- 1Department of Chemistry, Sir M. Visvesvaraya Institute of Technology, Affiliated to Visvesvaraya Technological University, Bengaluru, India
- 2Department of Biotechnology, School of Engineering & Technology, Sharda University, Greater Noida, India
- 3Department of Chemistry, Sai Vidya Institute of Technology, Affiliated to Visvesvaraya Technological University, Bengaluru, India
- 4Department of Pharmacology, School of Medical and Allied Sciences, KR Mangalam University, Gurgaon, India
- 5Department of Chemistry, PES College of Engineering, Affiliated to Visvesvaraya Technological University, Mandya, India
- 6Department of Chemistry, Nitte Meenakshi Institute of Technology, Affiliated to Visvesvaraya Technological University, Bengaluru, India
- 7Department of ECE, Surya Engineering College, Mettukadai, India
- 8Centre for Nano and Material Sciences, Jain University, Bengaluru, India
- 9Department of Bio Technology, Sir M. Visvesvaraya Institute of Technology, Affiliated to Visvesvaraya Technological University, Bengaluru, India
- 10Department of Biology, Faculty of Science, King Khalid University, Abha, Saudi Arabia
- 11Department of Botany and Microbiology, Faculty of Science, South Valley University, Qena, Egypt
- 12Department of Biotechnology, School of Applied and Life Sciences (SALS), Uttaranchal University, Dehradun, India
- 13Department of Biotechnology Engineering and Food Technology, Chandigarh University, Mohali, India
- 14Department of Pharmaceutics, School of Medical and Allied Sciences, KR Mangalam University, Gurgaon, India
- 15Department of ECE, Christu Jyothi Institute of Technology and Science, Janagon, India
Nanotechnology is a fast-expanding area with a wide range of applications in science, engineering, health, pharmacy, and other fields. Among many techniques that are employed toward the production of nanoparticles, synthesis using green technologies is the simplest and environment friendly. Nanoparticles produced from plant extracts have become a very popular subject of study in recent decades due to their diverse advantages such as low-cost synthesis, product stability, and ecofriendly protocols. These merits have prompted the development of nanoparticles from a variety of sources, including bacteria, fungi, algae, proteins, enzymes, etc., allowing for large-scale production with minimal contamination. However, nanoparticles obtained from plant extracts and phytochemicals exhibit greater reduction and stabilization and hence have proven the diversity of properties, like catalyst/photocatalyst, magnetic, antibacterial, cytotoxicity, circulating tumor deoxy ribo nucleic acid (CT-DNA) binding, gas sensing, etc. In the current scenario, nanoparticles can also play a critical role in cleaning wastewater and making it viable for a variety of operations. Nano-sized photocatalysts have a great scope toward the removal of large pollutants like organic dyes, heavy metals, and pesticides in an eco-friendly and sustainable manner from industrial effluents. Thus, in this review article, we discuss the synthesis of several metal nanoparticles using diverse plant extracts, as well as their characterization via techniques like UV–vis (ultraviolet–visible), XRD (X-ray diffraction), SEM (scanning electron microscopy), TEM (transmission electron microscopy), FTIR (Fourier transform infrared spectroscopy), etc., and catalytic activity on various hazardous systems.
Introduction
Clean water is important for human health and survival. Many countries are currently suffering from a shortage of drinking water. Water scarcity is not only the result of increased population densities, but also due to expansion of several manufacturing sectors such as textiles, automobiles, petrochemical, electronics, food, pharma, etc. (US Environmental Protection Agency, 1998b; World Health Organization, 2008; Herrera et al., 2013). As a result, water reuse has become an important issue in today’s global resource management system. In many developing countries, the industry accounts for about 22% of the world’s total water usage, but more than 70% of untreated industrial waste is simply wastewater (Lin et al., 2007). Dyes typically constitute a major pollutant in waste water. Textile and dye wastes are extremely dangerous to aquatic life and the surrounding environment (Ghorai and Biswas, 2013; Wang et al., 2014a). Such effluents are highly hazardous due to their toxicity, strong colour, high chemical oxygen demand (COD) content, and poor bio-degradability (Jing et al., 2011). Usually, naphthol orange (NO), methylene blue (MB), methyl orange (MO), congo red (CR), acid orange (AO), malachite green (MG), eosin Y (EY), and rhodamine B (RhB) are utilized in a variety of industries, including textile, food, and pharmaceutical. To control the contamination of the ecosystem by such hazardous chemicals, researchers have been exploring cost-effective degradation via photocatalytic techniques, which have been widely used over the last two decades as a strategy, especially for dye decomposition in wastewater and effluents (Binding and Steinbach, 1970; Daneshvar et al., 2005; Zhang et al., 2011; Wang et al., 2012; Zhang et al., 2012).
Nanoparticles (NPs) are a class of materials with properties distinctively different from their bulk and molecular counterparts. NPs are considered to be the building blocks for nanotechnology, and are referred to particles with at least one dimension less than 100 nm. Nanomaterials and nanotechnologies have attracted tremendous attention in recent years, due to their effective applications, ranging from traditional chemical to medicinal and environmental technologies (Sevilla et al., 2013; Shume et al., 2020). NPs with dimensions ranging from 1 to 100 nm that exhibit excellent qualities in terms of huge ratio of surface and volume, surface morphology and size (Afsharian and Khosravi-Daran, 2019; Fagier, 2021), have been used by several industries and humankind for thousands of years (Biswas and Wu, 2005). NPs surfaces themselves may be distinctive (Banfield and Zhang, 2001). However, there has been a recent resurgence because of the ability to synthesize on large scales and characterize with accuracies, so as to manipulate their surface properties via the particle’s atomic, electronic, and magnetic structures, physical and chemical natures, and reactivity relative to the bulk material (Cho et al., 2013).
A nanoparticle can be either a zero dimensional where the length, breadth and height is fixed at a single point as in nano dots; one dimensional where it can possess only one parameter like in graphene; two dimensional where it has length and breadth, as seen in carbon nanotubes; or three dimensional where it has all the parameters such as length, breadth and height as in gold NPs (Anu Mary Ealias and Saravanakumar, 2017). Hence, NPs are a class of materials with conspicuous properties distinctively different from their bulk and molecular counterparts. Their distinct functional qualities make them a viable candidate for a variety of applications for photocatalysis, catalysis, sensor technology, magnetic property, DNA binding, food, engineering, medical, disease diagnosis and treatment, anti-carcinogenic, antioxidant, anti-tubercular, and antimicrobial (Li et al., 2011; Bera and Belhaj, 2016; Sabouri et al., 2019; Sapawe et al., 2019; Ghosh et al., 2020a; Pillai et al., 2020; Abdullahi Ari et al., 2021; Chandraker et al., 2021a).
Organic compounds discovered as contaminants in industrial or domestic wastewater effluents must be eliminated or destroyed before being discharged into the environment (Prashanth et al., 2022a). The presence of such pollutants in ground and surface water necessitates treatment in order to reach acceptable drinking water quality. The rising public concern about these environmental contaminants has necessitated the development of novel treatment approaches, with photocatalysis gaining a lot of interest in the pollutant degradation sector. An ideal photocatalyst should be stable, inexpensive, non-toxic, and, of course, highly photoactive.
Interestingly, recent literature do not present the complete picture of photocatalytic activity of metal NPs synthesized with the aid of plant extracts, and hence, this review paper tries to address the gaps, by providing insights into the development of novel NPs towards treatment of dye-contaminated wastewater with low price and high efficiency, via photocatalysis.
Why Green Approach for NPs Synthesis?
NPs are synthesized by two methods: 1) top-down approach and 2) bottom-up approach. In top-down method, bulk materials are transformed to NPs through sputtering technique, grinding, and milling. In bottom-up method, NPs are synthesized through physical, chemical, and biological methods. Different physical and chemical methods include electrochemical changes, chemical reduction, spray pyrolysis, sonication, arc discharge, pulse wire discharge, pulsed laser ablation, radiation, electro-deposition, evaporation–condensation, vapor and gas phase, ball milling, lithography, photochemical reduction, sol–gel, pyrolysis, and combustion, etc. Microwave, precipitation, hydrothermal, combustion, and sonochemical methods are commonly employed for the preparation and stabilization of metallic NPs (Chen et al., 2001; Geethalakshmi and Sarada, 2012; Jamkhande et al., 2019, Krishna et al., 2016a; Prashanth et al., 2016b; Krishna et al., 2017a; Krishna et al., 2017b; Sathyananda et al., 2021a).
Since processes such as kinetics of the metal ion interaction with a reducing agent, the adsorption process of a stabilizing agent with metal NPs, and diverse experimental methodologies have a substantial influence on the shape of metallic NPs; the method of synthesis of NPs is very crucial (Vijayakumar et al., 2013; Ahmed et al., 2022).
There are several disadvantages of chemical methods like they are being toxic, harmful for society, high cost, etc. Chemical methods for the synthesis of metal NPs have been identified as toxic for the environment as well as the synthesis involves high temperature and pressure (Irshad et al., 2021). Further, biomedical applications of these NPs could be toxic (Thirumurugan et al., 2010; Herizchi et al., 2016; Muddapur et al., 2022).
Green synthesis is a subset of green chemistry, born after recognizing the need for sustainable processes in the chemical industry, with the goal of developing safer chemical products and processes that reduce or eliminate the generation and use of hazardous elements. Therefore, there are two goals for green synthesis of nanomaterials: 1) Creating nanomaterials to solve environmental problems, by preventing damage from known pollutants, or by incorporating nanomaterials into environmental technologies to clean up contaminated environments (Ahmed et al., 2022) and 2) minimize damage to human health and environment due to human activities (Sivaraj et al., 2015).
The biological approach of synthesis of NPs follows green chemistry because it is free of toxic substances, clean, and energy-efficient. Biological synthesis derives the creation of the basic principles of green chemistry such as prevention, less hazardous chemical syntheses, use of renewable feedstock, reductive derivatives, etc. As far as the biological approach is concerned, NPs are synthesized using fungi, bacteria, plant, animal sources, etc. The synthesis of NPs from plant extracts has more advantages than other biological sources due to the expensive maintenance of cultures and microbial isolation (Chandraker et al., 2019). Plant extract is commonly used as a crucial component for the NPs synthesis due to its safety and feasibility. Proteins, carbohydrates, enzymes, phenolic acids, and alkaloids are found in plants and are used in the reduction and stabilization processes. Plants are biological substrates that are rich in numerous critical phytochemicals, and hence decrease the need for chemicals as reducing, capping, and stabilising agents during the production of metal NPs from their respective precursor solutions (Singh, 2022).
Furthermore, because leaves contain a lot of metabolites, green synthesis usually uses leaf extracts and this route is safe, bio-compatible, and environment friendly (Thirumurugan et al., 2010; Irshad et al., 2021).
Synthesis process of NPs by various approaches is depicted in Figure 1. In this review article, we highlight the green synthesis process of various metal NPs and their characterization by different techniques such as XRD, FTIR, SEM, TEM, and UV–vis spectroscopy. Apart from this, we highlight their photocatalytic property against different organic pollutant dyes such as NO, MB, MO, CR, RhB, MG, EY, acid orange (AO), and the possible mechanism involved. We believe this review article will be helpful to the new researchers in this field to understand the phenomenon of metal NPs synthesis by green approaches and their photocatalytic activity.
Synthesis Process of NPs by Plant Extracts
Preparation of Plant Extract
In this process, leaves collection from plants is a crucial step. Herbal plants are identified by a subject expert before they are collected. Fresh leaves are cleaned with running water followed by double distilled water (DDW), chopped into very small pieces, and dried in shade or in the presence of sunshine. To prepare the extraction, usually a known weight of the powdered leaves (5, 10 g) is soaked in a known volume of DDW (100 mL) and incubated at 40–50°C for 80–100 min. Further, the leaf extract is stored at ambient temperature for cooling before being filtered using Whatman (No. 41) filter paper.
Phytochemical Tests
Plants are ubiquitous in nature, and many of them have yet to be fully studied for their great health benefits. Plants contain a wealth of essential phytochemicals with therapeutic and medical capabilities (Rizwana et al., 2022).
The medicinal properties of various medicinal plants are due to the phytochemicals contained in them. These phytochemicals are the primary source for treating devastating illnesses. Various phytochemicals have a wide range of activities that help strengthen the immune system and provide long-term disease resistance to protect the body from harmful pathogens (Khalid et al., 2018).
Phytochemical test is the more important aspect in the NPs synthesis from plant extracts. Phytochemical tests reveal the chemical presence in plant extracts. These tests should be performed by the standard protocol (Harbone, 1999; Kokate, 2000; Tiwari et al., 2011; Prashanth and Krishnaiah, 2014a; Prashanth et al., 2015a; Prashanth and Krishnaiah, 2014b). Table 1 provides the different phytochemical tests that are performed for any plant extract. The + ve and -ve signs indicate the presence and absence of the phytochemical of the plant extract, respectively. To examine and investigate the constituents present, phytochemical tests require to be performed.
Synthesis of NPs
The nature and concentration of the plant extract, pH, temperature, metal salt concentration, and contact time are known to affect the rate of NPs production (Christopher et al., 2015; Ekennia et al., 2022).
Proper amount of metal salt is dissolved in DDW, and appropriate amount of plant extract is added to it. The mixture is stirred for the accurate time, may be at a normal temperature or at 40–80°C. The synthesized NPs are initially observed by visual color change and detected by UV–vis spectroscopy. Finally, the formation of NPs is confirmed by other characterization techniques such as XRD, IR, SEM, and TEM. Plant extracts contain several phytochemicals like anthraquinone, flavonoids, anthocyanine, tannins, phytosterols, phlobatannins, alkaloids, phenols, glycosides, terpenoids, saponins, carbohydrates, and steroids. The phytochemicals from the leaves extract of the plants work as stabilizing and capping agents for synthesizing NPs and in the reduction of Mn+ to Mo, as shown in Figure 2. Therefore, capping, stabilizing, and reducing agents are not required for the synthesis of NPs from plant extracts. This is one of the most important advantages of green synthesis of NPs.
Few Reported NPs Synthesis From Plant Extracts
Sandip Kumar Chandraker et al. synthesized silver (Ag) NPs by using Justicia adhatoda (JA) leaf extract. They initially confirmed the formation of JA@Ag NPs by UV–vis spectroscopy and finally confirmed the same by XRD, IR, SEM, and TEM analyses. The nano-size of JA@Ag NPs was 19 nm, which was supported by SEM and TEM analyses. The spherical shape NPs had a semiconducting property with an optical band gap of 3.3 eV (Chandraker et al., 2021b).
Ghosh et al. synthesized copper (Cu) NPs by using the leaf extract of Jatropha curcas (JC). The JC-Cu NPs were characterized by XRD, UV–vis spectroscopy, FT-IR, SEM, and TEM (Ghosh et al., 2020b). The average crystallite size was 12 ± 1 nm. The JC-Cu NPs exhibited several applications like photocatalysis, semiconductor property, and CT-DNA binding (Ghosh et al., 2020b). Their band gap was found to be 3.6 eV at 337 nm, as shown in Figure 3A (blue: plant extract (JC); red: cuprous chloride; and black: JC-Cu NPs (Ghosh et al., 2020b). Jagpreet Singh et al. synthesized zinc oxide (ZnO) NPs, making use of aqueous leaves extract of Coriandum sativum. A sharp absorption peak observed at 364 nm confirmed the formation of zinc oxide (ZnO) NPs, as shown in Figure 3B. The optical band gap determined from absorption spectra was 3.4 eV, which is higher than that of bulk ZnO (3.32 eV). These NPs were employed for the photocatalytic degradation of yellow 186 dye under direct sunlight (Singh et al., 2019a).
