- College of Optoelectronic Engineering, Chengdu University of Information Technology, Chengdu, China
Exciplexes possessing thermally activated delayed fluorescence (TADF) characteristics have received much attention in the fields of organic light-emitting materials and devices over the past decade. In general, an exciplex is a physical mixture between a donor (D) with hole transport properties and an acceptor (A) with electron transport characteristics, and the energy difference between the lowest excited singlet state and the lowest excited triplet state is usually fairly small in terms of the long-range charge-transfer process from D to A. In the processes of photoluminescence and electroluminescence, triplet excitons can be converted to singlet excitons through reverse intersystem crossing and then radiate photons to achieve TADF. As a consequence, triplet excitons can be effectively harvested, and the exciton utilization can be significantly enhanced. Up to now, a large number of exciplexes have been developed and applied to organic light-emitting devices. Notably most of them showed green or red emission, while blue exciplexes are relatively few owing to the spectrum characteristics of the large red-shift and broadened emission. In this study, the latest progress of blue exciplex–based organic light-emitting materials and devices is briefly reviewed, and future research is prospected.
Introduction
Since the realization of the first organic light-emitting diode (OLED) possessing a high brightness of >1,000 cd m−2 and a low-driving voltage (Von) of <10 V (Tang and VanSlyke, 1987), OLEDs based on small molecules (Tang et al., 1989; Adachi et al., 1990), polymers (Burroughes et al., 1990; Peng et al., 1998), and metal–organic complexes (Baldo et al., 1998; Chang et al., 2013) have attracted tremendous attention in the fields of lighting and displays over the past few decades owing to their fascinating merits such as thinness, fast response, and flexibility (Hong et al., 2021). Among these OLEDs, several different kinds of luminescence mechanisms, including traditional fluorescence (Friend et al., 1999; Huang et al., 2012), phosphorescence (Bernhard et al., 2002; Zhou et al., 2014), triplet–triplet annihilation (TTA) (Fukagawa et al., 2012; Jankus et al., 2013), traditional thermally activated delayed fluorescence (TADF) (Endo et al., 2011; Uoyama et al., 2012; Zhang et al., 2012; Li et al., 2013; Li et al., 2021d), hyperfluorescence (Nakanotani et al., 2014; Chan et al., 2021), singlet–triplet inversion (Ehrmaier et al., 2019; Pollice et al., 2021; Li et al., 2022), exciplex-based TADF (Goushi et al., 2012; Li et al., 2014; Oh et al., 2015; Li et al., 2021c; Gu et al., 2022), aggregation-induced emission (AIE)–based TADF (Peng and Shuai, 2021; Suman et al., 2021), and multiple resonance (MR)–based TADF (Lee et al., 2020; Stavrou et al., 2021; Wu et al., 2021; Zou et al., 2022) have been reported. Thus, the exciplex used to be considered an important reason for poor OLED performance, and it thus should be avoided and eliminated (Adachi et al., 1990; Jenekhe, 1995; Morteani et al., 2003). Nevertheless, the studies on enhanced exciplex emission over the past decade suggested the possibility of exciplexes as unique light-emitting materials (Goushi and Adachi, 2012; Sarma and Wong, 2018; Zhang et al., 2021) or highly efficient cohost materials for OLEDs with high efficiencies, low Von, and low roll-offs (Liu et al., 2015b; Wu et al., 2017; Xiao et al., 2018; Wang et al., 2019; Zhao et al., 2019; Jung and Lee, 2020).
In general, an exciplex originates from the intermolecular charge-transfer (CT)–excited state between the highest occupied molecular orbital of an electron donor (D) and the lowest unoccupied molecular orbital of an electron acceptor (A), leading to a fairly small energy difference (ΔEST) between the lowest excited singlet state (S1) and the lowest excited triplet state (T1) by means of the long-range CT process from D to A (Sarma and Wong, 2018; Shao et al., 2022). In the processes of photoluminescence (PL) and electroluminescence (EL), nonradiative triplet excitons can be converted to be radiative singlet excitons through efficient reverse intersystem crossing (RISC). Thus, triplet excitons can be effectively harvested and the luminescence efficiencies can be significantly enhanced, thus ensuring that exciplexes are an important class of emitters in OLEDs (Hung et al., 2014; Kim and Kim, 2019; Guo et al., 2021). Up to now, a large number of exciplex-based molecular systems have been developed and applied to OLEDs. In particular, most of them are green or red exciplexes, while blue exciplexes are relatively few (Zhang et al., 2021). This is mainly because the realization of blue exciplexes is a herculean task on account of a large red-shifted and broadened exciplex emission spectrum as compared to those of the corresponding D and A compounds (Guo et al., 2021). In this study, the latest progress of blue exciplex–based organic light-emitting materials and devices is briefly reviewed, and future research is prospected. The chemical structures of compounds forming blue exciplexes involved in the following descriptions are depicted in Figure 1, and the EL performance of blue exciplex–based OLEDs is summarized in Table 1.
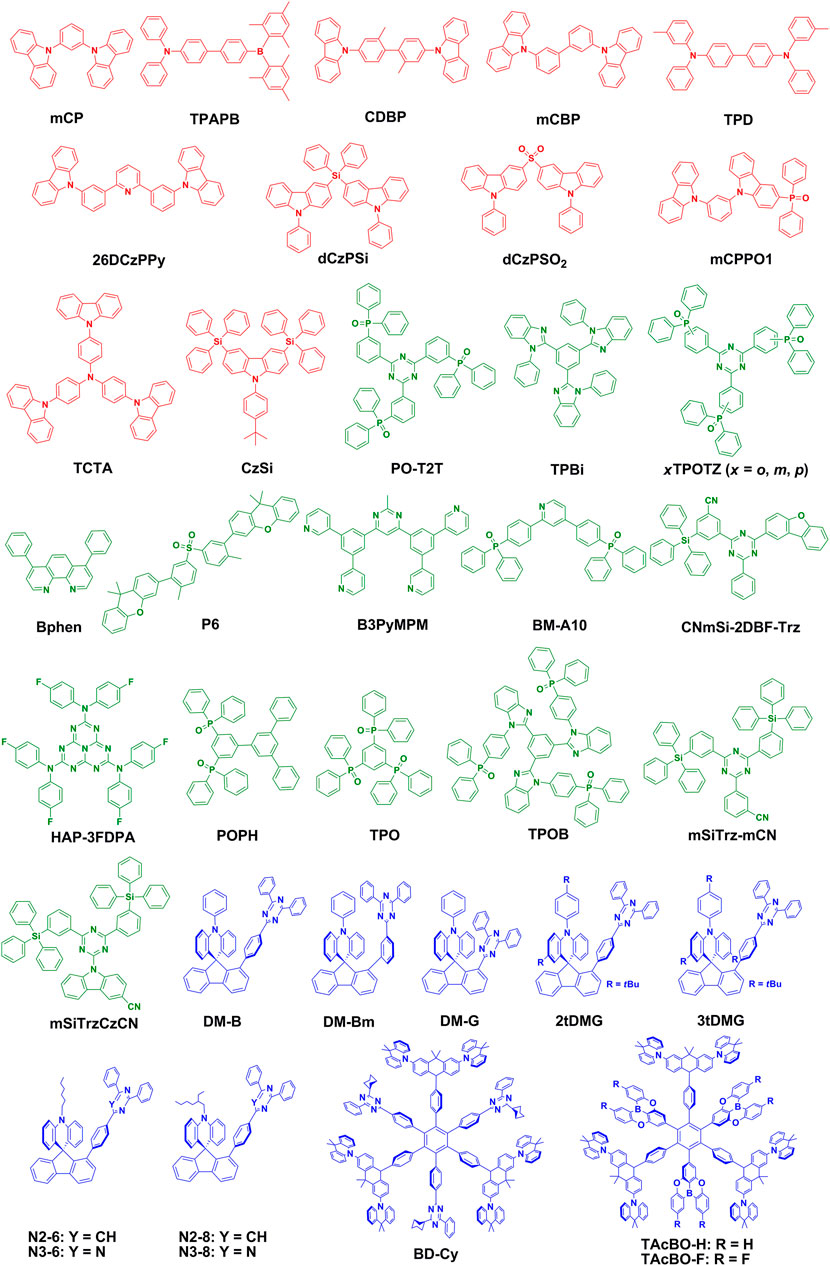
FIGURE 1. Chemical structures of compounds forming blue exciplexes. The red, green, and blue colors represent donors, acceptors, and intramolecular exciplexes, respectively.
