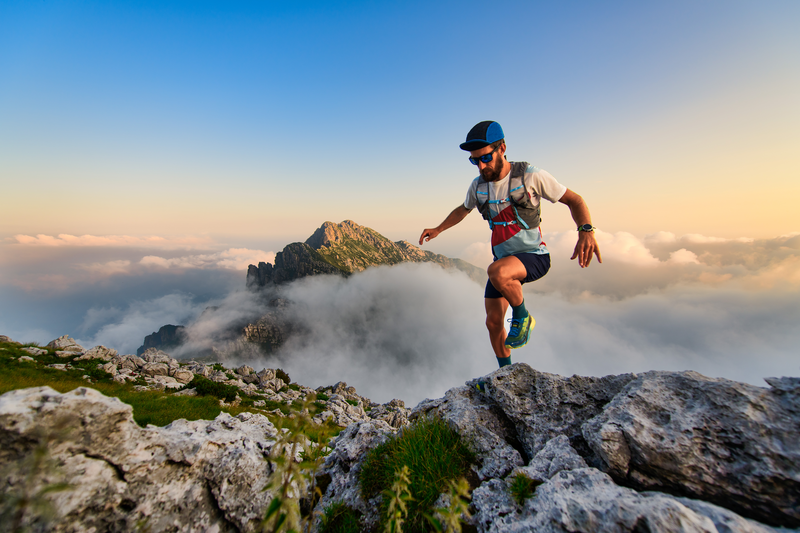
95% of researchers rate our articles as excellent or good
Learn more about the work of our research integrity team to safeguard the quality of each article we publish.
Find out more
MINI REVIEW article
Front. Chem. , 13 May 2022
Sec. Analytical Chemistry
Volume 10 - 2022 | https://doi.org/10.3389/fchem.2022.909110
This article is part of the Research Topic Optical and Electrochemical Biosensing View all 11 articles
Metal nanoparticles (NPs) with superior physicochemical properties and biocompatibility have shown great potential in theranostics. However, metal NPs show poor stability in some harsh conditions such as strong acid, oxidation, corrosion and high-temperature conditions, which limits their extensive bioapplications. To address such issue, a variety of superstable metal graphitic nanocapsules with the metal cores confined in the nanospace of few-layer graphitic shell have been developed for biodetection and therapy in harsh conditions. In this mini-review, we summarize the recent advances in metal graphitic nanocapsules for bioapplications in harsh conditions. Firstly, their theranostic performance in non-intrinsic physiological harsh environment, including oxidation, corrosion and high-temperature conditions, is systematically discussed. Then, we highlight their theranostic performance in the harsh stomach condition that is strong acidic and pepsin-rich. It is expected that this review will offer inspiration to facilitate the exploitation of novel theranostic agents that are stable in harsh conditions.
Metal nanoparticles (NPs) with different compositions and unique physicochemical properties are powerful tools for various biodetection and therapy (Azharuddin et al., 2019; Kim et al., 2019; Ma et al., 2021b). However, metal NPs show unsatisfactory stability in some non-intrinsic physiological harsh conditions (including strong oxidation, corrosion and high-temperature conditions) as well as harsh stomach condition (including strong acid (pH 0.9-1.5) and pepsin-rich conditions), and these harsh conditions hinder their broad applications in biomedicine. Hence, it is of great significance to develop effective strategies to improve the stability of metal NPs for reliable disease diagnosis and treatment in harsh conditions. The encapsulation of metal NPs is one of the most effective strategies to promote their stability (Gao et al., 2021). Currently, inert inorganic coating (such as silica (Li et al., 2010; Żygieło et al., 2021), titanium dioxide (Jin et al., 2021; Perumal et al., 2021) and graphene oxide (Xu et al., 2020; Kasztelan et al., 2021)) protection and organic coating (such as polyvinylpyrrolidone (Mirzaei et al., 2017)and lipids (Hsu et al., 2018)) functionalization strategies have been widely used to prevent the metal NPs from damage under external environments. However, these strategies make it difficult to completely isolate metal NPs from harsh conditions without affecting their inherent properties and functions.
