- 1Department of Medicinal Chemistry, School of Pharmacy, Xi’an Jiaotong University, Xi’an, SN, China
- 2Xi’an Changqing Chemical Group Co., Ltd, Xi’an, SN, China
- 3Qingyuan Edible Fungi Research Center, Lishui, ZJ, China
- 4Institute of Materia Medica, Chinese Academy of Sciences, Shanghai, China
- 5Zhongshan Institute for Drug Discovery, Shanghai Institute of Materia Medica, Chinese Academy of Sciences, Zhongshan, China
- 6Key Laboratory of Surface & Interface Science of Polymer Materials of Zhejiang Province, Department of Chemistry, Zhejiang Sci-Tech University, Hangzhou, China
A photo-induced C-S radical cross-coupling of aryl iodides and disulfides under transition-metal and external photosensitizer free conditions for the synthesis of aryl sulfides at room temperature has been presented, which features mild reaction conditions, broad substrate scope, high efficiency, and good functional group compatibility. The developed methodology could be readily applied to forge C-S bond in the field of pharmaceutical and material science.
Introduction
Aryl sulfides, as a ubiquitous structural motif in functional molecules, plays a unique role in pharmaceutical (Le Grand et al., 2008; Ilardi et al., 2014; Xiao et al., 2019; Golosov et al., 2021; Mourtas et al., 2020; Hai et al., 2021; Liu and Jiang, 2013; Barce Ferro et al., 2020) and material science (Figure 1). (Boyd 2016; Lu et al., 2017; Zhang et al., 2018; Hou et al., 2022) Hence, the development of efficient methods for the construction of C-S bond has attracted considerable attentions from chemical researchers. Traditionally, transition metal-catalyzed cross coupling of aryl halides and thiol/thiophenol constitutes the mainstream route to aryl sulfides (Figure 2A) (Fernández-Rodríguez et al., 2006; Alvaro and Hartwig, 2009; Timpa et al., 2014; Fu et al., 2015; Amiri et al., 2016a; Guzmán-Percástegui et al., 2016; Shiri et al., 2016; Chen et al., 2019; Gupta 2022; Jones et al., 2018; Liu et al., 2019; Isshiki et al., 2021; Cheng-Yi Wang et al., 2021; Yu et al., 2021). However, several troublesome drawbacks still exist, including expensive catalysts and ligands, high temperature and narrow substrate scope. In addition, the metal catalyst-poisoning enabled by thiols further limited the applicability of these reactions, so several alternatives to thiols had been explored (Clark et al., 1989; Akkilagunta and Kakulapati, 2011; Ke et al., 2011; Park et al., 2011; Prasad and Sekar, 2011; Reddy et al., 2011; Singh et al., 2013; Zhao et al., 2013; Firouzabadi et al., 2015; Tber et al., 2016; Ge et al., 2019; Liu et al., 2020). Although the use of thiol substitutes can eliminate the difficulties caused by thiols, these methods also have certain disadvantage, such as the tedious procedures for the synthesis of S-Alkylisothiouronium salt (Zhao et al., 2007), 1,3-propanedithiol equivalent (Liu et al., 2003) and 2-[bis (alkylthio) methylene]-3-oxo-N-o-tolylbutanamides (Dong et al., 2006). The pursuit of more efficient and environment-friendly approaches for the formation of C-S bond is of great urgency. Consequently, numerous newly developed methods emerge to replace the traditional methods. For instance, transition metal-catalyzed decarbonylation of thioester from readily available carboxylic acids has been regarded as another noteworthy strategy for achieving C–S bond formation (Figure 2B). (Ichiishi et al., 2018; Bie et al., 2021; Han Cao et al., 2021; Liu and Szostak, 2021) Reduction of sulfoxides also serves as a direct route to sulfides (Shiri and Kazemi, 2017; Lin et al., 2021; Zhang 2022). The synthesis of aryl sulfides using phenylboronic acid as substrate requires the participation of magnetic nanoparticles or expensive transition metals (Xu et al., 2012; Rostami et al., 2015a; Rostami et al., 2015b; Amiri et al., 2016a; Amiri et al., 2016b; Rostami et al., 2017; Wang et al., 2017; Cheng et al., 2018; Farzin et al., 2018; Atashkar et al., 2019; Gavhane et al., 2019; Khakyzadeh et al., 2019). With the development of photocatalytic reaction, photocatalytic synthesis of aryl sulfide has become an effective way. In the past few years, the photoredox transition metal-catalyzed C–S cross-coupling between aryl halides and thiols/disulfides have been widely developed, in which a series of Cu, Ni, Pd, and Rh transition metals are still utilized as catalysts (Figure 2C). (Uyeda et al., 2013; Wang et al., 2013; Johnson et al., 2016; Jouffroy et al., 2016; Jouffroy et al., 2016; Oderinde et al., 2016; Li et al., 2020; Sandfort et al., 2020; Brahmachari et al., 2021; Qin et al., 2021; Yang et al., 2021)
Meanwhile, A series of photo-induced transition-metal and photosensitizer free C−S cross-coupling methods has been developed (Bunnett and Creary, 1974; Liu et al., 2017; Pramanik et al., 2020; Dawei Cao et al., 2021; Nandy et al., 2021; Saroha et al., 2021; Shun Wang et al., 2021; Uchikura et al., 2021; Wang et al., 2022). For the metal-free synthesis of aryl sulfides, Hong and co-workers developed a convergent, organocatalytic visible-light-mediated process for the synthesis of diaryl sulfides (Hong et al., 2017). Kibriya’s group developed a metal-free visible-light-promoted oxidative coupling between thiols and arylhydrazines to afford diaryl sulfides using a catalytic amount of rose bengal as photocatalyst under aerobic conditions (Kibriya et al., 2018). Inspired by the aforementioned seminal studies and our pursuit of developing greener and more sustainable methods to forge C-S bonds, we have developed a metal-free photo-catalyzed C-S cross-coupling of aryl iodide and disulfides for the efficient synthesis of aryl sulfides under mild conditions (Figure 2D). It is worth noting that the present reaction features many advantages, including the use of clean and renewable light source, no participation of metal and photosensitizer, high efficiency, and excellent functional group compatibility, providing an environmentally friendly and expedient approach for the construction of aryl sulfides and congeners.
