- School of Biotechnology and Health Sciences, Wuyi University, Jiangmen, China
α-Glucosidase inhibitors are known to prevent the digestion of carbohydrates and reduce the impact of carbohydrates on blood glucose. To develop novel α-glucosidase inhibitors, a series of 5-fluoro-2-oxindole derivatives (3a ∼ 3v) were synthesized, and their α-glucosidase inhibitory activities were investigated. Biological assessment results showed that most synthesized compounds presented potential inhibition on α-glucosidase. Among them, compounds 3d, 3f, and 3i exhibited much better inhibitory activity with IC50 values of 49.89 ± 1.16 μM, 35.83 ± 0.98 μM, and 56.87 ± 0.42 μM, respectively, which were about 10 ∼ 15 folds higher than acarbose (IC50 = 569.43 ± 43.72 μM). A kinetic mechanism study revealed that compounds 3d, 3f, and 3i inhibited the α-glucosidase in a reversible and mixed manner. Molecular docking was carried out to simulate the affinity between the compound and α-glucosidase.
1 Introduction
Diabetes is a chronic metabolic disorder disease that increases the risk of cancer, stroke, peripheral arterial disease, cardiovascular disease, retinopathy, and kidney disease. (Wang et al., 2017; Sonia et al., 2019; Proença et al., 2019; Proença et al., 2017; Proença et al., 2018; Rocha et al., 2019; Santos et al., 2018; Wu et al., 2014; Wu et al., 2017). The prevalence of diabetes at all ages worldwide is rising. It is estimated that by 2030, the prevalence of diabetes may rise from 2.8% (171 million) in 2000 to 4.4% (366 million) (Zhong et al., 2019). Type 2 diabetes, which is characterized by insulin resistance, is the most common, which accounts for approximately 90% of all diabetic patients (Taha et al., 2015; Leong et al., 2019; Settypalli et al., 2019).
α-Glucosidase is an indispensable enzyme in the sugar metabolism pathway of organisms, and its main function is to hydrolyze glycosidic bonds into glucose (Chaudhry et al., 2019; Dan et al., 2019; Gollapalli et al., 2019; Krishna et al., 2019; Mendieta-Moctezuma et al., 2019; Spasov et al., 2019; Ye et al., 2019). Thus inhibiting the α-glucosidase would obviously control the postprandial hyperglycemia. α-Glucosidase inhibitors can block the hydrolysis of 1, 4-glycosidic bonds and delay the hydrolysis of carbohydrates into glucose, resulting in the effective reduction of postprandial blood sugar (Al-Salahi, et al., 2018; Qamar, et al., 2018; Shah, et al., 2018; Wang, et al., 2018). Up to now, a great number of naturally occurring and synthetic α-glucosidase inhibitors have been reported. However, only several well-known inhibitors, such as acarbose, voglibose, and miglitol, are used clinically as first-line drugs. Moreover, these drugs have uncomfortable side effects (e.g., flatulence, abdominal pain, and diarrhea) (Taha et al., 2018a; Kasturi et al., 2018; Prachumart et al., 2018). These prompt us to develop effective and safe α-glucosidase inhibitors from natural sources.
Lots of compounds from natural sources have shown potential inhibitory activity on α-glucosidase. Oxindoles, the important indole-based derivatives, widely exist in many natural alkaloids. It was reported that oxindoles have the ability to inhibit the α-glucosidase (Khan et al., 2014; Asadollahi-Baboli and Dehnavi, 2018; Taha et al., 2018b). Moreover, oxindoles have attracted much attention due to their broad-spectrum biological activity, such as anti-inflammatory, anti-bacterial, and anti-tumor. (Yang et al., 2014; Xu et al., 2016; Alvarez et al., 2018; Bao et al., 2018; Huang et al., 2019). In addition, fluorine, a key atom in medicine, might enhance metabolic stability, improve the pharmacodynamic effect, and eliminate active metabolic intermediates (Johnson et al., 2020). Hence, 5-fluoro-2-oxindole was selected as the leading structure to synthesize the title compounds (3a ∼ 3u) through the condensation with the substituted aromatic aldehydes, followed by the screening on α-glucosidase inhibitory activities and the molecular docking studies.
2 Results and Discussion
2.1 Chemistry
The 5-fluoro-2-oxindole derivatives (3a ∼ 3v) were prepared according to the synthetic route shown in Scheme 1. As the starting material, 5-fluoro-2-oxindole (1) was condensed with the substituted aromatic aldehydes (2a ∼ 2v) in the presence of KOH to produce the title compounds (3a ∼ 3v). The structures of compounds 3a ∼ 3v were characterized by 1H NMR, MS, and melting point.
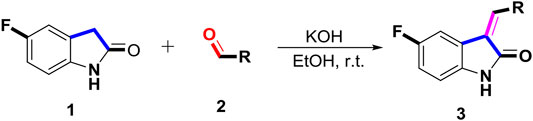
SCHEME 1. Synthetic route to 5-fluoro-2-oxindole derivatives (3a ∼ 3u). Reagents and conditions: 5-fluoro-2-oxindole (1.0 mmol, 1.0 equiv), substituted aldehydes (1.5 mmol, 1.5 equiv), KOH (6.0 mmol, 6.0 equiv), and EtOH, r. t., 3 h.
2.2 α-Glucosidase Inhibition Assay
α-Glucosidase from Saccharomyces cerevisiae (EC 3.2.1.20) was widely accepted and used to evaluate the inhibitory activity against α-glucosidase. Then, the inhibitory activity of compounds (3a ∼ 3v) on α-glucosidase from S. cerevisiae was investigated using p-NPG as the substrate. First, the inhibitory activities of compounds (3a ∼ 3v) were screened at a concentration of 100 μM. As shown in Table 1, compounds 3d, 3f, and 3i presented better activities, with inhibition of ∼90% at a concentration of 100 μM and those of compounds (3g, 3n, 3p, and 3r) were ∼50% at a concentration of 100 μM, while those of other compounds were below 50% at a concentration of 100 μM. Then, IC50 values of compounds 3d, 3f, 3i, 3n, 3p, and 3r were measured due to their better inhibitory activities. The IC50 values are summarized in Table 1, and the inhibitory activities of compounds 3d, 3f, and 3i on α-glucosidase are presented in Figure 1. For analyzing the inhibitory activities of compounds (3a ∼ 3v), the inhibitory activities of 5-fluoro-2-oxindole and acarbose were investigated. Among all compounds, compounds 3d, 3f, and 3i exhibited much better potent inhibitory activity with IC50 values of 56.87 ± 0.42 μM, 49.89 ± 1.16 μM, and 35.83 ± 0.98 μM, respectively, which were about 10 ∼ 15 folds higher than that of acarbose (IC50 = 569.43 ± 43.72 μM) and significantly better than that of 5-fluoro-2-oxindole (IC50 = (7.51 ± 0.17)×103 μM).