Metal graphitic nanocapsules, a new type of nanomaterials with metal cores confined in the nanospace of few-layer graphitic shell prepared by the chemical vapor deposition method, show superior stability in harsh conditions (Li et al., 2019, 2022; Liu et al., 2020; Tang et al., 2021; Zhu et al., 2021). The graphitic shell acts as an inert layer to protect the unique physicochemical properties and intact functions of the metal core. Moreover, the graphitic shell with a large specific surface area and delocalized π electronic structure offers a robust platform for targeted molecules or drugs loading, and it also acts as a stable Raman label or internal standard molecule for reliable Raman bioanalysis. Benefiting from the ultra-high stability of graphitic layer and versatility of metal cores, a variety of theranostic applications in harsh conditions have been implemented by metal graphitic nanocapsules in the past few years. In this mini-review, we first introduce the theranostic advances of the metal graphitic nanocapsules in non-intrinsic physiological harsh environment, including oxidation, corrosion and high-temperature conditions. Then, we highlight the theranostic performance of the metal graphitic nanocapsules in the strong acid (pH 0.9-1.5) and pepsin-rich stomach conditions. Finally, the potential challenge and development direction of their theranostic applications in harsh conditions are further discussed. We expect this review will attract readers to facilitate the exploitation of novel theranostic agents that are stable in harsh conditions.
Metal NPs-based theranostic agents are commonly subjected to a variety of non-intrinsic physiological harsh conditions, including strong oxidation, corrosion and high temperature conditions that exists during the occurrence, development and theranostics of diseases. These harsh conditions affect the stability of metal NPs to a certain extent, making direct biodetection and therapy challenging. Core-shell structured metal graphitic nanocapsules that integrated the multifunctional metal core in the nanospace of inert graphitic shell demonstrate exceptional theranostic potential in harsh conditions.
Oxidation conditions are able to affect the stability and property of metal NPs, especially the plasmonic-active Ag NPs that are prone to oxidation in air (Han et al., 2011). With the goal of protecting Ag NPs from oxidation, Song et al. (2014) prepared a highly surface enhanced Raman scattering (SERS)-active AgCu graphitic nanocapsules (AgCu@G). Using the intrinsic characteristic Raman bands from the graphitic shell of AgCu@G as the stable Raman label, high-resolution multimodal cellular Raman imaging was achieved (Figure 1A). Latterly, Li et al. (2021) reported a novel AuAg graphitic nanocapsules (AuAg@G) with superior anti-oxidation property and realized SERS quantitative analysis in homogeneous system and multimodal Raman imaging of MCF-7 cells.
FIGURE 1. Metal graphitic nanocapsules-based theranostics in non-intrinsic physiological harsh conditions. (A) Oxidation resistance of AgCu@G and AgCu@G for multimodal cellular Raman imaging (Song et al., 2014). (B) Illustration of the CoPt@G propelled navigator to enhance penetration and PTT action against tumor cells (Zhang et al., 2021a). (C) Illustration of the CoPt@G@GOx with magneto-actuated cascade catalytic activity for the therapy of Streptococcus mutans biofilm (Dong et al., 2022). (D) AuCo@G with corrosion resistance for direct capture and SERS analysis of the CN− (Zhang et al., 2019). (E) AuNR@G-P-aspirin for enhanced NIR-mediated PTT of solid tumor and simultaneously inhibit PTT-induced inflammatory response (Dong et al., 2018). (F) Thermostable Ag@G-GOx synergistic PTT platform for bacterial elimination (Liu et al., 2022).
Endogenous/exogenous H+ and H2O2 are other kinds corrosive substances that influence the stability and function of metal NPs (Mabilleau et al., 2006). Zhang et al. (2021a) constructed the catalase-like CoPt graphitic nanocapsules (CoPt@G) with superior corrosion resistance to H2O2, and it catalyzed H2O2 to produce O2, offering a driving force for enhanced tumor penetration. Coupled with the magnetic and photothermal properties of CoPt@G, enhanced penetration and efficient photothermal therapy (PTT) of solid tumors have been achieved with the assistance of H2O2 and an external magnetic field (Figure 1B). Based on the superior corrosion resistance to H2O2 of CoPt@G, Dong et al. (2022) further proposed a glucose oxidase loaded CoPt@G (CoPt@G@GOx) platform with cascade reaction activity for Streptococcus mutans biofilms treatment. GOx firstly oxidized glucose to generate H2O2 and gluconic acid in the presence of endogenous glucose. The lower pH of the local microenvironment caused by gluconic acid could enhance the peroxidase-like activity of CoPt@G, and catalyze H2O2 to produce a large amount of highly toxic •OH, thereby achieving efficient inhibition of bacteria (Figure 1C). Recently, Keoingthong et al. (2021) fabricated a novel peroxidase active Ru graphitic nanocapsules (Ru@G) with superior corrosion resistance to H2O2 for sensitive colorimetric detection of glutathione (GSH) at near-physiological pH. In the presence of H2O2, Ru@G could catalyze colorimetric probe 3,3′,5,5′-tetramethylbenzydine (TMB) into blue-colored products, which was inhibited in the presence of GSH, building a simple and sensitive method for the colorimetric detection of GSH. In addition to H+ and H2O2, CN− is also a common corrosive substance that could influence the stability and function of metal NPs-based bioanalytical platform (Wang et al., 2009). Zhang et al. (2019) developed a versatile AuCo graphitic nanocapsules (AuCo@G) with superior SERS activity, magnetic properties and corrosion resistance to CN−, and direct SERS analysis of the biomarker of CN− in Pseudomonas aeruginosa was achieved (Figure 1D). The superstable AuCo@G proposed a robust platform for detecting Pseudomonas aeruginosa infection.
