- School of Chemical and Printing-dyeing Engineering, Henan University of Engineering, Zhengzhou, China
The current study investigated host-guest complexation in 6-p-toluidinylnaphthalene-2-sulfonate (TNS), a fluorescence probe used to investigate hydrophobic regions that contain the water-soluble cationic pillar[6]arene (CP6). After complexation with CP6, the fluorescence intensity of TNS was significantly increased. The decreases in the fluorescence intensity of the TNS•CP6 complex when phenolic food-additives are added have been used in indicator displacement assays to detect food additives in the water.
Introduction
Fluorescent indicator displacement (FID) assays make use of fluorescent indicators and emission phenomena to detect important analytes by transiting different receptors to universal optical sensors. FID assays can bind to a wide variety of target molecules (Rather et al., 2021; Sedgwick et al., 2021). With the advancement of host-guest chemistry, macrocyclic host-based FID assays have garnered widespread attention for their potential application in the field of analytical testing, and several significant research results have been generated in recent years (Dsouza et al., 2011; Ghale et al., 2014; Cao et al., 2019; Jiang et al., 2020). Macrocyclic hosts, primarily cyclodextrins (Crini, et al., 2014; Pal, et al., 2015), calixarenes (Koh, et al., 1996; Hennig, et al., 2007; Guo, et al., 2014; Zheng, et al., 2018), cucurbiturils (Praetorius, et al., 2008; Florea, et al., 2011; Barrow, et al., 2015; Sonzini, et al., 2017), and pillararenes (Wang, et al., 2014; Bojtár, et al., 2015; Bojtár, et al., 2016; Hua, et al., 2016; Bojtár, et al., 2017; Hua, et al., 2018; Cai, et al., 2021; Wu, et al., 2021), are widely used as fluorescent probes in the majority of the FID-based sensing systems.
Phenolic food additives have been widely used in the food industry for their significant antioxidant, antimicrobial, and flavor-enhancing properties (Vinson, et al., 2012; Zhang, et al., 2014). Whereas the insolubility of food additives in water and their long-term stability contribute to their excessive use, ultimately resulting in their accumulation and negative effects on the biosphere (Tobacman, et al., 2001; Savjani, et al., 2012). Encapsulating small-molecule food-additives in non-toxic, water-soluble macrocyclic hosts improves their bioavailability and solubility by regulating their physical and chemical properties (Munin, et al., 2011). 2-Hydroxypropyl β-cyclodextrin (HP-β-CD) is one representative example, with a binding affinity of ∼ 102 M−1 to food-additives (Pal, et al., 2016). It is critical to investigate artificial receptors with extremely high affinity for food additives to improve sensitivity and detection efficiency in compound detection.
We developed a new FID assay with a water-soluble cationic pillar[6]arene (CP6) for the detection of three important phenolic food additives, namely p-coumaric acid (CA), trans-ferulic acid (FA), and gallic acid (GA). Because of its enhanced fluorescence in non-polar environments, the widely used fluorescent probe, 6-p-toluidinylnaphthalene-2-sulfonate (Dotsikas, et al., 2000) (TNS in Scheme 1), was used as the fluorescent indicator in our FID system. Due to the complexation of TNS and CP6, we use an FID strategy to perform sensitive fluorescence detection on CA, FA, and GA.
Materials and Methods
The reagents used were marketable and applied directly without further purification. CP6 (Duan et al., 2019) was synthesized by following the known procedures. Nuclear magnetic resonance (NMR) spectra were obtained using the Bruker Avance III HD 400 spectrometer with the deuterated solvent as the lock and the residual solvent as the internal reference. Fluorescence spectra were obtained by using the Agilent Cary Eclipse fluorescence spectrophotometer. To prevent the dilution effect during titration, CP6 stock solutions were produced using the same TNS solution. The measurement was repeated three times for each experiment. Displacement assays for CA, FA, and GA were performed at pH 6.8 with CP6 at varying concentrations of CA, FA, and GA, respectively. All the experiments were conducted at room temperature (298 K).