Blue Exciplex–Based Organic Light-Emitting Materials and Devices
Although exciplex emission has been studied for decades, exciplex-based organic light-emitting materials have not received extensive attention for a long time, mainly because of their relatively low luminous efficiency and poor color purity (Gould et al., 1992; Osaheni and Jenekhe, 1994; Gebler et al., 1997; Chao and Chen, 1998; Wang et al., 1998; Cocchi et al., 2002; Kulkarni and Jenekhe, 2008; Zhao et al., 2008; Zhu et al., 2009; Yang et al., 2010). Exciplex-based OLEDs have triggered much attention since Goushi and coworkers demonstrated a pronounced EL enhancement using the RISC process in the 4,4′,4″-tris [3-ethylphenyl (phenyl)amino]triphenylamine (m-MTDATA):tris-[3-(3-pyridyl)mesityl]borane (3TPYMB) exciplex system, which showed a relatively high maximum external quantum efficiency (EQEmax) of 5.4% in view of a rather low photoluminescence quantum efficiency (PLQE) of 26%, exceeding the corresponding limit of fluorescence-based OLEDs (Goushi et al., 2012). Afterward, a much higher EQEmax of 10.0% and a maximum power efficiency (PEmax) of 47.0 lm W−1 were achieved by changing the exciplex system from m-MTDATA:3TPYMB to m-MTDATA:2,8-bis(diphenylphosphoryl)dibenzo [b,d]thiophene (PPT) (Goushi and Adachi, 2012).
Since then, exciplex-based emitters have aroused widespread attention by virtue of their fascinating optoelectronic properties, and a number of efficient blue exciplex–based OLEDs have been realized (Shao et al., 2022). In 2014, Hung and coworkers attained a record-high blue exciplex OLED (λEL = 471 nm) with an EQEmax of 8.0% based on 1,3-bis(N-carbazolyl)benzene (mCP):2,4,6-tris[3-(1H-pyrazol-1-yl)phenyl]-1,3,5-triazine (PO-T2T) (Hung et al., 2014). In 2015, Zhao et al. (2015) achieved a blue exciplex–based OLED based on 4,4′,4″-tri (N-carbazolyl)triphenylamine (TCTA):4,7-di-phenyl-1,10-phenanthroline (Bphen), which displayed a low EQEmax of 2.65%. Chen et al. (2015) developed a highly efficient blue exciplex system using a novel electron donor molecule (4-dimesitylboryl)phenyltriphenylamine (TPAPB) and a conventional electron acceptor 1,3,5-tris(1-phenyl-1H-benzimidazol-2-yl)benzene (TPBi), while a blue-emitting device containing TPAPB:TPBi exhibited a low Von of 3.2 V, a high EQEmax of 7.0 ± 0.4%, and CIE coordinates of (0.14, 0.18). Liu and coworkers reported an efficient blue exciplex emitter consisting of 4,4′-bis(9-carbazolyl)-2,2′-dimethylbiphenyl (CDBP):PO-T2T, which showed obvious TADF emission and intrinsically high T1, making itself an excellent candidate as a blue emitter or a host for green and red phosphors (Liu et al., 2015a). Meanwhile, the CDBP:PO-T2T exciplex–based blue device delivered a record-high EQE of 13.0% with an EL emission peak at 480 nm and CIE coordinates of (0.17, 0.29). Zhang et al. (2015) demonstrated that the energy could transfer from blue exciplexes to both fluorescent and phosphorescent orange dopants using an efficient blue exciplex system of 9,9′-biphenyl-3,3′-diylbis-9H-carbazole (mCBP) and PO-T2T as the electron donor and acceptor, respectively, and a high EQEmax of 7.66% with CIE coordinates of (0.17, 0.23) were realized. Liu et al. (2016) investigated the EL property of a blue exciplex of 2,6-bis[3-(9H-carbazol-9-yl)phenyl]pyridine (26DCzPPy):POT2T and an OLED incorporating this exciplex as an emitting layer turned on at 3.1 V, and realized a high EQEmax of 7.8% with blue emission peaked at 488 nm. Based on the acceptors xTPOTZ (x = o, m, or p), which are triphenyltrazine derivatives substituted with diphenylphosphine oxide groups at ortho-, meta-, and para-positions, respectively (Jia et al., 2015), Duan et al. (2018) constructed a series of exciplexes mCP:xTPOTZ (x = o, m, or p). Thus, in virtue of the strongest electron-withdrawing effect and appropriate steric hindrance, an efficient sky-blue OLED based on mCP:pTPOTZ realized an ultralow Von of 2.5 eV, a high CEmax up to 26.2 cd A−1, a high PEmax of 32.4 lm W−1, and an EQEmax of 11.1%. Hung et al. (2018) designed two new nonconjugated linked dicarbazole materials, diphenylbis(9-phenyl-9H-carbazol-3-yl)silane (dCzPSi) and 3,3′-sulfonylbis(9-phenyl-9H-carbazole) (dCzPSO2). The electron-transporting acceptor, PO-T2T, was introduced to give two exciplex-forming systems, dCzPSi:PO-T2T and dCzPSO2:PO-T2T. The dCzPSi:PO-T2T–based device revealed a Von as low as 2.4 V and a high EQEmax of 8.6% with the CIE coordinates of (0.15, 0.21), significantly higher than that of the device based on dCzPSO2:PO-T2T (EQEmax = 1.8%), which is due to the fact that dCzPSO2:PO-T2T possessing a large ΔEST is unfavorable in forming a CT complex.
