- 1Institute of Carbon Materials Science, Shanxi Datong University, Datong, China
- 2Physics Department, Taiyuan Normal University, Taiyuan, China
- 3Institute of Theoretical Chemistry, College of Chemistry, Jilin University, Changchun, China
A series of hetero-binuclear superatom motifs involving chloride/bromide ligands, that is, MM′X4− (M = Li, Na; M′ = Be, Mg, Ca; X = Cl, Br) anions, have been characterized by using many-body perturbation theory calculations. Large vertical electron detachment energies (VDEs, 5.470–6.799 eV) confirm the superhalogen identity of these anions. A larger VDE value can be obtained by introducing small M or large M′ central atoms and small halogen ligand atoms. Thus, one isomer of LiCaCl4− possesses the largest VDE value. Besides, when the extra electron is shared by all ligand atoms or three bridging ligand atoms, the isomers have relatively larger VDE values.
Introduction
Superhalogens are unusual molecules possessing higher electron affinity (EA) than those of any halogen atom (Gutsev and Boldyrev, 1981). They were first proposed by Gutsev and Boldyrev in 1981 and verified by a variety of theoretical chemical methods (Gutsev and Boldyrev, 1981). Meanwhile, a new class of highly stable anions (superhalogen anions) were also reported. Now, superhalogen anions have been proved to possess very large vertical electron detachment energies (VDEs) (Anusiewicz et al., 2003; Koirala et al., 2010; Yang et al., 2017; Li et al., 2019; Li et al., 2020; Zhao et al., 2020), even approaching 14 eV in certain systems (Freza and Skurski, 2010). Moreover, it is found that the superhalogen anions have much high stability, such as BF4−, AlCl4−, and AsF6−, and other superhalogen anions have been confirmed to be stable in crystalline solids or gaseous molecules.
In 1981, Gutsev and Boldyrev proposed the representative formula MXk+1 for a class of superhalogens, in which M represents the central main group atom, K is the highest valence of M atom, and X is the halogen atom (Gutsev and Boldyrev, 1981). In 1999, the superhalogen anions MX2− (M = Li, Na; X = Cl, Br, and I) were reported by Wang et al., and their VDE values were experimentally measured for the first time and theoretically calculated applying the outer valence Green function (OVGF) method, which are consistent with each other well (Wang et al., 1999). Shortly afterward, the EA value of the BO2 superhalogen (Zhai et al., 2007) and the VDE value of the MX3− (M = Be, Mg, Ca; X = Cl, Br) superhalogen anion (Elliott et al., 2005) were determined by the same experimental means. During subsequent studies on superhalogens and their corresponding anions, the central atom M of MXk+1 formula was no longer limited to the main group metal atoms (Anusiewicz et al., 2003; Elliott et al., 2005), and the transition metal atoms (Gutsev et al., 1999; Gutsev et al., 2001; Yang et al., 2003), coinage metal atoms (Feng et al., 2011; Lu et al., 2019), and nonmetal atoms (Arnold et al., 2002) could act as central atoms to construct superhalogens. In addition, the researchers found that increasing the number of central atoms benefits the dispersion of extra negative charges without increasing the repulsion between ligands. Therefore, some binuclear/multinuclear superhalogen anions have been proposed by experimental synthesis or theoretical prediction (Anusiewicz and Skurski, 2007; Anusiewicz, 2008; Czapla, 2017; Yang et al., 2017; Yang et al., 2018).
Besides, it is realized that halogen atoms are not the necessary units for the construction of superhalogens. In recent years, the ligands of superhalogens have been extended from halogen atoms to oxygen atoms (Gutsev et al., 1999; Zhai et al., 2007), acid functional groups (Anusiewicz, 2009b), various monovalent groups (Smuczynska and Skurski, 2009), nine-electron ligands (Sikorska et al., 2011), hydroxyl groups (Świerszcz and Anusiewicz, 2011), and electrophilic substituents (Anusiewicz, 2009a). In addition, M@Nk-type superhalogens with inclusion complexes of metal (Zhai et al., 2004) and carborane cage superhalogens (Pathak et al., 2011) have also been proposed. Recently, a new class of cluster was designed in which the central atom was modified by superhalogen ligands replacing the halogen ligands. These clusters have higher EAs than their superhalogen ligands; thus, they are termed “hyperhalogen" (Willis et al., 2010). Subsequently, many hyperhalogens with various geometries of superhalogen ligands have been proposed (Paduani et al., 2011; Gutsev et al., 2012; Sun et al., 2015; Sun et al., 2016; Yang et al., 2021; Dong et al., 2022).
