- 1Chemistry and Material Science College, Huaibei Normal University, Huaibei, China
- 2Key Laboratory of Green and Precise Synthetic Chemistry and Applications, Ministry of Education, Huaibei Normal University, Huaibei, China
A series of novel α-aminophosphonate derivatives containing hydrazone were designed and synthesized based on active fragments. Bioassay results demonstrated that title compounds possessed good activities against tobacco mosaic virus. Among them, compounds 6a, 6g, 6i, and 6j were equivalent to the commercial antiviral agents like dufulin. On structure optimization-based molecular docking, compound 6k was synthesized and displayed excellent activity with values of 65.1% curative activity, 74.3% protective activity, and 94.3% inactivation activity, which were significantly superior to the commercial antiviral agents dufulin and ningnanmycin. Therefore, this study indicated that new lead compounds could be developed by adopting a joint strategy with active fragments and molecular docking.
1 Introduction
Tobacco mosaic virus (TMV) is one of the most widely studied plant viruses that can cause deformation and stunting of the leaves, flowers, and fruits of infected plants (Ritzenthaler, 2005). Plant diseases caused by tobacco mosaic virus (TMV) are difficult to control because TMV is absolutely parasitic, and transmissibility to host cells and plants may completely suppress immune system (Bos, 1982). Although several commercial antiviral agents against TMV have been used, efficient and practical varieties are few. The widely used antiviral agent ribavirin only gave less than 50% anti-TMV effect at 500 μg/ml (Hansen and Stace Smith, 1989). The developing novel structure, remarkable effect, and environmentally friendly anti-TMV agents are needed urgently. At present, the main representative research groups are Wang Qingmin’s group and Song Baoan’s group on domestic development of anti-plant virus agents. In 2019–2021, Song Baoan’s group mainly designed and synthesized antiviral compounds based on active fragments through in vitro activity screening by microscale thermophoresis (MST) (He et al., 2019; Zan et al., 2020; Liu et al., 2021; Zhang et al., 2021). During the same period, Wang Qingming’s group mainly designed and synthesized new and efficient antiviral lead compounds based on natural products through traditional in vivo activity screening by using the half leaf dry spot method (Li et al., 2019; Hao et al., 2020a; Chen et al., 2020; Li et al., 2021). Then, the primary action mechanism of antiviral agents was studied by the vivo interaction of viral coat protein and drug molecules. These results indicated that viral coat protein was a key target protein for antiviral agents. Therefore, molecular docking could accelerate the development of antiviral agents.
α-Aminophosphonates are considered to be structural analogs of α-amino acids. Compounds bearing the α-aminophosphonate moiety play an important role in biochemical and medicinal chemistry such as antitumor activity (Liu et al., 2010; Ye et al., 2014; Fang et al., 2016; Ewies et al., 2019; Zhang et al., 2020), antivirus activity (Zhang et al., 2010; Zhang and Liu, 2016; Zhang et al., 2017; Lan et al., 2017; Poola et al., 2020; Zhou et al., 2021), antimicrobial activity (He et al., 2015), and antibacterial activity (Dake et al., 2011; Hellal et al., 2017). In recent years, Song and coworkers (Chen et al., 2009; Yang et al., 2011; Zhang et al., 2016) reported that many α-aminophosphonates with anti-TMV and anti-CMV activity were synthesized via substructural splicing. Among them, dufulin (Figure 1), a new commercially registered plant antiviral product, was developed, which belongs to the α-aminophosphonate family (Song et al., 2006). In addition, it has been highly effective in preventing infection caused by rice viruses and tobacco mosaic virus and, as a result, has obtained a national invention patent in the People’s Republic of China. It was registered by the Ministry of Agriculture of China (LS 20071280, 20071282, and 20130359) and was subsequently industrialized for large-scale field application. It has been widely used to prevent and control rice, vegetable, and tobacco viral diseases in China. This may provide some useful information for the future design of novel structural aminophosphonates.
Hydrazone derivatives are biologically interesting compounds known for their antiviral (Massarani et al., 1970; Wang et al., 2019), anticancer (Mehlika et al., 2012), insecticidal (Liu et al., 2010b), and antimicrobial (Martin et al., 2021) effects. Among them, hydrazones with promising antiviral activity have attracted our attention. In order to discover new molecules with antiviral effects, we sought to incorporate the active substructural unit hydrazone into the backbone structure of α-aminophosphonate. Based on the aforementioned facts, we designed and synthesized the title compounds by a joint strategy with active fragments and molecular docking (Figure 2). This article describes the syntheses and bioactivities of the designed compounds. The structure–activity relationships of these phosphonate–hydrazone analogs are examined in comparison with their parent aminophosphonate analogs to further the design of more effective antiviral compounds.
2 Materials and Methods
2.1 Chemicals
All reagents were purchased from commercial suppliers and used without further purification.