2.3 Structure–Activity Relationships
Then, the structure–activity relationships of compounds (3a ∼ 3v) were analyzed according to the experimental data in Table 1. First, the steric effect of substituents at aldehydes was investigated based on the inhibitory activities of compounds 3a/3b/3c, 3d/3e/3f, 3g/3h/3i, and 3m/3n, with -F, -Cl, -Br, and -CF3 at ortho-, meta- and para-positions of the benzene ring, and the order of the inhibitory activities is 4- > 2- > 3-. When the substituent was OCH3 (3j/3k/3l), it turned out just the opposite. Second, the electronic effect of substituents was considered. The introduction of -F, -Cl, -Br, -CF3, and -OCH3 at phenyl para-position (compounds 3c, 3f, 3i, 3l, and 3r) could enhance the inhibitory activities with the inhibitory activity order of -Cl > -Br > -SCH3 > -OCH3 > -F. It could be seen that the inhibitory activity has no correlation with the steric and electronic effects of substituents at aldehydes. Furthermore, the introduction of various heterocycles (compounds 3s, 3t, 3u, and 3v) presented a negative effect on inhibitory activity. It could be concluded that the introduction of the substituents at the benzyl para-position of substituted aldehydes is beneficial to the improvement of the inhibitory activity. Therefore, the further derivatization of title compounds might be focused on the screening of substituents at the benzyl para-position of substituted aldehydes.
2.4 Inhibitory Mechanism Analysis
For further understanding the interaction mechanism of title compounds with α-glucosidase, compounds 3d, 3f, and 3i were selected to investigate the inhibition mechanism of α-glucosidase through revealing the linkage between enzyme activity and the enzyme concentration in the presence of test compounds (figures for the inhibitory mechanism analysis of compounds 3f was shown in Figure 2 and figures for the inhibitory mechanism analysis of compounds 3d and 3i have been shown in the supporting information). The increasing concentrations of compounds 3d, 3f, or 3i reduced the slope of the lines and the plots of the enzyme activity vs. the enzyme concentration at different concentrations of compounds 3d, 3f, or 3i gave a group of straight lines, which all passed through the origin, indicating that the inhibitor reduces the activity of the enzyme and the inhibition of compounds 3d, 3f, or 3i against α-glucosidase was reversible.
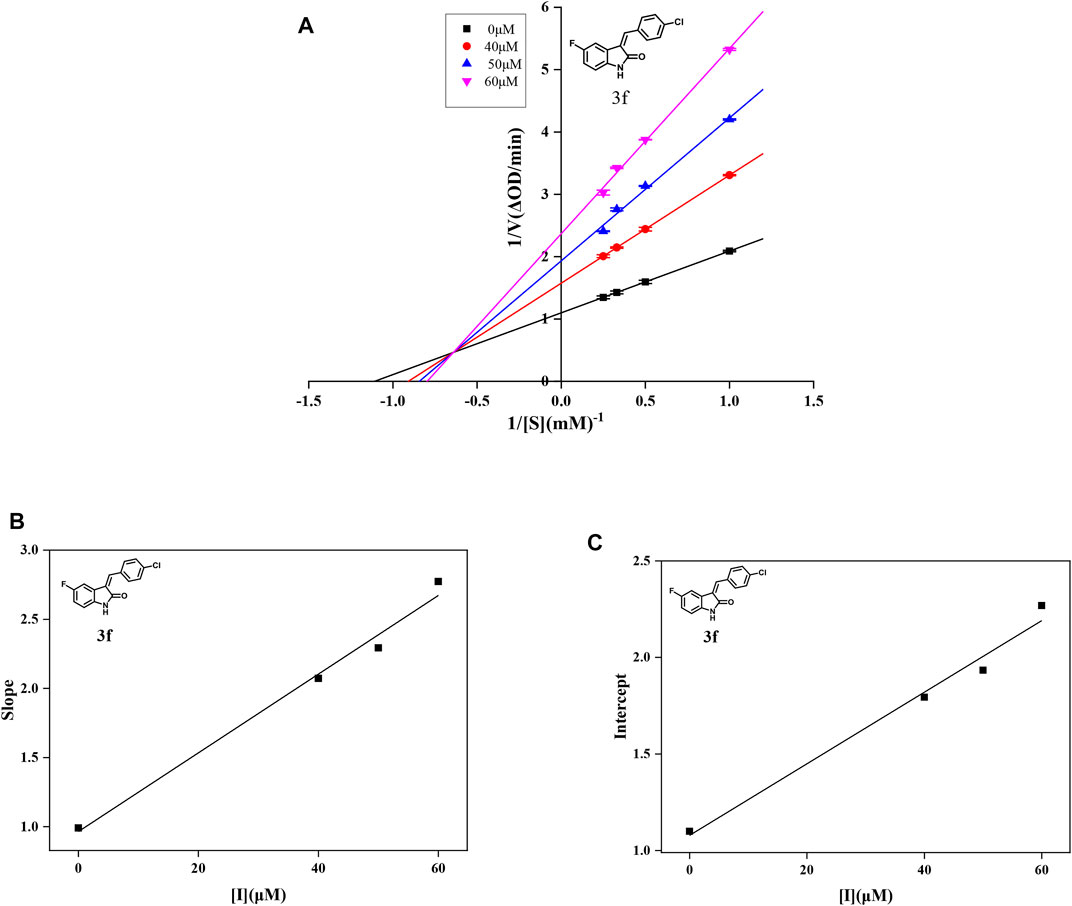
FIGURE 2. (A) Lineweaver–Burk plots of compound 3f on α-glucosidase. (B) Plot of slope vs. the concentration of compound 3f for the calculation of the inhibition constant KI. (C) Plot of intercept vs. the concentration of compound 3f for the determination of the inhibition constant KIS.
In order to obtain the inhibition kinetics type of compounds 3d, 3f, and 3i, the Lineweaver–Burk plot analysis method was carried out with different concentrations of test compounds and substrates. For compounds 3d, 3f, and 3i, the plots of 1/ν vs. 1/[S] gave a group of straight lines with different slopes that intersected the same point at the second quadrant, indicating that compounds 3d, 3f, and 3i were mixed-type inhibitors. Then, the KI values were calculated as 14.96, 33.85, and 22.72 μM, respectively, and the KIS values were calculated as 453.85, 58.31, and 24.74 μM, respectively, which are summarized in Table 2. These results showed that compounds 3d, 3f, and 3i could bind with the free enzyme as well as the enzyme–substrate complex of α-glucosidase. In addition, the inhibition types of compounds 3d, 3f, and 3i, different from that of acarbose, are the competitive inhibition type.