Results and Discussion
Fluorescent Probe 6-p-Toluidinylnaphthalene-2-Sulfonate Complexed With Cationic Pillar[6]Arene
UV-vis absorption spectroscopy was used to confirm the host-guest complexation of fluorescent probe TNS with CP6. Following successive additions of CP6 to the phosphate-buffered solution (PBS) of TNS at pH 6.8, hyperchromic effects at the maximum absorption wavelengths of 223, 263, and 318 nm occurred with a significant bathochromic shift (Figure 1A). The variations appeared to be greater than those when α-CD (Nishijo et al., 1992) or β-CD (Nishijo et al., 1995; Dotsikas et al., 2000) addition was used. The results indicate that TNS can form a stable complex with CP6. Additionally, a fluorescence titration on TNS with an increased CP6 concentration was performed in PBS with a pH of 6.8 at room temperature. According to Figure 1B, as the concentration of CP6 increased, a significant increase in fluorescence intensity was observed, along with a shift in the fluorescence maximum to shorter wavelengths. The results indicate that TNS molecules exist in a hydrophobic environment. Encapsulating TNS in CP6 protects it from solvent collisions while also providing a distinct local environment for TNS in terms of polarity, which significantly enhances (approximately 400 times) fluorescence intensity. Additionally, the significant pale blue fluorescence was evident in UV light (the inset of Figure 1B).
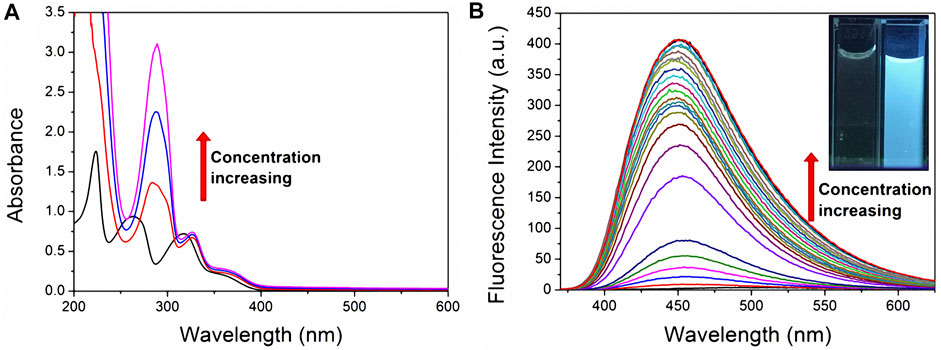
FIGURE 1. UV-vis and fluorescence titration on TNS with CP6 in PBS (pH 6.8). (A) Absorption spectra (20 µM TNS, 0–6.5 equiv. CP6) (B) fluorescence spectra (20 µM TNS, 0–2.6 equiv. CP6, λex = 318 nm). The inset illustrates enhanced fluorescence in water when excited at 365 nm with a UV lamp set to 298 K.
1H NMR tests were used to investigate the host-guest complexation. Because the solubility of the complex in neat D2O was insufficient to reach the mM level, DMSO-d6 cosolvents were added. As illustrated in Figure 2, the naphthyl proton signals of TNS in the inclusion complex underwent varying degrees of upward shifts. The largest shift occurred in the direction away from the sulfonate group, whereas the smallest shift occurred in the direction toward the sulfonate group. Proton signal variations in the methylphenyl group are insignificant, indicating that this group may be located outside the cavity. When combined with the protons’ shift and broadening in the sulfonate-naphthyl group, it is concluded that the fluorescence probe molecule is partially in the CP6 cavity, where the shielding effects of the aromatic host produce the characteristic signal broadening (Li et al., 2010). Additionally, the 2D ROESY data (Supplementary Figure S1) establish a correlation between the naphthyl protons (Ha-f) in the entrapped TNS and the aromatic proton H1 in CP6, revealing the interpenetrated geometry.