2.2 Instruments
1H NMR and 13C NMR spectra of the compounds were obtained using a Bruker DPX 400 MHz (Bruker, Germany) and Bruker DPX 600 MHzin CDCl3 or DMSO-d6 solution. HRMS was performed with a Thermo Scientific Q Exactive (Thermo Scientific, United States ). Infrared (IR) spectra were recorded on a Bruker VECTOR 22 spectrometer using KBr disks. The melting points of the compounds were measured using WRX-4 equipment.
2.3 General Procedures
2.3.1 Procedures for the Synthesis of Intermediates (4a–4h)
Aromatic aldehyde containing a hydroxyl group (1, 100 mmol) was added to a vial containing acetonitrile (50 ml) and potassium carbonate (100 mmol), and then ethyl bromoacetate (2, 110 mmol) was added in the reaction vessel. After the mixture was stirred and refluxed for 12 h, the solvent was removed in vacuo. The reaction mixture was poured into water (100 ml) and extracted with dichloromethane (50 ml×3). The dichloromethane solution was dried with anhydrous Na2SO4 and evaporated in a vacuum. The residue was recrystallized from acetonitrile to obtain the intermediates (3a∼3 h). A solution of intermediates 3 (80 mmol) and 2-amino-4-methylbenzothiazole (80 mmol) in toluene (50 ml) was refluxed for 3 h. Then, diethyl phosphite (120 mmol) was added to the reaction solution and refluxed for 6–12 h. The crude product was afforded through removing the solvent and recrystallized from acetonitrile to obtain the intermediates (4a∼4 h). Characterization data of the intermediate 4a are given as follows, and the data of other compounds are listed in Supplementary Material S1.
4a: Yield 78%, m. p. 128–130°C; 1H NMR (600 MHz, DMSO) δ 8.89 (dd, J = 9.6, 2.9 Hz, 1H), 7.43 (dd, J = 14.9, 7.5 Hz, 3H), 7.01 (d, J = 7.3 Hz, 1H), 6.96–6.84 (m, 3H), 5.58 (dd, J = 21.0, 9.6 Hz, 1H), 4.73 (s, 2H), 4.12 (q, J = 7.1 Hz, 2H), 4.08–3.96 (m, 2H), 3.94–3.86 (m, 1H), 3.84–3.76 (m, 1H), 2.41 (s, 3H), 1.16 (t, J = 7.1 Hz, 3H), 1.13 (t, J = 7.0 Hz, 3H), 1.03 (t, J = 7.0 Hz, 3H). 13C NMR (151 MHz, DMSO) δ 169.13 (s), 164.99 (d, J = 9.7 Hz), 157.67 (s), 150.92 (s), 130.76 (s), 129.91 (d, J = 5.7 Hz), 129.01 (s), 128.00 (s), 126.68 (s), 121.69 (s), 118.86 (s), 114.75 (s), 65.13 (s), 63.07 (d, J = 6.7 Hz), 62.86 (d, J = 6.8 Hz), 61.09 (s), 54.84 (s), 53.81 (s), 18.41 (s), 16.72 (d, J = 5.4 Hz), 16.53 (d, J = 5.4 Hz), 14.48 (s). IR (thin film, cm−1): 3233.5 (s), 2982.6 (s), 2928.9 (s), 1753.3 (s), 1587.9 (s), 1534.9 (s), 1446.3 (s), 1197.7 (s), 1053.1 (s), 1018.7 (s), 976.1 (s). HRMS (ESI) m/z for (C23H29N2O6PS [M + H]+ cacld. 493.1557, found 493.1553.
2.3.2 Procedures for the Synthesis of Intermediate 5a
The intermediate 4a (60 mmol) was added to the reaction flask with ethyl alcohol (50 ml), and then hydrazine hydrate (70 mmol) was added to the reaction mixture. After the mixture was stirred and refluxed for 12 h, ethyl alcohol was evaporated under reduced pressure to afford the crude product. The residue was purified by recrystallization affording acyl-hydrazine (5a) using alcohol. Characterization data of the intermediate 5a are given as follows.
5a: Yield 71%, m. p. 126–128°C; 1H NMR (600 MHz, DMSO-d6) δ: 9.29 (s, 1H), 8.92–8.85 (m, 1H), 7.49–7.38 (m, 3H), 7.01 (d, J = 7.4 Hz, 1H), 6.96–6.87 (m, 3H), 5.57 (dd, J = 21.0, 9.5 Hz, 1H), 4.44 (s, 2H), 4.32 (s, 2H), 4.08–3.95 (m, 2H), 3.93–3.87 (m, 1H), 3.83–3.77 (m, 1H), 2.40 (s, 3H), 1.13 (t, J = 7.0 Hz, 3H), 1.04 (t, J = 7.0 Hz, 3H); 13C NMR (151 MHz, DMSO-d6) δ: 167.03 (s), 165.00 (d, J = 9.8 Hz), 157.87 (s), 150.92 (s), 130.76 (s), 129.87 (d, J = 5.6 Hz), 128.94 (s), 127.99 (s), 126.68 (s), 121.69 (s), 118.87 (s), 114.88 (s), 66.74 (s), 63.07 (d, J = 6.7 Hz), 62.87 (d, J = 6.7 Hz), 56.50 (s), 54.89 (s), 53.86 (s), 19.03 (s), 18.41 (s), 16.73 (d, J = 5.2 Hz), 16.55 (d, J = 5.4 Hz). IR (thin film, cm−1): 3231.8 (s), 3037.2 (s), 2984.6 (s), 1671.9 (s), 1590.3 (s), 1536.9 (s), 1510.7 (s), 1446.6 (s), 1239.3 (s), 1050.8 (s), 1024.6 (s), 973.9 (s). HRMS (ESI) m/z for (C21H27N4O5PS [M + H]+ cacld. 479.1513, found. 479.1509.