2.5 Molecular Docking Studies
With the purpose of acquiring a better comprehension of the mutual effects between compounds 3d, 3f, and 3i and α-glucosidase, molecular docking studies were implemented using Sybyl tools. The 3D structures of S. cerevisiae α-glucosidase (EC 3.2.1.20) are unavailable, and oligo-1, 6-glucosidase from S. cerevisiae (PDB: 1UOK) was selected as the target protein. Also, the sequence similarity is about 62.0% and the sequence identity is about 38.0%, as compared with α-glucosidase. As demonstrated in Figure 3A, compounds 3d, 3f, and 3i were well inserted into the active pocket of α-glucosidase, with similar angles and positions. A hydrogen bond between carbonyl of compounds 3d, 3f, and 3i and amino acid sequences of GLN330 was formed to increase the affinity with α-glucosidase (Figure 3B). These similar integrated situations of compounds 3d, 3f, and 3i with α-glucosidase indicated the same inhibition mechanism. In addition, the lipophilic potential interaction between 3d, 3f, and 3i and the active pocket was investigated. As shown in Figure 3C, the active pocket external is more lipophilic than the interior. Then, in Figure 3D, the fluorophenyl as the lipophilic fraction of 3d, 3f, and 3i was close to the lipophilic potential region, while the pyrrole ring as the hydrophilic fraction was near to the hydrophilic region.
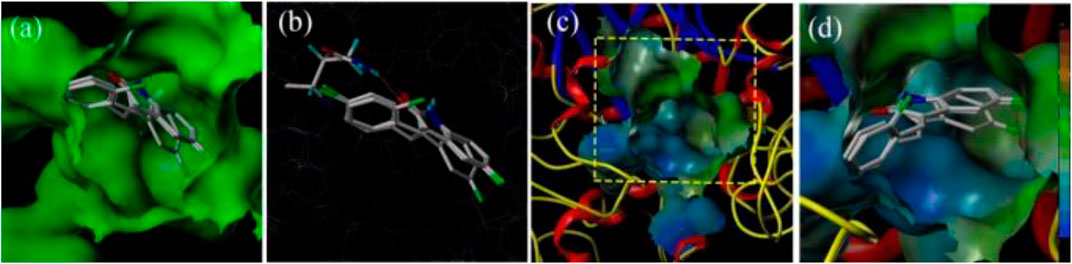
FIGURE 3. (A) The insertion of compounds 3d, 3f, and 3i into the active pocket of α-glucosidase; (B) The hydrogen-bond interaction between carbonyl of the compounds (3d, 3f, and 3i) and α-glucosidase; (C) The lipophilic interaction between the compounds (3d, 3f, and 3i) and α-glucosidase; (D) The fluorophenyl as the lipophilic fraction of compounds 3d, 3f, and 3i binding to α-glucosidase.
3 EXPERIMENTAL
3.1 Chemicals
α-Glucosidase from S. cerevisiae (EC 3.2.1.20) and 4-nitrophenyl-β-D-galactopyranoside (p-NPG) were supplied by Sigma-Aldrich. All other reagents were of analytical grade. The water used was re-distilled and ion-free.
3.2 Instruments
1H NMR was recorded by using a NMR spectrometer (DPX-500 MHz) in chloroform-d or DMSO-d6, with chemical shifts (d) given in parts per million (ppm) relative to TMS as internal standard and recorded. Mass spectrometry was determined on a (LCQTM) LC-MS supplied by Thermo Fisher Scientific (Shanghai) Co., Ltd. Melting points were measured on a micro melting point instrument, which was supplied by Shanghai Yidian Physical Optical Instrument Co., Ltd. The absorbance was recorded using a microplate reader supplied by Thermo Fisher Scientific (Shanghai) Co., Ltd.
3.3 Synthesis of Compounds 3a ∼ 3v
To a solution of 1 (1.0 mmol, 1.0 equiv.) and 2a ∼ 2v in 10 ml absolute ethanol was added KOH (6 mmol, 6.0 equiv.), followed by the addition of the corresponding substituted aldehydes. Then, the mixture was stirred at room temperature for 3 h and detected to be complete by TLC. The mixture was adjusted to the pH value between 2.0 and 3.0, followed by the evaporation of ethanol, and extraction with ethyl acetate. The ethyl acetate layer was washed with saturated NaHCO3 and brine and then was concentrated under vacuum to give the crude product, subsequently by the recrystallization with ethanol to give compounds 3a ∼ 3v.
(Z)-5-Fluoro-3-(2-Fluorobenzylidene) Indolin-2-One (3a). Orange-yellow crystal; yield 65.0%; m p.: 228.3–230.2°C; 1H NMR (500 MHz, DMSO-d6) δ 10.73 (s, 1H), 7.77 (td, J = 7.7, 1.7 Hz, 1H), 7.65–7.55 (m, 2H), 7.47–7.36 (m, 2H), 7.13 (td, J = 9.0, 2.6 Hz, 1H), 6.94 (dd, J = 9.0, 2.6 Hz, 1H), and 6.88 (dd, J = 8.5, 4.6 Hz, 1H); HRMS (ESI) calculated for C15H9F2NO [M - H]−: m/z = 256.24, found 255.85.
(Z)-5-Fluoro-3-(3-Fluorobenzylidene) Indolin-2-One (3b). Orange-yellow crystal; yield 41.2%; m p.: 191.4–192.2°C; 1H NMR (500 MHz, chloroform-d) δ 7.81 (s, 1H), 7.49 (td, J = 8.0, 5.7 Hz, 1H), 7.42 (dp, J = 7.6, 0.9 Hz, 1H), 7.30 (ddd, J = 8.9, 6.8, 2.4 Hz, 2H), 7.20–7.15 (m, 1H), 6.97 (td, J = 8.7, 2.5 Hz, 1H), 6.82 (dd, J = 8.6, 4.4 Hz, 1H); HRMS (ESI) calculated for C15H9F2NO [M - H]−: m/z = 256.24, found 256.09.
(Z)-5-Fluoro-3-(4-Fluorobenzylidene) Indolin-2-One (3c). Orange-yellow crystal; yield 55.7%; mp.: 217.0–219.6°C; 1H NMR (500 MHz, chloroform-d) δ 8.10 (s, 1H), 7.82 (s, 1H), 7.64 (dd, J = 8.5, 5.4 Hz, 2H), 7.32 (dd, J = 9.1, 2.5 Hz, 1H), 7.20 (t, J = 8.4 Hz, 2H), 6.96 (td, J = 8.8, 2.6 Hz, 1H), and 6.83 (dd, J = 8.5, 4.4 Hz, 1H); HRMS (ESI) calculated for C15H9F2NO [M-H]−: m/z = 256.24, found 256.17.
(Z)-3-(2-Chlorobenzylidene)-5-Fluoroindolin-2-One (3d). Orange-yellow crystal; yield 82.4%; m p.: 219.7–221.9°C; 1H NMR (500 MHz, chloroform-d) δ 8.12 (s, 1H), 7.92 (s, 1H), 7.68 (dd, J = 7.5, 1.8 Hz, 1H), 7.54 (dd, J = 7.9, 1.4 Hz, 1H), 7.40 (dtd, J = 20.5, 7.5, 1.6 Hz, 2H), 7.04 (dd, J = 8.9, 2.6 Hz, 1H), 6.94 (td, J = 8.8, 2.6 Hz, 1H), and 6.82 (dd, J = 8.5, 4.3 Hz, 1H); HRMS (ESI) calculated for C15H9ClFNO [M-H]−: m/z = 272.69, found 271.96.