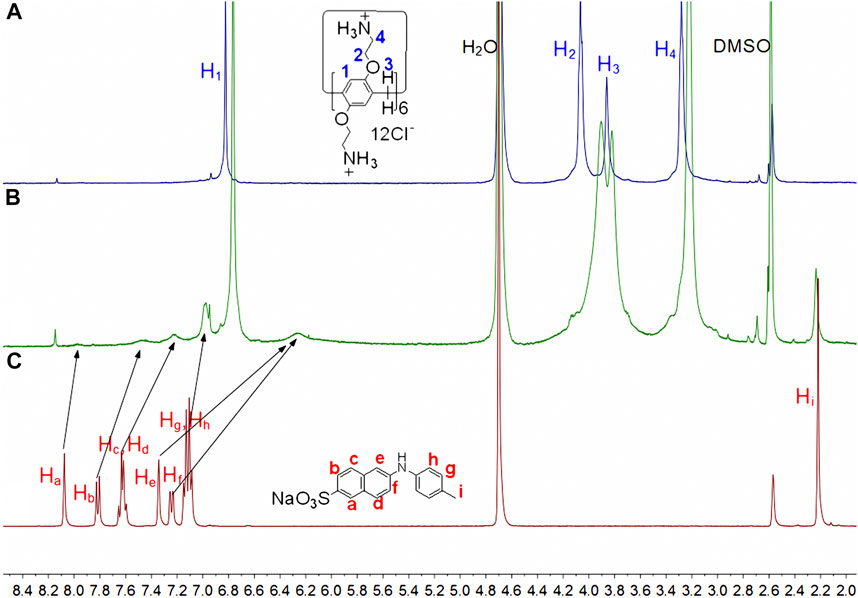
FIGURE 2. Partial 1H NMR spectra (400 MHz, D2O:DMSO-d6 = 3:1, 298 K) for (A) 5 mM CP6, (B) 5 mM CP6 and 15 mM TNS, (C) 15 mM TNS.
To quantify the binding of TNS to CP6, the association constant (Ka) was determined to be (4.51 ± 0.90) × 105 M−1 using titration tests and a non-linear curve-fitting to the fluorescence spectra (Supplementary Figure S4). The complex formed by CP6 and TNS had a 1:1 binding stoichiometry (Supplementary Figure S3A). We deduced that the complex formed between CP6 and TNS in aqueous solution as a result of multiple electrostatic interactions between the cationic ammonium groups on CP6 and the sulfonate anion on TNS, hydrophobic interactions, and π-π stacking interactions between the benzene rings on host CP6 and naphthalene ring on guest TNS. The cooperativity of these non-covalent interactions is attributed to the binding affinity in the host-guest system.
Detection of Food-Additives Using Fluorescent Indicator
TNS complexed with CP6 exhibits a significant fluorescence response, allowing it to be used for FID detection. The binding affinities of CP6 to CA, FA, and GA were determined in this study using FID detection (Scheme 1), and the fluorescent indicator TNS was first reversibly bound to the receptor CP6. The solution was then added with a weakly fluorescent or non-fluorescent analyte, which competitively displaced the highly fluorescent TNS from the indicator CP6 cavity, altering the optical signal (You et al., 2015). Notably, titration of a preformed TNS•CP6 complex with increasing concentrations of competitor food additive molecules results in a reversal of the fluorescence intensity, which is used to determine the competitor molecules’ binding affinity. Competitive displacement was used to investigate the binding of three major phenolic food-additives, namely CA, FA, and GA, to CP6. Figure 3A illustrates a typical fluorescence displacement titration with CA as a strong competitor. The quenching of fluorescence in the presence of CA was easily observed with the naked eye using a simple UV-lamp (the inset of Figure 3A).
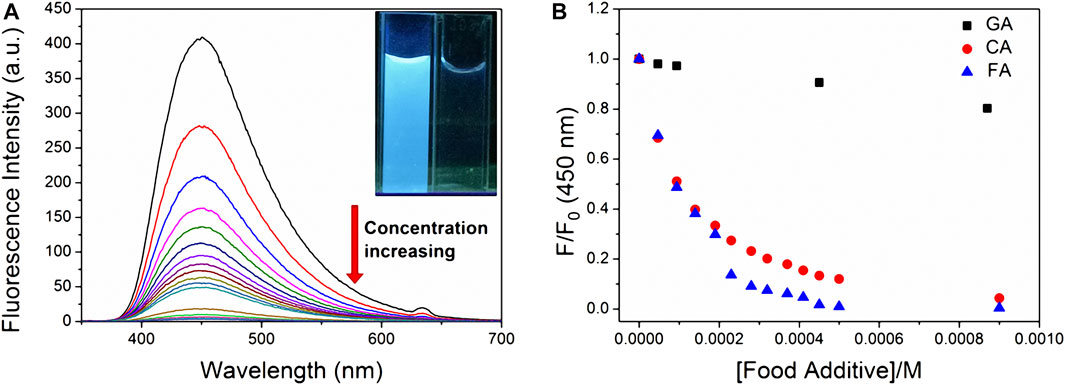
FIGURE 3. Displacement of the fluorescent indicator CP6•TNS by various food additives in PBS (pH 6.8). (A) Fluorescence spectra of CP6•TNS (20 µM TNS, 48 µM CP6, λex = 318 nm) upon addition of CA (0–2.3 mM). The inset reveals fluorescence quenching in water at excitation of 365 nm under the UV lamp at 298 K. (B) Fluorescence intensity changes at 450 nm of CP6•TNS (20 µM TNS, 48 µM CP6, λex = 318 nm) upon addition of CA, FA, and GA in different concentrations.
