- 1Key Laboratory of Child Cognition & Behavior Development of Hainan Province, Qiongtai Normal University, Haikou, China
- 2School of Science, Qiongtai Normal University, Haikou, China
- 3Shandong Institute for Food and Drug Control, Ji’nan, China
- 4Department of Medicinal Chemistry and Natural Medicine Chemistry, College of Pharmacy, Harbin Medical University, Harbin, China
The secondary C(OH)-C bonds are abundant in biomass such as lignin and cellulose. Thus, selective cleavage of the C(OH)-C bonds into value chemicals attracted much attention. Molecular iodine has received considerable attention as an inexpensive and readily available catalyst to yield the corresponding products in excellent yields with high selectivity, but it is highly corrosive and toxic, making its use somewhat unattractive. In this study, I2 was generated in situ from Fe(NO3)3.9H2O/NaI, which was further combined with Fe(NO3)3.9H2O to catalyze the oxidation process. In the reaction, the H2O molecule from the reaction and Fe(NO3)3.9H2O attacked the phenylglyoxal to form benzaldehyde, which was further oxidized to benzoic acid. Aryl primary and secondary benzylic alcohols from lignin were successfully transformed into aryl carboxylic acids by Fe(NO3)3.9H2O/NaI/DMSO. The catalytic system was green and efficient, avoiding the usage of toxic and corrosive molecular I2. From the experiments, it was clear that the yield of the product from the substrates with an electron-donating group was higher than that of electron-withdrawing substituted substrates, which was similar to the aryl secondary alcohols. Aryl alkyl ketones were also successfully conducted by the Fe(NO3)3.9H2O/NaI/DMSO catalytic system.
Introduction
Lignin is the largest renewable source with a large number of aromatic units; thus, it could serve as a sustainable candidate feedstock for aromatic chemicals (Ohlrogge et al., 2009). Inspired by this, there are more efforts to selectively transform lignin into value-added chemicals, especially aromatic chemicals. In natural lignin, C(OH)-C bonds are widespread. Thus, it is an attractive strategy for selective cleavage and functionalization of C(OH)-C bonds for converting lignin into value-added chemical products, such as aryl carboxylic acid. Aryl carboxylic acid is very common in many structures of bioactive molecules and can be transformed to esters, amides, acid halides, and so on (Peng et al., 2016; Nicolaou et al., 2009; Liu et al., 2020). Although the C(OH)–C bonds in lignin have inherent kinetic inertness and thermodynamic stability, it is still very challenging for selective functionalization of these bonds.
In recent years, because molecular iodine possesses some advantages such as low in cost and easy to obtain, it has attracted considerable attention. More importantly, it is very efficient to make use as a powerful catalyst for various organic transformations with excellent yields and high selectivity under mild conditions, owing to its oxidation ability and Lewis acidity (Iida and Togo, 2006; Hazra et al., 2017). For example, Hazra’s group has reported a metal-free catalytic system with iodine/NaOH, which could transform alcohols and aldehydes into carboxylic acids with excellent yield (Hazra et al., 2017). It is clear that I2 is very useful in various types of organic reactions, but molecular iodine is highly corrosive and toxic, resulting in somewhat undesirable results in many reactions. Researchers conducted some excellent works to overcome the direct usage of I2. Bailey et al. have reported that CuSO4/NaI used in combination could be an in situ generation of I2 (Mohan et al., 2006). Subsequently, another convenient system to generate I2 in situ was reported by Tamin’s group, which consisted of Fe(NO3)3.9H2O/NaI, and the amount of I2 generated in situ was measured by UV/Vis spectrophotometry (Rostami et al., 2009). Also, this group has applied this protocol for trimethylsilylation and formylation of various alcohols catalyzed by I2 generated in situ from Fe(NO3)3.9H2O/NaI (Amin et al., 2011).
