- 1State Key Laboratory Breeding Base of Green Pesticide and Agricultural Bioengineering, Key Laboratory of Green Pesticide and Agricultural Bioengineering, Ministry of Education, Guizhou University, Guiyang, China
- 2Guizhou Rice Research Institute, Guizhou Academy of Agricultural Sciences, Guiyang, China
Plant virus diseases, also known as “plant cancers”, cause serious harm to the agriculture of the world and huge economic losses every year. Antiviral agents are one of the most effective ways to control plant virus diseases. Ningnanmycin is currently the most successful anti-plant virus agent, but its field control effect is not ideal due to its instability. In recent years, great progress has been made in the research and development of antiviral agents, the mainstream research direction is to obtain antiviral agents or lead compounds based on structural modification of natural products. However, no antiviral agent has been able to completely inhibit plant viruses. Therefore, the development of highly effective antiviral agents still faces enormous challenges. Therefore, we reviewed the recent research progress of anti-plant virus agents based on natural products in the past decade, and discussed their structure-activity relationship (SAR) and mechanism of action. It is hoped that this review can provide new inspiration for the discovery and mechanism of action of novel antiviral agents.
1 Introduction
Plant viruses are a serious threat to the safe production of world agriculture, causing global economic losses as high as $60 billion every year (Bos, 2000; Barna et al., 2003; Zhao et al., 2017a). Tobacco mosaic virus (TMV), tomato spotted wilt virus (TSWV), tomato yellow leaf curl virus (TYLCV), cucumber mosaic virus (CMV), potato virus Y (PVY) are the top five most important plant viruses of the world according to science/economic importance (Scholthof et al., 2011). TMV is one of the oldest known plant viruses and ranks first among the top 10 plant viruses, causing economic losses in excess of $100 million per year. The host range of TMV exceeds 400 species, and TMV may alter the metabolism and impair the defense system of hosts (Silverman et al., 2005; Scholthof et al., 2011; Sharma et al., 2021). After the plant virus invades the host, the substances required for the life process are completely dependent on the host, and its replication may be combined with the metabolism of the host, making it difficult to prevent and control the viral diseases (Rodrigues et al., 2016; Wang D. et al., 2021). There is no antiviral agent that can completely inhibit plant viruses, and the development of high-efficiency antiviral agents still faces huge challenges (Gan et al., 2021).
Natural products have long been regarded as a source of inspiration for drug design, providing many unknown chemical scaffolds and pharmacophores (Eschenbrenner-Lux et al., 2014). In addition, natural products readily interact with biological targets, thereby exhibiting specific biological activities (Lowe, 2014; Bauer and Brӧnstrup, 2014). Identifying natural product structure and studying biological activity are of great significance for drug discovery (Chen and Song, 2021; Della-Felice et al., 2022). The discovery of anti-plant virus agents based on natural products is an important research direction in the prevention and control of plant virus diseases and has always attracted much attention (Eckert et al., 2003; Carli et al., 2012; Katayama et al., 2013; Wang et al., 2015). Ningnamycin (Figure 1A), isolated from Strepcomces noursei var xichangensisn for the first time, has broad-spectrum and excellent antiviral activity and is currently the most successful antiviral agent, playing a huge role in the control of plant virus diseases (Han et al., 2014). Ningnanmycin promotes the accumulation of pathogen-related proteins (PRs), a marker of systemic acquired resistance (SAR), by inhibiting the polymerization process of TMV coat protein (TMV-CP) (Han et al., 2014). In addition, ningnamycin can activate redox and metabolic processes in CMV-infected tobacco (Gao et al., 2019).
In recent years, great progress has been made in the research and development of anti-plant virus agents (Carli et al., 2010; Li and Song, 2017; Park et al., 2021). The discovery of some new antiviral agents based on natural products (Figure 1B) not only reflects the important role of natural products in the discovery of antiviral agents, but also provides great help for the control of plant viruses. Special natural molecular scaffolds can serve as bridges for the derivatization of antiviral agents (Jassbi et al., 2017; Chen J. et al., 2020), which can provide innovative solutions for the discovery of novel antiviral agents. We wish to analyze the research progress of chemical antiviral agents based on natural scaffold. However, most of these references come from China in recent 10 years. From the perspective of natural products, we reviewed the latest research progress of anti-plant virus chemical active compounds in recent years and discussed their anti-viral activity, structure-activity relationship and mechanism of action, aiming to provide new insights for the discovery of new anti-viral agents.
2 Antiviral Active Compounds
2.1 Acids
2.2.1 Fatty Acids or Carboxylic Acids
Some natural fatty acids or carboxylic acids have good anti-plant virus activity (Katayama et al., 2013; Deshoux et al., 2020). For example, compound 1 (Figure 2), isolated from cottonseed sludge was able to increase the phenylalanine ammonia lyase (PAL) and peroxidase (POD) activities of tobacco, as well as the expression levels of PR-1a and PR-5 genes. Its anti-plant virus activity may be related to the expression and activation of various defense-related genes in tobacco (Zhao et al., 2017b). Compound 2, a derivative of the marine natural product essramycin, exhibited 62, 64, and 68% of TMV inactivating, curative, and protective activities at 500 mg/L, respectively. Compound 2 showed antiviral activity by inhibiting viral assembly and promoting aggregation of 20S disk proteins (Wang T. et al., 2020).
2.2.2 Ferulic Acid Derivatives
Ferulic acid is widely found in plants, and its derivatives have broad-spectrum biological activities (Sonar et al., 2019; Boulebd et al., 2022). Ferulic acid derivatives have good performance in antiviral activity. For example, compound 3 (Figure 3) has EC50 values of 135.5 and 178.6 mg/L for TMV and CMV. Compound 3 can significantly alter the levels of tobacco gene transcription and protein expression, and enhance the defense response of tobacco by inducing the accumulation of secondary metabolites in the biosynthetic pathway of tobacco phenylpropanoid, thereby inhibiting virus infection (Gan et al., 2021). At a concentration of 500 mg/L, the curative, protective and inactivating activities of compound 4 against TMV were 62.5, 61.8 and 83.5%, respectively. Compound 4 is not only able to cause the breaking and bending of TMV, but also has a strong binding force on TMV-CP (Wang Y. et al., 2020). The EC50 values for the curative and protective activity of compound 5 against CMV were 284.67 and 216.30 mg/L, respectively (Lan et al., 2017). The EC50 value of compound 6 for TMV inactivating activity was 36.59 mg/L (Wang et al., 2017). The derivatization of ferulic acid is mainly phenolic hydroxyl and carboxyl moieties. In the phenolic hydroxyl part, benzyl, alkyl, and carbonyl groups are mainly introduced for derivatization, and in the carboxyl part, new ferulic acid derivatives are synthesized mainly through esterification, amidation and acylhydrazone.
2.2 Ketones
2.2.1 Chalcone Derivatives
Chalcone derivatives showed good antiviral activity (Sinha, et al., 2019). For example, compound 7 (Figure 4) showed 55.6, 71.2 and 92.4% of curative, protective, and inactivating activities against TMV, respectively. Compound 7 induced plant tolerance to mosaic virus by enhancing tobacco defense enzyme activity, chlorophyll content, and photosynthesis (Gan et al., 2017a). Compounds 8 and 9 not only have good passivation activities (EC50, 51.65 and 30.57 mg/L) for TMV, but also have a strong binding ability to TMV-CP (Gan et al., 2017b; Zhou et al., 2018). Compound 10 has good curative and protective activities against TMV (57.6 and 59.9%), which may trigger the breakdown of TMV by directly interacting with TMV, while also inducing plant resistance (Zhou et al., 2021). The derivatization direction of chalcone is mainly two benzene rings. The benzene ring connected to the carbonyl group mainly introduces halogen, alkoxy, alkyl, and aryl ether, while the benzene ring connected to the double bond mainly introduces halogen, alkoxy, benzyl, aryl ether and ester groups.
