- 1Mechanical Engineering Department, College of Engineering and Islamic Architecture, Umm Al-Qura University, Makkah, Saudi Arabia
- 2Department of Mathematics, Mohi-ud-Din Islamic University, Nerian Sharif, AJ&K, Pakistan
- 3Department of Mathematics, College of Science, King Khalid University, Abha, Saudi Arabia
- 4Research Center for Advanced Materials Science (RCAMS), King Khalid University, Abha, Saudi Arabia
- 5Faculty of Engineering and Technology, Future University in Egypt New Cairo, New Cairo, Egypt
- 6Department of Mathematics, College of Science and Humanities in Al-Kharj, Prince Sattam Bin Abdulaziz University, Al-Kharj, Saudi Arabia
- 7Department of Basic Engineering Science, Faculty of Engineering, Menoufia University, Shebin El-Kom, Egypt
- 8Department of Mathematics and Statistics, Hazara University, Mansehra, Pakistan
Nanofluids are a new generation of fluids which help in improving the efficiency of thermal systems by improving heat transport rate and extensive applications of this class extensively fall in biomedical engineering, the electronics industry, applied thermal and mechanical engineering, etc. The core concern of this study is to examine the interaction of Al2O3-Fe3O4 hybrid nanoparticles of lamina shaped with blood over a 3D surface by impinging novel impacts of non-linear thermal radiations, stretching, velocity slippage, and magnetic field. This leads to a mathematical flow model in terms of highly non-linear differential equations via nanofluid-effective characteristics and similarity rules. To know the actual behavior of (Al2O3-Fe3O4)/blood inside the concerned region, mathematical investigation is performed via numerical technique and the results are obtained for different parameter ranges. The imposed magnetic field of high strength is a better tool to control the motion of (Al2O3-Fe3O4)/blood inside the boundary layer, whereas, stretching of the surface is in direct proportion of the fluid movement. Furthermore, thermal radiations (Rd) and
Introduction
The world of nanotechnology is nothing without the investigation of the dynamics of nano and hybrid fluids (Alharbi et al., 2022). Now-a-days, we see that many researchers have come out with new technological ideas of a hybrid nanofluid, which is an upgraded version of common liquids. Hybrid nanofluids have high thermal conductivity due to the joint contribution of two types of nanoparticles. Therefore, researchers have seriously taken the analysis of such fluids from synthetization to applications and have performed the studies at a high level. Recently, Kashi et al. (Kashi et al., 2020) demonstrated the study of (Cu-Al2O3/water) in three dimensions over a slippage surface with uniform surface convection. Another imperative study related to the thermal behavior of Cu-Al2O3/water by taking flow assumptions on the surface was conveyed in (Khashi et al., 2020).
The transmission of heat in a hybrid nanoliquid with water as the base component and (Al2O3-Cu) hybrid nanoparticles was examined by Zainal et al. (Zaina et al., 2020). The inspection of Lorentz forces in the flow behavior over a 3D surface subject to resistive heating is done in Devi and Devi, 2016a. Hybrid nanofluids are a new generation of heat transport fluids with enriched energy storage ability. Therefore, Devi et al. (Devi and Devi, 2016b) reported a comparative heat transport performance of two nanofluids over a permeable surface. Recently, the stability analysis of (Cu-Al2O3/water) (Khan et al., 2022) over a non-linear shrinkable sheet and the heat dynamics under certain physical constraints is described in (Lund et al., 2019). Usman et al. (Usman et al., 2018) studied the significant effects of non-linear thermal radiations (Khan et al., 2021) with the contribution of thermal conductance on the temperature of nanoliquids and explored that the imposition of thermal radiation as an enriched natural source to boost the heat storage ability of the nanoliquids.
The investigation of momentum slippage and MHD (Ahmed, Adnan, and Mohyud-Din, 2020) on Cu-Al2O3/water nanofluid flow over a permeable stretching sheet is described in Wahid et al., 2020. Furthermore, a study of nanoliquids influenced by gravity is examined in Jamaludin et al., 2020. A hybrid nanofluid with surface temperature and Lorentz forces was examined by Prakash et al.,2016 and concluded that the hybrid nanofluid had better efficiency than traditional nanofluids. Another significant contribution in thermal enhancement is reported in Colak et al., 2020 to estimate the specific heat of Cu-Al2O3/water hybrid nanofluid based on temperature (T) and volume concentrations (φ). Mehryan et al. (Mehryan et al., 2017) studied the free convection thermal performance in a cavity with nanofluids and pointed out that the thermophysical attributes of the nanoparticles empower the thermal transport rate in nanoliquids.
Lund et al. (Lund et al., 2020) explored the thermal characteristics of Cu-Al2O3/water by considering MHD and viscous dissipation insights over a shrinkable sheet. Alshare et al. (Alshare et al., 2020) investigated the nano and hybrid nanofluid heat transport mechanisms in a periodic structure and found that enhancing % volume fraction will result increment in the temperature and frictional effects. A numerical analysis of an unsteady MHD mixed convection (Khan, Adnan, and Haleema, 2022) stagnation point flow heat transmission model (SPFM) for Cu-Al2O3/water over a 3D oriented geometry is described in Zainal et al., 2021. Nur et al. (Wahid et al., 2020) examined an analytic solution under slip momentum and thermal radiation influences on magnetized Cu-Al2O3/water nanofluid over a permeable stretching sheet (Adnan et al., 2022a). Force convection of turbulent flow of pure water, Al2O3/water nanofluid, and Cu-Al2O3/water hybrid nanofluid through a uniformly heated circular geometry is numerically analyzed in Takabi and Shokouhmand, 2015. Some other beneficial heat transport investigations in nanoliquids are reported in (Roy et al., 2020), (Adnan and Ahmed, 2022), (Leong et al., 2020), (Waqas et al., 2021), (Adnan and Ashraf, 2022a).
