- 1School of Chemistry and Chemical Engineering, Yangzhou University, Yangzhou, China
- 2School of Chemistry and Chemical Engineering, Nantong University, Nantong, China
Although the construction and application of pillar[5]arene-based [1]rotaxanes have been extensively studied, the types of stoppers for them are limited. In this work, we designed and prepared three series of pillar[5]arene-based [1]rotaxanes (P5[1]Rs) with pentanedione derivatives, azobenzene derivatives, and salicylaldehyde derivatives as the stoppers, respectively. The obtained P5[1]Rs were fully characterized by NMR (1H, 13C, and 2D), mass spectra, and single-crystal X-ray analysis. We found that the synergic C–H···π, C–H···O interactions and N–H···O, O–H···N hydrogen bonding are the key to the stability of [1]rotaxanes. This work not only enriched the diversity of pillar[n]arene family but also gave a big boost to the pillar[n]arene-based mechanically interlocked molecules
Introduction
Mechanically interlocked molecules (MIMs), mainly including knots, rotaxanes, and catenanes, are a new type of fascinating molecules that contain mechanical bonds and allow for large movements at the molecular level (Hu et al., 2017; Zhou et al., 2020). MIMs cannot be separated without breaking the participating covalent bonds due to the mechanical bonds forming an entanglement in space. During the past decades, MIMs have drawn a tremendous interest not only due to their smart architectures but also due to their potential applications in various fields (Sluysmans et al., 2019; Cornelissen et al., 2021; David et al., 2021). [1]Rotaxanes, which are composed of “T”-shaped axles and macrocyclic wheels and the axles are threaded by their own wheels and connected by covalent bonds, are considered as the fundamental supramolecular systems for the construction of diverse MIMs (Tian et al., 2018; Scheme 1). It is known that there is a huge challenge for the efficient synthesis of [1]rotaxanes due to their subtle structures.
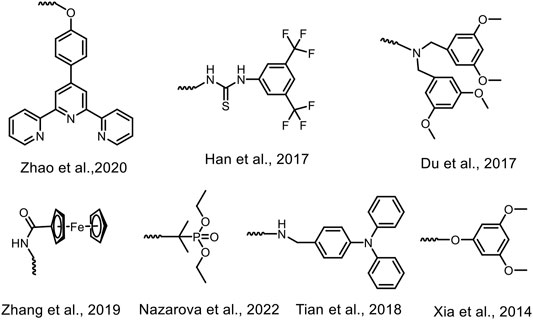
Scheme 1. Chemical structures of different types of reported stoppers for the formation of [1]rotaxanes.
Pillar[5]arenes, first reported by Ogoshi et al. (2008), are considered the fifth generation of classical macrocyclic compounds (An et al., 2021) after crown ethers (Liu et al., 2017; Wu et al., 2020; Bai et al., 2022), cyclodextrins (Bolton et al., 2020; Sugita et al., 2022), calixarenes (An et al., 2019; Li B. et al., 2020; Zhang et al., 2021), and cucurbiturils (Chernikova et al., 2020; Zhang et al., 2022). Pillar[5]arenes are composed of five hydroquinone derivatives, which are linked by –CH2– at the 2,5-positions (Shi et al., 2011; Strutt et al., 2012). During the past 14 years, great developments have been made in the synthesis (Yao et al., 2012; Fan et al., 2016; Wu et al., 2021), host–guest properties (Ogoshi et al., 2015; Zhou et al., 2016; Lu et al., 2022), self-assembly (Guo et al., 2020; Cai et al., 2021a; Cao et al., 2021; Wang et al., 2021; Wu et al., 2022), and applications (Tan et al., 2015; Li L. et al., 2020; Cai et al., 2021b; Guo et al., 2021; Yan et al., 2021) of pillar[5]arenes. Pillar[5]arene-based rotaxanes (P5Rs) have also attracted much attention. In 2011, Prof. Stoddart and co-workers prepared the first P5R in two steps. First, a host–guest complex was fabricated between DMpillar[5]arene and 1,8-diaminooctane. Then, the stopper 3,5-di-tertbutylbenzaldehyde reacted with the amino groups on the guest to block the cavity to form P5R (Strutt et al., 2011). After that, numerous types of P5[1]Rs with various longer axels and different sizes of stoppers have been designed and synthesized (Han et al., 2017; Nazarova et al., 2022; Scheme 1). For example, in 2014, Xia and co-workers designed and synthesized a P5[1]R with the yield up to 73% in three steps (Xia et al., 2014; Scheme 1). Firstly, they prepared a monocarboxylic acid-functionalized pillar[5]arene through the co-oligomerization reaction and a linear guest with a stopper on one side and a primary amine group on the other side. Next, due to the C–H⋯π interactions, the alkyl chain on the guest is passed through the cavity of the pillar[5]arene, where the primary amine group and the carboxylic acid group are expected to form an ion pair complex. Lastly, the primary amine group on the guest and the carboxylic acid group on pillar[5]arene reacted via Schiff-base formation to afford P5[1]R. Besides, our groups have constructed a couple of P5[1]Rs from their parent pseudo[1]rotaxanes in recent years through aldoamine condensation (Zhang et al., 2019; Zhao et al., 2020; Scheme 1).
Although great progress has been made in the research of P5[1]Rs (Du et al., 2017; Scheme 1), the species of P5[1]Rs, especially the types of the stoppers, are not abundant enough. In this work, we designed and prepared three series of P5[1]Rs with different stoppers from their parent pseudo[1]rotaxanes via the “threading-followed-by-stoppering” method. The obtained P5[1]Rs were characterized by various technologies, such as 1H NMR, 13C NMR, 2D-NOESY spectra, and X-ray single-crystal diffraction.
Experimental Section
Syntheses of Pillar[5]arene-Based [1]Rotaxanes
Based on previous reports (Zhang et al., 2019), pseudo[1]rotaxanes 1a and 1b were prepared directly from P5 and alkyl-diamine in CH3CH2OH (Scheme 2, and Supplementary Figures S1–S6). Then, P5[1]Rs were successfully synthesized by 1a or 1b reacted with the stoppers (2, 3, 4, 5) under the catalysis of CH3COOH. [1]Rotaxene 6e is taken as an example. Compound 1b (0.198 g, 0.2 mmol), stopper 2a (0.025 g, 0.2 mmol), and 0.1 ml CH3COOH were stirred in 10 ml of dry CH3CH2OH for 12 h at 80°C. The reaction solvent was evaporated, and the residue was purified by flash column chromatography on silica gel (CH2Cl2/CH3OH, v/v 20:1) to give 6e as a yellow solid (0.167 g). Other P5[1]Rs were prepared according to the same method (Scheme 2).
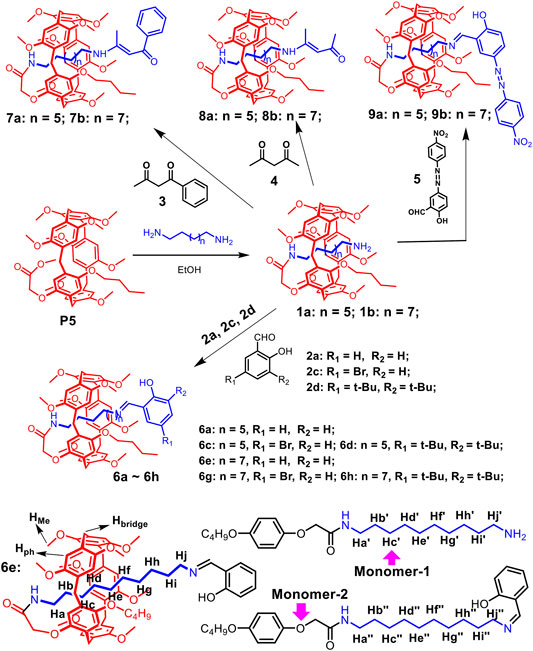
Scheme 2. Synthetic route to pillar[5]arene-based [1]rotaxanes and the chemical structures of 6e and monomers.