High temperature produced in the PTT process tends to affect the stability of photothermal reagents, meaning the development of stable photothermal reagents is of great significance (Gao et al., 2015). Metal graphitic nanocapsules present excellent thermostability and the graphitic layer has superior spectral absorption properties, thus showing extensive prospects in PTT applications. Dong et al. (2018) found that gold nanorod graphitic nanocapsules (AuNR@G) had superior near-infrared (NIR) light absorption property and had a better thermostability than AuNR. They further integrated AuNR@G with anti-inflammatory prodrugs (AuNR@G-P-aspirin) to realize enhanced NIR-mediated PTT of solid tumor and simultaneously inhibit PTT-induced inflammatory response (Figure 1E). Based on the superior thermostability and photothermal property of AuNR@G, Xu et al. (2019) reported a AuNR@G-doped hydrogel system for highly efficient photothermal antibacterial therapy for both Gram-negative Escherichia coli and Gram-positive Staphylococcus aureus. Recently, Liu et al. (2022) reported a GOx and Ag graphitic nanocapsules (Ag@G) co-loaded silk membrane theranostic system, the silk membrane enabled GOx to maintain good antibacterial activity during the photothermal antibacterial therapy process, the inert graphitic shell enabled Ag@G to keep excellent SERS performance and photothermal performance under high temperature and GOx-catalyzed production of large amounts of H2O2, SERS identification of bacteria in the bacteria-infected wound model and efficient synergistic treatment of bacteria were eventually achieved (Figure 1F).
Gastric environment is a harsh condition with extremely low pH (0.9-1.5) and abundant pepsin. Among these, H+ is a corrosive substance that affects the stability of theranostic reagents, and pepsin in gastric fluid can degrade or bind to theranostic reagents nonspecifically, resulting in instability and inefficiency of theranostics (Huang et al., 2015). Metal graphitic nanocapsules have excellent corrosion resistance to H+ and degradation resistance to pepsin, providing a robust platform for the theranostic of gastric diseases.
Helicobacter pylori (H. pylori) infection is implicated in the aetiology of many diseases (Sanders and Peura, 2002). Although a series of methods for the detection of H. pylori infection have been developed, researchers have never stopped exploring safer and more efficient in situ diagnostic methods (Abu Shady et al., 2015; Celik et al., 2021; Jain et al., 2021). Magnetic resonance imaging (MRI), a powerful technique with superior penetration depth, noninvasiveness, high spatial and temporal resolution, shows great promises for the in situ detection of H. pylori infection (Hsieh et al., 2019). However, the harsh gastric acid environment affects the application of conventional contrast agent such as Gd3+ complexes and superparamagnetic iron oxide (Stephen et al., 2011; Li and Meade, 2019). Previous works reported that the FeCo graphitic nanocapsules (FeCo@G) showed superior stability and magnetic properties in solutions with extensive pH range, including the 1 M HCl solution (Chen et al., 2012; Nie et al., 2014). Based on the unique properties of FeCo@G, Li et al. (2017) used the FeCo@G as a robust contrast agent, and further prepared the benzeneboronic acid-PEG (B-PEG, a molecule that could specifically bind to peptidoglycan in bacterial cells) modified FeCo@G system for in situ targeted MRI imaging detection of H. pylori in mice (Figure 2A). Triple therapy (a proton pump inhibitor and two antibiotics) is used as the standard first-line therapy in the clinical treatment of H. pylori infection, but its efficacy is greatly limited by the rapid degradation of antibiotics in gastric acid, the emergence of drug-resistant bacteria and the side effect to the intestinal flora (Wu et al., 2012; Brestoff and Artis, 2013). Nanozyme-based bacterial therapy has been developed rapidly in recent years, and it is expected to provide new options for the treatment of H. pylori (Huang et al., 2019; Jiang et al., 2019). Zhang et al. (2021b) developed a bacteria-targeting molecule C18-PEGn-benzeneboronic acid-functionalized CoPt graphitic nanocapsules (CoPt@G@CPB) platform for targeting and selective combating H. pylori infection in vivo. The CoPt@G showed superior corrosion resistance in acidic conditions and its oxidase-like activity was activated to catalyze the generation of superoxide radical species for antibacterial applications (Figure 2B). Meanwhile, its oxidase-like activity was suppressed under intestinal neutral conditions, showing minimal side effects. In addition, MRI and Raman imaging was used for monitoring the distribution of CoPt@G@CPB to guide further treatment.