Jamshed et al. (Jamshad et al., 2021) studied the Casson nanofluid and examined the results for entropy and heat transport under solar thermal radiations. Sajid et al. (Sajid et al., 2021) studied the second law for a parabolic trough surface collector (PTSC) located inside solar aircraft wings, by taking the homo/heterogeneous reaction. Kashi et al. (Khashi et al., 2021) formulated the model for Cu-Al2O3/water hybrid nanofluid using the single-phase technique and reported a detailed analysis. Recent investigations were revealed in the studies by Adnan et al., 2020a, Adnan et al., 2022b, Adnan et al., 2020b, and Ahmed et al., 2017.
The study of electroosmotic silver/water nanoliquids in peristaltic geometry via two distinct approaches is done by Akram et al. (Akram et al., 2022a). They treated the developed model through different approaches and analyzed the dynamics due to fluctuating peristalsis parameters. An experimental analysis regarding the resistance of anti-microbes for gold nanoparticles is described by Habib and Akbar (Habib and Akbar, 2021) and the results are explained in a comprehensive manner. The exploration of an exact solution for various fluid dynamic heat transmission models is of great significance to examine the behavior of the temperature inside the concerned region. In this regard, a significant analysis is reported by Akbar et al. (Akbar et al., 2022). The impacts of thermal radiations on the nanoliquid whose components are CNTs and water are explained in detail. The entropy investigation in a new Rabinowitsch nanoliquid due to peristaltic pumping is discussed by Akram et al. (Akram et al., 2022b). Some recent and well-contributed studies in the area of applied fluid mechanics from various physical aspects (thermal radiations, magnetic field, heat sink/source inside the fluid, viscous dissipation, joule heating, etc.) of flow and geometry are elaborated in Akram and Akbar, 2020; Akram et al., 2020; Butt et al., 2020 at various spans of time.
The dynamics of nanoliquid in a curved channel with a thermophoretic movement are disclosed by Akram et al. (Akram et al., 2022c). The authors determined that higher buoyancy forces strengthened the temperature and facilitated the fluid movement. The temperature due to thermal radiations, resistive heating (Abbasi et al., 2017), and dissipation function in a nanoliquid prepared by gold and blood were analyzed by Sridhar et al. (Sridhar et al., 2022). The magnetic field (Akram et al., 2022d) is an important perspective from an industrial view point and broadly applicable in a variety of industries. Therefore, the researchers made several attempts to inspect the temperature transmission and fluid movement under a variety of nanoliquids by taking the flow in different regimes. Such important studies are described in the Refs. (Akram et al., 2022e; Tripathi et al., 2022), (Saleem et al., 2021), (Javid et al., 2021), and (Tripathi et al., 2021).
Model development
Model statement and geometry
Consider a three-dimensional, steady, laminar flow over a surface with modified slip boundaries. The flow is incompressible and subject to the magnetic field. Furthermore, thermal radiations are also imposed over the surface for better thermal performance of the nanofluids. The x and y axes are designated along the length and width of the stretching sheet, respectively; while the z-direction is taken perpendicular to the sheet. We take u, v, and w, as the velocity components along the x, y, and z directions, respectively. Furthermore, we imposed a magnetic field Bo with uniform strength and aligned along z-axis. The flow region for the used nanofluids is depicted in Figure 1.
The steady Prandtl boundary layer flow of the nanoliquid can be described by the following PDEs (Devi and Devi, 2016a), (Hayat et al., 2015):
The flow on the boundaries is specified to the following rules (Hayat et al., 2015):
The quantities appearing in the aforementioned governing laws are
Thermo-physical attributes for nano and hybrid fluids
The following are thermophysical attributes of nano and hybrid fluids utilized to modify the problem for (Al2O3-Fe3O4)/blood and Al2O3/blood over a desired 3D stretchable surface (Khan et al., 2017; Adnan and Ashraf, 2022b):
And
Electrical conductivity
In thermal conductivity correlations,
Similarity rules
The following similarity equations are designated to perform the dimensional analysis of the model:
And, the continuity in Eq. 1 is clearly satisfied in the view of aforementioned similarity equations.
Final (Al2O3-Fe3O4)/blood hybrid model
Using similarity transformation and thermo-physical characteristics in the governing model, the final version of the model is achieved:
With the boundary conditions,
Furthermore,
Mathematical analysis
The problem in hand is solved numerically due to the high strength of the non-linear terms and it is followed by the following steps:
• Firstly, write the model in its appropriate form.
• Make substitutions according to the order of model.
• Using those substitutions, the higher-order model should be transformed into first order IVP.
• Adjust the BCs accordingly and set those conditions equal to unknowns which will be determined latter.
• Finally, run the code and plot the results for various physical constraints.
The complete working rules for Runge-Kutta scheme are given in Figure 2.
By following the flow chart, the model (Al2O3-Fe3O4)/blood adjust in the following pattern:
Now according to the model, the following are appropriate transformations:
Eqs 11–13 are then combined in the following form:
After this, numerical computation is performed and furnished for the results for various physical constraints over the desired region.