2.2.2 Pentadienone Derivatives
There have been many reports on the antiviral activity of pentadienone derivatives. For example, compound 11 (Figure 5) has an EC50 value of 52.9 mg/L for TMV inactivation activity and has a micromolar affinity for TMV-CP (Chen et al., 2015). At 500 mg/L, the in vivo curative activity of compound 12 against TMV and CMV was 48.2 and 59.84%, respectively, and the inactivation activity was 82.6 and 89.8%, respectively (Han et al., 2015). The EC50 values of the protective activity against TMV and the curative activity against CMV of compound 13 were 124.3 and 365.5 mg/L, respectively (Long et al., 2015). At a concentration of 500 mg/L, the curative and protective activities of compound 14 against TMV were 52.6 and 55.4%, respectively (Wu et al., 2016). Compound 15 has a strong binding affinity to CMV-CP with a dissociation constant of 0.071 μΜ (Li et al., 2019). The EC50 value of compound 16 for TMV curative activity was 132.2 mg/L (Ma et al., 2014). The EC50 values of compound 17 for the curative, protective and inactivating of TMV were 441.3, 364.6, 243.3 mg/L, and the EC50 values for the curative, protective and inactivating activity of CMV were 533.6, 490.7 and 471.6 mg/L, respectively (Luo et al., 2013). The backbone structure of 1,4-pentadien-3-one was obtained by curcumin derivatization. The modification of the 1,4-pentadien-3-one structure is mainly at the terminal positions of the two olefinic bonds. If one of the positions is a benzene ring, halogen, alkoxy, benzyl, and ester groups are mainly introduced into the benzene ring. And another position can be benzene ring, thiophene, furan, and pyridine ring, and mainly halogen is introduced in the ring.
2.2.3 Quinazolinone Derivatives
Quinazolinone is the backbone structure of many alkaloids, and its derivatives have good anti-plant virus activity. For example, compound 18 (Figure 6) not only exhibited good curative activity against CMV (EC50, 146.30 mg/L), but also had micromolar binding to CMV-CP (Chen L. et al., 2016). Compounds 19 and 20 have a strong binding ability to tomato chlorosis virus coat protein (ToCV-CP), and the relative expression of ToCV-CP gene in tomato was decreased by 93.3 and 81.0%, respectively (Ran et al., 2020; Zu et al., 2020). Compound 21 not only has good inactivating activity against TSWV (EC50, 188 mg/L), but also has a strong binding force to TSWV coat protein (Liu et al., 2021). At 500 mg/L, the inactivating, curative and protective activities of compound 22 against TMV were 51, 43 and 54%, respectively. In addition, compound 22 may show excellent antiviral activity by preventing viral assembly (Hao et al., 2020). Halogen, CF3, and alkyl are mainly introduced into the benzene ring of quinazolinone. The two- and 3-positions of quinazoline are mainly introduced into thioether, ether and benzene rings, while between the two- and 3-positions, new heterocycles can be obtained by cyclization.
2.2.4 Chromone Derivatives
Chromones, which are widely present in plants, have good antiviral activity. For example, compound 23 (Figure 7A) not only has good binding ability to ToCV-CP, but also reduces the relative expression of ToCV-CP gene by 67.2% (Jiang et al., 2021). The curative and protective EC50 values of compound 24 against TSWV were 124.2 and 109.3 gm/L, respectively. It may exert antiviral activity by blocking the binding of TSWV N to viral RNA (Zan et al., 2021). At a concentration of 500 mg/L, compound 25 exhibited good curative, protective and inactivating activities against TMV, which were 68.8, 58.8, and 86.0%, respectively. It may exert antiviral activity by disrupting the phenotype and integrity of TMV (Li et al., 2020). Halogen, CF3, alkyl and benzyl are mainly introduced into the benzene ring of chromone. The second-flow acetal was mainly introduced at the 3-position of the chromone, and the oxygen-containing flexible chain and the olefinic bond were derivatized.
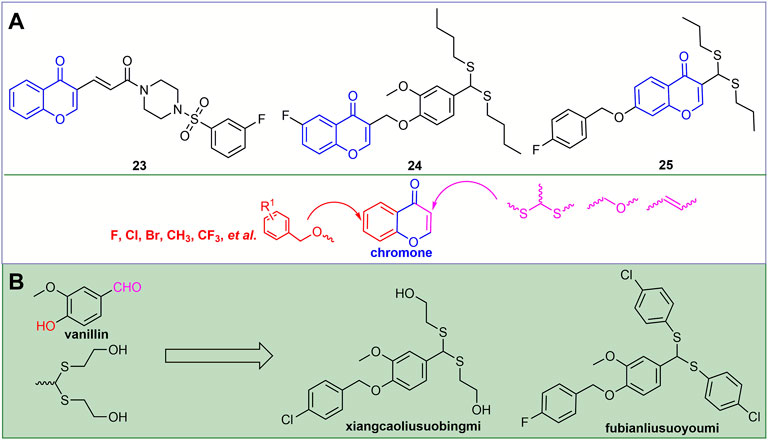
FIGURE 7. Structures of representative chromone derivatives in antiviral activities (A). Discovery of novel antiviral agents xiangcaoliusuobingmi and fubianliusuoyoumi (B).
2.3 Vanillin Derivatives
Vanillin is a natural fragrance with a strong aroma and is widely used in the production of cosmetics and fragrances (Libardi et al., 2011; Kayaci and Uyar, 2011). For example, the discovery of novel antiviral agents xiangcaoliusuobingmi and fubianliusuoyoumi (Figure 7B) benefits from this. The EC50 values of xiangcaoliusuobingmi for the curative and protective activities against PVY were 217.6 and 205.7 mg/L, respectively, and the EC50 values for the curative and protective activities against CMV were 206.3 and 186.2 mg/L, respectively (Zhang et al., 2017). In addition, it can regulate the expression of defense genes and increase the activity of defense enzymes to exert antiviral activity (Shi et al., 2018).
The EC50 for the curative, protective and inactivating activities of compound 26 (Figure 8) against TMV were 329.5, 269.2 and 48.1 mg/L (Yang Y. et al., 2020). The curative, protective and inactivating activities of compound 27 against TMV were 50.9, 58.9 and 81.8%, respectively. Compound 27 can not only destroy the morphology of TMV particles, but also has a strong binding effect with TMV-CP (Wang et al., 2019). At 500 mg/L, compound 28 exhibited 51.8 and 90.1% of the curative and inactivating activities against TMV, respectively, and it was able to hinder the self-assembly of TMV (Luo et al., 2020). Compound 29 inhibited ToCV infection in the host and decreased the expression level of ToCV-mCP gene (Yang H. et al., 2020). Compound 30 has good curative and protective activities against PVY, CMV and TMV, and can improve the resistance of tobacco to viruses (Chen et al., 2018).
The curative, protective and inactivating activities of compound 31 against TMV were 62.1, 54.5 and 94.2%, respectively, and it could disrupt the structure of TMV particles, thereby inhibiting virus infection (Zhao et al., 2020a; Zan et al., 2020). The modification sites of vanillin are mainly hydroxyl and aldehyde groups, and benzyl, alkoxy, imino, heterocyclic and carbonyl groups are mainly introduced into the hydroxyl part, while the aldehyde groups are mainly changed to dithioacetal and carbonyl. Among them, the antiviral activity was significantly improved after the aldehyde group was changed to dithioacetal, and the chain length and substituent of the acetal also significantly affected the antiviral activity of the compounds.
2.4 Indole Derivatives
Indole is the core backbone structure of many alkaloids and is widely used in the discovery of pesticides (Çokuğraş and Bodur, 2013; Ji et al., 2016; Rajasekharan et al., 2020; Dong et al., 2020). The inactivating, curative and protective activities of compound 33 against TMV were 54, 50, and 53%, respectively, and it may exert antiviral activity by preventing the movement of the virus in plants (Figure 9) (Guo et al., 2019). Compound 35 may show good anti-TMV activity by inhibiting the assembly of viral particles and the aggregation of 20S CP (Kang et al., 2020). The antiviral activity of compound 39 against TMV, CMV, and PVY was associated with an increase in chlorophyll content and defense-related enzyme activity (Wei et al., 2019). Antiviral activity of other representative indole derivatives is shown in Table 1. Halogen, alkyl and CF3 are mainly introduced into the benzene ring of indole. Benzyl, alkyl and sulfonyl groups are mainly introduced on the N atom. At the 3-position, heterocycle, benzene ring, dithioacetal, carbonyl and alkyl are mainly introduced for derivatization.
The inactivating, curative, and protective activities of the marine natural product debromohamacanthin A (Figure 10A) against TMV were 53, 51 and 56%, respectively. The inactivating, curative, and protective activities of its derivative 41 against TMV were 60, 59, and 63%, respectively, and the antiviral activity of the compound was improved through structural optimization (Wang T. et al., 2021). In addition, compound 41 can bind to TMV-CP and interfere with the assembly process of TMV-CP and RNA, thus showing antiviral resistance. The inactivating, curative, and protective activities of compound 42 (Figure 10B) against TMV were 51.2, 49.0, and 53.6%, respectively (Wang et al., 2022). The functional groups containing CF2, indole or cyano favored the antiviral activity of 3,3-helix cyclic indole derivatives. At 500 mg/L, the curative, protective, and inactivating activities of compound 43 against TMV were 47, 50, and 51%, respectively (Chen L. et al., 2020). At 500 mg/L, the inactivating, curative, and protective activities of compound 44 were 58, 55.2, and 49.7%, respectively (Chen M. et al., 2016).
