- 1Changshu No. 2 People’s Hospital, Changshu, Jiangsu, China
- 2Central Laboratory of Changshu Medical Examination Institute, Changshu, China
- 3School of Pharmacy, Guilin Medical University, Guilin, China
- 4Department of Materials Engineering, Changshu Institute of Technology, Changshu, China
Two Cu(II) compounds based on tetrazole-carboxylate ligands, [Cu(phtza)2(H2O)2]∙3H2O (1) and [Cu(atzipa)2]∙2H2O (2) (phtza = 2,2'-(5,5'-(1,3-phenylene)bis(2H-tetrazole-5,2-diyl))diacetate, atzipa = 3-(5-amino-1H-tetrazol-1-yl)isopropanoic anion), were designed and synthesized by hydrothermal reactions. The X-ray diffraction results show that the two compounds show two-dimensional (2D) layer structures. Nanoprecipitation with 1,2-distearoyl-sn-glycero-3-phosphoethanolamine-N-[methoxy(polyethylene glycol)-2000] (DSPE-PEG-2000) contributes to the formation of the nanoparticles (NPs) with excellent water dispersity. In vitro study indicates that the two NPs exert considerable cytotoxicity toward human hepatocellular carcinoma cells (HepG2 and Huh7) with low half-maximal inhibitory concentration (IC50). However, the cytotoxicity of such NPs is negligible in normal cells (HL-7702). The cytotoxicity of these NPs was also investigated by the flow cytometry and Calcein-AM/PI (live/dead) co-stained experiments. The results promise the great potential of these NPs for chemodynamic therapy against cancer cells.
Introduction
Cancer has already become a tremendous threat to health worldwide, following heart and cardiovascular diseases, and global mortality keeps rising (Siegel et al., 2018). Traditional therapeutic methods, for example, chemotherapy usually employs drugs, such as cisplatin (II) and doxorubicin, for the treatment of cancer (Tang et al., 2018; Song et al., 2019; Zhu et al., 2020). Normal cells may still suffer from side effects because the targeting ability of the compounds is very poor, though such drugs achieve considerable therapeutic efficacy to some extent. Therefore, designing and synthesizing anticancer drugs with specific targeting ability to avoid the side effects and enhance the therapeutic efficacy is an effective alternative to solve the problem (Fujita et al., 2014; Weiss et al., 2014; Sun et al., 2017a; Sun et al., 2017b; Yang et al., 2018; Yang et al., 2019a; Yang et al., 2019b; Yang et al., 2020; Zou et al., 2020). Tumor microenvironment (TME), usually features hypoxia and high hydrogen peroxide (H2O2) concentration, compared with that in normal tissues (Arneth, 2020; Deng et al., 2020). Chemodynamic therapy (denoted as CDT) can induce cell death by catalyzing H2O2 to generate cytotoxic hydroxyl radicals (⋅OH) through Fenton or Fenton-like reactions, typically Fe(II) or Cu(II) compounds (Fujita et al., 2014; Li et al., 2018; Yang et al., 2019c; Shen et al., 2019; Li et al., 2020). For example, Chen et al. designed a kind of nanosystem that is able to generate free radicals by iron pool for cancer chemodynamic therapy (H Zou et al., 2021). CDT is considered a non-invasive strategy to fight against cancer.
Coordination compounds, a class of functional materials, are attracting increasing interest owing to not only their diverse structures but also their great potential in the field of luminescence, adsorption, and catalysis (Aromi et al., 2011; Wriedt et al., 2012; Zou et al., 2014a; Zou et al., 2015a; Du et al., 2015; Shen et al., 2016; Zhang et al., 2016; Lin et al., 2019; Zou et al., 2021). Tetrazole carboxylates are bi-functional ligands with either flexible carboxylate groups or rigid tetrazole rings that have great potential for constructing a variety of coordination architectures. These ligands with abundant nitrogen and oxygen atoms have a high possibility to show diverse coordination modes, and the CH2 is capable of favoring the carboxylate with flexible orientations, contributing to the formation of novel crystal structures (Zou et al., 2014b; Zou et al., 2014c; Zou et al., 2014d; Zou et al., 2015b; Dai et al., 2021). In the previous study, tetrazole-based ligands have been universally employed as multi-building blocks for the construction of novel coordination architectures and in the fields of luminescence (Zou et al., 2014b; Zou et al., 2014c; Zou et al., 2014d; Yang et al., 2014; Zou et al., 2015b; Sun et al., 2016; Zhang et al., 2016; Dai et al., 2021), catalysis (Zou et al., 2015a), and adsorption (Wriedt et al., 2012). However, the biological applications of such compounds are relatively less reported. Based on the observations, we are devoted to designing and synthesizing novel Cu(II) compounds based on two different tetrazole carboxylates, 2,2'-(5,5'-(1,3-phenylene)bis(2H-tetrazole-5,2-diyl))diacetic acid (Hphtza) and Hatzipa = 3-(5-amino-1H-tetrazol-1-yl)isopropanoic acid) (Scheme 1). As a result, [Cu(phtza)2(H2O)2]∙3H2O (1) and [Cu(atzipa)2]∙2H2O (2) were obtained by hydrothermal reactions. The nanoparticles (NPs) of the two compounds were synthesized by a nano-precipitation method with encapsulation by 1,2-distearoyl-sn-glycero-3-phosphoethanolamine-N-[methoxy(polyethylene glycol)-2000] (DSPE-PEG-2000). Further in vitro study suggests the two NPs show high cytotoxicity toward human hepatocellular carcinoma cells (HepG2 and Huh7). In terms of cytotoxicity, compound 1 is superior to compound 2 on HepG2 (IC50: 58.3 μM for compound 1 NPs and 83.6 μM for compound 2 NPs), while both complexes have IC50 values of 45.5 μM on Huh7 cells. Eventually, the Calcein-AM/PI (PI = 3,8-diamino-5-(3-diethylaminopropyl)-6-phenylphenanthridinium iodide) staining and flow cytometry results demonstrate that these compounds are able to induce cell apoptosis for efficient chemodynamic therapy.