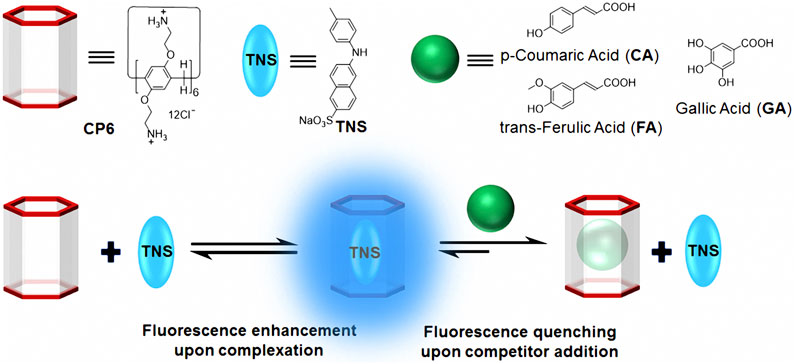
SCHEME 1. Chemical structures and cartoon representations of CP6, TNS, CA, FA, and GA, as well as an illustration of the procedure for fluorescence indicator displacement.
To avoid the effect of changes in pH and dilution on the displacement assay, the pH of complex and food-additive solutions was set to 6.8, and the concentrations of TNS and CP6 in food-additive solutions were kept constant. We used that previously reported competitive binding formula to fit the reduced fluorescence intensities at the band maximum against the concentration of competitor food additives (Bakirci et al., 2006). Using TNS•CP6 as the reporter pair, we determined the association constants (Ka) for CA, FA, and GA to be (1.24 ± 0.29) × 104 M−1, (1.19 ± 0.16) × 104 M−1, and (2.78 ± 0.18) × 102 M−1 (Supplementary Figures S5–S7), respectively. Except for GA, the binding affinities are approximately two orders of magnitude greater than those of previously studied HP-β-CD to the other two food additives, which are around 102 M−1 (Pal et al., 2016).
Additionally, the observed fluorescence response can also be used to quantify CA, FA, and GA. The fluorescence intensity plots increase linearly as the CA, FA, and GA concentrations increase (Supplementary Figure S8), respectively. 0.047–2.3 mM, 0.047–0.14 mM, and 0.047–2.5 mM were the linear ranges. The results indicate that the limit of detection (LOD) values was 0.012, 0.08, and 0.17 µM, respectively, using a 3σ/slope method (MacDougall et al., 1980).
NMR research with GA, CA, and FA was used to determine the complexation of food additives. Supplementary Figure S9 illustrates the 1H NMR spectra for GA in the presence of CP6. As illustrated in the figure, shielding caused a shift in the benzene proton signal of GA, conclusively confirming the inclusion complex between CP6 and GA. Additionally, the 2D NOESY data (Supplementary Figure S2) show NOE cross-peaks between the benzene proton (Ha) in entrapped GA and the protons H1–4 in CP6, indicating the inclusion of a benzene ring in the CP6 cavity. The signals in the NMR spectra of CA and FA changed similarly upon the addition of CP6 (Supplementary Figures S10,S11).
Conclusions
To summarize, we demonstrated a new fluorescence activation switch based on host-guest complexation between the fluorescent indicator probe TNS and cationic pillar[6]arene CP6. In TNS solution, the complexation significantly enhanced the fluorescence. A fluorescence switch-off displacement assay was used to detect three commonly used non-fluorescence phenolic food additives in the water. The study used molecular recognition and fluorescence indicator displacement assays to develop a prospective strategy for phenolic food additive detection.