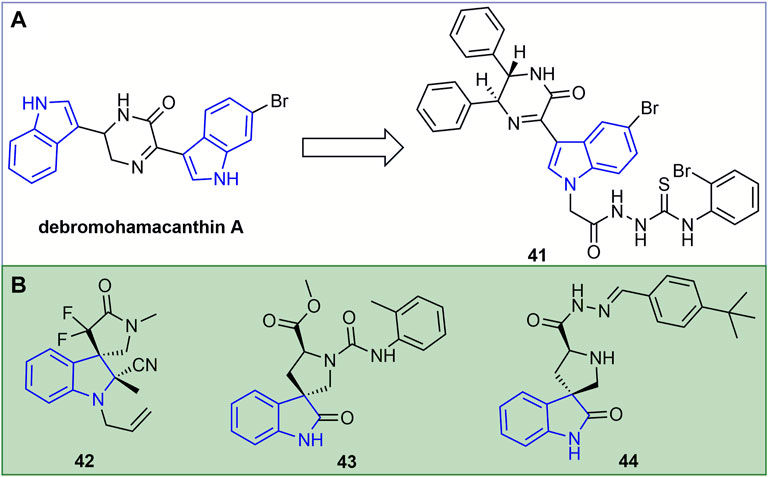
FIGURE 10. Structures of representative of debromohamacanthin A derivatives in antiviral activities (A). Structures of representative of indole spirocycles derivatives in antiviral activities (B).
A novel antiviral agent, chloroinconazide (Figure 11A), was discovered based on the natural product harmine. Its inactivating, curative, and protective activities at 500 mg/L against TMV were 70.4, 71.5, and 64.2%, respectively (Liu et al., 2014). Chloroinconazide can also activate reactive oxygen species and antioxidant levels, induce an increase in salicylic acid content and the expression of its response gene PR2 (Lv et al., 2021). Furthermore, it can attenuate the virulence of TMV by directly changing the morphological structure of the virion and increasing the activity of antioxidant enzymes, thereby reducing the production of TMV-induced reactive oxygen species (ROS) during plant infection (Lv et al., 2020).
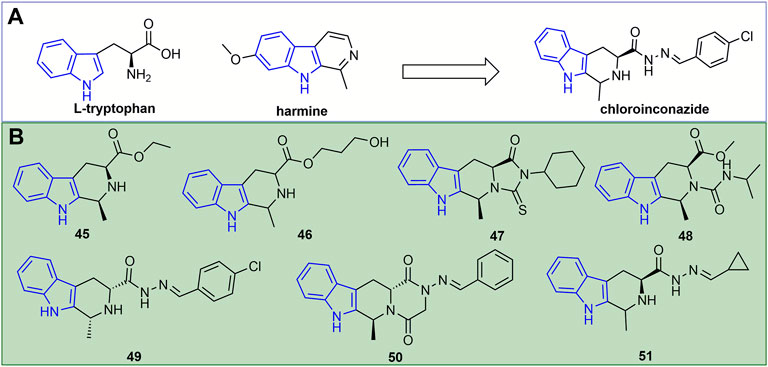
FIGURE 11. Discovery of a novel antiviral agent chloroinconazide (A). Structures of representative indole derivatives in antiviral activities (B).
At 500 mg/L, the inactivating, curative, and protective activities of compound 46 (Figure 11B) against TMV were 50.4, 43.9, and 47.9% (Song H. et al., 2014). The in vitro antiviral activity of compound 47 against TMV was 48.2% (Song H.-j. et al., 2014). The anti-TMV inactivating, curative, and protective activities of compound 48 were 59, 63 and 60% at 500 mg/L, respectively (Huang et al., 2018). Compound 49 showed excellent inactivating, curative, and protective activities against TMV with EC50 values of 127, 156, and 108 mg/L, respectively (Wang and Song, 2020c). Compound 50 not only retarded TMV proliferation, but also had a concentration-dependent effect on tobacco growth and biomass accumulation (Zhang X. et al., 2021). The inactivating, curative, and protective activities of compound 51 against TMV were 49, 50 and 52%, respectively (Xie et al., 2020).
2.5 Tylophorine Derivatives
Tylophorine has good antiviral activity, and there have been many reports on the antiviral activity of its derivatives (Figure 12) (Wang et al., 2010a). For example, the inactivating, curative, and protective activities of compound 52 against TMV were 78.1, 80.1 and 88.4%, respectively. The planarity of the molecule and the rigidity of the D-ring also have a strong effect on the activity, suggesting that the three-dimensional conformation is also very important for enhanced biological activity (Su et al., 2016). At 500 mg/L, the inactivating, curative, and protective activities of compound 53 against TMV were 67.7, 65.3, and 65.9%, respectively, and its EC50 value was 296 mg/L (Yan et al., 2021). The inactivating, curative and protective activities of compound 54 against TMV were 75.3, 76.2 and 68.4% at 500 mg/L, respectively. The methoxy group on the phenanthrene unit significantly affects the antiviral activity of the compounds (Su et al., 2014a). Antiviral activity of other representative tylophorine analogues is shown in Table 2.
2.6 Purine Nucleoside Derivatives
Purine nucleosides have excellent antiviral activity, and the curative and protective activities of its derivative 68 (Figure 13) against PVY and CMV were 52.5, 60.0, 60.2%, respectively. The excellent antiviral activity of compound 68 is related to its immune-inducing effect, which can regulate the activities of defense-related enzymes, defense-related genes, and photosynthesis-related proteins in plants (He et al., 2019). The EC50 values of the protective activity of compound 69 against CMV and PVY were 137 and 209 mg/L, respectively. The EC50 value of compound 69 for the inactivating activity of TMV was 48 mg/L. Compound 69 may further damage the viral structure TMV virus by binding to the coat protein of the virus, thus weakening its infectivity and infectivity (Zhang J. et al., 2021).
2.7 Esters or Lactones Derivatives
Esters or lactones have made great progress in the study of antiviral activity, which has attracted the attention of researchers (Olivon et al., 2015; Eriksson et al., 2008; Hellwig et al., 2003). At 500 mg/L, the inactivating, curative, and protective activities of compound 70 (Figure 14) were 52, 57, and 56%, respectively (Lu et al., 2014). The curative activity of compound 71 against TMV was 62.86% at 100 mg/L, and it could inhibit TMV infection by interfering with the expression of TMV-CP (Zhao et al., 2017c). Compound 72 inhibits the expression of tobacco TMV-CP with an IC50 value of 5.56 μM (Tan et al., 2018; Yan et al., 2010). Compound 73 has obvious inhibitory activity against TMV infection and replication with IC50 of 13.98 and 7.13 mg/L, respectively (Shen et al., 2008). Compound 74 inhibited gene expression of TSWV by more than 85%. Compound 74 activates the JA pathway, promotes PAL activity, induces systemic resistance, inhibits gene expression of TSWV, and defends against TSWV infection (Zhao et al., 2020b). Antiviral activity of other representative ester or lactone derivatives are shown in Table 3.
2.8 Berberine Analogs
The protective activity of berberine (Figure 15) against TMV was 62.8%. Berberine induces an immune response to TMV in tobacco and associated with systemic resistance through activation of salicylic acid signaling (Guo et al., 2020). At 500 mg/L, chelerythrine had obvious inactivation, proliferation inhibition, and protection effects on TMV, and the inhibition ratio were 72.67, 77.52, and 59.34%, respectively. (Guo et al., 2021).
3 Conclusion
In recent years, great progress has been made in the research and development of antiviral agents. The discovery of some new antiviral agents has provided more options for the prevention and control of plant virus diseases. These new antiviral agents are expected to become pillar products in the future. These novel antiviral agents are obtained by structural modification of natural products as lead compounds. In the past 10 years, some important natural products or backbone structures based on natural products in the research and development of anti-plant virus agents are mainly acids (fatty acids, carboxylic acids, and ferulic acids), ketones (chalcones, pentadienones, quinazolinones, and chromones), vanilloids, indoles, silmenines, purine nucleosides, esters or lactones, and berberine analogs. Among them, the derivatives based on vanillin and indole show great application prospects. For example, xiangcaoliusuobingmi and fubianliusuoyoumi are novel antiviral agents based on vanillin, and chloroinconazide is a novel antiviral agent based on indole. Currently, the discovery methods of anti-plant viral agents mainly include natural product isolation, natural product-based structural modification, protein-based structural design, and computational-based structural optimization. The research on the mechanism of action of antiviral agents mainly focuses on the relationship between drugs and RNA, proteins, and pathways, and the in-depth mechanism of action in living host plants needs to be further explored. The design of new scaffolds and lead compounds inspired by natural products has played an important role in the development of antiviral agents and has been demonstrated in practice. With the continuous discovery of new natural products, more anti-plant virus agents based on natural products will be discovered and applied in the future.
