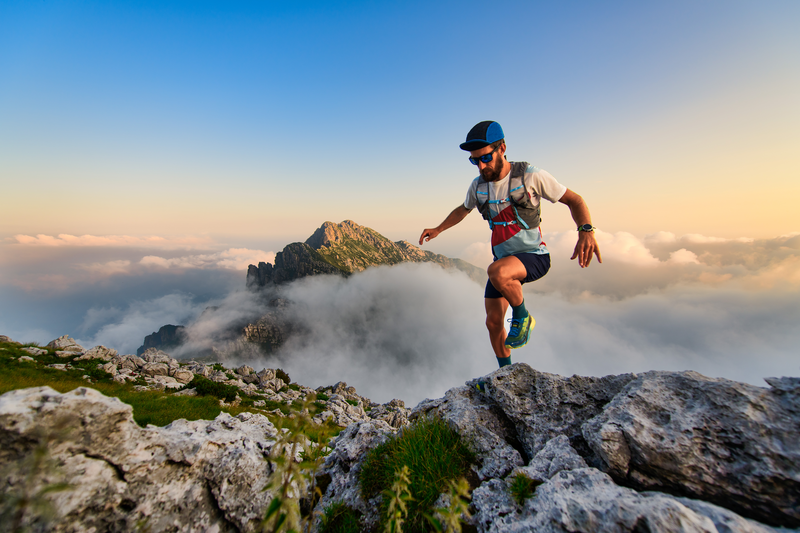
95% of researchers rate our articles as excellent or good
Learn more about the work of our research integrity team to safeguard the quality of each article we publish.
Find out more
ORIGINAL RESEARCH article
Front. Chem. , 12 July 2022
Sec. Medicinal and Pharmaceutical Chemistry
Volume 10 - 2022 | https://doi.org/10.3389/fchem.2022.953523
Diarylamines are a class of important skeleton widely existing in drugs or natural products. To discover novel diarylamine analogues as potential drugs, two series of diamide and carboxamide derivatives containing diarylamine scaffold were designed, synthesized and evaluated for their potential cytotoxic activities. The bioassay results indicated that some of the obtained compounds (C5, C6, C7, C11) exhibited good cytotoxic effect on cancer cell lines (SGC-7901, A875, HepG2), especially, compound C11 present significantly selective proliferation inhibition activity on cancer and normal cell lines (MARC145). In addition, the possible apoptosis induction for highly potential molecules was investigated, which present compound C11 could be used as novel lead compound for discovery of promising anticancer agents.
According to the progress report published by American Association for Cancer Research in 2019, cancer has become the leading cause of morbidity and mortality around the world, accounting for about 16 percent of deaths worldwide (American Cancer Society, 2018; AACR, 2019). Annual cancer cases and deaths have been increasing since 2000, and in 2018, 24% of estimated new cancer cases and 30% of cancer-related deaths globally occurred in China (Li et al., 2019). It is reported that the most common causes of cancer death were lung cancer, liver cancer and stomach cancer in China (Chen et al., 2016). The traditional treatment for cancer includes surgical resection combined with radiotherapy and chemotherapy; however, there are adverse drug reactions or therapeutic resistance. The devastating impact of cancer is predicated to grow significantly in the coming decades unless new and more effective anticancer drugs are developed. From 2009 to 2018, the number of cancer drug trails in China showed remarkable growth, with an average annual growth rate of 33% (Li et al., 2019). The China cancer drugs market size is projected to reach USD 30 billion by the year 2024. Thus, there is an urgent need for developing new anticancer agents with safer toxicological profiles and novel modes of action.
Diarylamines are a class of important scaffold widely existing in drugs and natural products, which can construct diverse molecular structures with extensive pharmacological activity (Kumar and Mishra, 2018; Abduelkarem et al., 2020). Diarylamines have also been used as typical synthons for construction of novel molecules due to their special multifunctional features, and many diarylamines analogues have been demonstrated to exhibit broad range of biological functions (Guan et al., 2013; Wang et al., 2013; Chai et al., 2014; Soussi et al., 2014; Wild et al., 2016; Wang et al., 2017; Zhang et al., 2017). Especially, some important diarylamine derivatives such as selumetinib, imatinib, dasatinib, niflumic acid, and flufenamic acid have been discovered and developed as commercial drugs (Figure 1), which further identify that this special scaffold is an attractive pharmacophore in the discovery of highly potential molecules, and will lead to some promising drug candidates. Therefore, diarylamines as easily available substrates have proven a convenient choice for construction of structural diversity molecules with promising bioactivity. In addition, carboxamides are also a useful class of molecules with potential pharmacological (Odingo et al., 2017; Tang et al., 2018; Barker et al., 2019; Ling et al., 2019; Sethy et al., 2019; Sun et al., 2019; Yang et al., 2019) and agroactive activities (Sartori et al., 2018; Tsikolia et al., 2018; Yu et al., 2018; Liu et al., 2019; Siber et al., 2019; Tsikolia et al., 2019; Liu et al., 2020), and many carboxamides have been developed as clinical drugs or agrochemicals, which demonstrate that amide units will play an important role in the development of drugs and pesticides.
Recently, during the course of our research on discovery of functional molecules, a series of diamides derivatives bearing nicotinamide unit were obtained (Peng et al., 2017), and an interesting phenomenon was found based on the preliminary results. All of the similar diamides without nicotinamide moiety lost the inhibitory activity except the compound bearing diarylamine unit (A2, R = Me), and this compound exhibited good cytotoxic activity against the tested lung cancer cell lines (NCI-H460, A549, and NCI-H1975) with IC50 values of 14.66–46.42 μM (Figure 2). However, the corresponding N-unsubstituted diarylamine-amide derivative A1 (R = H) almost lost activity (IC50 > 80 μM). These interesting findings may provide some useful information for developing diarylamine derivatives as potential anticancer agents, which also urge us to investigate the possible structure and activity relationships (SARs) for these diarylamine derivatives bearing diamide scaffold.
So, a series of extended diarylamine-guided diamides analogues based on the lead compound A2 was constructed for exploring prospective SARs with good cytotoxic activity as shown in Figure 3, and a series of diarylamine-guided carboxamide derivatives have also been designed for comparison with that of diarylamine-guided diamides. We report herein the synthesis and characterization of two series of diarylamine derivatives that have flexible substituent patterns, and their in vitro cytotoxic activities against SGC-7901 (human gastric cancer cell line), A875 (human melanoma cell line), HepG2 (human hepatocellular liver carcinoma cell line) and MARC145 (A subclone of African green monkey kidney cell line MA-104) cell lines were fully investigated. The results demonstrated that compound C11 may be a highly potential selective cytotoxic agent between cancer and normal cell lines.
According to the structure of lead molecule A2, various ortho-amino aryl acid 2 and diverse substituted aryl amines 5 were utilized as the building blocks to construct these diarylamine guided carboxamide or diamide derivatives. The target molecules B1-13 and C1-11 were conveniently prepared as described in Scheme 1.
First, the easily available flufenamic acid 1 (X = CH) was used as starting material for construction of diarylamine-guided diamides B1-13, which were reacted with various ortho-amino aryl acid 2 to obtain the corresponding oxazinone heterocyclic intermediates. Then these oxazinones 3 were treated with various amines resulting in the target diamides derivatives B1-13 via nucleophilic substitution reaction. Subsequently, diverse substituted arylamines 5 were directly adopted to construction diarylamine guided carboxamides C1-11 via nucleophilic substitution reaction of acids 1 (X = CH or N). The structures of all obtained molecules were characterized on the basis of satisfied spectral analysis, and the substituents of all the compounds were present in Scheme 1. All of the newly prepared diamides B1-13 and carboxamide derivatives C1-11 were confirmed by IR, 1H NMR, 13C NMR and ESI-MS analyses, and their chemical structures and basic physicochemical properties were summarized in Experimental. The 1H NMR of these compounds indicated typical proton signal peaks for different groups, and the ESI-MS spectrum indicated all these diarylamine-guided diamide derivatives B1-13 displayed an obvious [M + Na]+ addition ion fragmentation peak, however, the MS for diarylamine-containing carboxamide derivatives C1-11 exhibited [M + H]+ or [M−H]+ ion fragmentation peak.