6e: light yellow solid, yield 49%. m.p. 85–86°C; 1H NMR (Supplementary Figure S16) (400 MHz, CDCl3) δ 13.57 (s, 1H, OH), 8.39 (s, 1H, CH), 7.36–7.31 (m, 1H, ArH), 7.28 (m, 1H, ArH), 6.99 (d, J = 8.3 Hz, 1H, ArH), 6.91 (m, 6H, ArH), 6.86–6.82 (t, J = 2 Hz, 4H, ArH), 6.71 (s, 1H, ArH), 5.06 (s, 1H, NH), 4.57 (s, 2H, CH2), 3.88 (d, J = 7.0 Hz, 2H, CH2), 3.79–3.71 (m, 34H, 8CH3, 5CH2), 3.64 (t, J = 6.7 Hz, 2H, CH2), 2.45 (s, 2H, CH2), 1.86–1.78 (d, J = 2 Hz, 2H,CH2), 1.73 (t, J = 7.8 Hz, 2H, CH2), 1.61 (d, J = 7.4 Hz, 2H, CH2), 1.40 (t, J = 7.9 Hz, 2H, CH2), 1.19 (s, 2H, CH2), 1.02 (t, J = 7.4 Hz, 3H, CH3), 0.77 (s, 2H, CH2), −0.08 (s, 2H, CH2), −1.38 (s, 4H, CH2), −2.36 (s, 2H,CH2); 13C NMR (Supplementary Figure S17) (101 MHz, CDCl3) δ 167.2, 164.6, 161.3, 150.8, 150.4, 150.3, 150.3, 150.2, 150.0, 147.0, 132.2, 131.1, 129.4, 129.0, 128.3, 128.2, 128.1, 127.9, 127.8, 127.8, 127.1, 126.8, 118.8, 118.6, 117.0, 114.7, 113.9, 113.6, 113.3, 112.7, 112.4, 67.8, 65.8, 59.7, 55.5, 55.4, 55.3, 55.1, 38.0, 32.1, 31.3, 30.8, 30.6, 30.2, 29.7, 29.3, 28.9, 28.7, 28.3, 28.1, 26.5, 23.6, 19.6, 14.1; HRMS (ESI) (Supplementary Figure S18) calcd. for C66H82N2O12([M + Na]+): 1117.5765, found: 1117.5770.
6a: light yellow solid, yield 76%. m.p. 106–107°C; 1H NMR (Supplementary Figure S7) (400 MHz, CDCl3) δ 13.47 (s, 1H, OH), 8.38 (s, 1H, CH), 7.35 (t, J = 8 Hz, 1H, ArH), 7.28 (d, J = 6 Hz, 1H, ArH), 7.00 (d, J = 8.4 Hz, 1H, ArH), 6.92 (m, 5H, ArH), 6.88 (s, 1H, ArH), 6.84 (m, 4H, ArH), 6.73 (s, 1H, ArH), 5.09 (s, 1H, NH), 4.57 (s, 2H, CH2), 3.88 (d, J = 3.6 Hz, 2H, CH2), 3.79–3.69 (m, 34H, 8OCH3, 5CH2), 3.58 (d, J = 6.6 Hz, 2H, CH2), 2.48 (s, 2H, CH2), 1.82 (t, J = 14.7 Hz, 2H, CH2), 1.61 (s, 2H, CH2), 1.53 (d, J = 11.2 Hz, 2H, CH2), 0.99 (t, J = 7.4 Hz, 3H, CH3), 0.81 (s, 2H, CH2), −0.10 (s, 2H, CH2), −1.35 (s, 4H, CH2), −2.31 (s, 2H, CH2); 13C NMR (Supplementary Figure S8) (101 MHz, CDCl3) δ 167.3, 164.4, 161.2, 150.8, 150.4, 150.3, 150.2, 150.1, 150.0, 132.3, 131.0, 130.0, 129.0, 128.4, 128.3, 128.0, 127.9, 127.3, 126.9,118.7, 118.6, 117.1, 115.0, 114.1, 113.7, 113.5, 113.4, 112.8, 112.4, 68.1, 65.9, 60.4, 55.6, 55.5, 55.4, 55.3, 55.2, 38.1, 32.1, 31.9, 30.2, 29.2, 28.9, 28.7, 28.5, 28.3, 28.2, 26.4, 23.6, 19.7, 14.1; HRMS (ESI) (Supplementary Figure S9) calcd. for C64H78N2O12([M + Na]+): 1089.5452, found: 1089.5437.
6c: light yellow solid, yield 89%. m.p. 92–93°C; 1H NMR (Supplementary Figure S10) (400 MHz, CDCl3) δ 13.52 (s, 1H, OH), 8.31 (s, 1H, CH), 7.44–7.38 (m, 2H, ArH), 6.94–6.87 (m, 6H, ArH), 6.85–6.82 (t, J = 4 Hz, 4H, ArH), 6.72 (s, 1H, ArH), 5.09 (s, 1H, NH), 4.57 (s, 2H, CH2), 3.90–3.85 (m, 2H, CH2), 3.80–3.70 (m, 34H, 8OCH3,5CH2), 3.58 (t, J = 7.2 Hz, 2H, CH2), 2.48 (s, 2H, CH2), 1.81 (d, J = 15.5 Hz, 2H, CH2), 1.59 (t, J = 7.5 Hz, 2H, CH2), 1.50 (t, J = 7.3 Hz, 2H, CH2), 0.99 (t, J = 7.4 Hz, 3H, CH3), 0.80 (s, 2H, CH2), −0.11 (s, 2H, CH2), −1.34 (s, 4H, CH2), −2.28 (s, 2H, CH2); 13C NMR (Supplementary Figure S11) (101 MHz, CDCl3) δ 167.3, 163.1, 160.3, 150.8, 150.4, 150.4, 150.3, 150.3, 150.2, 150.0, 147.1, 134.9, 133.1, 129.5, 129.1, 128.4, 128.3, 128.2, 128.1, 127.9, 127.9, 127.3, 126.9, 120.1, 119.1, 115.0, 114.1, 113.8, 113.6, 113.5, 112.7, 112.4, 110.0, 68.1, 65.9, 60.3, 55.7, 55.6, 55.4, 55.3, 55.1, 38.1, 32.0, 31.8, 30.2, 29.3, 29.1, 28.8, 28.7, 28.4, 28.3, 28.1, 26.3, 23.6, 19.6, 14.1; HRMS (ESI) (Supplementary Figure S12) calcd. for C64H77BrN2O12([M + Na]+): 1167.4558, found: 1167.4537.