Author Contributions
JC: conceived and designed the research, JC and XL: wrote the manuscript, YC, YW, JP, and ZX: analyzed and interpreted the data.
Funding
This work was supported by the National Key R & D Program of China (2021YFD1400800), the Natural Science Foundation of Guizhou Province (QKHJC-ZK(2022)039), the Natural Science Foundation of Guizhou University (NO. (2021)01), the Cultivation Project of Guizhou University (NO. (2020)06).
Conflict of Interest
The authors declare that the research was conducted in the absence of any commercial or financial relationships that could be construed as a potential conflict of interest.
Publisher’s Note
All claims expressed in this article are solely those of the authors and do not necessarily represent those of their affiliated organizations, or those of the publisher, the editors and the reviewers. Any product that may be evaluated in this article, or claim that may be made by its manufacturer, is not guaranteed or endorsed by the publisher.
References
Barna, B., Fodor, J., Pogány, M., and Király, Z. (2003). Role of Reactive Oxygen Species and Antioxidants in Plant Disease Resistance. Pest. Manag. Sci. 59, 459–464. doi:10.1002/ps.706
Bauer, A., and Brönstrup, M. (2014). Industrial Natural Product Chemistry for Drug Discovery and Development. Nat. Prod. Rep. 31, 35–60. doi:10.1039/c3np70058e
Bos, L. (2000). 100 Years of Virology: From Vitalism via Molecular Biology to Genetic Engineering. Trends Microbiol. 8, 82–87. doi:10.1016/s0966-842x(99)01678-9
Boulebd, H., Mechler, A., Thi Hoa, N., and Vo, Q. V. (2022). Insights on the Kinetics and Mechanisms of the Peroxyl Radical Scavenging Capacity of Caftaric Acid: the Important Role of the Acid-Base Equilibrium. New J. Chem. 46, 7403–7409. doi:10.1039/d2nj00377e
Chen, J.-x., and Song, B.-a. (2021). Natural Nematicidal Active Compounds: Recent Research Progress and Outlook. J. Integr. Agric. 20, 2015–2031. doi:10.1016/s2095-3119(21)63617-1
Chen, J., Li, Q. X., and Song, B. (2020a). Chemical Nematicides: Recent Research Progress and Outlook. J. Agric. Food Chem. 68, 12175–12188. doi:10.1021/acs.jafc.0c02871
Chen, J., Shi, J., Yu, L., Liu, D., Gan, X., Song, B., et al. (2018). Design, Synthesis, Antiviral Bioactivity, and Defense Mechanisms of Novel Dithioacetal Derivatives Bearing a Strobilurin Moiety. J. Agric. Food Chem. 66, 5335–5345. doi:10.1021/acs.jafc.8b01297
Chen, L., Hao, Y., Song, H., Liu, Y., Li, Y., Zhang, J., et al. (2020b). Design, Synthesis, Characterization, and Biological Activities of Novel Spirooxindole Analogues Containing Hydantoin, Thiohydantoin, Urea, and Thiourea Moieties. J. Agric. Food Chem. 68, 10618–10625. doi:10.1021/acs.jafc.0c04488
Chen, L., Xie, J., Song, H., Liu, Y., Gu, Y., Wang, L., et al. (2016a). Design, Synthesis, and Biological Activities of Spirooxindoles Containing Acylhydrazone Fragment Derivatives Based on the Biosynthesis of Alkaloids Derived from Tryptophan. J. Agric. Food Chem. 64, 6508–6516. doi:10.1021/acs.jafc.6b02683
Chen, M., Hu, D., Li, X., Yang, S., Zhang, W., Li, P., et al. (2015). Antiviral Activity and Interaction Mechanisms Study of Novel Glucopyranoside Derivatives. Bioorg. Med. Chem. Lett. 25, 3840–3844. doi:10.1016/j.bmcl.2015.07.068
Chen, M., Li, P., Hu, D., Zeng, S., Li, T., Jin, L., et al. (2016b). Synthesis, Antiviral Activity, 3D-QSAR, and Interaction Mechanisms Study of Novel Malonate Derivatives Containing Quinazolin-4(3h)-One Moiety. Bioorg. Med. Chem. Lett. 26, 168–173. doi:10.1016/j.bmcl.2015.11.006
Chen, Y. H., Guo, D. S., Lu, M. H., Yue, J. Y., Liu, Y., Shang, C. M., et al. (2020c). Inhibitory Effect of Osthole from Cnidium Monnieri on Tobacco Mosaic Virus (TMV) Infection in Nicotiana Glutinosa. Molecules 25, 65. doi:10.3390/molecules25010065
Çokuğraş, A. N., and Bodur, E. (2013). Comparative Effects of Two Plant Growth Regulators; Indole-3-Acetic Acid and Chlorogenic Acid on Human and Horse Serum Butyrylcholinesterase. Pestic. Biochem. Physiol. 77, 24–33. doi:10.1016/S0048-3575(03)00071-3
Cooper, B., Eckert, D., Andon, N. L., Yates, J. R., and Haynes, P. A. (2003). Investigative Proteomics: Identification of an Unknown Plant Virus from Infected Plants Using Mass Spectrometry. J. Am. Soc. Mass Spectrom. 14, 736–741. doi:10.1016/S1044-0305(03)00125-9
Della-Felice, F., Bartolomeu, A. A., and Pilli, R. A. (2022). The Phosphate Ester Group in Secondary Metabolites. Nat. Prod. Rep. doi:10.1039/d1np00078k
Deshoux, M., Masson, V., Arafah, K., Voisin, S., Guschinskaya, N., van Munster, M., et al. (2020). Cuticular Structure Proteomics in the Pea Aphid Acyrthosiphon Pisum Reveals New Plant Virus Receptor Candidates at the Tip of Maxillary Stylets. J. Proteome Res. 19, 1319–1337. doi:10.1021/acs.jproteome.9b00851
Dong, J., Huang, S. S., Hao, Y. N., Wang, Z. W., Liu, Y. X., Li, Y. Q., et al. (2020). Marine‐natural‐products for Biocides Development: First Discovery of Meridianin Alkaloids as Antiviral and Anti‐phytopathogenic‐fungus Agents. Pest Manag. Sci. 76, 3369–3376. doi:10.1002/ps.5690
Eriksson, U., Peterson, L. W., Kashemirov, B. A., Hilfinger, J. M., Drach, J. C., Borysko, K. Z., et al. (2008). Serine Peptide Phosphoester Prodrugs of Cyclic Cidofovir: Synthesis, Transport, and Antiviral Activity. Mol. Pharm. 5, 598–609. doi:10.1021/mp8000099
Eschenbrenner-Lux, V., Küchler, P., Ziegler, S., Kumar, K., and Waldmann, H. (2014). An Enantioselective Inverse-Electron-Demand Imino Diels-Alder Reaction. Angew. Chem. Int. Ed. 53, 2134–2137. doi:10.1002/anie.201309022
Gan, X., Hu, D., Chen, Z., Wang, Y., and Song, B. (2017b). Synthesis and Antiviral Evaluation of Novel 1,3,4-Oxadiazole/thiadiazole-Chalcone Conjugates. Bioorg. Med. Chem. Lett. 27, 4298–4301. doi:10.1016/j.bmcl.2017.08.038
Gan, X., Hu, D., Wang, Y., Yu, L., and Song, B. (2017a). Novel Trans-ferulic Acid Derivatives Containing a Chalcone Moiety as Potential Activator for Plant Resistance Induction. J. Agric. Food Chem. 65, 4367–4377. doi:10.1021/acs.jafc.7b00958
Gan, X., Wang, Z., and Hu, D. (2021). Synthesis of Novel Antiviral Ferulic Acid-Eugenol and Isoeugenol Hybrids Using Various Link Reactions. J. Agric. Food Chem. 69, 13724–13733. doi:10.1021/acs.jafc.1c05521
Gao, D., Wang, D., Chen, K., Huang, M., Xie, X., and Li, X. (2019). Activation of Biochemical Factors in CMV-Infected Tobacco by Ningnanmycin. Pesticide Biochem. Physiology 156, 116–122. doi:10.1016/j.pestbp.2019.02.012