2.3.3 Procedures for the Synthesis of Title Compounds (6a∼6 m)
The intermediate 5a (2.0 mmol) and aldehyde (2.0 mmol) were added to 5 ml of alcohol. The mixture was stirred at 80°C for 6 h. The resulting mixture was concentrated under reduced pressure to give the crude product. A total of 5 ml of water was added to the crude product and stirred for 0.5 h. The crude product was purified by column chromatography using hexane/EtOAc (1:2, v/v) or recrystallization with alcohol. The data for the title compound 6a are shown as follows, and the data of other compounds are listed in Supplementary Material S1.
6a: Yield 86%, m. p. 184–186°C; 1H NMR (400 MHz, DMSO-d6) δ: 11.56 (trans), 11.51 (cis) (s, 1H, CONH), 8.90 (d, J = 9.6 Hz, 1H, NH-Hetero), 8.30 (cis), 7.97(trans) (s, 1H, CH = N), 7.74–7.60 (m, 2H, Ar-H), 7.47–7.36 (m, 6H, Ar-H), 7.07–6.84 (m, 4H, Ar-H), 5.58 (dd, J = 21.0, 9.6 Hz, 1H, CHP), 5.10 (trans), 4.63(cis) (s, 2H, COCH2O), 4.10–3.96 (m, 2H, CH2OP), 3.95–3.87 (m, 1H, CHOP), 3.86–3.75 (m, 1H, CHOP), 2.41 (s, 3H, CH3-Hetero), 1.13 (t, J = 7.0 Hz, 3H, CH3), 1.04 (t, J = 7.0 Hz, 3H, CH3). trans:cis=(0.61:0.39); 13C NMR (151 MHz, DMSO-d6) δ: 169.03 (s), 164.61 (d, J = 9.6 Hz), 164.25 (s), 157.89 (s), 157.43 (s), 150.55 (s), 148.04 (s), 143.86 (s), 134.18 (s), 134.04 (s), 130.37 (s), 130.24 (s), 130.01 (s), 129.56 (d, J = 5.3 Hz), 129.44 (d, J = 5.3 Hz), 128.88 (d, J = 4.6 Hz), 128.72 (s), 128.12 (s), 127.60 (s), 127.21 (s), 127.00 (s), 126.28 (s), 121.28 (s), 118.47 (s), 114.54 (s), 114.35 (s), 66.63 (s), 64.84 (s), 62.66 (d, J = 6.9 Hz), 62.46 (d, J = 6.5 Hz), 54.48 (s), 53.45 (s), 18.04 (s), 16.34 (d, J = 5.2 Hz), 16.16 (d, J = 5.3 Hz). IR (thin film, cm−1): 3273.9 (s), 3106.5 (s), 2979.7 (s), 2914.9 (s), 1695.5 (s), 1612.8 (s), 1586.6 (s), 1534.4 (s), 1511.5 (s), 1430.5 (s), 1229.8 (s), 1047.5 (s), 1023.7 (s); HRMS (ESI) m/z for (C28H31N4O5PS [M + H]+ cacld. 567.1826, found. 567.1824.
2.3.4 Evaluation of Phytotoxic Activities Against Tobacco and Anti-TMV Activity
Phytotoxic activities against tobacco and anti-TMV activity were assessed according to the aforementioned method (Ji et al., 2019; Wang et al., 2012). Tobacco mosaic virus (TMV) was purified. The biological activity of the compounds against TMV was evaluated by using a half-leaf method.
2.4 Molecular Docking
Molecular docking with AutoDock 4.0 (Huey et al., 2007; Trott and Olson, 2010) between compound 6a and TMV-CP was performed. The X-ray crystal structure of TMV-CP used for the computation was downloaded from RCSB (Bhyravbhatla et al., 1998). Most of the parameters for the docking calculation were set to the default values. Each docked structure was scored by the built-in scoring function and was clustered by 1 Å of RMSD criteria. Finally, the enzyme ligand complex structures were selected according to the criteria for autodocking score.