Superhalogens play an important role in chemistry given the strong oxidation capability. For example, they can be used as capable oxidants to oxidize substances that have relatively high ionization potentials (e.g., O2 (Bartlett and Lohmann, 1962), noble gas atom (Bartlett, 1962), and (H2O)n clusters (Marchaj et al., 2013)). They can also be used to synthesize and prepare noble gas compounds (Saha et al., 2018; Chang et al., 2019), supersalts (Giri et al., 2014a), ion battery electrolytes (Giri et al., 2014b), ionic liquids (Srivastava et al., 2021), liquid crystalline molecules (Srivastava, 2021), solar cells (Kim et al., 2022), and so on. Therefore, exploring various new species classified as superhalogens and studying their structures, stability, and properties has become a significant and attractive research topic in recent years.
To the best of our knowledge, most hitherto proposed superhalogens are mono- or homo-nuclear. The hetero-nuclear superhalogens involving different central atoms, however, have received very little attention. The investigation on the influence of different ligands on hetero-nuclear superhalogen properties, however, has not been reported yet. In this research, we aim to design a new class of superhalogen anions with two different central atoms using chloride or bromine atoms as ligands. Consequently, the MM′X4− (M = Li, Na; M′ = Be, Mg, Ca; X = Cl, Br) anions have been proposed and systematically investigated. The considerable VDE values of these anions confirm their superhalogen identity. The geometric features and relative stability of these anions were analyzed. Meanwhile, the correlations between their VDEs and structural features, ligand and central atoms, and extra electron distribution are also revealed. The present investigation predicts a new member of superhalogens and conduces to the development of new strong oxidizing agents.
Computational Details
Initially, the structures of the MM′X4− (M = Li, Na; M′ = Be, Mg, Ca; X = Cl, Br) anions were built by considering all the possible connection between M, M′, and X atoms. Then, all the constructed structures of anions were optimized using the Møller–Plesset perturbation method (MP2) (Møller and Plesset, 1934) together with the 6–311+G (3df) basis set (Yang et al., 2017; Yang et al., 2018). Meanwhile, frequency analysis was performed at the same computational level to ensure that the obtained structures are stable on potential energy surfaces without imaginary frequency. Natural bond orbital (NBO) (Reed et al., 1985) and single-point energy calculations were carried out at the same level.
The vertical electron detachment energies (VDEs) of the MM′X4− anions were calculated applying the outer valence Green function (OVGF) approximation (Cederbaum, 1975) with the 6–311+G (3df) basis set. The smallest pole strength (PS) in our study is 0.90, justifying the validity of the OVGF method (Zakrzewski et al., 1996).
The above-mentioned calculations were performed using the GAUSSIAN 16 program package (Frisch et al., 2016). The plots of molecular structures and orbitals were generated with the GaussView program (Dennington et al., 2016).
Results and Discussion
Geometrical Structures and Relative Stability
The optimized geometries of MM'X4−anions are depicted schematically in Figure 1. The relative energies, the lowest vibrational frequencies, bond lengths, and angles are listed in Tables 1, 2. As shown in the figure, each MM'X4− anion has two types of structures, that is, central atoms M and M′ are connected by two or three bridging X atoms. Notably, these two structures are also presented in the superhalogen anions with F ligands (Yang et al., 2017). Unlike MM'F4− anions (Yang et al., 2017), the structures involving one bridging ligand atom are not stable, which turn to the above two types of structures after optimization. In terms of the number of bridging X atoms, the isomers of MM'X4− are termed MM'X4−-2 and MM'X4−-3, respectively. For the sake of convenience, the terminal X atoms that bind with M and M′ atoms are named Xt and Xt’, respectively, and the bridging X atom that connects M and M′ atoms is named Xb.
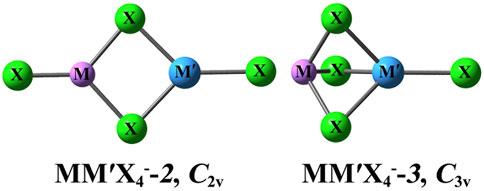
FIGURE 1. Optimized structures of the MM′X4− anions at the MP2/6–311+G (3df) level. Color legend: green for X atom, light purple for M atom, and blue for M′ atom.
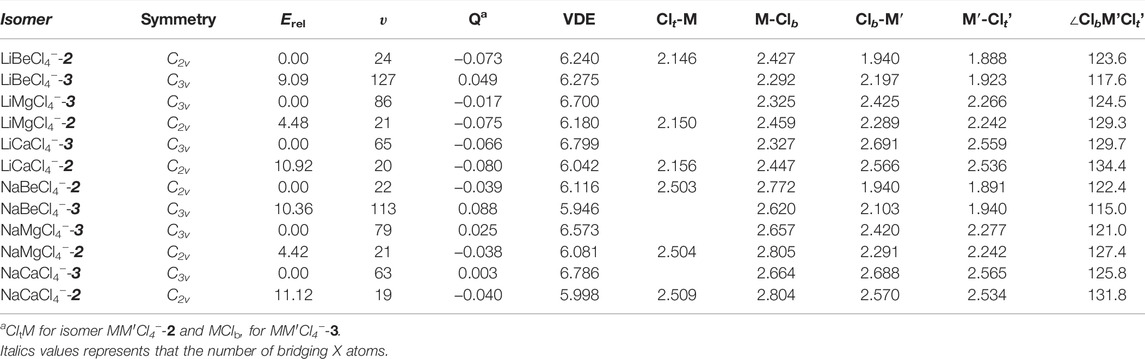
TABLE 1. Relative energies Erel (kcal/mol), the lowest vibrational frequencies υ (cm−1), total NBO charges on the MCl subunit (|e|), vertical detachment energies VDE (eV), bond lengths (Å), and select bond angles (degree) of the MM′Cl4− (M = Li, Na; M′ = Be, Mg, Ca) anions.