Ge, Y.-h., Liu, K.-x., Zhang, J.-x., Mu, S.-z., and Hao, X.-j. (2012). The Limonoids and Their Antitobacco Mosaic Virus (TMV) Activities from Munronia Unifoliolata Oliv. J. Agric. Food Chem. 60, 4289–4295. doi:10.1021/jf205362d
Guo, J., Hao, Y., Ji, X., Wang, Z., Liu, Y., Ma, D., et al. (2019). Optimization, Structure-Activity Relationship, and Mode of Action of Nortopsentin Analogues Containing Thiazole and Oxazole Moieties. J. Agric. Food Chem. 67, 10018–10031. doi:10.1021/acs.jafc.9b04093
Guo, W., Lu, X., Liu, B., Yan, H., and Feng, J. (2021). Anti‐TMV Activity and Mode of Action of Three Alkaloids Isolated from Chelidonium Majus. Pest Manag. Sci. 77, 510–517. doi:10.1002/ps.6049
Guo, W., Yan, H., Ren, X., Tang, R., Sun, Y., Wang, Y., et al. (2020). Berberine Induces Resistance against Tobacco Mosaic Virus in Tobacco. Pest Manag. Sci. 76, 1804–1813. doi:10.1002/ps.5709
Han, G., Chen, L., Wang, Q., Wu, M., Liu, Y., and Wang, Q. (2018). Design, Synthesis, and Antitobacco Mosaic Virus Activity of Water-Soluble Chiral Quaternary Ammonium Salts of Phenanthroindolizidines Alkaloids. J. Agric. Food Chem. 66, 780–788. doi:10.1021/acs.jafc.7b03418
Han, Y., Ding, Y., Xie, D., Hu, D., Li, P., Li, X., et al. (2015). Design, Synthesis, and Antiviral Activity of Novel Rutin Derivatives Containing 1, 4-Pentadien-3-One Moiety. Eur. J. Med. Chem. 92, 732–737. doi:10.1016/j.ejmech.2015.01.017
Han, Y., Luo, Y., Qin, S., Xi, L., Wan, B., and Du, L. (2014). Induction of Systemic Resistance against Tobacco Mosaic Virus by Ningnanmycin in Tobacco. Pesticide Biochem. Physiology 111, 14–18. doi:10.1016/j.pestbp.2014.04.008
Hao, Y., Wang, K., Wang, Z., Liu, Y., Ma, D., and Wang, Q. (2020). Luotonin A and its Derivatives as Novel Antiviral and Antiphytopathogenic Fungus Agents. J. Agric. Food Chem. 68, 8764–8773. doi:10.1021/acs.jafc.0c04278
He, F., Shi, J., Wang, Y., Wang, S., Chen, J., Gan, X., et al. (2019). Synthesis, Antiviral Activity, and Mechanisms of Purine Nucleoside Derivatives Containing a Sulfonamide Moiety. J. Agric. Food Chem. 67, 8459–8467. doi:10.1021/acs.jafc.9b02681
Hellwig, V., Mayer-Bartschmid, A., Müller, H., Greif, G., Kleymann, G., Zitzmann, W., et al. (2003). Pochonins A−F, New Antiviral and Antiparasitic Resorcylic Acid Lactones from Pochonia Chlamydosporia Var. Catenulata. J. Nat. Prod. 66, 829–837. doi:10.1021/np020556v
Huang, X., Li, T., Shan, X., Lu, R., Hao, M., Lv, M., et al. (2020). High Value-Added Use of Citrus Industrial Wastes in Agriculture: Semisynthesis and Anti-tobacco Mosaic Virus/Insecticidal Activities of Ester Derivatives of Limonin Modified in the B Ring. J. Agric. Food Chem. 68, 12241–12251. doi:10.1021/acs.jafc.0c05588
Huang, Y., Guo, Z., Song, H., Liu, Y., Wang, L., and Wang, Q. (2018). Design, Synthesis, and Biological Activity of β-Carboline Analogues Containing Hydantoin, Thiohydantoin, and Urea Moieties. J. Agric. Food Chem. 66, 8253–8261. doi:10.1021/acs.jafc.8b03087
Jassbi, A. R., Zare, S., Asadollahi, M., and Schuman, M. C. (2017). Ecological Roles and Biological Activities of Specialized Metabolites from the Genus Nicotiana. Chem. Rev. 117, 12227–12280. doi:10.1021/acs.chemrev.7b00001
Ji, X., Guo, J., Liu, Y., Lu, A., Wang, Z., Li, Y., et al. (2018). Marine-Natural-Product Development: First Discovery of Nortopsentin Alkaloids as Novel Antiviral, Anti-phytopathogenic-fungus, and Insecticidal Agents. J. Agric. Food Chem. 66, 4062–4072. doi:10.1021/acs.jafc.8b00507
Ji, X., Wang, Z., Dong, J., Liu, Y., Lu, A., and Wang, Q. (2016). Discovery of Topsentin Alkaloids and Their Derivatives as Novel Antiviral and Anti-phytopathogenic Fungus Agents. J. Agric. Food Chem. 64, 9143–9151. doi:10.1021/acs.jafc.6b04020
Jiang, D., Chen, J., Zan, N., Li, C., Hu, D., and Song, B. (2021). Discovery of Novel Chromone Derivatives Containing a Sulfonamide Moiety as Anti-ToCV Agents through the Tomato Chlorosis Virus Coat Protein-Oriented Screening Method. J. Agric. Food Chem. 69, 12126–12134. doi:10.1021/acs.jafc.1c02467
Kang, J., Gao, Y., Zhang, M., Ding, X., Wang, Z., Ma, D., et al. (2020). Streptindole and its Derivatives as Novel Antiviral and Anti-phytopathogenic Fungus Agents. J. Agric. Food Chem. 68, 7839–7849. doi:10.1021/acs.jafc.0c03994
Katayama, S., Ohno, F., Yamauchi, Y., Kato, M., Makabe, H., and Nakamura, S. (2013). Enzymatic Synthesis of Novel Phenol Acid Rutinosides Using Rutinase and Their Antiviral Activity In Vitro. J. Agric. Food Chem. 61, 9617–9622. doi:10.1021/jf4021703
Kayaci, F., and Uyar, T. (2011). Solid Inclusion Complexes of Vanillin with Cyclodextrins: Their Formation, Characterization, and High-Temperature Stability. J. Agric. Food Chem. 59, 11772–11778. doi:10.1021/jf202915c
Lan, X., Xie, D., Yin, L., Wang, Z., Chen, J., Zhang, A., et al. (2017). Novel α,β-unsaturated Amide Derivatives Bearing α-amino Phosphonate Moiety as Potential Antiviral Agents. Bioorg. Med. Chem. Lett. 27, 4270–4273. doi:10.1016/j.bmcl.2017.08.048
Li, G., Guo, J., Wang, Z., Liu, Y., Song, H., and Wang, Q. (2018). Marine Natural Products for Drug Discovery: First Discovery of Kealiinines A-C and Their Derivatives as Novel Antiviral and Antiphytopathogenic Fungus Agents. J. Agric. Food Chem. 66, 7310–7318. doi:10.1021/acs.jafc.8b02238
Li, M., Zan, N., Huang, M., Jiang, D., Hu, D., and Song, B. (2020). Design, Synthesis and Anti-TMV Activities of Novel Chromone Derivatives Containing Dithioacetal Moiety. Bioorg. Med. Chem. Lett. 30, 126945. doi:10.1016/j.bmcl.2019.126945
Li, X.-y., and Song, B.-a. (2017). Progress in the Development and Application of Plant-Based Antiviral Agents. J. Integr. Agric. 16, 2772–2783. doi:10.1016/s2095-3119(17)61788-x
Li, X., Wang, Y., Chen, K., Gao, D., Wang, D., and Xue, W. (2019). Cucumber Mosaic Virus Coat Protein: The Potential Target of 1, 4-Pentadien-3-One Derivatives. Pesticide Biochem. Physiology 155, 45–50. doi:10.1016/j.pestbp.2019.01.004
Libardi, S. H., Borges, J. C., Skibsted, L. H., and Cardoso, D. R. (2011). Deactivation of Ferrylmyoglobin by Vanillin as Affected by Vanillin Binding to β-Lactoglobulin. J. Agric. Food Chem. 59, 6202–6208. doi:10.1021/jf1047173
Liu, B., Li, R., Li, Y., Li, S., Yu, J., Zhao, B., et al. (2019). Discovery of Pimprinine Alkaloids as Novel Agents against a Plant Virus. J. Agric. Food Chem. 67, 1795–1806. doi:10.1021/acs.jafc.8b06175