Data Availability Statement
The original contributions presented in the study are included in the article/Supplementary Material, further inquiries can be directed to the corresponding author.
Author Contributions
QD designed the work. YX and KG made contributions to the experiments and collective data. The paper was written by QD. All authors extensively discussed the results, reviewed the manuscript, and approved the final version of the manuscript to be submitted.
Funding
This work was supported by Henan Province Science and Technology Research Program (No. 222102320067).
Conflict of Interest
The authors declare that the research was conducted in the absence of any commercial or financial relationships that could be construed as a potential conflict of interest.
Publisher’s Note
All claims expressed in this article are solely those of the authors and do not necessarily represent those of their affiliated organizations, or those of the publisher, the editors and the reviewers. Any product that may be evaluated in this article, or claim that may be made by its manufacturer, is not guaranteed or endorsed by the publisher.
Supplementary Material
The Supplementary Material for this article can be found online at: https://www.frontiersin.org/articles/10.3389/fchem.2022.925881/full#supplementary-material
References
Bakirci, H., and Nau, W. M. (2006). Fluorescence Regeneration as a Signaling Principle for Choline and Carnitine Binding: A Refined Supramolecular Sensor System Based on a Fluorescent Azoalkane. Adv. Funct. Mat. 16, 237–242. doi:10.1002/adfm.200500219
Barrow, S. J., Kasera, S., Rowland, M. J., Del Barrio, J., and Scherman, O. A. (2015). Cucurbituril-Based Molecular Recognition. Chem. Rev. 115, 12320–12406. doi:10.1021/acs.chemrev.5b00341
Bojtár, M., Kozma, J., Szakács, Z., Hessz, D., Kubinyi, M., and Bitter, I. (2017). Pillararene-Based Fluorescent Indicator Displacement Assay for the Selective Recognition of ATP. Sensors Actuators B Chem. 248, 305–310. doi:10.1016/j.snb.2017.03.163
Bojtár, M., Paudics, A., Hessz, D., Kubinyi, M., and Bitter, I. (2016). Amino Acid Recognition by Fine Tuning the Association Constants: Tailored Naphthalimides in Pillar[5]arene-Based Indicator Displacement Assays. RSC Adv. 6, 86269–86275. doi:10.1039/C6RA15003A
Bojtár, M., Szakács, Z., Hessz, D., Kubinyi, M., and Bitter, I. (2015). Optical Spectroscopic Studies on the Complexation of Stilbazolium Dyes with a Water Soluble Pillar[5]arene. RSC Adv. 5, 26504–26508. doi:10.1039/C4RA14809F
Cai, Y., Zhang, Z., Ding, Y., Hu, L., Wang, J., Chen, T., et al. (2021). Recent Development of Pillar[n]arene-Based Amphiphiles. Chin. Chem. Lett. 32, 1267–1279. doi:10.1016/j.cclet.2020.10.036
Cao, D., and Meier, H. (2019). Pillararene-Based Fluorescent Sensors for the Tracking of Organic Compounds. Chin. Chem. Lett. 30, 1758–1766. doi:10.1016/j.cclet.2019.06.026
Crini, G. (2014). Review: A History of Cyclodextrins. Chem. Rev. 114, 10940–10975. doi:10.1021/cr500081p
Dotsikas, Y., Kontopanou, E., Allagiannis, C., and Loukas, Y. L. (2000). Interaction of 6-P-Toluidinylnaphthalene-2-Sulphonate with β-cyclodextrin. J. Pharm. Biomed. Analysis 23, 997–1003. doi:10.1016/s0731-7085(00)00392-7
Dsouza, R. N., Pischel, U., and Nau, W. M. (2011). Fluorescent Dyes and Their Supramolecular Host/Guest Complexes with Macrocycles in Aqueous Solution. Chem. Rev. 111, 7941–7980. doi:10.1021/cr200213s