Results and discussion
The C-S cross-coupling between 4-iodophenol (1e) and 1,2- diphenyldisulfide (2a) was selected as the model reaction for the optimization of reaction conditions. The reaction parameters, including the equivalent of disulfide, light, solvent, base, reaction time, were examined and the results were summarized in Table 1; Supplementary Table S1, S2. The investigation towards the amounts of disulfides was first conducted, as demonstrated that 0.5 equiv. of diphenyldisulfide 2a enabled the formation of 4-(phenylthio) phenol (3e) in 56% yield (Table 1, Entry 1). The further increase of the disulfide amounts failed to improve the reaction yields (Table 1, Entry 2-3 and Supplementary Table S2). Then, the base effect of the reaction was investigated by using diverse organic and inorganic bases, which indicated that 50 mol% of TMG (1,1,3,3-Tetramethylguanidine) could give the best result (Table 1, entries 4-7 and Supplementary Table S2). Other additives were also tested and inferior yields were obtained (Supplementary Table S2). Subsequently, the screening of organic solvents revealed DMSO and ethyl acetate acted as the good medium, whereas other protic solvents and non-polar solvents led to the yield decline Table 1, entries 8-10 and Supplementary Table S2). Furthermore, shortening the reaction time had a detrimental impact on the reaction, whereas prolonging the reaction time to 18 h or 24 h could not obviously promote the reaction yield (Table 1, entries 12-14). The sources of light were also evaluated by the employment of 500 W Xe lamp, blue and green LED, 35 W white fluorescent lamp and in the dark, but no product 3e was detected under the above conditions. (Table 1, entry 14 and Supplementary Table S1). Finally, the air atmosphere could sharply inhibit the reaction. (Table 1, entry 15 and Supplementary Table S2).
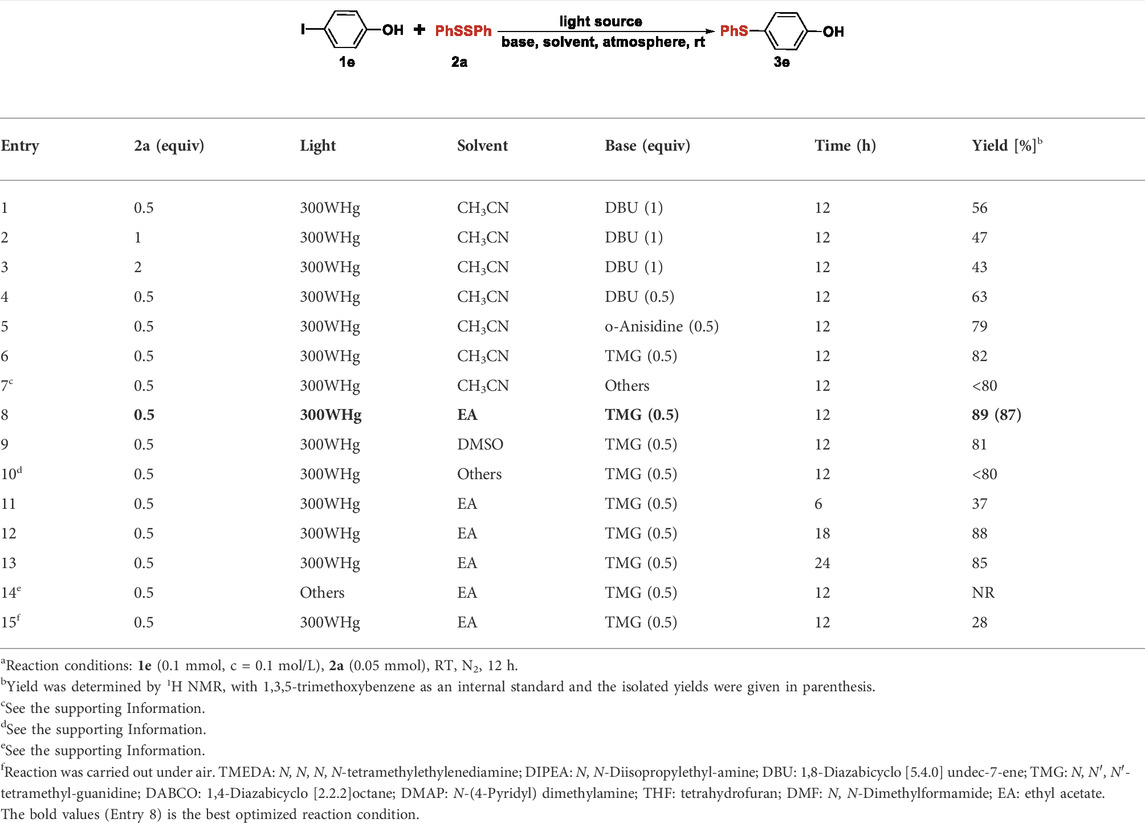
TABLE 1. Optimization of the reaction conditionsa.