3 Results and Discussion
3.1 Synthesis and Spectroscopy
The synthetic route of the target compounds (6a∼6 m) is shown in Figure 2. 4-hydroxybenzaldehyde 1) reacted with ethyl bromoacetate, 2) in acetonitrile to give aldehyde with an ester group, and 3) compound 3 condensed with 2-amino-4-methylbenzothiazole via a Schiff base condensation to give the intermediate of imine, which was followed by phosphine hydrogenation with diethyl phosphite to afford the compound 4. Compound 4 continued to react with hydrazine hydrate in alcohol to give the intermediate compound 5. Title compound 6 was smoothly prepared by the reaction of an aromatic aldehyde with the corresponding hydrazides. The chemical structures of these compounds were identified by NMR, IR, and HRMS (Supplementary Material). In 1H NMR of the title compounds, the CH-P proton appeared at δ 5.58–5.63 as dd, the NH-Ar proton appeared at δ 8.89–8.93 as double, and the CH = N proton of cis-isomer appeared at the higher magnetic field (8.17–8.70) than trans-isomer (7.85–8.34), the ratio of which was 3:2. In the 13C NMR spectra of compound 6, the typical carbon resonance at δ169 was indicative of a carbonyl group (C═O). The IR spectra of compound C showed bands at 1684–1701 cm−1 for C═O stretching.
3.2 Phytotoxic Activities and Antivirus Activities
First, the data on phytotoxic activity against tobacco indicated that compounds 6a–6k at 500 μg·mL− showed no toxicity. Then, the tested concentration of the compounds at 500 μg/ml was chosen, and the biological activities against TMV were evaluated. The results of anti-TMV activity are shown in Table1. The intermediates (4a∼4h) exhibited lower antiviral activities against TMV in vivo. Among them, the intermediate 4a displayed moderate activities, with values of 33.2% curative activity, 45.7% protective activity, and 78.7% inactivation activity. The intermediate 5a exhibited higher activities than compound 4a, especially in curative activity. The title compounds 6a, 6g, 6i, and 6j derived from α-aminophosphonate possessed good activities, which were similar to those controls of ningnanmycin and dufulin. Compound 6k exhibited excellent activity, with the values of 65.1% curative activity, 74.3% protective activity, and 94.3% inactivation activity, which were significantly greater than those of controls. Compounds 6b, 6f, and 6h possessed slightly lower activity than these controls. Other compounds showed lower activities. The antiviral activity results of the intermediates 4a∼4i and intermediate 5a suggest that the structure of α-aminophosphonate with benzothiazole is critical for the activity, and generally, in addition, the R2 substituted phenyl series of the title compounds influenced the antiviral activity for the derivatives (6a∼6 m). The R2 substitutions at the ortho-position of the phenyl ring connected to the hydrazone moiety (6b, 6c, and 6d) showed weaker curative activity than the R2 = H of the phenyl ring. As for the para-substituted derivatives, it is clear to see that the order of the curative activity against TMV was NO2-substituted (6g) > CH3-substituted (6f) > Br-substituted (6e). When the R2 group was at the meta-position of the phenyl ring, the compounds (6h∼6j) exhibited relatively similar curative activities to compound 6a (R2 = H). Fortunately, 2-OH-5-CH3-substituted compound 6k showed the best curative activity (65.1%) against TMV, which was significantly better than that of ningnanmycin (53.3%). Considering the prior discussion, we found that the antiviral activities of our designed compounds could be increased by the introduction of 2-OH and 5-CH3 on benzene rings. The results further suggested that small differences of substituted position on the phenyl ring could lead to large differences in the overall activities, which implies further possibilities for lead compound development.
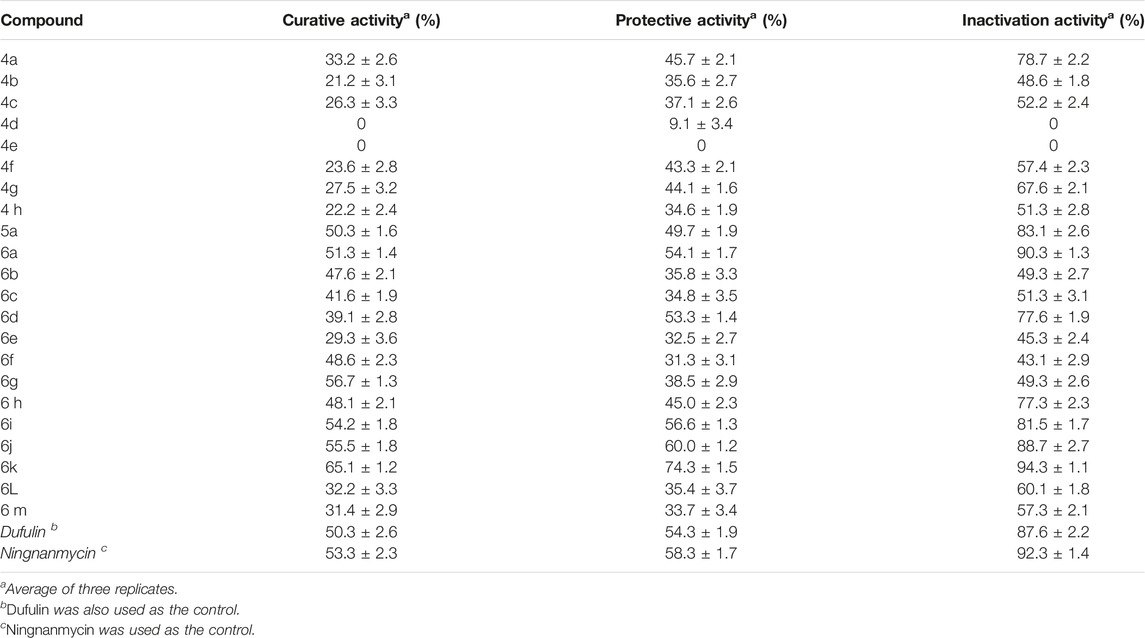
TABLE 1. Antiviral activity of the compounds (4a∼6m) against TMV at 500 μg/mLa.