In 2019, Chapran and coworkers investigated the exciplex properties by selecting PO-T2T as an electron acceptor with different electron donors, and the emissions of these exciplexes spanned from blue to orange-red regions (Chapran et al., 2019). The blue-emitting exciplexes CzSi:PO-T2T–, mCP:PO-T2T–, and mCPPO1:PO-T2T–based OLEDs exhibited high EQEmax of 6.1%, 16.0%, and 6.5%, respectively. Guo et al. (2020) reported a blue exciplex–based OLED based on N,N′-bis(3-methylphenyl)-N,N′-diphenylbenzidine (TPD):Bphen, which exhibited a relatively low EQEmax of 0.46%, possibly resulting from the low PLQE of the exciplex. Tan et al. (2020) designed and investigated a series of donor–acceptor–donor materials based on sulfone substituents as acceptors and found that one of these materials (P6) could form blue exciplexes with TCTA. The TCTA:P6-exciplex–based OLED showed a high EQEmax of 9.1% and deep-blue emission with λEL = 433 nm. Li et al. (2021b) designed and synthesized a heptazine-based electron acceptor, 2,5,8-tris[di (4-fluorophenyl)amine]-1,3,4,6,7,9,9b-heptaazaphenalene (HAP-3FDPA), and the exciplex system of 8 wt% mCP:HAP-3FDPA exhibited a high PLQE of 53.2%. More importantly, an OLED containing this exciplex system as an emitting layer showed deep-blue emission with CIE coordinates of (0.16, 0.12), and a rather high EQEmax of 10.2% along with a low roll-off.
Of late, intramolecular exciplexes based on through-space charge transfer (TSCT) are an attractive class of emitters with spatial D/A architecture and TADF characteristics (Shao and Wang, 2020; Li B. et al., 2021; Xue and Xie, 2021). For intramolecular exciplexes, the D and A segments are spatially proximate to each other but are physically separated by a nonconjugated structure (Shao and Wang, 2020; Yang et al., 2020). Tang and coworkers presented an intramolecular design strategy for exciplex-based emitters via a space-confined CT to enhance the light emission (Tang et al., 2020). By connecting the donor and acceptor units via a rigid linker, the electronic coupling between the donor and acceptor units is sufficient to allow for efficient direct absorption by the CT state. In contrast to more flexible or less strongly coupled samples, the three rigid sky-blue exciplex emitters, 1′-[4-(4,6-diphenyl-1,3,5-triazin-2-yl)phenyl]-10-phenyl-10H-spiro[acridine-9,9′-fluorene] (DM-B), 1′-[3-(4,6-diphenyl-1,3,5-triazin-2-yl)phenyl]-10-phenyl-10H-spiro[acridine-9,9′-fluorene] (DM-Bm) and 1′-(4,6-diphenyl-1,3,5-triazin-2-yl)-10-phenyl-10H-spiro[acridine-9,9′-fluorene] (DM-G) possess very high PLQEs of >90% when incorporated in a solid matrix. The sky-blue OLEDs based on DM-B achieved a fairly high EQEmax of 27.4% along with a small efficiency roll-off. Peng et al. (2020) developed two greenish-blue TADF emitters with a tilted face-to-face alignment of D/A units presenting intramolecular noncovalent interactions, 2-(tert-butyl)-10-[4-(tert-butyl)phenyl]-1′-[4-(4,6-diphenyl-1,3,5-triazin-2-yl)phenyl]-10H-spiro[acridine-9,9′-fluorene] (2tDMG) and 2,7-di-tert-butyl-10-[4-(tert-butyl)phenyl]-1′-[4-(4,6-diphenyl-1,3,5-triazin-2-yl)phenyl]-10H-spiro[acridine-9,9′-fluorene] (3tDMG). 2tDMG and 3tDMG achieved extremely high EQEmax of 30.8% (evaporation-process) and 20.2% (solution-process), respectively. These excellent results opened new avenues for the study of spatial electronic interactions in organic light-emitting materials. Wang T. T. et al. (2021) designed and synthesized four small blue-emitting molecules containing a spiro-scaffold based on fluorene, namely, N2-6, N2-8, N3-6, and N3-8, while the rather short D–A distance led to large steric hindrance as well as a π-stacking manner, favoring CT from D to A. The blue OLED based on N3-8 achieved a high EQEmax of 18.9%. Wang and coworkers reported the design of π-stacked dendrimers consisting of cofacially aligned D and A for highly efficient OLEDs, and the dendritic structure and orthogonal configuration led to the TSCT emission (Wang X. et al., 2021). Thus, the blue device based on the dendrimer BD-Cy showed promising performance with CEmax = 36.8 cd A−1, EQEmax of 18.2%, and PEmax = 36.1 lm W−1. Du et al. (2021) reported two blue TSCT dendrimers consisting of dendritic triacridan donors and oxygen-bridged triarylboron acceptors, TAcBO-H and TAcBO-F. More importantly, the solution-processed OLEDs based on these two dendrimers exhibited blue EL emission and a high EQEmax of >15%.
Blue Exciplexes as Cohost Materials in Blue Phosphorescent Organic Light-Emitting Diodes
Balanced carrier transporting for electron and hole in the emitting layer is significant for the OLED performance in the EL process. To achieve good electron and hole balance, various host materials possessing bipolar characteristics have been developed. There are two approaches to realize bipolar hosts. One is to design single molecules consisting of both hole and electron-transporting units (Su et al., 2008; Lee et al., 2009; Chou and Cheng, 2010). The other is to use exciplex-based cohosts inherently containing hole and electron-transporting molecules (Park et al., 2013a; Park et al., 2013b; Lee et al., 2013). In particular, the latter approach commonly does not require a new molecular synthesis, and the hole and electron mobilities of the exciplex can be tuned by adjusting the ratio of hole and electron-transporting molecules. Thus, exciplex-based cohost materials are conductive to the achievement of OLEDs with low Von and high efficiencies (Park et al., 2013b; Lee et al., 2013). To realize high-performance blue phosphorescent OLEDs (PhOLEDs) utilizing exciplex-based cohost materials, the T1 level of the exciplex should be lower than those of the consisting molecules in order to confine the excitation energy in the exciplex state, not to transfer to the D and A molecules. Meanwhile, the T1 level of the exciplex should also be higher than that of a phosphorescent dopant to guarantee the energy transfer from the exciplex to a blue dopant. Hence, it seems to be a challenging issue to attain an ideal exciplex system meeting these requirements (Jung and Lee, 2020; Tan et al., 2020).