Experimental section
Materials and apparatus
H2phtza and Hatzipa were prepared according to the literature (Yang et al., 2014; Sun et al., 2016). The chemicals were commercially available from Sigma-Aldrich (Shanghai). Elemental analyses (C, H, and N) were performed with a PE2400 elemental analyzer. The Fourier transform infrared (FT-IR) spectrum was measured on the Thermo NICOLET 380 instrument as a KBr disk (4,000–400 cm−1). The UV-vis spectra were obtained from a UV-3600 spectrometer (Shimadzu, Japan).
Preparation and characterization of compounds 1 and 2
A mixture of Cu(NO3)2·3H2O (241 mg, 0.1 mmol) and H2phtza (165 mg, 0.05 mmol) in a mixture of water (2 ml) and ethanol (2 ml) was sealed in a stainless steel container and heated at 120°C for 24 h. After cooling to room temperature, the solution was filtered for evaporation. Blue crystals were obtained. Elemental analyses Calcd. for C12H18CuN8O9 (%): C, 29.91; H, 3.74; N, 23.25. Found (%): C, 29.73; H, 3.77; N, 23.40. IR (KBr, cm−1): 3,022 (w), 2,901 (w), 2,245(w), 1,623 (m), 1,577 (m), 1,521 (s), 1,124 (vs), 1,088 (s), 935 (w), 868 (m), 725 (m), and 623 (s).
The synthesis procedure for compound 2 is similar to that of 1 except that H2phtza has been replaced by Hatzipa (79 mg, 0.05 mmol). Elemental analyses Calcd. for C36H32Cl2CuN8O10 (%): C, 23.33; H, 3.88; N, 34.00. Found (%): C, 23.43; H, 3.82; N, 34.50. IR (KBr, cm−1): 3,440 (m), 3,088 (w), 2,933 (w), 1,635 (w), 1,568 (m), 1,494 (s), 1,385 (m), 1,114 (vs), 925 (w), 855 (w), 738 (m), and 629 (s).
Crystal structure determination
Suitable single crystals of 1 and 2 were selected for collection of intensity data on a Bruker SMART APEX II CCD diffractometer using a ϕ-ω scan mode at 291 K for compound 1 and 296(2) K for compound 2 [monochromate Mo Kα radiation (λ = 0.71073 Å)]. SADABS was applied for multi-scan absorption corrections of all intensity data. The structures were solved by direct methods, and SHELXTL software was used to refine F2 by full-matrix least squares procedures (Sheldrick, 2008). All hydrogen atoms were fixed in calculated positions and refined isotropically. The crystallographic data of compounds 1 and 2 are listed in Table 1. The selected bond lengths and angles are shown in Table 2. The hydrogen bond parameters are listed in Supplementary Table S1.
Hydroxyl generation by Fenton-like reaction
10 μg mL−1 MB, 8 mM H2O2, and 0.5 mM compounds 1 or 2 NPs were allowed to stand at 37°C for 30 min. The OH-induced MB degradation was monitored by the absorbance change at 665 nm.
Synthesis of compounds 1 and 2 nanoparticles
The nanoparticles (NPs) were prepared by nanoprecipitation with DSPE-PEG-2000. A mixture of DSPE-PEG-2000 (10 mg) and compound 1 or 2 (2 mg) was dissolved in tetrahydrofuran (THF) under ultrasound (100 W). Then, such a solution was injected into 5 ml distilled water under ultrasound. After the mixture was sonicated in an ultrasonic washer for 3–5 days, THF was removed by purging nitrogen gas at room temperature. The solution was stored at 4°C for characterization and cytotoxicity experiment.
Cell culture and cytotoxicity assay
Hepatocellular carcinoma cell lines (HCC, including HepG2 and Huh7) and human normal cells (HL-7702) were available from the Shanghai Institute of Biochemistry and Cell Biology, Chinese Academy of Sciences (CAS). HepG2 and Huh7 cells were cultured in DMEM (Biosharp; Biosharp Life Sciences) with 10% FBS (Biosharp; Biosharp Life Sciences) at 37°C with 5% CO2.
Cell viability was determined using a Cell Counting Kit. HepG2 cells (2 × 103/well), Huh7 cells (2 × 103/well), and HL-7702 (2 × 103/well) were seeded into 96-well plates. The cells were cultured with the NPs of compounds 1 and 2 for 24 h, respectively. Cytotoxicity was evaluated using the Cell Counting Kit-8 (CCK-8, Abbkine Scientific). CCK-8 was added to the medium and incubated with the cells for 2 h. Then, the absorbance was measured on a microplate reader. The cell viability was calculated as follows:
where Atreatment = mean absorbance of the medium from cells incubated with NPs containing complex 1 or 2 and Acontrol = mean absorbance of the medium incubated without NPs of non-treated cells. The half-maximal inhibitory concentration (IC50) was calculated using SPSS 25.0 software.
The MTT assay was repeated three times.
Flow cytometry
Cell apoptosis of the nanoparticles was detected by flow cytometry. Generally, HepG2 cells (2 × 105/well) and Huh7 cells (2 × 105/well) were seeded in 6-well plates and cultured for 24 h. Then, the cells were co-cultured with NPs of compound 1 or 2 for 24 h at the concentration of IC50 and 2 × IC50, respectively. Cell apoptosis was detected by the FITC Annexin V Apoptosis Detection Kit I on the flow cytometry (Attune NxT, Invitrogen by Thermo Fisher Scientific). The flow cytometry was repeated three times.