FIGURE 2. Metal graphitic nanocapsules-based theranostics in harsh stomach conditions. (A) Stability of FeCo@G in 1 M HCl and FeCo@G-B-PEG for in situ MRI images of H. pylori (Li et al., 2017). (B) CoPt@G@CPB platform for targeted and selective combating H. pylori infection in vivo (Zhang et al., 2021b). (C) Hydrogel isolated HSA-Mn system for MRI monitoring of gastric pH (Xu et al., 2021). (D) Pepsin-assisted assembly of FeCo@G for enhanced mucosal penetration depth and prolonged drug retention (Cai et al., 2021).
Abundant pepsin in the gastric fluid can also affect the theranostics of gastric diseases because it tends to degrade or bind to the theranostic reagents nonspecifically. Many gastric diseases are highly correlated with abnormal pH, and in situ pH monitoring is therefore indispensable for prevention and treatment of gastric diseases (Ma et al., 2021a). To realize accurate and interference-free MRI detection of gastric pH, Xu et al. (2021) developed an orally administrated hydrogel capsule isolated human serum albumin−manganese (HSA-Mn) complex system, which could shield the interference of the pepsin in gastric fluid without severely hindering the penetration of H+, for sensitive MRI monitoring of gastric pH in vivo (Figure 2C). Magnetic nanomaterials show huge potential for enhanced targeted drug delivery, the FeCo@G with superior corrosion resistance to gastric fluid is therefore expected to be a robust tool for targeted drug delivery in the stomach (Yang et al., 2014; Xu et al., 2018). Cai et al. (2021) surprisingly discovered FeCo@G could not only avoid the interference, but also use the pepsin as a “bridge” to realize the self-assembly of FeCo@G under an external magnetic field, and enhanced mucosal penetration depth and prolonged drug retention time were finally achieved in vivo (Figure 2D). This magnetic field-mediated in situ self-assembly platform without the interference of extremely acidic and pepsin-rich stomach conditions provided new ideas for the delivery of oral drugs and site-selective treatment of gastric diseases.
Versatile metal graphitic nanocapsules have been widely used for theranostics in harsh conditions due to their excellent stability, good biocompatibility and unique physicochemical properties. In this mini-review, we have summarized the recent advances in metal graphitic nanocapsules for theranostics in different harsh conditions. Firstly, plasmonic metal graphitic nanocapsues that can resist corrosion and high temperature damage have been constructed for reliable SERS bioanalysis, efficient photothermal anticancer and photothermal antibacterial applications in harsh conditions. Secondly, metal graphitic nanocapsules with robust MRI contrast ability and nanozyme activity under strong acid conditions have shown superior performance in the theranostics of gastric diseases. Finally, magnetic and magnetocatalytic propelled metal graphitic nanocapsules that can be stabilized in harsh conditions have been developed as delivery platforms for enhanced gastric mucus penetration and tumor penetration.
Despite great progress has been made in metal graphitic nanocapsules for theranostics in harsh conditions, some critical issues are still needed to be resolved. Firstly, novel multifunctional metal graphitic nanocapsules should be developed to broaden their scope of disease theranostics applications in harsh conditions. Secondly, long-term toxicity of metal graphitic nanocapsules in vivo should be systematically explored for promoting expected clinical applications. Finally, the integration of metal graphitic nanocapsules with some advanced technology like Raman endoscope should be considered to acquire more accurate and abundant information for the theranostics of grastric diseases. We expect the superstable metal graphitic nanocapsules will offer robust nanoplatforms for future clinical theranostics without the interference of harsh conditions.