3.3 Molecular Modeling Analysis
TMV-CP is an important protein involved in plant virus infections and is being studied as a potential protein target to develop effective antivirus agents (Hao et al., 2020b; Kang et al., 2020). In order to gain more understanding of the structure–activity relationships and further structure optimization, molecular docking was performed on the binding mode of compound 6a into the binding pocket of TMV-CP using AutoDock 4.0 software. The 3D binding models of compound 6a with TMV-CP are shown in Figure 3. Results showed that the benzothiazole ring of 6a fit into the binding pocket, surrounded by the amino acid residues of SER138. Detailed analysis of the binding mode showed that the hydrazone of 6a was surrounded by the amino acid residues of ARG134 and ASP224. These docking results suggested that the benzothiazole ring in the title compound was critical for the activity and the phenyl ring of hydrazone influenced the antiviral activity. On structure optimization-based molecular docking, compound 6k was synthesized and displayed excellent activity, with values of 65.1% curative activity, 74.3% protective activity, and 94.3% inactivation activity, which were significantly superior to the commercial antiviral agents dufulin and ningnanmycin. Therefore, molecular docking could accelerate the development of lead compounds.
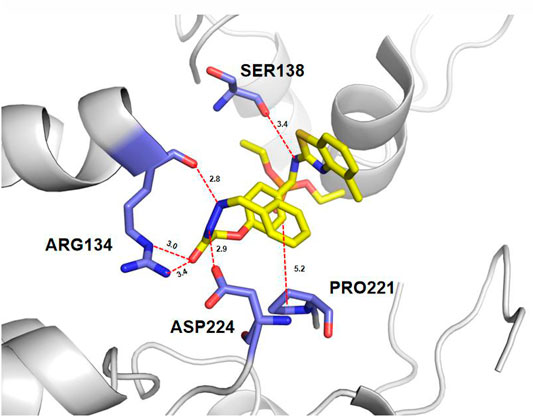
FIGURE 3. 3D mode of the interaction of compound 6a and receptor TMV-CP analyzed by AutoDock 4.0 software. Conventional hydrogen bond, carbon–hydrogen bond, and alkyl, as well as Pi–alkyl, are shown by green, light green, and pink, respectively.
4 Conclusion
A series of α-aminophosphonate-hydrazone derivatives were synthesized and evaluated for antiviral activities. Some derivatives showed good inhibitory activity. The SARS analysis showed that the volume and position of the substituted groups at the phenyl ring of hydrazones had significant influences on inhibitory activity. The docking studies showed that compound 6a was well bound to the TMV-CP via one hydrogen bond with SER 138, ARG 134, and ASP 224. This also indicated that the structures of benzothiazole and hydrazone play a key role in the activity of the title compound. Among them, compound 6k displayed excellent activity, with values of 65.1% curative activity, 74.3% protective activity, and 94.3% inactivation activity. Therefore, the basic motif of compound 6k can be used as a lead compound for further development.
Data Availability Statement
The original contributions presented in the study are included in the article/Supplementary Material, further inquiries can be directed to the corresponding author.
Author Contributions
All authors have contributed to the work, have read the manuscript, and have agreed to be listed as authors. The submitted manuscript has not been published elsewhere nor is it currently under review by another publication. GZ designed the project and wrote the manuscript; JT and RJ performed the experiments; and HW and SL analyzed the data.
Funding
This work was funded by the National Natural Science Foundation of China (No. 21807037) and the Provincial Graduate Innovation Program in Anhui (No. YX2021020).
Conflict of Interest
The authors declare that the research was conducted in the absence of any commercial or financial relationships that could be construed as a potential conflict of interest.
Publisher’s Note
All claims expressed in this article are solely those of the authors and do not necessarily represent those of their affiliated organizations, or those of the publisher, the editors, and the reviewers. Any product that may be evaluated in this article, or claim that may be made by its manufacturer, is not guaranteed or endorsed by the publisher.