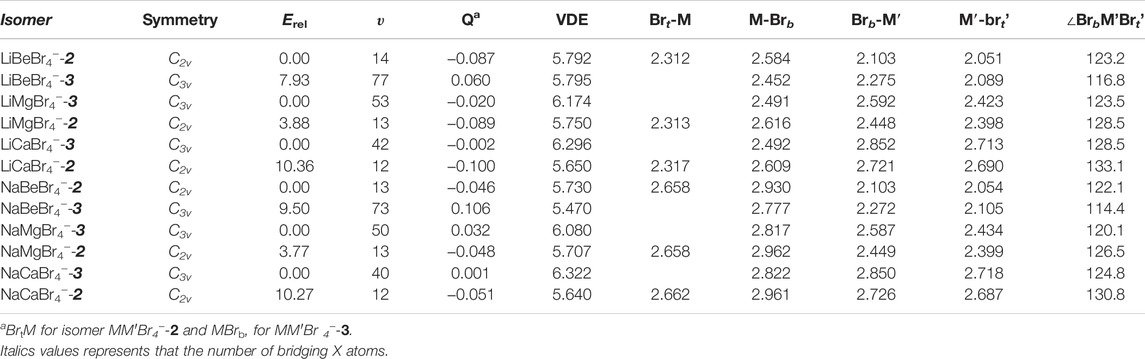
TABLE 2. Relative energies Erel (kcal/mol), the lowest vibrational frequencies υ (cm−1), total NBO charges on MBr subunit (|e|), vertical detachment energies VDE (eV), bond lengths (Å), and select bond angles (degree) of the MM′Br4− (M = Li, Na; M′ = Be, Mg, Ca) anions.
It can be seen in Figure 1 that the MM'Cl4−-2 and MM'Cl4−-3 isomers possess planar and three-dimensional structures with C2v and C3v symmetries, respectively. From the data in Table 1, it is found that for MM'Cl4−-2 and MM'Cl4−-3 isomers, when M′ atom varies from Be to Ca, the Clt-M and M-Clb bond lengths change very little, while the ∠ClbM'Clt angle showed a tendency of increasing; for example, the orders of ∠ClbM'Clt angle in NaM'Cl4−-2 and NaM'Cl4−-3 are 122.4° < 127.4° < 131.8° and 115.0° < 121.0° < 125.8° with varying M′ atoms, respectively. Thus, the MM'Cl4−-2 and MM'Cl4−-3 structures tend to elongate along the M-M′ axis with the increasing radius of M′ atoms. Besides, the Clt-M bond is shorter than the M-Clb bond in MM'Cl4−-2 isomers. For instance, the Clt-Na bonds are about 2.80 Å, while the Na-Clb bonds are 2.50 Å in NaM'Cl4−-2 isomers. On the other hand, when the M atom goes from Li to Na, the Clb-M′ and M′-Clt’ bond lengths also show minor difference in MM'Cl4− anions, but the ∠ClbM'Clt’ angles show a decrease, e.g., the ∠ClbM'Clt’ angles of LiBeCl4−-2 and LiBeCl4−-3 are 1.4 and 1.6° larger than that of the corresponding NaBeCl4−-2 and NaBeCl4−-3, respectively. In addition, the total NBO charges of CltM subunits are in the range of -0.080–0.088|e|, which are close to zero (see Table 1); consequently, the total NBO charges of M'Cl3 subunits approximate -1. In this sense, the MM'Cl4− structures can be regarded as a combination of an MCl molecule and a superhalogen anion M'Cl3−.
MM'Br4− series show similar structural characteristics with MM'Cl4− anions. From the data in Tables 1, 2, it is noticed that ∠XbM'Xt’ angles of MM'Cl4− anions are always larger than that of the corresponding MM'Br4− anions; for example, the ∠ClbM'Clt’ angles of NaMgCl4−-3 and NaMgCl4−-2 are 121.0° and 127.4°, respectively, which are larger than the ∠BrbM'Brt’ angles of NaMgBr4−-3 (120.1°) and NaMgBr4−-2 (126.5°), respectively.