YY and SL wrote the original draft of the manuscript. HB and XX edited the manuscript. ZC and YX discussed the scope and content of this review. ZC and LC reviewed the final version of the review.
This work was financially supported by the National Key Research and Development Program of China (2020YFA0210800), the National Natural Science Foundation of China (No. 21522501), the Science and Technology Innovation Program of Hunan Province (No. 2020RC4017), and the Hunan Provincial Education Office General Project of China (No. 21CO349).
The authors declare that the research was conducted in the absence of any commercial or financial relationships that could be construed as a potential conflict of interest.
All claims expressed in this article are solely those of the authors and do not necessarily represent those of their affiliated organizations, or those of the publisher, the editors and the reviewers. Any product that may be evaluated in this article, or claim that may be made by its manufacturer, is not guaranteed or endorsed by the publisher.
Abu Shady, M. M., Fathy, H. A., Ali, A., Galal, E. M., Fathy, G. A., and Sibaii, H. (2015). Comparison of Serum IgG Antibody Test with Gastric Biopsy for the Detection of Helicobacter pylori Infection Among Egyptian Children. Open Access Maced. J. Med. Sci. 3, 303–306. doi:10.3889/oamjms.2015.062
Azharuddin, M., Zhu, G. H., Das, D., Ozgur, E., Uzun, L., Turner, A. P. F., et al. (2019). A Repertoire of Biomedical Applications of Noble Metal Nanoparticles. Chem. Commun. 55, 6964–6996. doi:10.1039/c9cc01741k
Brestoff, J. R., and Artis, D. (2013). Commensal Bacteria at the Interface of Host Metabolism and the Immune System. Nat. Immunol. 14, 676–684. doi:10.1038/ni.2640
Cai, X., Xu, Y., Zhao, L., Xu, J., Li, S., Wen, C., et al. (2021). In Situ pepsin-assisted Needle Assembly of Magnetic-Graphitic-Nanocapsules for Enhanced Gastric Retention and Mucus Penetration. Nano Today 36, 101032. doi:10.1016/j.nantod.2020.101032
Celik, C., Can Sezgin, G., Kocabas, U. G., Gursoy, S., Ildiz, N., Tan, W., et al. (2021). Novel Anthocyanin-Based Colorimetric Assay for the Rapid, Sensitive, and Quantitative Detection of Helicobacter pylori. Anal. Chem. 93, 6246–6253. doi:10.1021/acs.analchem.1c00663
Chen, Z., Hong, G., Wang, H., Welsher, K., Tabakman, S. M., Sherlock, S. P., et al. (2012). Graphite-coated Magnetic Nanoparticle Microarray for Few-Cells Enrichment and Detection. ACS Nano 6, 1094–1101. doi:10.1021/nn2034692
Dong, Q., Wang, X., Hu, X., Xiao, L., Zhang, L., Song, L., et al. (2018). Simultaneous Application of Photothermal Therapy and an Anti-inflammatory Prodrug Using Pyrene-Aspirin-Loaded Gold Nanorod Graphitic Nanocapsules. Angew. Chem. 130, 183–187. doi:10.1002/ange.201709648
Dong, Q., Li, Z., Xu, J., Yuan, Q., Chen, L., and Chen, Z. (2022). Versatile Graphitic Nanozymes for Magneto Actuated Cascade Reaction-Enhanced Treatment of S. Mutans Biofilms. Nano Res. doi:10.1007/s12274-022-4258-x
Gao, W., Wang, X., Fan, H., Song, Z., Lai, X., Chen, Z., et al. (2015). Fabrication of Superstable Gold Nanorod-Carbon Nanocapsule as a Molecule Loading Material. Sci. Bull. 60, 1101–1107. doi:10.1007/s11434-015-0814-z
Gao, C., Lyu, F., and Yin, Y. (2021). Encapsulated Metal Nanoparticles for Catalysis. Chem. Rev. 121, 834–881. doi:10.1021/acs.chemrev.0c00237
