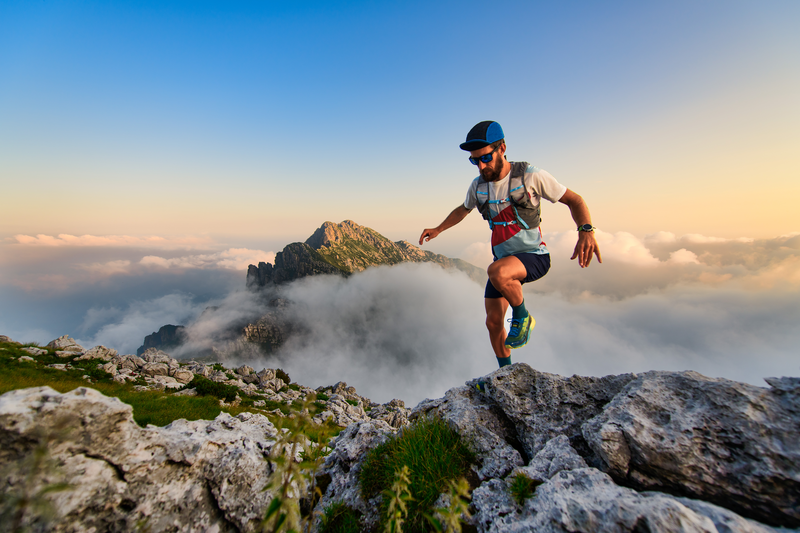
94% of researchers rate our articles as excellent or good
Learn more about the work of our research integrity team to safeguard the quality of each article we publish.
Find out more
ORIGINAL RESEARCH article
Front. Chem. , 08 June 2022
Sec. Electrochemistry
Volume 10 - 2022 | https://doi.org/10.3389/fchem.2022.915759
Carbon dioxide electroreduction (CO2RR) with renewable energy is of great significance to realize carbon neutralization. Traditional electrolysis devices usually need an ion exchange membrane to eliminate the interference of oxygen generated on the anode. Herein, the novel CuO/CeO2 composite was facilely prepared by anchoring small CuO nanoparticles on the surface of CeO2 nanocubes. In addition, CuO(002) crystal planes were induced to grow on CeO2(200), which was preferable for CO2 adsorption and C-C bond formation. As the catalyst in a membrane-free cell for CO2RR, the Cu+ was stabilized due to strong interactions between copper and ceria to resist the reduction of negative potentials and the oxidation of oxygen from the counter electrode. As a result, a high Faradaic efficiency of 62.2% toward C2 products (ethylene and ethanol) was achieved for the first time in the membrane-free conditions. This work may set off a new upsurge to drive the industrial application of CO2RR through membrane-free electrocatalysis.
• Nano CeO2 cube was applied as supports for highly dispersed CuO nanoparticles.
• The CuO(002) crystal faces were preferentially grown on CeO2(200).
• The Faradaic efficiency of CO2 to C2 exceeds 62% in a membrane-free cell.
Carbon dioxide electroreduction reaction (CO2RR) has attracted worldwide attention because of its promising application in suppressing carbon dioxide emission (Gong et al., 2019; Fan et al., 2020; Li H et al., 2021; Sun et al., 2021; Zhao et al., 2021; Li et al., 2022). However, it still needs high performance catalysts to accelerate the conversion of carbon dioxide and control the selectivity of products for the industrial application.