With the optimized reaction conditions in hand, the scope of the photo-catalyzed C-S cross-coupling was investigated with a variety of (hetero) aryl iodides and disulfides and the results were summarized in Table 2. Various substituted phenyl iodides reacted smoothly with diphenyldisulfide to deliver the corresponding aryl sulfides 3a–i in good yields, irrespective of the electronic effect of the substituents. Noteworthy is that several sensitive functional groups, such as −OH, −NO2 and allyl, were all tolerant in the current reaction system (3e–g, 3ab). The transformation is also applicable to the aryl iodides with strong electron-withdrawing groups, producing the sulfide products 3h–i in 68%–80% yields. In addition, p-tolyl disulfide and 4,4′- dithiodiphenol were viable substrates to participate in the reaction to lead to the desired products 3aa and 3ab with high efficiency.
The substrate scope of the photo-catalyzed C-S cross-coupling was further extended with different (hetero) aryl iodides and dimethyl disulfide. As shown in Table 3, the cross coupling of (hetero) aryl iodides with dimethyl disulfide proceeded to afford the methyl (aryl) sulfane products 3j-z in moderate to excellent yields. Various substituents, including −CN, −CO2Me, −CF3, naphthalene and heterocyclic scaffolds, were all compatible with the reaction system. The electron effect and the steric hindrance of the (hetero) aryl iodides exerted a marginal influence on the reaction, as verified by the comparable yields of the obtained products. The observation depicted in Tables 2, 3 exhibited the broad substrate scope and excellent functional group tolerance of the photo-catalyzed C-S bond cross coupling reaction.
The protocol could also be applied to construct C-Se and C-Te bond by using diselenide and ditelluride as coupling partners (Table 4). A series of aryl selenoethers 3ac-am were obtained in moderate to excellent yields, in which several sensitive functional groups and strong electron-withdrawing substituents were tolerant. In addition, two diaryltellane products 3an and 3ao were furnished with high efficiency under the current photo-reduced reaction system.
To investigate the mechanism of sulfuration reaction, the on-off experiment was carried out and no product formation was observed during the dark conditions in this experiment (Figure 3). It demonstrated that light irradiation is crucial for this sulfuration reaction and a radical chain propagation pathway is possibly not involved in the reaction.
To gain mechanistic understanding of this transformation, we turned our attention toward exploring the key intermediate and the nature of the reaction pathway. As depicted in Eq. 1 and Figure 4, when the radical scavengers of TEMPO (2,2,6,6-tetramethylpiperidine-N-oxide) was added into the reaction, the desired product 3e was not detected at all and the coupling product 4 of aryl radical with TEMPO was successfully detected by LC-MS, which indicated that the reaction possibly involved a radical process.
On the basis of the above experimental results and related literatures (Discekici et al., 2015; Dong et al., 2019; Wu et al., 2019), a plausible mechanism of this reaction has been proposed (Figure 5). Under the irradiation of ultraviolet light, the aryl iodide 1 absorbs energy to reach the excited state 1’, and the bond of disulfide subsequently undergoes homolysis process to produce sulfur radical 7 (Schmidt et al., 1964; Ogawa et al., 1998). Then, the strong base cleaves the carbon-iodine bond uniformly to produce aryl radical 5 and iodine radical 6. Finally, the cross coupling of free radicals 7 and 5 delivers the desired aryl sulfoether product 3. In addition, the remaining iodine radicals are transformed into elemental iodine through homocoupling reaction1.
Conclusion
In conclusion, an efficient and transition metal-free photo-catalyzed C-S cross-coupling reaction have been developed. An array of (hetero) aryl sulfides could be accessed from the readily available aryl/hetero iodides and disulfides in an easily-operative and environment-friendly manner. A series of aryl selenoethers and diaryltellanes were also delivered by the developed method. The protocol is also charactered by no participation of metal catalyst or photosensitizer, broad substrate scope and good functional group tolerance. It is expected that this methodology will have wide application in the synthesis of functional sulfur-containing molecules in the field of pharmaceutical and material science.
Data availability statement
The original contributions presented in the study are included in the article/Supplementary Material, further inquiries can be directed to the corresponding authors.
Author contributions
SM conceived and designed the experiments; YZ and ZL carried out the experiments and data test; RW and BY performed some synthesis; JH and XY performed compounds characterization; LH and SZ contributed to data analysis and discussion; SM, MW, and ZC wrote the paper.
Acknowledgments
This work is supported by the National Natural Science Foundation of China (21602169 and 81803490), Qingyuan Edible fungi Research Center (202107182) and Xi’an Jiaotong University (334100038). The authors also thank Chao Feng at the Instrument Analysis Center of XJTU for the NMR and IR analysis.
Conflict of interest
Author ZL was employed by Xi’an Changqing Chemical Group Co., Ltd.
The remaining authors declare that the research was conducted in the absence of any commercial or financial relationships that could be construed as a potential conflict of interest.