It is reported that the isomers could exhibit higher stability with more bridging ligands in the previous studies on the dual-nuclear superhalogen anions with F ligands, such as homonuclear Mg2F5−(Anusiewicz and Skurski, 2007) and heteronuclear ones NaM'F4−(M' = Mg, Ca) (Yang et al., 2017). MM'X4− anions also follow this rule except the MBeX4− series. However, MBeX4− anions show a reverse trend; that is, structure 2 is more stable than structure 3. In other words, Be atoms are more likely to bond with three ligands than four ligands. This may be due to the smaller atomic radius of the central Be atom, the three bridging ligands are more crowded in structure 3 of MBeX4− anions than in structure 3 of MMgX4− and MCaX4−. This can be confirmed by the shorter Clb-Clb and Brb-Brb distance in structures 3 of MBeX4− than MMgX4− and MCaX4−; for example, the Clb-Clb and Brb-Brb distances in LiBeCl4−-3 and LiBeBr4−-3 are 0.226 Å and 0.229 Å shorter than that in LiMgCl4−-3 and LiMgBr4−-3, respectively. Thereby, MBeX4−-3 is less stable than the MBeX4−-2.
Vertical Electron Detachment Energies (VDEs)
The VDE values of MM'X4− anions are gathered in Tables 1, 2. As one can notice, all anions have considerable VDE values (5.470–6.799 eV) exceeding the electron affinity of the Cl atom; thus undoubtedly, these anions can be identified as superhalogen anions. In addition, it is found that the factors affecting the VDE values of these studied anions were as follows:
(1) When the M atom varies from the Li atom to Na atom, the VDE values of the studied anions show a tendency of decreasing in similar structures. For example, the VDE values of the isomers LiMgCl4−-3 and LiMgCl4−-2 are greater than those of NaMgCl4−-3 and NaMgCl4−-2, respectively. However, the only one exception is the VDE values of isomers LiCaBr4−-3 and NaCaBr4−-3. This is probably due to the different extra electron distribution of these two isomers, which will be discussed in the following. Therefore, the hetero-binuclear superhalogen anions with large VDE values could be constructed by introducing small alkali metal atoms into the system. It is worth noting that the same trend was found for the other hetero-binuclear anions with the F atom, cyanide, and isocyanide as ligands (Yang et al., 2017; Yang et al., 2018).
(2) The largest VDE values for each MM'X4− anions are presented in Table 3. From the table, the VDE values increase in the order: MBeX4−→MMgX4−→MCaX4−. Hence, the hetero-binuclear superhalogen anion MM'X4− could possess a larger VDE value by involving larger alkaline earth metal atoms. Note that it also holds true for the superhalogen anions with other ligands (Yang et al., 2017; Yang et al., 2018).
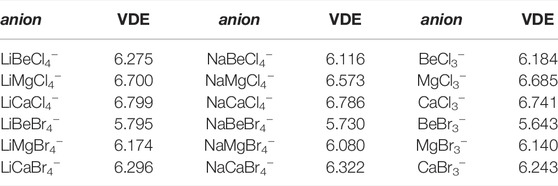
TABLE 3. The largest vertical detachment energies VDE (eV) of superhalogen anions MM′X4− (M = Li, Na; M′ = Be, Mg, Ca, X = Cl, Br) and M′X3− (M′ = Be, Mg, Ca, X = Cl, Br).
As pointed out earlier, the MM'X4− anions can be regarded as MX (M'X3)−; thus, the comparison between MM'X4− anions and their corresponding mononuclear superhalogen anions M'X3− is also necessary. For this reason, the VDE values of M'X3− (X = Cl, Br) anions were also calculated at the same level and are listed in Table 3 as well. From the table, the VDE values of mononuclear anions M'X3− also increase from BeX3− to CaX3−. Besides, the mononuclear anions M'X3− possess lower VDE values than their corresponding hetero-nuclear anions MM'X4− (except for NaBeCl4− and NaMgX4− series). So again, the superhalogen anions could gain larger VDE values by increasing the number of central atoms.
(3) The relationship between the VDE values and the ligand atoms is plotted in Figure 2. The six curves show similar varying trends, that is, the largest VDE values of each MM'X4− (X = F (Yang et al., 2017), Cl, Br) species show a decreasing order: MM'F4− > MM'Cl4− > MM'Br4−. This may be attributed to the different electronegativity of X atoms. To be specific, the F atom possesses larger electronegativity and stronger electron-accepting ability than Cl and Br atoms, which is more beneficial for the anions to bind with the extra electron. Thereby, the larger electronegativity the ligand atom possesses, the higher VDE value the MM'X4− anion has.