Liu, Y., Chen, J., Xie, D., Song, B., and Hu, D. (2021). First Report on Anti-TSWV Activities of Quinazolinone Derivatives Containing a Dithioacetal Moiety. J. Agric. Food Chem. 69, 12135–12142. doi:10.1021/acs.jafc.1c03171
Liu, Y., Song, H., Huang, Y., Li, J., Zhao, S., Song, Y., et al. (2014). Design, Synthesis, and Antiviral, Fungicidal, and Insecticidal Activities of Tetrahydro-β-Carboline-3-Carbohydrazide Derivatives. J. Agric. Food Chem. 62, 9987–9999. doi:10.1021/jf503794g
Long, C., Li, P., Chen, M., Dong, L., Hu, D., and Song, B. (2015). Synthesis, Anti-tobacco Mosaic Virus and Cucumber Mosaic Virus Activity, and 3D-QSAR Study of Novel 1,4-Pentadien-3-One Derivatives Containing 4-Thioquinazoline Moiety. Eur. J. Med. Chem. 102, 639–647. doi:10.1016/j.ejmech.2015.08.029
Lu, A., Wang, J., Liu, T., Han, J., Li, Y., Su, M., et al. (2014). Small Changes Result in Large Differences: Discovery of (−)-Incrustoporin Derivatives as Novel Antiviral and Antifungal Agents. J. Agric. Food Chem. 62, 8799–8807. doi:10.1021/jf503060k
Lu, A., Wang, T., Hui, H., Wei, X., Cui, W., Zhou, C., et al. (2019). Natural Products for Drug Discovery: Discovery of Gramines as Novel Agents against a Plant Virus. J. Agric. Food Chem. 67, 2148–2156. doi:10.1021/acs.jafc.8b06859
Luo, D., Guo, S., He, F., Chen, S., Dai, A., Zhang, R., et al. (2020). Design, Synthesis, and Bioactivity of α-Ketoamide Derivatives Bearing a Vanillin Skeleton for Crop Diseases. J. Agric. Food Chem. 68, 7226–7234. doi:10.1021/acs.jafc.0c00724
Luo, H., Liu, J., Jin, L., Hu, D., Chen, Z., Yang, S., et al. (2013). Synthesis and Antiviral Bioactivity of Novel (1E, 4E)-1-Aryl-5-(2-(quinazolin-4-Yloxy)phenyl)-1,4-Pentadien-3-One Derivatives. Eur. J. Med. Chem. 63, 662–669. doi:10.1016/j.ejmech.2013.02.035
Lv, X., Xiang, S., Wang, X., Wu, L., Liu, C., Yuan, M., et al. (2020). Synthetic Chloroinconazide Compound Exhibits Highly Efficient Antiviral Activity against Tobacco Mosaic Virus. Pest Manag. Sci. 76, 3636–3648. doi:10.1002/ps.5910
Lv, X., Yuan, M., Pei, Y., Liu, C., Wang, X., Wu, L., et al. (2021). The Enhancement of Antiviral Activity of Chloroinconazide by Aglinate-Based Nanogel and its Plant Growth Promotion Effect. J. Agric. Food Chem. 69, 4992–5002. doi:10.1021/acs.jafc.1c00941
Ma, J., Li, P., Li, X., Shi, Q., Wan, Z., Hu, D., et al. (2014). Synthesis and Antiviral Bioactivity of Novel 3-((2-((1e,4e)-3-Oxo-5-Arylpenta-1,4-Dien-1-Yl)phenoxy)methyl)-4(3h)-Quinazolinone Derivatives. J. Agric. Food Chem. 62, 8928–8934. doi:10.1021/jf502162y
Ni, W., Li, C., Liu, Y., Song, H., Wang, L., Song, H., et al. (2017). Various Bioactivity and Relationship of Structure-Activity of Matrine Analogues. J. Agric. Food Chem. 65, 2039–2047. doi:10.1021/acs.jafc.6b05474
Olivon, F., Palenzuela, H., Girard-Valenciennes, E., Neyts, J., Pannecouque, C., Roussi, F., et al. (2015). Antiviral Activity of Flexibilane and Tigliane Diterpenoids fromStillingia Lineata. J. Nat. Prod. 78, 1119–1128. doi:10.1021/acs.jnatprod.5b00116
Park, J., Wen, A. M., Gao, H., Shin, M. D., Simon, D. I., Wang, Y., et al. (2021). Designing S100A9-Targeted Plant Virus Nanoparticles to Target Deep Vein Thrombosis. Biomacromolecules 22, 25822594. doi:10.1021/acs.biomac.1c00303
Rajasekharan, S. K., Kim, S., Kim, J.-C., and Lee, J. (2020). Nematicidal Activity of 5-iodoindole against Root-Knot Nematodes. Pesticide Biochem. Physiology 163, 76–83. doi:10.1016/j.pestbp.2019.10.012
Ran, L., Yang, H., Luo, L., Huang, M., and Hu, D. (2020). Discovery of Potent and Novel Quinazolinone Sulfide Inhibitors with Anti-tocv Activity. J. Agric. Food Chem. 68, 5302–5308. doi:10.1021/acs.jafc.0c00686
Rodrigues, T., Reker, D., Schneider, P., and Schneider, G. (2016). Counting on Natural Products for Drug Design. Nat. Chem. 8, 531–541. doi:10.1038/nchem.2479
Ryu, S. M., Lee, H. M., Song, E. G., Seo, Y. H., Lee, J., Guo, Y., et al. (2017). Antiviral Activities of Trichothecenes Isolated from Trichoderma Albolutescens against Pepper Mottle Virus. J. Agric. Food Chem. 65, 4273–4279. doi:10.1021/acs.jafc.7b01028
Scholthof, K.-B. G., Adkins, S., Czosnek, H., Palukaitis, P., Jacquot, E., Hohn, T., et al. (2011). Top 10 Plant Viruses in Molecular Plant Pathology. Mol. Plant Pathol. 12, 938–954. doi:10.1111/j.1364-3703.2011.00752.x
Sharma, J., Bhardwaj, V. K., Das, P., and Purohit, R. (2021). Plant-Based Analogues Identified as Potential Inhibitor against Tobacco Mosaic Virus: A Biosimulation Approach. Pesticide Biochem. Physiology 175, 104858. doi:10.1016/j.pestbp.2021.104858
Shen, J.-G., Zhang, Z.-K., Wu, Z.-J., Ouyang, M.-A., Xie, L.-H., and Lin, Q.-Y. (2008). Antiphytoviral Activity of Bruceine-D fromBrucea Javanica Seeds. Pest. Manag. Sci. 64, 191–196. doi:10.1002/ps.1465
Shi, J., Yu, L., and Song, B. (2018). Proteomics Analysis of Xiangcaoliusuobingmi-Treated Capsicum Annuum L. Infected with Cucumber Mosaic Virus. Pesticide Biochem. Physiology 149, 113–122. doi:10.1016/j.pestbp.2018.06.008
Silverman, F. P., Petracek, P. D., Heiman, D. F., Fledderman, C. M., and Warrior, P. (2005). Salicylate Activity. 3. Structure Relationship to Systemic Acquired Resistance. J. Agric. Food Chem. 53, 9775–9780. doi:10.1021/jf051383t
Sinha, S., Manju, S. L., and Doble, M. (2019). Chalcone-Thiazole Hybrids: Rational Design, Synthesis, and Lead Identification against 5-Lipoxygenase. ACS Med. Chem. Lett. 10, 1415–1422. doi:10.1021/acs.jpca.8b0678710.1021/acsmedchemlett.9b00193
Sonar, V. P., Fois, B., Distinto, S., Maccioni, E., Meleddu, R., Cottiglia, F., et al. (2019). Ferulic Acid Esters and Withanolides: In Search of Withania Somnifera GABAA Receptor Modulators. J. Nat. Prod. 82, 1250–1257. doi:10.1021/acs.jnatprod.8b01023
Song, H.-j., Liu, Y.-x., Liu, Y.-x., Huang, Y.-q., Li, Y.-q., and Wang, Q.-m. (2014b). Design, Synthesis, Anti-TMV, Fungicidal, and Insecticidal Activity Evaluation of 1,2,3,4-Tetrahydro-β-Carboline-3-Carboxylic Acid Derivatives Based on Virus Inhibitors of Plant Sources. Bioorg. Med. Chem. Lett. 24, 5228–5233. doi:10.1016/j.bmcl.2014.09.063
Song, H., Liu, Y., Liu, Y., Wang, L., and Wang, Q. (2014a). Synthesis and Antiviral and Fungicidal Activity Evaluation of β-Carboline, Dihydro-β-Carboline, Tetrahydro-β-Carboline Alkaloids, and Their Derivatives. J. Agric. Food Chem. 62, 1010–1018. doi:10.1021/jf404840x
Su, B., Cai, C., Deng, M., Liang, D., Wang, L., and Wang, Q. (2014a). Design, Synthesis, Antiviral Activity, and Sars of 13a-Substituted Phenanthroindolizidine Alkaloid Derivatives. Bioorg. Med. Chem. Lett. 24, 2881–2884. doi:10.1016/j.bmcl.2014.04.101