Publisher’s note
All claims expressed in this article are solely those of the authors and do not necessarily represent those of their affiliated organizations, or those of the publisher, the editors and the reviewers. Any product that may be evaluated in this article, or claim that may be made by its manufacturer, is not guaranteed or endorsed by the publisher.
Supplementary material
The Supplementary Material for this article can be found online at: https://www.frontiersin.org/articles/10.3389/fchem.2022.941016/full#supplementary-material
Footnotes
1According to standard conditions, the system was dark-brown after the reaction was completed. When an appropriate amount of sodium thiosulfate solution was added, the system became colourless and transparent. The comparison before and after the reaction can be found in the SI.
References
Akkilagunta, V. K., and Kakulapati, R. R. (2011). Synthesis of unsymmetrical sulfides using ethyl potassium xanthogenate and recyclable copper catalyst under ligand-free conditions. J. Org. Chem. 76, 6819–6824. doi:10.1021/jo200793k
Alvaro, E., and Hartwig, J. F. (2009). Resting state and elementary steps of the coupling of aryl halides with thiols catalyzed by alkylbisphosphine complexes of palladium. J. Am. Chem. Soc. 131, 7858–7868. doi:10.1021/ja901793w
Amiri, K., Rostami, A., and Rostami, A. (2016b). CuFe 2 O 4 magnetic nanoparticle catalyzed odorless synthesis of sulfides using phenylboronic acid and aryl halides in the presence of S 8. New J. Chem. 40, 7522–7528. doi:10.1039/c6nj01434h
Amiri, K., Rostami, A., Samadi, S., and Rostami, A. (2016a). Cu-ZSM5 as reusable catalyst for the one-pot, odorless and ligand-free C-S bond formation. Catal. Commun. 86, 108–112. doi:10.1016/j.catcom.2016.07.019
Atashkar, B., Rostami, A., Rostami, A., and Zolfigol, M. A. (2019). NiFe2O4 as a magnetically recoverable nanocatalyst for odourless C–S bond formation via the cleavage of C–O bond in the presence of S8 under mild and green conditions. Appl. Organomet. Chem. 33, e4691. doi:10.1002/aoc.4691
Barce Ferro, C. T., Dos Santos, B. F., da Silva, C. D. G., Brand, G., da Silva, B. A. L., de Campos Domingues, N. L., et al. (2020). Review of the syntheses and activities of some sulfur-containing drugs. Curr. Org. Synth. 17, 192–210. doi:10.2174/1570179417666200212113412
Bie, F., Liu, X., Cao, H., Shi, Y., Zhou, T., Szostak, M., et al. (2021). Pd-catalyzed double-decarbonylative aryl sulfide synthesis through aryl exchange between amides and thioesters. Org. Lett. 23, 8098–8103. doi:10.1021/acs.orglett.1c03232
Boyd, D. A. (2016). Sulfur and its role in modern materials science. Angew. Chem. Int. Ed. 55, 15486–15502. doi:10.1002/anie.201604615
Brahmachari, G., Bhowmick, A., and Karmakar, I. (2021). Visible light-driven and singlet oxygen-mediated photochemical cross-dehydrogenative C3–H sulfenylation of 4-hydroxycoumarins with thiols using rose bengal as a photosensitizer. J. Org. Chem. 86, 9658–9669. doi:10.1021/acs.joc.1c00919
Bunnett, J., and Creary, X. (1974). Arylation of arenethiolate ions by the SRNl mechanism. Convenient synthesis of diaryl sulfides. J. Org. Chem. 39, 3173–3174. doi:10.1021/jo00935a037
Chen, L., Noory Fajer, A., Yessimbekov, Z., Kazemi, M., and Mohammadi, M. (2019). Diaryl sulfides synthesis: Copper catalysts in C–S bond formation. J. Sulfur Chem. 40, 451–468. doi:10.1080/17415993.2019.1596268
Cheng, Y., Liu, X., and Dong, Z. B. (2018). Phenyldithiocarbamates: Efficient sulfuration reagents in the Chan–Lam coupling reaction. Eur. J. Org. Chem., 815–820. doi:10.1002/ejoc.201701631
Cheng-Yi Wang, C.-Y., Tian, R., and Zhu, Y.-M. (2021). Ni-catalyzed C–S bond cleavage of aryl 2-pyridyl thioethers coupling with alkyl and aryl thiols. Tetrahedron 99, 132453. doi:10.1016/j.tet.2021.132453
Clark, J. H., Jones, C. W., Duke, C. V. A., and Miller, J. M. (1989). Aromatic thiocyanation using supported copper(I) thiocyanate. J. Chem. Soc. Chem. Commun., 81–82. doi:10.1039/c39890000081
Dawei Cao, D., Pan, P., Li, C.-J., and Zeng, H. (2021). Photo-induced transition-metal and photosensitizer free cross–coupling of aryl halides with disulfides. Green Synth. Catal. 2, 303–306. doi:10.1016/j.gresc.2021.04.006