(Z)-3-(3-Chlorobenzylidene)-5-Fluoroindolin-2-One (3e). Orange-yellow crystal; yield 47.8%; mp: 237.4–238.9°C; 1H NMR (500 MHz, chloroform-d) δ 7.97 (s, 1H), 7.79 (s, 1H), 7.58 (dd, J = 2.1, 1.1 Hz, 1H), 7.54–7.50 (m, 1H), 7.46–7.43 (m, 2H), 7.25 (d, J = 2.5 Hz, 1H), 6.97 (td, J = 8.7, 2.6 Hz, 1H), and 6.82 (dd, J = 8.5, 4.4 Hz, 1H); HRMS (ESI) calculated for C15H9ClFNO [M-H]−: m/z = 272.69, found 272.10.
(Z)-3-(4-Chlorobenzylidene)-5-Fluoroindolin-2-One (3f). Orange-yellow crystal; yield 44.2%; mp: 200.6–202.5°C; 1H NMR (500 MHz, chloroform-d) δ 7.80 (s, 1H), 7.58 (d, J = 8.3 Hz, 2H), 7.51–7.45 (m, 2H), 7.30 (dd, J = 8.9, 2.5 Hz, 1H), 6.96 (td, J = 8.7, 2.6 Hz, 1H), and 6.82(dd, J = 8.5, 4.4 Hz, 1H); HRMS (ESI) calculated for C15H9ClFNO [M-H]−: m/z = 272.69, found 272.39.
(Z)-3-(2-Bromobenzylidene)-5-Fluoroindolin-2-One (3g). Orange-yellow crystal; yield 69.7%; mp: 194.9–197.1°C; 1H NMR (500 MHz, chloroform-d) δ 7.90–7.82 (m, 1H), 7.73 (dd, J = 8.1, 1.1 Hz, 1H), 7.66 (dt, J = 7.7, 1.9 Hz, 1H), 7.44 (td, J = 7.4, 1.1 Hz, 1H), 7.34 (td, J = 7.7, 1.6 Hz, 1H), 7.00 (dd, J = 8.9, 2.6 Hz, 1H), and 6.94 (td, J = 8.7, 2.6 Hz, 1H); HRMS (ESI) calculated for C15H9BrFNO [M + Na]+: m/z = 340.15, found 340.59.
(Z)-3-(3-Bromobenzylidene)-5-Fluoroindolin-2-One (3h). Orange-yellow crystal; yield 70.4%; mp: 222.6–223.8°C; 1H NMR (500 MHz, chloroform-d) δ 8.31 (s, 1H), 7.86–7.67 (m, 2H), 7.66–7.48 (m, 2H), 7.38 (t, J = 7.9 Hz, 1H), 7.24 (d, J = 2.6 Hz, 1H), 6.97 (td, J = 8.7, 2.6 Hz, 1H), and 6.84 (dd, J = 8.5, 4.4 Hz, 1H); HRMS (ESI) calculated for C15H9BrFNO [M + Na]+: m/z = 357.15, found 357.97.
(Z)-3-(4-Bromobenzylidene)-5-Fluoroindolin-2-One (3i). Orange-yellow crystal; yield 65.7%; mp: 249.3–251.6°C; 1H NMR (500 MHz, chloroform-d) δ 7.77 (s, 1H), 7.67–7.61 (m, 2H), 7.51 (d, J = 8.4 Hz, 2H), 7.30 (dd, J = 9.0, 2.6 Hz, 1H), 6.96 (td, J = 8.7, 2.6 Hz, 1H), and 6.81 (dd, J = 8.5, 4.4 Hz, 1H); HRMS (ESI) calculated for C15H9BrFNO [M + Na]+: m/z = 341.15, found 340.97.
(Z)-5-Fluoro-3-(2-Methoxybenzylidene) Indolin-2-One (3j). Orange-yellow crystal; yield 52.7%; mp: 227.1–227.8°C; 1H NMR (500 MHz, chloroform-d) δ 8.02 (s, 1H), 7.97 (s, 1H), 7.67 (dd, J = 7.7, 1.7 Hz, 1H), 7.49–7.42 (m, 1H), 7.28 (dd, J = 9.2, 2.7 Hz, 1H), 7.06 (td, J = 7.5, 1.0 Hz, 1H), 7.00 (dd, J = 8.3, 1.0 Hz, 1H), 6.91 (td, J = 8.8, 2.6 Hz, 1H), 6.80 (dd, J = 8.5, 4.4 Hz, 1H), and 3.89 (s, 3H); HRMS (ESI) calculated for C16H12FNO2 [M-H]−: m/z = 268.28, found 268.19.
(Z)-5-Fluoro-3-(3-Methoxybenzylidene) Indolin-2-One (3k). Orange-yellow crystal; yield 73.3%; mp: 200.1–202.1°C; 1H NMR (500 MHz, chloroform-d) δ 7.86 (s, 1H), 7.45–7.38 (m, 2H), 7.23 (d, J = 7.5 Hz, 1H), 7.14 (t, J = 2.0 Hz, 1H), 7.04–6.90 (m, 2H), 6.82 (dd, J = 8.6, 4.4 Hz, 1H), and 3.86 (s, 3H); HRMS (ESI) calculated for C16H12FNO2 [M-H]−: m/z = 268.28, found 268.01.
(Z)-5-Fluoro-3-(4-Methoxybenzylidene) Indolin-2-One (3l). Orange-yellow crystal; yield 42.8%; mp: 358.8–359.9°C; 1H NMR (500 MHz, chloroform-d) δ 7.84 (s, 1H), 7.68–7.62 (m, 2H), 7.49 (dd, J = 9.3, 2.6 Hz, 1H), 7.04–7.00 (m, 2H), 6.93 (dd, J = 8.8, 2.6 Hz, 1H), 6.83 (dt, J = 9.0, 3.6 Hz, 1H), and 3.91 (s, 3H); HRMS (ESI) calculated for C16H12FNO2 [M-H]−: m/z = 268.28, found 268.11.
(Z)-5-Fluoro-3-[2-(Trifluoromethyl) Benzylidene) Indolin-2-One (3m). Orange-yellow crystal; yield 53.1%; mp: 235.2–236.4°C; 1H NMR (500 MHz, chloroform-d) δ 8.33–8.22 (m, 1H), 8.01 (q, J = 2.5 Hz, 1H), 7.83 (d, J = 7.8 Hz, 1H), 7.70–7.65 (m, 2H), 7.63–7.55 (m, 1H), 6.93 (td, J = 8.8, 2.6 Hz, 1H), 6.83 (dd, J = 8.5, 4.3 Hz, 1H), and 6.66 (dd, J = 8.7, 2.6 Hz, 1H); HRMS (ESI) calculated for C16H9F4NO [M-H]−: m/z = 306.25, found 305.91.