Live/dead co-staining by Calcein-AM and PI
HepG2 and Huh7 (both concentration ∼2 × 104/well) were seeded in 24-well plates. In this experiment, we set up control groups, IC50 groups and 2 × IC50 groups, respectively. Calcein AM is a cell-permeant dye that can be used to determine cell viability in most eukaryotic cells. In live cells, the non-fluorescent calcein AM is converted to a green-fluorescent calcein after acetoxymethyl ester hydrolysis by intracellular esterases. Calcein AM is used to stain live cells (green channel), while PI is used to stain dead cells (red channel). In the control group, the cells were cultured, and then the cells were treated with compounds 1 and 2 NPs for 24 h at the concentration of IC50 and 2 × IC50, respectively. Then, the medium was discarded, and the cells were washed with PBS three times. The cells were co-stained with a Living/Dead cell double staining kit (Sigma-Aldrich (Shanghai) Trading Co., Ltd.) with the concentration of calcein-AM at 2.5 μM and PI 4.5 μM, respectively. After 20 min, the cells were washed with PBS three times. The photographs were captured on an inverted fluorescence microscope, Olympus FV1000 confocal microscope.
Statistical analysis
All numeric data are expressed as mean ± s.d. unless otherwise indicated. The significance between the two groups was analyzed by a two-tailed Student’s t-test. Statistical analysis was performed using GraphPad Prism 6.0. p values of less than 0.05 were considered significant (**p < 0.01 and ***p < 0.01).
Results and discussions
Description of crystal structures of [Cu(phtza)2(H2O)2]∙3H2O (1) and [Cu(atzipa)2]∙2H2O (2)
X-ray crystallography results reveal that both compounds 1 and 2 crystallize in monoclinic lattice with space group P21/c. Compound 1 is made up of one Cu(II), two phtza− anions, two coordinated water, and three lattice water molecules, while compound 2 consists of one Cu(II), two atzipa− anions, and two lattice water (Figures 1A, 2A). The phtza ligand is a tetra-dentate to bridge the adjacent Cu(II) centers by two carboxylate oxygen atoms in a μ1,1,3-COO or a μ1-COO mode, respectively, to generate a two-dimensional (2D) layer structure parallel to the ac plane (Figure 1B). In contrast, atzipa in compound 2 adopts a bridging mode by the tetrazole nitrogen atoms one carboxylate oxygen, thereby also forming a 2D layer structure parallel to the bc plane (Figure 2B). The Cu-O bond distance of compound 1 is 1.943 Å and that of compound 2 ranges from 1.936 to 2.210 Å, while the Cu-N bond distance of compound 1 is 1.999 Å. Adjacent 2D layers are connected together by hydrogen bonding to generate a three-dimensional (3D) supramolecular structure (Supplementary Figures S1, S2; Supplementary Table S1). Compared with the previously reported mononuclear [Cu(2-pytzipa)2(H2O)2]·2H2O (Zhai et al., 2017) (2-pytzipa = 5-(2-pyridyl)tetrazole-2-isopropanoic anion) in which 2-pytzipa only adopts the N (pyridyl) and N(tetrazole) chelating mode, the coordination modes of phtza and atzipa are more complicated. 3-pytza in [Cu(3-pytza)2(H2O)]n·2nH2O (Zou et al., 2014e) shows a N(pyridyl) and O (COO−) bridging mode, which is similar to that of atzipa in compound 2. All the nitrogen atoms in phtza in compound 1 are all uncoordinated.
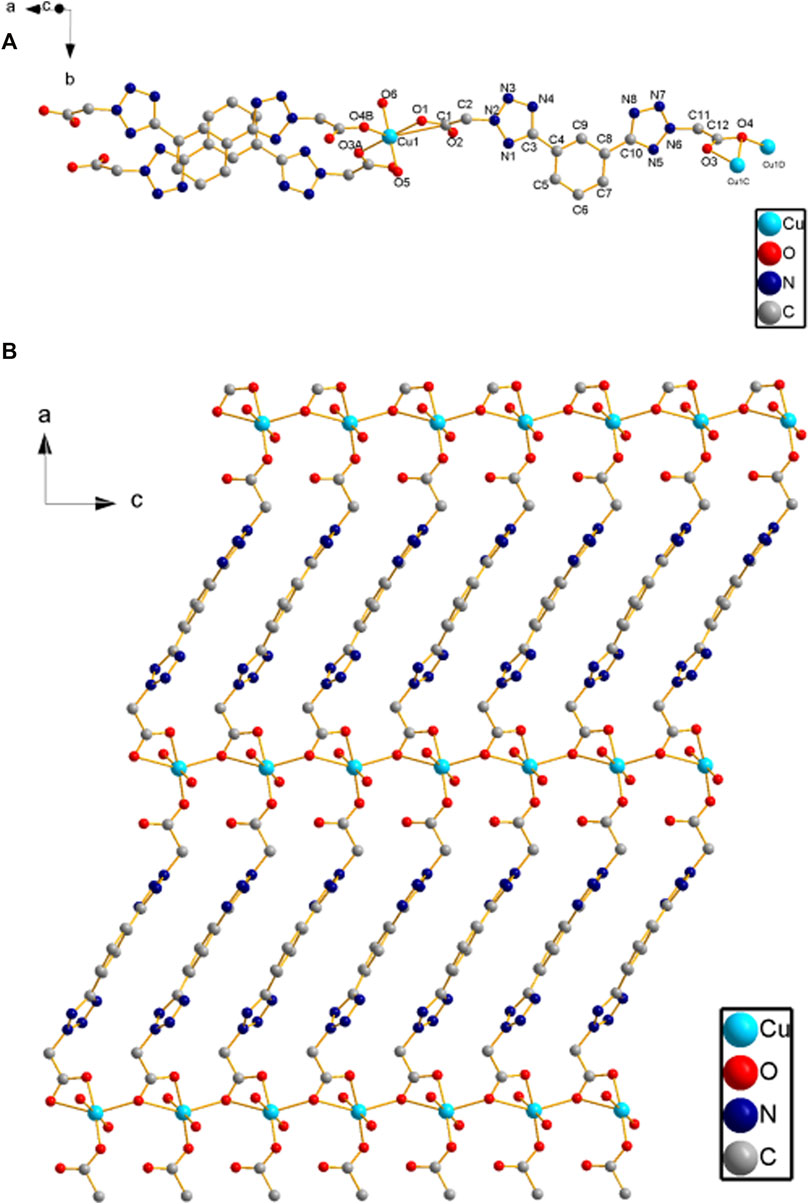
FIGURE 1. (A) Coordination environment of Cu(II) in compound 1. (B) 2D layer structure of compound 1 parallel to the ac plane. Hydrogen atoms are omitted for clarity.