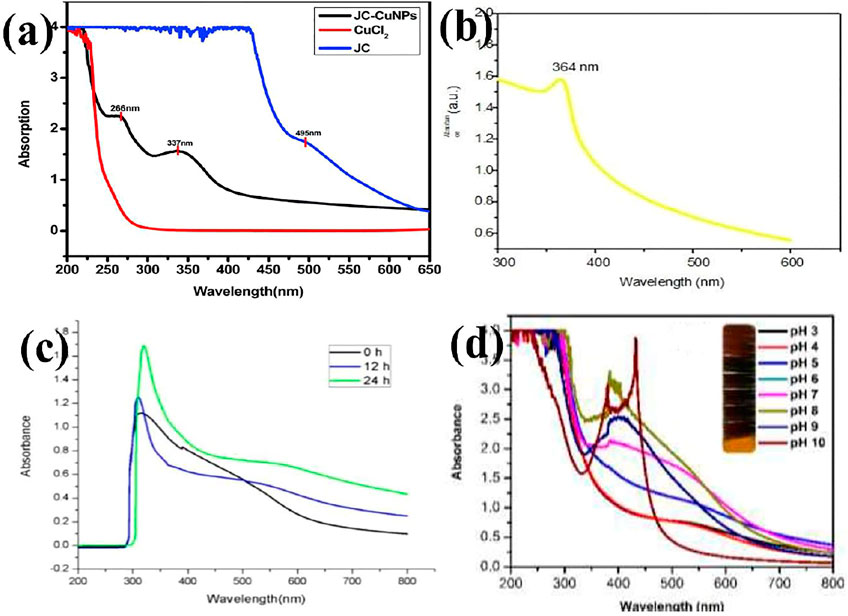
FIGURE 3. UV–vis absorption spectra of (A) JC-Cu NPs (Ghosh et al., 2020b), (B) ZnO NPs (Singh et al., 2019a), (C) Se NPs at different time intervals (0, 12, and 24 h) (Alagesan and Venugopal, 2019), and (D) optimizing parameters to produce Ag2O NPs with different pH values by UV–vis spectroscopy (Manikandan et al., 2017).
Selenium (Se) NPs were synthesized by Venkatesan Alagesan and Sujatha Venugopal with the aid of aqueous leaves extract of Withania somnifera (Alagesan and Venugopal, 2019) The UV–vis spectrum of Se NPs at different time intervals (0, 12, and 24 h) is given in Figure 3C. The synthesized Se NPs were tested for the antioxidant activity, antibacterial activity against pathogenic bacterial strains, Bacillus subtilis, Escherichia coli, Klebsiella pneumoniae, and Staphylococcus aureus, antiproliferative activity, and photocatalytic activity on MB under sunlight. The results suggested that these NPs effectively degraded MB under sunlight irradiation.
Silver oxide (Ag2O) NPs were synthesized using Artocarpus heterophyllus rind extract, and their antifungal activity was evaluated. Their UV–vis absorption spectra are presented in Figure 3D (Manikandan et al., 2017).
Jyoti et al. synthesized Ag NPs through 10–3 M of 95% silver nitrate (AgNO3) and 5% leaves extract of Zanthoxylum armatum at 40°C. They initially monitored the formation of NPs by visual color change from brownish to colorless, which was confirmed by UV–vis spectroscopy. Finally, the formation of NPs was asserted by XRD, TEM, SAED, and SEM–EDX (energy-dispersive X-ray spectroscopy). Sizes of the NPs were found to be from 10 to 50 nm. The NPs had a potential photocatalytic activity against organic pollutant dyes (Jyoti and Singh, 2016).
ZnO NPs were synthesized by the solution combustion method with the aid of Abutilon indicum (AI), Melia azedarach (MA), and Indigofera tinctoria (IT) leaves extracts with their different concentrations. The average crystallite sizes of the samples were found to be 7, 11, and 12 nm (with different volumes of AI), 7, 9, and 18 nm (with different volumes of MA), and 8, 11, and 12 nm (with different volumes of IT). The samples were characterized by XRD, SEM, TEM, X-ray photoelectron spectroscopy, thermogravimetric analysis, and Brunauer–Emmett–Teller (BET). They were found to exhibit anticancer activity against DU-145 and Calu-6 cancer cell lines (Prashanth et al., 2018).
Lemon juice was employed as a reductant in synthesizing ZnO NPs (Gopala Krishna et al., 2017; Prashanth et al., 2019; Prashanth et al., 2020). The samples were characterized by XRD, SEM with EDS (energy-dispersive spectroscopy) and elemental mapping, TEM, and BET. The samples were tested against DU-145, Calu-6, PC-3, HCT116, A549, MDA-MB-231, and MCF-7 cell lines. The green synthesized ZnO NPs were found to possess antitubercular activity against Mycobacterium tuberculosis H37 Ra. They were also found to possess antibacterial activity against different organisms.
ZnO NPs were synthesized from the aqueous fruit extracts of Punica granatum and Tamarindus indica (Prashanth et al., 2015b). The samples were found to have crystallite sizes ranging from 4-20 nm. They were tested against bacterial strains and MCF-7 cell line.
Synthesis of cobalt (Co)-doped copper oxide (CuO) NPs was carried out using lemon juice as a reductant, and the samples were characterized by XRD and SEM with EDS and elemental mapping. They were tested against Mycobacterium tuberculosis, H37Rv, Mycobacterium abscessus, Mycobacterium fortuitum, Mycobacterium chelonae, and anticancer activity on MDA-MB-231 (Sathyananda et al., 2021b).
ZnO NPs were synthesized from Syzgium cumini (S. cumini). FTIR spectroscopy analysis ascertained the existence of flavonoids, phenolic acids, enzymes, and steroids in leaves extract of S. cumini which aided in reducing zinc salt into zinc ions and acted as capping agents during the synthesis of NPs. The NPs were tested for seed germination and used as a catalyst for the degradation of RhB (Rafique et al., 2022).
Prashanth et al. reported the synthesis of Ag/CuO nanocomposites by solution combustion method using lemon juice as a reductant (Prashanth et al., 2022b). The samples were subjected to investigation for their antimycobacterial activity on Mycobacterium tuberculosis H37Rv, Mycobacterium abscessus, Mycobacterium fortuitum, Mycobacterium chelonae, anticarcinogenic activity on breast cancer cell line MDA-MB-231, and scavenging activity by the 2, 2-diphenyl-1-picrylhydrazyl hydrate (DPPH) method (Prashanth et al., 2022b).
Several mechanisms have been proposed to explain the antimicrobial mechanism of different metal NPs: inhibition of cell wall/membrane synthesis, disruption of energy transduction, production of toxic reactive oxygen species (ROS), photocatalysis, enzyme inhibition, and reduced DNA production (Weir et al., 2008; Shaikh et al., 2019). The probable antimicrobial mechanism of different metal NPs as explained by Shaikh et al. is shown in Figure 4 (Shaikh et al., 2019).
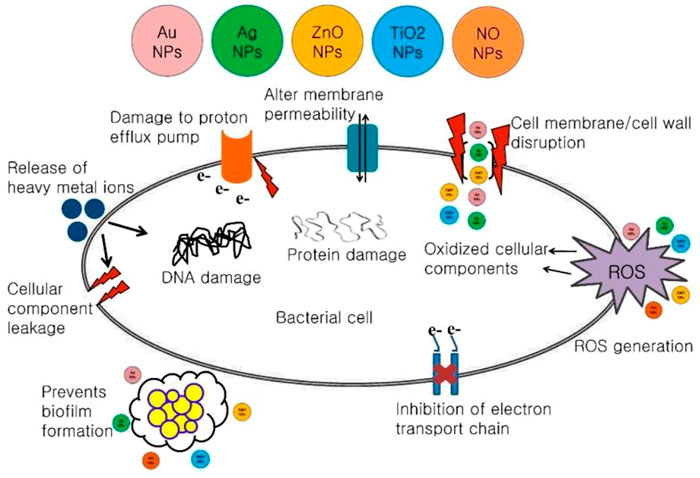
FIGURE 4. Probable antimicrobial mechanism of different metal NPs (Shaikh et al., 2019).
The anticancer potential of these NPs was attributed to the production of ROS in cellular compartments that eventually leads to the activation of autophagic, apoptotic, and necrotic death pathways (Andleeb et al., 2021). The plausible anticancer mechanism of CuO NPs proposed by Letchumanan et al. is presented in Figure 5 (Letchumanan et al., 2021).
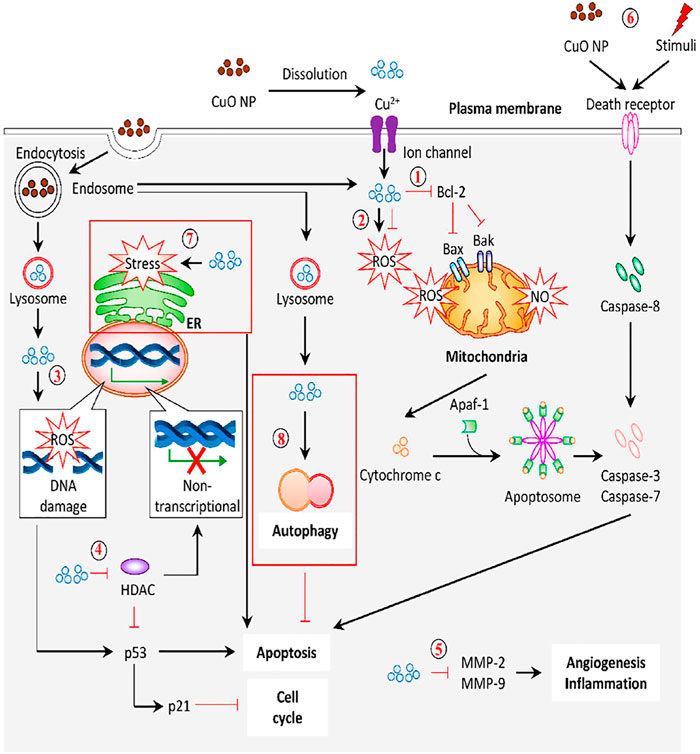
FIGURE 5. Proposed anticancer mechanism of metal oxide NPs (Letchumanan et al., 2021).
In summary, during the last decade, many efforts have been made in developing variety of metal NPs by green routes, making use of plant materials as reducing/stabilizing agents. Leaves, root, bark, stem, and fruits of various plants have been employed to develop different metal NPs, which possess multiple applications. The distinctive features of NPs synthesized using plant extracts enhance their applications in many fields. Efforts need to be intensified in manipulating the morphological characteristics of NPs by means of various parameters such as the nature of the plant materials, reaction time, reactant concentrations, pH, and temperature. Such parameters are very important to understand their crucial roles and effects during the synthesis of NPs.
Characterization
Any scientific research work depends on the characterization of the samples. The formation of NPs, their size, morphology, and surface area are determined from the instrumental characterization. NPs are characterized by different techniques like FTIR, UV–vis spectroscopy, SEM, TEM, energy-dispersive X-ray (EDX), and XRD. The UV–vis spectra confirms the formation of NPs by comparison peaks of metal salt and leaves extract. The XRD analysis is of elucidating the crystal parameter, structure, and size. The SEM analysis is for measuring the surface morphology of the NPs and size. The TEM determines the exact size of the synthesized NPs. The EDX defines the percentage of elements present in the NPs. UV–vis spectroscopy, FTIR spectroscopy, TEM, and SEM are among the few that are the commonly used techniques for NPs characterization (Chandraker et al., 2021c).
XRD Analysis
Ghosh et al. characterized the JC-Cu NPs by XRD as shown in Figure 6A (Ghosh et al., 2020b). They calculated the nano-size from XRD, and it was found to be 12 ± 1 nm. Figures 6B–D describe the XRD analysis of Ag NP synthesized using Cydonia oblong seed extract (CO-Ag NPs) (Zia et al., 2016), Ageratum conyzoides leaf extract (AC-Ag NPs) (Chandraker et al., 2019) and Ageratum houstonianum leaf extract (AH-Cu NPs) (Chandraker et al., 2020) respectively. The crystallite sizes of CO-Ag NPs and AC-Ag NPs were 38 and 35 nm, respectively.
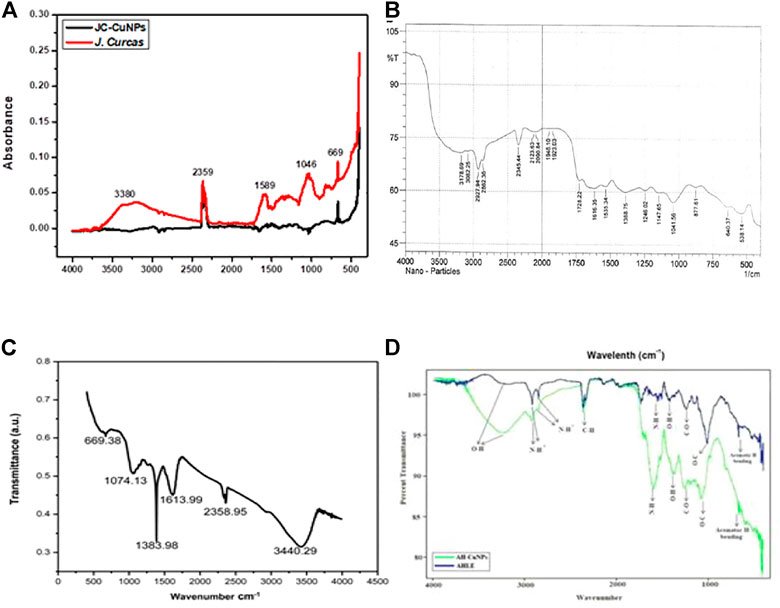
FIGURE 6. FT-IR spectra of (A) JC-Cu NPs (Ghosh et al., 2020b), (B) CO-Ag NPs (Zia et al., 2016), (C) AC-Ag NPs (Chandraker et al., 2019), and (D) AH-Cu NPs (Chandraker et al., 2020).
FT-IR
The absorption bands for JC-Cu NPs at 418, 669, 1046, 1589, 2359, and 3380 cm−1 were due to C-Cl, C-O, C=N, C-H, and O-H functional groups, as depicted in Figure 7A (Ghosh et al., 2020b). The functional groups in JC might be accountable for the bio-reduction of Cu+ to JC-Cu NPs (Ghosh et al., 2020b). The peak for the NH stretching was observed at 3178.60 cm−1. The peaks at 3082.25 and 2862.36 cm−1 were associated with the symmetric CH2 stretching (Zia et al., 2016). The peak at 2927.94 cm−1 was associated with the CH2 asymmetric stretching. The peak at 2123.63 cm−1 indicated the C:C stretching and that at 1948.10 cm−1 was associated with allenes (C:C:C). The peak for the carbonyl group was obtained at 1728.22 cm−1. The carbonyl groups proved the presence of flavanones or terpenoids that are adsorbed on the surface of the metal nano-sized particles by interaction through p-electrons in the carbonyl groups in the absence of a sufficient concentration of chelating agents [Figure 7B (Zia et al., 2016)].