Moreover, iron is low in cost and toxicity and has rapidly developed in various fields (Martin and Suarez, 2002; Plietker and Dieskau, 2009; Czaplik et al., 2010). Compared with other transition metals, most of the iron species show low toxicity (Perutz, 1979). The oral permitted daily exposure limit of elemental iron is high at 13,000 Ug/day; thus, the application of elemental iron in the pharmaceutical and food industries is attractive (Bauer and Knölker, 2015). In recent years, iron-catalyzed oxidation reactions to pharmaceutical molecules have attracted significant attention. Also, Li’ group reported a procedure of aerobic oxidative deoximation reactions that were catalyzed by iron (Li et al., 2018). In 2013, Ma’s group have reported Fe(NO3)3.9H2O/TEMPO/MCl (M = Na or K) oxidized corresponding alcohols to carbonyl compounds using O2 or air as a terminal oxidant at room temperature (Jiang et al., 2016; Liu and Ma, 2013a; Liu and Ma, 2013b). Also, Xu and co-workers reported a strategy of I2/Fe(NO3)3.9H2O-catalyzed C–C bond cleavage of aryl alkyl ketones and secondary benzylic alcohols (Xu et al., 2018a). Notably, all these related research studies have confirmed that iron salts are efficient in catalytic oxidation reactions, but all the catalytic systems need other types of co-oxidants to promote catalytic activity.
Inspired by those finding, we wish to report a facile and efficient approach for the oxidation of secondary C(OH)-C bonds in lignin to aryl carboxylic acid by using cheap and nontoxic Fe(NO3)3 and NaI as a catalyst, in which I2 was generated in situ under the reaction, avoiding the usage of toxic and corrosive molecular I2. A variety of secondary and primary benzylic alcohols could be transformed into corresponding acids in moderate-to-excellent yields.
Experimental Section
Materials
All of the materials were purchased from Beijing Innochem Company and used as received.
Characterization
Other than the 9H-fluoren-9-ol, mass spectra were obtained on an SCIEX X500R QTOF high-resolution mass spectrometry instrument with negative ion mode. The 0.1 acetic acid/5 mM amine acetate in CH3CN/H2O (20:80) was used as the eluent.
General Procedures for Aerobic Oxidation
Typical procedure: the desired amount of secondary alcohol substrate (0.5 mmol), Fe(NO3)3.9H2O (0.15 mmol), NaI (0.075 mmol), and DMSO (2 ml) was added into a 25-ml reaction bottle. Then, the mixture was degassed three times with the oxygen balloon, and the reaction was held under 130°C for the desired time. After being acidified with 2 mol/L HCl (3 ml), the solution was extracted by ethyl acetate (5 ml) twice, and the organic phase was washed with saturated brine once and dried by Na2SO4. The combined organic phase was removed from the solvent by a rotary evaporator. The desired product was obtained through column chromatography using ethyl acetate/petroleum ether as an eluent.
Results and Discussion
Initially, 1-phenyl-1-propanol was taken as the model substrate to optimize the reaction conditions (Table 1). We tried to optimize the reaction with a suitable amount and ratio of Fe(NO3)3.9H2O/NaI; when the catalytic system consisted of 20 mol% of Fe(NO3)3.9H2O and 10 mol% of NaI, the desired product could be successfully obtained with 67% yield in 18 h (Table 1, entry 1). When the loading of Fe(NO3)3.9H2O was further increased to 30 mol% and NaI was increased to 15 mol%, the yield was sharply increased to 83% (Table 1, entry 2). But, while maintaining the loading of Fe(NO3)3.9H2O at 30 mol%, the loading of NaI was further increased to 30 mol%, and then the benzoic acid was yielded at 72% (Table 1, entry 3). While other types of nitrate catalysts were used as catalyst, the yield of acid was lower than when the Fe(NO3)3.9H2O was used as the catalyst (Table 1, entries 4–5). We also test other types of salts as the co-catalyst, such as LiI, KI, NaBr, or NaCl, but all those salts cannot offer a satisfactory yield of acid (Table 1, entries 6–10).