6d: yellow solid, yield 47%. m.p. 108–109°C; 1H NMR (Supplementary Figure S13) (400 MHz, CDCl3) δ 13.89 (s, 1H, OH), 8.39 (s, 1H, CH), 7.41 (s, 1H, ArH), 7.11 (s, 1H, ArH), 6.91 (d, J = 15.0 Hz, 5H, ArH), 6.86–6.82 (t, 4H, ArH), 6.73 (s, 1H, ArH), 5.07 (s, 1H, NH), 4.57 (s, 2H, CH2), 3.88 (d, J = 12.5 Hz, 2H, CH2), 3.79–3.69 (m, 34H, 8OCH3, 5CH2), 3.60–3.55 (d, J = 4 Hz, 2H), 2.45 (s, 2H, CH2), 1.82 (s, 2H, CH2), 1.59 (s, 4H, CH2), 1.49 (t, J = 6 Hz, 9H, CH3), 1.34 (d, J = 2.4 Hz, 9H, CH3), 1.02–0.98 (m, 3H, CH3), 0.86 (s, 2H, CH2), −0.07 (s, 2H, CH2), −1.29 (s, 4H, CH2), −2.32 (s, 2H, CH2); 13C NMR (Supplementary Figure S14) (101 MHz, CDCl3) δ 167.3, 165.5, 158.1, 150.8, 150.3, 150.2, 150.1, 150.0, 147.0, 140.1, 136.8, 129.5, 129.1, 128.3, 128.2, 128.0, 127.9, 127.2, 126.9, 125.6, 117.8, 114.9, 114.0, 113.6, 113.4, 112.7, 112.4, 68.0, 65.9, 60.3, 55.6, 55.4, 55.3, 55.1, 38.1, 35.1, 32.0, 31.5, 30.2, 29.4, 29.3, 28.8, 28.6, 28.3, 26.3, 23.6, 19.6, 14.1; HRMS (ESI) (Supplementary Figure S15) calcd. for C72H94N2O12([M + Na]+): 1201.6704, found: 1201.6683.
6g: yellow solid, yield 58%. m.p. 126–127°C; 1H NMR (Supplementary Figure S19) (400 MHz, CDCl3) δ 13.59 (s, 1H, OH), 8.30 (s, 1H, CH), 7.40 (d, J = 10.0 Hz, 2H, ArH), 6.98–6.77 (m, 10H, ArH), 6.71 (s, 1H, ArH), 5.06 (s, 1H, NH), 4.56 (s, 2H, CH2), 3.75 (m, 38H, 8OCH3, 7CH2), 2.45 (s, 2H, CH2), 1.87–1.79 (m, 2H, CH2), 1.72 (m, 2H, CH2), 1.57 (t, J = 7.4 Hz, 2H, CH2), 1.38 (m, 2H, CH2), 1.18 (m, 2H, CH2), 1.02 (t, J = 7.3 Hz, 3H, CH3), 0.77 (s, 2H, CH2), −0.08 (s, 2H, CH2), −1.36 (s, 4H, CH2), −2.35 (s, 2H, CH2); 13C NMR (Supplementary Figure S20) (101 MHz, CDCl3) δ 167.3, 163.4, 160.4, 150.8, 150.4, 150.3, 150.1, 130.0, 147.0, 134.9, 133.2, 129.4, 129.0, 128.3, 128.2, 128.1, 128.0, 127.8, 127.2, 126.8, 120.1, 119.1, 114.7, 113.9, 113.6, 113.3, 112.7, 112.4, 119.9, 67.8, 65.9, 59.7, 55.5, 55.4, 55.3, 55.1, 38.0, 32.1, 31.2, 30.8, 30.6, 30.2, 29.7, 29.3, 28.9, 28.7, 28.3, 28.1, 26.5, 23.6, 19.7, 14.1; HRMS (ESI) (Supplementary Figure S21) calcd. for C66H81BrN2O12([M + Na]+): 1195.4871, found: 1195.4853.
6h: yellow solid, yield 63%. m.p. 102–103°C; 1H NMR (Supplementary Figure S22) (400 MHz, CDCl3) δ 13.94 (s, 1H, OH), 8.39 (s, 1H, CH), 7.40 (d, J = 2.4 Hz, 1H, ArH), 7.11 (d, J = 2.4 Hz, 1H, ArH), 6.95–6.81 (m, 9H, ArH), 6.74 (d, J = 10.9 Hz, 1H, ArH), 5.12 (s, 1H, NH), 4.57 (s, 2H, CH2), 3.90–3.61 (m, 38H, 8OCH3, 7CH2), 2.51 (s, 2H, CH2), 1.83 (d, J = 14.9 Hz, 2H, CH2), 1.73 (t, J = 7.7 Hz, 2H, CH2), 1.61 (t, J = 7.6 Hz, 2H, CH2), 1.48 (s, 9H, CH3), 1.44–1.38 (t, J = 12 Hz, 2H, CH2), 1.32 (s, 9H, CH3), 1.18 (s, 2H, CH2), 1.02 (t, J = 7.4 Hz, 3H, CH3), 0.75 (s, 2H, CH2), −0.14 (s, 2H, CH2), −1.20–1.47 (t, J = 52 Hz, 4H, CH2), −2.36 (s, 2H, CH2); 13C NMR (Supplementary Figure S23) (101 MHz, CDCl3) δ 167.3, 165.7, 158.1, 150.9, 150.3, 150.3, 150.2, 150.0, 147.0, 140.1, 136.8, 129.3, 129.0, 128.4, 128.2, 128.1, 128.0, 127.9, 127.8, 127.2, 126.8, 125.6, 117.8, 114.7, 114.1, 114.0, 113.6, 113.3, 112.7, 112.7, 112.3, 67.8, 65.8, 59.6, 55.4, 55.4, 55.4, 55.3, 55.2, 55.1, 38.0, 35.1, 32.1, 31.5, 31.4, 30.8, 30.7, 30.1, 29.7, 29.4, 29.2, 28.9, 28.6, 28.5, 28.3, 28.2, 26.6, 23.6, 19.6, 14.1; HRMS (ESI) (Supplementary Figure S24) calcd. for C74H98N2O12([M + Na]+): 1229.7017, found: 1229.6999.
7a: white solid, yield 36%. m.p. 186–187°C; 1H NMR (Supplementary Figure S25) (400 MHz, CDCl3) δ 11.51 (s, 1H, NH), 7.89 (d, J = 6.6 Hz, 2H, ArH), 7.42 (t, J = 8 Hz, 3H, ArH), 6.94–6.82 (m, 9H, ArH), 6.72 (s, 1H, ArH), 5.71 (s, 1H, CH), 5.05 (s, 1H, NH), 4.57 (s, 2H, CH2), 3.89 (m, 2H, CH2), 3.80–3.71 (m, 34H, 8OCH3, 5CH2), 3.32 (m, 2H, CH2), 2.44 (s, 2H, CH2), 2.13 (s, 3H, CH3), 1.83 (m, 2H, CH2), 1.59–1.48 (m, 4H, CH2), 1.01 (t, J = 7.4 Hz, 3H, CH3), 0.87 (s, 2H, CH2), −0.03 (s, 2H, CH2), −1.37 (d, J = 60.8 Hz, 4H, CH2), −2.32 (s, 2H, CH2); 13C NMR (Supplementary Figure S26) (101 MHz, CDCl3) δ 187.6, 164.2, 150.9, 150.5, 150.4, 150.2, 150.0, 147.2, 140.4, 130.5, 129.7, 129.1, 128.4, 128.2, 128.1, 128.0, 127.9, 127.3, 126.8, 115.2, 114.3, 113.9, 113.7, 112.8, 112.5, 91.9, 68.3, 65.9, 55.8, 55.6, 55.4, 55.3, 55.2, 43.8, 38.0, 32.1, 31.1, 30.1, 29.3, 29.1, 28.9, 28.6, 28.3, 27.9, 19.7, 19.3, 14.1; MS (m/z): HRMS (ESI) (Supplementary Figure S27) calcd. for C67H82N2O12([M + Na]+): 1129.5765, found: 1129.5748.