Su, B., Cai, C., Deng, M., and Wang, Q. (2016). Spatial Configuration and Three-Dimensional Conformation Directed Design, Synthesis, Antiviral Activity, and Structure-Activity Relationships of Phenanthroindolizidine Analogues. J. Agric. Food Chem. 64, 2039–2045. doi:10.1021/acs.jafc.5b06112
Su, B., Cai, C., Li, Y., and Wang, Q. (2021). Design, Synthesis, Antivirus Activity, and Sars of Phenanthroquinolizidine Alkaloid Derivatives. ACS Agric. Sci. Technol. 1, 222–229. doi:10.1021/acsagscitech.1c00032
Su, B., Chen, F., Wang, L., and Wang, Q. (2014b). Design, Synthesis, Antiviral Activity, and Structure-Activity Relationships (Sars) of Two Types of Structurally Novel Phenanthroindo/Quinolizidine Analogues. J. Agric. Food Chem. 62, 1233–1239. doi:10.1021/jf405562r
Tan, Q.-W., Ni, J.-C., Zheng, L.-P., Fang, P.-H., Shi, J.-T., and Chen, Q.-J. (2018). Anti-Tobacco Mosaic Virus Quassinoids from Ailanthus Altissima (Mill.) Swingle. J. Agric. Food Chem. 66, 7347–7357. doi:10.1021/acs.jafc.8b01280
Wang, D., Liu, B., Ma, Z., Feng, J., and Yan, H. (2021a). Reticine A, a New Potent Natural Elicitor: Isolation from the Fruit Peel of Citrus Reticulate and Induction of Systemic Resistance against Tobacco Mosaic Virus and Other Plant Fungal Diseases. Pest Manag. Sci. 77, 354–364. doi:10.1002/ps.6025
Wang, H., and Song, H. (2020c). Synthesis of Four Optical Isomers of Antiviral Agent NK0209 and Determination of Their Configurations and Activities against a Plant Virus. J. Agric. Food Chem. 68, 2631–2638. doi:10.1021/acs.jafc.9b07694
Wang, J., Yu, G., Li, Y., Shen, L., Qian, Y., Yang, J., et al. (2015). Inhibitory Effects of Sulfated Lentinan with Different Degree of Sulfation against Tobacco Mosaic Virus (TMV) in Tobacco Seedlings. Pesticide Biochem. Physiology 122, 38–43. doi:10.1016/j.pestbp.2014.12.027
Wang, K., Hu, Y., Liu, Y., Mi, N., Fan, Z., Liu, Y., et al. (2010b). Design, Synthesis, and Antiviral Evaluation of Phenanthrene-Based Tylophorine Derivatives as Potential Antiviral Agents. J. Agric. Food Chem. 58, 12337–12342. doi:10.1021/jf103440s
Wang, K., Su, B., Wang, Z., Wu, M., Li, Z., Hu, Y., et al. (2010a). Synthesis and Antiviral Activities of Phenanthroindolizidine Alkaloids and Their Derivatives. J. Agric. Food Chem. 58, 2703–2709. doi:10.1021/jf902543r
Wang, Q., Song, H., and Wang, Q. (2022). Studies on the Biological Activity of Gem-Difluorinated 3,3′-spirocyclic Indole Derivatives. Chin. Chem. Lett. 33, 859–862. doi:10.1016/j.cclet.2021.08.005
Wang, T., Li, L., Zhou, Y., Lu, A., Li, H., Chen, J., et al. (2021b). Structural Simplification of Marine Natural Products: Discovery of Hamacanthin Derivatives Containing Indole and Piperazinone as Novel Antiviral and Anti-phytopathogenic-fungus Agents. J. Agric. Food Chem. 69, 10093–10103. doi:10.1021/acs.jafc.1c04098
Wang, T., Yang, S., Li, H., Lu, A., Wang, Z., Yao, Y., et al. (2020a). Discovery, Structural Optimization, and Mode of Action of Essramycin Alkaloid and its Derivatives as Anti-tobacco Mosaic Virus and Anti-phytopathogenic Fungus Agents. J. Agric. Food Chem. 68, 471–484. doi:10.1021/acs.jafc.9b06006
Wang, Y., He, F., Wu, S., Luo, Y., Wu, R., Hu, D., et al. (2020b). Design, Synthesis, Anti-TMV Activity, and Preliminary Mechanism of Cinnamic Acid Derivatives Containing Dithioacetal Moiety. Pesticide Biochem. Physiology 164, 115–121. doi:10.1016/j.pestbp.2020.01.002
Wang, Y., Zhang, J., He, F., Gan, X., Song, B., and Hu, D. (2019). Design, Synthesis, Bioactivity and Mechanism of Dithioacetal Derivatives Containing Dioxyether Moiety. Bioorg. Med. Chem. Lett. 29, 2218–2223. doi:10.1016/j.bmcl.2019.06.030
Wang, Z., Feng, A., Cui, M., Liu, Y., Wang, L., and Wang, Q. (2014a). Design, Synthesis, Anti-tobacco Mosaic Virus (TMV) Activity, and Sars of 7-Methoxycryptopleurine Derivatives. Chem. Biol. Drug. Des. 84, 531–542. doi:10.1111/cbdd.12340
Wang, Z., Wang, L., Ma, S., Liu, Y., Wang, L., and Wang, Q. (2012a). Design, Synthesis, Antiviral Activity, and Sars of 14-Aminophenanthroindolizidines. J. Agric. Food Chem. 60, 5825–5831. doi:10.1021/jf3013376
Wang, Z., Wei, P., Liu, Y., and Wang, Q. (2014b). D and E Rings May Not Be Indispensable for Antofine: Discovery of Phenanthrene and Alkylamine Chain Containing Antofine Derivatives as Novel Antiviral Agents against Tobacco Mosaic Virus (TMV) Based on Interaction of Antofine and TMV RNA. J. Agric. Food Chem. 62, 10393–10404. doi:10.1021/jf5028894
Wang, Z., Wei, P., Wang, L., and Wang, Q. (2012c). Design, Synthesis, and Anti-tobacco Mosaic Virus (TMV) Activity of Phenanthroindolizidines and Their Analogues. J. Agric. Food Chem. 60, 10212–10219. doi:10.1021/jf303550a
Wang, Z., Wei, P., Xizhi, X., Liu, Y., Wang, L., and Wang, Q. (2012b). Design, Synthesis, and Antiviral Activity Evaluation of Phenanthrene-Based Antofine Derivatives. J. Agric. Food Chem. 60, 8544–8551. doi:10.1021/jf302746m
Wang, Z., Xie, D., Gan, X., Zeng, S., Zhang, A., Yin, L., et al. (2017). Synthesis, Antiviral Activity, and Molecular Docking Study of Trans-ferulic Acid Derivatives Containing Acylhydrazone Moiety. Bioorg. Med. Chem. Lett. 27, 4096–4100. doi:10.1016/j.bmcl.2017.07.038
Wei, C., Zhang, J., Shi, J., Gan, X., Hu, D., and Song, B. (2019). Synthesis, Antiviral Activity, and Induction of Plant Resistance of Indole Analogues Bearing Dithioacetal Moiety. J. Agric. Food Chem. 67, 13882–13891. doi:10.1021/acs.jafc.9b05357
Wei, C., Zhao, L., Sun, Z., Hu, D., and Song, B. (2020). Discovery of Novel Indole Derivatives Containing Dithioacetal as Potential Antiviral Agents for Plants. Pesticide Biochem. Physiology 166, 104568. doi:10.1016/j.pestbp.2020.104568
Wu, J., Zhu, Y.-Y., Zhao, Y.-H., Shan, W.-L., Hu, D.-Y., Chen, J.-X., et al. (2016). Synthesis and Antiviral Activities of Novel 1,4-Pentadien-3-One Derivatives Bearing an Emodin Moiety. Chin. Chem. Lett. 27, 948–952. doi:10.1016/j.cclet.2016.01.051
Wu, M., Han, G., Meng, C., Wang, Z., Liu, Y., and Wang, Q. (2014). Design, Synthesis, and Anti-tobacco Mosaic Virus (TMV) Activity of Glycoconjugates of Phenanthroindolizidines Alkaloids. Mol. Divers 18, 25–37. doi:10.1007/s11030-013-9484-4
Wu, M., Han, G., Wang, Z., Liu, Y., and Wang, Q. (2013). Synthesis and Antiviral Activities of Antofine Analogues with Different C-6 Substituent Groups. J. Agric. Food Chem. 61, 1030–1035. doi:10.1021/jf304905k