(4) For the two isomers of LiM'X4−, the VDE values of LiM'X4−-3 are always larger than those of LiM'X4−-2. This is probably due to the fact that the extra electron distribution in two isomers is different. To analyze this clearly, the highest occupied molecular orbitals (HOMOs) of some representative MM'X4− isomers are depicted in Figure 3. As can be seen from the figure, the extra electron is confined to a single Xt atom in LiBeCl4−-2, while localized on the three bridging Xb atoms in LiBeCl4—3, which is a benefit for the extra negative charge dispersion, and thus, LiBeCl4−-3 possesses a larger VDE value. For NaBeX4− anions, the extra electrons of two isomers are all distributed on the terminal X atom. Interestingly, the isomer NaBeX4−-2 in which the extra electron goes on Xt atom has a higher VDE value than isomer NaBeX4—3, which goes on the Xt’ atom (see Figure 3). As to NaMgX4− and NaCaX4− species, the situation is similar to that of the LiM'X4− anions. However, unlike LiM'X4−-3 and NaMgX4−-3, the extra electron is shared by all X ligand atoms instead of three Xb ligands in NaCaX4−-3, which leads to the extra negative charge being more evenly distributed (see Figure 3), and, hence, a relatively larger VDE values for these isomers. This may also explain why NaCaBr4−-3 exhibits larger VDE values than LiCaBr4−-3. Therefore, the extra electron distribution is an important factor affecting the VDE values of the hetero-binuclear superhalogen anions.
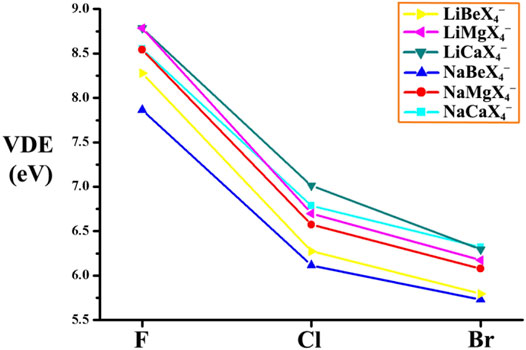
FIGURE 2. The evolutions of VDE values of the MM′X4− and M′X3− (M = Li, Na; M′ = Be, Mg, Ca, X = F ((from ref. [4]), Cl, Br) anions.
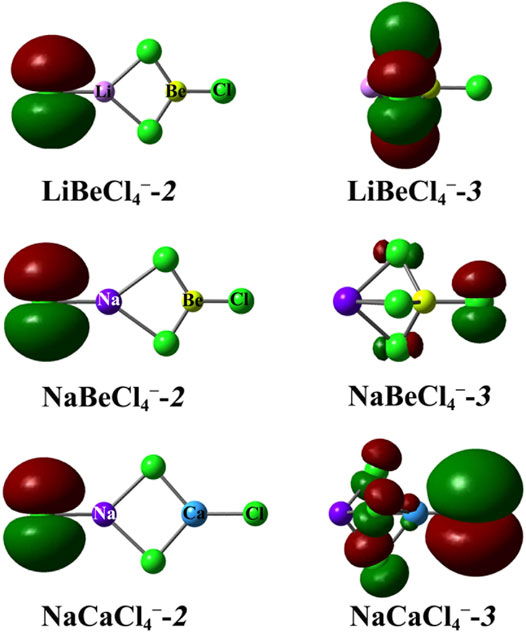
FIGURE 3. HOMO orbitals of superhalogen anions LiBeCl4−, NaBeCl4−, and NaCaCl4−.Color legend: green for Cl atom, light purple for Li atom, dark purple for Na atom, and blue for Ca atom.
Conclusion
Our systematic investigation of the MM′X4− (M = Li, Na; M′ = Be, Mg, Ca; X = Cl, Br) species has theoretically proposed a series of hetero-binuclear superhalogen anions. The results show that these heteronuclear superhalogen anions could gain larger VDE values by involving a smaller alkali metal atom M, a larger alkaline earth metal atom M′, and a higher electronegative ligand atom X. Thereby, of all the anions studied, an isomer of LiCaCl4− anions possesses the largest VDE value (6.799 eV). Moreover, the extra electron distribution is a very influential factor in the VDE values of structural isomers. For the NaBeX4− anions, the isomers have larger VDE values when the extra electron is distributed on the terminal Xt ligand atom instead of the Xt’ ligand atom. For the other anions, the isomers possess larger VDE values when the extra electron is shared by all ligand atoms or three bridging ligand atoms.
Data Availability Statement
The original contributions presented in the study are included in the article/supplementary material; further inquiries can be directed to the corresponding authors.
Author Contributions
All authors listed have made a substantial, direct, and intellectual contribution to the work and approved it for publication.
Funding
This work was supported by the Scientific and Technological Innovation Programs of Higher Education Institutions in Shanxi (2021L370, 2020L0507, and 2020L0516), the National Natural Science Foundation of China (Grant Nos. 21573089 and 51872057), the Doctoral Scientific Foundation Research Foundation of Shanxi Datong University (Grant Nos. 2019-B-11 and 2017-B-04), and the Basic Research Project Fund of Shanxi Province (20210302124491 and 20210302123341).
Conflict of Interest
The authors declare that the research was conducted in the absence of any commercial or financial relationships that could be construed as a potential conflict of interest.
Publisher’s Note
All claims expressed in this article are solely those of the authors and do not necessarily represent those of their affiliated organizations, or those of the publisher, the editors, and the reviewers. Any product that may be evaluated in this article, or claim that may be made by its manufacturer, is not guaranteed or endorsed by the publisher.