7b: white solid, yield 36%. m.p. 212–213°C; 1H NMR (Supplementary Figure S28) (400 MHz, CDCl3) δ 11.53 (s, 1H, NH), 7.91–7.86 (t, J = 5.6 Hz, 2H, ArH), 7.42 (t, J = 6.4 Hz, 3H, ArH), 6.94–6.82 (m, 9H, ArH), 6.71 (s, 1H, ArH), 5.71 (s, 1H, CH), 5.04 (s, 1H, NH), 4.57 (s, 2H, CH2), 3.88 (t, J = 6.6 Hz, 2H, CH2), 3.80–3.71 (m, 34H, 8OCH3, 5CH2), 3.38 (m, 2H, CH2), 2.43 (s, 2H, CH2), 2.12 (s, 3H, CH3), 1.83 (s, 2H, CH2), 1.70 (t, J = 7.8 Hz, 2H, CH2), 1.62–1.57 (t, J = 7.2 Hz, 2H, CH2), 1.44 (t, J = 7.8 Hz, 2H, CH2), 1.20 (t, J = 7.7 Hz, 2H, CH2), 1.03 (t, J = 7.4 Hz, 3H, CH3), 0.81 (s, 2H, CH2), −0.05 (s, 2H, CH2), −1.39 (d, J = 47.7 Hz, 4H, CH2), −2.36 (s, 2H, CH2); 13C NMR (Supplementary Figure S29) (101 MHz, CDCl3) δ 187.7, 167.2, 164.6, 150.8, 150.4, 150.3, 150.2, 150.1, 150.0, 147.0, 140.4, 130.5, 129.4, 129.1, 128.6, 128.4, 128.2, 128.0, 127.9, 127.8, 127.1, 127.0, 126.8, 114.8, 114.0, 113.7, 113.3, 112.8, 112.4, 92.0, 77.3, 67.9, 55.5, 55.4, 55.3, 55.1, 43.4, 38.0, 32.1, 30.8, 30.7, 30.4, 30.2, 29.7, 29.3, 28.8, 28.7, 27.8, 19.7, 19.4, 14.1; MS (m/z): HRMS (ESI) (Supplementary Figure S30) calcd. for C69H86N2O12([M + Na]+): 1157.6078, found: 1157.6054.
8a: white solid, yield 54%. m.p. 87–88°C; 1H NMR (Supplementary Figure S31) (400 MHz, CDCl3) δ 10.86 (s, 1H, NH), 6.94–6.81 (m, 9H, ArH), 6.71 (s, 1H, ArH), 5.07 (s, 1H, NH), 5.00 (s, 1H, CH), 4.57 (s, 2H, CH2), 3.88 (t, J = 6.6 Hz, 2H, CH2), 3.81–3.67 (m, 34H, 8OCH3, 5CH2), 3.21 (m, 2H, CH2), 2.45 (s, 2H, CH2), 2.04 (s, 3H, CH3), 1.98 (s, 3H, CH3), 1.82 (m, 2H, CH2), 1.62–1.58 (t, J = 7.6 Hz, 2H, CH2), 1.42 (t, J = 7.8 Hz, 2H, CH2), 1.02 (t, J = 7.3 Hz, 3H, CH3), 0.79 (s, 2H, CH2), −0.08 (s, 2H, CH2), −1.32 (s, 4H, CH2), −2.33 (s, 2H, CH2); 13C NMR (Supplementary Figure S32) (101 MHz, CDCl3) δ 194.8, 167.2, 162.4, 150.9, 150.5, 150.4, 150.3, 150.2, 150.1, 150.0, 147.2, 129.6, 129.1, 128.4, 128.3, 128.2, 128.1, 128.0, 127.9, 127.3, 126.9, 115.7, 115.2, 114.3, 113.9, 113.7, 113.6, 112.8, 112.4, 95.1, 68.2, 55.7, 55.6, 55.5, 55.4, 55.3, 55.1, 43.6, 38.0, 32.05, 31.2, 29.3, 29.1, 28.8, 28.5, 28.3, 27.8, 19.7, 18.7, 14.1; MS (m/z): HRMS (ESI) (Supplementary Figure S33) calcd. for C62H80N2O12([M + Na]+): 1067.5609, found: 1067.5613.
8b: white solid, yield 39%. m.p. 86–87°C; 1H NMR (Supplementary Figure S34) (400 MHz, CDCl3) δ 10.92 (s, 1H, NH), 6.94–6.82 (m, 9H, ArH), 6.70 (s, 1H, ArH), 5.04 (s, 1H, NH), 5.00 (s, 1H, CH), 4.57 (s, 2H, CH2), 3.87 (t, J = 6.5 Hz, 2H, CH2), 3.80–3.68 (m, 34H, 8OCH3, 5CH2), 3.28 (m, 2H, CH2), 2.42 (s, 2H, CH2), 2.03 (s, 3H, CH3), 1.97 (s, 3H, CH3), 1.83 (s, 2H, CH2), 1.64 (d, J = 7.4 Hz, 2H, CH2), 1.58 (d, J = 7.4 Hz, 2H, CH2), 1.42–1.36 (m, 2H, CH2), 1.20–1.15 (t, J = 8 Hz, 2H, CH2), 1.03 (t, J = 7.4 Hz, 3H, CH3), 0.83–0.77 (d, J = 8.8 Hz, 2H,CH2), −0.06 (s, 2H, CH2), −1.40 (d, J = 53.4 Hz, 4H, CH2), −2.37 (s, 2H, CH2); 13C NMR (Supplementary Figure S35) (101 MHz, CDCl3) δ 194.9, 167.2, 162.9, 150.8, 150.4, 150.3, 150.2, 150.1, 150.0, 147.0, 129.4, 129.0, 128.4, 128.2, 128.2, 128.0, 127.9, 127.8, 127.1, 126.8, 114.8, 114.0, 113.7, 113.3, 112.7, 112.4, 95.2, 67.9, 65.9, 55.5, 55.4, 55.3, 55.1, 43.1, 38.0, 32.1, 30.8, 30.6, 30.5, 30.2, 29.7, 29.3, 28.8, 28.8, 28.7, 28.2, 27.8, 26.4, 19.7, 18.8, 14.1; MS (m/z): HRMS (ESI) (Supplementary Figure S36) calcd. for C64H84N2O12([M + Na]+): 1095.5922, found: 1095.5912.
9a: red solid, yield 56%. m.p. 100–102°C; 1H NMR (Supplementary Figure S37) (400 MHz, CDCl3) δ 8.45 (s, 1H, CH), 8.39 (m, 2H, ArH), 8.09–7.98 (m, 5H,ArH), 7.10 (d, J = 9.0 Hz, 1H, ArH), 6.94–6.89 (t, J = 14.4 Hz, 4H, ArH), 6.84 (d, J = 5.5 Hz, 4H, ArH), 6.74 (s, 1H, ArH), 5.15 (s, 1H, NH), 4.57 (s, 2H, CH2), 3.89 (s, 2H, CH2), 3.80–3.72 (m, 34H, 8OCH3, 5CH2), 3.55 (s, 2H, CH2), 2.53 (s, 2H,CH2), 1.82 (s, 2H, CH2), 1.61–1.57 (t, J = 7.6 Hz, 2H,CH2), 1.47 (s, 2H, CH2), 1.00 (t, J = 7.4 Hz, 3H, CH3), 0.76 (s, 2H, CH2), −0.11 (s, 2H, CH2), −1.18 (d, J = 62.5 Hz, 4H, CH2), −2.16 (s, 2H, CH2); 13C NMR (Supplementary Figure S38) (101 MHz, CDCl3) δ 196.3, 168.4, 167.4, 164.1, 156.0, 150.8, 150.5, 150.4, 150.3, 150.2, 150.0, 148.2, 147.2, 144.6, 130.6, 129.6, 129.1, 128.4, 128.3, 128.2, 128.1, 127.9, 127.4, 127.2, 127.0, 124.8, 124.8, 123.3, 123.0, 119.7, 119.0, 117.6, 115.1, 114.2, 114.0, 113.8, 113.6, 112.8, 112.4, 77.2, 68.2, 55.9, 55.8, 55.6, 55.5, 55.3, 55.1, 38.1, 32.1, 31.4, 30.2, 29.3, 28.9, 28.3, 27.8, 19.6, 14.1; MS (m/z): HRMS (ESI) (Supplementary Figure S39) calcd. for C70H81N5O14([M + Na]+): 1238.5678, found: 1238.5674.