(Z)-5-Fluoro-3-[3-(Trifluoromethyl) Benzylidene] Indolin-2-One (3n). Orange-yellow crystal; yield 42.9%; mp: 199.7–200.5°C; 1H NMR (500 MHz, chloroform-d) δ 7.87 (s, 1H), 7.85 (s, 1H), 7.81 (d, J = 7.7 Hz, 1H), 7.73 (d, J = 7.8 Hz, 1H), 7.64 (t, J = 7.8 Hz, 1H), 7.18 (dd, J = 8.9, 2.6 Hz, 1H), 6.97 (td, J = 8.7, 2.6 Hz, 1H), and 6.83 (dd, J = 8.6, 4.3 Hz, 1H); HRMS (ESI) calculated for C16H9F4NO [M-H]−: m/z = 306.25, found 306.01.
(Z)-3-(2,4-Difluorobenzylidene)-5-Fluoroindolin-2-One (3o). Orange-yellow crystal; yield 55.3%; mp: 221.1–224.3°C; 1H NMR (500 MHz, chloroform-d) δ 8.31–8.21 (s, 1H), 8.01 (s, 1H), 7.83 (d, J = 7.8 Hz, 1H), 7.68–7.65 (m, 2H), 7.62–7.58 (m, 1H), 6.93 (td, J = 8.8, 2.6 Hz, 1H), 6.83 (dd, J = 8.5, 4.3 Hz, 1H), and 6.66 (dd, J = 8.7, 2.6 Hz, 1H); HRMS (ESI) calculated for C16H9F4NO [M + H]+: m/z = 276.23, found 278.01.
(Z)-3-(3,4-Difluorobenzylidene)-5-Fluoroindolin-2-One (3p). Orange-yellow crystal; yield 44.6%; mp: 189.2–190.2°C; 1H NMR (500 MHz, chloroform-d) δ 8.99 (dd, J = 10.1, 2.7 Hz, 1H), 8.91 (ddd, J = 4.6, 2.0, 0.8 Hz, 1H), 7.83 (td, J = 7.7, 1.9 Hz, 1H), 7.73 (s, 1H), 7.63 (dd, J = 7.7, 1.1 Hz, 1H), 7.36 (ddd, J = 7.7, 4.7, 1.1 Hz, 1H), 7.01 (td, J = 8.6, 2.7 Hz, 1H), and 6.80 (dd, J = 8.5, 4.4 Hz, 1H); HRMS (ESI) calculated for C15H8F3NO [M-H]−: m/z = 274.23, found 274.02.
(Z)-3-Benzylidene-5-Fluoroindolin-2-One (3q). Orange-yellow crystal; yield 76.3%; mp: 198.9–199.7°C; 1H NMR (500 MHz, chloroform-d) δ 8.93 (s, 1H), 7.90 (s, 1H), 7.67–7.62 (m, 2H), 7.53–7.46 (m, 3H), 7.36 (dd, J = 9.1, 2.6 Hz, 1H), 6.94 (td, J = 8.7, 2.5 Hz, 1H), and 6.86 (dd, J = 8.5, 4.5 Hz, 1H); HRMS (ESI) calculated for C15H8F3NO [M-H]−: m/z = 238.25, found 237.99.
(Z)-5-Fluoro-3-[4-(Methylthio) Benzylidene] Indolin-2-One (3r). Orange-yellow crystal; yield 41.8%; mp: 246.1–247.1°C; 1H NMR (500 MHz, chloroform-d) δ 8.13 (s, 1H), 7.81 (s, 1H), 7.61–7.55 (m, 2H), 7.44 (dd, J = 9.0, 2.6 Hz, 1H), 7.36–7.31 (m, 2H), 6.94 (td, J = 8.7, 2.5 Hz, 1H), 6.82 (dd, J = 8.5, 4.4 Hz, 1H), and 2.56 (s, 3H); HRMS (ESI) calculated for C16H12FNOS [M-H]−: m/z = 284.34, found 284.13.
(Z)-5-Fluoro-3-(Furan-2-ylmethylene) Indolin-2-One (3s). Orange-yellow crystal; yield 72.5%; mp: 237.9–238.8°C; 1H NMR (500 MHz, chloroform-d) δ 7.76 (s, 1H), 7.41 (s, 1H), 7.24–7.16 (m, 2H), 6.88 (td, J = 8.8, 2.5 Hz, 1H), 6.82 (dd, J = 8.5, 4.2 Hz, 2H), and 6.42 (dt, J = 4.0, 2.1 Hz, 1H); HRMS (ESI) calculated for C16H12FNOS [M-H]−: m/z = 228.21, found 227.95.
(Z)-5-Fluoro-3-(Thiophen-2-ylmethylene) Indolin-2-One (3t). Orange-yellow crystal; yield 44.6%; mp: 219.0–223.4°C; 1H NMR (500 MHz, chloroform-d) δ 8.01 (dd, J = 9.4, 2.5 Hz, 1H), 7.97 (s, 1H), 7.85 (s, 1H), 7.65 (dd, J = 23.4, 4.4 Hz, 2H), 7.23 (dd, J = 5.1, 3.7 Hz, 1H), 6.99 (td, J = 8.7, 2.5 Hz, 1H), and 6.84 (dd, J = 8.5, 4.5 Hz, 1H); HRMS (ESI) calculated for C16H12FNOS [M-H]−: m/z = 244.27, found 244.14.
(Z)-3-[(1H-Pyrrol-2-yl)methylene]-5-Fluoroindolin-2-One (3u). Orange-yellow crystal; yield 54.4%; mp: 198.9–201.6°C; 1H NMR (500 MHz, chloroform-d) δ 13.30 (s, 1H), 7.76 (s, 1H), 7.41 (s, 1H), 7.22 (s, 1H), 7.19 (dd, J = 8.7, 2.5 Hz, 1H), 6.88 (td, J = 8.8, 2.5 Hz, 1H), 6.82 (d, J = 4.2 Hz, 2H), and 6.42 (dt, J = 4.0, 2.1 Hz, 1H); HRMS (ESI) calculated for C13H9FN2O [M-H]−: m/z = 227.23, found 227.02.
(Z)-5-Fluoro-3-(Pyridin-2-ylmethylene) Indolin-2-One (3v). Orange-yellow crystal; yield 47.9%; mp: 248.5–251.2°C; 1H NMR (500 MHz, chloroform-d) δ 8.99 (dd, J = 10.1, 2.7 Hz, 1H), 8.91 (ddd, J = 4.6, 2.0, 0.8 Hz, 1H), 7.83 (td, J = 7.7, 1.9 Hz, 1H), 7.73 (s, 1H), 7.63 (dd, J = 7.7, 1.1 Hz, 1H), 7.36 (ddd, J = 7.7, 4.7, 1.1 Hz, 1H), 7.01 (td, J = 8.6, 2.7 Hz, 1H), and 6.80 (dd, J = 8.5, 4.4 Hz, 1H); HRMS (ESI) calculated for C13H9FN2O [M-H]−: m/z = 239.24, found 238.93.