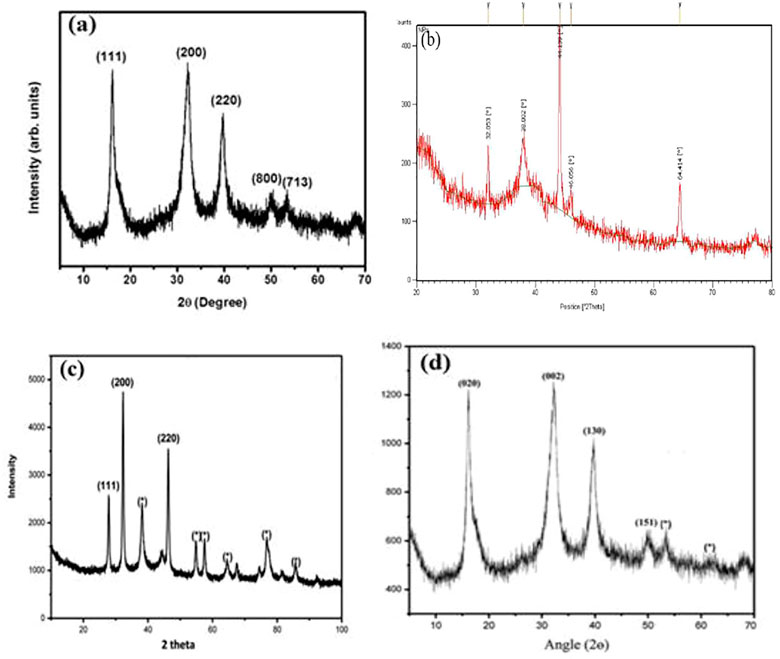
FIGURE 7. XRD analysis of (A) JC-Cu NPs (Ghosh et al., 2020b), (B) CO-Ag NPs (Zia et al., 2016), (C) AC-Ag NPs (Chandraker et al., 2019), and (D) AH-Cu NPs (Chandraker et al., 2020).
The IR-spectrum at 1074.83, 1383.98, 1613.99, 2358.95, and 3440.29 cm−1 were identified as C-OH, alcohol/ethers/esters/carboxylic acids/amino acids, C=O, C-H, N-H stretching respectively, as shown in Figure 7C (Chandraker et al., 2019).
The IR-spectra at 3236.34, 2918.71, 2359.93, 1595.13, 1377.76, 1260.17, 1074.64, and 667.81 cm−1 were identified as the O–H stretch, N+-H, C-H, N-H, O-H, C-O, O-C, and aromatic H respectively [Figure 7D (Chandraker et al., 2020)].
SEM
The morphology and shape of NPs are described by the SEM images. Few SEM images of NPs obtained from plant extracts are presented in Figures 8A–D. SEM images showed that Cydonia oblong (CO)-Ag NPs have been formed and Ag+ ions have been completely consumed, as presented in Figure 8B (Chandraker et al., 2019). The shapes of AH-Cu NPs were hexagonal, rectangular, and cubic (Figure 8D) (Chandraker et al., 2020).
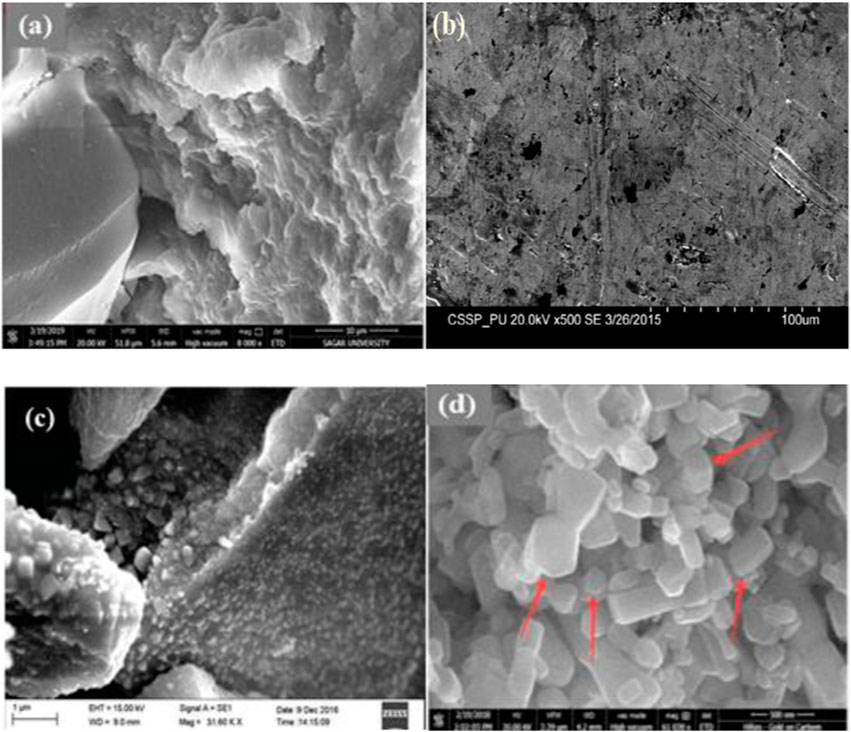
FIGURE 8. SEM analysis of (A) JC-Cu NPs (Ghosh et al., 2020b), (B) CO-Ag NPs (Zia et al., 2016), (C) AC-Ag NPs (Chandraker et al., 2019), and (D) AH-Cu NPs (Chandraker et al., 2020).
Photocatalytic Activity
Optical Band Gap
The optical band gap of NPs is a critical factor in photocatalytic activity. The optical band gap may predict the photocatalytic activity of the NPs. When the band gap value is less than 5 eV, it favors the photocatalytic activity of the NPs. The optical band gap is calculated from the UV–vis spectrum of the NPs. It is calculated by using Tauc’s formula, as shown in Eq. 1 (Ghosh et al., 2020b):
where α is the absorption coefficient, h is Plank’s constant, υ is the frequency, A is a constant, Eg is the band gap, and n signifies the exponent value.
Ghosh et al. reported that the optical band of JC-CuNPs was 3.6 eV and it was very active in photocatalysis (Ghosh et al., 2020b). Chandraker et al. reported a band gap value of 3.33 eV for Ag NPs synthesized from plant extract (Chandraker et al., 2021c). From the plot (Figure 9), the band gap energy of Se NPs was found to be 2.75 eV (Alagesan and Venugopal, 2019).
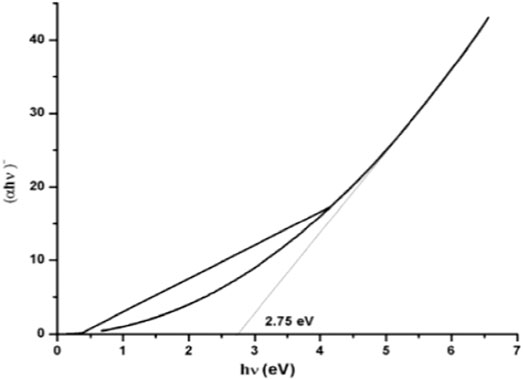
FIGURE 9. Band gap energy of Se NPs at 24 h (Alagesan and Venugopal, 2019).
Method of Photocatalytic Reaction by NPs
Photocatalytic tests are carried out by employing NPs, an aqueous solution of organic dye, and in the presence of sunlight. The reactions are carried out by the addition of NPs as a photocatalyst (0.05 g) into each batch of 30 mL aqueous solution of the organic dyes, and then the reaction mixtures are exposed to sunlight. The reaction mixtures are monitored by UV–vis spectroscopy at a constant time interval (Jiang et al., 2015).
Kinetics of the Reaction
Pseudo-first-order, pseudo-second-order, and Langmuir–Hinshelwood kinetic expressions are widely used to describe the kinetics study of the photocatalytic oxidation of organic compounds. The reaction of kinetics can be evaluated by Eq. 2 (Ahmad and Majid, 2018):
where k is the rate constant of pseudo-first-order, [A0] is the initial concentration of organic dyes at time t = 0, and [A] is the concentration of organic dyes at time t. The rate constant of the reaction is determined by plotting ln [A]/[A0] vs. t and slope with rate constant (k).
Efficiency of Degradation of Dyes by NPs
According to Beer’s law, the concentration of the organic dye is linearly proportional to the intensity of the dye’s absorption peak and the effectiveness of NPs for organic dye degradation, which may be computed as follows (Eq. 3) (Jayappa et al., 2020):
where At is the absorbance at t time and A0 is the initial absorbance at zero time.
The Possible Mechanism
The first step involves the adsorption of the dye onto the surface of NPs and the generation of electron hole (e−/h+) in the presence of sunlight (Eq. 4). The electron (e−) in conductance band (CB) reacts with oxygen and superoxide anionic radical (O2−) (Eq. 5). The hole (h+) in the valence band (VB) reacts with water molecules and forms a hydrogen ion (H+) and hydroxyl anion (OH−) (Eq. 6). At the same time, another hole (h+) reacts with water molecules and produces hydroxyl radicals (OH) (Eq. 7). Concurrently, dye is activated by the absorption of sunlight and produces dye* (Eq. 8) while instantaneously discharging electrons to form dye+ (Eq. 9). The dye+ reacts with highly unstable species, hydroxyl radicals (OH.) and superoxide anionic radical (O2−), and produces the degradation products in the form of CO2 and H2O (Eqs 10, 11) (Lam et al., 2012; Ibhadon and Fitzpatrick, 2013; Ong et al., 2018). The possible mechanism is shown in Figure 10:
Some Case Studies of Photocatalytic Activity of Green Synthesized NPs
Cu NPs were synthesized by the green method. The optical band gap (4.5 eV) favored the photocatalytic activity. CR was degraded by AH-CuNPs, and the rate of reaction was 3.1 × 10–4 s−1 (Chandraker et al., 2020).
CuO NPs were synthesized by using plant extract of Ruellia tuberosa and Abutilon indicum. CuO NPs exhibited photocatalytic activity against Crystal Violet (CV) and Acid Black 210 (Ijaz et al., 2017; Vasantharaj et al., 2019a).
Biogenic synthesis of CuO NPs using Psidium guajava leaf extract as a reducing agent has been reported (Singh et al., 2019b). The CuO NPs exhibited excellent degradation efficiency for the industrial dyes, i.e., Nile blue (NB) (93% removal in 120 min) and reactive yellow 160 (RY160) (81% removal in 120 min) with apparent rate constants of 0.023 and 0.014 min−1, respectively. The CuO catalyst was found to be reusable for photocatalytic dye degradation even after five consecutive cycles.
Biocompatible CuO NPs were produced with the aid of Rubia cordifolia bark extract (Vinothkanna et al., 2022). The photocatalytic activity of the CuO NPs demonstrated the MB (81.84%) and CV (64.0%) dye degradation potentials, indicating the environmental bioremediation efficacy (Vinothkanna et al., 2022).
Phytocombustion method was used to produce CuO NPs, making use of Pouteria campechiana leaves extract (Navada et al., 2021). The NPs degraded MB and MO with magnificent degradation more than 84% and its reusability over five cycles owing to its stability (Navada et al., 2021).
CuO and Ag/CuO NPs were synthesized using Cyperus pangorei extract by co-precipitation method. The plant extract acted as a reducing and stabilizing agent. The photocatalytic activity of CuO and Ag/CuO NPs was analyzed against RhB under visible light irradiation. The presence of metallic silver on the surface of CuO increased the electron‐hole pair life and improved the effective catalytic activity (Parvathiraja and Shailajha, 2021).
Suresh ChandMali reported the photocatalytic degradation of MB by Cu NPs synthesized by the green approach using Celastrus paniculatus willd leaf extract. The experiment used a 10 mg L−1 concentration of MB mixed with 10 mg dosages of photocatalyst. Almost complete degradation of MB was seen for 120 min. In the presence of Cu NPs, the photodegradation was significantly enhanced at basic pH (pH = 9) (Mali et al., 2020).
MnO2, CuO, and Ag NPs were synthesized by Kalopanax pictus leaf extract. In this study, manganese dioxide (MnO2) NPs had potential photocatalytic activity against CR than the other two NPs (Moon et al., 2018).
SA-Ag NPs were synthesized by using AgNO3 and plant extract of Sonchus arvensis (SA). SA-Ag NPs had effective photocatalytic activity against MB due to a lower band gap (3.2eV) (Chandraker et al., 2021c; Chandraker et al., 2019).
BP-Ag NPs were formed by using AgNO3 and leaf extract of Bryophyllum pinnatum (BP). Spherical shaped BP-Ag NPs degraded MB and RhB in the presence of sunlight (Chandraker et al., 2021a).
Ag NPs were prepared by using a green route with extracts of onion, tomato acacia catechu, and their mixed extracts by Kishore Chand et al. (Chand et al., 2020). These Ag NPs were tested for the degradation of MO, MR, and CR room temperature. In the presence of Ag NPs prepared with onion and acacia catechu, MO and MR dyes were completely degraded within a very short reactive time of 20 min, whereas in the case of CR dye, it was 15 min. Ag NPs synthesized with the mixture had increased effect on the degradation of all dyes as compared to Ag NPs synthesized alone in any one extract.
Green synthesis of Ag NPs using the leaf extract of Kalanchoe Daigremontiana was carried out, and the photocatalytic activity of the Ag NPs was evaluated through the degradation of MB, where the degradation time as low as 1 min was reported (Molina et al., 2019).
Shaikh et al. have reported the biosynthesis of Ag NPs using Shorea robusta leaf extract (Shaikh et al., 2020). The photocatalytic degradation process by the bio-synthesized Ag NPs exhibited a significant degradation potential for RhB (maximum degradation efficiency up to 90.41%) (Shaikh et al., 2020).
Green synthesis of Ag NPs from procera plant latex has been reported (Chandhru et al., 2021). The Ag NPs were applied as photocatalysts for the degradation of MO dye and also as anti-biofilm agents. The photocatalytic degradation efficiency was determined to be 0.0076.
Leaves of Cordia dichotoma were employed in the biosynthesis of Ag NPs (Dave et al., 2021). The degradation process of MO was completed at 70 h and was recognized by the change of reaction mixture color to colorless.
Rama Sharma et al. reported the synthesis of Ag NPs using Terminalia bellirica fruit extract (Sharma, 2021). The photocatalytic degradation of MB by these biosynthesized Ag NPs was evaluated by UV–vis spectroscopy.
Biosynthesis of Ag NPs using Berberis vulgaris extract was reported (Hashemi et al., 2022a). The results showed the average crystallite size of 45–60 nm and spherical morphology with only a few agglomerated particles. These NPs exhibited high photocatalytic degradation (97.82%) in short time on MO.
Panneerselvi et al. reported Mangifera indica resin-assisted synthesis of Ag NPs (Panneerselvi et al., 2022). The biosynthesized Ag NPs effectively degraded MB.
Green synthesis of Ag NPs was achieved using the Catharanthus roseus flower extract as the reducing agent (Muthu et al., 2021). These NPs had excellent photocatalytic activity in the reduction of methyl orange.
Synthesis of Ag NPs using Sambucus ebulus phenolic extract has been reported (Hashemi et al., 2022b). The biogenic Ag NPs were used as a nanocatalyst, which showed a high catalytic activity for the degradation of MO under sunlight irradiation.
Phytosynthesis of silver/silver chloride particles (Ag/AgCl) NPs was carried out using Cissus quadrangularis stem extract under microwave irradiation (Priyadarshini et al., 2021). Ag/AgCl NPs demonstrated a high photocatalytic activity toward the degradation of environmental pollutant MB under visible light illumination.
Ag/AgCl nanocomposites were obtained from the Azadirachta indica plant fruit extract (Panchal et al., 2021). The sample exhibited an excellent dye decomposition efficiency of 92.5% on MB in 80 min, which was better than the performance of Ag NPs and AgCl NPs.