The lead molecules A2 and all newly prepared diarylamine derivatives B1-13 and C1-11 were evaluated for their in vitro cytotoxic effects against SGC-7901, A875, HepG2 and MARC145 cell lines by the classical MTT [3-(4,5-dimethylthiazol-2-yl)-2,5-diphenyl tetrazolium bromide] assay (Alley et al., 1988; Ke et al., 2012; Shi et al., 2012; Yu et al., 2015; Ke et al., 2019; Xu et al., 2019; Huang et al., 2021) using 5-FU (5-Fluorouracil) as a control.
First, the lead molecule A2 exhibit good in vitro cytotoxic activity against lung cancer cell lines including NCI-H460, A549, and NCI-H1975 based on our previous investigation (Peng et al., 2017). As far as we know, the substituents attached to aryl ring usually have obvious influence on the activity. So, in order to investigate the possible effect of this unit on the activity of final molecules, a series of diarylamine guided diamide derivatives B1-13 were extended synthesized, and their cytotoxic activity was tested against SGC-7901, A875, HepG2, and MARC145 cell lines. Unfortunately, all newly prepared diarylamine-guided diamide derivatives B1-13 present poor effects on these tested cell lines as indicated in the Table 1, however, the lead compound A2 still exhibit very good cytotoxicity with IC50 values of 7.59–12.43 μM. These results demonstrated that the change of an appropriate substituents on the aryl ring could dramatically change the biological activity.
With these results in hand, we think that the part of ortho-amino aryl acid may be important for the bioactivity, and so the molecular simplification strategy was adopted to investigate the potential active molecules. As shown in Scheme 1, a series of diarylamine guided carboxamides C1-11 were further constructed using diverse substituted aryl amines, and the preliminary screening for these molecules were treated at the concentration of 100 µM (Table 2). What’s interesting is that some of the compounds (C5, C6, C7, C10, and C11) exhibit good inhibition activity, and especially the inhibition rate is even higher than that of the control 5-FU (Figure 4). We also can find from Figure 4 that compound C11 indicated good inhibition on all three cancer cell lines (SGC-7901, A875, and HepG2), however, which has low inhibition on normal cell lines (MARC145).
FIGURE 4. Cytotoxic activities of compounds C1-11 at the concentration of 100 µM. Abbreviations: SGC-7901—Human gastric cancer cell line; A875—Human melanoma cell line; HepG2—Human hepatocellular liver carcinoma cell line; MARC145—A subclone of African green monkey kidney cell line MA-104; 5-FU—5-Fluorouracil, used as a positive control.
Base on the aforementioned results, some of the diarylamine-guided carboxamide derivatives have been demonstrated to present good inhibitory activities against all tested cancer cell lines, so in order to further investigate the potential activities, the IC50 values were tested based on the above cell-based method. The in vitro activities described as IC50 values for these compounds were present in Table 3.
As shown in Table 3, the results further confirmed that some of these diarylamine-guided carboxamide derivatives (C5, C6, C7, C10, and C11) indicated higher inhibition activities compared to the control 5-FU. Generally, the compounds bearing p-isopropylaniline and o-trifluormethylaniline present poor inhibition activities against all tested cell lines (Entries 1–4). The compounds C6 containing m-trifluormethylaniline exhibited significant cytotoxic activities with the IC50 values of 5.53–12.77 μM (Entry 6), respectively. However, for the compounds containing p-trifluormethylaniline, the compound bearing flufenamic acid (X = CH) present the highest potential activities (Entry 7) than that of the compound containing niflumic acid (X = N). Especially, compound C11 bearing 6-chloropyridin-3-amine had a stronger inhibition on cancer cell lines (SGC-7901 IC50 = 9.13 μM; A875 IC50 = 12.34 μM; HepG2 IC50 = 10.15 μM) than the normal cells line MARC145 (IC50 > 102 μM), and it displayed a significantly selective proliferation inhibition activity on cancer and normal cell lines, and the selectivity index up to 8.26 (Figure 5). These interesting finds may provide some useful information for developing potential cytotoxicity agents.
In addition, the dose-response analysis of cell growth inhibition for highly potential molecules C5, C6, C7, C11, and 5-FU have been displayed in Figure 6, which identified that these compounds exhibited obvious cytotoxic effects on SGC-7901, A875, HepG2, and MARC145 cell lines with a significant concentration dependence.
FIGURE 6. Dose-response analysis of cell growth inhibition activity for compounds C5, C6, C7, and 5-FU (positive control) against SGC-7901 cells (upper left), A875 cells (upper right), HepG2 cells (lower left) and MARC145 cells (lower right).
Apoptosis induction is one of the important modes of action for antitumor drugs. So, the potential mechanism of highly effective compounds (C6 and C11) on A875 cells were investigated by flow cytometry. The apoptotic effect of compounds C6 and C11 was evaluated and analyzed following 24 h of treatment with 0.5×IC50, IC50 or 2×IC50 concentrations using Annexin V-FITC/PI dual staining assay.
All data obtained in this work are described in Figure 7, and we can find that compounds C6 and C11 induced apoptotic changes after 24 h treatment, and both compounds initiated apoptosis, in terms of FITC(+)PI(−) staining, to a larger extent than the control. 12% and 27% apoptotic rates are observed with treatment of compounds C6 and C11 at 2×IC50 concentrations respectively, whereas 2.14% of apoptosis was observed in control (0.1% DMSO), which indicated that these two potential molecules can induce apoptosis in A875 cells at least partly. However, the clearer mechanism of cell death induction by these compounds still remain to be further investigated.
FIGURE 7. Annexin V-FITC flow cytometry. Annexin V-FITC/PI staining was monitored on A875 cells following 24 h treatment with compounds C6 or C11 at concentrations corresponding to their 0.5 × IC50s, IC50s or 2 × IC50s (B–D,F–H). Representative dot plots of three independent experiments are given, presenting intact cells at lower-left quadrant, FITC(−)/PI(−); early apoptotic cells at lower-right quadrant, FITC(+)/PI(−); late apoptotic or necrotic cells at upper-right quadrant, FITC(+)/PI(+); necrotic cells at upper-left quadrant, FITC(−)/PI(+). (I,J) Apoptotic effect of compounds C6 and C11 was evaluated after 24 h treatment; bar graphs represent mean ± SD in at least three independent experiments.
From the preliminary analysis of apoptosis, the results encourage us to further investigate the type of cell death induced by the potential compounds (C6 and C11). As we know, the release of LDH is a typical characteristic feature of necrotic cell death, and so which was detected. From the results indicated in Figure 8, we can find that the release of LDH was significantly increased at 24 h after treatment with compounds C6, C11, and 5-FU (Figure 8), which were consistent with those of flow cytometry.
Based on the aforementioned results for the potential mechanism of action, the kinase inhibitory profile of compounds C6 and C11 were screened at two dose concentration of 10 and 1 µM over a panel of 15 kinases, and the kinase inhibitory activities are listed in supporting information (Supplementary Table S1). It was found that compound C6 exerted certain inhibitions on the tested MAPK1 and CDK2/A2 protein kinases at 1 μM, and compound C11 also indicated some inhibitions on the tested CDK1/A2, CDK2/A2, MAPK1 protein kinases at 1 μM, however, the inhibitory activity is not very good. These results will provide some reference for further mechanism research.