Results and discussion against the physical constraints
The physical flow constraints are imperative to analyze the fluid motion and thermal behavior over a desired region. For the sake of this purpose, the results are organized to examine the hybrid nanofluid dynamics.
The velocity behavior of (Al2O3-Fe3O4)/blood and Al2O3/blood
Figure 3 demonstrates the velocity of [(Al2O3-Fe3O4)/blood]hnf and [(Al2O3)/blood]nf against the imposed magnetic field (Figure 3) aligned vertically to the plane of flow. The results reveal that the velocity (
The results for
Stretching of the surface is another physical aspect to observe the fluid movement over the region. Therefore, Figure 5 is furnished to examine the behavior of [(Al2O3-Fe3O4)/blood]hnf and [(Al2O3)/blood]nf. Very fascinating changes in the fluid movement are observed due to stretching of the surface. It is explored that the velocity
Thermal behavior of (Al2O3-Fe3O4)/blood and Al2O3/blood
The analysis of thermal enhancement in [(Al2O3-Fe3O4)/blood]hnf and [(Al2O3)/blood]nf is the heart of the study while dealing with nano and hybrid nanofluids. Therefore, a subsequent discussion is about the thermal enhancement in under consideration nanofluid with varying attributes of Rd,
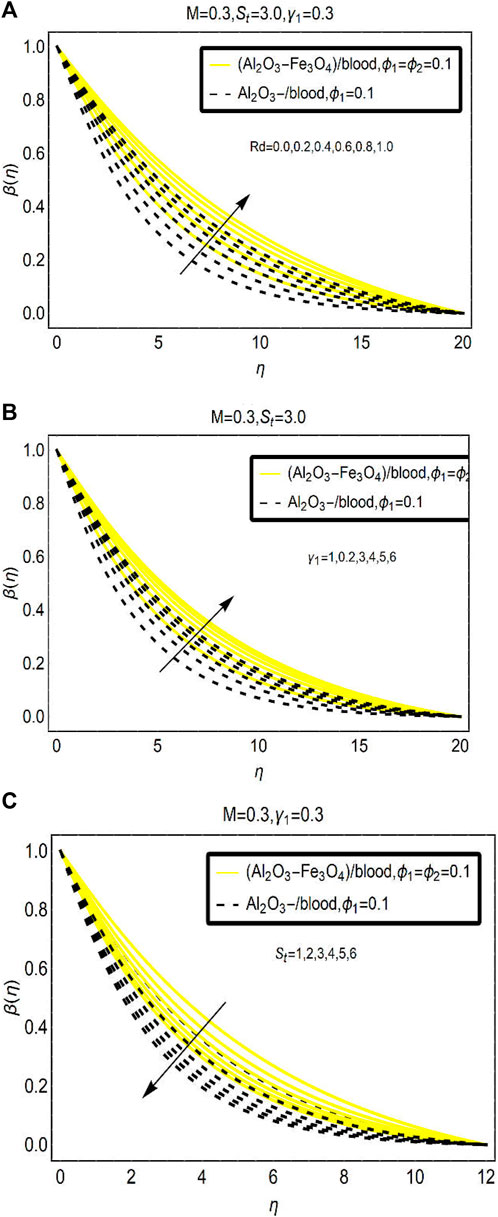
FIGURE 6. The temperature changes under varying (A) Rd (B)
From Figure 6A, it is analyzed that applied thermal radiations over the flow configuration is a very important parameter that significantly alters the temperature characteristics of [(Al2O3-Fe3O4)/blood]hnf and [(Al2O3)/blood]nf over a stretching radiated surface. Figure 6A discloses that the temperature increases by strengthening the applied thermal radiations (Rd). In [(Al2O3-Fe3O4)/blood]hnf, the temperature intensifies rapidly than conventional nanoliquid [(Al2O3)/blood]nf. Physically, thermal radiations and thermal conductivities of bi and mono nanoparticles in the base solvent (blood) improve thermal storage of the fluid. Therefore, the temperature enhances in both the nanoliquids. In a hybrid nanoliquid, the temperature changes are observed more rapidly than mono nanoliquid due to the difference between their thermal conductivities.
Similarly, Figure 6B and Figure 6C demonstrate the temperature alterations for
Quantities of practical interest and thermophysical attributes
The study of skin friction and local heat transport rate achieved much attention of the researchers, and more specifically, engineers because of their significant contribution in various engineering applications. Thus, the behavior of shear stresses and local heat transport rate for [(Al2O3-Fe3O4)/blood]hnf and [(Al2O3)/blood]nf over a radiated and slippery stretchable surface is pictured in Figures 7, 8, respectively. The parameters of interest in the particular model are the stretching surface ratio (
The analysis of Figure 8 ensures that the shear drag at the slippery surface upturns for a more stretchable and magnetized surface. The rapid growth of shear stresses is inspected for hybrid nanofluid [(Al2O3-Fe3O4)/blood]hnf than regular fluid [(Al2O3)/blood]nf. Being a denser solution, hybrid nanofluid has this characteristic whereas; shear stress decays for growing slippery effects and these are elaborated in Figure 9A. The behavior of the local heat transport rate due to imposed thermal radiation (Rd) is furnished in Figure 9B for both [(Al2O3-Fe3O4)/blood]hnf and [(Al2O3)/blood]nf. The results expose that induction of the thermal radiation in the constitutive model is an important physical aspect to enhance the heat transport rate in nanofluids.