Xia, Q., Dong, J., Li, L., Wang, Q., Liu, Y., and Wang, Q. (2018). Discovery of Glycosylated Genipin Derivatives as Novel Antiviral, Insecticidal, and Fungicidal Agents. J. Agric. Food Chem. 66, 1341–1348. doi:10.1021/acs.jafc.7b05861
Xie, J., Xu, W., Song, H., Liu, Y., Zhang, J., and Wang, Q. (2020). Synthesis and Antiviral/Fungicidal/Insecticidal Activities Study of Novel Chiral Indole Diketopiperazine Derivatives Containing Acylhydrazone Moiety. J. Agric. Food Chem. 68, 5555–5571. doi:10.1021/acs.jafc.0c00875
Yan, C., Dong, J., Liu, Y., Li, Y., and Wang, Q. (2021). Target-Directed Design, Synthesis, Antiviral Activity, and Sars of 9-Substituted Phenanthroindolizidine Alkaloid Derivatives. J. Agric. Food Chem. 69, 7565–7571. doi:10.1021/acs.jafc.1c02276
Yan, X.-H., Chen, J., Di, Y.-T., Fang, X., Dong, J.-H., Sang, P., et al. (2010). Anti-Tobacco Mosaic Virus (TMV) Quassinoids from Brucea Javanica (L.) Merr. J. Agric. Food Chem. 58, 1572–1577. doi:10.1021/jf903434h
Yang, H., Zu, G., Liu, Y., Xie, D., Gan, X., and Song, B. (2020b). Tomato Chlorosis Virus Minor Coat Protein as A Novel Target to Screen Antiviral Drugs. J. Agric. Food Chem. 68, 3425–3433. doi:10.1021/acs.jafc.9b08215
Yang, Y., Zhang, J., Li, X., He, F., Wu, R., Hu, D., et al. (2020a). Discovery of Dithioacetal Derivatives Containing Sulfonamide Moiety of Novel Antiviral Agents by TMV Coat Protein as A Potential Target. ACS Omega 5, 22596–22602. doi:10.1021/acsomega.0c03306
Yu, X., Wei, P., Wang, Z., Liu, Y., Wang, L., and Wang, Q. (2016). Design, Synthesis, Antiviral Activity and Mode of Action of Phenanthrene-containingN-Heterocyclic Compounds Inspired by the Phenanthroindolizidine Alkaloid Antofine. Pest. Manag. Sci. 72, 371–378. doi:10.1002/ps.4008
Zan, N., Li, J., He, H., Hu, D., and Song, B. (2021). Discovery of Novel Chromone Derivatives as Potential Anti-TSWV Agents. J. Agric. Food Chem. 69, 10819–10829. doi:10.1021/acs.jafc.1c03626
Zan, N., Xie, D., Li, M., Jiang, D., and Song, B. (2020). Design, Synthesis, and Anti-tocv Activity of Novel Pyrimidine Derivatives Bearing a Dithioacetal Moiety that Targets ToCV Coat Protein. J. Agric. Food Chem. 68, 6280–6285. doi:10.1021/acs.jafc.0c00987
Zhang, J., He, F., Chen, J., Wang, Y., Yang, Y., Hu, D., et al. (2021b). Purine Nucleoside Derivatives Containing a Sulfa Ethylamine Moiety: Design, Synthesis, Antiviral Activity, and Mechanism. J. Agric. Food Chem. 69, 5575–5582. doi:10.1021/acs.jafc.0c06612
Zhang, J., Zhao, L., Zhu, C., Wu, Z., Zhang, G., Gan, X., et al. (2017). Facile Synthesis of Novel Vanillin Derivatives Incorporating a Bis(2-Hydroxyethyl)dithhioacetal Moiety as Antiviral Agents. J. Agric. Food Chem. 65, 4582–4588. doi:10.1021/acs.jafc.7b01035
Zhang, X., Huang, W., Lu, X., Liu, S., Feng, H., Yang, W., et al. (2021a). Identification of Carbazole Alkaloid Derivatives with Acylhydrazone as Novel Anti-TMV Agents with the Guidance of a Digital Fluorescence Visual Screening. J. Agric. Food Chem. 69, 7458–7466. doi:10.1021/acs.jafc.1c00897
Zhang, X., Zhou, Y., Wei, Z., Shen, J., Wang, L., Ma, Z., et al. (2018). Antiphytoviral Toxins of Actinidia Chinensis Root Bark (ACRB) Extract: Laboratory and Semi-field Trials. Pest. Manag. Sci. 74, 1630–1636. doi:10.1002/ps.4854
Zhao, L., Chen, Y., Wu, K., Yan, H., Hao, X., and Wu, Y. (2017a). Application of Fatty Acids as Antiviral Agents against Tobacco Mosaic Virus. Pesticide Biochem. Physiology 139, 87–91. doi:10.1016/j.pestbp.2017.05.005
Zhao, L., Dong, J., Hu, Z., Li, S., Su, X., Zhang, J., et al. (2017c). Anti-TMV Activity and Functional Mechanisms of Two Sesquiterpenoids Isolated from Tithonia Diversifolia. Pesticide Biochem. Physiology 140, 24–29. doi:10.1016/j.pestbp.2017.05.009
Zhao, L., Feng, C., Wu, K., Chen, W., Chen, Y., Hao, X., et al. (2017b). Advances and Prospects in Biogenic Substances against Plant Virus: A Review. Pesticide Biochem. Physiology 135, 15–26. doi:10.1016/j.pestbp.2016.07.003
Zhao, L., Hu, Z., Li, S., Zhang, L., YuZhang, P. J., Zhang, J., et al. (2020b). Tagitinin A from Tithonia Diversifolia Provides Resistance to Tomato Spotted Wilt Orthotospovirus by Inducing Systemic Resistance. Pesticide Biochem. Physiology 169, 104654. doi:10.1016/j.pestbp.2020.104654
Zhao, L., Zhang, J., Liu, T., Mou, H., Wei, C., Hu, D., et al. (2020a). Design, Synthesis, and Antiviral Activities of Coumarin Derivatives Containing Dithioacetal Structures. J. Agric. Food Chem. 68, 975–981. doi:10.1021/acs.jafc.9b06861
Zhou, D., Xie, D., He, F., Song, B., and Hu, D. (2018). Antiviral Properties and Interaction of Novel Chalcone Derivatives Containing a Purine and Benzenesulfonamide Moiety. Bioorg. Med. Chem. Lett. 28, 2091–2097. doi:10.1016/j.bmcl.2018.04.042
Zhou, X., Ye, Y., Liu, S., Shao, W., Liu, L., Yang, S., et al. (2021). Design, Synthesis and Anti-TMV Activity of Novel α-aminophosphonate Derivatives Containing a Chalcone Moiety that Induce Resistance against Plant Disease and Target the TMV Coat Protein. Pesticide Biochem. Physiology 172, 104749. doi:10.1016/j.pestbp.2020.104749
Zhu, Y. J., Wu, Q. F., Fan, Z. J., Huo, J. Q., Zhang, J. L., Zhao, B., et al. (2019). Synthesis, Bioactivity and Mode of Action of 5 A 5 B 6 C Tricyclic Spirolactones as Novel Antiviral Lead Compounds. Pest. Manag. Sci. 75, 292–301. doi:10.1002/ps.5115
Keywords: plant virus, natural products, antiviral agents, SAR, mechanism
Citation: Chen J, Luo X, Chen Y, Wang Y, Peng J and Xing Z (2022) Recent Research Progress: Discovery of Anti-Plant Virus Agents Based on Natural Scaffold. Front. Chem. 10:926202. doi: 10.3389/fchem.2022.926202
Received: 22 April 2022; Accepted: 13 May 2022;
Published: 26 May 2022.
Edited by:
Pei Li, Kaili University, ChinaReviewed by:
Chen Jingsheng, Chongqing Three Gorges University, ChinaYahui Li, Anhui Agricultural University, China
Copyright © 2022 Chen, Luo, Chen, Wang, Peng and Xing. This is an open-access article distributed under the terms of the Creative Commons Attribution License (CC BY). The use, distribution or reproduction in other forums is permitted, provided the original author(s) and the copyright owner(s) are credited and that the original publication in this journal is cited, in accordance with accepted academic practice. No use, distribution or reproduction is permitted which does not comply with these terms.
*Correspondence: Jixiang Chen, anhjaGVuQGd6dS5lZHUuY24=