3.4 α-Glucosidase Inhibitory Assay
The α-glucosidase inhibition of synthetic compounds was performed as previously reported methods with minor modification (Deng et al., 2022) which is as follows: briefly, 130 μl of phosphate buffer (10 mM, pH 6.8), 10 μl of α-glucosidase (1 U/ml), and 10 μl of test compound solution were added into the wells of a 96-well plate, followed by incubation for 10 min at 37°C. Then, 50 μl of p-NPG (1 mM) was added, and the plate was further incubated for 30 min at 37°C. Finally, the absorbance of each well was recorded at 405 nm using a microplate reader. Acarbose was used as the reference. The inhibition of the test compound on α-glucosidase was calculated as follows: inhibition ratio (%) = [(A− B)/A] × 100, where A is the absorbance of blank and B is the absorbance of the test compound. Each concentration was experimented four times in parallel. Half inhibitory concentration (IC50) was obtained from the fitting curve of inhibition ratio vs. test compound with different concentrations.
3.5 Kinetics Mechanism Analysis
Compounds 3d, 3f, and 3i with much better α-glucosidase inhibitory activity were selected for kinetic analysis. The experiments were performed to investigate the kinetics mechanism of compounds 3d, 3f, and 3i by the previously reported method (Xu et al., 2019). To determine the inhibition mechanism, the final concentrations for 3d were 0, 40, 50, 60 μM, for 3f were 0, 40, 50, 60 μM, and for 3i were 0, 30, 40, 50 μM, the final substrate p-NPG concentration was 0.25 mM, and the final concentrations for α-glucosidase were 3.75 × 10–2, 5.00 × 10–2, 6.25 × 10–2, and 7.50 × 10–2 U/ml. Then, the inhibition rates were measured by the aforementioned method.
To analyze the inhibition type, the final concentrations for 3d were 0, 40, 50, and 60 μM, for 3f were 0, 40, 50, and 60 μM, and for 3i were 0, 30, 40, and 50 μM, the final α-glucosidase concentration was 5.00 × 10–2 U/ml, and final concentrations for substrate p-NPG concentration were 0.25, 0.50, 0.75, and 1.00 mM. The inhibition rates were obtained by the aforementioned method. The inhibition type on α-glucosidase was analyzed by using Lineweaver–Burk plots of the inverse of velocities (1/v) vs. the inverse of substrate concentration 1/[S]. The KI and KIS were obtained from the slope and the vertical intercept vs. the inhibitor concentration, respectively.
3.6 Molecular Docking
The molecular docking between compounds 3d, 3f, and 3i and α-glucosidase were simulated with Sybyl-2.1.1 (Tripos, Shanghai, China) (Hu et al., 2021). First, compounds 3d, 3f, and 3i were prepared by hydrogenation and energy minimization using the MM2 program. In the energy minimization program, the energy convergence criterion was revised to 0.001 kcal/mol, optimizing the energy gradient that was revised to 2,500 times, and the charge was run with the Gasteiger–Huckle charges method. Next, after being retrieved from the RCSB Protein Database (PDB: 1UOK), the α-glucosidase structure was prepared, followed by the procedure of removing water, termini treatment, adding hydrogens, adding charges with the MMFF94, fixing side chain amides, and staged minimization. The active pocket of α-glucosidase was generated with the automatic mode. Then, the molecular docking between compounds 3d, 3f, and 3i and α-glucosidase were operated in the default format.
4 Conclusion
In summary, a series of α-glucosidase inhibitors based on 5-fluoro-2-oxindole have been synthesized and evaluated. Most synthesized compounds presented better potential inhibitory on α-glucosidase than the parent compound. Among them, compounds 3d, 3f, and 3i exhibited much better inhibitory activity with IC50 values of 49.89 ± 1.16, 35.83 ± 0.98 and 56.87 ± 0.42 μM, respectively, which were about 10 ∼ 15 folds higher activities than acarbose (IC50 = 569.43 ± 43.72 μM) that was used as reference. The kinetics mechanism study revealed that compounds 3d, 3f, and 3i inhibited the α-glucosidase in a reversible and mixed manner. Molecular docking confirmed that compounds could effectively integrate with α-glucosidase. These results indicated that these synthesized compounds could be used as the leading structure in the research and development of α-glucosidase inhibitors for the prevention and treatment of type 2 diabetes.
Data Availability Statement
The original contributions presented in the study are included in the article/Supplementary Material; further inquiries can be directed to the corresponding authors.
Author Contributions
JL, Q-ML, and Y-NY contributed to the synthesis and inhibitory activity evaluation. DX, LL, M-YL, J-PL, and Y-Z contributed to the characterization and analysis of 1H NMR, 13C NMR, and MS. ZX, NF, and CL supervised the work and prepared the manuscript.
Funding
This work was financially supported by the Department of Education of Guangdong Province (Nos. 2019KZDXM035, 2021KTSCX135, and 2021KCXTD044) and Special Funds for the Cultivation of Guangdong College Students’ Scientific and Technological Innovation (“Climbing Program” Special Funds, pdjh 2021a0504 and pdjh 2022b0532).
Conflict of Interest
The authors declare that the research was conducted in the absence of any commercial or financial relationships that could be construed as a potential conflict of interest.
Publisher’s Note
All claims expressed in this article are solely those of the authors and do not necessarily represent those of their affiliated organizations, or those of the publisher, the editors, and the reviewers. Any product that may be evaluated in this article, or claim that may be made by its manufacturer, is not guaranteed or endorsed by the publisher.