In summary, two series of diarylamine-guided diamide and carboxamide derivatives based on lead molecule have been designed and synthesized, and their potential cytotoxic activities were fully investigated by cell-based assay. The results demonstrated that some of the obtained diarylamine-guided carboxamide derivatives (C5, C6, C7, C11) had good cytotoxic effect on cancer cell lines (SGC-7901, A875, HepG2) compared with 5-FU in vitro, especially, compound C11 present significantly selective proliferation inhibition activity between cancer and normal cell lines, and the selectivity index was greater than 8.26, and these interesting results might be helpful to develop diarylamine-guided carboxamides as potential anticancer agents.
Melting points (m.p.) were measured using a digital model X-5 apparatus (Shanghai Instrument Physical Optics Instrument Co., LTD., Shanghai, China) and were uncorrected. Infrared (IR) Spectra were recorded using Shimadzu FTIR 8400S spectrophotometer. 1H NMR and 13C NMR spectra were recorded on a Bruker spectrometer at 600 MHz (Bruker, Bremen, Germany) with CDCl3, DMSO-d6 or CD3OD as the solvent. Liquid chromatography-tandem mass spectrometry (LC−MS/MS) analysis were performed on a Waters ACQUITY UPLC® H-CLASS PDA (Waters®, Milford, MA, United States) instrument. Analytical thin-layer chromatography was carried out on precoated plates, and spots were visualized with ultraviolet light. All chemicals or reagents used for syntheses were commercially available.
All the diarylamine-guided diamides derivatives B1-13 were prepared according to the similar procedures described in our previous reports (Shi et al., 2012; Peng et al., 2017), and were purified by recrystallization with methanol. The structures of all target compounds were confirmed by their spectral analysis, and all data for target molecules B1-13 are as following:
N-(2-Carbamoylphenyl)-2-((3- (trifluoromethyl)phenyl)amino)benzamide B1. Yield: 63%; m. p. 136–137°C; FTIR υmax (cm−1): 3,400, 3,260, 1,643, 1,575, 1,528, 1,334, 1,106; 1H NMR (600 MHz, CDCl3): δ 12.14 (s, 1H), 9.80 (s, 1H), 8.74 (d, J = 8.6 Hz, 1H), 7.82 (d, J = 7.3 Hz, 1H), 7.61–7.56 (m, 2H), 7.46 (s, 1H), 7.42–7.34 (m, 4H), 7.22 (d, J = 5.0 Hz, 1H), 7.15 (t, J = 7.2 Hz, 1H), 6.98–6.92 (m, 1H), 6.20 (s, 1H), 5.64 (s, 1H); 13C NMR (150 MHz, CDCl3): δ 171.32, 168.15, 145.22, 142.29, 140.34, 133.53, 132.79, 129.78, 128.31, 127.39, 123.18, 123.03, 121.78, 119.44, 119.08, 118.93, 118.58, 118.55, 116.74, 115.87, 99.99; MS (ESI) m/z 422.34 (M + Na)+, calcd. for C21H16F3N3O2 m/z = 399.12.
N-Methyl-2-(2-((3-(trifluoromethyl)phenyl) amino)benzamido)benzamide B2. Yield: 67%; m.p. 127–128°C; FTIR υmax (cm−1): 3,295, 1,637, 1,596, 1,505, 1,406, 1,280, 1,159; 1H NMR (600 MHz, CDCl3): δ 12.03 (s, 1H), 9.82 (s, 1H), 8.65 (d, J = 8.4 Hz, 1H), 7.84 (d, J = 7.9 Hz, 1H), 7.57–7.47 (m, 2H), 7.46 (s, 1H), 7.38 (p, J = 8.1 Hz, 4H), 7.22 (t, J = 6.7 Hz, 1H), 7.13 (t, J = 7.6 Hz, 1H), 6.97 (t, J = 7.2 Hz, 1H), 6.29 (s, 1H), 3.03 (d, J = 4.7 Hz, 3H); 13C NMR (150 MHz, CDCl3): δ 169.74, 168.07, 145.15, 142.35, 139.48, 132.72, 132.61, 131.83, 131.62, 129.78, 128.36, 126.49, 124.96, 123.15, 123.05, 121.86, 121.01, 119.58, 119.16, 118.50, 116.64, 115.86, 100.00, 26.94; MS (ESI) m/z 436.38 (M + Na)+, calcd. for C22H18F3N3O2 m/z = 413.14.
3-Bromo-5-fluoro-2- (2-((3-(trifluoromethyl)phenyl)amino)benzamido)benzamide B3. Yield: 81%; m.p. 198–199°C; FTIR υmax (cm−1): 3,371, 3,263, 1,650, 1,599, 1,504, 1,454, 1,305, 1,115; 1H NMR (600 MHz, DMSO-d6): δ 10.21 (s, 1H), 9.37 (s, 1H), 7.85–7.01 (m, 12H); 13C NMR (150 MHz, DMSO-d6): δ 168.07, 167.53, 143.30, 143.09, 138.54, 132.76, 131.14, 130.95, 130.04, 125.17, 122.49, 121.59, 121.42, 121.24, 120.18, 117.78, 116.49, 115.38, 115.23, 114.90; MS (ESI) m/z 518.21 (M + Na)+, calcd. for C21H14BrF4N3O2 m/z = 495.02.
3-Bromo-5-fluoro-N-methyl-2-(2-((3- (trifluoromethyl)phenyl)amino)benzamido)benzamide B4. Yield: 84%; m.p. 189–190°C; FTIR υmax (cm−1): 3,281, 1,628, 1,580, 1,456, 1,325, 1,159, 1,122; 1H NMR (600 MHz, DMSO-d6): δ 10.26 (s, 1H), 9.14 (s, 1H), 8.39 (s, 1H), 7.84 (d, J = 4.5 Hz, 1H), 7.78 (d, J = 7.1 Hz, 1H), 7.52–7.38 (m, 6H), 7.23 (d, J = 6.4 Hz, 1H), 7.03 (t, J = 6.4 Hz, 1H), 2.61 (d, J = 2.9 Hz, 3H); 13C NMR (150 MHz, DMSO-d6): δ 168.03, 166.26, 153.12, 143.49, 142.54, 135.27, 132.49, 130.92, 129.83, 123.72, 122.35, 121.98, 121.53, 121.36, 120.42, 117.61, 116.85, 115.17, 114.76, 26.54; MS (ESI) m/z 532.16 (M + Na)+, calcd. for C22H16BrF4N3O2 m/z = 509.04.
N-(2-Carbamoyl-6-methylphenyl)-2-((3-(trifluoromethyl)phenyl)amino)benzamide B5. Yield: 77%; m.p. 191–192°C; FTIR υmax (cm−1): 3,377, 3,276, 1,646, 1,596, 1,507, 1,470, 1,289, 1,157; 1H NMR (600 MHz, DMSO-d6): δ 10.17 (s, 1H), 9.36 (s, 1H), 7.82–7.80 (m, 2H), 7.48–7.38 (m, 8H), 7.26 (t, J = 7.6 Hz, 1H), 7.22 (d, J = 7.5 Hz, 1H), 7.02 (t, J = 7.8 Hz, 1H), 2.20 (s, 3H); 13C NMR (150 MHz, DMSO-d6): δ 170.45, 167.46, 143.71, 142.63, 136.42, 134.48, 133.54, 132.61, 132.44, 130.88, 130.65, 130.44, 129.91, 126.59, 126.35, 122.86, 122.12, 120.63, 117.45, 117.11, 114.49, 18.65; MS (ESI) m/z 436.38 (M + Na)+, calcd. for C22H18F3N3O2 m/z = 413.14.