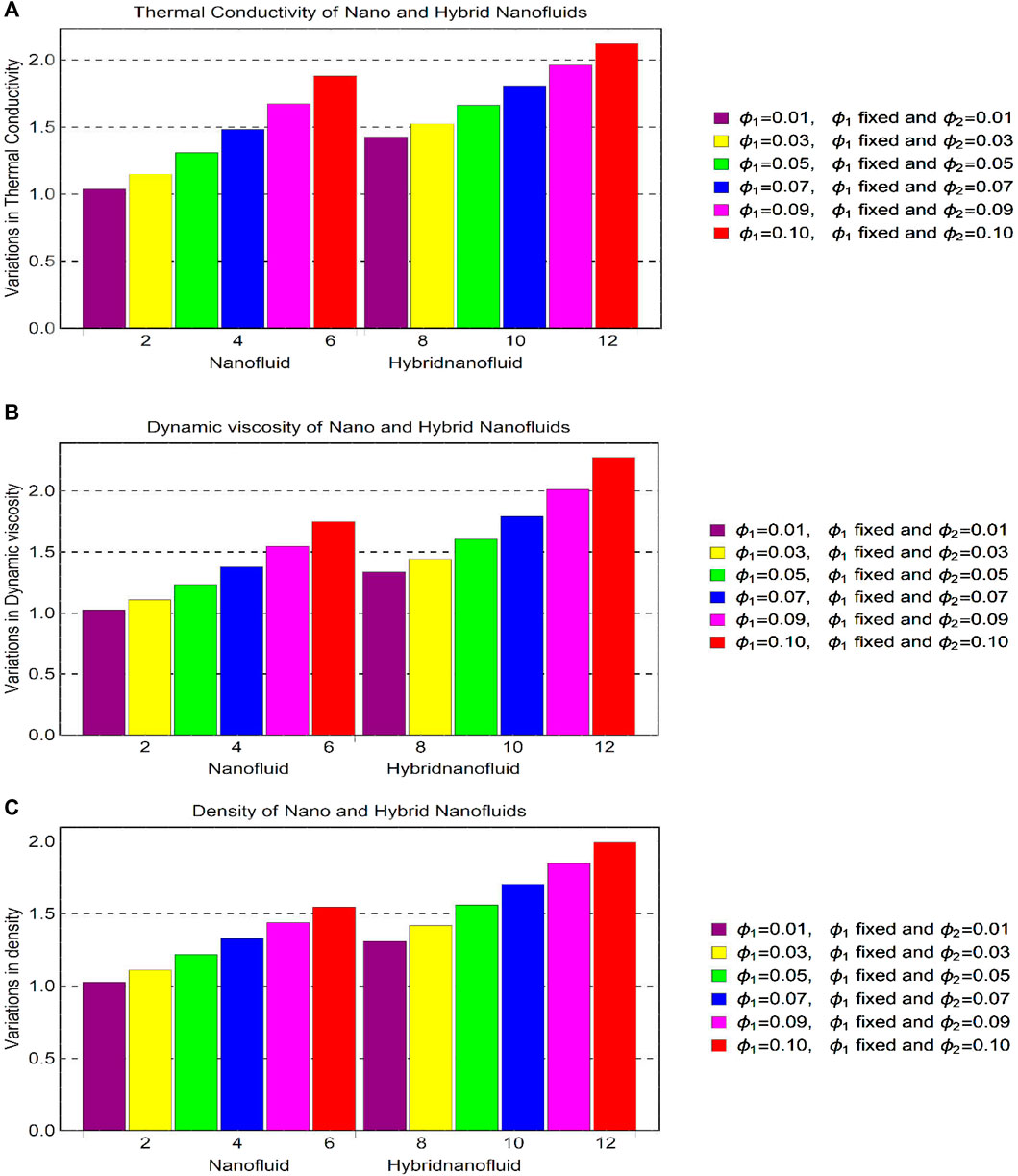
FIGURE 9. The changes in thermophysical values for (A) thermal conductivity (B) dynamic viscosity, and (C) density.
Thermophysical attributes of [(Al2O3-Fe3O4)/blood]hnf and [(Al2O3)/blood]nf knowingly depend on the thermophysical empirical correlations. Therefore, the behavior of these quantities due to volume fraction is depicted in Figure 9 for thermal conductance, dynamic viscosity, and density. It is inspected that, by the strengthen volume fraction within a reasonable domain, the thermophysical attributes increase which lead to a significant contribution in the nano and hybrid nanoliquids. It is also evident that, due to high thermal conductance of the hybrid nanoparticles, hybrid nanoliquid has much greater ability to store thermal energy.
Code and study of validation
The code and study validation with previously published data is an important factor in numerical investigation. Thus, the results of the model and code are validated with the data of Devi et al. (Devi and Devi, 2016a) by restricting the present model to some flow parameters. The comparative results for altering the stretching parameter (St = 0.0, 0.3, 0.6, 1.0) and fixed magnetic strength in Figure 10 are the evidence that the developed code and the results are valid and these can be replicated in the future.
Conclusion
The analysis of [(Al2O3-Fe3O4)/blood]hnf and [(Al2O3)/blood]nf over a 3D extendable surface is conducted. The heat transport problem formulation is carried out with proper utilization of similarity equations and thermophysical models of conventional and hybrid nanofluids. Thereafter, the model is analyzed systematically through a numerical technique and the results furnished for the parameters involved determined that:
• The movement of [(Al2O3-Fe3O4)/blood]hnf and [(Al2O3)/blood]nf could be controlled against the high strength of the magnetic field which is beneficial for industrial applications.