Supplementary Material
The Supplementary Material for this article can be found online at: https://www.frontiersin.org/articles/10.3389/fchem.2022.911453/full#supplementary-material
References
Altıntop, M. D., Özdemir, A., Turan-Zitouni, G., Ilgın, S., Atlı, Ö., İşcan, G., et al. (2012). Synthesis and Biological Evaluation of Some Hydrazone Derivatives as New Anticandidal and Anticancer Agents. Eur. J. Med. Chem. 58, 299–307. doi:10.1016/j.ejmech.2012.10.011
Bhyravbhatla, B., Watowich, S. J., and Caspar, D. L. D. (1998). Refined Atomic Model of the Four-Layer Aggregate of the Tobacco Mosaic Virus Coat Protein at 2.4-Å Resolution. Biophysical J. 74, 604–615. doi:10.1016/s0006-3495(98)77819-1
Bos, L. (1982). Crop Losses Caused by Viruses. Crop Prot. 1, 263–282. doi:10.1016/0261-2194(82)90002-3
Chen, L., Hao, Y., Song, H., Liu, Y., Li, Y., Zhang, J., et al. (2020). Design, Synthesis, Characterization, and Biological Activities of Novel Spirooxindole Analogues Containing Hydantoin, Thiohydantoin, Urea, and Thiourea Moieties. J. Agric. Food Chem. 68, 10618–10625. doi:10.1021/acs.jafc.0c04488
Chen, M.-H., Chen, Z., Song, B.-A., Bhadury, P. S., Yang, S., Cai, X.-J., et al. (2009). Synthesis and Antiviral Activities of Chiral Thiourea Derivatives Containing an α-Aminophosphonate Moiety. J. Agric. Food Chem. 57, 1383–1388. doi:10.1021/jf803215t
Dake, S. A., Raut, D. S., Kharat, K. R., Mhaske, R. S., Deshmukh, S. U., and Pawar, R. P. (2011). Ionic Liquid Promoted Synthesis, Antibacterial and In Vitro Antiproliferative Activity of Novel α-aminophosphonate Derivatives. Bioorg. Med. Chem. Lett. 21 (8), 2527–2532. doi:10.1016/j.bmcl.2011.02.039
Ewies, E. F., El-Hussieny, M., El-Sayed, N. F., and Fouad, M. A. (2019). Design, Synthesis and Biological Evaluation of Novel α-aminophosphonate Oxadiazoles via Optimized Iron Triflate Catalyzed Reaction as Apoptotic Inducers. Eur. J. Med. Chem. 180 (15), 310–320. doi:10.1016/j.ejmech.2019.07.029
Fang, Y.-L., Wu, Z.-L., Xiao, M.-W., Tang, Y.-T., Li, K.-M., Ye, J., et al. (2016). One-Pot Three-Component Synthesis of Novel Diethyl((2-Oxo-1,2-Dihydroquinolin-3-Yl)(arylamino)methyl)phosphonate as Potential Anticancer Agents. Ijms 17 (5), 653–667. doi:10.3390/ijms17050653
Hansen, A. J., and Stace Smith, R. (1989). Antiviral Chemicals for Plant Disease Control. Crit. Rev. Plant Sci. 8, 45–88. doi:10.1080/07352688909382270
Hao, Y., Guo, J., Wang, Z., Liu, Y., Li, Y., Ma, D., et al. (2020b). Discovery of Tryptanthrins as Novel Antiviral and Anti-phytopathogenic-fungus Agents. J. Agric. Food Chem. 68, 5586–5595. doi:10.1021/acs.jafc.0c02101
Hao, Y., Wang, K., Wang, Z., Liu, Y., Ma, D., and Wang, Q. (2020a). Luotonin A and its Derivatives as Novel Antiviral and Antiphytopathogenic Fungus Agents. J. Agric. Food Chem. 68, 8764–8773. doi:10.1021/acs.jafc.0c04278
He, F., Shi, J., Wang, Y., Wang, S., Chen, J., Gan, X., et al. (2019). Synthesis, Antiviral Activity, and Mechanisms of Purine Nucleoside Derivatives Containing a Sulfonamide Moiety. J. Agric. Food Chem. 67 (31), 8459–8467. doi:10.1021/acs.jafc.9b02681
He, S., Ouyang, X., Huang, X., Hu, W., Dai, W., Tian, X., et al. (2015). Synthesis of Derivatives of Artesunate α-Aminophosphonate and Their Antimicrobial Activities. Lddd 12, 408–416. doi:10.2174/1570180812666141125004502