9b: red solid, yield 89%. m.p. 100–101°C; 1H NMR (Supplementary Figure S40) (400 MHz, CDCl3) δ 8.46 (s, 1H, CH), 8.37 (d, J = 8.5 Hz, 2H, ArH), 8.06 (d, J = 9.4 Hz, 1H, ArH), 7.98 (d, J = 8.6 Hz, 3H, ArH), 7.09 (d, J = 9.0 Hz, 1H, ArH), 6.94–6.83 (m, 9H, ArH), 6.72 (s, 1H, ArH), 5.08 (s, 1H, NH), 4.57 (s, 2H, CH2), 3.88 (s, 2H, CH2), 3.75 (m, 34H, 8OCH3, 5CH2), 3.68 (s, 2H, CH2), 2.47 (s, 2H, CH2), 1.79 (d, J = 33.9 Hz, 4H, CH2), 1.60 (d, J = 7.5 Hz, 2H, CH2), 1.41 (s, 2H, CH2), 1.20 (s, 2H, CH2), 1.02 (t, J = 7.5 Hz, 3H, CH3), 0.80 (s, 2H, CH2), −0.06 (s, 2H, CH2), −1.31 (s, 4H, CH2), −2.31 (s, 2H, CH2); 13C NMR (Supplementary Figure S41) (101 MHz, CDCl3) δ 164.3, 156.0, 150.8, 150.4, 150.3, 150.2, 150.1, 150.0, 148.2, 147.0, 144.6, 129.6, 129.4, 129.0, 128.3, 128.2, 128.0, 127.9, 127.8, 127.2, 126.8, 124.7, 123.3, 123.0, 119.6, 117.6, 114.8, 114.0, 113.7, 113.3, 112.7, 112.4, 77.2, 67.9, 58.2, 55.5, 55.4, 55.3, 55.1, 38.0, 32.1, 31.0, 30.8, 30.5, 30.2, 29.7, 29.3, 28.8, 28.6, 28.3, 28.0, 23.6, 19.6, 14.1; MS (m/z): HRMS (ESI) (Supplementary Figure S42) calcd. for C70H81N5O14([M + Na]+): 1266.5991, found: 1266.5969.
Synthesis of Monomers
Synthesis of compound Monomer-1: methyl 2-(4-butoxyphenoxy) acetate (1 g, 4.2 mmol) and 1,10-decanediamine (7.7 g, 44.8 mmol) were added to 20 ml of anhydrous ethanol solution and reacted at 75°C for 12 h. The organic solvent was removed by rotation under reduced pressure, and compound Monomer-1 was obtained by column chromatography (volume ratio: dichloromethane: methanol = 10: 1). White solid, 60%; 1H NMR (Supplementary Figure S43) (400 MHz, CDCl3) δ 6.84 (s, 4H, ArH), 6.65 (s, 1H, NH2), 5.31 (s, 1H, NH), 4.43 (s, 2H, CH2), 3.91 (t, J = 6.5 Hz, 2H, CH2), 3.69 (m, 2H, CH2), 3.36–3.30 (m, 2H, CH2), 1.75–1.72 (m, 6H, CH2), 1.51–1.40 (m, 10H, CH2), 1.23 (t, J = 7.0 Hz, 4H, CH2), 0.97 (t, J = 7.4 Hz, 3H, CH3).
Synthesis of compound Monomer-2: Monomer-1 (0.208 g, 0.55 mmol) and salicylaldehyde (0.06 g, 0.5 mmol) were added to 20 ml of anhydrous ethanol solution and reacted at 80°C for 4 h. The organic solvent was removed by rotating under reduced pressure, and the compound Monomer-2 was obtained by column chromatography (volume ratio: ethyl acetate: petroleum ether = 1:5). Yellow solid, 50%; 1H NMR (Supplementary Figure S44) (400 MHz, CDCl3) δ 13.73 (s, 1H, OH), 8.33 (s, 1H, CH), 7.32–7.27 (m, 1H, ArH), 6.95 (d, J = 8.2 Hz, 1H, ArH), 6.84 (s, 4H, ArH), 6.60 (s, 1H, ArH), 4.43 (s, 2H, CH2), 3.91 (t, J = 6.5 Hz, 2H, CH2), 3.58 (t, J = 6.9 Hz, 2H, CH2), 3.33 (m, 2H, CH2), 1.79–1.64 (m, 4H, CH2), 1.49 (m, 6H, CH2), 1.28 (t, J = 4.9 Hz, 10H, CH2), 0.97 (t, J = 7.4 Hz, 3H, CH3).
Materials and Methods
Stoppers 2a, 2c, 2d, 3, 4, and 5 and reagents [1,10-decanediamine, methyl 2-(4-butoxyphenoxy)acetate, and so on] were commercially available (99%) and used as received. Further purification and drying of the solvents by standard methods were employed and distilled prior to use when necessary.
1H NMR and 13C NMR spectra were recorded on a Bruker AVIII-400 MHz spectrometer. 2D NMR spectra were recorded on a Bruker AV-600 MHz spectrometer. All NMR used tetramethylsilane (TMS) as the internal standard.
A Bruker Micro-TOF spectrometer was used to investigate the high-resolution mass (ESI) of the compounds.
A Bruker Smart APEX-2 CCD diffractometer was used to investigate the X-ray single-crystal structures.
Results and Discussion
1H NMR Investigation
As shown in Scheme 2, P5[1]Rs were synthesized from pseudo[1]rotaxane 1a or 1b reacted with different stoppers in one step. We used 1H NMR spectra to characterize the obtained P5[1]Rs firstly. It can be clearly found that there were several groups of protons in the high magnetic field (δ < 0 ppm) of the 1H NMR spectra of 6a, 6c, 6d, 6e, 6g, 6h, 7a, 7b, 8a, 8b, 9a, and 9b, indicating that the alkyl chain was penetrated into the cavity of pillar[5]arene (Zhang et al., 2020; Ding et al., 2021). [1]Rotaxane 6e is taken as an example; monomer-2 and 6e in CDCl3 at 293 K are shown in Figures 1D,C. Compared with monomer-2, we found that the signals of protons Hd, He, Hf, Hg, and Hh on the alkyl chain attached onto the pillar[5]arene platform shifted upfield from 1.277 to 0.756, −0.094, −1.292, −1.382, and −2.401 ppm due to the shielding effect, indicating the formation of a mechanically interlocked structure. Then, we used DMSO-d6 as the solvent for 1H NMR investigation to confirm the formation of [1]rotaxane. As is known that DMSO is a de-complexometric solvent, in DMSO-d6, the signals of protons on the alkyl chains upfield obviously below 0 ppm due to the shielding effect (Figure 1B), confirming that the stopper units are large enough for blocking the cavity of pillar[5]arene. Further 13C NMR and HR-MS studies also confirmed that these P5[1]Rs were prepared successfully.