In 2014, Shin et al. (2014) reported a high efficiency blue-emitting PhOLED approaching the theoretical efficiency limit (EQEmax = 29.5%) using a blue exciplex cohost of mCP:bis-4,6-(3,5-di-3-pyridylphenyl)-2-methylpyrimi-dine (B3PYMPM) and a phosphorescent emitter of iridium (III)bis[(4,6-difluorophenyl)-pyridinato-N,C2′]picolinate (FIrpic), and meanwhile, the OLED exhibited a low Von of 3 V and low-efficiency roll-off. In 2015, Ban and coworkers designed and synthesized a novel electron-transporting molecule (5-terphenyl-1,3-phenylene)bis(diphenylphosphine oxide) (POPH), and the solution-processed blue PhOLED incorporating a blue exciplex–based cohost TCTA:POPH displayed an extremely low Von of 2.7 V, a high PEmax of 22.5 lm W−1, and a very low-efficiency roll-off even the luminance was up to 10,000 cd m−1 (Ban et al., 2015). Lee et al. (2015) reported an efficient exciplex–based cohost system of mCP:PO-T2T, and a high-performance blue PhOLED using the exciplex cohost doped with FIrpic possessing a remarkably high EQEmax of 30.3%, PEmax of 66 lm W−1, and a low Von of 2.4 V was realized. Based on the time resolved PL measurement, these results should be ascribed to the suitable T1 level of the exciplex (2.64 eV) ,which is lower than the T1 levels of the consisting molecules of mCP (2.94 eV) and PO-T2T (2.99 eV), and higher than that of FIrpic (2.63 eV), so that the exciplex system well confines the excitons in the exciplex state, followed by energy transfer to a blue dopant of FIrpic. In 2016, Ban et al. realized a highly efficient blue PhOLED based on a blue exciplex cohost system of TCTA:1,3,5-tris(diphenylphosphoryl)benzene (TPO) with the CEmax of 23.8 cd A−1 and PEmax of 15.8 lm W−1 (Ban X. X. et al., 2016). Afterward, they designed and synthesized a novel electron acceptor 1,3,5-tris(1-[4-(diphenylphosphoryl)phenyl]-1H-benzo [d]imidazol-2-yl)benzene (TPOB) to form an exciplex-type cohost with TCTA, and the solution-processed blue PhOLED achieved an extremely low Von of 2.8 V and a high PEmax of 22 lm W−1 along with a low-efficiency roll-off (Ban X. et al., 2016). Lim et al. (2017) developed an exciplex-forming cohost composed of mCP as the donor and 2,4-bis[4-(diphenylphosphoryl)phenyl]pyridine (BM-A10) as the acceptor for deep-blue PhOLEDs achieving a Von of 2.9 eV, a rather high EQEmax of 24% with CIE coordinates of (0.15, 0.21). Yun and coworkers designed n-type molecules with isomeric molecular structure, while the corresponding exciplex cohosts formed by mCBP:3-(4,6-bis[3-(triphenylsilyl)phenyl]-1,3,5-triazin-2-yl)benzonitrile (mSiTrz-mCN) showed blue emission (Yun et al., 2021a). The deep-blue PhOLED employing this exciplex as a cohost showed a low Von of 2.8 V and a high EQEmax of 21.0% with a color coordinate of (0.14, 0.18). Afterward, Yun et al. developed a bipolar n-type host material, 9-(4,6-bis[3-(triphenylsilyl)phenyl]-1,3,5-triazin-2-yl)-9H-carbazole-3-carbonitrile (mSiTrzCzCN), and the blue-emitting mCBP:mSiTrzCzCN exciplex system showed a high T1 energy close to 3.0 eV (Yun et al., 2021b). The mCBP:mSiTrzCzCN exciplex–based deep-blue PhOLED realized a high EQEmax of 21.8% and a lifetime elongation of more than double relative to the conventional n-type host-based device. Kim et al. developed three n-type hosts to form blue exciplex with mCBP (Kim et al., 2022). Among them, the exciplex developed by mCBP:CNmSi-2DBF-Trz showed a high T1 of 2.95 eV and the fabricated blue PhOLED showed a rather high EQEmax over 23%.
Conclusion and Outlook
In summary, this study provided an overview of blue exciplex–based organic light-emitting materials and devices. The research background and luminescence mechanism were briefly introduced. Benefiting from the intriguing merits of exciplex-based OLEDs including low-driving voltages and low-efficiency roll-offs, simultaneously, as well as simple device structures, exciplexes have drawn significant attention on account of the potentials for efficient electroluminescence or for the use as high-performance cohost materials. Manipulating blue exciplex emissions by adjusting molecular structures gives an ideal strategy to fully utilize all exciton energies for high-performance OLEDs. We believe that our work will be conductive to the future development of blue exciplex–based OLEDs with high efficiencies and simplified device structures. Meanwhile, we expect that further systematic investigations of the excited-state dynamics and the structure-property relationships will be of benefit for the development of more efficient exciplex–based emitters and cohost materials oriented for WOLEDs and solution-processed OLEDs.
Author Contributions
JL and QG conceived the idea and supervised the whole work. ZL, HL, HG, and JZ collected the articles and revised the manuscript. All authors contributed to the manuscript revision and approved the submitted version.
Funding
This work was financially supported by the National Natural Science Foundation of China (61505015, 21801028), and Department of Science and Technology of Sichuan Province (2022NSFSC1200, 2019YJ0358).
Conflict of Interest
The authors declare that the research was conducted in the absence of any commercial or financial relationships that could be construed as a potential conflict of interest.
Publisher’s Note
All claims expressed in this article are solely those of the authors and do not necessarily represent those of their affiliated organizations, or those of the publisher, the editors, and the reviewers. Any product that may be evaluated in this article, or claim that may be made by its manufacturer, is not guaranteed or endorsed by the publisher.