• The stretching parameter is useful for rapid movement of the nanofluids over a 3D surface.
• The heat transmission ability of [(Al2O3-Fe3O4)/blood]hnf due to the radiated surface is much higher than [(Al2O3)/blood]nf.
• The % volume concentration is a core factor in hybrid and common liquids particularly in the heat storage ability.
• The skin friction and Nusselt number upsurge by intensifying the strength of the magnetic field and thermal radiations, respectively.
• The interaction of oxide nanomaterials with blood as presented in the study will contribute potentially in the field of medical and biomedical engineering.
Data availability statement
The raw data supporting the conclusions of this article will be made available by the authors, without undue reservation. The data will be provided upon reasonable request.
Author contributions
All authors listed have made a substantial, direct, and intellectual contribution to the work and approved it for publication. Adnan and WA wrote original draft, UK done mathematical analysis, KG, ZR, EE, and ME-S potentially contributed revision, language editing and study validation.
Funding
The authors would like to thank the Deanship of Scientific Research at Umm Al-Qura University for supporting this work by Grant Code: 22UQU4331317DSR51. This work was supported by the King Khalid University through a Grant KKU/RCAMS/22 under the Research Center for Advanced Materials (RCAMS) at King Khalid University, Saudi Arabia.
Conflict of interest
The authors declare that the research was conducted in the absence of any commercial or financial relationships that could be construed as a potential conflict of interest.
Publisher’s note
All claims expressed in this article are solely those of the authors and do not necessarily represent those of their affiliated organizations, or those of the publisher, the editors, and the reviewers. Any product that may be evaluated in this article, or claim that may be made by its manufacturer, is not guaranteed or endorsed by the publisher.
References
Abbasi, A., Ahmed, N., and Mohyud-Din, S. T. (2017). Flow of Magneto-Nanofluid over a Thermally Stratified Bi-directional Stretching Sheet in the Presence of Ohmic Heating: A Numerical Study of Particle Shapes. Eng. Comput. Swans. 34 (8), 2499–2513. doi:10.1108/ec-04-2017-0127
Adnan, , , and Ashraf, W. (2022a). Numerical Thermal Featuring in γAl2O3-C2H6O2 Nanofluid under the Influence of Thermal Radiation and Convective Heat Condition by Inducing Novel Effects of Effective Prandtl Number Model (EPNM). Adv. Mech. Eng. 14, 168781322211065. doi:10.1177/16878132221106577
Adnan, , , and Ashraf, W. (2022b). Thermal Efficiency in Hybrid (Al2O3-CuO/H2O) and Ternary Hybrid Nanofluids (Al2O3-CuO-Cu/H2O) by Considering the Novel Effects of Imposed Magnetic Field and Convective Heat Condition. Waves Random Complex Media. doi:10.1080/17455030.2022.2092233
Adnan, S. Z. A., Khan, U., Abdeljawad, T., Ahmed, N., Khan, I., Nisar, K. S., et al. (2020). Investigation of Thermal Transport in Multi-Shaped Cu Nanomaterial-Based Nanofluids. Materials 13, 2737. doi:10.3390/ma13122737
Adnan, S. Z. A., Zaidi, U., Khan, N., Ahmed, Y., Chu, M., Khan, I., et al. (2020). Impacts of Freezing Temperature Based Thermal Conductivity on the Heat Transfer Gradient in Nanofluids: Applications for a Curved Riga Surface. Molecules 25, 2152. doi:10.3390/molecules25092152
Adnan, Khan, U., and Ahmed, N. (2022). Thermal Enhancement and Entropy Investigation in Dissipative ZnO-SAE50 under Thermal Radiation: a Computational Paradigm. Waves Random Complex Media. doi:10.1080/17455030.2022.2053243
Adnan, W. A., Andualem, M., and Khan, I. (2022). Thermal Transport Investigation and Shear Drag at Solid–Liquid Interface of Modified Permeable Radiative-SRID Subject to Darcy–Forchheimer Fluid Flow Composed by γ-nanomaterial. Sci. Rep. 12, 3564. doi:10.1038/s41598-022-07045-2
Adnan, W. Ashraf, Alghtani, A. H., Khan, I., and Andualem, M. (2022). Thermal Transport in Radiative Nanofluids by Considering the Influence of Convective Heat Condition. J. Nanomater. 2022, 1–11. doi:10.1155/2022/1854381
Ahmed, N., Abbasi, A., Saba, F., and Mohyud-Din, S. T. (2018). Flow of Ferro-Drmagnetic Nanoparticles in a Rotating System: a Numerical Investigation of Particle Shapes. Indian J. Phys. 92, 969–977. doi:10.1007/s12648-018-1186-4