Hellal, A., Chafaa, S., Chafai, N., and Touafri, L. (2017). Synthesis, Antibacterial Screening and DFT Studies of Series of α-amino-phosphonates Derivatives from Aminophenols. J. Mol. Struct. 1134, 217–225. doi:10.1016/j.molstruc.2016.12.079
Huey, R., Morris, G. M., Olson, A. J., and Goodsell, D. S. (2007). A Semiempirical Free Energy Force Field with Charge-Based Desolvation. J. Comput. Chem. 28, 1145–1152. doi:10.1002/jcc.20634
Ji, R. J., Shi, W. M., Tian, D. Y., Zhang, G. P., and Wang, H. (2019). Facile Synthesis of Novel Dithioacetal-Naphthalene Derivatives as Potential Activators for Plant Resistance Induction. RSC Adv. 9, 32375–32381. doi:10.1039/c9ra06843k
Kang, J., Gao, Y., Zhang, M., Ding, X., Wang, Z., Ma, D., et al. (2020). Streptindole and its Derivatives as Novel Antiviral and Anti-phytopathogenic Fungus Agents. J. Agric. Food Chem. 68, 7839–7849. doi:10.1021/acs.jafc.0c03994
Krátký, M., Štěpánková, Š., Konečná, K., Svrčková, K., Maixnerová, J., Švarcová, M., et al. (2021). Novel Aminoguanidine Hydrazone Analogues: from Potential Antimicrobial Agents to Potent Cholinesterase Inhibitors. Pharmaceuticals 14, 1229–1259. doi:10.3390/ph14121229
Lan, X., Xie, D., Yin, L., Wang, Z., Chen, J., Zhang, A., et al. (2017). Novel α,β-unsaturated Amide Derivatives Bearing α-amino Phosphonate Moiety as Potential Antiviral Agents. Bioorg. Med. Chem. Lett. 27 (18), 4270–4273. doi:10.1016/j.bmcl.2017.08.048
Li, L., Zou, J., Xu, C., You, S., Deng, Z., Chen, G., et al. (2021). Preparation and Anti-tobacco Mosaic Virus Activities of Crocetin Diesters. J. Agric. Food Chem. 69 (45), 13637–13643. doi:10.1021/acs.jafc.1c03884
Li, L., Zou, J., You, S., Deng, Z., Liu, Y., and Wang, Q. (2019). Natural Product Cerbinal and its Analogues Cyclopenta[c]pyridines: Synthesis and Discovery as Novel Pest Control Agents. J. Agric. Food Chem. 67, 10498–10504. doi:10.1021/acs.jafc.9b03699
Liu, J.-Z., Song, B.-A., Fan, H.-T., Bhadury, P. S., Wan, W.-T., Yang, S., et al. (2010a). Synthesis and In Vitro Study of Pseudo-peptide Thioureas Containing α-aminophosphonate Moiety as Potential Antitumor Agents. Eur. J. Med. Chem. 45 (11), 5108–5112. doi:10.1016/j.ejmech.2010.08.021
Liu, M., Wang, Y., Wangyang, W.-z., Liu, F., Cui, Y.-l., Duan, Y.-s., et al. (2010b). Design, Synthesis, and Insecticidal Activities of Phthalamides Containing a Hydrazone Substructure. J. Agric. Food Chem. 58, 6858–6863. doi:10.1021/jf1000919
Liu, Y., Chen, J., Xie, D., Song, B., and Hu, D. (2021). First Report on Anti-TSWV Activities of Quinazolinone Derivatives Containing a Dithioacetal Moiety. J. Agric. Food Chem. 69, 12135–12142. doi:10.1021/acs.jafc.1c03171
Massarani, E., Nardi, D., Pozzi, R., and Degen, L. (1970). Arylglyoxal N,N-disubstituted Hydrazones with Antiviral and Antifungal Activity. J. Med. Chem. 13 (1), 157–159. doi:10.1021/jm00295a052
Poola, S., Nagaripati, S., Tellamekala, S., Chintha, V., Kotha, P., Yagani, J. R., et al. (2020). Green Synthesis, Antibacterial, Antiviral and Molecular Docking Studies of α-aminophosphonates. Synth. Commun. 50, 2655–2672. doi:10.1080/00397911.2020.1753079
Ritzenthaler, C. (2005). Resistance to Plant Viruses: Old Issue, News Answers? Curr. Opin. Biotechnol. 16, 118–122. doi:10.1016/j.copbio.2005.02.009
Song, B. A., Zhang, G. P., Hu, D. Y., Pang, L., Yang, S., Liu, G., et al. (2006). N-Substituted Benzothiazoline-1-Substituted Phenyl -O,O- Dialkyl -α- Amino Phosphonate Derivatives and Their Preparation Method and Application. CN 1291993C.