References
Adachi, C., Tsutsui, T., and Saito, S. (1990). Blue Light‐emitting Organic Electroluminescent Devices. Appl. Phys. Lett. 56, 799–801. doi:10.1063/1.103177
Baldo, M. A., O'Brien, D. F., You, Y., Shoustikov, A., Sibley, S., Thompson, M. E., et al. (1998). Highly Efficient Phosphorescent Emission from Organic Electroluminescent Devices. Nature 395, 151–154. doi:10.1038/25954
Ban, X., Sun, K., Sun, Y., Huang, B., and Jiang, W. (2016a). Enhanced Electron Affinity and Exciton Confinement in Exciplex-type Host: Power Efficient Solution-Processed Blue Phosphorescent Oleds with Low Turn-On Voltage. ACS Appl. Mat. Inter. 8, 2010–2016. doi:10.1021/acsami.5b10335
Ban, X. X., Sun, K. Y., Sun, Y. M., Huang, B., and Jiang, W. (2016b). Design of High Triplet Energy Electron Transporting Material for Exciplex-type Host: Efficient Blue and White Phosphorescent Oleds Based on Solution Processing. Org. Electron. 33, 9–14. doi:10.1016/j.orgel.2016.02.041
Ban, X. X., Sun, K. Y., Sun, Y. M., Huang, B., Ye, S. H., Yang, M., et al. (2015). High Power Efficiency Solution-Processed Blue Phosphorescent Organic Light-Emitting Diodes Using Exciplex-type Host with a Turn-On Voltage Approaching the Theoretical Limit. ACS Appl. Mat. Inter. 7, 25129–25138. doi:10.1021/acsami.5b06424
Bernhard, S., Gao, X., Malliaras, G. G., and Abruña, H. D. (2002). Efficient Electroluminescent Devices Based on a Chelated Osmium(ii) Complex. Adv. Mat. 14, 433–436. doi:10.1002/1521-4095(20020318)14:6<433::aid-adma433>3.0.co;2-w
Burroughes, J. H., Bradley, D. D. C., Brown, A. R., Marks, R. N., Mackay, K., Friend, R. H., et al. (1990). Light-emitting-diodes Based on Conjugated Polymers. Nature 347, 539–541. doi:10.1038/347539a0
Chan, C. Y., Tanaka, M., Lee, Y. T., Wong, Y. W., Nakanotani, H., Hatakeyama, T., et al. (2021). Stable Pure-Blue Hyperfluorescence Organic Light-Emitting Diodes with High-Efficiency and Narrow Emission. Nat. Photonics 15, 203–207. doi:10.1038/s41566-020-00745-z
Chang, S. H., Chang, C. F., Liao, J. L., Chi, Y., Zhou, D. Y., Liao, L. S., et al. (2013). Emissive Osmium(ii) Complexes with Tetradentate Bis(pyridylpyrazolate) Chelates. Inorg. Chem. 52, 5867–5875. doi:10.1021/ic302829e
Chao, C., and Chen, S. (1998). White Light Emission from Exciplex in a Bilayer Device with Two Blue Light-Emitting Polymers. Appl. Phys. Lett. 73, 426–428. doi:10.1063/1.121888
Chapran, M., Pander, P., Vasylieva, M., Wiosna-Salyga, G., Ulanski, J., Dias, F. B., et al. (2019). Realizing 20% External Quantum Efficiency in Electroluminescence with Efficient Thermally Activated Delayed Fluorescence from an Exciplex. ACS Appl. Mat. Inter. 11, 13460–13471. doi:10.1021/acsami.8b18284
Chen, Z., Liu, X. K., Zheng, C. J., Ye, J., Liu, C. L., Li, F., et al. (2015). High Performance Exciplex-Based Fluorescence-Phosphorescence White Organic Light-Emitting Device with Highly Simplified Structure. Chem. Mat. 27, 5206–5211. doi:10.1021/acs.chemmater.5b01188
Chou, H. H., and Cheng, C. H. (2010). A Highly Efficient Universal Bipolar Host for Blue, Green, and Red Phosphorescent Oleds. Adv. Mat. 22, 2468–2471. doi:10.1002/adma.201000061
Cocchi, M., Virgili, D., Giro, G., Fattori, V., Di Marco, P., Kalinowski, J., et al. (2002). Efficient Exciplex Emitting Organic Electroluminescent Devices. Appl. Phys. Lett. 80, 2401–2403. doi:10.1063/1.1467614
Du, B., Wang, X., Chen, F., Yang, Q., Shao, S., Wang, L., et al. (2021). Through-space Charge Transfer Dendrimers Employing Oxygen-Bridged Triarylboron Acceptors for Efficient Deep-Blue Electroluminescence. Chem. Commun. 57, 7144–7147. doi:10.1039/d1cc02311j
Duan, C. B., Han, C. M., Du, R. M., Wei, Y., and Xu, H. (2018). High-efficiency Blue Dual-Emissive Exciplex Boosts Full-Radiative White Electroluminescence. Adv. Opt. Mat. 6, 1800437. doi:10.1002/Adom.201800437
Ehrmaier, J., Rabe, E. J., Pristash, S. R., Corp, K. L., Schlenker, C. W., Sobolewski, A. L., et al. (2019). Singlet-triplet Inversion in Heptazine and in Polymeric Carbon Nitrides. J. Phys. Chem. A 123, 8099–8108. doi:10.1021/acs.jpca.9b06215
Endo, A., Sato, K., Yoshimura, K., Kai, T., Kawada, A., Miyazaki, H., et al. (2011). Efficient Up-Conversion of Triplet Excitons into a Singlet State and its Application for Organic Light Emitting Diodes. Appl. Phys. Lett. 98, 083302. doi:10.1063/1.3558906
Friend, R. H., Gymer, R. W., Holmes, A. B., Burroughes, J. H., Marks, R. N., Taliani, C., et al. (1999). Electroluminescence in Conjugated Polymers. Nature 397, 121–128. doi:10.1038/16393
Fukagawa, H., Shimizu, T., Ohbe, N., Tokito, S., Tokumaru, K., and Fujikake, H. (2012). Anthracene Derivatives as Efficient Emitting Hosts for Blue Organic Light-Emitting Diodes Utilizing Triplet-Triplet Annihilation. Org. Electron. 13, 1197–1203. doi:10.1016/j.orgel.2012.03.019
Gebler, D. D., Wang, Y. Z., Blatchford, J. W., Jessen, S. W., Fu, D. K., Swager, T. M., et al. (1997). Exciplex Emission in Bilayer Polymer Light-Emitting Devices. Appl. Phys. Lett. 70, 1644–1646. doi:10.1063/1.118657
Gould, I. R., Farid, S., and Young, R. H. (1992). Relationship between Exciplex Fluorescence and Electron Transfer in Radical Ion Pairs. J. Photochem. Photobiol. A 65, 133–147. doi:10.1016/1010-6030(92)85038-v
Goushi, K., and Adachi, C. (2012). Efficient Organic Light-Emitting Diodes through Up-Conversion from Triplet to Singlet Excited States of Exciplexes. Appl. Phys. Lett. 101, 023306. doi:10.1063/1.4737006
Goushi, K., Yoshida, K., Sato, K., and Adachi, C. (2012). Organic Light-Emitting Diodes Employing Efficient Reverse Intersystem Crossing for Triplet-To-Singlet State Conversion. Nat. Photonics 6, 253–258. doi:10.1038/nphoton.2012.31