N,3-Dimethyl-2-(2-((3-(trifluoromethyl)phenyl)amino)benzamido)benzamide B6. Yield: 82%; m.p. 195–196°C; FTIR υmax (cm−1): 3,317, 1,651, 1,611, 1,529, 1,322, 1,158, 1,114; 1H NMR (600 MHz, DMSO-d6): δ 10.15 (s, 1H), 9.19 (s, 1H), 8.30 (d, J = 4.5 Hz, 1H), 7.75 (dd, J = 7.7, 1.1 Hz, 1H), 7.54–7.32 (m, 7H), 7.26 (t, J = 7.6 Hz, 1H), 7.21 (d, J = 7.4 Hz, 1H), 7.03 (t, J = 7.0 Hz, 1H), 2.65 (d, J = 4.6 Hz, 3H), 2.22 (s, 3H); 13C NMR (150 MHz, DMSO-d6): δ 168.95, 167.47, 143.82, 142.20, 136.38, 134.32, 133.91, 132.47, 132.18, 130.85, 129.73, 126.67, 126.11, 123.69, 121.75, 120.75, 117.23, 26.56, 18.60; MS (ESI) m/z 450.33 (M + Na)+, calcd. for C23H20F3N3O2 m/z = 427.15.
N-(2-Carbamoyl-5- chlorophenyl)-2-((3-(trifluoromethyl)phenyl)amino)benzamide B7. Yield: 64%; m.p. 176–177°C; FTIR υmax (cm−1): 3,345, 3,153, 1,668, 1,640, 1,515, 1,389, 1,325, 1,159, 1,117; 1H NMR (600 MHz, DMSO-d6): δ 12.82 (s, 1H), 9.30 (s, 1H), 8.67 (d, J = 2.2 Hz, 1H), 8.43 (s, 1H), 7.88 (d, J = 8.6 Hz, 2H), 7.74 (dd, J = 7.9, 1.4 Hz, 1H), 7.55–7.33 (m, 5H), 7.26 (dd, J = 8.5, 2.2 Hz, 1H), 7.21 (d, J = 7.6 Hz, 1H), 7.09–7.05 (m, 1H); 13C NMR (150 MHz, DMSO-d6): δ 170.54, 167.25, 143.67, 143.14, 141.40, 137.19, 133.30, 130.81, 130.78, 129.13, 125.50, 123.70, 122.95, 122.55, 122.33, 121.17, 120.10, 118.64, 118.50, 117.75, 114.96; MS (ESI) m/z 456.27 (M + Na)+, calcd. for C21H15ClF3N3O2 m/z = 433.08.
4-Chloro-N-methyl-2-(2-((3-(trifluoromethyl)phenyl)amino)benzamido)benzamide B8. Yield: 71%; m.p. 152–153°C; FTIR υmax (cm−1): 3,316, 1,633, 1,572, 1,515, 1,330, 1,221, 1,166, 1,117; 1H NMR (600 MHz, DMSO-d6): δ 12.50 (s, 1H), 9.21 (s, 1H), 8.85 (d, J = 4.2 Hz, 1H), 8.63 (d, J = 2.2 Hz, 1H), 7.77 (d, J = 8.5 Hz, 1H), 7.74 (dd, J = 7.8, 1.4 Hz, 1H), 7.52–7.48 (m, 1H), 7.45 (t, J = 8.2 Hz, 1H), 7.40–7.34 (m, 3H), 7.26 (dd, J = 8.5, 2.2 Hz, 1H), 7.19 (d, J = 7.6 Hz, 1H), 7.15–7.07 (m, 1H), 2.74 (d, J = 4.5 Hz, 3H); 13C NMR (150 MHz, DMSO-d6): δ 168.36, 167.06, 143.92, 142.69, 140.71, 136.76, 133.25, 130.73, 130.59, 130.39, 130.10, 129.32, 125.52, 123.27, 123.07, 121.96, 121.54, 120.25, 119.64, 118.99, 114.62, 26.70; MS (ESI) m/z 470.32 (M + Na)+, calcd. for C22H17ClF3N3O2 m/z = 447.10.
N-(2-Carbamoyl-4-chlorophenyl) -2-((3-(trifluoromethyl)phenyl)amino)benzamide B9. Yield: 68%; m.p. 183–184°C; FTIR υmax (cm−1): 3,405, 3,197, 1,643, 1,611, 1,518, 1,385, 1,295, 1,112; 1H NMR (600 MHz, DMSO-d6): δ 12.53 (s, 1H), 9.30 (s, 1H), 8.55 (d, J = 9.0 Hz, 1H), 8.45 (s, 1H), 7.92 (d, J = 2.5 Hz, 1H), 7.90 (s, 1H), 7.73 (dd, J = 7.9, 1.4 Hz, 1H), 7.61 (dd, J = 9.0, 2.5 Hz, 1H), 7.50–7.45 (m, 2H), 7.41–7.37 (m, 3H), 7.20 (d, J = 7.6 Hz, 1H), 7.11–7.04 (m, 1H); 13C NMR (150 MHz, DMSO-d6): δ 170.00, 167.02, 143.72, 143.02, 138.85, 133.18, 132.38, 130.82, 130.64, 129.14, 128.75, 127.10, 123.70, 122.78, 122.65, 122.21, 122.14, 121.22, 118.52, 117.69, 114.77; MS (ESI) m/z 456.27 (M + Na)+, calcd. for C21H15ClF3N3O2 m/z = 433.08.
5-Chloro-N-methyl-2-(2-((3-(trifluoromethyl)phenyl)amino)benzamido)benzamide B10. Yield: 74%; m.p. 190–191°C; FTIR υmax (cm−1): 3,300, 1,657, 1,629, 1,515, 1,328, 1,157, 1,110; 1H NMR (600 MHz, DMSO-d6): δ 12.21 (s, 1H), 9.21 (s, 1H), 8.87 (d, J = 4.4 Hz, 1H), 8.51 (d, J = 9.0 Hz, 1H), 7.80 (d, J = 2.5 Hz, 1H), 7.74 (dd, J = 7.8, 1.4 Hz, 1H), 7.59 (dd, J = 9.0, 2.5 Hz, 1H), 7.53–7.47 (m, 1H), 7.45 (t, J = 8.2 Hz, 1H), 7.40–7.34 (m, 3H), 7.18 (d, J = 7.7 Hz, 1H), 7.13–7.08 (m, 1H), 2.73 (d, J = 4.5 Hz, 3H); 13C NMR (150 MHz, DMSO-d6): δ 167.85, 166.83, 143.96, 142.58, 138.16, 133.12, 131.95, 130.76, 130.59, 129.34, 128.16, 127.20, 125.52, 123.51, 123.16, 122.80, 121.83, 121.57, 118.98, 117.43, 114.48, 26.71; MS (ESI) m/z 470.32 (M + Na)+, calcd. for C22H17ClF3N3O2 m/z = 447.10.
N-(2-Carbamoyl-3-chlorophenyl)-2-((3-(trifluoromethyl)phenyl)amino)benzamide B11. Yield: 62%; m.p. 194–195°C; FTIR υmax (cm−1): 3,325, 3,171, 1,678, 1,581, 1,510, 1,326, 1,162, 1,111; 1H NMR (600 MHz, DMSO-d6): δ 10.05 (s, 1H), 9.15 (s, 1H), 7.98 (s, 1H), 7.86 (s, 1H), 7.74 (dd, J = 7.8, 1.2 Hz, 1H), 7.70 (d, J = 8.0 Hz, 1H), 7.52–7.44 (m, 2H), 7.43–7.32 (m, 5H), 7.21 (d, J = 7.7 Hz, 1H), 7.10–7.04 (m, 1H); 13C NMR (150 MHz, DMSO-d6): δ 167.26, 166.87, 144.02, 142.67, 136.49, 133.06, 132.01, 130.84, 130.62, 130.39, 129.82, 126.67, 125.52, 124.24, 123.72, 123.04, 122.01, 121.36, 118.75, 117.59, 114.55; MS (ESI) m/z 456.27 (M + Na)+, calcd. for C21H15ClF3N3O2 m/z = 433.08.