On different catalysts, various products may be obtained, such as carbon monoxide, formic acid, methanol, methane, ethylene, ethanol, oxalic acid, and acetic acid. Among them, the C2+ products were desired because of their much higher added value and more important theoretical significance of understanding C-C bond formation (Ma et al., 2020; Li Z et al., 2021; Zhang et al., 2021). Among all the elements, copper is considered as the only candidate as a high selective catalyst for C2+ products. In order to achieve better catalytic performance of copper-based catalysts, tremendous works have been carried out from the aspect of crystal faces (Suen et al., 2019), size (Wang et al., 2019), morphology (De Luna et al., 2018), defects (Gu et al., 2021), valence state (Mistry et al., 2016), and surface modification (Li F et al., 2020). Unfortunately, nearly all the pioneer works are executed in a two-chamber cell system, which need a cation or anion exchange membrane to separate the anode and cathode chambers. From a practical and industrial point of view, membranes make the CO2RR reactor more complex, increase cost, and limits large-scale application. Therefore, the design of a membrane-free electroreduction process is of great significance. Though with more concise and compact structure, the membrane-free cell still faces several critical challenges, such as the separation of products and the coupling with anodic reaction. For instance, the water oxidation reaction is inevitably on the anode when using aqueous electrolyte. In this condition, the oxygen produced and dissolved in the electrolyte may oxidize the active sites and lead to the decomposition of products in the liquid phase. In order to improve the oxygen tolerance of catalysts for CO2RR, several interesting works have been reported (Lu et al., 2019; He et al., 2020). For example, Sun et al. (Li P et al., 2020) developed a CO2-selective layer by introducing aniline into the pores of a microporosity polymer to permeate CO2 from O2 mixture. It was found that the acid–base interaction between CO2 and aniline enhances CO2 separation from O2. Even if the polymer coating is a feasible strategy to protect the active sites from interacting with oxygen, it is not conducive to the effective exposure of active sites. Therefore, new strategies need to be developed to achieve oxygen tolerance and full exposure of active sites simultaneously.
In addition to oxidation, the reduction of active sites in the CO2RR process also needs to be considered, whether there is a membrane or not. Especially for the oxide-derived copper (OD-Cu) catalysts, the Cu+ has been proposed as the most important active site in the formation of C-C bond, while its stability has always been a great challenge due to its easy reduction under negative applied potentials in the CO2RR procedure (Yuan et al., 2018). The strong metal–support interactions have been proved a promising way to improve catalytic activity of metal active sites, which is also applied to upgrade the catalytic performance of copper-based catalysts for the CO2RR (Geioushy et al., 2017). For example, Lee et al. (2019) sintered the CuO nanocrystals onto CeO2 and found that the ceria is proposed to weaken the hydrogen binding energy of adjacent Cu sites to stabilize the *OCCO intermediate via an additional chemical interaction with an oxygen atom in the *OCCO. Zheng et al. (Wang et al., 2018) designed single-atomic Cu–substituted mesoporous CeO2 nanorods with multiple oxygen vacancy bond. They found each Cu atom substituted in the CeO2(110) surface can be stabilized by coordinating with three oxygen vacancies, yielding a highly effective catalytic center for CO2 adsorption and activation to methane. On the contrary, Qiao et al. (Wu et al., 2018) proved the isolated cuprous ions doped in ceria nanorods exhibit strong capability to capture CO2 and CO molecules and decrease the energy barrier for producing C2 products, leading to a high Faradaic efficiency (FE) of 47.6% toward ethylene. On the other hand, the stabilization effects of ceria substrate toward cuprous ions are responsible for the long-term durability. Sun et al. (Chu et al., 2020a) found that the selectivity and activity of the CO2RR on Cu/CeO2 composites are depended strongly on the exposed crystal facets of CeO2. By tuning the CuO/CeO2 interfacial interaction, they further improved the selectivity of ethylene by stabilizing Cu+ at the CuO–CeO2 interface (Chu et al., 2020b). Han et al. (Yan et al., 2021) modified copper oxide with cerium oxide to enhanced water activation and accelerate the formation rate of *CHO, thus enhance the selectivity and activity of C2+ products by promoting the hydrogenation of *CHO. Though the existing works have proved that CeO2 is a promising candidate as the supports of copper in the traditional H-cell (with a membrane to separate two champers) for the CO2RR process, few works have been reported achieving a high selectivity toward C2 products in a membrane-free cell.
Herein, we synthesized a series of novel CuO/CeO2 composites by dispersing CuO nanoparticles (NPs) on CeO2 nonocubes (NCs), which is then used as catalysts for the CO2RR in a single chamber cell without membrane. Surprisingly, the Faradaic efficiency of C2 was found exceeding 62% for the first time. This new finding may set off a new research climax in a simple membrane-free cell for industrial perspective of CO2 electrocatalysis.
The fabrication of CuO/CeO2 is illustrated in Figure 1. The CeO2 NCs were synthesis by a modified hydrothermal method (Zhu et al., 2020). Through an impregnation–precipitation process, copper (II) ions were introduced into ceria lattice. After annealing at 773 K for 3 h, the CuO/CeO2 samples were obtained. The detailed composition of CuO/CeO2 composites is listed in Supplementary Table S1.