Supplementary Material
The Supplementary Material for this article can be found online at: https://www.frontiersin.org/articles/10.3389/fchem.2022.928295/full#supplementary-material
References
Al-Salahi, R., Ahmad, R., Anouar, E., Iwana Nor Azman, N. I., Marzouk, M., and Abuelizz, H. A. (2018). 3-Benzyl(phenethyl)-2-thioxobenzo[g]quinazolines as a New Class of Potent α-glucosidase Inhibitors: Synthesis and Molecular Docking Study. Future Med. Chem. 10, 1889–1905. doi:10.4155/fmc-2018-0141
Álvarez, R., Gajate, C., Puebla, P., Mollinedo, F., Medarde, M., and Peláez, R. (2018). Substitution at the Indole 3 Position Yields Highly Potent Indolecombretastatins against Human Tumor Cells. Eur. J. Med. Chem. 158, 167–183. doi:10.1016/j.ejmech.2018.08.078
Asadollahi-Baboli, M., and Dehnavi, S. (2018). Docking and QSAR Analysis of Tetracyclic Oxindole Derivatives as α-glucosidase Inhibitors. Comput. Biol. Chem. 76, 283–292. doi:10.1016/j.compbiolchem.2018.07.019
Bao, W., Wang, J.-Q., Xu, X.-T., Zhang, B.-H., Liu, W.-T., Lei, L.-S., et al. (2018). Copper-catalyzed Cyclization of 2-cyanobenzaldehydes and 2-isocyanoacetates: an Efficient Strategy for the Synthesis of Substituted 1-aminoisoquinolines. Chem. Commun. 54, 8194–8197. doi:10.1039/c8cc04733b
Chaudhry, F., Naureen, S., Ashraf, M., Al-Rashida, M., Jahan, B., Munawar, M. A., et al. (2019). Imidazole-pyrazole Hybrids: Synthesis, Characterization and In-Vitro Bioevaluation against α-glucosidase Enzyme with Molecular Docking Studies. Bioorg. Chem. 82, 267–273. doi:10.1016/j.bioorg.2018.10.047
Dan, W.-J., Zhang, Q., Zhang, F., Wang, W.-W., and Gao, J.-M. (2019). Benzonate Derivatives of Acetophenone as Potent α-glucosidase Inhibitors: Synthesis, Structure-Activity Relationship and Mechanism. J. Enzyme Inhibition Med. Chem. 34, 937–945. doi:10.1080/14756366.2019.1604519
Deng, X. Y., Ke, J. J., Zheng, Y. Y., Li, D. L., Zhang, K., Zheng, X., et al. (2022). Synthesis and Bioactivities Evaluation of Oleanolic Acid Oxime Ester Derivatives as α-glucosidase and α-amylase Inhibitors. J. Enzyme Inhib. Med. Chem. 37, 451–461. doi:10.1080/14756366.2021.2018682
Gollapalli, M., Taha, M., Javid, M. T., Almandil, N. B., Rahim, F., Wadood, A., et al. (2019). Synthesis of Benzothiazole Derivatives as a Potent α-glucosidase Inhibitor. Bioorg. Chem. 85, 33–48. doi:10.1016/j.bioorg.2018.12.021
Hu, C. M., Wang, W. J., Ye, Y. N., Kang, Y., Lin, J., Wu, P. P., et al. (2021). Novel Cinnamic Acid Magnolol Derivatives as Potent α-glucosidase and α-amylase Inhibitors: Synthesis, In Vitro and In Silico Studies. Bioorgan. Chem. 116, 105435. doi:10.1016/j.bioorg.2021.105291
Huang, Y., Zhang, B., Li, J., Liu, H., Zhang, Y., Yang, Z., et al. (2019). Design, Synthesis, Biological Evaluation and Docking Study of Novel Indole-2-Amide as Anti-inflammatory Agents with Dual Inhibition of COX and 5-LOX. Eur. J. Med. Chem. 180, 41–50. doi:10.1016/j.ejmech.2019.07.004
Johnson, B. M., Shu, Y.-Z., Zhuo, X., and Meanwell, N. A. (2020). Metabolic and Pharmaceutical Aspects of Fluorinated Compounds. J. Med. Chem. 63, 6315–6386. doi:10.1021/acs.jmedchem.9b01877
Kasturi, S. P., Surarapu, S., Uppalanchi, S., Dwivedi, S., Yogeeswari, P., Sigalapalli, D. K., et al. (2018). Synthesis, Molecular Modeling and Evaluation of α-glucosidase Inhibition Activity of 3,4-dihydroxy Piperidines. Eur. J. Med. Chem. 150, 39–52. doi:10.1016/j.ejmech.2018.02.072
Khan, M., Yousaf, M., Wadood, A., Junaid, M., Ashraf, M., Alam, U., et al. (2014). Discovery of Novel Oxindole Derivatives as Potent α-glucosidase Inhibitors. Bioorg. Med. Chem. 22, 3441–3448. doi:10.1016/j.bmc.2014.04.033
Leong, S. W., Awin, T., Mohd Faudzi, S. M., Maulidiani, M., Shaari, K., and Abas, F. (2019). Synthesis and Biological Evaluation of Asymmetrical Diarylpentanoids as Antiinflammatory, Anti-α-glucosidase, and Antioxidant Agents. Med. Chem. Res. 28, 2002–2009. doi:10.1007/s00044-019-02430-5
Mendieta-Moctezuma, A., Rugerio-Escalona, C., Villa-Ruano, N., Gutierrez, R. U., Jiménez-Montejo, F. E., Fragoso-Vázquez, M. J., et al. (2019). Synthesis and Biological Evaluation of Novel Chromonyl Enaminones as α-glucosidase Inhibitors. Med. Chem. Res. 28, 831–848. doi:10.1007/s00044-019-02320-w
Prachumrat, P., Kobkeatthawin, T., Ruanwas, P., Boonnak, N., Laphookhieo, S., Kassim, M. B., et al. (2018). Synthesis, Crystal Structure, Antioxidant, and α-Glucosidase Inhibitory Activities of Methoxy-Substituted Benzohydrazide Derivatives. Crystallogr. Rep+ 63, 405–411. doi:10.1134/s1063774518030227
Proença, C., Freitas, M., Ribeiro, D., Oliveira, E. F. T., Sousa, J. L. C., Tomé, S. M., et al. (2017). α-Glucosidase Inhibition by Flavonoids: an In Vitro and In Silico Structure-Activity Relationship Study. J. Enzyme Inhibition Med. Chem. 32, 1216–1228. doi:10.1080/14756366.2017.1368503
Proença, C., Freitas, M., Ribeiro, D., Sousa, J. L. C., Carvalho, F., Silva, A. M. S., et al. (2018). Inhibition of Protein Tyrosine Phosphatase 1B by Flavonoids: A Structure - Activity Relationship Study. Food Chem. Toxicol. 111, 474–481. doi:10.1016/j.fct.2017.11.039
Proença, C., Freitas, M., Ribeiro, D., Tomé, S. M., Oliveira, E. F. T., Viegas, M. F., et al. (2019). Evaluation of a Flavonoids Library for Inhibition of Pancreatic α-amylase towards a Structure-Activity Relationship. J. Enzyme Inhibition Med. Chem. 34, 577–588. doi:10.1080/14756366.2018.1558221
Qamar, R., Saeed, A., Saeed, M., Shah, B. H., Ashraf, Z., Abbas, Q., et al. (2018). Synthesis and Enzyme Inhibitory Kinetics of Some Novel 3-(substituted Benzoyl)-2-Thioxoimidazolidin-4-One Derivatives as α-glucosidase/α-amylase Inhibitors. Med. Chem. Res. 27, 1528–1537. doi:10.1007/s00044-018-2170-4
Rama Krishna, B., Ramakrishna, S., Rajendra, S., Madhusudana, K., and Mallavadhani, U. V. (2019). Synthesis of Some Novel Orsellinates and Lecanoric Acid Related Depsides as α-glucosidase Inhibitors. J. Asian Nat. Prod. Res. 21, 1013–1027. doi:10.1080/10286020.2018.1490274