2-Chloro-N-methyl-6-(2-((3-(trifluoromethyl)phenyl)amino)benzamido)benzamide B12. Yield: 73%; m.p. 178–179°C; FTIR υmax (cm−1): 3,273, 1,667, 1,641, 1,505, 1,331, 1,171, 1,108; 1H NMR (600 MHz, DMSO-d6): δ 10.14 (s, 1H), 8.95 (s, 1H), 8.44 (d, J = 4.6 Hz, 1H), 7.79 (d, J = 8.2 Hz, 1H), 7.73 (d, J = 7.8 Hz, 1H), 7.50–7.45 (m, 2H), 7.42 (t, J = 8.1 Hz, 1H), 7.36–7.33 (m, 4H), 7.20 (d, J = 7.7 Hz, 1H), 7.14–7.09 (m, 1H), 2.61 (d, J = 4.6 Hz, 3H); 13C NMR (150 MHz, DMSO-d6): δ 167.03, 165.25, 144.40, 142.27, 136.92, 132.94, 131.52, 130.79, 130.68, 130.59, 130.08, 126.46, 125.54, 124.25, 123.82, 123.74, 121.93, 121.75, 119.47, 117.39, 114.41, 26.26; MS (ESI) m/z 470.32 (M + Na)+, calcd. for C22H17ClF3N3O2 m/z = 447.10.
3,5-Dichloro-N- methyl-2-(2-((3-(trifluoromethyl)phenyl)amino)benzamido)benzamide B13. Yield: 76%; m.p. 180–181°C; FTIR υmax (cm−1): 3,287, 1,632, 1,582, 1,524, 1,328, 1,162, 1,115; 1H NMR (600 MHz, CDCl3): δ 9.44 (s, 1H), 9.21 (s, 1H), 7.80 (d, J = 7.8 Hz, 1H), 7.57 (d, J = 2.2 Hz, 1H), 7.44 (s, 1H), 7.40 (dd, J = 5.7, 2.2 Hz, 4H), 7.35 (d, J = 8.3 Hz, 1H), 7.23 (d, J = 7.5 Hz, 1H), 6.95–6.91 (m, 1H), 6.29 (s, 1H), 2.90 (d, J = 4.0 Hz, 3H); 13C NMR (150 MHz, CDCl3): δ 168.25, 167.11, 145.16, 141.99, 134.03, 133.46, 133.28, 132.35, 132.00, 131.93, 131.69, 129.88, 129.07, 126.04, 123.19, 119.47, 118.79, 117.66, 116.75, 115.71, 26.97; MS (ESI) m/z 504.25 (M + Na)+, calcd. for C22H16Cl2F3N3O2 m/z = 481.06.
To a solution of diarylamine carboxylic acid 1 (1 mmol) in 8 ml anhydrous acetonitrile was added pyridine (3 mmol), and then the reaction mixture was cooled to 0 C. Whereafter, methanesulfonyl chloride (1.5 mmol) was added dropwise to the reaction mixture over 15–20 min. After addition, the reaction mixture was then allowed to warm to room temperature and stirred for additional hours, the corresponding multi-substituted amines 5 (1.05 mmol) was added, and the reaction mixture was heated to 40–45°C and detected by thin-layer chromatography. After completion of the reaction, the mixture was quenched by the addition of water and was stirred for 20 min. The suspended solid was collected by filtration and washed with water to afford the crude products, which can be purified by silica gel column chromatography (petroleum ether/ethyl acetate) or recrystallization (methanol) to give the target molecules. Their physico-chemical properties and the spectra data are as follows:
N-(4-Isopropylphenyl)-2-((3-(trifluoromethyl)phenyl) amino)benzamide C1. Yield: 72%; m.p. 74–75°C; FTIR υmax (cm−1): 3,274, 1,633, 1,595, 1,514, 1,323, 1,113; 1H NMR (600 MHz, CDCl3): δ 9.30 (s, 1H), 7.73 (s, 1H), 7.53 (d, J = 7.2 Hz, 1H), 7.41–7.25 (m, 7H), 7.18–7.10 (m, 2H), 6.99 (m, 1H), 6.85 (t, J = 7.2 Hz, 1H), 2.86–2.82 (m, 1H), 1.18 (d, J = 6.6 Hz, 6H); 13C NMR (150 MHz, CDCl3): δ 167.63, 145.89, 144.52, 142.17, 134.97, 132.72, 129.86, 127.61, 127.22, 127.11, 122.93, 121.01, 119.70, 119.32, 118.57, 116.27, 116.15, 33.70, 24.03; MS (ESI) m/z 399.55 (M + H)+, calcd. for C23H21F3N2O m/z = 398.16.
N-(4-Isopropylphenyl)-2-((3-(trifluoromethyl) phenyl)amino)nicotinamide C2. Yield: 76%; m.p. 161–162°C; FTIR υmax (cm−1): 3,318, 1,634, 1,588, 1,507, 1,443, 1,331, 1,114; 1H NMR (600 MHz, DMSO-d6): δ 10.98 (s, 1H), 9.36 (bs, 1H), 8.43–7.34 (m, 7H), 7.18 (d, J = 7.2 Hz, 2H), 6.82 (s, 1H), 2.87–2.82 (m, 1H), 1.19 (d, J = 6.6 Hz, 6H); 13C NMR (150 MHz, CD3OD): δ 168.58, 156.04, 151.88, 146.91, 142.49, 138.54, 137.18, 132.19, 131.98, 130.51, 127.74, 124.07, 122.84, 119.20, 119.18, 117.07, 115.44, 114.14, 35.02, 24.52; MS (ESI) m/z 400.63 (M + H)+, calcd. for C22H20F3N3O m/z = 399.16.
N-(2-(Trifluoromethyl)phenyl)-2-((3-(trifluoromethyl) phenyl)amino)benzamide C3. Yield: 78%; m.p. 82–83°C; FTIR υmax (cm−1): 3,286, 1,636, 1,580, 1,518, 1,314, 1,100; 1H NMR (600 MHz, CDCl3): δ 9.33 (s, 1H), 8.20 (d, J = 8.4 Hz, 1H), 8.16 (s, 1H), 7.62–7.52 (m, 3H), 7.39 (s, 1H), 7.35–7.29 (m, 4H), 7.23 (t, J = 7.2 Hz, 1H), 7.17 (s, 1H), 6.89–6.87 (m, 1H); 13C NMR (150 MHz, CDCl3): δ 167.51, 145.15, 141.95, 135.04, 133.33, 132.94, 129.91, 127.48, 126.34, 126.30, 125.01, 124.85, 123.34, 119.57, 118.95, 118.53, 116.82, 116.79, 116.22; MS (ESI) m/z 425.47 (M + H)+, calcd. for C21H14F6N2O m/z = 424.10.
N-(2-(Trifluoromethyl)phenyl)-2-((3-(trifluoromethyl) phenyl)amino)nicotinamide C4. Yield: 62%; m.p. 139–140°C; FTIR υmax (cm−1): 3,292, 1,638, 1,599, 1,517, 1,442, 1,330, 1,109; 1H NMR (400 MHz, DMSO-d6): δ 10.79 (s, 1H), 10.54 (s, 1H), 8.47 (dd, J = 7.2 Hz, 1H), 8.34 (dd, J = 7.2 Hz, 1H), 8.28 (s, 1H), 7.86–7.77 (m, 3H), 7.61 (q, J = 12 Hz, 2H), 7.52 (t, J = 12 Hz, 1H), 7.31 (d, J = 12 Hz, 1H), 7.06 (q, J = 12 Hz, 1H); 13C NMR (100 MHz, DMSO-d6) δ 168.14, 154.73, 151.69, 141.26, 138.26, 135.49, 133.76, 132.01, 130.26, 130.14, 129.83, 128.47, 127.09, 127.04, 126.09, 125.41, 123.49, 122.69, 118.32, 115.69, 115.65, 115.03, 111.66; MS (ESI) m/z 426.55 (M + H)+, calcd. for C20H13F6N3O m/z = 425.10.