The crystal structure of samples as made was firstly investigated by XRD. As depicted in Figure 2A, the characteristic peaks of 28.6°, 33.1°, 47.5°, and 56.4° were ascribed to the (111), (200), (220) and (311) planes of CeO2, which is accurately corresponds to the standard spectrum of CeO2 (JCPDS No.03-065-0459, space group: Fd-3m Ia3̅, a = 10.774 Å). As a control experiment, we also synthesized CuO following the same procedure but in the absence of CeO2. The peaks at 38.8° was corresponded to the (111) planes of CuO (JCPDS No.80-1916). Interestingly, no characteristic peaks of CuO or Cu2O were found in the CuO/CeO2 composites, which need further characterization.
FIGURE 2. (A) XRD spectra of CuO, CuO/CeO2 and CeO2; (B) XPS survey spectra of CuO/CeO2; (C) XPS of Ce 3d spectra of CuO/CeO2 and CeO2; (D) XPS of Cu 2p spectra of CuO and CuO/CeO2.
The detailed element composition of CuO/CeO2 was analyzed by XPS spectrum. In Figure 2B, the characteristic peaks of Cu, Ce, and O elements were observed clearly, indicating that the CuO/CeO2 composites were successful prepared. The high-resolution XPS spectrum of Ce elopement is shown in Figure 2C. The Ce 3d curve was fitted to 10 peaks corresponding to Ce 3d5/2 (μ1, 880.5 eV; νo, 882.2 eV; μ0, 884.1 eV; ν1,888.7 eV; and ν2, 897.8 eV) and Ce 3d3/2 state (μ1′, 898.4 eV; ν0′, 900.7 eV; μ0′, 902.5 eV; ν1′, 907.5 eV; and ν2′, 916.6 eV). The peaks of μ0, μ1, μ0′, and μ1′ are attributed to Ce3+ species, and the remaining peaks are attributed to the Ce4+ species (Wang et al., 2018). According to the peak area ratio of the Ce 3d region of CuO/CeO2, the relative Ce3+ percentage in CeO2 is 17.6%, which is much less than that in the pure CeO2 (20.8% of Ce3+). This phenomenon is derived from the substitution of Ce ions by Cu ions during the synthesis procedure (Wu et al., 2018). The Cu 2p spectra of CuO/CeO2 and CuO show distinct copper oxide features in Figure 2D. In addition to the satellite peaks of Cu2+, the binding energies (BEs) of 934.2 eV in the Cu 2p3/2 region can be attributed to the Cu2+ and the peak at 932.7 eV is attributed to the Cu+ (Lei et al., 2020) According to the peak area ratio of copper oxidation states in the Cu 2p3/2 region, the relative Cu+ percentage of CuO dispersed on CeO2 is determined to be 38.4%, while the relative Cu+ percentage of pure copper oxide is 28.3%. The increased content of Cu+ was derived from the transfer of electrons from Ce3+ to Cu2+ (Chu et al., 2020b).
The morphology of CuO/CeO2 was verified by the SEM and TEM images. As shown in Figure 3A, the CeO2 NPs obtained in this work have an obviously cubic morphology with smooth surface, which size is ca. 23 nm. The morphology of CeO2 was confirmed by TEM (Figure 3B) to be classical cubes with clear boundaries. In the HRTEM image, the CeO2(200) crystal planes were mainly exposed (Figure 3C), which is confirmed by the SAED pattern (Figure 3D). After compositing with CuO, the cubic morphology and size of CeO2 was maintained but the smooth surface was mostly covered by the irregular CuO NPs with the diameter from 20 to 30 nm (Figures 3E,F).
FIGURE 3. (A) SEM images of CeO2; (B) TEM images of CeO2; (C) HRTEM images of CeO2; (D) SAED pattern of CeO2; (E) SEM images of CuO/CeO2; (F) TEM images of CuO/CeO2; (G) HRTEM images of CuO/CeO2; (H) SAED pattern of CuO/CeO2; (I–L) EDS mapping of CuO/CeO2.
It is worth noting that there is a clear boundary between CuO(002) and CeO2(200), as shown in Figure 3G. Furthermore, the SAED pattern of CuO/CeO2 (Figure 3H) confirmed that the CeO2(200) and CuO(002) planes exist simultaneously and the EDS mapping (Figures 3I–L) indicates the CuO NPs were uniformly dispersed on CeO2.