References
Anusiewicz, I., and Skurski, P. (2007). Unusual Structures of Mg2F5- Superhalogen Anion. Chem. Phys. Lett. 440, 41–44. doi:10.1016/j.cplett.2007.04.016
Anusiewicz, I., Sobczyk, M., Dąbkowska, I., and Skurski, P. (2003). An Ab Initio Study on MgX3− and CaX3− Superhalogen Anions (X=F, Cl, Br). Chem. Phys. 291, 171–180. doi:10.1016/s0301-0104(03)00208-8
Anusiewicz, I. (2008). Mg2Cl5 - and Mg3Cl7 - Superhalogen Anions. Aust. J. Chem. 61, 712–717. doi:10.1071/ch08212
Anusiewicz, I. (2009a). Electrophilic Substituents as Ligands in Superhalogen Anions. J. Phys. Chem. A 113, 6511–6516. doi:10.1021/jp901910q
Anusiewicz, I. (2009b). Superhalogen Anions Utilizing Acidic Functional Groups as Ligands. J. Phys. Chem. A 113, 11429–11434. doi:10.1021/jp907246w
Arnold, S. T., Miller, T. M., and Viggiano, A. A. (2002). A Theoretical Study of High Electron Affinity Sulfur Oxyfluorides: SO3F, SO2F3, and SOF5. Int. J. Mass Spectrom. 218, 207–215. doi:10.1016/s1387-3806(02)00713-3
Bartlett, N., and Lohmann, D. H. (1962). Fluorides of the Noble Metals. Part II. Dioxygenyl Hexafluoroplatinate(V), O2 +[PtF6]? J. Chem. Soc. 0, 5253–5261. doi:10.1039/jr9620005253
Cederbaum, L. S. (1975). One-body Green's Function for Atoms and Molecules: Theory and Application. J. Phys. B 8, 290–303. doi:10.1088/0022-3700/8/2/018
Chang, X.-T., Li, Y., Liu, J.-Y., Ma, H.-D., and Wu, D. (2019). Noble Gas Insertion Compounds of Hydrogenated and Lithiated Hyperhalogens. Phys. Chem. Chem. Phys. 21, 20156–20165. doi:10.1039/c9cp01284b
Czapla, M. (2017). Dinuclear Superhalogen Anions Containing Two Different Central Atoms. J. Fluor. Chem. 199, 97–102. doi:10.1016/j.jfluchem.2017.05.003
Dennington, R., Keith, T., and Millam, J. (2016). GaussView. version 6. Shawnee Mission, KS: Semichem Inc.
Dong, X.-X., Zhao, Y., Li, J., Wang, H., Bu, Y., and Cheng, S.-B. (2022). Dual External Field-Engineered Hyperhalogen. J. Phys. Chem. Lett. 13, 3942–3948. doi:10.1021/acs.jpclett.2c00916
Elliott, B. M., Koyle, E., Boldyrev, A. I., Wang, X.-B., and Wang, L.-S. (2005). MX3- Superhalogens (M = Be, Mg, Ca; X = Cl, Br): A Photoelectron Spectroscopic and Ab Initio Theoretical Study. J. Phys. Chem. A 109, 11560–11567. doi:10.1021/jp054036v
Feng, Y., Xu, H.-G., Zheng, W., Zhao, H., Kandalam, A. K., and Jena, P. (2011). Structures and Photoelectron Spectroscopy of Cun(BO2)m− (N, M = 1, 2) Clusters: Observation of Hyperhalogen Behavior. J. Chem. Phys. 134, 094309. doi:10.1063/1.3556818
Freza, S., and Skurski, P. (2010). Enormously Large (Approaching 14 eV!) Electron Binding Energies of [HnFn+1]- (N= 1-5, 7, 9, 12) Anions. Chem. Phys. Lett. 487, 19–23. doi:10.1016/j.cplett.2010.01.022
Frisch, M. J., Trucks, G. W., Schlegel, H. B., Scuseria, G. E., Robb, M. A., Cheeseman, J. R., et al. (2016). GAUSSIAN 16. Wallingford CT: Gaussian, Inc.