N-(3-(Trifluoromethyl)phenyl)-2-((3-(trifluoromethyl) phenyl)amino)benzamide C5. Yield: 70%; m.p. 75–76°C; FTIR υmax (cm−1): 3,292, 1,638, 1,519, 1,443, 1,325, 1,154, 1,118; 1H NMR (600 MHz, CDCl3): δ 9.19 (s, 1H), 7.91 (s, 1H), 7.84 (s, 1H), 7.69 (d, J = 7.8 Hz, 1H), 7.54 (d, J = 7.8 Hz, 1H), 7.43 (t, J = 7.8 Hz, 1H), 7.37–7.26 (m, 6H), 7.18–7.16 (m, 1H), 6.87–6.85 (m, 1H); 13C NMR (150 MHz, CDCl3): δ 167.66, 144.83, 141.95, 138.07, 133.22, 131.90, 131.70, 129.94, 129.71, 127.61, 124.89, 124.71, 123.59, 123.31, 121.41, 119.41, 118.93, 117.32, 116.62, 116.36; MS (ESI) m/z 425.56 (M + H)+, calcd. for C21H14F6N2O m/z = 424.10.
N-(3-(Trifluoromethyl)phenyl)-2-((3-(trifluoromethyl) phenyl)amino)nicotinamide C6. Yield: 73%; m.p. 130–131°C; FTIR υmax (cm−1): 3,293, 1,638, 1,599, 1,517, 1,462, 1,330, 1,156, 1,109; 1H NMR (600 MHz, CDCl3): δ 10.35 (s, 1H), 8.36 (t, J = 4.8 Hz, 1H), 8.02 (s, 1H), 7.90 (s, 1H), 7.83–7.71 (m, 4H), 7.48–7.34 (m, 3H), 7.21 (d, J = 7.8 Hz, 1H), 6.78 (s, 1H); 13C NMR (150 MHz, CDCl3): δ 166.48, 155.14, 153.22, 152.20, 140.22, 137.68, 135.68, 131.61, 129.83, 129.57, 129.23, 123.93, 123.46, 121.86, 120.70, 119.08, 117.63, 117.09, 113.86, 110.94; MS (ESI) m/z 426.55 (M + H)+, calcd. for C20H13F6N3O m/z = 425.10.
N-(4-(Trifluoromethyl)phenyl)-2-((3-(trifluoromethyl) phenyl)amino)benzamide C7. Yield: 76%; m.p. 102–103°C; FTIR υmax (cm−1): 3,332, 1,644, 1,594, 1,505, 1,325, 1,157, 1,108; 1H NMR (600 MHz, CDCl3): δ 9.18 (s, 1H), 7.94 (s, 1H), 7.65–7.54 (m, 5H), 7.37–7.32 (m, 4H), 7.27 (d, J = 8.4 Hz, 1H), 7.18 (d, J = 7.8 Hz, 1H), 6.88–6.85 (m, 1H); 13C NMR (150 MHz, CDCl3): δ 167.67, 144.86, 141.93, 140.63, 133.29, 131.92, 131.71, 129.96, 127.64, 126.44, 126.42, 123.29, 120.11, 119.43, 119.00, 118.98, 118.93, 116.63, 116.60, 116.38; MS (ESI) m/z 425.65 (M + H)+, calcd. for C21H14F6N2O m/z = 424.10.
N-(4-(Trifluoromethyl)phenyl)-2-((3-(trifluoromethyl) phenyl)amino)nicotinamide C8. Yield: 73%; m.p. 171–172°C; FTIR υmax (cm−1): 3,319, 1,645, 1,585, 1,508, 1,442, 1,309, 1,119; 1H NMR (600 MHz, DMSO-d6): 1H NMR (600 MHz, CDCl3): δ 10.81 (s, 1H), 10.30 (s, 1H), 8.43 (dd, J = 4.2 Hz, 1H), 8.29–8.25 (m, 2H), 7.98 (d, J = 8.4 Hz, 2H), 7.87 (d, J = 8.4 Hz, 1H), 7.76 (d, J = 8.4 Hz, 2H), 7.52 (t, J = 8.4 Hz, 1H), 7.31 (d, J = 7.2 Hz, 1H), 7.04 (dd, J = 4.8 Hz, 1H); 13C NMR (150 MHz, DMSO-d6): δ 153.68, 151.36, 145.14, 141.78, 137.92, 130.20, 126.92, 124.13, 121.28, 118.98, 115.87, 115.00, 114.14; MS (ESI) m/z 426.55 (M + H)+, calcd. for C20H13F6N3O m/z = 425.10.
N-(Pyridin-2-yl)-2-((3-(trifluoromethyl)phenyl)amino)benzamide C9. Yield: 55%; m.p. 65–66°C; FTIR υmax (cm−1): 2,958, 1,663, 1,575, 1,493, 1,435, 1,340, 1,298, 1,109; 1H NMR (600 MHz, CDCl3): δ 9.40 (s, 1H), 9.25 (br, 1H), 8.30 (d, J = 8.4Hz, 1H), 8.19 (d, J = 4.2Hz, 1H), 7.76–7.68 (m, 3H), 7.47–7.31 (m, 3H), 7.18 (t, J = 6.6 Hz, 1H), 7.04 (t, J = 6.6 Hz, 1H), 6.85–6.83 (m, 1H); 13C NMR (150 MHz, CDCl3): δ 167.92, 151.22, 146.76, 145.20, 141.96, 139.37, 133.39, 131.91, 131.70, 129.90, 128.35, 123.39, 119.97, 119.32, 118.96, 118.23, 116.93, 116.01, 114.72; MS (ESI) m/z 358.60 (M + H)+, calcd. for C19H14F3N3O m/z = 357.11.
N-(2-Chloropyridin-4-yl)-2-((3-(trifluoromethyl)phenyl)amino)benzamide C10. Yield: 68%; m.p. 97–98°C; FTIR υmax (cm−1): 2,959, 1,663, 1,575, 1,525, 1,493, 1,435, 1,340, 1,298, 1,109; 1H NMR (600 MHz, CDCl3): δ 9.19 (s, 1H), 8.31 (d, J = 5.4Hz, 1H), 8.17 (s, 1H), 7.76 (d, J = 1.8 Hz, 1H), 7.60 (d, J = 7.8Hz, 1H), 7.45–7.35 (m, 6H), 7.28 (d, J = 7.2 Hz, 1H), 6.93 (t, J = 7.8 Hz, 1H); 13C NMR (150 MHz, CDCl3): δ 167.76, 152.64, 150.31, 146.93, 145.29, 141.70, 133.84, 130.04, 127.71, 123.69, 119.44, 118.00, 117.00, 116.53, 113.86, 112.79; MS (ESI) m/z 392.26 (M + H)+, calcd. for C19H13ClF3N3O m/z = 391.07.