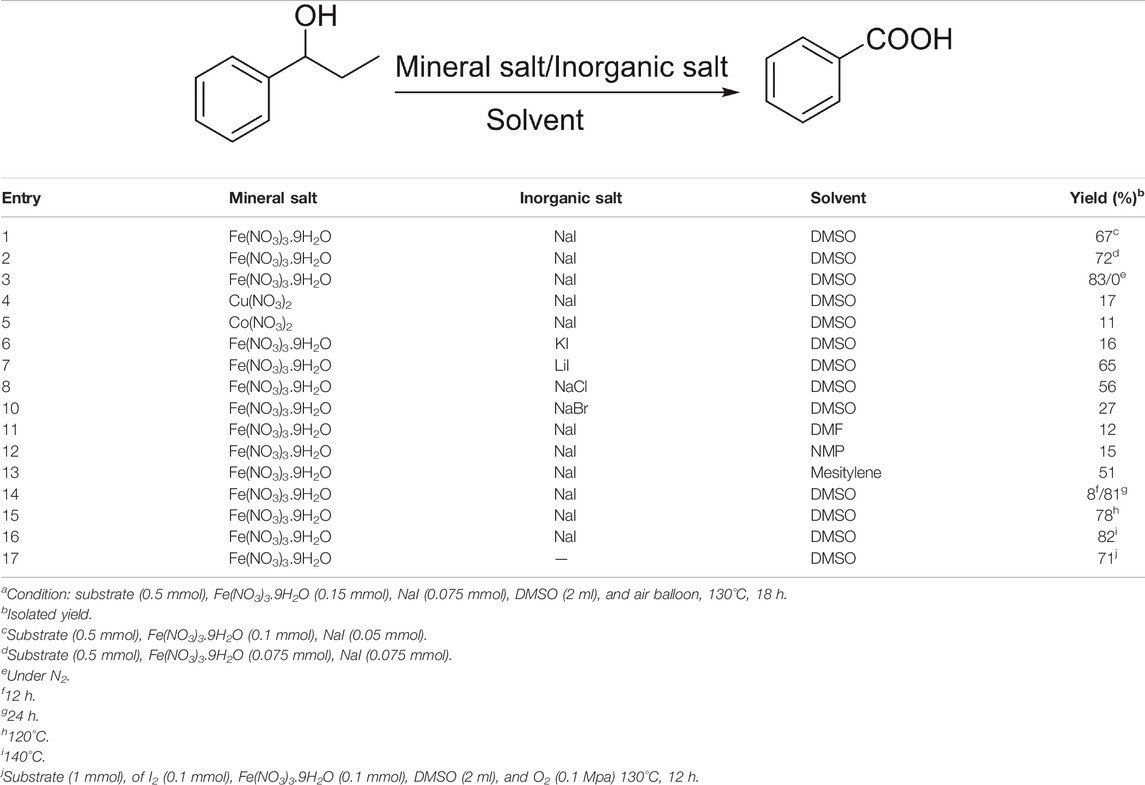
TABLE 1. Optimization of reaction conditionsa.
A series of solvents including DMF, NMP, and mesitylene were screened, and it was clearly shown that DMSO was the best solvent among them (Table 1, entries 11–13). When the reaction was conducted in the absence of oxygen, the yield of benzoic acid was not detected, but benzaldehyde was formed with a 65% yield (Table 1, entry 3). Then, we proceeded with the reaction under different temperatures and times, and it was found that the yield of the product was lower under 120°C (Table 1, entry 15). The yield of the desired product was not improved when the reaction temperature was increased to 140°C or the reaction time was increased to 24 h (Table 1, entries 14 and 16). More interestingly, when the reaction time was decreased to 12 h, the yield of acid was just 8%, and most of them was benzaldehyde, indicating that benzaldehyde was transformed to the desired product faster.
Thus, it was obvious that NaI (15 mol%)/Fe(NO3)3.9H2O (30 mol%) as the catalyst and DMSO as solvent under O2 balloon at 130°C for 18 h could efficiently catalyze aerobic oxidation of alcohol. Subsequently, the standard reaction condition was chosen to explore the scope and generality of this protocol. The various aryl alkyl alcohols were further investigated, with the results shown in Table 2.
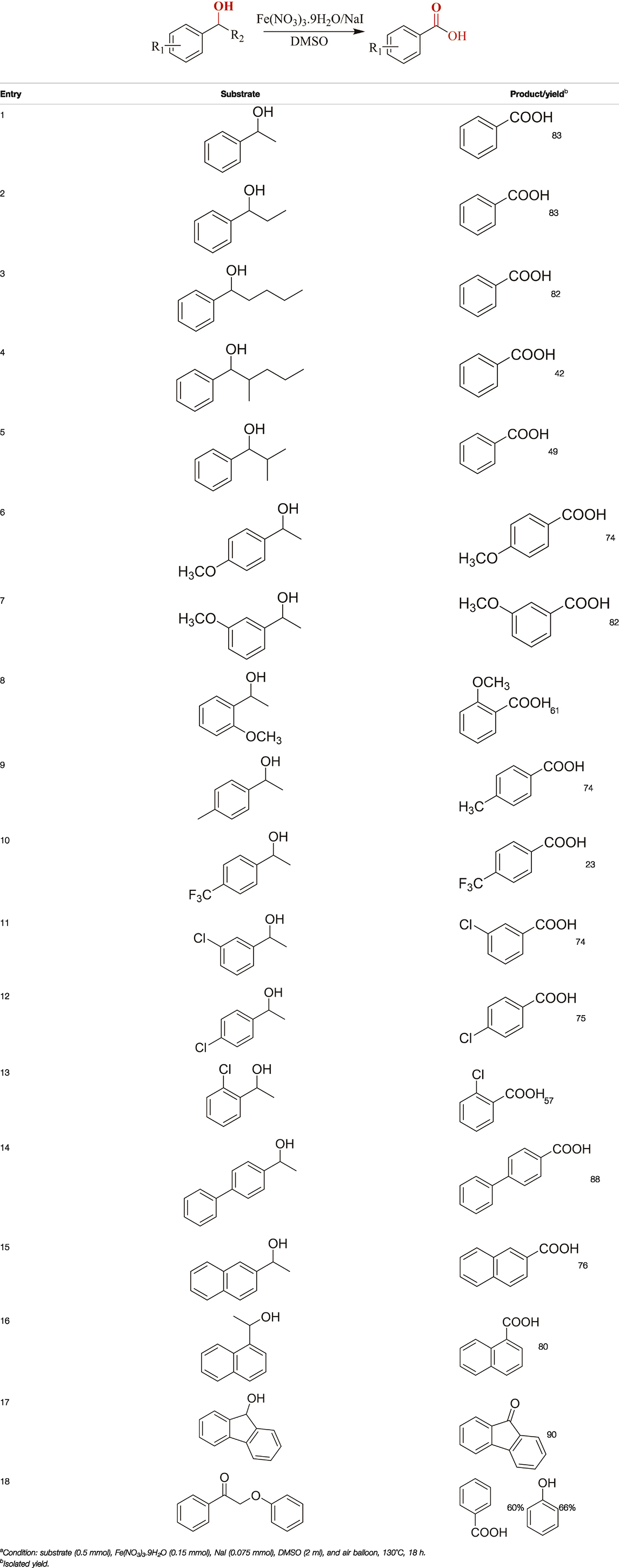
TABLE 2. Catalytic aerobic oxidation of secondary alcoholsa.
The aryl alkyl alcohols with different alkyl substituent groups on its β-position of the methyl group were then used as substrates, and all of the aryl alkyl alcohols could be oxidized to desired aryl carboxylic acids in moderate-to-good yields ranging from 42–83%. (Table 2, entries 1–5). Compared with phenyl-1-pentanol, 2-methyl-1-phenyl- propanol with a steric hindrance group on the β-position was transformed into the desired product in 49% yield (Table 2, entry 5). A similar result was obtained from 1-phenyl-2-methyl-1-propanol (42%), which was also with a steric hindrance group in the same position.