Discekici, E. H., Treat, N. J., Poelma, S. O., Mattson, K. M., Hudson, Z. M., Luo, Y., et al. (2015). A highly reducing metal-free photoredox catalyst: Design and application in radical dehalogenations. Chem. Commun. 51, 11705–11708. doi:10.1039/c5cc04677g
Dong, D., Yu, H., Ouyang, Y., Liu, Q., Bi, X., Lu, Y., et al. (2006). Thia-michael addition reactions using 2-[Bis(alkylthio)methylene]-3-oxo-N-o-tolylbutanamides as odorless and efficient thiol equivalents. Synlett 2006, 283–287. doi:10.1055/s-2005-923602
Dong, Y., Su, Y., Du, L., Wang, R., Zhang, L., Zhao, D., et al. (2019). Plasmon-enhanced deuteration under visible-light irradiation. ACS Nano 13, 10754–10760. doi:10.1021/acsnano.9b05523
Farzin, S., Rahimi, A., Amiri, K., Rostami, A., and Rostami, A. (2018). Synthesis of diaryl sulfides via nickel ferrite‐catalysed C─ S bond formation in green media. Appl. Organomet. Chem. 32, e4409. doi:10.1002/aoc.4409
Fernández-Rodríguez, M. A., Shen, Q., and Hartwig, J. F. (2006). A general and long-lived catalyst for the palladium-catalyzed coupling of aryl halides with thiols. J. Am. Chem. Soc. 128, 2180–2181. doi:10.1021/ja0580340
Firouzabadi, H., Iranpoor, N., Gorginpour, F., and Samadi, A. (2015). Dithiooxamide as an effective sulfur surrogate for odorless high-yielding carbon–sulfur bond formation in wet PEG200 as an eco-friendly, safe, and recoverable solvent. Eur. J. Org. Chem., 2914–2920. doi:10.1002/ejoc.201500156
Fu, W., Liu, T., Fang, Z., Ma, Y., Zheng, X., Wang, W., et al. (2015). High activity and stability in the cross-coupling of aryl halides with disulfides over Cu-doped hierarchically porous zeolite ZSM-5. Chem. Commun. 51, 5890–5893. doi:10.1039/c4cc10417j
Gavhane, D. S., Sarkate, A. P., Karnik, K. S., Jagtap, S. D., Ansari, S. H., Izankar, A. V., et al. (2019). Nano copper catalyzed microwave assisted coupling of benzene boronic acids with thiophenols. Lett. Org. Chem. 16, 491–494. doi:10.2174/1570178616666181116113243
Ge, X., Cheng, L., Sun, F., Liu, X., Chen, X., Qian, C., et al. (2019). Mechanistic and experimental study on copper-catalyzed C3-sulfenylation of indoles with sulfur powder and aryl iodides. Catal. Commun. 123, 32–37. doi:10.1016/j.catcom.2019.01.015
Golosov, A. A., Flyer, A. N., Amin, J., Babu, C., Gampe, C., Li, J., et al. (2021). Design of thioether cyclic peptide scaffolds with passive permeability and oral exposure. J. Med. Chem. 64, 2622–2633. doi:10.1021/acs.jmedchem.0c01505
Gupta, S. (2022). Magnetic nanoparticles supported sulfuric acid as a green and efficient nanocatalyst for oxidation of sulfides and oxidative coupling of thiols. J. Synth. Chem. 1, 16–21.
Guzmán-Percástegui, E., Hernández, D. J., and Castillo, I. (2016). Calix[8]arene nanoreactor for Cu(i)-catalysed C–S coupling. Chem. Commun. 52, 3111–3114. doi:10.1039/c5cc09232a
Hai, Y., Wei, M.-Y., Wang, C.-Y., Gu, Y.-C., and Shao, C.-L. (2021). The intriguing chemistry and biology of sulfur-containing natural products from marine microorganisms (1987–2020). Mar. Life Sci. Technol. 3, 488–518. doi:10.1007/s42995-021-00101-2
Han Cao, H., Liu, X., Bie, F., Shi, Y., Han, Y., Yan, P., et al. (2021). Rh(I)-Catalyzed intramolecular decarbonylation of thioesters. J. Org. Chem. 86, 10829–10837. doi:10.1021/acs.joc.1c01117
Hong, B., Lee, J., and Lee, A. (2017). Visible-light-promoted synthesis of diaryl sulfides under air. Tetrahedron Lett. 58, 2809–2812. doi:10.1016/j.tetlet.2017.06.006
Hou, J.-T., Kwon, N., Wang, S., Wang, B., He, X., Yoon, J., et al. (2022). Sulfur-based fluorescent probes for HOCl: Mechanisms, design, and applications. Coord. Chem. Rev. 450, 214232. doi:10.1016/j.ccr.2021.214232
Ichiishi, N., Malapit, C. A., Woźniak, Ł., and Sanford, M. S. (2018). Palladium- and nickel-catalyzed decarbonylative C–S coupling to convert thioesters to thioethers. Org. Lett. 20, 44–47. doi:10.1021/acs.orglett.7b03305
Ilardi, E. A., Vitaku, E., and Njardarson, J. T. (2014). Data-mining for sulfur and fluorine: An evaluation of pharmaceuticals to reveal opportunities for drug design and discovery. J. Med. Chem. 57, 2832–2842. doi:10.1021/jm401375q
Isshiki, R., Kurosawa, M. B., Muto, K., and Yamaguchi, J. (2021). Ni-catalyzed aryl sulfide synthesis through an aryl exchange reaction. J. Am. Chem. Soc. 143, 10333–10340. doi:10.1021/jacs.1c04215