N-(6-Chloropyridin-3-yl)-2-((3-(trifluoromethyl)phenyl)amino)nicotinamide C11. Yield: 74%; m.p. 166–167°C; FTIR υmax (cm−1): 3,316, 1,643, 1,605, 1,581, 1,505, 1,443, 1,282, 1,110; 1H NMR (600 MHz, DMSO-d6): δ 10.81 (s, 1H), 10.36 (s, 1H), 8.76 (d, J = 2.7 Hz, 1H), 8.44 (dd, J = 4.8, 1.8 Hz, 1H), 8.29–8.21 (m, 3H), 7.87 (dd, J = 8.2, 1.4 Hz, 1H), 7.57–7.51 (m, 2H), 7.31 (d, J = 7.7 Hz, 1H), 7.04 (dd, J = 7.7, 4.8 Hz, 1H); 13C NMR (150 MHz, DMSO-d6): δ 167.22, 154.25, 151.49, 144.99, 142.61, 141.34, 138.63, 135.46, 132.13, 130.00, 125.67, 124.59, 123.86, 123.70, 118.38, 115.91, 114.97, 113.07; MS (ESI) m/z 393.34 (M + H)+, calcd. for C18H12ClF3N4O m/z = 392.07.
The cytotoxicity of target compounds was determined with MTT assay (Alley et al., 1988; Ke et al., 2012; Shi et al., 2012; Yu et al., 2015; Ke et al., 2019; Xu et al., 2019; Huang et al., 2021). SGC-7901, A875, HepG2 and MARC145 cell lines were obtained from Hubei Biopesticide Engineering Research Centre. All data of the experiment were analyzed with SPSS software, and all assay were performed in triplicate on three independent experiments, and measurement data were expressed as the mean ± S.D.
Quantitative analysis of apoptotic and necrotic cell death induced by potential molecules was investigated using Annexin V-FITC/PI dual staining assay (Yu et al., 2015; Huang et al., 2021).
A875 cells grown in 96-well plates were treated with serial dilutions of each tested compound for 24 h. The culture media were collected, and the concentrations of LDH were determined based on our previous research (Huang et al., 2021).
The kinase inhibitory profile of compounds C6 and C11 were screened at two dose concentration of 10 and 1 µM over a panel of 15 kinases, and each assay was repeated twice. All the inhibitory assays were carried out through kinase profiling services provided by HY Biotech (Chinese), in which ADP-GLO kinase assays were used.
The original contributions presented in the study are included in the article/Supplementary Material, further inquiries can be directed to the corresponding authors.
All authors listed have made a substantial, direct, and intellectual contribution to the work and approved it for publication.
The authors declare that the research was conducted in the absence of any commercial or financial relationships that could be construed as a potential conflict of interest.
All claims expressed in this article are solely those of the authors and do not necessarily represent those of their affiliated organizations, or those of the publisher, the editors and the reviewers. Any product that may be evaluated in this article, or claim that may be made by its manufacturer, is not guaranteed or endorsed by the publisher.
The authors are grateful to the Program for Leading Talents of Hubei Academy of Agricultural Sciences (L2018031), the Youth Science Foundation of Hubei Academy of Agricultural Sciences (2021NKYJJ17), the Innovation and Application of Key Technologies of Quality-improving and Efficiency-increasing of Fengtou Ginger Industry (2020-620-002-06) and Hubei Biopesticide Engineering Research Centre (HBERC-RC-202002, HBERC-RC-202104) for their financial support.
The Supplementary Material for this article can be found online at: https://www.frontiersin.org/articles/10.3389/fchem.2022.953523/full#supplementary-material
AACR (2019). AACR Cancer Progress Report. Available at: https://www.cancerprogressreport.org/Pages/cpr19-cancer-in-2019.
Abduelkarem, A. R., Anbar, H. S., Zaraei, S. O., Alfar, A. A., Al-Zoubi, O. S., Abdelkarem, E. G., et al. (2020). Diarylamides in Anticancer Drug Discovery: A Review of Pre-clinical and Clinical Investigations. Eur. J. Med. Chem. 188, 112029. doi:10.1016/j.ejmech.2019.112029
Alley, M. C., Scudiero, D. A., Monks, A., Hursey, M. L., Czerwinski, M. J., Fine, D. L., et al. (1988). Feasibility of Drug Screening with Panels of Human Tumor Cell Lines Using a Microculture Tetrazolium Assay. Cancer Res. 48, 589–601.
American Cancer Society (2018). Global Cancer Facts & Figures. 4th Edition. Atlanta: American Cancer Society.
Barker, W. T., Chandler, C. E., Melander, R. J., Ernst, R. K., and Melander, C. (2019). Tryptamine Derivatives Disarm Colistin Resistance in Polymyxin-Resistant Gram-Negative Bacteria. Bioorg. Med. Chem. 27, 1776–1788. doi:10.1016/j.bmc.2019.03.019
Chai, B., Liu, C., Li, H., Liu, S., Xu, Y., Song, Y., et al. (2014). Synthesis and Acaricidal Activity of Strobilurin-Pyrimidine Derivatives. Chin. Chem. Lett. 25, 137–140. doi:10.1016/j.cclet.2013.10.006
Chen, W., Zheng, R., Baade, P. D., Zhang, S., Zeng, H., Bray, F., et al. (2016). Cancer Statistics in China, 2015. CA A Cancer J. Clin. 66, 115–132. doi:10.3322/caac.21338
Guan, A., Liu, C., Huang, G., Li, H., Hao, S., Xu, Y., et al. (2013). Design, Synthesis, and Structure-Activity Relationship of Novel Aniline Derivatives of Chlorothalonil. J. Agric. Food Chem. 61, 11929–11936. doi:10.1021/jf403739e
Huang, W., Gao, Z., Zhang, Z., Fang, W., Wang, Z., Wan, Z., et al. (2021). Selective and Effective Anticancer Agents: Synthesis, Biological Evaluation and Structure-Activity Relationships of Novel Carbazole Derivatives. Bioorg. Chem. 113, 104991. doi:10.1016/j.bioorg.2021.104991
Ke, S., Shi, L., Cao, X., Yang, Q., Liang, Y., and Yang, Z. (2012). Heterocycle-functional Gramine Analogues: Solvent- and Catalyst-free Synthesis and Their Inhibition Activities against Cell Proliferation. Eur. J. Med. Chem. 54, 248–254. doi:10.1016/j.ejmech.2012.05.003
Ke, S., Zhang, Z., Liu, M., Fang, W., Huang, D., Wan, Z., et al. (2019). Synthesis and Bioevaluation of Novel Steroidal Isatin Conjugates Derived from Epiandrosterone/androsterone. J. Enzyme Inhibition Med. Chem. 34, 1607–1614. doi:10.1080/14756366.2019.1659790
Kumar, A., and Mishra, A. K. (2018). Pharmacological Applications of Diphenylamine and its Derivative as Potent Bioactive Compound: A Review. Cbc 14, 217–233. doi:10.2174/1573407213666170301155550
Li, N., Huang, H. Y., Wu, D. W., Yang, Z. M., Wang, J., Wang, J. S., et al. (2019). Changes in Clinical Trials of Cancer Drugs in Mainland China over the Decade 2009-18: a Systematic Review. Lancet Oncol. 20, e619–e626. doi:10.1016/s1470-2045(19)30491-7
Ling, Y., Gao, W., Ling, C., Liu, J., Meng, C., Qian, J., et al. (2019). β-Carboline and N-Hydroxycinnamamide Hybrids as Anticancer Agents for Drug-Resistant Hepatocellular Carcinoma. Eur. J. Med. Chem. 168, 515–526. doi:10.1016/j.ejmech.2019.02.054