Han, Y., Lupitskyy, R., Chou, T.-M., Stafford, C. M., Du, H., and Sukhishvili, S. (2011). Effect of Oxidation on Surface-Enhanced Raman Scattering Activity of Silver Nanoparticles: a Quantitative Correlation. Anal. Chem. 83, 5873–5880. doi:10.1021/ac2005839
Hsieh, V., Okada, S., Wei, H., García-Álvarez, I., Barandov, A., Alvarado, S. R., et al. (2019). Neurotransmitter-responsive Nanosensors for T2-Weighted Magnetic Resonance Imaging. J. Am. Chem. Soc. 141, 15751–15754. doi:10.1021/jacs.9b08744
Hsu, J. C., Naha, P. C., Lau, K. C., Chhour, P., Hastings, R., Moon, B. F., et al. (2018). An All-In-One Nanoparticle (AION) Contrast Agent for Breast Cancer Screening with DEM-CT-MRI-NIRF Imaging. Nanoscale 10, 17236–17248. doi:10.1039/c8nr03741h
Huang, J., Shu, Q., Wang, L., Wu, H., Wang, A. Y., and Mao, H. (2015). Layer-by-layer Assembled Milk Protein Coated Magnetic Nanoparticle Enabled Oral Drug Delivery with High Stability in Stomach and Enzyme-Responsive Release in Small Intestine. Biomaterials 39, 105–113. doi:10.1016/j.biomaterials.2014.10.059
Huang, Y., Ren, J., and Qu, X. (2019). Nanozymes: Classification, Catalytic Mechanisms, Activity Regulation, and Applications. Chem. Rev. 119, 4357–4412. doi:10.1021/acs.chemrev.8b00672
Jain, U., Saxena, K., and Chauhan, N. (2021). Helicobacter pylori Induced Reactive Oxygen Species: a New and Developing Platform for Detection. Helicobacter 26, e12796. doi:10.1111/hel.12796
Jiang, D., Ni, D., Rosenkrans, Z. T., Huang, P., Yan, X., and Cai, W. (2019). Nanozyme: New Horizons for Responsive Biomedical Applications. Chem. Soc. Rev. 48, 3683–3704. doi:10.1039/c8cs00718g
Jin, L., Shaaban, E., Bamonte, S., Cintron, D., Shuster, S., Zhang, L., et al. (2021). Surface Basicity of Metal@TiO2 to Enhance Photocatalytic Efficiency for CO2 Reduction. ACS Appl. Mat. Interfaces 13, 38595–38603. doi:10.1021/acsami.1c09119
Kasztelan, M., Studzinska, A., Żukowska, G. Z., and Pałys, B. (2021). Silver-Graphene Oxide Nanohybrids for Highly Sensitive, Stable SERS Platforms. Front. Chem. 9, 665205. doi:10.3389/fchem.2021.665205
Keoingthong, P., Hao, Q., Li, S., Zhang, L., Xu, J., Wang, S., et al. (2021). Graphene Encapsuled Ru Nanocrystal with Highly-Efficient Peroxidase-like Activity for Glutathione Detection at Near-Physiological pH. Chem. Commun. 57, 7669–7672. doi:10.1039/d1cc02953c
Kim, M., Lee, J. H., and Nam, J. M. (2019). Plasmonic Photothermal Nanoparticles for Biomedical Applications. Adv. Sci. 6, 1900471. doi:10.1002/advs.201900471
Li, H., and Meade, T. J. (2019). Molecular Magnetic Resonance Imaging with Gd(III)-based Contrast Agents: Challenges and Key Advances. J. Am. Chem. Soc. 141, 17025–17041. doi:10.1021/jacs.9b09149
Li, J. F., Huang, Y. F., Ding, Y., Yang, Z. L., Li, S. B., Zhou, X. S., et al. (2010). Shell-isolated Nanoparticle-Enhanced Raman Spectroscopy. Nature 464, 392–395. doi:10.1038/nature08907
Li, Y., Hu, X., Ding, D., Zou, Y., Xu, Y., Wang, X., et al. (2017). In Situ targeted MRI Detection of Helicobacter pylori with Stable Magnetic Graphitic Nanocapsules. Nat. Commun. 8, 1–12. doi:10.1038/ncomms15653
Li, S., Xu, J., Wang, S., Xia, X., Chen, L., and Chen, Z. (2019). Versatile Metal Graphitic Nanocapsules for SERS Bioanalysis. Chin. Chem. Lett. 30, 1581–1592. doi:10.1016/j.cclet.2019.05.049
Li, S., Zhu, Z., Cai, X., Song, M., Wang, S., Hao, Q., et al. (2021). Versatile Graphene‐Isolated AuAg‐Nanocrystal for Multiphase Analysis and Multimodal Cellular Raman Imaging †. Chin. J. Chem. 39, 1491–1497. doi:10.1002/cjoc.202000734