In general, substrates with electron-donating or electron-withdrawing groups could be smoothly converted by this catalytic system, and the desired aryl carboxylic acids were obtained in moderate-to-good yields. 1-phenylethanol bearing electron-donating substituents such as 4-CH3 and 4-OCH3 were transformed to the corresponding benzoic acids in 74 and 74% isolated yields, respectively (Table 2, entries 6 and 9). The 3-OCH3 and 2-OCH3 substituted 1-phenylethanol gave corresponding benzoic acids in 82 and 61% isolated yields, respectively (Table 2, entries 7–8).
It is obvious that the product from the 2-OCH3 substituted substrate is in a lower yield, which might be owing to the ortho-effect. 1-phenylethanol with strong electron-withdrawing groups (4-CF3) could also afford the desired product in a low yield (23%) (Table 2, entry 10). In addition, the aryl alkyl alcohols bearing a p- or m-chloro substituent were also transformed into corresponding benzoic acid in moderate yields under standard conditions (Table 2, entries 11 and 12). However, like o-OCH3 substituted 1-phenylethanol, the substrate bearing a chloro group in the ortho position was with lower reactivity, and the isolated yield of the product was 57% (Table 2, entry 13).
For 1-phenylethanol derivative with a phenyl substitute group on p-position (Table 2, entry 14), their catalytic oxidation of the substrate to the benzoic acid product resulted in excellent yield (88%). Meanwhile, the 1-(1-naphthyl) ethanol and 1-(2-naphthyl) ethanol also bear an aryl ring substitute with yields upto 80 and 76%, respectively (Table 2, entry 15 and 16). But the 9H-fluoren-9-ol, which was a representative diaryl secondary alcohol, could not be converted to the corresponding acid but to the corresponding ketone in a high yield (93%) (Table 2, entry 17). More interestingly, the important fragment of lignin (Table 2, entry 18) could be converted smoothly to the corresponding benzoic acid and phenol in moderate yield.