Gu, J. N., Tang, Z. Y., Guo, H. Q., Chen, Y., Xiao, J., Chen, Z. J., et al. (2022). Intermolecular Tadf: Bulk and Interface Exciplexes. J. Mat. Chem. C 10, 4521–4532. doi:10.1039/d1tc04950j
Guo, J. F., Zhen, Y. G., Dong, H. L., and Hu, W. P. (2021). Recent Progress on Organic Exciplex Materials with Different Donor-Acceptor Contacting Modes for Luminescent Applications. J. Mat. Chem. C 9, 16843–16858. doi:10.1039/d1tc04330g
Guo, Y. Y., Zhao, Y. P., Miao, Y. Q., Wang, L. S., Li, T. B., Wang, H., et al. (2020). All-exciplex-based White Organic Light-Emitting Diodes by Employing an Interface-free Sandwich Light-Emitting Unit Achieving High Electroluminescence Performance. J. Mat. Chem. C 8, 12247–12256. doi:10.1039/d0tc02915g
Hong, G., Gan, X., Leonhardt, C., Zhang, Z., Seibert, J., Busch, J. M., et al. (2021). A Brief History of Oleds-Emitter Development and Industry Milestones. Adv. Mat. 33, e2005630. doi:10.1002/adma.202005630
Huang, J. H., Su, J. H., and Tian, H. (2012). The Development of Anthracene Derivatives for Organic Light-Emitting Diodes. J. Mat. Chem. 22, 10977–10989. doi:10.1039/c2jm16855c
Hung, W. Y., Fang, G. C., Lin, S. W., Cheng, S. H., Wong, K. T., Kuo, T. Y., et al. (2014). The First Tandem, All-Exciplex-Based Woled. Sci. Rep. 4, 5161. doi:10.1038/srep05161
Hung, Y. T., Chen, Z. Y., Hung, W. Y., Chen, D. G., and Wong, K. T. (2018). Exciplex Cohosts Employing Nonconjugated Linked Dicarbazole Donors for Highly Efficient Thermally Activated Delayed Fluorescence-Based Organic Light-Emitting Diodes. ACS Appl. Mat. Inter. 10, 34435–34442. doi:10.1021/acsami.8b14070
Jankus, V., Chiang, C. J., Dias, F., and Monkman, A. P. (2013). Deep Blue Exciplex Organic Light-Emitting Diodes with Enhanced Efficiency; P-type or E-type Triplet Conversion to Singlet Excitons? Adv. Mat. 25, 1455–1459. doi:10.1002/adma.201203615
Jenekhe, S. A. (1995). Excited-state Complexes of Conjugated Polymers. Adv. Mat. 7, 309–311. doi:10.1002/adma.19950070314
Jia, J. L., Zhu, L. P., Wei, Y., Wu, Z. B., Xu, H., Ding, D. X., et al. (2015). Triazine-phosphine Oxide Electron Transporter for Ultralow-Voltage-Driven Sky Blue Pholeds. J. Mat. Chem. C 3, 4890–4902. doi:10.1039/c4tc02993c
Jung, M., and Lee, J. Y. (2020). Exciplex Hosts for Blue Phosphorescent Organic Light-Emitting Diodes. J. Inf. Disp. 21, 11–18. doi:10.1080/15980316.2019.1680453
Kim, H. B., and Kim, J. J. (2019). Recent Progress on Exciplex-Emitting Oleds. J. Inf. Disp. 20, 105–121. doi:10.1080/15980316.2019.1650838
Kim, Y. S., Lim, J., Lee, J. Y., Lee, Y., and Choo, C. (2022). Benzonitirile Modified N Type Host for Exciplex Host to Enhance Efficiency and Lifetime in Blue Phosphorescent Organic Light-Emitting Diodes. Chem. Eng. J. 429, 132584. doi:10.1016/j.cej.2021.132584
Kulkarni, A. P., and Jenekhe, S. A. (2008). Blue-green, Orange, and White Organic Light-Emitting Diodes Based on Exciplex Electroluminescence of an Oligoquinoline Acceptor and Different Hole-Transport Materials. J. Phys. Chem. C 112, 5174–5184. doi:10.1021/jp076480z
Lee, H., Karthik, D., Lampande, R., Ryu, J. H., and Kwon, J. H. (2020). Recent Advancement in Boron-Based Efficient and Pure Blue Thermally Activated Delayed Fluorescence Materials for Organic Light-Emitting Diodes. Front. Chem. 8, 373. doi:10.3389/fchem.2020.00373
Lee, J. H., Cheng, S. H., Yoo, S. J., Shin, H., Chang, J. H., Wu, C. I., et al. (2015). An Exciplex Forming Host for Highly Efficient Blue Organic Light Emitting Diodes with Low Driving Voltage. Adv. Funct. Mat. 25, 361–366. doi:10.1002/adfm.201402707
Lee, J., Lee, J. I., Lee, J. Y., and Chu, H. Y. (2009). Stable Efficiency Roll-Off in Blue Phosphorescent Organic Light-Emitting Diodes by Host Layer Engineering. Org. Electron. 10, 1529–1533. doi:10.1016/j.orgel.2009.08.020
Lee, S., Kim, K. H., Limbach, D., Park, Y. S., and Kim, J. J. (2013). Low Roll-Off and High Efficiency Orange Organic Light Emitting Diodes with Controlled Co-doping of Green and Red Phosphorescent Dopants in an Exciplex Forming Co-host. Adv. Funct. Mat. 23, 4105–4110. doi:10.1002/adfm.201300187
Li, B., Yang, Z., Gong, W. Q., Chen, X. H., Bruce, D. W., Wang, S. Y., et al. (2021a). Intramolecular Through-Space Charge Transfer Based Tadf-Active Multifunctional Emitters for High Efficiency Solution-Processed Oled. Adv. Opt. Mat. 9, 2100180. doi:10.1002/Adom.202100180
Li, J., Gong, H., Zhang, J., Liu, H., Tao, L., Wang, Y., et al. (2021b). Efficient Exciplex-Based Deep-Blue Organic Light-Emitting Diodes Employing a Bis(4-Fluorophenyl)amine-Substituted Heptazine Acceptor. Molecules 26, 5568. doi:10.3390/molecules26185568
Li, J., Gong, H., Zhang, J., Zhou, S., Tao, L., Jiang, L., et al. (2021c). Enhanced Electroluminescence Based on a Pi-Conjugated Heptazine Derivative by Exploiting Thermally Activated Delayed Fluorescence. Front. Chem. 9, 693813. doi:10.3389/fchem.2021.693813
Li, J., Li, Z., Liu, H., Gong, H., Zhang, J., Li, X., et al. (2022). Down-conversion-induced Delayed Fluorescence via an Inverted Singlet-Triplet Channel. Dyes Pigments 203, 110366. doi:10.1016/j.dyepig.2022.110366
Li, J., Nakagawa, T., MacDonald, J., Zhang, Q., Nomura, H., Miyazaki, H., et al. (2013). Highly Efficient Organic Light-Emitting Diode Based on a Hidden Thermally Activated Delayed Fluorescence Channel in a Heptazine Derivative. Adv. Mat. 25, 3319–3323. doi:10.1002/adma.201300575
Li, J., Nomura, H., Miyazaki, H., and Adachi, C. (2014). Highly Efficient Exciplex Organic Light-Emitting Diodes Incorporating a Heptazine Derivative as an Electron Acceptor. Chem. Commun. 50, 6174–6176. doi:10.1039/c4cc01590h
Li, J., Zhang, J. C., Gong, H. Q., Tao, L., Wang, Y. Q., and Guo, Q. (2021d). Efficient Deep-Blue Electroluminescence Employing Heptazine-Based Thermally Activated Delayed Fluorescence. Photonics 8, 293. doi:10.3390/Photonics8080293