Li, S., Yang, Y., Wang, S., Gao, Y., Song, Z., Chen, L., et al. (2022). Advances in Metal Graphitic Nanocapsules for Biomedicine. Exploration, 20210223. doi:10.1002/EXP.20210223
Liu, Z., Li, S., Xia, X., Zhu, Z., Chen, L., and Chen, Z. (2020). Recent Advances in Multifunctional Graphitic Nanocapsules for Raman Detection, Imaging, and Therapy. Small Methods 4, 1900440. doi:10.1002/smtd.201900440
Liu, Z., Li, S., Yin, Z., Zhu, Z., Chen, L., Tan, W., et al. (2022). Stabilizing Enzymes in Plasmonic Silk Film for Synergistic Therapy of In Situ SERS Identified Bacteria. Adv. Sci. 9, 2104576. doi:10.1002/advs.202104576
Mabilleau, G., Bourdon, S., Joly-Guillou, M. L., Filmon, R., Baslé, M. F., and Chappard, D. (2006). Influence of Fluoride, Hydrogen Peroxide and Lactic Acid on the Corrosion Resistance of Commercially Pure Titanium. Acta Biomater. 2, 121–129. doi:10.1016/j.actbio.2005.09.004
Ma, Y., Liu, Y., Jiang, Z., Lv, H., Wang, J., Wang, T., et al. (2021a). Visualization of the pH-Fluctuations in Gastric Ulcer Living Mice by the In Situ Near-Infrared Imaging. Sensors Actuators B Chem. 349, 130747. doi:10.1016/j.snb.2021.130747
Ma, Z., Mohapatra, J., Wei, K., Liu, J. P., and Sun, S. (2021b). Magnetic Nanoparticles: Synthesis, Anisotropy, and Applications. Chem. Rev. doi:10.1021/acs.chemrev.1c00860
Mirzaei, A., Janghorban, K., Hashemi, B., Bonyani, M., Leonardi, S. G., and Neri, G. (2017). Characterization and Optical Studies of PVP-Capped Silver Nanoparticles. J. Nanostruct Chem. 7, 37–46. doi:10.1007/s40097-016-0212-3
Nie, X. K., Xu, Y. T., Song, Z. L., Ding, D., Gao, F., Liang, H., et al. (2014). Magnetic-graphitic-nanocapsule Templated Diacetylene Assembly and Photopolymerization for Sensing and Multicoded Anti-counterfeiting. Nanoscale 6, 13097–13103. doi:10.1039/c4nr03837a
Perumal, A., Kannan, S., and Nallaiyan, R. (2021). Silver Nanoparticles Incorporated Polyaniline on TiO2 Nanotube Arrays: A Nanocomposite Platform to Enhance the Biocompatibility and Antibiofilm. Surfaces Interfaces 22, 100892. doi:10.1016/j.surfin.2020.100892
Sanders, M. K., and Peura, D. A. (2002). Helicobacter Pylori-Associated Diseases. Curr. Gastroenterol. Rep. 4, 448–454. doi:10.1007/s11894-002-0019-x
Song, Z. L., Chen, Z., Bian, X., Zhou, L. Y., Ding, D., Liang, H., et al. (2014). Alkyne-functionalized Superstable Graphitic Silver Nanoparticles for Raman Imaging. J. Am. Chem. Soc. 136, 13558–13561. doi:10.1021/ja507368z
Stephen, Z. R., Kievit, F. M., and Zhang, M. (2011). Magnetite Nanoparticles for Medical MR Imaging. Mater. Today 14, 330–338. doi:10.1016/S1369-7021(11)70163-8
Tang, W., Li, S., Wang, S., Chen, L., and Chen, Z. (2021). Laser-mediated Enrichment Based Surface Enhanced Raman Analysis. Chem. J. Chin. Univ. 42, 3054–3061. doi:10.7503/cjcu20210153
Wang, X.-B., Wang, Y.-L., Yang, J., Xing, X.-P., Li, J., and Wang, L.-S. (2009). Evidence of Significant Covalent Bonding in Au(CN)2−. J. Am. Chem. Soc. 131, 16368–16370. doi:10.1021/ja908106e
Wu, W., Yang, Y., and Sun, G. (2012). Recent Insights into Antibiotic Resistance inHelicobacter pyloriEradication. Gastroenterology Res. Pract. 2012, 1–8. doi:10.1155/2012/723183
Xu, X., Hou, S., Wattanatorn, N., Wang, F., Yang, Q., Zhao, C., et al. (2018). Precision-guided Nanospears for Targeted and High-Throughput Intracellular Gene Delivery. ACS Nano 12, 4503–4511. doi:10.1021/acsnano.8b00763