Cadmium sulfide (CdS)-NPs were synthesized by Dicliptera Roxburghiana plant extract. It degraded MB in the presence of sunlight, and the efficiency of degradation was 87.12% (Ullah et al., 2021).
Copper–cobalt–nickel (Cu–Co–Ni) NPs were synthesized by Origanum vulgare leaves extract. The NPs had a photocatalytic activity against MB, and the order of reaction was pseudo-first-order (Alshehri and Malik, 2020).
Cobalt (Co) NPs were synthesized by Taraxacum officinale. Co NPs degraded the MO dye within 60 min in the presence of sunlight (Rasheed et al., 2019a).
α-ferric oxide (α-Fe2O3) NPs were synthesized by using ferric chloride and leaf extract of Carica papaya. Remazol yellow RR dye was degraded to 76.6% extent by α-Fe2O3 NPs in the presence of light (Bhuiyan et al., 2020).
Iron oxide NPs (IO NPs) were synthesized by Teucrium polium leaf extract. IO NPs had a photocatalytic activity against MO. The degradation efficiency was 73.6% within 6 hours (Kouhbanani et al., 2019).
Ferric oxide (FO) NPs were synthesized by Peltophorum pterocarpum leaf extract. MB was degraded by FO NPs in the presence of sunlight (Anchan et al., 2019).
FO NPs were formed with the aid of the leaf extract of Ruellia tuberosa. CV was degraded to 80% by FO NPs in the presence of sunlight (Vasantharaj et al., 2019b).
Iron-polyphenol nanoparticles (Fe-P-NPs) were synthesized by three plant extracts Melaleuca nesophila, Rosemarinus officinalis, and Eucalyptus tereticornis. Fe-P-NPs degraded 80% (AB 194) (Wang et al., 2014b).
Iron oxide (Fe3O4) NPs developed by different plant extracts (leaves of Mentha pulegium, Artemisia herba-alba, fruit peels of Punica granatum) were proved to possess catalytic activity for the dye degradation of MB and CR stains under environmental conditions. The NPs were proved to be useful in the treatment of wastewater/dye degradation (Meneceur et al., 2022).
Novel iron oxide NPs were produced, making use of Peltophorum pterocarpum plant extract (Shah et al., 2022). The as-prepared NPs effectively removed the RhB molecules with a high removal rate of 95.1% under UV irradiation.
Palladium (Pd) NPs were formed by the plant extract of Pimpinella tirupatiensis. Pd NPs had a photocatalytic activity against CR (Narasaiah et al., 2017).
ZnO NPs were synthesized by using the plant extract of Plectranthus amboinicus. ZnONPs showed photocatalytic activity against MR (Fu and Fu, 2015).
Panous extract-mediated ZnO nanoflowers were synthesized and tested for the removal of MB, eosin Y (EY), and MG (Kaliraj et al., 2019). The nanoflowers degraded the 15 mg L−1 MB, EY, and MG to >99% within 80, 90, and 110 min of contact time, respectively. In addition, the ZnO photocatalyst removed the low concentrated 5 mg L−1 of MB, EY, and MG within 30, 35, and 40 min of contact time, respectively (Kaliraj et al., 2019).
Aloe vera gel was used in the sol–gel synthesis of tin oxide and zinc oxide (SnO2-ZnO) nanocomposites. They were tested for their photocatalytic activity on MO (Sudhaparimala and Vaishnavi, 2016).
ZnO NPs synthesized using the leaf extract of Cyanometra ramiflora were tested for the photocatalytic degradation of pollutant dye, RhB. A remarkable degradation efficiency of 98% within 200 min was achieved under sunlight irradiation, and a degradation constant of 0.017 min−1 was obtained (Varadavenkatesan et al., 2019).
ZnO nanoflowers were obtained using Bridelia retusa leaf extract. Within a span of 165 min, under solar irradiation, ZnO NPs showed the photocatalytic degradation of RhB dye up to 94.74%. Exhibiting pseudo-first-order kinetics, the process had a degradation constant of 0.0109 min−1 (Vinayagam et al., 2021).
Karhik et al. reported the development of Cu-doped ZnO NPs from Synadium grantii plant extract (Karthik et al., 2022). The photocatalytic studies of the NPs were studied using MB, Indigo Carmine (IC), and RhB organic pollutants. 3% and 5% Cu-doped samples exhibited a superior photocatalytic activity for MB, IC, and RhB dyes.
Ag/ZnO nanocomposites were prepared using Urtica dioica leaf extract (Sohrabnezhad and Seifi, 2016). Their photocatalytic activity was assessed on MB.
Synthesis of nickel oxide (NiO), CuO, and ZnO was carried out by the solution combustion method, making use of Centella asiatica extract as a fuel/reductant (Alam et al., 2021). ZnO exhibited a greater photocatalytic activity than NiO and CuO on acid red 88 (AR88).
Cauliflower-shaped ZnO NPs were developed using Alchemilla vulgaris (Rajendrachari et al., 2021). The band gap of ZnO NPs was studied by UV–vis spectroscopy via Tauc’s method, and it was determined to be 3.27 eV. The photocatalytic activity of ZnO NPs was executed against RhB under the illumination of an AM 1.5 solar simulator.
ZnO- and manganese (Mn)-doped ZnO NPs using the seed extract of Cassia angustifolia were synthesized (Dhivya et al., 2022). The effective photocatalytic activity of ZnO NPs and Mn-doped ZnO achieved 84 and 95% decolorations of water pollutant MB dye, respectively, with H2O2 and UV radiation by the changing parameters like dye concentration, amount of catalyst, and pH.
Reduced graphene oxide-zinc oxide (RGO-ZnO) NPs were prepared using basilicum leaves extract (Raouf Malik et al., 2022). In photocatalytic activity, the ZnO NPs and RGO-ZnO NPs were tested as the catalyst and degraded the RhB dye 91.4% and 96.7% under UV–vis light. RGO-ZnO showed better results in photocatalytic activity against the pure ZnO NPs.
Binary metal oxide zinc oxide-cobalt oxide (ZnO-Co3O4) nanocomposites were prepared by the sol–gel method using the organic compounds of Abies pindrow plant leaves (Shaheen et al., 2022). The ZnO-Co3O4 catalyst exhibited excellent catalytic potential to degrade MO with 73% and 99% degradation efficiencies under dark and light conditions, respectively, within 15 min.
Ag-doped ZnO nanowires were developed using the medicinal plant Pedalium murex (Mangala Nagasundari et al., 2021). The NPs exhibited a photocatalytic degradation of about 92.43% of MB dye in 150 min under UV irradiation.
Biosynthesis of ZnO NPs making use of Sonneratia alba has been reported (Khan et al., 2022). The as-synthesized ZnO NPs showed excellent photocatalytic activity against Ethidium bromide (EtBr) and Brilliant green (BG) dye. Kinetic studies showed degradation efficiency toward EtBr (97.07%) and BG (95.01%).
SnO2-ZnO heterojunction nanocomposites were prepared using Urtica dioica leaf extract (Ebrahimian et al., 2021). The photocatalytic activity of the sample was investigated for the degradation of RhB under UV irradiation. According to the results, the RhB degradation efficiency was obtained to be 91.9% within 55 min, which can be compared with 91.7% at 190 min and 85% at 140 min over SnO2 and ZnO, respectively.
Cu-doped NiO NPs were synthesized by the Okra plant extract. XRD results showed that the size of NPs under optimal conditions (at the temperature of 400°C and % 3 Cu-doped) was 10.66 nm with a face-centered cubic structure (Ghazal et al., 2021). Photocatalytic activity of these NPs on MB, studied under UV light indicated 78% of degradation throughout 105 min.
The extract of the medicinal plant Tribulus terrestris was employed to synthesize NiO NPs (Khan et al., 2021). The ecotoxicity of CR and components derived from the dye was investigated using the Ecological Structure Activity Relationship (ECOSAR) program (Khan et al., 2021).
Ni/NiO NPs were prepared in an eco-friendly way using the seed extract of Lactuca Serriola (Ali et al., 2022). These NPs exhibited very good photocatalytic activity on CV.
Gold-titanium dioxide (Au-TiO2)-NPs were synthesized by the green method using Avverhoa bilimbi and Pandanus amaryllifolius. MB was degraded 80% by Au-TiO2-NPs, and the reaction followed the second order (Yulizar et al., 2019).
Acorus calamus leaf extract was used to develop TiO2 NPs (Ansari et al., 2022). The dye degradation activity of the synthesized NPs suggested that TiO2 NPs were more involved in RhB degradation than conventional hydrogen peroxide, which needs the use of a catalyst. Under visible light irradiation, the increased photocatalytic action of the biosynthesized TiO2 NPs was responsible for the degradation efficiency of 96.59% of the RhB.
Cedrous deodara extract was used to synthesize Cu@TiO2 NPs (Ramzan et al., 2021). Under sunlight irradiation, Cu@TiO2 NPs showed 95% of MB; on the other hand, it showed 73% dye degradation under visible light irradiation. The difference of dye degradation was because of the active participation shown by e− and h+ separations, which resulted in slowing the recombination rate of electron hole pairs as well.
Lemon peel extract was employed to synthesize TiO2 NPs for the first time (Nabi et al., 2022). The Tauc plot prepared from the UV–vis spectrum showed a band gap of 3.08 eV. The particles were spherical anatase crystals with the size 80–140 nm. The particles exhibited more than 70% photocatalytic activity on RhB.
Pulicaria undulata extract-mediated eco-friendly synthesis of TiO2 has been reported by Khaleel Al-hamoud et al (Al-hamoud et al., 2022). They were tested for the degradation of MB and MO under UV–vis light irradiation. Due to the small size and high dispersion of NPs, almost complete degradation (∼95%) was achieved in a short period of time (between 1 and 2 h). No significant difference in the photocatalytic activity of the TiO2 NPs was observed even after repeated use (three times) of the photocatalyst.
Magnesium oxide (MgO) NPs were developed using Texas sage plant extract (Ahmad et al., 2022). A characteristic metal and oxygen binding peak was observed at 296 nm in the FTIR spectrum confirmed the Mg-O formation. The 90% of degradation efficiency against MB was observed within 120 min of visible light irradiation (Ahmad et al., 2022).
Cobalt-doped cerium oxide (Co-CeO2) NPs were prepared in a media containing Salvadoral persica extract. The photocatalytic activity of Co-CeO2 NPs was tested on the AO7 dye, and it was observed that the amount of Co. doped CeO2 can be effective on the photocatalyst performance. The best photocatalytic performance was observed and recorded in the case of 7% Co. doped CeO2 NPs (Hamidian et al., 2021).
CeO2 NPs were prepared using banana peel. SEM results showed that the size of synthesized NPs was in the range of 4–13 nm (Miri et al., 2021). The experiment of photocatalytic activity showed that the synthesized NPs can remove 81.7% of AO7 in 180 min under visible light.
Green synthesis of ceria (CeO2) by Phoenix dactylifera (Ajwa dates), Coriandrum sativum (Coriander), Ocimum tenuiflorum (Black tulsi), Camellia sinensis (green tea), and Hibiscus flower has been reported (Iqbal et al., 2021). The photocatalytic activity of various green synthesized nanoceria for the photodegradation of amido black dye was investigated, and the optimum degradation was obtained for Phoenix dactylifera synthesized nanoceria.
Kannan et al. have reported the synthesis of zinc sulfide (ZnS) NPs using extracts of Acalypha indica and Tridax procumbens (Kannan et al., 2020). The samples were found to have excellent photodegradation efficiency for MB dye. From this investigation, 1.0 mg of ZnS-40 mL NPs exhibited an excellent MB dye degradation efficiency (98%) under 180 min of the visible light irradiation.
Au NPs were developed by green synthesis using green tea leaf extract as a reducing and stabilizing agent (Singh et al., 2021). These Au NPs were studied to understand their catalytic role in the photocatalytic degradation of MB under daylight conditions.
Tungsten oxide (WO3) NPs were prepared hydrothermally by basil leaves extract (Bayahia, 2022). WO3 NPs were examined for their photocatalytic activity on MB.
Tin tungstate (SnWO4)/ZnO nanocomposites were prepared using Muntingia calabura leaf extract (Elviera et al., 2022). Results of the photocatalytic experiment using MB as a model pollutant showed good results.
Copper iodide (CuI) NPs were synthesized using Hibiscus rosa-sinensis flower extract (Archana et al., 2022). Batch adsorption studies of Reactive Red 256 (RR) and Reactive Black 5 (RBL) were probed in the presence of CuI, and the respective adsorption capacity of RR and RBL were found to be 135.22 and 176.42 mg/g.
Biosynthesis of zirconium oxide (ZrO2) NPs was carried out using Wrightia tinctoria leaf extract (Al-Zaqri et al., 2021). The photocatalytic degradation of organic dye Reactive Yellow (RY 160) under sunlight irradiation using biosynthesized ZrO2-NPs was performed. The biosynthesized ZrO2-NPs exhibited 94% degradation for RY 160 dye for 120 min.
Platinum (Pt) NPs were synthesized by using Salix Tetraspeama leaf extract (Ramachandiran et al., 2021). The effective parameters on the Pt catalytic reduction were fully optimized under MB with the higher degradation efficiency being observed at 90%.
NiO nanosticks were synthesized with the aid of graviola leaf extract (Rajan et al., 2017). These nanosticks showed excellent photocatalytic activity on Rose Bengal dye (Rajan et al., 2017).
Cobalt ferrite (CoFe2O4) NPs were prepared using the aqueous solution of torajabin (Miri et al., 2022). The photocatalytic degradation activity of CoFe2O4 NPs was assessed on Acid Orange 7 (AO7) dye under simulated sunlight exposure where a high level of photodegradation was displayed by the NPs.
Ocimum tenuiflorum assisted green synthesis of CuO NPs has been reported (Sharma et al., 2021). Studies on photocatalytic activity indicated that CuO NPs could be employed as a photocatalyst in the degradation process MO. Up to 96.4 ± 0.83% degradation of MO was obtained in just 24 min.
The above examples very clearly indicate that various metals, metal oxide NPs, and composites could be obtained from green synthetic approaches that can be used for wastewater treatment. Distinctive methods are available to remove the organic dye contaminants from wastewater, including filtration, ion exchange, coagulation, aerobic/anaerobic decomposition, ozone treatment, and photocatalytic processes. Among these methods, nanoparticle photocatalysts show great potential as an alternative to physical, chemical, and biological methods. In addition, nanocatalysts are inexpensive, chemically stable, environmentally friendly, and rapidly oxidizable.
Photocatalytic Degradation of Pesticides and Oil by NPs Developed by Green Approaches
Heavy metals, fertilizers, and pesticides used in agriculture have lowered the availability of clear water for drinking and crop irrigation. Nanotechnology can play an essential role in ensuring the quality and purity of a long-term productivity system. Pesticide levels in water have risen as a result of their widespread use in contemporary agriculture. Pesticides have been shown in several studies to be hazardous to individuals and the environment. The type of pesticide and the performance of the treatment process influence which water treatment method is best for pesticide removal (Rasheed et al., 2019b).
One of the issues in water resource management is oil-contaminated water. To comply with the environmental authorities’ discharge requirements, it is critical to remove oil droplets from water. Apart from removing dyes from contaminated water, green synthesized NPs can be employed to remove pesticides and oils and they were found to be very effective.