Duan, Q., Zhang, H., Mai, W., Wang, F., and Lu, K. (2019). Acid/Base- and Base/Acid-Switchable Complexation between Anionic-/Cationic-Pillar[6]arenes and A Viologen Ditosylate Salt. Org. Biomol. Chem. 17, 4430–4434. doi:10.1039/c9ob00398c
Florea, M., and Nau, W. M. (2011). Strong Binding of Hydrocarbons to Cucurbituril Probed by Fluorescent Dye Displacement: A Supramolecular Gas-Sensing Ensemble. Angew. Chem. Int. Ed. 50, 9338–9342. doi:10.1002/anie.201104119
Ghale, G., and Nau, W. M. (2014). Dynamically Analyte-Responsive Macrocyclic Host-Fluorophore Systems. Acc. Chem. Res. 47, 2150–2159. doi:10.1021/ar500116d
Guo, D.-S., and Liu, Y. (2014). Supramolecular Chemistry of P-Sulfonatocalix[n]arenes and its Biological Applications. Acc. Chem. Res. 47, 1925–1934. doi:10.1021/ar500009g
Hennig, A., Bakirci, H., and Nau, W. M. (2007). Label-Free Continuous Enzyme Assays with Macrocycle-Fluorescent Dye Complexes. Nat. Methods 4, 629–632. doi:10.1038/nmeth1064
Hua, B., Shao, L., Yu, G., and Huang, F. (2016). Fluorescence Indicator Displacement Detection Based on Pillar[5]arene-Assisted Dye Deprotonation. Chem. Commun. 52, 10016–10019. doi:10.1039/c6cc04919b
Hua, B., Shao, L., Zhang, Z., Sun, J., and Yang, J. (2018). Pillar[6]arene/Acridine Orange Host-Guest Complexes as Colorimetric and Fluorescence Sensors for Choline Compounds and Further Application in Monitoring Enzymatic Reactions. Sensors Actuators B Chem. 255, 1430–1435. doi:10.1016/j.snb.2017.08.141
Jiang, C., Song, Z., Yu, L., Ye, S., and He, H. (2020). Fluorescent Probes Based on Macrocyclic Hosts: Construction, Mechanism and Analytical. TrAC Trends Anal. Chem. 133, 116086. doi:10.1016/j.trac.2020.116086
Koh, K. N., Araki, K., Ikeda, A., Otsuka, H., and Shinkai, S. (1996). Reinvestigation of Calixarene-Based Artificial-Signaling Acetylcholine Receptors Useful in Neutral Aqueous (Water/Methanol) Solution. J. Am. Chem. Soc. 118, 755–758. doi:10.1021/ja951488k
Li, C., Zhao, L., Li, J., Ding, X., Chen, S., Zhang, Q., et al. (2010). Self-assembly of [2]Pseudorotaxanes Based on Pillar[5]arene and Bis(imidazolium) Cations. Chem. Commun. 46, 9016–9018. doi:10.1039/C0CC03575K
MacDougall, D., Crummett, W. B., Amore, F. J., Cox, G. V., Crosby, D. G., and Estes, F. L. (1980). Guidelines for Data Acquisition and Data Quality Evaluation in Environmental Chemistry. Anal. Chem. 52, 2242–2249. doi:10.1021/ac50064a004
Munin, A., and Edwards-Lévy, F. (2011). Encapsulation of Natural Polyphenolic Compounds; a Review. Pharmaceutics 3 (4), 793–829. doi:10.3390/pharmaceutics3040793
Nishijo, J., Nagai, M., Yasuda, M., Ohno, E., and Ushiroda, Y. (1995). Interaction of 6-P-Toluidinylnaphthalene-2-Sulfonate with β-Cyclodextrin. J. Pharm. Sci. 84, 1420–1426. doi:10.1002/jps.2600841207
Nishijo, J., Yasuda, M., Nagai, M., and Ohno, E. (1992). Interaction of 6-P-Toluidinylnaphthalene-2-Sulfonate with .ALPHA.-Cyclodextrin. Chem. Pharm. Bull. 40 (10), 2591–2596. doi:10.1248/cpb.39.510.1248/cpb.40.2591
Pal, K., Chandra, F., Mallick, S., and Koner, A. L. (2016). pH Dependent Supramolecular Recognition of Dapoxyl Sodium Sulfonate with 2-hydroxypropyl β-cyclodextrin: an Application towards Food-Additive Formulation. New J. Chem. 40, 6093–6100. doi:10.1039/c5nj03415a
Pal, K., Mallick, S., and Koner, A. L. (2015). Complexation Induced Fluorescence and Acid-Base Properties of Dapoxyl Dye with γ-cyclodextrin: a Drug-Binding Application Using Displacement Assays. Phys. Chem. Chem. Phys. 17, 16015–16022. doi:10.1039/C5CP01696G
