared from parent 3D laminate with in-plane chemical ordering. Nat. Commun. 8, 14949. doi:10.1038/ncomms14949
VahidMohammadi, A., Rosen, J., and Gogotsi, Y. (2021). The world of two-dimensional carbides and nitrides (MXenes). Science 372, eabf1581. doi:10.1126/science.abf1581
Wang, C., Shou, H., Chen, S., Wei, S., Lin, Y., Zhang, P., et al. (2021). HCl-based hydrothermal etching strategy toward fluoride-free MXenes. Adv. Mat. 33, 2101015. doi:10.1002/adma.202101015
Wang, X., Kajiyama, S., Iinuma, H., Hosono, E., Oro, S., Moriguchi, I., et al. (2015). Pseudocapacitance of MXene nanosheets for high-power sodium-ion hybrid capacitors. Nat. Commun. 6, 6544. doi:10.1038/ncomms7544
Wang, Z., Xuan, J., Zhao, Z., Li, Q., and Geng, F. (2017). Versatile cutting method for producing fluorescent ultrasmall MXene sheets. ACS Nano 11, 11559–11565. doi:10.1021/acsnano.7b06476
Wei, Y., Zhang, P., Soomro, R. A., Zhu, Q., and Xu, B. (2021). Advances in the synthesis of 2D MXenes. Adv. Mat. 2021, 2103148. doi:10.1002/adma.202103148
Xu, M., Liang, L., Qi, J., Wu, T., Zhou, D., and Xiao, Z. (2021). Intralayered ostwald ripening‐induced self‐catalyzed growth of CNTs on MXene for robust lithium–sulfur batteries. Small 17, 2007446. doi:10.1002/smll.202007446
Xu, M., Wu, T., Qi, J., Zhou, D., and Xiao, Z. (2021). V2C/VO2 nanoribbon intertwined nanosheet dual heterostructure for highly flexible and robust lithium-sulfur batteries. J. Mat. Chem. A 9, 21429–21439. doi:10.1039/d1ta05693j
Xu, M., Zhou, D., Wu, T., Qi, J., Du, Q., and Xiao, Z. (2022). Self-regulation of spin polarization density propelling the ion diffusion kinetics for flexible potassium-ion batteries. Adv. Funct. Mat. 2022, 2203263. doi:10.1002/adfm.202203263
Zhang, M., Yang, D., Dong, C., Huang, H., Feng, G., Chen, Q., et al. (2022). Two-dimensional MXene-originated in situ nanosonosensitizer generation for augmented and synergistic sonodynamic tumor nanotherapy. ACS Nano 16, 9938–9952. doi:10.1021/acsnano.2c04630
Keywords: Ti3AlC2 powders, Ti3C2Tx sheets, H2SO4/H2O2 mixture, selective etching, HF-containing etchants
Citation: Tian Z, Tian H, Cao K, Bai S, Peng Q, Wang Y and Zhu Q (2022) Facile preparation of Ti3C2Tx sheets by selectively etching in a H2SO4/H2O2 mixture. Front. Chem. 10:962528. doi: 10.3389/fchem.2022.962528
Received: 06 June 2022; Accepted: 04 October 2022;
Published: 19 October 2022.
Edited by:
Qingzhi Wu, Wuhan University of Technology, ChinaCopyright © 2022 Tian, Tian, Cao, Bai, Peng, Wang and Zhu. This is an open-access article distributed under the terms of the Creative Commons Attribution License (CC BY). The use, distribution or reproduction in other forums is permitted, provided the original author(s) and the copyright owner(s) are credited and that the original publication in this journal is cited, in accordance with accepted academic practice. No use, distribution or reproduction is permitted which does not comply with these terms.
*Correspondence: Zhengshan Tian, tianzhengshan@163.com; Suzhen Bai, szb0211@163.com