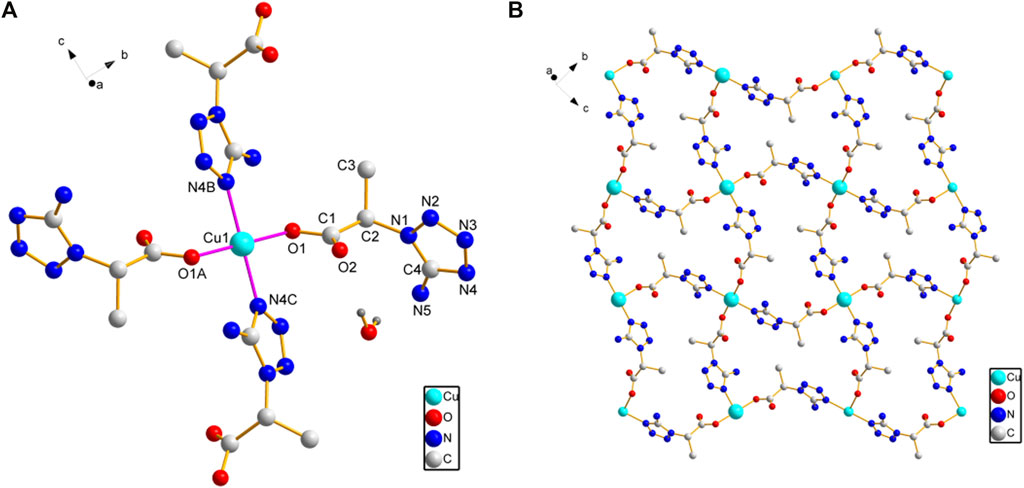
FIGURE 2. (A) Coordination environment of Cu(II) in compound 2. (B) 2D layer structure of compound 2 parallel to the bc plane. Hydrogen atoms are omitted for clarity.
Since methylene blue (MB) can be degraded by hydroxyl radicals, the hydroxyl radicals’ generation ability of compounds 1 and 2 NPs was investigated by recording the absorbance of methylene blue (MB) in the presence of H2O2 (Figure 3). The absorbance of MB continued to decrease because of the generation of hydroxyl radicals catalyzed by the Fenton-like reaction of compounds 1 or 2 NPs and H2O2. In addition, compound 1 NPs are superior to compound 2 NPs in terms of catalysis.
Cytotoxicity and flow cytometry
The nanoparticles (NPs) of compounds 1 and 2 were prepared by nanoprecipitation with DSPE-PEG-2000 due to their excellent dispersity and stability in aqueous solution. Two different human hepatocellular carcinoma cells, HepG2 and Huh7, were chosen to investigate the chemodynamic therapeutic efficacy of NPs of compounds 1 and 2 by the Cell Counting Kit-8 (CCK-8) assay. CCK-8 allows sensitive colorimetric assays for the determination of cell viability in cell proliferation and cytotoxicity assays. The results showed concentration-dependent cell death after two types of liver cancer cells were treated with NPs of compound 1 or 2 under different concentrations (Figure 4). With the increase of the concentrations, the viability of HepG2 and Huh7 cells tends to gradually reduce. For HepG2 cells, the IC50 values of compounds 1 and 2 NPs are 58.3 and 83.6 μM, respectively. For Huh7 cells, the IC50 of both NPs is 45.5 μM. Both compounds have strong inhibitory effects on the cell activity of the two cell lines. Compound 2 is superior to compound 1 on HepG2 cells, while it is parallel to compound 1 on Huh7 cells in terms of cytotoxicity. Huh7 cells are more sensitive to the NPs containing complexes 1 and 2 than HepG2 cells. Compared with the relevant Cu(II) compounds based on tetrazole ligands, the cytotoxicity of the two compounds is lower than that of [Cu(atzpa)2] and [Cu(pytzipa)2] (Zhang et al., 2021) but higher than that of [Cu(2-pytzipa)2(H2O)2]·2H2O (Zhai et al., 2017) (Table 3). The cytotoxicity of these NPs is very low toward healthy hepatocytes because the cell viability remained high (Supplementary Figures S3, S4).
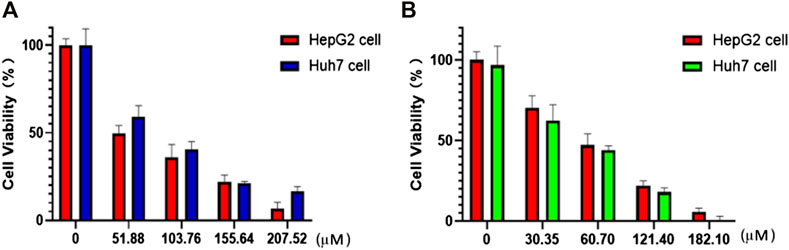
FIGURE 4. In vitro CCK8 assay of HepG2 and Huh7 cells treated with (A) compound 1 NPs and (B) compound 2 NPs.
The cytotoxicity of compounds 1 and 2 NPs was further confirmed by flow cytometry, a technique used to detect and measure the physical and chemical characteristics of a population of cells or particles. The results show that the cell apoptotic rates of HepG2 and Huh7 cells in the groups treated with compounds 1 and 2 were significantly increased but markedly different. In particular, all the groups of 2 × IC50 were significantly higher than IC50 groups, and the apoptosis rates (Q2 + Q3) of the control groups were rather low (Table 4). For the control group, both HepG2 and Huh7 cell viability are very high (87.27% for HepG2 and 93.99% for Huh7), while concentration-dependent cell viability was observed for those treated with compounds 1 or 2 NPs. These results demonstrated that the compound 1 and compound 2 NPs could induce and promote cell apoptosis of Huh7 and HepG2 cells (Figure 5). Late apoptosis of the cells treated with compound 1 NPs was detected, while both necrosis and late apoptosis were detected for the cells incubated with compound 2 NPs.