Praetorius, A., Bailey, D. M., Schwarzlose, T., and Nau, W. M. (2008). Design of a Fluorescent Dye for Indicator Displacement from Cucurbiturils: A Macrocycle-Responsive Fluorescent Switch Operating through a pKa Shift. Org. Lett. 10, 4089–4092. doi:10.1021/ol8016275
Rather, I. A., and Ali, R. (2021). Indicator Displacement Assays: From Concept to Recent Developments. Org. Biomol. Chem. 19, 5926–5981. doi:10.1039/d1ob00518a
Savjani, K. T., Gajjar, A. K., and Savjani, J. K. (2012). Drug Solubility: Importance and Enhancement Techniques. ISRN Pharm. 2012, 1–10. doi:10.5402/2012/195727
Sedgwick, A. C., Brewster, J. T., Wu, T., Feng, X., Bull, S. D., Qian, X., et al. (2021). Indicator Displacement Assays (IDAs): the Past, Present and Future. Chem. Soc. Rev. 50, 9–38. doi:10.1039/c9cs00538b
Sonzini, S., McCune, J. A., Ravn, P., Scherman, O. A., and van der Walle, C. F. (2017). A Simple Supramolecular Assay for Drug Detection in Urine. Chem. Commun. 53, 8842–8845. doi:10.1039/C7CC04081D
Tobacman, J. K., Wallace, R. B., and Zimmerman, M. B. (2001). Consumption of Carrageenan and Other Water-Soluble Polymers Used as Food Additives and Incidence of Mammary Carcinoma. Med. Hypotheses 56, 589–598. doi:10.1054/mehy.2000.1208
Vinson, J. A., and Cai, Y. (2012). Nuts, Especially Walnuts, Have Both Antioxidant Quantity and Efficacy and Exhibit Significant Potential Health Benefits. Food Funct. 3, 134–140. doi:10.1039/C2FO10152A
Wang, P., Yao, Y., and Xue, M. (2014). A Novel Fluorescent Probe for Detecting Paraquat and Cyanide in Water Based on Pillar[5]arene/10-Methylacridinium Iodide Molecular Recognition. Chem. Commun. 50, 5064–5067. doi:10.1039/C4CC01403K
Wu, L., Han, C., Jing, X., and Yao, Y. (2021). Rim-Differentiated Pillar[5]arenes. Chin. Chem. Lett. 32, 3322–3330. doi:10.1016/j.cclet.2021.04.046
You, L., Zha, D., and Anslyn, E. V. (2015). Recent Advances in Supramolecular Analytical Chemistry Using Optical Sensing. Chem. Rev. 115, 7840–7892. doi:10.1021/cr5005524
Zhang, X., Chen, F., and Wang, M. (2014). Antioxidant and Antiglycation Activity of Selected Dietary Polyphenols in a Cookie Model. J. Agric. Food Chem. 62, 1643–1648. doi:10.1021/jf4045827
Keywords: pillararene, host-guest complex, fluorescence, indicator displacement assay, food additives
Citation: Duan Q, Xing Y and Guo K (2022) The Detection of Food Additives Using a Fluorescence Indicator Based on 6– p–Toluidinylnaphthalence-2-sulfonate and Cationic Pillar[6]arene. Front. Chem. 10:925881. doi: 10.3389/fchem.2022.925881
Received: 22 April 2022; Accepted: 12 May 2022;
Published: 31 May 2022.
Edited by:
Yong Yao, Nantong University, ChinaReviewed by:
Yincheng Chang, Beijing University of Chemical Technology, ChinaXie Han, Wuhan University of Science and Technology, China
Copyright © 2022 Duan, Xing and Guo. This is an open-access article distributed under the terms of the Creative Commons Attribution License (CC BY). The use, distribution or reproduction in other forums is permitted, provided the original author(s) and the copyright owner(s) are credited and that the original publication in this journal is cited, in accordance with accepted academic practice. No use, distribution or reproduction is permitted which does not comply with these terms.
*Correspondence: Qunpeng Duan, cXBkdWFuQGhhdWUuZWR1LmNu