Trott, O., and Olson, A. J. (2009). AutoDock Vina: Improving the Speed and Accuracy of Docking with a New Scoring Function, Efficient Optimization, and Multithreading. J. Comput. Chem. 31, NA. doi:10.1002/jcc.21334
Wang, Y., Xu, F., Luo, D., Guo, S., He, F., Dai, A., et al. (2019). Synthesis of Anthranilic Diamide Derivatives Containing Moieties of Trifluoromethylpyridine and Hydrazone as Potential Anti-viral Agents for Plants. J. Agric. Food Chem. 67, 13344–13352. doi:10.1021/acs.jafc.9b05441
Wang, Z., Wei, P., Xizhi, X., Liu, Y., Wang, L., and Wang, Q. (2012). Design, Synthesis, and Antiviral Activity Evaluation of Phenanthrene-Based Antofine Derivatives. J. Agric. Food Chem. 60, 8544–8551. doi:10.1021/jf302746m
Yang, X., Song, B., Jin, L., Wei, X., Bhadury, S. P., Li, X., et al. (2011). Synthesis and Antiviral Bioactivities of Novel Chiral Bis-thiourea-type Derivatives Containing α-aminophosphonate Moiety. Sci. China Chem. 54 (1), 103–109. doi:10.1007/s11426-010-4179-5
Ye, M.-Y., Yao, G.-Y., Pan, Y.-M., Liao, Z.-X., Zhang, Y., and Wang, H.-S. (2014). Synthesis and Antitumor Activities of Novel α-aminophosphonate Derivatives Containing an Alizarin Moiety. Eur. J. Med. Chem. 83 (18), 116–128. doi:10.1016/j.ejmech.2014.02.067
Zan, N., Xie, D., Li, M., Jiang, D., and Song, B. (2020). Design, Synthesis, and Anti-ToCV Activity of Novel Pyrimidine Derivatives Bearing a Dithioacetal Moiety that Targets ToCV Coat Protein. J. Agric. Food Chem. 68, 6280–6285. doi:10.1021/acs.jafc.0c00987
Zhang, B., Hu, X.-T., Gu, J., Yang, Y.-S., Duan, Y.-T., and Zhu, H.-L. (2020). Discovery of Novel Sulfonamide-Containing Aminophosphonate Derivatives as Selective COX-2 Inhibitors and Anti-tumor Candidates. Bioorg. Chem. 105, 104390–110400. doi:10.1016/j.bioorg.2020.104390
Zhang, G.-P., and Liu, M. (2016). Novel Biphenyl Derivatives with ?-Aminophosphonate Moiety: Design, Synthesis and Antivirus Activity. Lddd 13, 706–714. doi:10.2174/1570180813666160125222813
Zhang, G.-P., Pan, J.-K., Zhang, J., Wu, Z.-X., Liu, D.-Y., and Zhao, L. (2017). Design, Synthesis, Antiviral Activities of Novel Phosphonate Derivatives Containing Quinazoline Based on Chalone Motif. J. Heterocycl. Chem. 54, 2548–2555. doi:10.1002/jhet.2849
Zhang, G., Hao, G., Pan, J., Zhang, J., Hu, D., and Song, B. (2016). Asymmetric Synthesis and Bioselective Activities of α-Amino-phosphonates Based on the Dufulin Motif. J. Agric. Food Chem. 64, 4207–4213. doi:10.1021/acs.jafc.6b01256
Zhang, J., He, F., Chen, J., Wang, Y., Yang, Y., Hu, D., et al. (2021). Purine Nucleoside Derivatives Containing a Sulfa Ethylamine Moiety: Design, Synthesis, Antiviral Activity, and Mechanism. J. Agric. Food Chem. 69, 5575–5582. doi:10.1021/acs.jafc.0c06612
Zhang, X., Qu, Y., Fan, X., Bores, C., Feng, D., Andrei, G., et al. (2010). Solvent-free Synthesis of Pyrimidine Nucleoside-Aminophosphonate Hybrids and Their Biological Activity Evaluation. Nucleosides, Nucleotides Nucleic Acids 29, 616–627. doi:10.1080/15257770.2010.496281
Zhou, X., Ye, Y., Liu, S., Shao, W., Liu, L., Yang, S., et al. (2021). Design, Synthesis and Anti-TMV Activity of Novel α-aminophosphonate Derivatives Containing a Chalcone Moiety that Induce Resistance against Plant Disease and Target the TMV Coat Protein. Pesticide Biochem. Physiology 172, 104749. doi:10.1016/j.pestbp.2020.104749
Keywords: α-aminophosphonate, hydrazone, synthesis, antiviral, docking
Citation: Tian J, Ji R, Wang H, Li S and Zhang G (2022) Discovery of Novel α-Aminophosphonates with Hydrazone as Potential Antiviral Agents Combined With Active Fragment and Molecular Docking. Front. Chem. 10:911453. doi: 10.3389/fchem.2022.911453
Received: 02 April 2022; Accepted: 13 April 2022;
Published: 20 May 2022.
Edited by:
Pei Li, Kaili University, ChinaCopyright © 2022 Tian, Ji, Wang, Li and Zhang. This is an open-access article distributed under the terms of the Creative Commons Attribution License (CC BY). The use, distribution or reproduction in other forums is permitted, provided the original author(s) and the copyright owner(s) are credited and that the original publication in this journal is cited, in accordance with accepted academic practice. No use, distribution or reproduction is permitted which does not comply with these terms.
*Correspondence: Guoping Zhang, hbzgp-1@163.com
†These authors have contributed equally to this work and share first authorship