To verify the catalytic performance of the catalysts as made, the LSV curves of all the samples in 0.1 M KHCO3 with Ar- and CO2-saturated were recorded, as shown in Figure 4A. For the CuO/CeO2, larger current density was obtained in CO2-saturated 0.1 M KHCO3 than in the Ar-saturated conditions, indicating the CuO/CeO2 composites have obviously catalytic activity toward CO2RR (Chu et al., 2020b). As a control example, the LSV curves of CeO2 almost coincide in the Ar- and CO2-saturated conditions, indicating the ceria has no activity toward CO2RR.
FIGURE 4. (A) LSV curves of CeO2 and CuO/CeO2 under Ar- and CO2-saturated 0.1 M KHCO3 in a single cell; (B) Faradaic efficiency and C2 products current density of CuO/CeO2; (C) Faradaic efficiency and C2 products current density of CuO; (D) Faradaic efficiency of CeO2; (E) Comparison of Faraday efficiency of CuO/CeO2 and CuO at different voltages; (F) stability curve and Faradaic efficiency of C2 during 9 h.
Furthermore, the comprehensive catalytic performance of CO2RR on CuO/CeO2 under different applied potentials was investigated in a home-made single chamber electrolytic cell without membrane (Supplementary Figure S1). The Faradaic efficiencies of all the products were recorded in Figures 4B–D. Only a small amount of CO and methane were detected in the gas products, which means the CeO2 as catalyst has low activity toward CO2 electroreduction. While for the CuO/CeO2 samples, various products were detected by chromatography, including CO, CH4, C2H4, C2H5OH, and HCOOH. Take C2 (C2H4 + C2H5OH) as the target product, as shown in Figures 4C,F, the maximum Faraday efficiency of C2 always appears at −1.4 V vs. the reversible hydrogen electrode (RHE) and the FE of C2 arrived at the highest value of 62.2%. As control experiments, the maximum FE of C2 on pristine CuO under −1.4 V vs. RHE is only 40%, indicating the CeO2 as supports can increase the selectivity of CuO toward CO2 electroreduction to C2. For comparison, the FEs of products on the mixture of CuO and CeO2 (with the same ratio of Cu/Ce as CuO/CeO2) were also recorded and depicted in Supplementary Figure S3. The FE of C2 is 51.1% at −1.4 V vs. RHE, which is lower than that of CuO/CeO2 but higher than that of CuO, proving that the presence of CeO2 is conducive to the selectivity of C2 while the composited state of CuO and CeO2 has better performance in producing C2 products than the mixed state. Next, the partial current density was applied to evaluate the formation rate of target products. Specially at −1.4 V vs. RHE, the partial current density of C2 is 4.5 mA cm−2 on CuO/CeO2, which is higher than that on CuO (3.9 mA cm−2). While in the H-type cell, with the optimum CuO/CeO2 catalyst, the FE of C2 is 58.4% with a partial current density of 4.3 mA cm−2, as shown in Supplementary Figure S4. The higher selectivity and activity further prove the superiority of our catalyst in a single cell than the H-type cell.
We also tested the long-time durability of different catalysts in the membrane-free condition (Figure 4F and Supplementary Figure S5). After 9 h’ electrocatalysis at −1.4 V vs. RHE, the catalytic performance of CuO/CeO2 is stable with no significant change and the Faradaic efficiency for C2 remains around 60%. On the contrary, when the pristine CuO was used as catalyst, the current density had a noticeable increasing over time and the FE of C2 decreased from 40 to 21%, which mean the Cu+ in CuO cannot be stabilized and the selectivity of C2 was synchronously reduced.
In order to unveil the reason of different performance between CuO/CeO2 and pristine CuO, the composition and morphology of catalyst after electrocatalysis were characterized. As proved by SEM and TEM image in Figures 5A,B, the morphology of CuO/CeO2 was well maintained after reaction. In addition, the XPS results further revealed that the ratio of Cu+/(Cu+ + Cu2+) has no significant change compared to the fresh catalyst, indicates Cu+ can be well stabilized by CeO2 during the CO2RR process. The stability of the morphology and valence state of the CuO/CeO2 composite further proves that the stabilization effects of ceria toward Cu+, which provides favorable catalysts for the high-efficiency electroreduction of carbon dioxide in a membrane-free electrolyzer.