Giri, S., Behera, S., and Jena, P. (2014a). Superalkalis and Superhalogens as Building Blocks of Supersalts. J. Phys. Chem. A 118, 638–645. doi:10.1021/jp4115095
Giri, S., Behera, S., and Jena, P. (2014b). Superhalogens as Building Blocks of Halogen-free Electrolytes in Lithium-Ion Batteries. Angew. Chem. Int. Ed. 53, 13916–13919. doi:10.1002/anie.201408648
Gutsev, G. L., and Boldyrev, A. I. (1981). DVM-xα Calculations on the Ionization Potentials of MXk+1− Complex Anions and the Electron Affinities of MXk+1 "superhalogens". Chem. Phys. 56, 277–283. doi:10.1016/0301-0104(81)80150-4
Gutsev, G. L., Rao, B. K., Jena, P., Wang, X.-B., and Wang, L.-S. (1999). Origin of the Unusual Stability of MnO4−. Chem. Phys. Lett. 312, 598–605. doi:10.1016/s0009-2614(99)00976-8
Gutsev, G. L., Jena, P., Zhai, H.-J., and Wang, L.-S. (2001). Electronic Structure of Chromium Oxides, CrOn− and CrOn (N=1-5) from Photoelectron Spectroscopy and Density Functional Theory Calculations. J. Chem. Phys. 115, 7935–7944. doi:10.1063/1.1405438
Gutsev, G. L., Weatherford, C. A., Johnson, L. E., and Jena, P. (2012). Structure and Properties of the Aluminum Borates Al(BO2)n and Al(BO2)n−, (N = 1-4). J. Comput. Chem. 33, 416–424. doi:10.1002/jcc.21984
Kim, H., Lim, J., Sohail, M., and Nazeeruddin, M. K. (2022). Superhalogen Passivation for Efficient and Stable Perovskite Solar Cells. Sol. RRL, 2200013. doi:10.1002/solr.202200013
Koirala, P., Willis, M., Kiran, B., Kandalam, A. K., and Jena, P. (2010). Superhalogen Properties of Fluorinated Coinage Metal Clusters. J. Phys. Chem. C 114, 16018–16024. doi:10.1021/jp101807s
Li, J., Huang, H.-C., Wang, J., Zhao, Y., Chen, J., Bu, Y.-X., et al. (2019). Polymeric Tungsten Carbide Nanoclusters: Structural Evolution, Ligand Modulation, and Assembled Nanomaterials. Nanoscale 11, 19903–19911. doi:10.1039/c9nr05613k
Li, J., Zhao, Y., Bu, Y.-F., Chen, J., Wei, Q., and Cheng, S.-B. (2020). On the Theoretical Construction of Nb2N2-Based Superatoms by External Field Strategies. Chem. Phys. Lett. 754, 137709. doi:10.1016/j.cplett.2020.137709
Lu, S.-J., Wu, L.-S., and Lin, F. (2019). Structural, Bonding, and Superhalogen Properties of Au4X 4 −/0 (X = F, Cl, Br, and I) Clusters. Theor. Chem. Acc. 138, 51. doi:10.1007/s00214-019-2442-1
Marchaj, M., Freza, S., Rybacka, O., and Skurski, P. (2013). Superhalogen Oxidizers Capable of Ionizing Water Molecules. Chem. Phys. Lett. 574, 13–17. doi:10.1016/j.cplett.2013.05.009
Møller, C., and Plesset, M. S. (1934). Note on an Approximation Treatment for Many-Electron Systems. Phys. Rev. 46, 618. doi:10.1103/PhysRev.46.618
Paduani, C., Wu, M. M., Willis, M., and Jena, P. (2011). Theoretical Study of the Stability and Electronic Structure of Al(BH4)n=1→4 and Al(BF4)n=1→4 and Their Hyperhalogen Behavior. J. Phys. Chem. A 115, 10237–10243. doi:10.1021/jp206330d
Pathak, B., Samanta, D., Ahuja, R., and Jena, P. (2011). Borane Derivatives: A New Class of Super- and Hyperhalogens. ChemPhysChem 12, 2423–2428. doi:10.1002/cphc.201100320
Reed, A. E., Weinstock, R. B., and Weinhold, F. (1985). Natural Population Analysis. J. Chem. Phys. 83, 735–746. doi:10.1063/1.449486
Saha, R., Mandal, B., and Chattaraj, P. K. (2018). HNgBeF3 (Ng = Ar-Rn): Superhalogen-Supported Noble Gas Insertion Compounds. Int. J. Quantum Chem. 118, e25499. doi:10.1002/qua.25499
Sikorska, C., Freza, S., Skurski, P., and Anusiewicz, I. (2011). Theoretical Search for Alternative Nine-Electron Ligands Suitable for Superhalogen Anions. J. Phys. Chem. A 115, 2077–2085. doi:10.1021/jp2000392
Smuczynska, S., and Skurski, P. (2009). Halogenoids as Ligands in Superhalogen Anions. Inorg. Chem. 48, 10231–10238. doi:10.1021/ic901253r
Srivastava, A. K., Kumar, A., and Misra, N. (2021). Superhalogens as Building Blocks of Ionic Liquids. J. Phys. Chem. A 125, 2146–2153. doi:10.1021/acs.jpca.1c00599