Johnson, M. W., Hannoun, K. I., Tan, Y., Fu, G. C., and Peters, J. C. (2016). A mechanistic investigation of the photoinduced, copper-mediated cross-coupling of an aryl thiol with an aryl halide. Chem. Sci. 7, 4091–4100. doi:10.1039/c5sc04709a
Jones, K. D., Power, D. J., Bierer, D., Gericke, K. M., and Stewart, S. G. (2018). Nickel phosphite/phosphine-catalyzed C–S cross-coupling of aryl chlorides and thiols. Org. Lett. 20, 208–211. doi:10.1021/acs.orglett.7b03560
Jouffroy, M., Kelly, C. B., and Molander, G. A. (2016). Thioetherification via photoredox/nickel dual catalysis. Org. Lett. 18, 876–879. doi:10.1021/acs.orglett.6b00208
Ke, F., Qu, Y., Jiang, Z., Li, Z., Wu, D., Zhou, X., et al. (2011). An efficient copper-catalyzed Carbon−Sulfur bond formation protocol in water. Org. Lett. 13, 454–457. doi:10.1021/ol102784c
Khakyzadeh, V., Rostami, A., Veisi, H., Shaghasemi, B. S., Reimhult, E., Luque, R., et al. (2019). Direct C–S bond formation via C–O bond activation of phenols in a crossover Pd/Cu dual-metal catalysis system. Org. Biomol. Chem. 17, 4491–4497. doi:10.1039/c9ob00313d
Kibriya, G., Mondal, S., and Hajra, A. (2018). Visible-light-mediated synthesis of unsymmetrical diaryl sulfides via oxidative coupling of arylhydrazine with thiol. Org. Lett. 20, 7740–7743. doi:10.1021/acs.orglett.8b03549
Le Grand, B., Pignier, C., Létienne, R., Cuisiat, F., Rolland, F., Mas, A., et al. (2008). Sodium late current blockers in ischemia reperfusion: Is the bullet magic? J. Med. Chem. 51, 3856–3866. doi:10.1021/jm800100z
Li, J., Yang, X.-E., Wang, S.-L., Zhang, L.-L., Zhou, X.-Z., Wang, S.-Y., et al. (2020). Visible-light-promoted cross-coupling reactions of 4-alkyl-1, 4-dihydropyridines with thiosulfonate or selenium sulfonate: A unified approach to sulfides, selenides, and sulfoxides. Org. Lett. 22, 4908–4913. doi:10.1021/acs.orglett.0c01776
Lin, H., Wu, L., and Kazemi, M. (2021). A decade updates (2011–2020): Reduction of sulfoxides to sulfides. Synth. Commun. 51, 1609–1635.
Liu, B., Lim, C.-H., and Miyake, G. M. (2017). Visible-light-promoted C–S cross-coupling via intermolecular charge transfer. J. Am. Chem. Soc. 139, 13616–13619. doi:10.1021/jacs.7b07390
Liu, C., and Szostak, M. (2021). Forging C−S bonds through decarbonylation: New perspectives for the synthesis of privileged aryl sulfides. ChemCatChem 13, 4878–4881. doi:10.1002/cctc.202101206
Liu, H., and Jiang, X. (2013). Transfer of sulfur: From simple to diverse. Chem. Asian J. 8, 2546–2563. doi:10.1002/asia.201300636
Liu, Q., Che, G., Yu, H., Liu, Y., Zhang, J., Zhang, Q., et al. (2003). The first nonthiolic, odorless 1, 3-propanedithiol equivalent and its application in thioacetalization. J. Org. Chem. 68, 9148–9150. doi:10.1021/jo034702t
Liu, D., Ma, H.-X., Fang, P., and Mei, T.-S. (2019). Nickel-catalyzed thiolation of aryl halides and heteroaryl halides through electrochemistry. Angew. Chem. Int. Ed. 58, 5033–5037. doi:10.1002/anie.201900956
Liu, Y., Lam, L. Y., Ye, J., Blanchard, N., and Ma, C. (2020). DABCO-Promoted diaryl thioether formation by metal-catalyzed coupling of sodium sulfinates and aryl iodides. Adv. Synth. Catal. 362, 2326–2331. doi:10.1002/adsc.202000221
Lu, S., Hu, Y., Wan, S., McCaffrey, R., Jin, Y., Gu, H., et al. (2017). Synthesis of ultrafine and highly dispersed metal nanoparticles confined in a thioether-containing covalent organic framework and their catalytic applications. J. Am. Chem. Soc. 139, 17082–17088. doi:10.1021/jacs.7b07918
Mourtas, S., Katakalou, C., Gatos, D., and Barlos, K. (2020). Convergent synthesis of thioether containing peptides. Molecules 25, 218. doi:10.3390/molecules25010218
Nandy, A., Kazi, I., Guha, S., and Sekar, G. (2021). Visible-light-driven halogen-bond-assisted direct synthesis of heteroaryl thioethers using transition-metal-free one-pot C–I bond formation/C–S cross-coupling reaction. J. Org. Chem. 86, 2570–2581. doi:10.1021/acs.joc.0c02672
Oderinde, M. S., Frenette, M., Robbins, D. W., Aquila, B., and Johannes, J. W. (2016). Photoredox mediated nickel catalyzed cross-coupling of thiols with aryl and heteroaryl iodides via thiyl radicals. J. Am. Chem. Soc. 138, 1760–1763. doi:10.1021/jacs.5b11244