Ahmed, N., Adnan, , , Khan, U., and Mohyud-Din, S. T. (2017). Influence of Thermal Radiation and Viscous Dissipation on Squeezed Flow of Water between Riga Plates Saturated with Carbon Nanotubes. Colloids Surfaces A Physicochem. Eng. Aspects 522, 389–398. doi:10.1016/j.colsurfa.2017.02.083
Ahmed, N., Adnan, , , Khan, U., and Mohyud-Din, S. T. (2020). Hidden Phenomena of MHD on 3D Squeezed Flow of Radiative-H2o Suspended by Aluminum Alloys Nanoparticles, Eur. Phys. J. Plus 135, 875. doi:10.1140/epjp/s13360-020-00870-2
Akbar, N. S., Maraj, E. N., Noor, N. F. M., and Habib, M. B. (2022). Exact Solutions of an Unsteady Thermal Conductive Pressure Driven Peristaltic Transport with Temperature-dependent Nanofluid Viscosity. Case Stud. Therm. Eng. 35, 102124. doi:10.1016/j.csite.2022.102124
Akram, J., Akbar, N. S., Alansari, M., and Tripathi, D. (2022). Electroosmotically Modulated Peristaltic Propulsion of TiO2/10W40 Nanofluid in Curved Microchannel. Int. Commun. Heat Mass Transf. 136, 106208. doi:10.1016/j.icheatmasstransfer.2022.106208
Akram, J., and Akbar, N. S. (2020). Biological Analysis of Carreau Nanofluid in an Endoscope with Variable Viscosity. Phys. Screen. 95, 055201–055205. doi:10.1088/1402-4896/ab74d7
Akram, J., Akbar, N. S., and Maraj, E. (2020). Chemical Reaction and Heat Source/sink Effect on Magnetonano Prandtl-Eyring Fluid Peristaltic Propulsion in an Inclined Symmetric Channel. Chin. J. Phys. 65, 300–313. doi:10.1016/j.cjph.2020.03.004
Akram, J., Akbar, N. S., and Tripathi, D. (2022). Analysis of Electroosmotic Flow of Silver-Water Nanofluid Regulated by Peristalsis Using Two Different Approaches for Nanofluid. J. Comput. Sci. 62, 101696. doi:10.1016/j.jocs.2022.101696
Akram, J., Akbar, N. S., and Tripathi, D. (2022). Electroosmosis Augmented MHD Peristaltic Transport of SWCNTs Suspension in Aqueous Media. J. Therm. Anal. Calorim. 147, 2509–2526. doi:10.1007/s10973-021-10562-3
Akram, J., Akbar, N. S., and Tripathi, D. (2022). Entropy Generation in Electroosmotically Aided Peristaltic Pumping of MoS2 Rabinowitsch Nanofluid. Fluid Dyn. Res. 54, 15507–15511. doi:10.1088/1873-7005/ac4e7b
Akram, J., Akbar, N. S., and Tripathi, D. (2022). Thermal Analysis on MHD Flow of Ethylene Glycol-Based BNNTs Nanofluids via Peristaltically Induced Electroosmotic Pumping in a Curved Microchannel. Arab. J. Sci. Eng. 47, 7487–7503. doi:10.1007/s13369-021-06173-7
Alharbi, K. A. M., Khan, U., Ahammad, N. A., Adnan, , , Ullah, B., Wahab, H. A., et al. (2022). Heat Transport Mechanism in Cu/water and (Cu–Al2O3)/water under the Influence of Thermophysical Characteristics and Non-linear Thermal Radiation for Blasius/Sakiadis Models: Numerical Investigation. J. Indian Chem. Soc. 99, 100578–8. doi:10.1016/j.jics.2022.100578
Alshare, A., Al-Kouz, W., and Khan, W. (2020). Cu-Al2O3Water Hybrid Nanofluid Transport in a Periodic Structure. Processes 8 (3), 285. doi:10.3390/pr8030285
Ashraf, W., Al-Johani, A. S., Khan, I., Andualem, M., Ahmed, N., Mohyud-Din, S. T., et al. (2022). Impact of Freezing Temperature (Tfr) of Al2O3 and Molecular Diameter (H2O)d on Thermal Enhancement in Magnetized and Radiative Nanofluid with Mixed Convection. Sci. Rep. 12, 703. doi:10.1038/s41598-021-04587-9
Butt, A. W., Akbar, N. S., and Mir, N. A. (2020). Heat Transfer Analysis of Peristaltic Flow of a Phan-Thien-Tanner Fluid Model Due to Metachronal Wave of Cilia. Biomech. Model. Mechanobiol. 19 (5), 1925–1933. doi:10.1007/s10237-020-01317-4
Colak, A. B., Yildiz, O., Bayrak, M., and Tezekici, B. S. (2020). Experimental Study for Predicting the Specific Heat of Water Based Cu-Al2O3 Hybrid Nanofluid Using Artificial Neural Network and Proposing New Correlation. Int. J. Energy Res. 44 (9), 7198–7215. doi:10.1002/er.5417
Devi, S. P. A., and Devi, S. S. U. (2016). Numerical Investigation of Hydromagnetic Hybrid Cu–Al2O3/Water Nanofluid Flow over a Permeable Stretching Sheet with Suction. Int. J. Nonlinear Sci. Numer. Simul. 17 (5), 249–257. doi:10.1515/ijnsns-2016-0037
Devi, S. U., and Devi, S. P. A. (2016). Numerical Investigation on Three Dimensional Hybrid Cu−Al2O3/Water Nanofluid Flow over a Stretching Sheet with Effecting Lorentz Force Subject to Newtonian Heating. Can. J. Phys. 94, 490–496. doi:10.1139/cjp-2015-0799
Habib, M. B., and Akbar, N. S. (2021). New Trends of Nanofluids to Combat Staphylococcus aureus in Clinical Isolates. J. Therm. Anal. Calorim. 143, 1893–1899. doi:10.1007/s10973-020-09502-4
Hayat, T., Imtiaz, M., Alsaedi, A., and Kutbi, M. A. (2015). MHD Three-Dimensional Flow of Nanofluid with Velocity Slip and Nonlinear Thermal Radiation. J. Magnetism Magnetic Mater. 396, 31–37. doi:10.1016/j.jmmm.2015.07.091