Rocha, S., Sousa, A., Ribeiro, D., Correia, C. M., Silva, V. L. M., Santos, C. M. M., et al. (2019). A Study towards Drug Discovery for the Management of Type 2 Diabetes Mellitus through Inhibition of the Carbohydrate-Hydrolyzing Enzymes α-amylase and α-glucosidase by Chalcone Derivatives. Food Funct. 10, 5510–5520. doi:10.1039/C9FO01298B
Santos, C. M. M., Freitas, M., and Fernandes, E. (2018). A Comprehensive Review on Xanthone Derivatives as α-glucosidase Inhibitors. Eur. J. Med. Chem. 157, 1460–1479. doi:10.1016/j.ejmech.2018.07.073
Settypalli, T., Chunduri, V. R., Maddineni, A. K., Begari, N., Kotha, P., Chippada, A. R., et al. (2019). Design, Synthesis, In Silico Docking Studies and Biological Evaluation of Novel Quinoxaline-Hydrazide Hydrazone-1,2,3-Triazole Hybrids as α-glucosidase Inhibitors and Antioxidants. New J. Chem. 43, 15435–15452. doi:10.1039/c9nj02580d,
Shah, S., Arshia, , Javaid, K., Zafar, H., Mohammed Khan, K., Khalil, R., et al. (2018). Synthesis, and In Vitro and In Silico α-Glucosidase Inhibitory Studies of 5-Chloro-2-Aryl Benzo[d]thiazoles. Bioorg. Chem. 78, 269–279. doi:10.1016/j.bioorg.2018.02.013
Sonia, R., Daniela, R., Eduarda, F., and Marisa, F. (2019). Meet Our Editorial Board Member. Curr. Med. Chem. 26, 1–64. doi:10.2174/092986732601190314143611
Spasov, A. A., Babkov, D. A., Osipov, D. V., Klochkov, V. G., Prilepskaya, D. R., Demidov, M. R., et al. (2019). Synthesis, In Vitro and In Vivo Evaluation of 2-Aryl-4h-Chromene and 3-Aryl-1h-Benzo[f]chromene Derivatives as Novel α-glucosidase Inhibitors. Bioorg. Med. Chem. Lett. 29, 119–123. doi:10.1016/j.bmcl.2018.10.018
Taha, M., Imran, S., Rahim, F., Wadood, A., and Khan, K. M. (2018b). Oxindole Based Oxadiazole Hybrid Analogs: Novel α -glucosidase Inhibitors. Bioorg. Chem. 76, 273–280. doi:10.1016/j.bioorg.2017.12.001
Taha, M., Ismail, N. H., Imran, S., Wadood, A., Rahim, F., Ali, M., et al. (2015). Novel Quinoline Derivatives as Potent In Vitro α-glucosidase Inhibitors: In Silico Studies and SAR Predictions. Med. Chem. Commun. 6, 1826–1836. doi:10.1007/s00044-019-02430-510.1039/c5md00280j
Taha, M., Shah, S. A. A., Afifi, M., Imran, S., Sultan, S., Rahim, F., et al. (2018a). Synthesis, α-glucosidase Inhibition and Molecular Docking Study of Coumarin Based Derivatives. Bioorg. Chem. 77, 586–592. doi:10.1016/j.bioorg.2018.01.033
Wang, G.-c., Peng, Y.-p., Xie, Z.-z., Wang, J., and Chen, M. (2017). Synthesis, α-glucosidase Inhibition and Molecular Docking Studies of Novel Thiazolidine-2,4-Dione or Rhodanine Derivatives. Med. Chem. Commun. 8, 1477–1484. doi:10.1039/C7MD00173H
Wang, M.-Y., Cheng, X.-C., Chen, X.-B., Li, Y., Zang, L.-L., Duan, Y.-Q., et al. (2018). Synthesis and Biological Evaluation of novelN-Aryl-ω-(benzoazol-2-Yl)-Sulfanylalkanamides as Dual Inhibitors of α-glucosidase and Protein Tyrosine Phosphatase 1B. Chem. Biol. Drug Des. 92, 1647–1656. doi:10.1111/cbdd.13331
Wu, P.-P., Zhang, B.-J., Cui, X.-P., Yang, Y., Jiang, Z.-Y., Zhou, Z.-H., et al. (2017). Synthesis and Biological Evaluation of Novel Ursolic Acid Analogues as Potential α-glucosidase Inhibitors. Sci. Rep. 7, 45578. doi:10.1038/srep45578
Wu, P.-P., Zhang, K., Lu, Y.-J., He, P., and Zhao, S.-Q. (2014). In Vitro and In Vivo Evaluation of the Antidiabetic Activity of Ursolic Acid Derivatives. Eur. J. Med. Chem. 80, 502–508. doi:10.1016/j.ejmech.2014.04.073
Xu, X.-T., Mou, X.-Q., Xi, Q.-M., Liu, W.-T., Liu, W.-F., Sheng, Z.-J., et al. (2016). Anti-inflammatory Activity Effect of 2-Substituted-1,4,5,6-Tetrahydrocyclopenta[b]pyrrole on TPA-Induced Skin Inflammation in Mice. Bioorg. Med. Chem. Lett. 26, 5334–5339. doi:10.1016/j.bmcl.2016.09.034
Xu, X. T., Deng, X. Y., Chen, J., Liang, Q. M., Zhang, K., Li, D. L., et al. (2019). Synthesis and Biological Evaluation of Coumarin Derivatives as α-glucosidase Inhibitors. Eur. J. Med. Chem. 189, 112013. doi:10.1016/j.ejmech.2019.112013
Yang, C., Yu, Y., Sun, W., and Xia, C. (2014). Indole Derivatives Inhibited the Formation of Bacterial Biofilm and Modulated Ca2+ Efflux in Diatom. Mar. Pollut. Bull. 88, 62–69. doi:10.1016/j.marpolbul.2014.09.027
Ye, G.-J., Lan, T., Huang, Z.-X., Cheng, X.-N., Cai, C.-Y., Ding, S.-M., et al. (2019). Design and Synthesis of Novel Xanthone-Triazole Derivatives as Potential Antidiabetic Agents: α-Glucosidase Inhibition and Glucose Uptake Promotion. Eur. J. Med. Chem. 177, 362–373. doi:10.1016/j.ejmech.2019.05.045
Keywords: oxindole, α-glucosidase, inhibition, docking, kinetics
Citation: Lin J, Liang Q-M, Ye Y-N, Xiao D, Lu L, Li M-Y, Li J-P, Zhang Y-F, Xiong Z, Feng N and Li C (2022) Synthesis and Biological Evaluation of 5-Fluoro-2-Oxindole Derivatives as Potential α-Glucosidase Inhibitors. Front. Chem. 10:928295. doi: 10.3389/fchem.2022.928295
Received: 25 April 2022; Accepted: 09 May 2022;
Published: 23 June 2022.
Edited by:
Shao-Hua Wang, Lanzhou University, ChinaReviewed by:
Wenneng Wu, Guiyang University, ChinaDanying Huang, Guangdong University of Petrochemical Technology, China
Copyright © 2022 Lin, Liang, Ye, Xiao, Lu, Li, Li, Zhang, Xiong, Feng and Li. This is an open-access article distributed under the terms of the Creative Commons Attribution License (CC BY). The use, distribution or reproduction in other forums is permitted, provided the original author(s) and the copyright owner(s) are credited and that the original publication in this journal is cited, in accordance with accepted academic practice. No use, distribution or reproduction is permitted which does not comply with these terms.
*Correspondence: Zhuang Xiong, d3l1Y2hlbXh6QDEyNi5jb20=; Na Feng, d3l1Y2hlbWZuQDEyNi5jb20=; Chen Li, d3l1Y2hlbWxjQDEyNi5jb20=
†These authors have contributed equally to this work