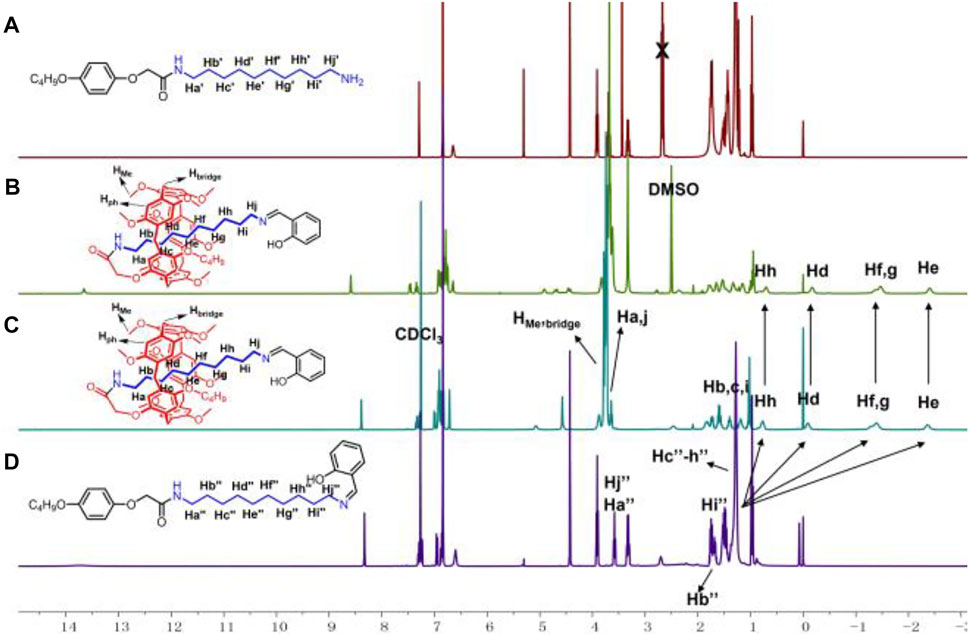
FIGURE 1. 1H NMR spectra (400 MHz, 298K) of (A) monomer-1 in CDCl3, (B) 6e in DMSO-d6, (C) 6e in CDCl3, and (D) monomer-2 in CDCl3.
2D NOESY Investigation
2D nuclear overhauser effect spectroscopy (NOESY) was then used to characterize the stereochemical structure of the obtained [1]rotaxanes. We also take 6e as an example. As shown in Figure 2, the hydrogens (Hb, Hc, Hd, He, Hf, Hg, Hh, and Hi) of the alkyl chain on 6e showed strong correlation with the bridged -CH2- (Hbridge) and the hydrogen–OCH3 and -OCH2- (HMe) on the 1,4-dimethoxybenzene, indicating that the alkyl chain was passed through the cavity and consisted of the above 1H NMR results. The NOESY spectrum of 6e in DMSO-d6 also confirmed the interlocked structure (Supplementary Figure S45).
Single-Crystal Structures
With the aim to further study the mechanically interlocked structure of 6e, a colorless crystal was grown by a vapor diffusion method. As shown in Figure 3A, the alkyl chain penetrated into the cavity of pillar[5]arene and the salicylaldiminato unit acted as a stopper to form [1]rotaxane. One amide N–H···O hydrogen bonding and an O–H···N hydrogen bonding were observed in Figure 3A. Besides the weak C–H···O interactions between the CH2 of the axle and the O atoms of the host (Figures 3B,C), multiple C–H···π interactions between CH2 of the axle and benzene units of the host (Figure 3D) were also observed. So, the driving forces for the formation of [1]rotaxane was the synergic C–H···π, C–H···O interactions and N–H···O hydrogen bonding.
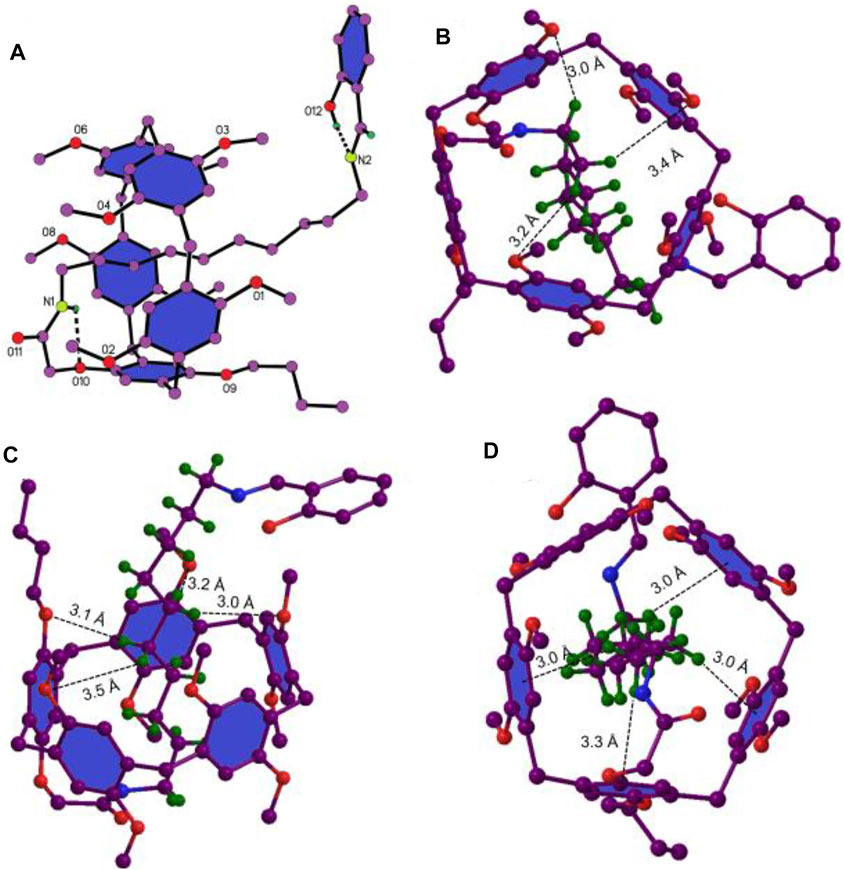
FIGURE 3. The single-crystal structure of 6e, where only the hydrogens in question are given for clarity. (A) N–H···O and O–H···N hydrogen bonding. (B) and (C) C–H···O interaction, H···O distances, 3.0 Å, 3.2 Å, 3.4 Å, 3.0 Å, 3.2 Å, 3.1 Å, and 3.5 Å. (D) C–H···π interactions, H···ring center distances, 3.0 Å, 3.3 Å 3.0 Å, and 3.0 Å.
Conclusion
In this article, we have successfully designed and synthesized three series of pillar [5]arene-based [1]rotaxanes (P5[1]Rs) with pentanedione derivatives, azobenzene derivatives, and salicylaldehyde derivatives as the stoppers, respectively. 1H NMR, 13C NMR, 2D-NOESY, MS, and single-crystal X-ray analysis were used to characterize the obtained P5[1]Rs. We found that the driving forces for the formation of [1]rotaxane were the synergic C–H···π, C–H···O interactions and N–H···O, O–H···N hydrogen bonding. This work not only enriched the diversity of pillar[n]arene family but also gave a big boost to the pillar[n]arene-based MIMs.