Synthesis of flower-shaped CuO and NiO was carried out, making use of Capparis decidua leaf extract. These NPs were employed for the photocatalytic degradation of Lambda-cyhalothrin (L-CHT) (Iqbal et al., 2021c).
Aegle marmelos leaf extract was used to develop NiO NPs by the green approach. They were evaluated for their in vitro cytotoxicity against A549 cancer cell line. They were also proved to be an efficient photocatalyst for the degradation of 4-chlorophenol (Angel Ezhilarasi et al., 2018).
Josiane Peternela et al. reported the impregnation of CuO NPs on activated carbon, making use of Pomegranate leaf extract. Atrazine pesticide, diclofenac, caffeine, and nitrate were used as pollutants to test the adsorption capacity of the impregnated carbons (Peternela et al., 2017).
Pijushkanti Purkait et al. reported the synthesis of ZnO NPs using Trema orientalis leaf extract. They reported that the rate of photocatalytic degradation of zoxamide increased with the increasing concentration of ZnO from 10 mg/L to 50 mg/L (Purkait et al., 2020).
Synthesis of NiO NPs using Solanum trilobatum extract has been reported. They were examined for the photocatalytic degradation of 4-chlorophenol (Angel Ezhilarasi et al., 2020).
Papaya peel extract-mediated green synthesis of CuO NPs has been reported (Phang et al., 2021). The sample was found to be crystalline with a band gap energy of 3.3 eV. The photocatalytic activity of the sample was assessed by the photodegradation of Palm oil mill effluent (POME). The results revealed that CuO NPs had a significant photocatalytic performance in degrading POME with reduced phytotoxicity and can thus be used as a promising photocatalyst in POME wastewater treatment (Phang et al., 2021).
Bimetallic metal oxides namely nickel-copper oxide (NiCuO), copper-chromium oxide (CuCr2O4), and nickel-chromium oxide NPs were developed by the green approach with the aid of Aegle marmelos leaf extract. These NPs were evaluated for the removal of various harmful phenols (phenol, 2, 4-dinitrophenol, and 3-aminophenol) from simulated water (Rani and Shanker, 2018).
Graphene oxide–Ag nanoparticle composites were developed with the aid of Cucurbita maxima leaves extract. Photocatalytic degradation of Chlorpyrifos [O, O-diethy O-(3,5,6 trcholr-2-pyridyl) phosphorothioate)] was carried out with the produced graphene oxide–Ag NPs. (Chinnappa et al., 2022).
The photocatalytic activity of various green synthesized NPs against different dyes, pesticides, and oils is presented in Table 2.
Conclusion and Future Plan
Green synthesis technology is a clean, non-toxic, and environmentally friendly technology for synthesizing metal NPs and is of great interest due to its commercial perspective and feasibility. In this review, we document the production of different metal NPs from various plant extracts and their characterization by UV–vis spectroscopy, XRD, FT-IR, SEM, and TEM. When the optical band value is less than 5 eV, it favors the photocatalytic activity. The NPs from plant extracts had a very effective degradation activity on pollutant organic dyes and purification process on the contaminated water. Recent advances in research in metallic NPs suggest that the integration of metallic NPs and semiconductors is a promising strategy in the development of efficient visible light-responsive materials. Research on the challenges that arise in this area will lead to rapid progress in this area. It is reasonable to assume that such efforts will enable obvious breakthroughs in the development of metallic NPs and make them an important strategy in visible light photocatalysts. To date, the photocatalytic efficiency of the green synthetic photocatalysts available is noteworthy. However, their stability, and ease of use are still inadequate. To achieve the photocatalytic sustainability of these photocatalysts, scavengers must be added continuously to improve the photocatalytic efficiency. The lack of industrial application of green synthetic photocatalysts is mainly due to the reasons such as low yields of NPs, workshop setups on a scientifically relevant scale, and low recycling capacity of green synthetic photocatalysts. Thus, protocols need to be modified further for making these methods cost-effective and comparable with traditional methods for the large-scale production of efficient NPs. Improvement of reliable and eco-friendly processes for the synthesis of metallic NPs is a significant step in the field of applied nanotechnology. We believe this review will help new researchers in this field to understand the development of metal NPs in a cleaner way and their applications.
Author Contributions
PK conceived the concept of review. PK and HN wrote the article. All authors discussed, reviewed, and commented on the article.
Funding
The authors thank the Deanship of Scientific Research, King Khalid University, Saudi Arabia, for funding this work under Grant No. RGP. 2/90/43.
Conflict of Interest
The authors declare that the research was conducted in the absence of any commercial or financial relationships that could be construed as a potential conflict of interest.
Publisher’s Note
All claims expressed in this article are solely those of the authors and do not necessarily represent those of their affiliated organizations, or those of the publisher, the editors, and the reviewers. Any product that may be evaluated in this article, or claim that may be made by its manufacturer, is not guaranteed or endorsed by the publisher.
References
Abdullahi Ari, H., Adewole, A. O., Ugya, A. Y., Asipita, O. H., Musa, M. A., and Feng, W. (2021). Biogenic Fabrication and Enhanced Photocatalytic Degradation of Tetracycline by Bio Structured ZnO Nanoparticles. Environ. Technol. 2021, 1–16. doi:10.1080/09593330.2021.2001049
Afsharian, Z., and Khosravi-Daran, K. (2019). Application of nanoclays in food packaging Biointerface Res. Appl. Chem. 10 (1), 4790–4802. doi:10.33263/BRIAC101.790802
Ahmad, A., Khan, M., Khan, S., Luque, R., Almutairi, T. M., and Karami, A. M. (2022). Bio-construction of MgO nanoparticles using Texas sage plant extract for catalytical degradation of methylene blue via photocatalysis Int. J. Environ. Sci. Technol.. doi:10.1007/s13762-022-04090-2
Ahmad, J., and Majid, K. (2018). In-situ Synthesis of Visible-Light Responsive Ag2O/graphene Oxide Nanocomposites and Effect of Graphene Oxide Content on its Photocatalytic Activity. Adv. Compos Hybrid. Mater 1 (2), 374–388. doi:10.1007/s42114-018-0025-6
Ahmed, S. F., Mofijur, M., Rafa, N., Chowdhury, A. T., Chowdhury, S., Nahrin, M., et al. (2022). Green Approaches in Synthesising Nanomaterials for Environmental Nanobioremediation: Technological Advancements, Applications, Benefits and Challenges. Environ. Res. 204 (Part A), 111967. doi:10.1016/j.envres.2021.111967
Al-hamoud, K., Shaik, M. R., Khan, M., Alkhathlan, H. Z., Adil, S. F., Kuniyil, M., et al. (2022). Pulicaria Undulata Extract-Mediated Eco-Friendly Preparation of TiO2 Nanoparticles for Photocatalytic Degradation of Methylene Blue and Methyl Orange. ACS Omega 7 (6), 4812–4820. doi:10.1021/acsomega.1c05090
Al-Zaqri, N., Muthuvel, A., Jothibas, M., Ali, A., Alharthi, F. A., and Mohana, V. (2021). Biosynthesis of Zirconium Oxide Nanoparticles Using Wrightia Tinctoria Leaf Extract: Characterization, Photocatalytic Degradation and Antibacterial Activities. Inorg. Chem. Commun. 127. doi:10.1016/j.inoche.2021.108507
Alam, M. W., Aamir, M., Farhan, M., Albuhulayqah, M., Ahmad, M. M., Ravikumar, C. R., et al. (2021). Green Synthesis of Ni-Cu-Zn Based Nanosized Metal Oxides for Photocatalytic and Sensor Applications. Crystals 11, 1467. doi:10.3390/cryst11121467
Alagesan, V., and Venugopal, S. (2019). Green Synthesis of Selenium Nanoparticle Using Leaves Extract of Withania somnifera and Its Biological Applications and Photocatalytic Activities. BioNanoSci. 9, 105–116. doi:10.1007/s12668-018-0566-8
Ali, T., Farooq Warsi, M., Zulfiqar, S., Sami, A., Ullah, S., Rasheed, A., et al. (2022). Green Nickel/nickel Oxide Nanoparticles for Prospective Antibacterial and Environmental Remediation Applications. Ceram. Int. 48 (6). doi:10.1016/j.ceramint.2021.12.039
Alshehri, A. A., and Malik, M. A. (2020). Facile One-Pot Biogenic Synthesis of Cu-Co-Ni Trimetallic Nanoparticles for Enhanced Photocatalytic Dye Degradation. Catalysts 10 (10), 1138. doi:10.3390/catal10101138
Anchan, S., Pai, S., Sridevi, H., Varadavenkatesan, T., Vinayagam, R., and Selvaraj, R. (2019). Biogenic Synthesis of Ferric Oxide Nanoparticles Using the Leaf Extract of Peltophorum pterocarpum and Their Catalytic Dye Degradation Potential. Biocatal. Agric. Biotechnol. 20, 101251. doi:10.1016/j.bcab.2019.101251
Andleeb, A., Andleeb, A., Asghar, S., Zaman, G., Tariq, M., Mehmood, A., et al. (2021). A Systematic Review of Biosynthesized Metallic Nanoparticles as a Promising Anti-cancer-strategy. Cancers 13, 2818. doi:10.3390/cancers13112818
Angel Ezhilarasi, A., Judith Vijaya, J., Kaviyarasu, K., John Kennedy, L., Jothi Ramalingam, R., and Al-Lohedan, H. A. (2018). Green Synthesis of NiO Nanoparticles Using Aegle Marmelos Leaf Extract for the Evaluation of In-Vitro Cytotoxicity, Antibacterial and Photocatalytic Properties. J. Photochem. Photobiol. B Biol. 180. doi:10.1016/j.jphotobiol.2018.01.023
Angel Ezhilarasi, A., Judith Vijaya, J., Kaviyarasu, K., Zhang, X., and John Kennedy, L. (2020). Green Synthesis of Nickel Oxide Nanoparticles Using Solanum Trilobatum Extract for Cytotoxicity, Antibacterial and Photocatalytic Studies. Surfaces Interfaces 20. doi:10.1016/j.surfin.2020.100553
Ansari, A., Siddiqui, V. U., Rehman, W. U., Akram, M. K., Siddiqi, W. A., Alosaimi, A. M., et al. (2022). Green Synthesis of TiO2 Nanoparticles Using Acorus calamus Leaf Extract and Evaluating its Photocatalytic and In Vitro Antimicrobial Activity. Catalysts 12, 181. doi:10.3390/catal12020181
Anu Mary Ealia, S., and Saravanakumar, M. P. (2017). A Review on the Classification, Characterisation, Synthesis of Nanoparticles and Their Application. IOP Conf. Ser. Mat. Sci. Eng. 263, 032019. doi:10.1088/1757-899X/263/3/032019
Archana, K. M., Rajagopal, R., Kavitha, R., and Gayathri Krishnaswamy, V. (2022). Green Synthesized CuI as an Adsorbent and a Photocatalyst in the Removal of Aqueous Reactive Red 256 and Reactive Black 5 dyes. Surfaces Interfaces 29, 101724. doi:10.1016/j.surfin.2022.101724
Banfield, J. F., and Zhang, H. (2001). Nanoparticles in the Environment. Rev. Mineralogy Geochem. 44 (1), 1–58. doi:10.2138/rmg.2001.44.01
Bayahia, H. (2022). Green Synthesis of Activated Carbon Doped Tungsten Trioxide Photocatalysts Using Leaf of Basil (Ocimum Basilicum) for Photocatalytic Degradation of Methylene Blue under Sunlight. J. Saudi Chem. Soc. 26 (2). doi:10.1016/j.jscs.2022.101432
Bera, A., and Belhaj, H. (2016). Application of Nanotechnology by Means of Nanoparticles and Nanodispersions in Oil Recovery - A Comprehensive Review. J. Nat. Gas Sci. Eng. 34, 1284–1309. doi:10.1016/j.jngse.2016.08.023
Bhuiyan, M. S. H., Miah, M. Y., Paul, S. C., Aka, T. D., Saha, O., Rahaman, M. M., et al. (2020). Green Synthesis of Iron Oxide Nanoparticle Using Carica Papaya Leaf Extract: Application for Photocatalytic Degradation of Remazol Yellow RR Dye and Antibacterial Activity. Heliyon 6 (8), e04603. doi:10.1016/j.heliyon.2020.e04603
Binding, D., and Steinbach, F. (1970). Homogeneous Photocatalysis by Organic Dyes in the Liquid Phase. Nature 227 (5260), 832–833. doi:10.1038/227832a0
Biswas, P., and Wu, C.-Y. (2005). Nanoparticles and the Environment. J. Air & Waste Manag. Assoc. 55 (6), 708–746. doi:10.1080/10473289.2005.10464656
Chand, K., Cao, D., Eldin Fouad, D., Hussain Shah, A., Qadeer Dayo, A., Zhu, K., et al. (2020). Green Synthesis, Characterization and Photocatalytic Application of Silver Nanoparticles Synthesized by Various Plant Extracts. Arabian J. Chem. 13 (11), 8248–8261. doi:10.1016/j.arabjc.2020.01.009
Chandhru, M., Logesh, R., Kutti Rani, S., Ahmed, N., and Vasimalai, N. (2021). Green Synthesis of Silver Nanoparticles from Plant Latex and Their Antibacterial and Photocatalytic Studies. Environ. Technol. 2021, 1–11. doi:10.1080/09593330.2021.1914181
Chandraker, S. K., Ghosh, M. K., Lal, M., and Shukla, R. (2021). A Review on Plant-Mediated Synthesis of Silver Nanoparticles, Their Characterization and Applications. Nano Ex. 2 (2), 022008. doi:10.1088/2632-959x/ac0355
Chandraker, S. K., Ghosh, M. K., Lal, M., Ghorai, T. K., and Shukla, R. (2019). Colorimetric sensing of Fe3+ and Hg2+ and photocatalytic activity of green synthesized silver nanoparticles from the leaf extract of Sonchus arvensis L New J. Chem. 46. doi:10.1039/C9NJ01338E