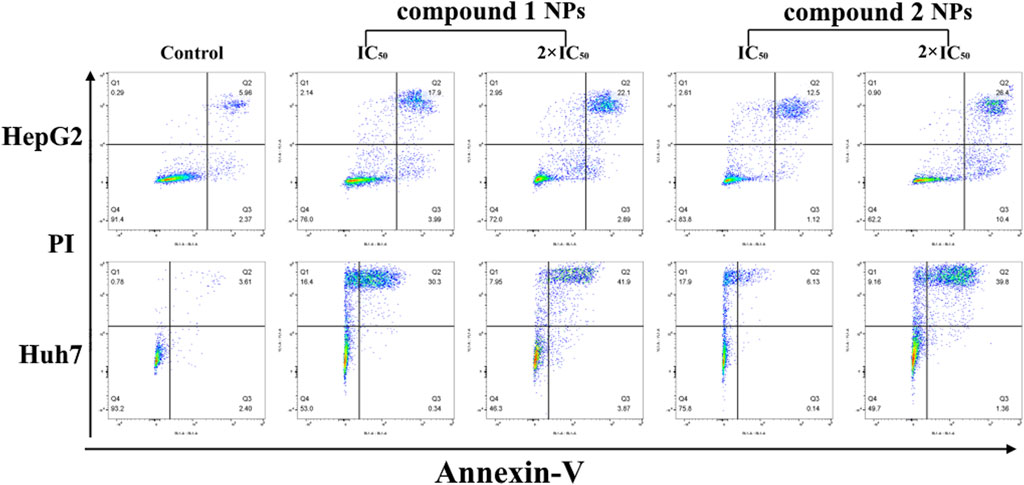
FIGURE 5. Flow cytometry results of compounds 1 and 2 NPs on HepG2 and Huh7 under different concentrations.
Live/dead co-staining by Calcein-AM and PI
After the discovery of the inhibitory and toxicity effects of compounds 1 and 2 on the HCC cells. The cell viability was investigated by the live/dead co-staining (Figure 6), where living cells can be stained with calcein AM to show green fluorescence and dead cells with PI to exhibit red fluorescence. The cells in control groups expressed large areas of strong green fluorescence, and the faint red fluorescence was almost negligible, indicating that all cells were alive. However, in the groups treated with compound 1 and compound 2 NPs, a large number of cells were stained with red fluorescence. Cell death is more obvious in the treatment groups with a concentration of 2 × IC50 than that with IC50 (Figure 6). The quantitative fluorescence intensity of the calcein AM and PI also indicates that compounds 1 and 2 NPs can induce cell apoptosis by CDT (Figure 7).
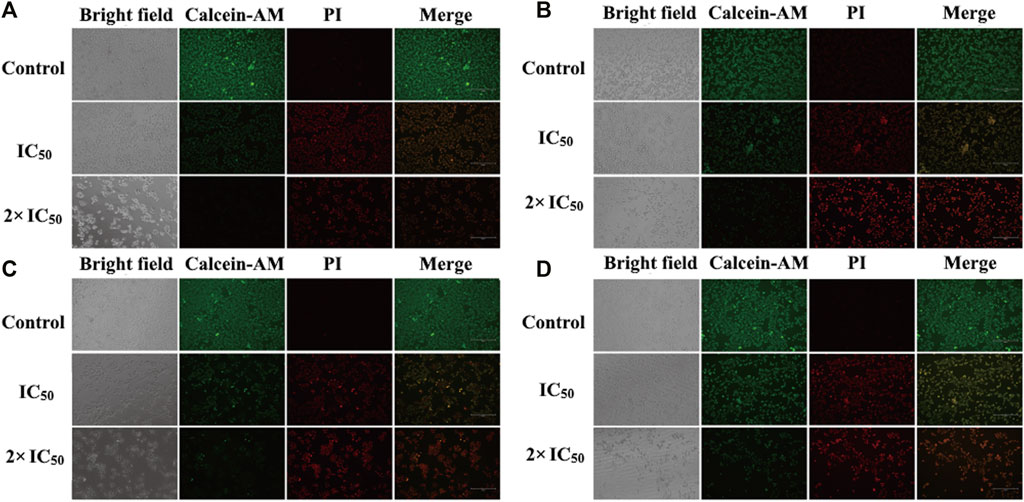
FIGURE 6. In vitro calcein AM and PI co-staining with compound 1 NPs (A) HepG2 cells and (B) Huh7 cells. Compound 2 NPs (C) HepG2 cells and (D) Huh7 cells.