Lim, H., Shin, H., Kim, K. H., Yoop, S. J., Huh, J. S., and Kim, J. J. (2017). An Exciplex Host for Deep-Blue Phosphorescent Organic Light-Emitting Diodes. ACS Appl. Mat. Inter. 9, 37883–37887. doi:10.1021/acsami.7b10914
Liu, X. K., Chen, W., Thachoth Chandran, H., Qing, J., Chen, Z., Zhang, X. H., et al. (2016). High-performance, Simplified Fluorescence and Phosphorescence Hybrid White Organic Light-Emitting Devices Allowing Complete Triplet Harvesting. ACS Appl. Mat. Inter. 8, 26135–26142. doi:10.1021/acsami.6b07629
Liu, X. K., Chen, Z., Qing, J., Zhang, W. J., Wu, B., Tam, H. L., et al. (2015a). Remanagement of Singlet and Triplet Excitons in Single-Emissive-Layer Hybrid White Organic Light-Emitting Devices Using Thermally Activated Delayed Fluorescent Blue Exciplex. Adv. Mat. 27, 7079–7085. doi:10.1002/adma.201502897
Liu, X. K., Chen, Z., Zheng, C. J., Chen, M., Liu, W., Zhang, X. H., et al. (2015b). Nearly 100% Triplet Harvesting in Conventional Fluorescent Dopant-Based Organic Light-Emitting Devices through Energy Transfer from Exciplex. Adv. Mat. 27, 2025–2030. doi:10.1002/adma.201500013
Morteani, A., Dhoot, A., and Friend, R. (2003). Barrier-free Electron-Hole Capture in Polymer Blend Heterojunction Light-Emitting Diodes. Adv. Mat. 15, 1708–1712. doi:10.1002/adma.200305618
Nakanotani, H., Higuchi, T., Furukawa, T., Masui, K., Morimoto, K., Numata, M., et al. (2014). High-efficiency Organic Light-Emitting Diodes with Fluorescent Emitters. Nat. Commun. 5, 4016. doi:10.1038/ncomms5016
Oh, C. S., Kang, Y. J., Jeon, S. K., and Lee, J. Y. (2015). High Efficiency Exciplex Emitters Using Donor-Acceptor Type Acceptor Material. J. Phys. Chem. C 119, 22618–22624. doi:10.1021/acs.jpcc.5b05292
Osaheni, J. A., and Jenekhe, S. A. (1994). Efficient Blue Luminescence of a Conjugated Polymer Exciplex. Macromolecules 27, 739–742. doi:10.1021/ma00081a018
Park, Y. S., Kim, K. H., and Kim, J. J. (2013a). Efficient Triplet Harvesting by Fluorescent Molecules through Exciplexes for High Efficiency Organic Light-Emitting Diodes. Appl. Phys. Lett. 102, 5. doi:10.1063/1.4802716
Park, Y. S., Lee, S., Kim, K. H., Kim, S. Y., Lee, J. H., and Kim, J. J. (2013b). Exciplex-forming Co-host for Organic Light-Emitting Diodes with Ultimate Efficiency. Adv. Funct. Mat. 23, 4914–4920. doi:10.1002/adfm.201300547
Peng, C. C., Yang, S. Y., Li, H. C., Xie, G. H., Cui, L. S., Zou, S. N., et al. (2020). Highly Efficient Thermally Activated Delayed Fluorescence via an Unconjugated Donor-Acceptor System Realizing Eqe of over 30. Adv. Mat. 32, 2003885. doi:10.1002/adma.202003885
Peng, Q., and Shuai, Z. (2021). Molecular Mechanism of Aggregation‐induced Emission. Aggregate 2, e91. doi:10.1002/agt2.91
Peng, Z., Bao, Z., and Galvin, M. E. (1998). Oxadiazole-containing Conjugated Polymers for Light-Emitting Diodes. Adv. Mat. 10, 680–684. doi:10.1002/(sici)1521-4095(199806)10:9<680::aid-adma680>3.0.co;2-h
Pollice, R., Friederich, P., Lavigne, C., Gomes, G. D., and Aspuru-Guzik, A. (2021). Organic Molecules with Inverted Gaps between First Excited Singlet and Triplet States and Appreciable Fluorescence Rates. Matter 4, 1654–1682. doi:10.1016/j.matt.2021.02.017
Sarma, M., and Wong, K. T. (2018). Exciplex: An Intermolecular Charge-Transfer Approach for Tadf. ACS Appl. Mat. Inter. 10, 19279–19304. doi:10.1021/acsami.7b18318
Shao, J., Chen, C., Zhao, W., Zhang, E., Ma, W., Sun, Y., et al. (2022). Recent Advances of Interface Exciplex in Organic Light-Emitting Diodes. Micromachines 13, 298. doi:10.102903390/mi13020298
Shao, S., and Wang, L. (2020). Through‐space Charge Transfer Polymers for Solution‐processed Organic Light‐emitting Diodes. Aggregate 1, 45–56. doi:10.1002/agt2.4
Shin, H., Lee, S., Kim, K. H., Moon, C. K., Yoo, S. J., Lee, J. H., et al. (2014). Blue Phosphorescent Organic Light-Emitting Diodes Using an Exciplex Forming Co-host with the External Quantum Efficiency of Theoretical Limit. Adv. Mat. 26, 4730–4734. doi:10.1002/adma.201400955
Stavrou, K., Danos, A., Hama, T., Hatakeyama, T., and Monkman, A. (2021). Hot Vibrational States in a High-Performance Multiple Resonance Emitter and the Effect of Excimer Quenching on Organic Light-Emitting Diodes. ACS Appl. Mat. Inter. 13, 8643–8655. doi:10.1021/acsami.0c20619
Su, S. J., Sasabe, H., Takeda, T., and Kido, J. (2008). Pyridine-containing Bipolar Host Materials for Highly Efficient Blue Phosphorescent Oleds. Chem. Mat. 20, 1691–1693. doi:10.1021/cm703682q
Suman, G. R., Pandey, M., and Chakravarthy, A. S. J. (2021). Review on New Horizons of Aggregation Induced Emission: From Design to Development. Mat. Chem. Front. 5, 1541–1584. doi:10.1039/d0qm00825g
Tan, X. F., Volyniuk, D., Matulaitis, T., Keruckas, J., Ivaniuk, K., Helzhynskyy, I., et al. (2020). High Triplet Energy Materials for Efficient Exciplex-Based and Full-Tadf-Based White Oleds. Dyes Pigments 177, 108259. doi:10.1016/J.Dyepig.2020.108259
Tang, C. W., Vanslyke, S. A., and Chen, C. H. (1989). Electroluminescence of Doped Organic Thin-Films. J. Appl. Phys. 65, 3610–3616. doi:10.1063/1.343409
Tang, C. W., and VanSlyke, S. A. (1987). Organic Electroluminescent Diodes. Appl. Phys. Lett. 51, 913–915. doi:10.1063/1.98799
Tang, X., Cui, L. S., Li, H. C., Gillett, A. J., Auras, F., Qu, Y. K., et al. (2020). Highly Efficient Luminescence from Space-Confined Charge-Transfer Emitters. Nat. Mat. 19, 1332–1338. doi:10.1038/s41563-020-0710-z
Uoyama, H., Goushi, K., Shizu, K., Nomura, H., and Adachi, C. (2012). Highly Efficient Organic Light-Emitting Diodes from Delayed Fluorescence. Nature 492, 234–238. doi:10.1038/nature11687
Wang, J., Kawabe, Y., and Peyghambarian, N. (1998). Exciplex Electroluminescence from Organic Bilayer Devices Composed of Triphenyldiamine and Quinoxaline Derivatives. Adv. Mat. 10, 230–233. doi:10.1002/(sici)1521-4095(199802)10:3<230::aid-adma230>3.0.co;2-y