Then, various benzyl alcohols and aryl alkyl ketones also could be converted into carboxylic acids in moderate-to-good yields (45–84%) (Table 3, entries 1–8). For example, benzyl alcohols bearing electron-donating substituents such as 4-OCH3, 3-OCH3, and naphthyl alcohol substrates were smoothly oxidized to benzoic acid analogs in 69–80% isolated yields. Benzyl alcohols with an electron-withdrawing group (4-CF3) could also afford the desired products in a low yield (46%). From the experiments, it was clear that the benzyl alcohols bearing the electron-donating substituent gave higher yields of products than substituted substrates bearing electron-withdrawing substituent, which was similar to the aryl secondary alcohols. Aryl alkyl ketones were also successfully conducted by the Fe(NO3)3.9H2O/NaI/DMSO catalytic system (Table 3, entries 9–11).
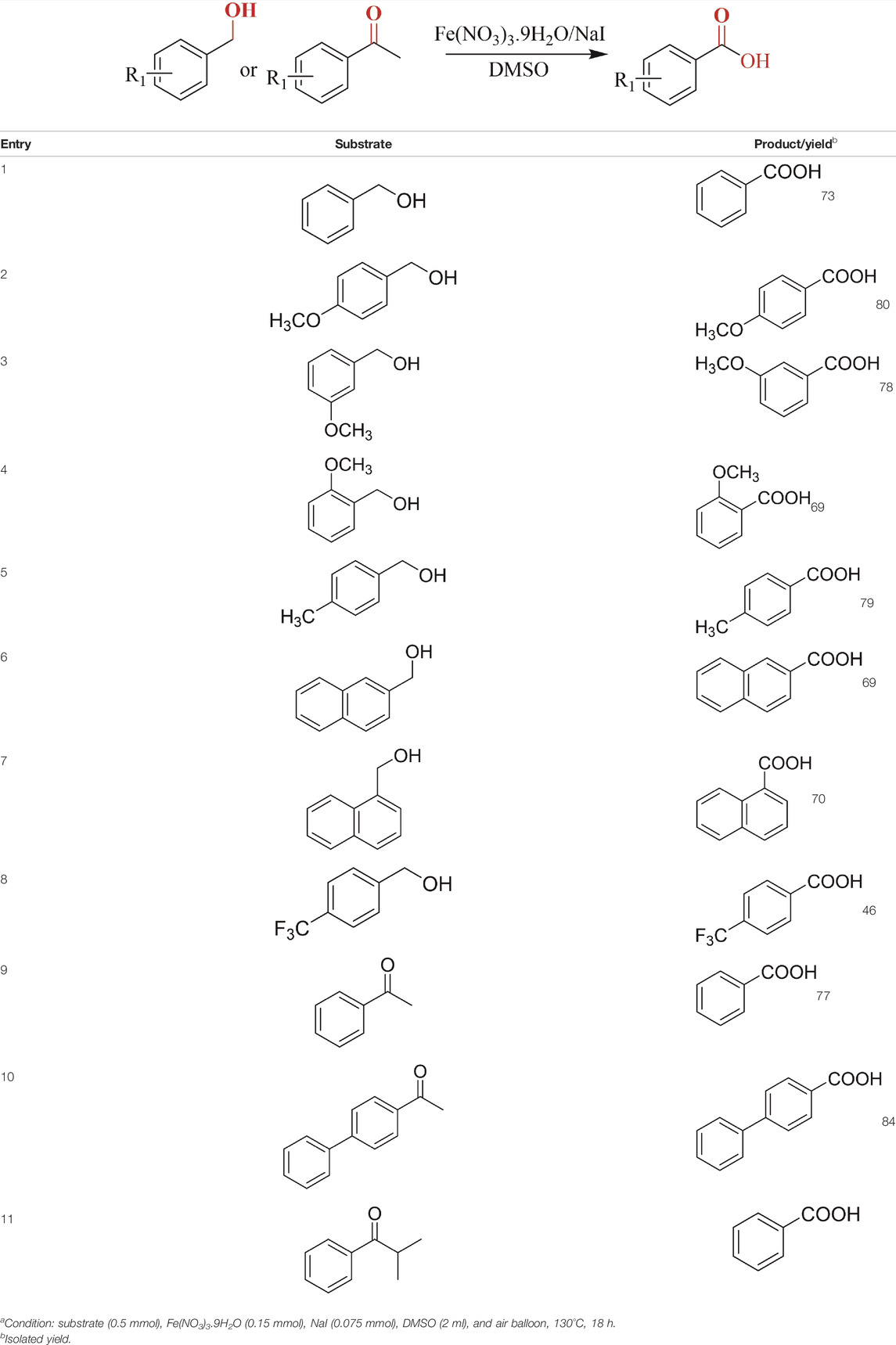
TABLE 3. Catalytic aerobic oxidation of primary alcohols and ketonesa.