Hosseinzadeh, K., Asadi, A., Mogharrebi, A. R., Azari, M. E., and Ganji, D. D. (2021). Investigation of Mixture Fluid Suspended by Hybrid Nanoparticles over Vertical Cylinder by Considering Shape Factor Effect. J. Therm. Anal. Calorim. 143, 1081–1095. doi:10.1007/s10973-020-09347-x
Jamaludin, A., Naganthran, K., Nazar, R., and Pop, I. (2020). MHD Mixed Convection Stagnation-point Flow of Cu-Al2O3/water Hybrid Nanofluid over a Permeable Stretching/shrinking Surface with Heat Source/sink. Eur. J. Mech. - B/Fluids 84, 71–80. doi:10.1016/j.euromechflu.2020.05.017
Jamshad, W., Devi, S. U., Boukili, A., Nisar, K. S., Sooppy Nisar, K., Zakarya, M., et al. (2021). Evaluating the Unsteady Casson Nanofluid over a Stretching Sheet with Solar Thermal Radiation: An Optimal Case Study. Case Stud. Therm. Eng. 26, 101160–101210. doi:10.1016/j.csite.2021.101160
Javid, K., Hassan, M., Tripathi, D., Khan, S., Bobescu, E., and Bhatti, M. M. (2021). Double-diffusion Convective Biomimetic Flow of Nanofluid in a Complex Divergent Porous Wavy Medium under Magnetic Effects. J. Biol. Phys. 47, 477–498. doi:10.1007/s10867-021-09583-8
Kashi, N. S., Arifin, N. M., Pop, L., Nazar, R., Hafidzuddin, E. H., and Wahi, N. (2020). Three-Dimensional Hybrid Nanofluid Flow and Heat Transfer Past a Permeable Stretching/Shrinking Sheet with Velocity Slip and Convective Condition. Chin. J. Phys. 66, 157–171. doi:10.1016/j.cjph.2020.03.032
Khan, U., Abbasi, A., Ahmed, N., and Mohyud-Din, S. T. (2017). Particle Shape, Thermal Radiations, Viscous Dissipation and Joule Heating Effects on Flow of Magneto-Nanofluid in a Rotating System. Eng. Comput. Swans. 34 (8), 2479–2498. doi:10.1108/ec-04-2017-0149
Khan, U., Adnan, , , and Haleema, B. (2022). Thermal Performance in Nanofluid and Hybrid Nanofluid under the Influence of Mixed Convection and Viscous Dissipation: Numerical Investigation. Waves Random Complex Media. doi:10.1080/17455030.2022.2036389
Khan, U., Ullah, B., Wahab, H. A., Ullah, I., Almuqrin, M. A., and Khan, I. (2022). Comparative Thermal Transport Mechanism in Cu-H2o and Cu- Al2O3/H2O Nanofluids: Numerical Investigation. Waves Random Complex Media. doi:10.1080/17455030.2021.2023783
Khan, W., Khan, I., Fayz-Al-Asad, M., and Adnan, (2021). Applied Mathematical Modelling and Heat Transport Investigation in Hybrid Nanofluids under the Impact of Thermal Radiation: Numerical Analysis. Math. Problems Eng. 1, 10. doi:10.1155/2021/2180513
Khashi, N, S., Arifin, N, M., and Pop, I. (2020). Mixed Convective Stagnation Point Flow towards a Vertical Riga Plate in Hybrid Cu- Al2O3/Water Nanofluid. Mathematics 8 (6), 912. doi:10.3390/math8060912
Khashi, N. S., Arifin, N. M., Pop, I., Nazar, R., and Hafidzuddin, E. H. (2021). A New Similarity Solution with Stability Analysis for the Three-Dimensional Boundary Layer of Hybrid Nanofluids. Int. J. Numer. Methods Heat. Fluid Flow. 31 (9), 809–828. doi:10.1108/hff-04-2020-0200
Leong, L. S., Basie, M. F. M., Jaafar, N. A., Chaharborj, S. S., Khairuddin, T. K. A., and Naganthran, K. (2020). Numerical Solutions for the Thin Film Hybrid Nanofluid Flow and Heat Transfer over an Unsteady Stretching Sheet. Open J. Sci. Technol. 3, 4..
Lund, L. A., Omar, Z., Dero, S., Khan, I., Baleanu, D., and Nisar, K. S. (2020). Magnetized Flow of Cu+ Al2O3+ H2O Hybrid Nanofluid in Porous Medium: Analysis of Duality and Stability. Symmetry 12 (9), 1513. doi:10.3390/sym12091513
Lund, L. A., Omar, Z., Khan, I., Seikh, A. H., Sherif, E. S. M., and Nasir, A. S. (2019). Stability Analysis and Multiple Solution of Cu–Al2O3/H2O Nanofluid Contains Hybrid Nanomaterials over a Shrinking Surface in the Presence of Viscous Dissipation. J. Mater. Res. Technol. 9 (1), 421–432. doi:10.1016/j.jmrt.2019.10.071
Mehryan, S. A. M., Kashkooli, F. M., Ghalambaz, M., and Chamkha, A. (2017). Free Convection of Hybrid Al2O3-Cu Water Nanofluid in a Differentially Heated Porous Cavity. Adv. Powder Technol. 28 (9), 2295–2305. doi:10.1016/j.apt.2017.06.011
Prakash, M., Devil, S., and Uma, S. (2016). Hydromagnetic Hybrid Al2O3-Cu/Water Nanofluid Flow over a Slendering Stretching Sheet with Prescribed Surface Temperature. Asian J. Res. Soc. Sci. humanit. 6 (9), 1921–1936. doi:10.5958/2249-7315.2016.00915.1
Roy, N. C., Saha, L. K., and Sheikholeslami, M. (2020). Heat Transfer of a Hybrid Nanofluid Past a Circular Cylinder in the Presence of Thermal Radiation and Viscous Dissipation. AIP Adv. 10 (9), 095208.