Srivastava, A. K. (2021). Prediction of Novel Liquid Crystalline Molecule Based on BO2 Superhalogen. J. Mol. Liq. 344, 117968. doi:10.1016/j.molliq.2021.117968
Sun, W.-M., Hou, D., Wu, D., Li, X.-H., Li, Y., Chen, J.-H., et al. (2015). Theoretical Characterization of a Series of N5-Based Aromatic Hyperhalogen Anions. Dalton Trans. 44, 19901–19908. doi:10.1039/c5dt03575a
Sun, W.-M., Li, X.-H., Li, Y., Wu, D., Li, C.-Y., Chen, J.-H., et al. (2016). Can Fluorinated Molecular Cages Be Utilized as Building Blocks of Hyperhalogens? ChemPhysChem 17, 1468–1474. doi:10.1002/cphc.201600052
Świerszcz, I., and Anusiewicz, I. (2011). Neutral and Anionic Superhalogen Hydroxides. Chem. Phys. 383, 93–100. doi:10.1016/j.chemphys.2011.04.018
Wang, X.-B., Ding, C.-F., Wang, L.-S., Boldyrev, A. I., and Simons, J. (1999). First Experimental Photoelectron Spectra of Superhalogens and Their Theoretical Interpretations. J. Chem. Phys. 110, 4763–4771. doi:10.1063/1.478386
Willis, M., Götz, M., Kandalam, A. K., Ganteför, G. F., and Jena, P. (2010). Hyperhalogens: Discovery of a New Class of Highly Electronegative Species. Angew. Chem. Int. Ed. 49, 8966–8970. doi:10.1002/anie.201002212
Yang, X., Wang, X.-B., Wang, L.-S., Niu, S., and Ichiye, T. (2003). On the Electronic Structures of Gaseous Transition Metal Halide Complexes, FeX4− and MX3− (M=Mn, Fe, Co, Ni, X=Cl, Br), Using Photoelectron Spectroscopy and Density Functional Calculations. J. Chem. Phys. 119, 8311–8320. doi:10.1063/1.1610431
Yang, H., Li, Y., He, H.-M., Tong, J., Wu, D., and Li, Z.-R. (2017). Superhalogen Properties of Hetero-Binuclear Anions MM′F4− and MM″F5− (M = Li, Na, M′ = Be, Mg, Ca; M″ = B, Al, Ga). Chem. Phys. Lett. 684, 273–278. doi:10.1016/j.cplett.2017.07.010
Yang, H., Li, Y., He, H.-M., Yu, D., Wu, D., and Li, Z.-R. (2018). Hetero-binuclear Superhalogen Anions with Cyanide And/or Isocyanide as Ligands. Chem. Phys. Lett. 713, 203–209. doi:10.1016/j.cplett.2018.10.039
Yang, H., Li, Y., Zhao, J.-G., Xing, B.-Y., He, H.-M., Jiang, S., et al. (2021). On Structure and Hyperhalogen Properties of Hetero-Binuclear Superatoms MM′(BO2)− (M = Na, Mg; M′ = Mg, Al; N = 4-6). Polyhedron 209, 115456. doi:10.1016/j.poly.2021.115456
Zakrzewski, V. G., Dolgounitcheva, O., and Ortiz, J. V. (1996). Ionization Energies of Anthracene, Phenanthrene, and Naphthacene. J. Chem. Phys. 105, 8748–8753. doi:10.1063/1.472654
Zhai, H.-J., Li, J., and Wang, L.-S. (2004). Icosahedral Gold Cage Clusters: M@Au12−(M = V, Nb, and Ta). J. Chem. Phys. 121, 8369–8374. doi:10.1063/1.1799574
Zhai, H.-J., Wang, L.-M., Li, S.-D., and Wang, L.-S. (2007). Vibrationally Resolved Photoelectron Spectroscopy of BO- and BO2-: A Joint Experimental and Theoretical Study. J. Phys. Chem. A 111, 1030–1035. doi:10.1021/jp0666939
Keywords: superhalogen, chloride or bromine ligands, binuclear, vertical electron detachment energy, theoretical calculation
Citation: Yang H, He H-M, Li N, Jiang S, Pang M-J, Li Y and Zhao J-G (2022) Design of a Novel Series of Hetero-Binuclear Superhalogen Anions MM′X4− (M = Li, Na; M′ = Be, Mg, Ca; X = Cl, Br). Front. Chem. 10:936936. doi: 10.3389/fchem.2022.936936
Received: 05 May 2022; Accepted: 07 June 2022;
Published: 01 July 2022.
Edited by:
Iwona Anusiewicz, University of Gdansk, PolandReviewed by:
Jakub Brzeski, University of Pittsburgh, United StatesShi-Bo Cheng, Shandong University, China
Copyright © 2022 Yang, He, Li, Jiang, Pang, Li and Zhao. This is an open-access article distributed under the terms of the Creative Commons Attribution License (CC BY). The use, distribution or reproduction in other forums is permitted, provided the original author(s) and the copyright owner(s) are credited and that the original publication in this journal is cited, in accordance with accepted academic practice. No use, distribution or reproduction is permitted which does not comply with these terms.
*Correspondence: Ning Li, bGluMjI0QG5lbnUuZWR1LmNu; Jian-Guo Zhao, amd6aGFvc2hpQDE2My5jb20=