Next, to gain insight into the mechanism of this oxygenation reaction, some control experiments were set up. Using phenylglyoxal instead of 1-phenyl-1-ethanol as the reactant, it was shown that the phenylglyoxal could be transformed to benzoic acid in 99% yield under standard conditions. Also, benzaldehyde was subjected as the substrate, and a quantitative yield of 99% was produced by benzoic acid under standard conditions. When the reaction was conducted in the absence of NaI, the yield of benzoic acid was low to 56%, while no product was observed without Fe(NO3)3.9H2O. Also, while the reaction was carried out without oxygen, no benzoic acid was obtained, along with a 58% yield of benzaldehyde. From the previous results and the references (Xu et al., 2018a), we proposed a reaction mechanism as follows. Under the reaction condition, the 1-phenylethanol was first oxidized into acetophenone. Initially, I2 was generated in situ from Fe(NO3)3.9H2O/NaI, and then acetophenone was transformed into a-iodoketone with the help of I2 (Elmasry et al., 1998; Naimi-Jamal et al., 2009; Dressen et al., 2009; Martin et al., 2008). Then, a-iodoketone was oxidized into phenylglyoxal by DMSO and releases HI (Wu et al., 2016; Xiang et al., 2016; Wu and Natte, 2016; Xu et al., 2018b). With the Fe salt and H2O molecule, the C–C bond was cleaved to give benzaldehyde and release a molecule of formic acid. Benzaldehyde can be oxidized into benzoic acid by the Fe(NO3)3.9H2O/NaI/DMSO catalytic system (see Supplementary Material).
Conclusion
In summary, the Fe(NO3)3.9H2O/NaI/DMSO catalytic system successfully transformed the aryl alcohols into the desired benzoic acids. In this catalyst system, I2 was generated in situ from Fe(NO3)3.9H2O/NaI, which was further combined with Fe(NO3)3.9H2O to catalyze the oxidation process. Compared with the Fe(NO3)3.9H2O/I2 catalytic system, Fe(NO3)3.9H2O/NaI/DMSO was found with a similar catalytic activity, avoiding direct usage of toxic and corrosive molecular I2. Aryl primary and secondary alcohols bearing an electron-donating substituent or electron-withdrawing substituent could be concerted to the corresponding benzoic acid in moderate-to-high yields under standard conditions. Also, the represented lignin model compounds were all successfully transformed into the desired products. Fortunately, it was obvious that the catalytic system had great potential application in terms of green process and technological innovation for the transformation of lignin.
Data Availability Statement
The original contributions presented in the study are included in the article/Supplementary Material; further inquiries can be directed to the corresponding authors.
Author Contributions
XW, HS, CL, YG, and YC: experimental operation and data collection; SN: the LC-MS data collection; JW: the conception and design of the study; JW and HX: article writing and revision; and TX: experimental data collection and analysis. All authors read and approved the submitted version.
Funding
This work was financially supported by the Hainan Provincial Natural Science Foundation of China (2019RC250 and 220QN281), National Science and Technology Major Project of the Ministry of Science and Technology of China (2018ZX09735005), National Natural Science Foundation of China (22165009), the Scientific Research Foundation of the Higher Education Institutions of Hainan Province (Hnky2021ZD-22), the Scientific Research Project of Qiongtai Normal University (qtyb201908), and the National Undergraduate Training Program for Innovation and Entrepreneurship (S202113811005, 202113811022).
Conflict of Interest
The authors declare that the research was conducted in the absence of any commercial or financial relationships that could be construed as a potential conflict of interest.
Publisher’s Note
All claims expressed in this article are solely those of the authors and do not necessarily represent those of their affiliated organizations, or those of the publisher, the editors, and the reviewers. Any product that may be evaluated in this article, or claim that may be made by its manufacturer, is not guaranteed or endorsed by the publisher.