Chandraker, S. K., Lal, M., Dhruve, P., Singh, R. P., and Shukla, R. (2021). Cytotoxic, Antimitotic, DNA Binding, Photocatalytic, H2O2 Sensing, and Antioxidant Properties of Biofabricated Silver Nanoparticles Using Leaf Extract of Bryophyllum Pinnatum (Lam.) Oken. Front. Mol. Biosci. 7, 593040. doi:10.3389/fmolb.2020.593040
Chandraker, S. K., Lal, M., Ghosh, M. K., Tiwari, V., Ghorai, T. K., and Shukla, R. (2020). Green Synthesis of Copper Nanoparticles Using Leaf Extract of Ageratum houstonianum Mill. And Study of Their Photocatalytic and Antibacterial Activities. Nano Ex. 1 (1), 010033. doi:10.1088/2632-959x/ab8e99
Chandraker, S. K., Lal, M., Kumar, A., and Shukla, R. (2021). Justicia Adhatoda L. Mediated Green Synthesis of Silver Nanoparticles and Assessment of Their Antioxidant, Hydrogen Peroxide Sensing and Optical Properties. Mater. Technol. 2021, 1–11. doi:10.1080/10667857.2021.1949525
Chandraker, S. K., Lal, M., and Shukla, R. (2019). DNA-binding, Antioxidant, H2O2 Sensing and Photocatalytic Properties of Biogenic Silver Nanoparticles Using Ageratum Conyzoides L. Leaf Extract. RSC Adv. 9 (40), 23408–23417. doi:10.1039/c9ra03590g
Chen, W., Cai, W., Zhang, L., Wang, G., and Zhang, L. (2001). Sonochemical Processes and Formation of Gold Nanoparticles within Pores of Mesoporous Silica. J. Colloid Interface Sci. 238 (2), 291–295. doi:10.1006/jcis.2001.7525
Chinnappa, K., Karuna Ananthai, P., Srinivasan, P. P., and Dharmaraj Glorybai, C. (2022). Green Synthesis of rGO-AgNP Composite Using Curcubita Maxima Extract for Enhanced Photocatalytic Degradation of the Organophosphate Pesticide Chlorpyrifos. Environ. Sci. Pollut. Res. doi:10.1007/s11356-022-19917-1
Cho, E. J., Holback, H., Liu, K. C., Abouelmagd, S. A., Park, J., and Yeo, Y. (2013). Nanoparticle Characterization: State of the Art, Challenges, and Emerging Technologies. Mol. Pharm. 10, 2093–2110. doi:10.1021/mp300697h
Christopher, J. G., Saswati, B., and Ezilrani, P. (2015). Optimization of Parameters for Biosynthesis of Silver Nanoparticles Using Leaf Extract of Aegle Marmelos. Braz. Arch. Biol. Technol. 58 (5), 702–710. doi:10.1590/s1516-89132015050106
Daneshvar, N., Salari, D., Niaei, A., Rasoulifard, M. H., and Khataee, A. R. (2005). Immobilization of TiO2 Nanopowder on Glass Beads for the Photocatalytic Decolorization of an Azo Dye C.I. Direct Red 23. J. Environ. Sci. Health, Part A 40 (8), 1605–1617. doi:10.1081/ese-200060664
Dave, S., Khan, A. M., Purohit, S. D., and Suthar, D. L. (2021). Application of Green Synthesized Metal Nanoparticles in the Photocatalytic Degradation of Dyes and its Mathematical Modelling Using the Caputo-Fabrizio Fractional Derivative without the Singular Kernel. J. Math. 2021, 1–8. doi:10.1155/2021/9948422
Dhivya, A., Yadav, R., and Stella Packiam, C. (2022). An Eco-Approach Synthesis of Undoped and Mn Doped ZnO Nano-Photocatalyst for Prompt Decoloration of Methylene Blue Dye. Mater. Today Proc. 48 (2), 494–501. doi:10.1016/j.matpr.2021.02.751
Ebrahimian, J., Mohsennia, M., and Khayatkashani, M. (2021). Green Synthesis and Characterization of Ud-SnO2-ZnO Using Urtica Dioica Leaf Extract: A Nanocomposite Photocatalyst for Degradation of Rhodamine B Dye. Res. Chem. Intermed. 47, 4789–4802. doi:10.1007/s11164-021-04546-z
Ekennia, A. C., Uduagwu, D. N., Nwaji, N. N., Olowu, O. J., Nwanji, O. L., Ejimofor, M., et al. (2022). Green Synthesis of Silver Nanoparticles Using Leaf Extract of Euphorbia Sanguine: an In Vitro Study of its Photocatalytic and Melanogenesis Inhibition Activity. Inorg. Nano-Metal Chem. 52 (2), 195–203. doi:10.1080/24701556.2021.1891100
Elviera, Y. Y., Apriandanu, D. O. B., and Marcony Surya, R. (2022). Fabrication of Novel SnWO4/ZnO Using Muntingia calabura L. Leaf Extract with Enhanced Photocatalytic Methylene Blue Degradation under Visible Light Irradiation. Ceram. Int. 48 (3). doi:10.1016/j.ceramint.2021.10.135
Fagier, M. A. (2021). Plant-Mediated Biosynthesis and Photocatalysis Activities of Zinc Oxide Nanoparticles: A Prospect towards Dyes Mineralization. J. Nanotechnol. 2021, 6629180. doi:10.1155/2021/6629180
Fu, L., and Fu, Z. (2015). Plectranthus Amboinicus Leaf Extract-Assisted Biosynthesis of ZnO Nanoparticles and Their Photocatalytic Activity. Ceram. Int. 41 (2), 2492–2496. doi:10.1016/j.ceramint.2014.10.069
Geethalakshmi, R., and Sarada, D. V. (2012). Gold and Silver Nanoparticles from Trianthema Decandra: Synthesis, Characterization, and Antimicrobial Properties. Int. J. Nanomedicine 7, 5375–5384. doi:10.2147/ijn.s36516
Ghazal, S., Khandannasab, N., Hosseini, H. A., Sabouri, Z., Rangrazi, A., and Darroudi, M. (2021). Green Synthesis of Copper-Doped Nickel Oxide Nanoparticles Using Okra Plant Extract for the Evaluation of Their Cytotoxicity and Photocatalytic Properties. Ceram. Int. 47 (19). doi:10.1016/j.ceramint.2021.06.135
Ghorai, T. K., and Biswas, N. (2013). Photodegradation of Rhodamine 6G in Aqueous Solution via SrCrO4 and TiO2 Nano-Sphere Mixed Oxides. J. Mater. Res. Technol. 2 (1), 10–17. doi:10.1016/j.jmrt.2013.03.002
Ghosh, M. K., Jain, K., Khan, S., Das, K., and Ghorai, T. K. (2020). New Dual-Functional and Reusable Bimetallic Y2ZnO4 Nanocatalyst for Organic Transformation under Microwave/Green Conditions. ACS Omega 5 (10), 4973–4981. doi:10.1021/acsomega.9b03875
Ghosh, M. K., Sahu, S., Gupta, I., and Ghorai, T. K. (2020). Green Synthesis of Copper Nanoparticles from an Extract of Jatropha Curcas Leaves: Characterization, Optical Properties, CT-DNA Binding and Photocatalytic Activity. RSC Adv. 10 (37), 22027–22035. doi:10.1039/d0ra03186k
Gopala Krishna, P., Paduvarahalli Ananthaswamy, P., Trivedi, P., Chaturvedi, V., Bhangi Mutta, N., Sannaiah, A., et al. (2017). Antitubercular Activity of ZnO Nanoparticles Prepared by Solution Combustion Synthesis Using Lemon Juice as Bio-Fuel. Mater. Sci. Eng. C 75, 1026–1033. doi:10.1016/j.msec.2017.02.093
Hamidian, K., Ahmad, N., Miri, A., and Sarani, M. (2021). Photocatalytic Performance on Degradation of Acid Orange 7 Dye Using Biosynthesized Un-doped and Co Doped CeO2 Nanoparticles. Mater. Res. Bull. 138, 111206. doi:10.1016/j.materresbull.2021.111206
Hashemi, Z., Mizwari, Z. M., Mohammadi-Aghdam, S., Mortazavi-Derazkola, S., and Ali Ebrahimzadeh, M. (2022). Sustainable Green Synthesis of Silver Nanoparticles Using Sambucus Ebulus Phenolic Extract (AgNPs@SEE): Optimization and Assessment of Photocatalytic Degradation of Methyl Orange and Their In Vitro Antibacterial and Anticancer Activity. Arabian J. Chem. 15 (1), 103525. doi:10.1016/j.arabjc.2021.103525
Hashemi, Z., Shirzadi-Ahodashti, M., Mortazavi-Derazkola, S., and Ebrahimzadeh, M. A. (2022). Sustainable Biosynthesis of Metallic Silver Nanoparticles Using Barberry Phenolic Extract: Optimization and Evaluation of Photocatalytic, In Vitro Cytotoxicity, and Antibacterial Activities against Multidrug-Resistant Bacteria. Inorg. Chem. Commun. 139, 109320. doi:10.1016/j.inoche.2022.109320
Herizchi, R., Abbasi, E., Milani, M., and Akbarzadeh, A. (2016). Current Methods for Synthesis of Gold Nanoparticles. Artif. Cells, Nanomedicine, Biotechnol. 44, 596–602. doi:10.3109/21691401.2014.971807
Herrera, G., Montoya, N., Doménech-Carbó, A., and Alarcón, J. (2013). Synthesis, Characterization and Electrochemical Properties of Iron-Zirconia Solid Solution Nanoparticles Prepared Using a Sol-Gel Technique. Phys. Chem. Chem. Phys. 15 (44), 19312–19321. doi:10.1039/c3cp53216j
Ibhadon, A., and Fitzpatrick, P. (2013). Heterogeneous Photocatalysis: Recent Advances and Applications. Catalysts 3 (1), 189–218. doi:10.3390/catal3010189
Ijaz, F., Shahid, S., Khan, S. A., Ahmad, W., and Zaman, S. (2017). Green Synthesis of Copper Oxide Nanoparticles Using Abutilon Indicum Leaf Extract: Antimicrobial, Antioxidant and Photocatalytic Dye Degradation Activitie. Trop. J. Pharm. Res. 16 (4), 743–753. doi:10.4314/tjpr.v16i4.2
Iqbal, A., Ahmed, A. S., Ahmad, N., Adil, S., Ahamad, T., and Khan, M. Z. (2021). Biogenic synthesis of CeO2 nanoparticles and its potential application as an efficient photocatalyst for the degradation of toxic amido black dye Environ. Nanotechnol. Monit. Manag. 16. doi:10.1016/j.enmm.2021.100505
Iqbal, A., Haq, A. U., Cerron-Calle, G. A., Naqvi, S. A. R., Westerhoff, P., and Garcia-Segura, S. (2021). Green Synthesis of Flower-Shaped Copper Oxide and Nickel Oxide Nanoparticles via Capparis Decidua Leaf Extract for Synergic Adsorption-Photocatalytic Degradation of Pesticides
Irshad, M. A., Nawaz, R., Rehman, M. Z. U., Adrees, M., Rizwan, M., Ali, S., et al. (2021). Synthesis, Characterization and Advanced Sustainable Applications of Titanium Dioxide Nanoparticles: A Review. Ecotoxicol. Environ. Saf. 212, 111978. doi:10.1016/j.ecoenv.2021.111978
Jadoun, S., Arif, R., Jangid, N. K., and Meena, R. K. (2021). Green Synthesis of Nanoparticles Using Plant Extracts: a Review. Environ. Chem. Lett. 19, 355–374. doi:10.1007/s10311-020-01074-x
Jamkhande, P. G., Ghule, N. W., Bamer, A. H., and Kalaskar, M. G. (2019). Metal Nanoparticles Synthesis: An Overview on Methods of Preparation, Advantages and Disadvantages, and Applications. J. Drug Deliv. Sci. Technol. 53, 101174. doi:10.1016/j.jddst.2019.101174
Jayappa, M. D., Ramaiah, C. K., Kumar, M. A. P., Suresh, D., Prabhu, A., Devasya, R. P., et al. (2020). Green Synthesis of Zinc Oxide Nanoparticles from the Leaf, Stem and In Vitro Grown Callus of Mussaenda Frondosa L.: Characterization and Their Applications. Appl. Nanosci. 10 (8), 3057–3074. doi:10.1007/s13204-020-01382-2
Jiang, W., Wang, X., Wu, Z., Yue, X., Yuan, S., Lu, H., et al. (2015). Silver Oxide as Superb and Stable Photocatalyst under Visible and Near-Infrared Light Irradiation and its Photocatalytic Mechanism. Ind. Eng. Chem. Res. 54 (3), 832–841. doi:10.1021/ie503241k
Jing, Y., Li, L., Zhang, Q., Lu, P., Liu, P., and Lü, X. (2011). Photocatalytic ozonation of dimethyl phthalate with TiO2 prepared by a hydrothermal method. J. Hazard. Mater. 189 (1-2), 40–47. doi:10.1016/j.jhazmat.2011.01.132
Jyoti, K., and Singh, A. (2016). Green Synthesis of Nanostructured Silver Particles and Their Catalytic Application in Dye Degradation. J. Genet. Eng. Biotechnol. 14 (2), 311–317. doi:10.1016/j.jgeb.2016.09.005
Kaliraj, L., Ahn, J. C., Rupa, E. J., Abid, S., Lu, J., and Yang, D. C. (2019). Synthesis of Panos Extract Mediated ZnO Nano-Flowers as Photocatalyst for Industrial Dye Degradation by UV Illumination. J. Photochem. Photobiol. B Biol. 199, 111588. doi:10.1016/j.jphotobiol.2019.111588
Kannan, S., Subiramaniyam, N. P., and Sathishkumar, M. (2020). A Novel Green Synthesis Approach for Improved Photocatalytic Activity and Antibacterial Properties of Zinc Sulfide Nanoparticles Using Plant Extract of Acalypha indica and Tridax Procumbens. J. Mater Sci. Mater Electron 31, 9846–9859. doi:10.1007/s10854-020-03529-x
Karthik, K. V., Raghu, A. V., Reddy, K. R., Ravishankar, R., Sangeeta, M., Shetti, N. P., et al. (2022). Green Synthesis of Cu-Doped ZnO Nanoparticles and its Application for the Photocatalytic Degradation of Hazardous Organic Pollutants. Chemosphere 287 (Part 2), 132081. doi:10.1016/j.chemosphere.2021.132081
Khalid, S., Adil, S., Basharat, N., Abubakar, M., and Anwar, P. (2018). Phytochemical Screening and Analysis of Selected Medicinal Plants in Gujrat. J. Phytochem. Biochem. 2, 108.
Khan, M. S., Dhavan, P. P., Ratna, D., and Shimpi, N. G. (2022). Ultrasonic-assisted Biosynthesis of ZnO Nanoparticles Using Sonneratia Alba Leaf Extract and Investigation of its Photocatalytic and Biological Activities. J. Clust. Sci. 33, 1007–1023. doi:10.1007/s10876-021-02036-1
Khan, Z. U. H., Khan, A., Shah, N. S., Din, I. U., Abdel Salam, M., Iqbal, J., et al. (2021). Photocatalytic and Biomedical Investigation of Green Synthesized NiONPs: Toxicities and Degradation Pathways of Congo Red Dye. Surfaces Interfaces 23. doi:10.1016/j.surfin.2021.100944
Kouhbanani, M. A. J., Beheshtkhoo, N., Taghizadeh, S., Amani, A. M., and Alimardani, V. (2019). One-step Green Synthesis and Characterization of Iron Oxide Nanoparticles Using Aqueous Leaf Extract of Teucrium Polium and Their Catalytic Application in Dye Degradation. Adv. Nat. Sci. Nanosci. Nanotechnol. 10 (1), 015007. doi:10.1088/2043-6254/aafe74
Krishna, P. G., Ananthaswamy, P. P., Yadavalli, T., Mutta, N. B., Sannaiah, A., and Shivanna, Y. (2016a). ZnO Nanopellets have Selective Anticancer Activity Mater. Sci. Eng. C 62, 919–926. doi:10.1016/j.msec.2016.02.039
Krishna, P. G., Ananthaswamy, P. P., Gadewar, M., Bora, U., and Mutta, N. B. (2017a). In Vitro Antibacterial And Anticancer Studies Of ZnO Nanoparticles Prepared By Sugar Fueled Combustion Synthesis 8, 24–29. doi:10.5185/amlett.2017.6424
Krishna, P. G., Ananthaswamy, P. P., Mutta, N. B., Mariyappa, K. G., and Singh, R. (2017b). Comparison of Antimicrobial and Anticancer Activity of ZnO Nanoparticles Prepared Using Different Precursors by Hydrothermal Synthesis J. Chem. Pharm. Sci. 10, 192–197.