Liu, C., Tian, J., An, T., Lyu, F., Jia, P., Zhou, M., et al. (2020). Secondary Metabolites from Solanum Rostratum and Their Antifeedant Defense Mechanisms against Helicoverpa Armigera. J. Agric. Food Chem. 68, 88–96. doi:10.1021/acs.jafc.9b06768
Liu, J., Li, F., Li, Y., Zhang, X., Hua, X., Xiong, L., et al. (2019). Synthesis, Insecticidal Evaluation and 3D-QSAR Study of Novel Anthranilic Diamide Derivatives as Potential Ryanodine Receptor Modulators. Pest. Manag. Sci. 75, 1034–1044. doi:10.1002/ps.5213
Odingo, J., Bailey, M. A., Files, M., Early, J. V., Alling, T., Dennison, D., et al. (2017). In Vitro evaluation of Novel Nitazoxanide Derivatives against mycobacterium Tuberculosis. ACS Omega 2, 5873–5890. doi:10.1021/acsomega.7b00892
Peng, M., Shi, L., and Ke, S. (2017). Nicotinamide-based Diamides Derivatives as Potential Cytotoxic Agents: Synthesis and Biological Evaluation. Chem. Central J. 11, 109. doi:10.1186/s13065-017-0338-5
Sartori, S. K., Alvarenga, E. S., Franco, C. A., Ramos, D. S., and Oliveira, D. F. (2018). One-pot Synthesis of Anilides, Herbicidal Activity and Molecular Docking Study. Pest. Manag. Sci. 74, 1637–1645. doi:10.1002/ps.4855
Sethy, B., Hsieh, C. F., Lin, T. J., Hu, P. Y., Chen, Y. L., Lin, C. Y., et al. (2019). Design, Synthesis, and Biological Evaluation of Itaconic Acid Derivatives as Potential Anti-influenza Agents. J. Med. Chem. 62, 2390–2403. doi:10.1021/acs.jmedchem.8b01683
Shi, L., Hu, R., Wei, Y., Liang, Y., Yang, Z., and Ke, S. (2012). Anthranilic Acid-Based Diamides Derivatives Incorporating Aryl-Isoxazoline Pharmacophore as Potential Anticancer Agents: Design, Synthesis and Biological Evaluation. Eur. J. Med. Chem. 54, 549–556. doi:10.1016/j.ejmech.2012.06.001
Siber, T., Bušić, V., Zobundžija, D., Roca, S., Vikić-Topić, D., Vrandečić, K., et al. (2019). An Improved Method for the Quaternization of Nicotinamide and Antifungal Activities of its Derivatives. Molecules 24, 1001. doi:10.3390/molecules24061001
Soussi, M. A., Provot, O., Bernadat, G., Bignon, J., Wdzieczak-Bakala, J., Desravines, D., et al. (2014). Discovery of Azaisoerianin Derivatives as Potential Antitumors Agents. Eur. J. Med. Chem. 78, 178–189. doi:10.1016/j.ejmech.2014.03.032
Sun, S., Jia, Q., and Zhang, Z. (2019). Applications of Amide Isosteres in Medicinal Chemistry. Bioorg. Med. Chem. Lett. 29, 2535–2550. doi:10.1016/j.bmcl.2019.07.033
Tang, Y., Zhang, X., Chen, Z., Yin, W., Nan, G., Tian, J., et al. (2018). Novel Benzamido Derivatives as PTP1B Inhibitors with Anti-hyperglycemic and Lipid-Lowering Efficacy. Acta Pharm. Sin. B 8, 919–932. doi:10.1016/j.apsb.2018.05.001
Tsikolia, M., Bernier, U. R., Agramonte, N. M., Estep, A. S., Becnel, J. J., Linthicum, K. J., et al. (2019). Insecticidal and Repellent Properties of Novel Trifluoromethylphenyl Amides III. Pesticide Biochem. Physiology 161, 5–11. doi:10.1016/j.pestbp.2019.06.012
Tsikolia, M., Bernier, U. R., Agramonte, N. M., Estep, A. S., Becnel, J. J., Tabanca, N., et al. (2018). Insecticidal and Repellent Properties of Novel Trifluoromethylphenyl Amides II. Pesticide Biochem. Physiology 151, 40–46. doi:10.1016/j.pestbp.2018.08.006
Wang, H., Gao, X., Zhang, X., Jin, H., Tao, K., and Hou, T. (2017). Design, Synthesis and Antifungal Activity of Novel Fenfuram-Diarylamine Hybrids. Bioorg. Med. Chem. Lett. 27, 90–93. doi:10.1016/j.bmcl.2016.11.026
Wang, S., Wang, X., and Xie, L. (2013). Synthesis and Biological Evaluation of Diarylamines with Antitumor Activity. Yao Xue Xue Bao 48, 1273–1280.
Wild, C. T., Zhu, Y., Na, Y., Mei, F., Ynalvez, M. A., Chen, H., et al. (2016). Functionalized N,N-diphenylamines as Potent and Selective EPAC2 Inhibitors. ACS Med. Chem. Lett. 7, 460–464. doi:10.1021/acsmedchemlett.5b00477
Xu, T., Shi, L., Zhang, Y., Wang, K., Yang, Z., and Ke, S. (2019). Synthesis and Biological Evaluation of Marine Alkaloid-Oriented β-carboline Analogues. Eur. J. Med. Chem. 168, 293–300. doi:10.1016/j.ejmech.2019.02.060
Yang, W., Li, Y., Ai, Y., Obianom, O. N., Guo, D., Yang, H., et al. (2019). Pyrazole-4-Carboxamide (YW2065): A Therapeutic Candidate for Colorectal Cancer via Dual Activities of Wnt/β-Catenin Signaling Inhibition and AMP-Activated Protein Kinase (AMPK) Activation. J. Med. Chem. 62, 11151–11164. doi:10.1021/acs.jmedchem.9b01252
Yu, X., Shi, L., and Ke, S. (2015). Acylhydrazone Derivatives as Potential Anticancer Agents: Synthesis, Bio-Evaluation and Mechanism of Action. Bioorg. Med. Chem. Lett. 25, 5772–5776. doi:10.1016/j.bmcl.2015.10.069
Yu, X., Teng, P., Zhang, Y., Xu, Z., Zhang, M., and Zhang, W. (2018). Design, Synthesis and Antifungal Activity Evaluation of Coumarin-3-Carboxamide Derivatives. Fitoterapia 127, 387–395. doi:10.1016/j.fitote.2018.03.013
Keywords: diarylamine, diamides, carboxamide, synthesis, bioactivity
Citation: Ke S, Huang W, Zhang Z, Wang Y, Zhang Y, Wu Z, Fang W, Wan Z, Gong Y, Yang J, Wang K and Shi L (2022) Diarylamine-Guided Carboxamide Derivatives: Synthesis, Biological Evaluation, and Potential Mechanism of Action. Front. Chem. 10:953523. doi: 10.3389/fchem.2022.953523
Received: 26 May 2022; Accepted: 23 June 2022;
Published: 12 July 2022.
Edited by:
Marco Tutone, University of Palermo, ItalyReviewed by:
Alessandra Montalbano, University of Palermo, ItalyCopyright © 2022 Ke, Huang, Zhang, Wang, Zhang, Wu, Fang, Wan, Gong, Yang, Wang and Shi. This is an open-access article distributed under the terms of the Creative Commons Attribution License (CC BY). The use, distribution or reproduction in other forums is permitted, provided the original author(s) and the copyright owner(s) are credited and that the original publication in this journal is cited, in accordance with accepted academic practice. No use, distribution or reproduction is permitted which does not comply with these terms.
*Correspondence: Shaoyong Ke, c2hhb3lvbmcua2VAbmJlcmMuY29t; Kaimei Wang, a2FpbWVpLndhbmdAbmJlcmMuY29t; Liqiao Shi, bGlxaWFvLnNoaUBuYmVyYy5jb20=
Disclaimer: All claims expressed in this article are solely those of the authors and do not necessarily represent those of their affiliated organizations, or those of the publisher, the editors and the reviewers. Any product that may be evaluated in this article or claim that may be made by its manufacturer is not guaranteed or endorsed by the publisher.
Research integrity at Frontiers
Learn more about the work of our research integrity team to safeguard the quality of each article we publish.