Sajid, T., Jamshed, W., Shahzad, F., Boukili, A. E., Ez-Zahraouy, H., Nisar, K. S., et al. (2021). Study on Heat Transfer Aspects of Solar Aircraft Wings for the Case of Reiner-Philippoff Hybrid Nanofluid Past a Parabolic Trough: Keller Box Method. Phys. Screen. 96, 095220–095229. doi:10.1088/1402-4896/ac0a2a
Saleem, N., Munawar, S., and Tripathi, D. (2021). Entropy Analysis in Ciliary Transport of Radiated Hybrid Nanofluid in Presence of Electromagnetohydrodynamics and Activation Energy. Case Stud. Therm. Eng. 28, 101665. doi:10.1016/j.csite.2021.101665
Sridhar, V., Ramesh, K., Tripathi, D., and Vivekanand, V. (2022). Analysis of Thermal Radiation, Joule Heating, and Viscous Dissipation Effects on Blood-Gold Couple Stress Nanofluid Flow Driven by Electroosmosis. Heat. Trans. 51, 4080–4101. doi:10.1002/htj.22490
Takabi, B., and Shokouhmand, H. (2015). Effects of Al2O3–Cu/water Hybrid Nanofluid on Heat Transfer and Flow Characteristics in Turbulent Regime. Int. J. Mod. Phys. C 26, 1550047. doi:10.1142/S0129183115500473
Tripathi, D., Jayavel, P., Osman, A. B., and Srivastava, V. (2022). EMHD Casson Hybrid Nanofluid Flow over an Exponentially Accelerated Rotating Porous Surface. J. Porous Media. doi:10.1615/JPorMedia.2022041050
Tripathi, D., Prakash, J., Reddy, M. G., and Kumar, R. (2021). Numerical Study of Electroosmosis-Induced Alterations in Peristaltic Pumping of Couple Stress Hybrid Nanofluids through Microchannel. Indian J. Phys. 95, 2411–2421. doi:10.1007/s12648-020-01906-0
Usman, M., Zubair, T., Haq, R., and Wang, W. (2018). Cu-Al2O3/Water Hybrid Nanofluid through a Permeable Surface in the Presence of Nonlinear Radiation and Variable Thermal Conductivity via LSM. Int. J. Heat Mass Transf. 126, 1347–1356. doi:10.1016/j.ijheatmasstransfer.2018.06.005
Wahid, N. S., Arifin, N. M., Turkyilmazoglu, M., Hafidzuddin, M. E. H., and Rahmin, N. A. A. (2020). MHD Hybrid Cu-Al2O3/Water Nanofluid Flow with Thermal Radiation and Partial Slip Past a Permeable Stretching Surface: Analytical Solution. JNanoR. 64, 75–91. doi:10.4028/www.scientific.net/jnanor.64.75
Waqas, H., Farooq, U., Alghamdi, M., Muhammad, T., and Alshomrani, A. S. (2021). On the Magnetized 3D Flow of Hybrid Nanofluids Utilizing Nonlinear Radiative Heat Transfer. Phys. Screen. 96 (9), 095202. doi:10.1088/1402-4896/ac0272
Zaina, N. K., Nazar, R., Naganthran, K., and Pop, I. (2020). Impact of Anisotropic Slip on the Stagnation-point Flow Past a Stretching/shrinking Surface of the Al2O3-Cu/H2O Hybrid Nanofluid. Appl. Math. Mech. 41, 1401–1416. doi:10.1007/s10483-020-2642-6
Keywords: thermal enhancement, Al2O3-Fe3O4 hybrid nanoparticles, blood, thermal radiation, slip boundaries
Citation: Guedri K, Adnan , Raizah Z, Eldin ET, EL-Shorbagy MA, Abbas W and Khan U (2022) Thermal mechanism in magneto radiated [(Al2O3-Fe3O4)/blood]hnf over a 3D surface: Applications in Biomedical Engineering. Front. Chem. 10:960349. doi: 10.3389/fchem.2022.960349
Received: 02 June 2022; Accepted: 26 July 2022;
Published: 06 October 2022.
Edited by:
Karthik Ramasamy, UbiQD, Inc., United StatesReviewed by:
Noreen Sher Akbar, National University of Sciences and Technology (NUST), PakistanDharmendra Tripathi, National Institute of Technology Delhi, India
Copyright © 2022 Guedri, Adnan, Raizah, Eldin, EL-Shorbagy, Abbas and Khan. This is an open-access article distributed under the terms of the Creative Commons Attribution License (CC BY). The use, distribution or reproduction in other forums is permitted, provided the original author(s) and the copyright owner(s) are credited and that the original publication in this journal is cited, in accordance with accepted academic practice. No use, distribution or reproduction is permitted which does not comply with these terms.
*Correspondence: Adnan, adnan_abbasi89@yahoo.com