FIGURE 5. (A) SEM images of CuO/CeO2; (B) TEM images of CuO/CeO2; (C) XPS of Ce 3d spectra of CuO/CeO2; (D) Cu 2p spectra of CuO/CeO2 after CO2RR.
In this work, the CeO2 NCs with average size of 230 nm were facilely synthesized by a hydrothermal method. Further through an impregnation–calcination process, the CuO NPs with diameters from 20 to 30 nm was highly dispersed on the surface of CeO2 to fabricate novel composites of CuO/CeO2, in which the mainly exposed crystal faces was CuO(002) and CeO2(200). Due to the strong metal–metal oxide interaction between copper and ceria, the content of Cu+ was increased in the CuO/CeO2 composite and stabilized in the CO2RR process, which makes the CuO/CeO2 an excellent catalyst for CO2RR toward C2 products with high selectivity and stability in a single cell without membrane. This study opens up a new way to realize the high-efficiency electroreduction of CO2 by using a simple membrane-free system.
The original contributions presented in the study are included in the article/Supplementary Material; further inquiries can be directed to the corresponding authors.
YT: experiments and writing. XF and DFT: calculation. HN: experimental guidance. WW: experimental assistant. XT: experimental assistant. XW: experimental assistant. ZM: experimental assistant. ZG: experimental assistant. MW: article review.
This work is financially supported by the National Natural Science Foundation of China (52072409), Major Scientific and Technological Innovation Project of Shandong Province (2020CXGC010403), and Taishan Scholar Project (No. ts201712020).
The authors declare that the research was conducted in the absence of any commercial or financial relationships that could be construed as a potential conflict of interest.
All claims expressed in this article are solely those of the authors and do not necessarily represent those of their affiliated organizations, or those of the publisher, the editors, and the reviewers. Any product that may be evaluated in this article, or claim that may be made by its manufacturer, is not guaranteed or endorsed by the publisher.
The Supplementary Material for this article can be found online at: https://www.frontiersin.org/articles/10.3389/fchem.2022.915759/full#supplementary-material
Chu, S., Li, X., W. Robertson, A., and Sun, Z. (2020a). Electrocatalytic CO2 Reduction to Ethylene over CeO2-Supported Cu Nanoparticles: Effect of Exposed Facets of CeO2. Acta Phys. Chim. Sin. 37, 2009023–2009020. doi:10.3866/pku.whxb202009023
Chu, S., Yan, X., Choi, C., Hong, S., Robertson, A. W., Masa, J., et al. (2020b). Stabilization of Cu+ by Tuning a CuO-CeO2 Interface for Selective Electrochemical CO2 Reduction to Ethylene. Green Chem. 22, 6540–6546. doi:10.1039/d0gc02279a
De Luna, P., Quintero-Bermudez, R., Dinh, C.-T., Ross, M. B., Bushuyev, O. S., Todorović, P., et al. (2018). Catalyst Electro-Redeposition Controls Morphology and Oxidation State for Selective Carbon Dioxide Reduction. Nat. Catal. 1, 103–110. doi:10.1038/s41929-017-0018-9
Fan, Q., Hou, P., Choi, C., Wu, T. S., Hong, S., Li, F., et al. (2020). Activation of Ni Particles into Single Ni-N Atoms for Efficient Electrochemical Reduction of CO2. Adv. Energy Mat. 10, 1903068. doi:10.1002/aenm.201903068
Geioushy, R. A., Khaled, M. M., Alhooshani, K., Hakeem, A. S., and Rinaldi, A. (2017). Graphene/ZnO/Cu2O Electrocatalyst for Selective Conversion of CO2 into N-Propanol. Electrochimica Acta 245, 456–462. doi:10.1016/j.electacta.2017.05.185