Data Availability Statement
The data presented in the study are deposited in the Cambridge Crystallographic Data Centre repository, accession number 2120389.
Author Contributions
LM, TC, and YW prepared all the compounds. YH, CY, and YY analyzed the data. YH and YY wrote this article.
Funding
This work was supported by the National Natural Science Foundation of China (32101215 and 22007052), the Natural Science Foundation of Jiangsu Province (BK20190917), and the Natural Science Foundation of Nantong City (MS12021079).
Conflict of Interest
The authors declare that the research was conducted in the absence of any commercial or financial relationships that could be construed as a potential conflict of interest.
Publisher’s Note
All claims expressed in this article are solely those of the authors and do not necessarily represent those of their affiliated organizations, or those of the publisher, the editors, and the reviewers. Any product that may be evaluated in this article, or claim that may be made by its manufacturer, is not guaranteed or endorsed by the publisher.
Acknowledgments
The authors also thank the Nantong University Analysis and Testing Center for characterization.
Supplementary Material
The Supplementary Material for this article can be found online at: https://www.frontiersin.org/articles/10.3389/fchem.2022.908773/full#supplementary-material
References
An, L., Wang, C., Han, L., Liu, J., Huang, T., Zheng, Y., et al. (2019). Structural Design, Synthesis, and Preliminary Biological Evaluation of Novel Dihomooxacalix[4]arene-Based Anti-tumor Agents. Front. Chem. 7, 856. doi:10.3389/fchem.2019.00856
An, L., Wang, C., Zheng, Y.-G., Liu, J.-d., and Huang, T.-h. (2021). Design, Synthesis and Evaluation of Calix[4]arene-Based Carbonyl Amide Derivatives with Antitumor Activities. Eur. J. Med. Chem. 210, 112984. doi:10.1016/j.ejmech.2020.112984
Bai, R., Zhang, H., Yang, X., Zhao, J., Wang, Y., Zhang, Z., et al. (2022). Supramolecular Polymer Networks Crosslinked by crown Ether-Based Host-Guest Recognition: Dynamic Materials with Tailored Mechanical Properties in the Bulk. Polym. Chem. 13, 1253–1259. doi:10.1039/D1PY01536B
Bolton, S. G., and Pluth, M. D. (2020). Modified Cyclodextrins Solubilize Elemental Sulfur in Water and Enable Biological Sulfane Sulfur Delivery. Chem. Sci. 11, 11777–11784. doi:10.1039/D0SC04137H
Cai, Y., Yan, X., Wang, S., Zhu, Z., Cen, M., Ou, C., et al. (2021a). Pillar[5]arene-Based 3D Hybrid Supramolecular Polymer for Green Catalysis in Water. Inorg. Chem. 60, 2883–2887. doi:10.1021/acs.inorgchem.0c03645
Cai, Y., Zhang, Z., Ding, Y., Hu, L., Wang, J., Chen, T., et al. (2021b). Recent Development of Pillar[n]arene-Based Amphiphiles. Chin. Chem. Lett. 32, 1267–1279. doi:10.1016/j.cclet.2020.10.036
Cao, Y., Chen, Y., Zhang, Z., Wang, J., Yuan, X., Zhao, Q., et al. (2021). CO2 and Photo-Controlled Reversible Conversion of Supramolecular Assemblies Based on Water Soluble Pillar[5]arene and Coumarin-Containing Guest. Chin. Chem. Lett. 32, 349–352. doi:10.1016/j.cclet.2020.03.058
Chernikova, E. Y., and Berdnikova, D. V. (2020). Cucurbiturils in Nucleic Acids Research. Chem. Commun. 56, 15360–15376. doi:10.1039/D0CC06583H
David, A. H. G., and Stoddart, J. F. (2021). Chiroptical Properties of Mechanically Interlocked Molecules. Isr. J. Chem. 61, 608–621. doi:10.1002/ijch.202100066
Ding, Y., Wang, C., Lu, B., and Yao, Y. (2021). Enhancing the Stability and Photothermal Conversion Efficiency of ICG by Pillar[5]arene-Based Host-Guest Interaction. Front. Chem. 9, 775436. doi:10.3389/fchem.2021.775436
Du, X.-S., Wang, C.-Y., Jia, Q., Deng, R., Tian, H.-S., Zhang, H.-Y., et al. (2017). Pillar[5]arene-based [1]rotaxane: High-Yield Synthesis, Characterization and Application in Knoevenagel Reaction. Chem. Commun. 53, 5326–5329. doi:10.1039/C7CC02364B
Fan, C., Wu, W., Chruma, J. J., Zhao, J., and Yang, C. (2016). Enhanced Triplet-Triplet Energy Transfer and Upconversion Fluorescence through Host-Guest Complexation. J. Am. Chem. Soc. 138, 15405–15412. doi:10.1021/jacs.6b07946
Guo, H., Ye, J., Zhang, Z., Wang, Y., Yuan, X., Ou, C., et al. (2020). Pillar[5]arene-Based [2]Rotaxane: Synthesis, Characterization, and Application in a Coupling Reaction. Inorg. Chem. 59, 11915–11919. doi:10.1021/acs.inorgchem.0c01752
Guo, S., Huang, Q., Chen, Y., Wei, J., Zheng, J., Wang, L., et al. (2021). Synthesis and Bioactivity of Guanidinium‐Functionalized Pillar[5]arene as a Biofilm Disruptor. Angew. Chem. Int. Ed. 60, 618–623. doi:10.1002/anie.202013975
Hu, F., Li, Z., Li, X., Yin, J., and Liu, S. (2017). Photochromism in Mechanically Interlocked Molecules. Coc 21, 450–462. doi:10.2174/1385272820666160919105428
Li, B., Cui, L., and Li, C. (2020). Macrocycle Co‐Crystals Showing Vapochromism to Haloalkanes. Angew. Chem. Int. Ed. 59, 22012–22016. doi:10.1002/anie.202010802
Li, L., Tuo, W., Zhu, Q., Sepehrpour, H., Yao, Y., Yan, C., et al. (2020). Resorcinarene Induced Assembly of Carotene and Lutein into Hierarchical Superstructures. J. Am. Chem. Soc. 142, 20583–20587. doi:10.1021/jacs.0c10901
Liu, Z., Nalluri, S. K. M., and Stoddart, J. F. (2017). Surveying Macrocyclic Chemistry: from Flexible crown Ethers to Rigid Cyclophanes. Chem. Soc. Rev. 46, 2459–2478. doi:10.1039/C7CS00185A
Lu, B., Yan, X., Wang, J., Jing, D., Bei, J., Cai, Y., et al. (2022). Rim-differentiated Pillar[5]arene Based Nonporous Adaptive Crystals. Chem. Commun. 58, 2480–2483. doi:10.1039/D1CC07124F
Ogoshi, T., Kanai, S., Fujinami, S., Yamagishi, T.-a., and Nakamoto, Y. (2008). para-Bridged Symmetrical Pillar[5]arenes: Their Lewis Acid Catalyzed Synthesis and Host-Guest Property. J. Am. Chem. Soc. 130, 5022–5023. doi:10.1021/ja711260m
Ogoshi, T., Sueto, R., Yoshikoshi, K., Sakata, Y., Akine, S., and Yamagishi, T. a. (2015). Host-Guest Complexation of Perethylated Pillar[5]arene with Alkanes in the Crystal State. Angew. Chem. Int. Ed. 54, 9849–9852. doi:10.1002/anie.201503489