Lam, S. M., Sin, J. C., Abdullah, A. Z., and Mohamed, A. R. (2012). Degradation of Wastewaters Containing Organic Dyes Photocatalysed by Zinc Oxide: a Review. Desalination Water Treat. 41 (1-3), 131–169. doi:10.1080/19443994.2012.664698
Letchumanan, D., Sok, S. P. M., Ibrahim, S., Nagoor, N. H., and Arshad, N. M. (2021). Plant-Based Biosynthesis of Copper/Copper Oxide Nanoparticles: An Update on Their Applications in Biomedicine, Mechanisms, and Toxicity. Biomolecules 11 (4), 564. doi:10.3390/biom11040564
Li, X., Xu, H., Chen, Z. S., and Chen, G. (2011). Biosynthesis of Nanoparticles by Microorganisms and Their Applications. J. Nanomater. 2011, 270974. doi:10.1155/2011/270974
Lin, C. H., Lin, Y. C., Chang, C. L., Chen, W. C., Cheng, S. Y., Wang, Y. H., et al. (2007). Photodecomposition of Methylene-Blue by Highly-Dispersed Nano TiO2/Ag Catalyst. React. Kinet. Catal. Lett. 90 (2), 267–273. doi:10.1007/s11144-007-4936-4
Mali, S. C., Dhaka, A., Githala, C. K., and Trivedi, R. (2020). Green Synthesis of Copper Nanoparticles Using Celastrus Paniculatus Willd. Leaf Extract and Their Photocatalytic and Antifungal Properties. Biotechnol. Rep. 27, e00518. doi:10.1016/j.btre.2020.e00518
Mangala Nagasundari, S., Muthu, K., Kaviyarasu, K., Al Farraj, D. A., and Alkufeidy, R. M. (2021). Current Trends of Silver Doped Zinc Oxide Nanowires Photocatalytic Degradation for Energy and Environmental Application. Surfaces Interfaces 23. doi:10.1016/j.surfin.2021.100931
Manikandan, V., Yi, P. I., Velmurugan, P., Jayanthi, P., Hong, S. C., Jang, S. H., et al. (2017). Production, Optimization and Characterization of Silver Oxide Nanoparticles Using Artocarpus Heterophyllus Rind Extract and Their Antifungal Activity. Afr. J. Bio Technol. 16 (36), 1819–1825. doi:10.5897/AJB2017.15967
Meneceur, S., Hemmami, H., Bouafia, A., Laouini, S. E., Tedjani, M. L., Berra, D., et al. (2022). Photocatalytic Activity of Iron Oxide Nanoparticles Synthesized by Different Plant Extracts for the Degradation of Diazo Dyes Evans Blue and Congo Red. Biomass Conv. bioref.. doi:10.1007/s13399-022-02734-4
Miri, A., Beiki, H., Najafidoust, A., Khatami, M., and Sarani, M. (2021). Cerium Oxide Nanoparticles: Green Synthesis Using Banana Peel, Cytotoxic Effect, UV Protection and Their Photocatalytic Activity. Bioprocess Biosyst. Eng. 44, 1891–1899. doi:10.1007/s00449-021-02569-9
Miri, A., Sarani, M., Najafidoust, A., Mehrabani, M., Zadeh, F. A., and Varma, R. S. (2022). Photocatalytic Performance and Cytotoxic Activity of Green-Synthesized Cobalt Ferrite Nanoparticles. Mater. Res. Bull. 149, 111706. doi:10.1016/j.materresbull.2021.111706
Molina, G. A., Esparza, R., López-Miranda, J. L., Hernández-Martínez, A. R., España-Sánchez, B. L., Elizalde-Peña, E. A., et al. (2019). Green Synthesis of Ag Nanoflowers Using Kalanchoe Daigremontiana Extract for Enhanced Photocatalytic and Antibacterial Activities. Colloids Surfaces B Biointerfaces 180, 141–149. doi:10.1016/j.colsurfb.2019.04.044
Moon, S. A., Salunke, B. K., Saha, P., Deshmukh, A. R., and Kim, B. S. (2018). Comparison of Dye Degradation Potential of Biosynthesized Copper Oxide, Manganese Dioxide, and Silver Nanoparticles Using Kalopanax Pictus Plant Extract. Korean J. Chem. Eng. 35 (3), 702–708. doi:10.1007/s11814-017-0318-4
Muddapur, U. M., Alshehri, S., Ghoneim, M. M., Mahnashi, M. H., Alshahrani, M. A., Khan, A. A., et al. (2022). Plant-Based Synthesis of Gold Nanoparticles and Theranostic Applications: A Review. Molecules 27, 1391. doi:10.3390/molecules27041391
Muthu, K., Rini, S., Nagasundari, S. M., and Akilandaeaswari, B. (2021). Photocatalytic Reduction and Antioxidant Potential of Green Synthesized Silver Nanoparticles from Catharanthus Roseus Flower Extract. Inorg. Nano-Metal Chem. 51 (4), 579–589. doi:10.1080/24701556.2020.1799404
Nabi, G., Ul- Ain, Q., Tahir, M. B., Riaz, K. N., Iqbal, T., and Rafique, M. (2022). Green synthesis of TiO2 nanoparticles using lemon peel extract: their optical and photocatalytic properties Int. J. Environ. Anal. Chem. 102, 434–442. doi:10.1080/03067319.2020.1722816
Narasaiah, P., Kumar Mandal, B., and Sarada, N. C. (2017). Green Synthesis of Pd NPs from Pimpinella Tirupatiensis Plant Extract and Their Application in Photocatalytic Activity Dye Degradation. IOP Conf. Ser. Mat. Sci. Eng. 263 (2), 022013. doi:10.1088/1757-899x/263/2/022013
Navada, K. M., Nagaraja, G. K., Ranjitha, R., D’Souza, J. N., Kouser, S., and Manasa, D. J. (2021). Synthesis, Characterization of Phyto-Functionalized CuO Nano Photocatalysts for Mitigation of Textile Dyes in Waste Water Purification, Antioxidant, Anti-inflammatory and Anticancer Evaluation. Appl. Nanosci. 11, 1313–1338. doi:10.1007/s13204-021-01688-9
Ong, C. B., Ng, L. Y., and Mohammad, A. W. (2018). A Review of ZnO Nanoparticles as Solar Photocatalysts: Synthesis, Mechanisms and Applications. Renew. Sustain. Energy Rev. 81, 536–551. doi:10.1016/j.rser.2017.08.020
Panchal, P., Meena, P., and Nehra, S. P. (2021). A Rapid Green Synthesis of Ag/AgCl-NC Photocatalyst for Environmental Applications. Environ. Sci. Pollut. Res. 28, 3972–3982. doi:10.1007/s11356-020-11834-5
Panneerselvi, V., Shankar, K., Muthukrishnan, P., and Prabhu, A. (2022). Mangifera Indica Resin Assisted Synthesis of Nano Silver: Assessing Their Photocatalytic Degradation of Methylene Blue, Anticorrosive and Antioxidant Activity. J. Clust. Sci. 33, 123–133. doi:10.1007/s10876-020-01965-7
Parvathiraja, C., and Shailajha, S. (2021). Bioproduction of CuO and Ag/CuO Heterogeneous Photocatalysis-Photocatalytic Dye Degradation and Biological Activities. Appl. Nanosci. 11, 1411–1425. doi:10.1007/s13204-021-01743-5
Peternela, J., Silva, M. F., Vieira, M. F., Bergamasco, R., and Vieira, A. M. S. (2017). Synthesis and Impregnation of Copper Oxide Nanoparticles on Activated Carbon through Green Synthesis for Water Pollutant Removal. Mater. Res. 21. doi:10.1590/1980-5373-mr-2016-0460
Phang, Y.-K., Aminuzzaman, M., Akhtaruzzaman, M., Muhammad, G., Ogawa, S., Watanabe, A., et al. (2021). Green Synthesis and Characterization of CuO Nanoparticles Derived from Papaya Peel Extract for the Photocatalytic Degradation of Palm Oil Mill Effluent (POME). Sustainability 13, 796. doi:10.3390/su13020796
Pillai, A. M., Sivasankarapillai, V. S., Rahdar, A., Joseph, J., Sadeghfar, F., Anuf A, R., et al. (2020). Green Synthesis and Characterization of Zinc Oxide Nanoparticles with Antibacterial and Antifungal Activity. J. Mol. Struct. 1211, 128107. doi:10.1016/j.molstruc.2020.128107
Prashanth, G. K., Prashanth, P. A., Singh, P., Nagabhushana, B. M., Shivakumara, C., Krishnaiah, G. M., et al. (2020). Effect of Doping (With Cobalt or Nickel) and UV Exposure on the Antibacterial, Anticancer, and ROS Generation Activities of Zinc Oxide Nanoparticles. J. Asian Ceram. Soc. 8 (4), 1175–1187. doi:10.1080/21870764.2020.1824328
Prashanth, G. K., and Krishnaiah, G. M. (2014). Chemical Composition of the Leaves of Azadiracta indica Linn (Neem). Int. J. Adv. Eng. Technol. Manag. Appl. Sci. 1 (5), 21–31.
Prashanth, G. K., Krishnaiah, G. M., and Sathyananda, H. M. (2015). Qualitative Phytochemical Screening and GCMS Analysis of the Leaves of Indigofera tinctoria. Int. J. Innov. Res. Sci. Eng. Technol. 4, 7544–7547. doi:10.15680/IJIRSET.2015.0408080
Prashanth, G. K., and Krishnaiah, G. M. (2014). Phytochemical Screening and GC-MS Analysis of the Leaves of Pongamia Pinnata Linn. Int. J. Innovative Res. Sci. Eng. Technol. 3 (11), 17329–17334. doi:10.15680/IJIRSET.2014.0311034
Prashanth, G. K., Prashanth, P. A., Bora, U., Gadewar, M., Nagabhushana, B. M., Ananda, S., et al. (2015). In Vitro antibacterial and Cytotoxicity Studies of ZnO Nanopowders Prepared by Combustion Assisted Facile Green Synthesis. Karbala Int. J. Mod. Sci. 1, 67–77. doi:10.1016/j.kijoms.2015.10.007
Prashanth, G. K., Prashanth, P. A., Nagabhushana, B. M., Ananda, S., Nagendra, H. G., and Singh, R. C. (2016b). In Vitro Antimicrobial, Antioxidant and Anticancer Studies of ZnO Nanoparticles Synthesized by Precipitation Method Adv. Sci. Eng. Medicine 8, 306–313. doi:10.1166/asem.2016.1854
Prashanth, G. K., Dileep, M. S., Prashanth, P. A., Gadewar, M., Nagabhushana, B. M., and Prabhu, S. R. B. (2022). Applications of Inorganic Polymers in Textile Wastewater Treatment. Polymer Technology in Dye-Containing Wastewater. Sustainable Textiles: Production, Processing, Manufacturing and Chemistry. Editors A. Khadir, and S. S. Muthu (Singapore: Springer), 227–245. doi:10.1007/978-981-19-0886-6_10
Prashanth, G. K., Prashanth, P. A., Nagabhushana, B. M., Ananda, S., Krishnaiah, G. M., Nagendra, H. G., et al. (2018). Comparison of Anticancer Activity of Biocompatible ZnO Nanoparticles Prepared by Solution Combustion Synthesis Using Aqueous Leaf Extracts of Abutilon Indicum, Melia Azedarach and Indigofera Tinctoria as Biofuels. Artif. Cells, Nanomedicine, Biotechnol. 46, 968–979. doi:10.1080/21691401.2017.1351982
Prashanth, G. K., Prashanth, P. A., Ramani, M., Ananda, S., Nagabhushana, B. M., Krishnaiah, G. M., et al. (2019). Comparison of antimicrobial, antioxidant and anticancer activities of ZnO nanoparticles prepared by lemon juice and citric acid fueled solution combustion synthesis. BioNanoScience 9 (4), 799–812. doi:10.1007/s12668-019-00670-8
Prashanth, G. K., Sathyananda, H. M., Prashanth, P. A., Gadewar, M., Mutthuraju, M., Boselin Prabhu, S. R., et al. (2022). Controlled Synthesis of Ag/CuO Nanocomposites: Evaluation of their Antimycobacterial, Antioxidant, and Anticancer Activities. Appl. Phys. A 128, 614. doi:10.1007/s00339-022-05748-x
Priyadarshini, S., Sonsudin, F., Mainal, A., Yahya, R., Gopinath, V., Vadivelu, J., et al. (2021). Phytosynthesis of Biohybrid Nano-Silver Anchors Enhanced Size Dependent Photocatalytic, Antibacterial, Anticancer Properties and Cytocompatibility. Process Biochem. 101, 59–71. doi:10.1016/j.procbio.2020.11.008
Purkait, P., Bhattacharyya, A., Roy, S., Maitra, S., Das, G. C., and Chaudhuri, M. G. (2020). Green Synthesis of ZnO Nanoparticle Using Trema Orientalis (L) Leaf: an Efficient Photocatalyst for Degradation of Zoxamide Fungicide in Aqueous Organic Media under UV Light Irradiation. Int. J. Environ. Anal. Chem. doi:10.1080/03067319.2020.1857750
Rafique, M., Tahir, R., Gillani, S. S. A., Tahir, M. B., Shakil, M., Iqbal, T., et al. (2022). Plant-mediated Green Synthesis of Zinc Oxide Nanoparticles from Syzygium Cumini for Seed Germination and Wastewater Purification. Int. J. Environ. Anal. Chem. 102 (1), 23–38. doi:10.1080/03067319.2020.1715379
Rajan, P. I., Vijaya, J. J., Jesudoss, S. K., Kaviyarasu, K., Kennedy, L. J., Jothiramalingam, R., et al. (2017). Green-fuel-mediated Synthesis of Self-Assembled NiO Nano-Sticks for Dual Applications-Photocatalytic Activity on Rose Bengal Dye and Antimicrobial Action on Bacterial Strains. Mat. Res. Express 4, 085030. doi:10.1088/2053-1591/aa7e3c
Rajendrachari, S., Taslimi, P., Cahit Karaoglanli, A., Orhan, U., Alp, E., and Kudur Jayaprakash, G. (2021). Photocatalytic Degradation of Rhodamine B (RhB) Dye in Waste Water and Enzymatic Inhibition Study Using Cauliflower Shaped ZnO Nanoparticles Synthesized by a Novel One-Pot Green Synthesis Method. Arabian J. Chem. 14 (6). doi:10.1016/j.arabjc.2021.103180
Ramachandiran, D., Elangovan, M., and Rajesh, K. (2021). Structural, Optical, Biological and Photocatalytic Activities of Platinum Nanoparticles Using Salixtetrasperma Leaf Extract via Hydrothermal and Ultrasonic Methods. Optik 244. doi:10.1016/j.ijleo.2021.167494
Ramzan, M., Obodo, R. M., Shahzad, M. I., Mukhtar, S., Ilyas, S. Z., and Mahmood, T. (2021). Green Synthesis of Cu@TiO2 via Cedrus Deodara Leaf Extract: A: Novel Composite with High Photocatalytic and Antibacterial Activity. Curr. Res. Green Sustain. Chem. 4, 100137. doi:10.1016/j.crgsc.2021.100137
Rani, M., and Shanker, U. (2018). Photocatalytic Degradation of Toxic Phenols from Water Using Bimetallic Metal Oxide Nanostructures. Colloids Surfaces A Physicochem. Eng. Aspects 553. doi:10.1016/j.colsurfa.2018.05.071
Raouf Malik, A., Sharif, S., Shaheen, F., Khalid, M., Iqbal, Y., Faisal, A., et al. (2022). Green synthesis of RGO-ZnO mediated Ocimum basilicum leaves extract nanocomposite for antioxidant, antibacterial, antidiabetic and photocatalytic activity. J. Saudi Chem. Soc. 26 (2). doi:10.1016/j.jscs.2022.101438
Rasheed, T., Bilal, M., Nabeel, F., Adeel, M., and Iqbal, H. M. N. (2019). Environmentally-related Contaminants of High Concern: Potential Sources and Analytical Modalities for Detection, Quantification, and Treatment. Environ. Int. 122, 52–66. doi:10.1016/j.envint.2018.11.038
Rasheed, T., Nabeel, F., Bilal, M., and Iqbal, H. M. N. (2019). Biogenic Synthesis and Characterization of Cobalt Oxide Nanoparticles for Catalytic Reduction of Direct Yellow-142 and Methyl Orange Dyes. Biocatal. Agric. Biotechnol. 19, 101154. doi:10.1016/j.bcab.2019.101154
Rizwana, H., Alwhibi, M. S., Al-Judaie, R. A., Aldehaish, H. A., and Alsaggabi, N. S. (2022). Sunlight-Mediated Green Synthesis of Silver Nanoparticles Using the Berries of Ribes Rubrum (Red Currants): Characterisation and Evaluation of Their Antifungal and Antibacterial Activities. Molecules 27 (7), 2186. doi:10.3390/molecules27072186
Sabouri, Z., Akbari, A., Hosseini, H. A., Hashemzadeh, A., and Darroudi, M. (2019). Eco-Friendly Biosynthesis of Nickel Oxide Nanoparticles Mediated by Okra Plant Extract and Investigation of Their Photocatalytic, Magnetic, Cytotoxicity, and Antibacterial Properties. J. Clust. Sci. 30 (6), 1425–1434. doi:10.1007/s10876-019-01584-x
Sapawe, N., Ariff Rustam, M., Hafizan Hakimin Mahadzir, M., Kamal Ezzat Mohamad Lani, M., Raidin, A., and Farhan Hanafi, M. (2019). A Novel Approach of In-Situ Electrobiosynthesis of Metal Oxide Nanoparticles Using Crude Plant Extract as Main Medium for Supporting Electrolyte. Mater. Today Proc. 19, 1441–1445. doi:10.1016/j.matpr.2019.11.166
Sathyananda, H. M., Prashanth, P. A., Prashanth, G. K., Nagabhushana, B. M., Shivakumara, C., Boselin Prabhu, S. R., et al. (2021). Evaluation of Antimycobacterial, Antioxidant, and Anticancer Activities of CuO Nanoparticles through Cobalt Doping. Appl. Nanosci. doi:10.1007/s13204-021-02156-0
Sathyananda, H. M., Prashanth, P. A., Prashanth, G. K., Nagabhushana, B. M., Krishnaiah, G. M., and Nagendra, H. G. (2021a). Antimicrobial, Antioxidant, and Cytotoxicity Activities of CuO Nanopellets Synthesized by Surfactant-Free Hydrothermal Method J. Testing Evalaution 49. doi:10.1520/JTE20200538
Sevilla, P., Hernández, M., Corda, E., García-Ramos, J. V., and Domingo, C. (2013). Molecular characterization of drug nanocarriers based on Plasmon Enhanced Spectroscopies: Fluorescence (SEF) and Raman (SERS). MAGINE NANO 2013, European Event in Nanoscience and Nanotechnology, 23rd - 26th of April 2013 at Bilbao, Spain. http://hdl.handle.net/10261/104668.