Gong, Q., Ding, P., Xu, M., Zhu, X., Wang, M., Deng, J., et al. (2019). Structural Defects on Converted Bismuth Oxide Nanotubes Enable Highly Active Electrocatalysis of Carbon Dioxide Reduction. Nat. Commun. 10, 2807. doi:10.1038/s41467-019-10819-4
Gu, Z., Shen, H., Chen, Z., Yang, Y., Yang, C., Ji, Y., et al. (2021). Efficient Electrocatalytic CO2 Reduction to C2+ Alcohols at Defect-Site-Rich Cu Surface. Joule 5, 429–440. doi:10.1016/j.joule.2020.12.011
He, M., Li, C., Zhang, H., Chang, X., Chen, J. G., Goddard, W. A., et al. (2020). Oxygen Induced Promotion of Electrochemical Reduction of CO2 via Co-electrolysis. Nat. Commun. 11, 3844. doi:10.1038/s41467-020-17690-8
Lee, C. W., Shin, S.-J., Jung, H., Nguyen, D. L. T., Lee, S. Y., Lee, W. H., et al. (2019). Metal-Oxide Interfaces for Selective Electrochemical C-C Coupling Reactions. ACS Energy Lett. 4, 2241–2248. doi:10.1021/acsenergylett.9b01721
Lei, Q., Zhu, H., Song, K., Wei, N., Liu, L., Zhang, D., et al. (2020). Investigating the Origin of Enhanced C2+ Selectivity in Oxide-/Hydroxide-Derived Copper Electrodes during CO2 Electroreduction. J. Am. Chem. Soc. 142, 4213–4222. doi:10.1021/jacs.9b11790
Li, F., Thevenon, A., Rosas-Hernández, A., Wang, Z., Li, Y., Gabardo, C. M., Ozden, A., Dinh, C. T., Li, J., Wang, Y., Edwards, J. P., Xu, Y., McCallum, C., Tao, L., Liang, Z.-Q., Luo, M., Wang, X., Li, H., O’Brien, C. P., Tan, C.-S., Nam, D.-H., Quintero-Bermudez, R., Zhuang, T.-T., Li, Y. C., Han, Z., Britt, R. D., Sinton, D., Agapie, T., Peters, J. C., and Sargent, E. H. (2020). Molecular Tuning of CO2-to-ethylene Conversion. Nature 577, 509–513. doi:10.1038/s41586-019-1782-2
Li, H., Liu, T., Wei, P., Lin, L., Gao, D., Wang, G., et al. (2021). High‐Rate CO2 Electroreduction to C2+ Products over a Copper‐Copper Iodide Catalyst. Angew. Chem. Int. Ed. 60, 14329–14333. doi:10.1002/anie.202102657
Li, P., Lu, X., Wu, Z., Wu, Y., Malpass‐Evans, R., McKeown, N. B., et al. (2020). Acid-Base Interaction Enhancing Oxygen Tolerance in Electrocatalytic Carbon Dioxide Reduction. Angew. Chem. Int. Ed. 59, 10918–10923. doi:10.1002/anie.202003093
Li, Y., Zhang, K., Yu, Y., Zhan, X., Gui, J., Xue, J., et al. (2022). Pd Homojunctions Enable Remarkable CO2 Electroreduction. Chem. Commun. 58, 387–390. doi:10.1039/d1cc04780a
Li, Z., Yang, Y., Yin, Z., Wei, X., Peng, H., Lyu, K., et al. (2021). Interface-Enhanced Catalytic Selectivity on the C2 Products of CO2 Electroreduction. ACS Catal. 11, 2473–2482. doi:10.1021/acscatal.0c03846
Lu, X., Jiang, Z., Yuan, X., Wu, Y., Malpass-Evans, R., Zhong, Y., et al. (2019). A Bio-Inspired O2-Tolerant Catalytic CO2 Reduction Electrode. Sci. Bull. 64, 1890–1895. doi:10.1016/j.scib.2019.04.008
Ma, W., Xie, S., Liu, T., Fan, Q., Ye, J., Sun, F., et al. (2020). Electrocatalytic Reduction of CO2 to Ethylene and Ethanol through Hydrogen-Assisted C-C Coupling over Fluorine-Modified Copper. Nat. Catal. 3, 478–487. doi:10.1038/s41929-020-0450-0
Mistry, H., Varela, A. S., Bonifacio, C. S., Zegkinoglou, I., Sinev, I., Choi, Y.-W., et al. (2016). Highly Selective Plasma-Activated Copper Catalysts for Carbon Dioxide Reduction to Ethylene. Nat. Commun. 7, 12123. doi:10.1038/ncomms12123
Suen, N.-T., Kong, Z.-R., Hsu, C.-S., Chen, H.-C., Tung, C.-W., Lu, Y.-R., et al. (2019). Morphology Manipulation of Copper Nanocrystals and Product Selectivity in the Electrocatalytic Reduction of Carbon Dioxide. ACS Catal. 9, 5217–5222. doi:10.1021/acscatal.9b00790