Ogawa, A., Obayashi, R., Doi, M., Sonoda, N., and Hirao, T. (1998). A novel photoinduced thioselenation of allenes by use of a Disulfide−Diselenide binary system. J. Org. Chem. 63, 9144. doi:10.1021/jo984988f
Park, N., Park, K., Jang, M., and Lee, S. (2011). One-pot synthesis of symmetrical and unsymmetrical aryl sulfides by Pd-catalyzed couplings of aryl halides and thioacetates. J. Org. Chem. 76, 4371–4378. doi:10.1021/jo2007253
Pramanik, M., Choudhuri, K., and Mal, P. (2020). Metal-free C–S coupling of thiols and disulfides. Org. Biomol. Chem. 18, 8771–8792. doi:10.1039/d0ob01741h
Prasad, D. J. C., and Sekar, G. (2011). Cu-catalyzed one-pot synthesis of unsymmetrical diaryl thioethers by coupling of aryl halides using a thiol precursor. Org. Lett. 13, 1008–1011. doi:10.1021/ol103041s
Qin, Y., Sun, R., Gianoulis, N. P., and Nocera, D. G. (2021). Photoredox nickel-catalyzed C–S cross-coupling: Mechanism, kinetics, and generalization. J. Am. Chem. Soc. 143, 2005–2015. doi:10.1021/jacs.0c11937
Reddy, K. H. V., Reddy, V. P., Shankar, J., Madhav, B., Anil Kumar, B. S. P., Nageswar, Y. V. D., et al. (2011). Copper oxide nanoparticles catalyzed synthesis of aryl sulfides via cascade reaction of aryl halides with thiourea. Tetrahedron Lett. 52, 2679–2682. doi:10.1016/j.tetlet.2011.03.070
Rostami, A., Rostami, A., and Ghaderi, A. (2015a). Copper-catalyzed thioetherification reactions of alkyl halides, triphenyltin chloride, and arylboronic acids with nitroarenes in the presence of sulfur sources. J. Org. Chem. 80, 8694–8704. doi:10.1021/acs.joc.5b01248
Rostami, A., Rostami, A., Ghaderi, A., and Zolfigol, M. A. (2015b). Ligand-free Cu-catalyzed odorless synthesis of unsymmetrical sulfides through cross-coupling reaction of aryl/benzyl/alkyl halides with an aryl boronic acid/S 8 system as a thiolating agent in PEG. RSC Adv. 5, 37060–37065. doi:10.1039/c5ra05244k
Rostami, A., Rostami, A., Ghaderi, A., Gholinejad, M., and Gheisarzadeh, S. (2017). Copper-catalyzed C–S bond formation via the cleavage of C–O bonds in the presence of S8 as the sulfur source. Synthesis 49, 5025–5038. doi:10.1055/s-0036-1588508
Sandfort, F., Knecht, T., Pinkert, T., Daniliuc, C. G., and Glorius, F. (2020). Site-selective thiolation of (Multi)halogenated heteroarenes. J. Am. Chem. Soc. 142, 6913–6919. doi:10.1021/jacs.0c01630
Saroha, M., Sindhu, J., Kumar, S., Bhasin, K. K., Khurana, J. M., Varma, R. S., et al. (2021). Transition metal‐free sulfenylation of C− H bonds for C− S bond formation in recent years: Mechanistic approach and promising future. ChemistrySelect 6, 13077–13208. doi:10.1002/slct.202102042
Schmidt, U., Müller, A., and Markau, K. (1964). Über organische Schweflradikale, VI. Isolierung von Arylschwefel-Radikalen, Benzylschwefel- und Phenylselen-Radikal. Chem. Ber. 97, 405–414. doi:10.1002/cber.19640970213
Shiri, L., Ghorbani-Choghamarani, A., and Kazemi, M. (2016). Sulfides synthesis: Nanocatalysts in C–S cross-coupling reactions. Aust. J. Chem. 69, 585. doi:10.1071/ch15528
Shiri, L., and Kazemi, M. (2017). Deoxygenation of sulfoxides. Res. Chem. Intermed. 43, 6007–6041. doi:10.1007/s11164-017-2976-6
Shun Wang, S., Wang, H., and König, B. (2021). Light-induced single-electron transfer processes involving sulfur anions as catalysts. J. Am. Chem. Soc. 143, 15530–15537. doi:10.1021/jacs.1c07785
Singh, N., Singh, R., Raghuvanshi, D. S., and Singh, K. N. (2013). Convenient MW-assisted synthesis of unsymmetrical sulfides using sulfonyl hydrazides as aryl thiol surrogate. Org. Lett. 15, 5874–5877. doi:10.1021/ol402948k
Tber, Z., Hiebel, M. A., El Hakmaoui, A., Akssira, M., Guillaumet, G., Berteina-Raboin, S., et al. (2016). Fe–Cu catalyzed synthesis of symmetrical and unsymmetrical diaryl thioethers using 1, 3-benzoxazole-2-thiol as a sulfur surrogate. RSC Adv. 6, 72030–72036. doi:10.1039/c6ra15335f
Timpa, S. D., Pell, C. J., and Ozerov, O. V. (2014). A well-defined (POCOP)Rh catalyst for the coupling of aryl halides with thiols. J. Am. Chem. Soc. 136, 14772–14779. doi:10.1021/ja505576g
Uchikura, T., Hara, Y., Tsubono, K., and Akiyama, T. (2021). Visible-light-Driven C–S bond formation based on electron donor–acceptor excitation and hydrogen atom transfer combined system. ACS Org. Inorg. Au 1, 23–28. doi:10.1021/acsorginorgau.1c00007