Shah, Y., Maharana, M., and Sen, S. (2022). Peltophorum pterocarpum Leaf Extract Mediated Green Synthesis of Novel Iron Oxide Particles for Application in Photocatalytic and Catalytic Removal of Organic Pollutants. Biomass Conv. bioref. 333. doi:10.1007/s13399-021-02189-z
Shaheen, I., Ahmad, K. S., Thomas, A., Ali, D., and Iram, S. (2022). Phytogenic Synthesis and Enhanced Photocatalytic Properties of ZnOCo3O4 P-N Junction: Biomimetic Water Remediators. Ionics 28, 1999–2006. doi:10.1007/s11581-021-04407-0
Shaikh, S., Nazam, N., Rizvi, S. M. D., Ahmad, K., Baig, M. H., Lee, E. J., et al. (2019). Mechanistic Insights into the Antimicrobial Actions of Metallic Nanoparticles and Their Implications for Multidrug Resistance. Int. J. Mol. Sci. 20, 2468. doi:10.3390/ijms20102468
Shaikh, W. A., Chakraborty, S., and Islam, R. U. (2020). Photocatalytic Degradation of Rhodamine B under UV Irradiation Using Shorea Robusta Leaf Extract-Mediated Bio-Synthesized Silver Nanoparticles. Int. J. Environ. Sci. Technol. 17, 2059–2072. doi:10.1007/s13762-019-02473-6
Sharma, R. (2021). Synthesis of Terminalia bellirica fruit extract mediated silver nanoparticles and application in photocatalytic degradation of wastewater from textile industries Materials Today: Proceedings 44, 1995–1998. doi:10.1016/j.matpr.2020.12.118
Sharma, S., Kumar, K., Thakur, N., Chauhan, S., and Singh Chauhan, M. (2021). Eco-friendly Ocimum Tenuiflorum Green Route Synthesis of CuO Nanoparticles: Characterizations on Photocatalytic and Antibacterial Activities. Eco-friendly J. Environ. Chem. Eng. 9 (4). doi:10.1016/j.jece.2021.105395
Shume, W. M., Murthy, H. C., and Zereffa, E. A. (2020). A Review on Synthesis and Characterization of Ag2O Nanoparticles for Photocatalytic Applications. J. Chem. 2020, 5039479. doi:10.1155/2020/5039479
Singh, A. K. (2022). A Review on Plant Extract-Based Route for Synthesis of Cobalt Nanoparticles: Photocatalytic, Electrochemical Sensing and Antibacterial Applications. Curr. Res. Green Sustain. Chem. 5, 100270. doi:10.1016/j.crgsc.2022.100270
Singh, J., Kaur, S., Kaur, G., Basu, S., and Rawat, M. (2019). Biogenic ZnO Nanoparticles: a Study of Blueshift of Optical Band Gap and Photocatalytic Degradation of Reactive Yellow 186 Dye under Direct Sunlight. Green Process Synth. 8, 272–280. doi:10.1515/gps-2018-0084
Singh, J., Kumar, V., Kim, K. H., and Rawat, M. (2019). Biogenic Synthesis of Copper Oxide Nanoparticles Using Plant Extract and its Prodigious Potential for Photocatalytic Degradation of Dyes. Environ. Res. 177, 108569. doi:10.1016/j.envres.2019.108569
Singh, T., Jayaprakash, A., Alsuwaidi, M., and Anish Madhavan, A. (2021). Green Synthesized Gold Nanoparticles with Enhanced Photocatalytic Activity. Mater. Today Proc. 42 (2). doi:10.1016/j.matpr.2020.12.531
Sivaraj, R., Salam, H. A., Rajiv, P., and Rajendran, V. (2015). Green Nanotechnology: the Solution to Sustainable Development of Environment. Environ. Sustain. 2015, 311–324. doi:10.1007/978-81-322-2056-5_18
Sohrabnezhad, S., and Seifi, A. (2016). The Green Synthesis of Ag/ZnO in Montmorillonite with Enhanced Photocatalytic Activity. Appl. Surf. Sci. 386, 33–40. doi:10.1016/j.apsusc.2016.05.102
Sudhaparimala, S., and Vaishnavi, M. (2016). Biological Synthesis of Nano Composite SnO2- ZnO - Screening for Efficient Photocatalytic Degradation and Antimicrobial Activity. Mater. Today Proc. 3 (6), 2373–2380. doi:10.1016/j.matpr.2016.04.150
Thirumurugan, A., Jiflin, G. J., Rajagomathi, G., Tomy, N. A., Ramachandran, S., and Jaiganesh, R. (2010). Plants: Emerging as Green Source toward Biosynthesis of Metallic Nanoparticles and its Applications. Int. J. Biol. Technol. 1, 75–77. doi:10.15297/JBCE.V2I1.02
Tiwari, P., Kumar, B., Kaur, M., Kaur, G., and Kaur, H. (2011). Phytochemical Screening and Extraction: A Review. Int. Pharm. Sci. 1.
Ullah, A., Rasheed, S., Ali, I., and Ullah, N. (2021). Plant Mediated Synthesis of CdS Nanoparticles, Their Characterization and Application for Photocatalytic Degradation of Toxic Organic Dye. Chem. Rev. Lett. 4 (2), 98–107. doi:10.22034/CRL.2021.262754.1097
US Environmental Protection Agency (1998b). Microbial and Disinfection By-Product Rules. Fed. Regist. 63, 69389–69476.
Varadavenkatesan, T., Pai, E., Pugazhendhi, S., Ramesh, A., Vinayagam, R., and Selvaraj, R. (2019). Photocatalytic Degradation of Rhodamine B by Zinc Oxide Nanoparticles Synthesized Using the Leaf Extract of Cyanometra Ramiflora. J. Photochem. Photobiol. B Biol. 199, 111621. doi:10.1016/j.jphotobiol.2019.111621
Vasantharaj, S., Sathiyavimal, S., Saravanan, M., Senthilkumar, P., Gnanasekaran, K., Shanmugavel, M., et al. (2019). Synthesis of Ecofriendly Copper Oxide Nanoparticles for Fabrication over Textile Fabrics: Characterization of Antibacterial Activity and Dye Degradation Potential. J. Photochem. Photobiol. B Biol. 191, 143–149. doi:10.1016/j.jphotobiol.2018.12.026
Vasantharaj, S., Sathiyavimal, S., Senthilkumar, P., LewisOscar, F., and Pugazhendhi, A. (2019). Biosynthesis of Iron Oxide Nanoparticles Using Leaf Extract of Ruellia Tuberosa: Antimicrobial Properties and Their Applications in Photocatalytic Degradation. J. Photochem. Photobiol. B Biol. 192, 74–82. doi:10.1016/j.jphotobiol.2018.12.025
Vijayakumar, M., Priya, K., Nancy, F. T., Noorlidah, A., and Ahmed, A. B. A. (2013). Biosynthesis, Characterisation and Anti-bacterial Effect of Plant-Mediated Silver Nanoparticles Using Artemisia Nilagirica. Industrial Crops Prod. 41, 235–240. doi:10.1016/j.indcrop.2012.04.017
Vinayagam, R., Pai, S., Varadavenkatesan, T., Pugazhendhi, A., and Selvaraj, R. (2021). Characterization and Photocatalytic Activity of ZnO Nanoflowers Synthesized Using Bridelia Retusa Leaf Extract. Appl. Nanosci. doi:10.1007/s13204-021-01816-5
Vinothkanna, A., Mathivanan, K., Ananth, S., Ma, Y., and Sekar, S. (2022). Biosynthesis of Copper Oxide Nanoparticles Using Rubia Cordifolia Bark Extract: Characterization, Antibacterial, Antioxidant, Larvicidal and Photocatalytic Activities. Environ. Sci. Pollut. Res. doi:10.1007/s11356-022-18996-4
Wang, D.-H., Jia, L., Wu, X.-L., Lu, L.-Q., and Xu, A.-W. (2012). One-step Hydrothermal Synthesis of N-Doped TiO2/C Nanocomposites with High Visible Light Photocatalytic Activity. Nanoscale 4 (2), 576–584. doi:10.1039/c1nr11353d
Wang, D., Li, L., Luo, Q., An, J., Li, X., Yin, R., et al. (2014). Enhanced Visible-Light Photocatalytic Performances of Ag3PO4 Surface-Modified with Small Amounts of TiO2 and Ag. Appl. Surf. Sci. 321, 439–446. doi:10.1016/j.apsusc.2014.10.055
Wang, Z., Fang, C., and Megharaj, M. (2014). Characterization of Iron-Polyphenol Nanoparticles Synthesized by Three Plant Extracts and Their Fenton Oxidation of Azo Dye. ACS Sustain. Chem. Eng. 2 (4), 1022–1025. doi:10.1021/sc500021n
Weir, E., Lawlor, A., Whelan, A., and Regan, F. (2008). The Use of Nanoparticles in Anti-microbial Materials and Their Characterization. Analyst 133, 835–845. doi:10.1039/b715532h
World Health Organization (2008). Guidelines for Drinking-Water Quality. Third Edition. Geneva: WHO. Incorporating the first and second ammenda , Volume 1, Recommendations.
Yulizar, Y., Sudirman, D. O. B., Apriandanu, D. O. B., and Wibowo, A. P. (2019). Plant Extract Mediated Synthesis of Au/TiO2 Nanocomposite and its Photocatalytic Activity under Sodium Light Irradiation. Compos. Commun. 16, 50–56. doi:10.1016/j.coco.2019.08.006
Zhang, M., Shao, C., Guo, Z., Zhang, Z., Mu, J., Zhang, P., et al. (2011). Highly Efficient Decomposition of Organic Dye by Aqueous-Solid Phase Transfer and In Situ Photocatalysis Using Hierarchical Copper Phthalocyanine Hollow Spheres. ACS Appl. Mat. Interfaces 3 (7), 2573–2578. doi:10.1021/am200412t
Zhang, Z., Yu, Y., and Wang, P. (2012). Hierarchical Top-Porous/bottom-Tubular TiO2 Nanostructures Decorated with Pd Nanoparticles for Efficient Photoelectrocatalytic Decomposition of Synergistic Pollutants. ACS Appl. Mat. Interfaces 4 (2), 990–996. doi:10.1021/am201630s
Keywords: nanoparticles, plant extract, characterization, photocatalyst, green synthesis, hazard remediation
Citation: Krishna PG, Chandra Mishra P, Naika MM, Gadewar M, Ananthaswamy PP, Rao S, Boselin Prabhu SR, Yatish KV, Nagendra HG, Moustafa M, Al-Shehri M, Jha SK, Lal B and Stephen Santhakumari SM (2022) Photocatalytic Activity Induced by Metal Nanoparticles Synthesized by Sustainable Approaches: A Comprehensive Review. Front. Chem. 10:917831. doi: 10.3389/fchem.2022.917831
Received: 11 April 2022; Accepted: 06 June 2022;
Published: 02 September 2022.
Edited by:
Mithun Kumar Ghosh, Govt College Hatta, IndiaReviewed by:
Sandip Kumar Chandraker, Indira Gandhi National Tribal University, IndiaRaja Selvaraj, Manipal Institute of Technology, India
Copyright © 2022 Krishna, Chandra Mishra, Naika, Gadewar, Ananthaswamy, Rao, Boselin Prabhu, Yatish, Nagendra, Moustafa, Al-Shehri, Jha, Lal and Stephen Santhakumari. This is an open-access article distributed under the terms of the Creative Commons Attribution License (CC BY). The use, distribution or reproduction in other forums is permitted, provided the original author(s) and the copyright owner(s) are credited and that the original publication in this journal is cited, in accordance with accepted academic practice. No use, distribution or reproduction is permitted which does not comply with these terms.
*Correspondence: Prashanth Gopala Krishna, cHJhc2hhbnRoX2NoZW1Ac2lybXZpdC5lZHU=, cHJhc2hhYW50aGdrQGdtYWlsLmNvbQ==; Saurabh Kumar Jha, c2F1cmFiaC5qaGFAc2hhcmRhLmFjLmlu
†ORCID: Prashanth Gopala Krishna, http://orcid.org/0000-0001-6691-4030; Saurabh Kumar Jha, https://orcid.org/0000-0002-7437-0755