Supplementary Material
The Supplementary Material for this article can be found online at: https://www.frontiersin.org/articles/10.3389/fchem.2022.933763/full#supplementary-material
References
Amin, R., Ardeshir, K., Heidar Ali, A.-N., and Zahra, T.-R. (2011). Formylation of Alcohol with Formic Acid under Solvent-free and Neutral Conditions Catalyzed by Free I2 or I2 Generated In Situ from Fe(NO3)3·9H2O/NaI. Chin. J. Catal. 32, 60–64. doi:10.1016/s1872-2067(10)60160-x
Bauer, I., and Knölker, H.-J. (2015). Iron Catalysis in Organic Synthesis. Chem. Rev. 115, 3170–3387. doi:10.1021/cr500425u
Czaplik, W. M., Mayer, M., Jacobi von Wangelin, A., and Wangelin, J. V. (2010). Iron-Catalyzed Reductive Aryl–Alkenyl Cross-Coupling Reactions. ChemCatChem 3, 135–138. doi:10.1002/cctc.201000276
Dressen, M. H. C. L., Stumpel, J. E., Van de Kruijs, B. H. P., Meuldijk, J., Vekemans, J. A. J. M., and Hulshof, L. A. (2009). The Mechanism of the Oxidation of Benzyl Alcohol by iron(III)nitrate: Conventional versus Microwave Heating. Green Chem. 11, 60–64. doi:10.1039/B813030B
Elmasry, M. A. A., Gaber, A., and Khater, E. M. H. (1998). Thermal Decomposition of Ni(II) and Fe(III) Nitrates and Their Mixture. J. Therm. Anal. Calorim. 52, 489–495. doi:10.1023/a:1010155203247
Hazra, S., Deb, M., and Elias, A. J. (2017). Iodine Catalyzed Oxidation of Alcohols and Aldehydes to Carboxylic Acids in Water: a Metal-free Route to the Synthesis of Furandicarboxylic Acid and Terephthalic Acid. Green Chem. 19, 5548–5552. doi:10.1039/C7GC02802D
Iida, S., and Togo, H. (2006). Direct and Facile Oxidative Conversion of Primary, Secondary, and Tertiary Amines to Their Corresponding Nitriles. Synlett 16, 2633–2635. doi:10.1055/s-2006-951491
Jiang, X., Zhang, J., and Ma, S. (2016). Iron Catalysis for Room-Temperature Aerobic Oxidation of Alcohols to Carboxylic Acids. J. Am. Chem. Soc. 138, 8344–8347. doi:10.1021/jacs.6b03948
Li, Y., Xu, N., Mei, G., Yun, Z., Zhao, Y., Lyu, J., et al. (2018). Fe(NO3)3·9H2O-catalyzed Aerobic Oxidative Deoximation of Ketoximes and Aldoximes under Mild Conditions. Can. J. Chem. 96, 810–814. doi:10.1139/cjc-2017-0567
Liu, J., and Ma, S. (2013b). Room Temperature Fe(NO3)3·9H2O/TEMPO/NaCl-catalyzed Aerobic Oxidation of Homopropargylic Alcohols. Tetrahedron 2013 (69), 10161–10167. doi:10.1016/j.tet.2013.08.082
Liu, J., and Ma, S. (2013a). Aerobic Oxidation of Indole Carbinols Using Fe(NO3)3·9H2O/TEMPO/NaCl as Catalysts. Org. Biomol. Chem. 11, 4186–4193. doi:10.1039/C3OB40226F
Liu, M., Zhang, Z., Yan, J., Liu, S., Liu, H., Liu, Z., et al. (2020). Aerobic Oxidative Cleavage and Esterification of C(OH)-C Bonds. Chem 6, 3288–3296. doi:10.1016/j.chempr.2020.09.006
Martin, S. E., and Suarez, D. (2002). Catalytic Aerobic Oxidation of Alcohols by Fe(NO3)3–FeBr3. Tetrahedron Lett. 43, 4475–4479. doi:10.1016/S0040-4039(02)00829-8
Mohan, R. S., Bailey, A. D., Cherney, S. M., Anzalone, P. W., Anderson, E. D., and Ernat, J. J. (2006). A Convenient Method for In Situ Generation of I2 Using CuSO4/NaI and its Applications to the Deprotection of Acetals, Etherifications and Iodolactonizations. Synlett 2, 215–218. doi:10.1055/s-2005-923586
Naimi-Jamal, M. R., Hamzeali, H., Mokhtari, J., Boy, J., and Kaupp, G. (2009). Sustainable Synthesis of Aldehydes, Ketones or Acids from Neat Alcohols Using Nitrogen Dioxide Gas, and Related Reactions. ChemSusChem 2, 83–88. doi:10.1002/cssc.200800193