Xu, M.-L., Guan, L.-Y., Li, S.-K., Chen, L., and Chen, Z. (2019). Stable Gold Graphitic Nanocapsule Doped Hydrogels for Efficient Photothermal Antibacterial Applications. Chem. Commun. 55, 5359–5362. doi:10.1039/c9cc01933b
Xu, L., Zhang, H., Tian, Y., Jiao, A., Li, S., Tan, Y., et al. (2020). Modified Photochemical Strategy to Support Highly-Purity, Dense and Monodisperse Au Nanospheres on Graphene Oxide for Optimizing SERS Detection. Talanta 209, 120535. doi:10.1016/j.talanta.2019.120535
Xu, Y., Yang, Y., Yin, Z., Cai, X., Xia, X., Donovan, M. J., et al. (2021). In Situ gastric pH Imaging with Hydrogel Capsule Isolated Paramagnetic Metallo-Albumin Complexes. Anal. Chem. 93, 5939–5946. doi:10.1021/acs.analchem.1c00538
Yang, Z., Deng, L., Lan, Y., Zhang, X., Gao, Z., Chu, C.-W., et al. (2014). Molecular Extraction in Single Live Cells by Sneaking in and Out Magnetic Nanomaterials. Proc. Natl. Acad. Sci. U.S.A. 111, 10966–10971. doi:10.1073/pnas.1411802111
Zhang, L., Zhang, J., Zheng, Z., Liao, Y., Xu, Y., Li, Z., et al. (2019). Interaction-transferable Graphene-Isolated Superstable AuCo Nanocrystal-Enabled Direct Cyanide Capture. Anal. Chem. 91, 8762–8766. doi:10.1021/acs.analchem.9b01811
Zhang, L., Dong, Q., Zhang, H., Xu, J., Wang, S., Zhang, L., et al. (2021a). A Magnetocatalytic Propelled Cobalt-Platinum@Graphene Navigator for Enhanced Tumor Penetration and Theranostics. CCS Chem. 3, 2382–2395. doi:10.31635/ccschem.021.202101219
Zhang, L., Zhang, L., Deng, H., Li, H., Tang, W., Guan, L., et al. (2021b). In Vivo activation of pH-Responsive Oxidase-like Graphitic Nanozymes for Selective Killing of Helicobacter pylori. Nat. Commun. 12, 1–10. doi:10.1038/s41467-021-22286-x
Zhu, Z., Li, S., Song, M., Cai, X., Song, Z., Chen, L., et al. (2021). Recent Progress of Versatile Metal Graphitic Nanocapsules in Biomedical Applications. Chem. J. Chin. Univ. 42, 2701–2716. doi:10.7503/cjcu20210118
Keywords: metal graphitic nanocapsules, theranostics, harsh condition, gastric environment, stability
Citation: Yang Y, Li S, Bu H, Xia X, Chen L, Xu Y and Chen Z (2022) Metal Graphitic Nanocapsules for Theranostics in Harsh Conditions. Front. Chem. 10:909110. doi: 10.3389/fchem.2022.909110
Received: 31 March 2022; Accepted: 21 April 2022;
Published: 13 May 2022.
Edited by:
Junjie Zhu, Nanjing University, ChinaReviewed by:
Zhimei He, Nanjing University of Posts and Telecommunications, ChinaCopyright © 2022 Yang, Li, Bu, Xia, Chen, Xu and Chen. This is an open-access article distributed under the terms of the Creative Commons Attribution License (CC BY). The use, distribution or reproduction in other forums is permitted, provided the original author(s) and the copyright owner(s) are credited and that the original publication in this journal is cited, in accordance with accepted academic practice. No use, distribution or reproduction is permitted which does not comply with these terms.
*Correspondence: Zhuo Chen, emh1b2NoZW5AaG51LmVkdS5jbg==
†These authors have contributed equally to this work
Disclaimer: All claims expressed in this article are solely those of the authors and do not necessarily represent those of their affiliated organizations, or those of the publisher, the editors and the reviewers. Any product that may be evaluated in this article or claim that may be made by its manufacturer is not guaranteed or endorsed by the publisher.
Research integrity at Frontiers
Learn more about the work of our research integrity team to safeguard the quality of each article we publish.