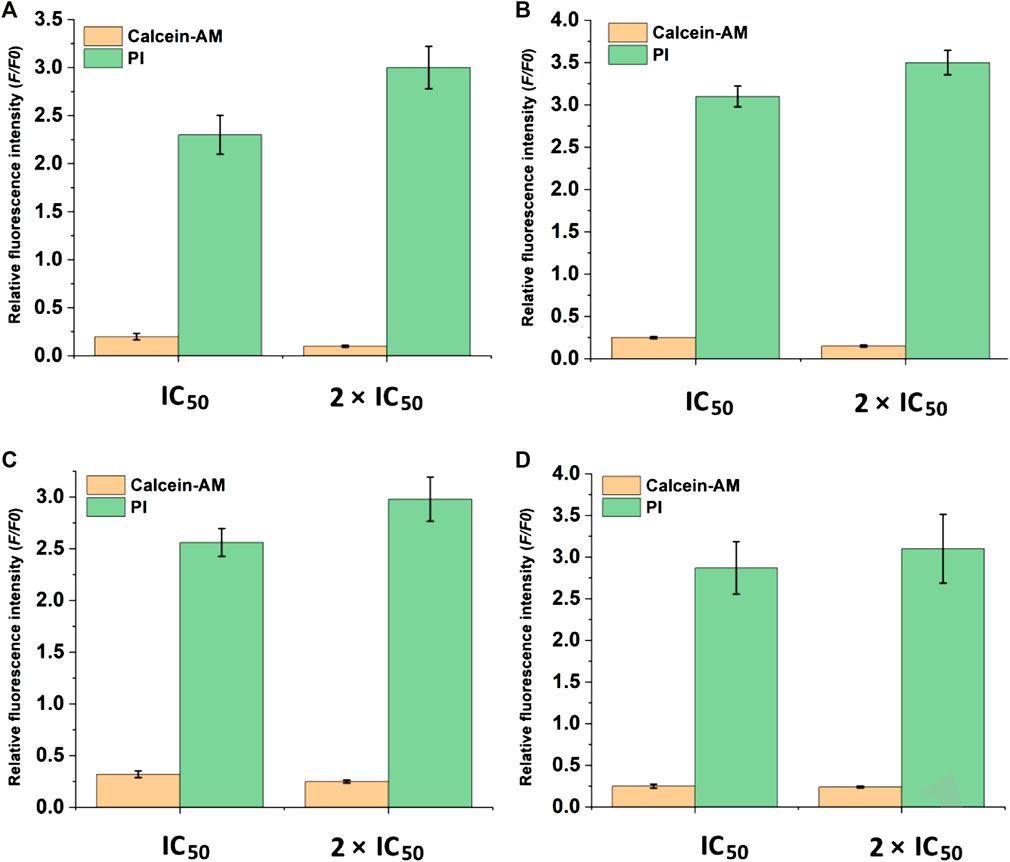
FIGURE 7. Quantification of fluorescence intensity of calcein AM and PI-stained cells. (A) HepG2 cells and (B) Huh7 cells treated with compound 1 NPs. (C) HepG2 cells and (D) Huh7 cells treated with compound 2 NPs.
Conclusion
To conclude, two new Cu(II) tetrazole carboxylates were designed and prepared for chemodynamic therapy against HCC. These compounds with new 2D structures are capable of catalyzing H2O2 to form cytotoxic hydroxyl radicals, promising their excellent cytotoxicity toward HepG2 and Huh7 cells. Nanoprecipitation with DSPE-PEG-2000 was used to prepare compounds 1 and 2 NPs with good water dispersity. Both the CCK-8 and Calcein-AM/PI co-staining assay confirmed the cytotoxicity of compounds 1 and 2 NPs, which was consistent with the flow cytometry results. Further study is still underway in our group to overcome the H2O2 consumption-induced shortage for enhanced chemodynamic therapy, such as H2O2 self-supply.
Data availability statement
The data presented in the study are deposited in the https://pan.baidu.com/s/1pgI7nZDVN1IXKZRmgYcPOA repository, accession number fic2.
Author contributions
XS, YG, CW, and DZ conceived the study and wrote the manuscript. LS, YZ, and LT synthesized the materials. XS and XJ did the cell experiments. DF re-checked these data.
Acknowledgments
The authors acknowledge financial support from the Scientific Research Pre-Technology Development project of Guilin city (No. 20180104-10), First-class discipline in Guangxi of Traditional Chinese Pharmacology (Direction of Ethnic Medicine) (2018No. 12), and the Autonomous Region Level College Students Innovation and Entrepreneurship Training Program (202010601093 and 202010601164).
Conflict of interest
The authors declare that the research was conducted in the absence of any commercial or financial relationships that could be construed as a potential conflict of interest.
Publisher’s note
All claims expressed in this article are solely those of the authors and do not necessarily represent those of their affiliated organizations, or those of the publisher, the editors, and the reviewers. Any product that may be evaluated in this article, or claim that may be made by its manufacturer, is not guaranteed or endorsed by the publisher.
Supplementary material
The Supplementary Material for this article can be found online at: https://www.frontiersin.org/articles/10.3389/fchem.2022.915247/full#supplementary-material
References
Aromi, G., Barrios, L. A., and Roubeau, O. (2011). Triazoles and tetrazoles: Prime ligands to generate remarkable coordination materials. Coord. Chem. Rev. 255, 485–546. doi:10.1016/j.ccr.2010.10.038
Dai, Y., Shen, T. X., Min, J., Zhang, L. S., Gu, Y. L., and Yang, J. (2021). Synthesis and anticancer property of two Sm(III) compounds based on tetrazole ligands. Inorganica Chim. Acta 528, 120598. doi:10.1016/j.ica.2021.120598
Deng, H. Z., Yang, W. J., Zhou, Z. J., Tian, R., Lin, L. S., Ma, Y., et al. (2020). Targeted scavenging of extracellular ROS relieves suppressive immunogenic cell death. Nat. Commun. 11, 4951. doi:10.1038/s41467-020-18745-6
Du, B. B., Zhu, Y. X., Pan, M., Yue, M. Q., Hou, Y. J., Wu, K., et al. (2015). Direct white-light and a dual-channel barcode module from Pr(iii)-MOF crystals. Chem. Commun. 51, 12533–12536. doi:10.1039/c5cc04468e
Fujita, K., Tanaka, Y. Y., Sho, T., Ozeki, S. C., Abe, S., Hikage, T., et al. (2014). Intracellular CO release from composite of ferritin and ruthenium carbonyl complexes. J. Am. Chem. Soc. 136, 16902–16908. doi:10.1021/ja508938f
H Zou, J., Li, L., Zhu, J. W., Li, X. Z., Yang, Z., Huang, W., et al. (2021). Singlet oxygen “afterglow” therapy with NIR-II fluorescent molecules. Adv. Mat. 33, 2103627. doi:10.1002/adma.202103627
Li, L., Zou, J. H., Dai, Y. L., Fan, W. P., Niu, G., Yang, Z., et al. (2020). Burst release of encapsulated annexin A5 in tumours boosts cytotoxic T-cell responses by blocking the phagocytosis of apoptotic cells. Nat. Biomed. Eng. 4, 1102–1116. doi:10.1038/s41551-020-0599-5
Li, X. S., Lee, D. Y., Huang, J. D., and Yoon, J. (2018). Phthalocyanine-assembled nanodots as photosensitizers for highly efficient Type I photoreactions in photodynamic therapy. Angew. Chem. Int. Ed. 57, 9885–9890. doi:10.1002/anie.201806551