Nicolaou, K. C., Chen, J. S., Edmonds, D. J., and Estrada, A. A. (2009). Recent Advances in the Chemistry and Biology of Naturally Occurring Antibiotics. Angew. Chem. Int. Ed. 48, 660–719. doi:10.1002/anie.200801695
Ohlrogge, J., Allen, D., Berguson, B., DellaPenna, D., Shachar-Hill, Y., and Stymne, S. (2009). Driving on Biomass. Science 324, 1019–1020. doi:10.1126/science.1171740
Peng, J.-B., Qi, X., and Wu, X.-F. (2016). Visible Light-Induced Carbonylation Reactions with Organic Dyes as the Photosensitizers. ChemSusChem 9, 2279–2283. doi:10.1002/cssc.201600625
Perutz, M. F. (1979). Regulation of Oxygen Affinity of Hemoglobin: Influence of Structure of the Globin on the Heme Iron. Annu. Rev. Biochem. 48, 327–386. doi:10.1146/annurev.bi.48.070179.001551
Plietker, B., and Dieskau, A. (2009). The Reincarnation of the Hieber Anion [Fe(CO) 3 (NO)] - - a New Venue in Nucleophilic Metal Catalysis. Eur. J. Org. Chem. 2009, 775–787. doi:10.1002/ejoc.200800893
Rostami, A., Rahmati, S., and Khazaei, A. (2009). A Highly Efficient and Ecofriendly Procedure for Tetrahydropyranylation of Alcohols and Phenols in the Presence of In-Situ Generated I2 under Heterogeneous and Neutral Conditions. Monatsh Chem. 140, 663–667. doi:10.1007/s00706-009-0117-7
Wu, X.-F., and Natte, K. (2016). The Applications of Dimethyl Sulfoxide as Reagent in Organic Synthesis. Adv. Synth. Catal. 358, 336–352. doi:10.1002/adsc.201501007
Wu, X., Gao, Q., Geng, X., Zhang, J., Wu, Y.-d., and Wu, A.-x. (2016). Iodine-Promoted Oxidative Cross-Coupling of Unprotected Anilines with Methyl Ketones: A Site-Selective Direct C-H Bond Functionalization to C4-Dicarbonylation of Anilines. Org. Lett. 18, 2507–2510. doi:10.1021/acs.orglett.6b01162
Xiang, J. C., Cheng, Y., Wang, M., Wu, Y. D., and Wu, A. (2016). XDirect Construction of 4-Hydroxybenzils via Para-Selective C–C Bond Coupling of Phenols and Aryl Methyl Ketones. Lett. Org. Lett. 18, 4360–4363. doi:10.1021/acs.orglett.6b02118
Xu, L., Chen, Y., Shen, Z., Wang, Y., and Li, M. (2018a). I2/Fe(NO3)3·9H2O-catalyzed Oxidative Synthesis of Aryl Carboxylic Acids from Aryl Alkyl Ketones and Secondary Benzylic Alcohols. Lett. Tetrahedron Lett. 59, 4349–4354. doi:10.1016/j.tetlet.2018.10.060
Keywords: catalytic oxidative cleavage, lignin model compounds, carboxylic acids, Fe(NO3)3.9H2O/NaI/DMSO, C(OH)-C bonds
Citation: Wang X, Sun H, Li C, Niu S, Gao Y, Chen Y, Xu T, Wang J and Xu H (2022) Catalytic Oxidative Cleavage of C(OH)-C Bonds in Lignin Model Compounds to Carboxylic Acids by Fe(NO3)3.9H2O/NaI/DMSO. Front. Chem. 10:933763. doi: 10.3389/fchem.2022.933763
Received: 01 May 2022; Accepted: 16 May 2022;
Published: 01 July 2022.
Edited by:
Jinliang Song, Guangdong University of Technology, ChinaCopyright © 2022 Wang, Sun, Li, Niu, Gao, Chen, Xu, Wang and Xu. This is an open-access article distributed under the terms of the Creative Commons Attribution License (CC BY). The use, distribution or reproduction in other forums is permitted, provided the original author(s) and the copyright owner(s) are credited and that the original publication in this journal is cited, in accordance with accepted academic practice. No use, distribution or reproduction is permitted which does not comply with these terms.
*Correspondence: Tianwei Xu, 27296930@qq.com; Jinhui Wang, 15999290001@163.com; Huanjun Xu, 15798946232@163.com
†These authors have contributed equally to this work and share first authorship