Si, W., Chen, L., Hu, X.-B., Tang, G., Chen, Z., Hou, J.-L., et al. (2011). Selective Artificial Transmembrane Channels for Protons by Formation of Water Wires. Angew. Chem. Int. Ed. 50, 12564–12568. doi:10.1002/anie.201106857
Sluysmans, D., and Stoddart, J. F. (2019). The Burgeoning of Mechanically Interlocked Molecules in Chemistry. Trends Chem. 1, 185–197. doi:10.1016/j.trechm.2019.02.013
Strutt, N. L., Fairen-Jimenez, D., Iehl, J., Lalonde, M. B., Snurr, R. Q., Farha, O. K., et al. (2012). Incorporation of an A1/A2-Difunctionalized Pillar[5]arene into a Metal-Organic Framework. J. Am. Chem. Soc. 134, 17436–17439. doi:10.1021/ja3082523
Strutt, N. L., Forgan, R. S., Spruell, J. M., Botros, Y. Y., and Stoddart, J. F. (2011). Monofunctionalized Pillar[5]arene as a Host for Alkanediamines. J. Am. Chem. Soc. 133, 5668–5671. doi:10.1021/ja111418j
Sugita, Y., Aoki, D., Tokita, M., and Otsuka, H. (2022). Mechanochromic Cyclodextrins. Chem. Commun. 58, 3067–3070. doi:10.1039/D2CC00363E
Tan, L.-L., Li, H., Qiu, Y.-C., Chen, D.-X., Wang, X., Pan, R.-Y., et al. (2015). Stimuli-responsive Metal-Organic Frameworks Gated by Pillar[5]arene Supramolecular Switches. Chem. Sci. 6, 1640–1644. doi:10.1039/C4SC03749A
Tian, H., Wang, C., Lin, P.-H., and Meguellati, K. (2018). Synthesis and Characterization of a New Pillar[5]arene-Based [1]rotaxane. Tetrahedron Lett. 59, 3416–3422. doi:10.1016/j.tetlet.2018.08.004
van Maarseveen, J. H., Cornelissen, M. D., and Pilon, S. (2021). Covalently Templated Syntheses of Mechanically Interlocked Molecules. Synthesis 53, 4527–4548. doi:10.1055/a-1665-4650
Wang, X.-H., Lou, X.-Y., Lu, T., Wang, C., Tang, J., Liu, F., et al. (2021). Supramolecular Engineering of Efficient Artificial Light-Harvesting Systems from Cyanovinylene Chromophores and Pillar[5]arene-Based Polymer Hosts. ACS Appl. Mater. Inter. 13, 4593–4604. doi:10.1021/acsami.0c21651
Wu, H., and Xiao, T. (2020). Supramolecular Polymers with AIE Property Fabricated from a Cyanostilbene Motif-Derived Ditopic Benzo-21-Crown-7 and a Ditopic Dialkylammonium Salt. Front. Chem. 8, 610093. doi:10.3389/fchem.2020.610093
Wu, L., Han, C., Jing, X., and Yao, Y. (2021). Rim-differentiated Pillar[5]arenes. Chin. Chem. Lett. 32 (11), 3322–3330. doi:10.1016/j.cclet.2021.04.046
Wu, Y., Qin, H., Shen, J., Li, H., Shan, X., Xie, M., et al. (2022). Pillararene-containing Polymers with Tunable Fluorescence Properties Based on Host-Guest Interactions. Chem. Commun. 58, 581–584. doi:10.1039/D1CC05962A
Xia, B., and Xue, M. (2014). Design and Efficient Synthesis of a Pillar[5]arene-Based [1]rotaxane. Chem. Commun. 50, 1021–1023. doi:10.1039/C3CC48014C
Yan, X., Huang, Y., Cen, M., Wang, J., Shi, J., Lu, B., et al. (2021). Pillar[6]arene-based Supramolecular Polymeric Materials Constructed via Electrostatic Interactions for Rapid and Efficient Organic Dye Removal from Water. Nanoscale Adv. 3, 1906–1909. doi:10.1039/D0NA00938E
Yao, Y., Xue, M., Chen, J., Zhang, M., and Huang, F. (2012). An Amphiphilic Pillar[5]arene: Synthesis, Controllable Self-Assembly in Water, and Application in Calcein Release and TNT Adsorption. J. Am. Chem. Soc. 134, 15712–15715. doi:10.1021/ja3076617
Zhang, D.-Y., Zheng, Z., Zhao, H., Wang, H.-Y., Ding, F., Li, H.-B., et al. (2021). Structurally Screening Calixarenes as Peptide Transport Activators. Chem. Commun. 57, 12627–12630. doi:10.1039/D1CC05414G
Zhang, R., Wang, C., Long, R., Chen, T., Yan, C., and Yao, Y. (2019). Pillar[5]arene Based [1]rotaxane Systems with Redox-Responsive Host-Guest Property: Design, Synthesis and the Key Role of Chain Length. Front. Chem. 7, 508. doi:10.3389/fchem.2019.00508
Zhang, R., Wang, C., Sun, J., Yan, C., and Yao, Y. (2019). Design and Construction of Pillar[5]arene-Based Bis-[1]rotaxane. Chin. J. Org. Chem.J. Org. Chem. 39, 3483–3489. doi:10.6023/cjoc201906006
Zhang, R., Yan, X., Guo, H., Hu, L., Yan, C., Wang, Y., et al. (2020). Supramolecular Polymer Networks Based on Pillar[5]arene: Synthesis, Characterization and Application in the Fenton Reaction. Chem. Commun. 56, 948–951. doi:10.1039/C9CC09155F
Zhang, X., Qi, S., Liu, D., Du, J., and Jin, J. (2022). PSMA-targeted Supramolecular Nanoparticles Prepared from Cucurbit[8]uril-Based Ternary Host-Guest Recognition for Prostate Cancer Therapy. Front. Chem. 10, 847523. doi:10.3389/fchem.2022.847523
Zhao, L.-L., Han, Y., and Yan, C.-G. (2020). Construction of [1]rotaxanes with Pillar[5]arene as the Wheel and Terpyridine as the Stopper. Chin. Chem. Lett. 31, 81–83. doi:10.1016/j.cclet.2019.04.024
Zhou, H.-Y., Han, Y., and Chen, C.-F. (2020). pH-Controlled Motions in Mechanically Interlocked Molecules. Mater. Chem. Front. 4, 12–28. doi:10.1039/C9QM00546C
Keywords: pillar[5]arene, mechanically interlocked molecule, self-inclusion, host–guest interaction, [1]rotaxanes
Citation: Ma L, Han Y, Yan C, Chen T, Wang Y and Yao Y (2022) Construction and Property Investigation of Serial Pillar[5]arene-Based [1]Rotaxanes. Front. Chem. 10:908773. doi: 10.3389/fchem.2022.908773
Received: 31 March 2022; Accepted: 08 April 2022;
Published: 07 June 2022.
Edited by:
Wei Wang, East China Normal University, ChinaReviewed by:
Pi Wang, Taiyuan University of Technology, ChinaChengyou Han, China University of Petroleum, China
Copyright © 2022 Ma, Han, Yan, Chen, Wang and Yao. This is an open-access article distributed under the terms of the Creative Commons Attribution License (CC BY). The use, distribution or reproduction in other forums is permitted, provided the original author(s) and the copyright owner(s) are credited and that the original publication in this journal is cited, in accordance with accepted academic practice. No use, distribution or reproduction is permitted which does not comply with these terms.
*Correspondence: Ying Han, Hanying@yzu.edu.cn; Yong Yao, yaoyong1986@ntu.edu.cn