Lin, L. S., Huang, T., Song, J. B., Ou, X. Y., Wang, Z. T., Deng, H. Z., et al. (2019). Synthesis of copper peroxide nanodots for H2O2 self-supplying chemodynamic therapy. J. Am. Chem. Soc. 141 (25), 9937–9945. doi:10.1021/jacs.9b03457
Sheldrick, G. M. (2008). A short history of SHELX. Acta Crystallogr. A A64, 112–122. doi:10.1107/s0108767307043930
Shen, J., Chen, J. J., Ke, Z., Zou, D. F., Sun, L. G., and Zou, J. H. (2019). Heavy atom-free semiconducting polymer with high singlet oxygen quantum yield for prostate cancer synergistic phototherapy. Mat. Chem. Front. 3, 1123–1127. doi:10.1039/c9qm00158a
Shen, L., Cao, M. J., Zhang, F. F., Wu, Q., Zhao, L. Y., Lu, Y. M., et al. (2016). Three new manganese(II) coordination complexes based on tetrazole carboxylate ligands. Transit. Metall. Chem. 41, 125–131. doi:10.1007/s11243-015-0003-6
Siegel, R. L., Miller, K. D., and Dvm, A. J. (2018). Cancer statistics, 2018. Ca. Cancer J. Clin. 68, 7–30. doi:10.3322/caac.21442
Song, J. B., Lin, L. S., Yang, Z., Zhu, R., Zhou, Z. J., Li, Z. W., et al. (2019). Self-assembled responsive bilayered vesicles with adjustable oxidative stress for enhanced cancer imaging and therapy. J. Am. Chem. Soc. 141, 8158–8170. doi:10.1021/jacs.8b13902
Sun, F., Yuan, J. W., Li, Y. K., Ju, C. D., Guan, W. H., Yang, G. W., et al. (2016). Substituent-dependent formation of Co(II) complexes based on phenyl-tetrazole acetic acid: From bis- to tristetrazole acetic acid. Transit. Metall. Chem. 41, 943–949. doi:10.1007/s11243-016-0099-3
Sun, W., Li, S. Y., Haupler, B., Liu, J., Jin, S. B., Steffen, W., et al. (2017). Drug therapy: An amphiphilic ruthenium polymetallodrug for combined photodynamic therapy and photochemotherapy in vivo (adv. Mater. 6/2017). Adv. Mat. 29, 1603. doi:10.1002/adma.201603702
Sun, W., Thiramanas, R., Slep, L. D., Zeng, X. L., Mail-nder, V., and Wu, S. (2017). Chem. Eur. J. 23, 10832–10837. doi:10.1002/chem.201701224
Tang, W., Yang, Z., Wang, S., Wang, Z., Song, J. B., Yu, G. C., et al. (2018). Organic semiconducting photoacoustic nanodroplets for laser-activatable ultrasound imaging and combinational cancer therapy. ACS Nano 12, 2610–2622. doi:10.1021/acsnano.7b08628
Weiss, A., Berndsen, R., Dubois, M., Müller, C., Schibli, R., Griffioen, A., et al. (2014). In vivo anti-tumor activity of the organometallic ruthenium(ii)-arene complex [Ru(η6-p-cymene)Cl2(pta)] (RAPTA-C) in human ovarian and colorectal carcinomas. Chem. Sci. 5, 4742–4748. doi:10.1039/c4sc01255k
Wriedt, M., Sculley, J. P., Yakovenko, A. A., Ma, Y. G., Halder, G. J., Balbuena, P. B., et al. (2012). Low-Energy selective capture of carbon dioxide by a pre-designed elastic single-molecule trap. Angew. Chem. Int. Ed. 51, 9804–9808. doi:10.1002/anie.201202992
Yang, J., Gu, X. Q., Su, W. T., Hao, X. Y., Shi, Y. J., Zhao, L. Y., et al. (2018). (2-(4-Bromophenyl)ethene-1, 1, 2-triyl)tribenzene with aggregation induced emission for ablation of HeLa cells. Mat. Chem. Front. 2, 1842–1846. doi:10.1039/c8qm00304a
Yang, J., He, X., Ke, Z., Chen, J. J., Zou, Z. Y., Wei, B., et al. (2020). Two photoactive Ru (II) compounds based on tetrazole ligands for photodynamic therapy. J. Inorg. Biochem. 210, 111127. doi:10.1016/j.jinorgbio.2020.111127
Yang, J., Min, Y. T., Chen, Y., Wang, J., Tian, H., Shen, L., et al. (2014). Syntheses, structures and properties of five coordinating metallic complexes with the new carboxylate-bis(tetrazolyl)amine ligand. Inorganica Chim. Acta 419, 73–81. doi:10.1016/j.ica.2014.04.013
Yang, J., Xu, Y., Jiang, M., Zou, D. F., Yang, G. W., Shen, L., et al. (2019). Photochemical property of two Ru(II) compounds based on 5-(2-pyrazinyl)tetrazole for cancer phototherapy by changing auxiliary ligand. J. Inorg. Biochem. 193, 124–129. doi:10.1016/j.jinorgbio.2019.01.015
Yang, Z., Fan, W. P., Zou, J. H., Tang, W., Li, L., He, L. C., et al. (2019). Precision cancer theranostic platform by in situ polymerization in perylene diimide-hybridized hollow mesoporous organosilica nanoparticles. J. Am. Chem. Soc. 141, 14687–14698. doi:10.1021/jacs.9b06086
Yang, Z., Song, J. B., Tang, W., Fan, W. P., Dai, Y. L., Shen, Z. Y., et al. (2019). Stimuli-responsive nanotheranostics for real-time monitoring drug release by photoacoustic imaging. Theranostics 9, 526–536. doi:10.7150/thno.30779