Wang, Q., Tian, Q. S., Zhang, Y. L., Tang, X., and Liao, L. S. (2019). High-efficiency Organic Light-Emitting Diodes with Exciplex Hosts. J. Mat. Chem. C 7, 11329–11360. doi:10.1039/c9tc03092a
Wang, T. T., Xie, G. H., Li, H. C., Yang, S. Y., Li, H., Xiao, Y. L., et al. (2021a). Pi-stacked Thermally Activated Delayed Fluorescence Emitters with Alkyl Chain Modulation. CCS Chem. 3, 1757–1763. doi:10.31635/ccschem.020.202000355
Wang, X., Hu, J., Lv, J., Yang, Q., Tian, H., Shao, S., et al. (2021b). Pi-stacked Donor-Acceptor Dendrimers for Highly Efficient White Electroluminescence. Angew. Chem. Int. Ed. 60, 16585–16593. doi:10.1002/anie.202104145
Wu, X. G., Su, B. K., Chen, D. G., Liu, D. H., Wu, C. C., Huang, Z. X., et al. (2021). The Role of Host-Guest Interactions in Organic Emitters Employing Mr-Tadf. Nat. Photonics 15, 780–786. doi:10.1038/s41566-021-00870-3
Wu, Z. B., Yu, L., Zhao, F. C., Qiao, X. F., Chen, J. S., Ni, F., et al. (2017). Precise Exciton Allocation for Highly Efficient White Organic Light-Emitting Diodes with Low Efficiency Roll-Off Based on Blue Thermally Activated Delayed Fluorescent Exciplex Emission. Adv. Opt. Mat. 5, 1700415. doi:10.1002/adom.201700415
Xiao, P., Huang, J. H., Yu, Y. C., Yuan, J., Luo, D. X., Liu, B. Q., et al. (2018). Recent Advances of Exciplex-Based White Organic Light-Emitting Diodes. Appl. Sci. 8, 1449. doi:10.3390/App8091449
Xue, Q., and Xie, G. H. (2021). Thermally Activated Delayed Fluorescence beyond Through-Bond Charge Transfer for High-Performance Oleds. Adv. Opt. Mat. 9, 2002204. doi:10.1002/Adom.202002204
Yang, C. C., Hsu, C. J., Chou, P. T., Cheng, H. C., Su, Y. O., and Leung, M. K. (2010). Excited State Luminescence of Multi-(5-Phenyl-1,3,4-Oxadiazo-2-Yl)benzenes in an Electron-Donating Matrix: Exciplex or Electroplex? J. Phys. Chem. B 114, 756–768. doi:10.1021/jp9093063
Yang, S. Y., Wang, Y. K., Peng, C. C., Wu, Z. G., Yuan, S., Yu, Y. J., et al. (2020). Circularly Polarized Thermally Activated Delayed Fluorescence Emitters in Through-Space Charge Transfer on Asymmetric Spiro Skeletons. J. Am. Chem. Soc. 142, 17756–17765. doi:10.1021/jacs.0c08980
Yun, J. H., Lee, K. H., Chung, W. J., Lee, J. Y., Lee, Y., and Lyu, J. J. (2021a). Thermally Activated Delayed Fluorescence Type Exciplex Host for Long Lifetime in Deep Blue Phosphorescent Organic Light-Emitting Diodes. Chem. Eng. J. 417, 128086. doi:10.1016/j.cej.2020.128086
Yun, J. H., Lim, J., Lee, J. Y., Lee, Y., and Chu, C. (2021b). Triplet Exciton Upconverting Blue Exciplex Host for Deep Blue Phosphors. Chem-Eur. J. 27, 12642–12648. doi:10.1002/chem.202101819
Zhang, M., Zheng, C. J., Lin, H., and Tao, S. L. (2021). Thermally Activated Delayed Fluorescence Exciplex Emitters for High-Performance Organic Light-Emitting Diodes. Mat. Horiz. 8, 401–425. doi:10.1039/d0mh01245a
Zhang, Q. S., Li, J., Shizu, K., Huang, S. P., Hirata, S., Miyazaki, H., et al. (2012). Design of Efficient Thermally Activated Delayed Fluorescence Materials for Pure Blue Organic Light Emitting Diodes. J. Am. Chem. Soc. 134, 14706–14709. doi:10.1021/ja306538w
Zhang, T., Zhao, B., Chu, B., Li, W., Su, Z., Yan, X., et al. (2015). Simple Structured Hybrid Woleds Based on Incomplete Energy Transfer Mechanism: From Blue Exciplex to Orange Dopant. Sci. Rep. 5, 10234. doi:10.1038/srep10234
Zhao, B., Zhang, T. Y., Chu, B., Li, W. L., Su, Z. S., Luo, Y. S., et al. (2015). Highly Efficient Tandem Full Exciplex Orange and Warm White Oleds Based on Thermally Activated Delayed Fluorescence Mechanism. Org. Electron. 17, 15–21. doi:10.1016/j.orgel.2014.11.014
Zhao, C. Y., Yan, D. H., Ahamad, T., Alshehri, S. M., and Ma, D. G. (2019). High Efficiency and Low Roll-Off Hybrid White Organic Light Emitting Diodes by Strategically Introducing Multi-Ultrathin Phosphorescent Layers in Blue Exciplex Emitter. J. Appl. Phys. 125, 045501. doi:10.1063/1.5047861
Zhao, D. W., Zhang, F. J., Xu, C., Sun, J. Y., Song, S. F., Xu, Z., et al. (2008). Exciplex Emission in the Blend of Two Blue Luminescent Materials. Appl. Surf. Sci. 254, 3548–3552. doi:10.1016/j.apsusc.2007.11.049
Zhou, L., Kwong, C. L., Kwok, C. C., Cheng, G., Zhang, H., and Che, C. M. (2014). Efficient Red Electroluminescent Devices with Sterically Hindered Phosphorescent Platinum(ii) Schiff Base Complexes and Iridium Complex Codopant. Chem. Asian J. 9, 2984–2994. doi:10.1002/asia.201402618
Zhu, J. Z., Li, W. L., Han, L. L., Chu, B., Zhang, G., Yang, D. F., et al. (2009). Very Broad White-Emission Spectrum Based Organic Light-Emitting Diodes by Four Exciplex Emission Bands. Opt. Lett. 34, 2946–2948. doi:10.1364/ol.34.002946
Keywords: blue exciplex, organic light-emitting diode, cohost, reverse intersystem crossing, thermally activated delayed florescence
Citation: Li J, Li Z, Liu H, Gong H, Zhang J and Guo Q (2022) Advances in Blue Exciplex–Based Organic Light-Emitting Materials and Devices. Front. Chem. 10:952116. doi: 10.3389/fchem.2022.952116
Received: 24 May 2022; Accepted: 03 June 2022;
Published: 12 July 2022.
Edited by:
Haichang Zhang, Qingdao University of Science and Technology, ChinaCopyright © 2022 Li, Li, Liu, Gong, Zhang and Guo. This is an open-access article distributed under the terms of the Creative Commons Attribution License (CC BY). The use, distribution or reproduction in other forums is permitted, provided the original author(s) and the copyright owner(s) are credited and that the original publication in this journal is cited, in accordance with accepted academic practice. No use, distribution or reproduction is permitted which does not comply with these terms.
*Correspondence: Qiang Guo, cWlhbmdndW9AY3VpdC5lZHUuY24=