Uyeda, C., Tan, Y., Fu, G. C., and Peters, J. C. (2013). A new family of nucleophiles for photoinduced, copper-catalyzed cross-couplings via single-electron transfer: Reactions of thiols with aryl halides under mild conditions (O °C). J. Am. Chem. Soc. 135, 9548–9552. doi:10.1021/ja404050f
Wang, X., Cuny, G. D., and Noël, T. (2013). A mild, one-pot stadler–ziegler synthesis of arylsulfides facilitated by photoredox catalysis in batch and continuous-flow. Angew. Chem. Int. Ed. 52, 7860–7864. doi:10.1002/anie.201303483
Wang, L., Xie, Y. B., Huang, N. Y., Zhang, N. N., Li, D. J., Hu, Y. L., et al. (2017). Disulfide‐directed C–H hydroxylation for synthesis of sulfonyl diphenyl sulfides and 2‐(phenylthio) phenols with oxygen as oxidant. Adv. Synth. Catal. 359, 779–785. doi:10.1002/adsc.201600861
Wang, R., Wang, X., Mao, S., Zhao, Y., Yuan, B., Yang, X. Y., et al. (2022). Metal‐free photochemical C− Se cross‐coupling of aryl halides with diselenides. Adv. Synth. Catal. 364, 1607–1612. doi:10.1002/adsc.202200042
Wu, W., Cui, E., Zhang, Y., Zhang, C., Zhu, F., Tung, C.-H., et al. (2019). Involving single-atom silver(0) in selective dehalogenation by AgF under visible-light irradiation. ACS Catal. 9, 6335–6341. doi:10.1021/acscatal.9b01929
Xiao, D., Wang, Y.-j., Hu, X.-b., Kan, W.-j., Zhang, Q., Jiang, X., et al. (2019). Design, synthesis and biological evaluation of the thioether-containing lenalidomide analogs with anti-proliferative activities. Eur. J. Med. Chem. 176, 419–430. doi:10.1016/j.ejmech.2019.05.035
Xu, H.-J., Zhao, Y.-Q., Feng, T., and Feng, Y.-S. (2012). Chan–lam-type S-arylation of thiols with boronic acids at room temperature. J. Org. Chem. 77, 2878–2884. doi:10.1021/jo300100x
Yang, D., Yan, Q., Zhu, E., Lv, J., and He, W.-M. (2021). Carbon–sulfur bond formation via photochemical strategies: An efficient method for the synthesis of sulfur-containing compounds. Chin. Chem. Lett. 33, 1798–1816. doi:10.1016/j.cclet.2021.09.068
Yu, T.-Y., Pang, H., Cao, Y., Gallou, F., and Lipshutz, B. H. (2021). Safe, scalable, inexpensive, and mild nickel-catalyzed migita-like C−S cross-couplings in recyclable water. Angew. Chem. Int. Ed. 60, 3708–3713. doi:10.1002/anie.202013017
Zhang, H.-F. (2022). InBr3-Catalyzed deoxygenation of sulfoxides and carboxylic acids with a hydrosilane. J. Synth. Chem. 1, 42–47.
Zhang, J., Weng, L., Su, X., Lu, G., Liu, W., Tang, Y., et al. (2018). Cisplatin and doxorubicin high-loaded nanodrug based on biocompatible thioether- and ethane-bridged hollow mesoporous organosilica nanoparticles. J. Colloid Interface Sci. 513, 214–221. doi:10.1016/j.jcis.2017.10.116
Zhao, Y., Ge, Z.-M., Cheng, T.-M., and Li, R.-T. (2007). Thia-michael addition using cheap and odorless S-alkylisothiouronium salts as thiol equivalents in water. Synlett 2007, 1529–1532. doi:10.1055/s-2007-982530
Keywords: metal-free, photo-induced, aryl sulfides, cross-coupling, disulfides
Citation: Mao S, Zhao Y, Luo Z, Wang R, Yuan B, Hu J, Hu L, Zhang S-Q, Ye X, Wang M and Chen Z (2022) Metal-free photo-induced sulfidation of aryl iodide and other chalcogenation. Front. Chem. 10:941016. doi: 10.3389/fchem.2022.941016
Received: 11 May 2022; Accepted: 30 June 2022;
Published: 26 July 2022.
Edited by:
Chanatip Samart, Thammasat University, ThailandReviewed by:
Mosstafa Kazemi, University of Ilam, IranChanat Aonbangkhen, Chulalongkorn University, Thailand
Copyright © 2022 Mao, Zhao, Luo, Wang, Yuan, Hu, Hu, Zhang, Ye, Wang and Chen. This is an open-access article distributed under the terms of the Creative Commons Attribution License (CC BY). The use, distribution or reproduction in other forums is permitted, provided the original author(s) and the copyright owner(s) are credited and that the original publication in this journal is cited, in accordance with accepted academic practice. No use, distribution or reproduction is permitted which does not comply with these terms.
*Correspondence: Shuai Mao, c21hbzAxMTVAeGp0dS5lZHUuY24=; Mingliang Wang, d2FuZ21pbmdsaWFuZ0BzaW1tLmFjLmNu; Zhengkai Chen, emtjaGVuQHpzdHUuZWR1LmNu
†These authors have contributed equally to this work