Zhai, C., Zhao, L., Hao, X. Y., Shi, Y. J., Xu, D., Yang, Z. Y., et al. (2017). One Cu(II) compound derived from 5-(2-pyridyl)tetrazole-2-isopropanoic acid against Hela cells. Inorg. Chem. Commun. 84, 150–152. doi:10.1016/j.inoche.2017.08.018
Zhang, A. L., Li, X. C., Min, J., Tan, L. T., Xu, H. L., Zhu, X. G., et al. (2021). Synthesis and anticancer property of three new Cu(II) coordination polymers constructed by the bifunctional substituted-polynitrogen heterocyclic ligands. Inorganica Chim. Acta 522, 120380. doi:10.1016/j.ica.2021.120380
Zhang, S., Yang, Q., Liu, X. Y., Qu, X. N., Wei, Q., Xie, G., et al. (2016). High-energy metal–organic frameworks (HE-MOFs): Synthesis, structure and energetic performance. Coord. Chem. Rev. 307, 292–312. doi:10.1016/j.ccr.2015.08.006
Zhu, H. T. C., Li, Q., Shi, B. B., Ge, F. J., Liu, Y. Z., Mao, Z. W., et al. (2020). Dual-emissive platinum(II) metallacage with a sensitive oxygen response for imaging of hypoxia and imaging-guided chemotherapy. Angew. Chem. Int. Ed. 59, 20208–20214. doi:10.1002/anie.202009442
Zou, J. H., Chen, D. Y., Yang, G. W., Li, Q. Y., Yang, J., and Shen, L. (2015). Synthesis, crystal structure and catalytic property of a new samarium compound derived from 5-(pyrazin-2-yl)tetrazole-2-acetic acid. RSC Adv. 5, 27887–27890. doi:10.1039/c5ra03296b
Zou, J. H., Cui, H. J., Xu, B., Zhu, D. L., Li, Q. Y., Yang, G. W., et al. (2014). Three new alkaline Earth coordination compounds based on 5-(2-pyrimidyl)tetrazole-2-acetic acid. Inorganica Chim. Acta 423, 430–434. doi:10.1016/j.ica.2014.08.058
Zou, J. H., Li, L., Yang, Z., and Chen, X. Y. (2021). Phototherapy meets immunotherapy: A win–win strategy to fight against cancer. Nanophotonics 10, 3229–3245. doi:10.1515/nanoph-2021-0209
Zou, J. H., Wu, H., Zhu, D. L., Tian, H., Zhang, P., Zhao, L. Y., et al. (2014). pH-dependent synthesis of a cadmium coordination compound from a compound based on Hpytz ligand [Hpytz = 5-(4-pyridyl)tetrazole]. J. Coord. Chem. 67, 3444–3453. doi:10.1080/00958972.2014.965163
Zou, J. H., Zhu, D. L., Li, F. F., Li, F. S., Wu, H., Li, Q. Y., et al. (2014). Three new coordination compounds with neodymium based on tetrazole containing carboxylic acid ligands. Z. Anorg. Allg. Chem. 640, 2226–2231. doi:10.1002/zaac.201400106
Zou, J. H., Zhu, D. L., Liu, Q., Li, S., Mei, G. D., Zhu, J. N., et al. (2014). Four new zinc coordination compounds derived from various tetrazole-containing carboxylic acids and 4, 4′-bipyridine. Inorganica Chim. Acta 421, 451–458. doi:10.1016/j.ica.2014.06.029
Zou, J. H., Zhu, D. L., Tian, H., Li, F. F., Zhang, F. F., Yang, G. W., et al. (2014). Construction of six new coordination complexes with 5-(3-pyridyl) tetrazole-2-acetato. Inorganica Chim. Acta 423, 87–94. doi:10.1016/j.ica.2014.07.034
Zou, J. H., Zhu, J. N., Cui, H. J., Wang, Z., Zhu, D. L., Zhang, F. F., et al. (2015). Group position-dependent structurally diverse coordination compounds based on isomeric ligands. Aust. J. Chem. 68, 889. doi:10.1071/ch14442
Keywords: Cu(II), tetrazole carboxylate, human hepatocellular carcinoma cells, chemodynamic therapy, in vitro
Citation: Shi X, Gu Y, Wan C, Jiang X, Shen L, Tan L, Zhong Y and Zou D (2022) Two copper(II) compounds derived from tetrazole carboxylates for chemodynamic therapy against hepatocellular carcinoma cells. Front. Chem. 10:915247. doi: 10.3389/fchem.2022.915247
Received: 11 April 2022; Accepted: 26 July 2022;
Published: 29 August 2022.
Edited by:
Nenad Filipović, Faculty of Agriculture, University of Belgrade, SerbiaReviewed by:
Ozlem Sen, Italian Institute of Technology (IIT), ItalyJohn Gallagher, Dublin City University, Ireland
Zanka Bojic-Trbojevic, Institute for the Application of Nuclear Energy (INEP), Serbia
Copyright © 2022 Shi, Gu, Wan, Jiang, Shen, Tan, Zhong and Zou. This is an open-access article distributed under the terms of the Creative Commons Attribution License (CC BY). The use, distribution or reproduction in other forums is permitted, provided the original author(s) and the copyright owner(s) are credited and that the original publication in this journal is cited, in accordance with accepted academic practice. No use, distribution or reproduction is permitted which does not comply with these terms.
*Correspondence: Yulan Gu, guyulan@263.net; Chuandan Wan, cd_wan@163.com; Dengfeng Zou, zdf1226@126.com