Sun, Q., Ren, W., Zhao, Y., and Zhao, C. (2021). Gram-scale Synthesis of Single-Atom Metal-N-CNT Catalysts for Highly Efficient CO2 Electroreduction. Chem. Commun. 57, 1514–1517. doi:10.1039/d0cc07263j
Wang, W., Ning, H., Yang, Z., Feng, Z., Wang, J., Wang, X., et al. (2019). Interface-induced Controllable Synthesis of Cu2O Nanocubes for Electroreduction CO2 to C2H4. Electrochimica Acta 306, 360–365. doi:10.1016/j.electacta.2019.03.146
Wang, Y., Chen, Z., Han, P., Du, Y., Gu, Z., Xu, X., et al. (2018). Single-Atomic Cu with Multiple Oxygen Vacancies on Ceria for Electrocatalytic CO2 Reduction to CH4. ACS Catal. 8, 7113–7119. doi:10.1021/acscatal.8b01014
Wu, D., Dong, C., Wu, D., Fu, J., Liu, H., Hu, S., et al. (2018). Cuprous Ions Embedded in Ceria Lattice for Selective and Stable Electrochemical Reduction of Carbon Dioxide to Ethylene. J. Mat. Chem. A 6, 9373–9377. doi:10.1039/c8ta01677a
Yan, X., Chen, C., Wu, Y., Liu, S., Chen, Y., Feng, R., et al. (2021). Efficient Electroreduction of CO2 to C2+ Products on CeO2 Modified CuO. Chem. Sci. 12, 6638–6645. doi:10.1039/d1sc01117k
Yuan, J., Zhang, J.-J., Yang, M.-P., Meng, W.-J., Wang, H., and Lu, J.-X. (2018). CuO Nanoparticles Supported on TiO2 with High Efficiency for CO2 Electrochemical Reduction to Ethanol. Catalysts 8, 171. doi:10.3390/catal8040171
Zhang, J., Li, Z., Xia, S., Zhang, T., Wang, Y., Wu, Y., et al. (2021). Reconstructing Two-Dimensional Defects in CuO Nanowires for Efficient CO2 Electroreduction to Ethylene. Chem. Commun. 57, 8276–8279. doi:10.1039/d1cc03171f
Zhao, Z.-H., Zheng, K., Huang, N.-Y., Zhu, H.-L., Huang, J.-R., Liao, P.-Q., et al. (2021). A Cu(111)@metal-Organic Framework as a Tandem Catalyst for Highly Selective CO2 Electroreduction to C2H4. Chem. Commun. 57, 12764–12767. doi:10.1039/d1cc05376k
Keywords: carbon dioxide, membrane free, electrocatalysis, copper, ceria
Citation: Tian Y, Fei X, Ning H, Wang W, Tan X, Wang X, Ma Z, Guo Z and Wu M (2022) Membrane-free Electrocatalysis of CO2 to C2 on CuO/CeO2 Nanocomposites. Front. Chem. 10:915759. doi: 10.3389/fchem.2022.915759
Received: 08 April 2022; Accepted: 29 April 2022;
Published: 08 June 2022.
Edited by:
Juqin Zeng, Italian Institute of Technology (IIT), ItalyReviewed by:
Yuxiang Hu, The University of Queensland, AustraliaCopyright © 2022 Tian, Fei, Ning, Wang, Tan, Wang, Ma, Guo and Wu. This is an open-access article distributed under the terms of the Creative Commons Attribution License (CC BY). The use, distribution or reproduction in other forums is permitted, provided the original author(s) and the copyright owner(s) are credited and that the original publication in this journal is cited, in accordance with accepted academic practice. No use, distribution or reproduction is permitted which does not comply with these terms.
*Correspondence: Hui Ning, bmluZ2h1aUB1cGMuZWR1LmNu; Mingbo Wu, d3VtYkB1cGMuZWR1LmNu
Disclaimer: All claims expressed in this article are solely those of the authors and do not necessarily represent those of their affiliated organizations, or those of the publisher, the editors and the reviewers. Any product that may be evaluated in this article or claim that may be made by its manufacturer is not guaranteed or endorsed by the publisher.
Research integrity at Frontiers
Learn more about the work of our research integrity team to safeguard the quality of each article we publish.