- 1Department of Organic Chemistry, College of Pharmacy, Harbin Medical University, Harbin, China
- 2MIIT Key Laboratory of Critical Materials Technology for New Energy Conversion and Storage, School of Chemistry and Chemical Engineering, Harbin Institute of Technology, Harbin, China
- 3State Key Laboratory of Urban Water Resource and Environment, Harbin Institute of Technology, Harbin, China
Spiro-OMeTAD is the well-known hole transporting material (HTM) in perovskite solar cells. In this work, its derivatives, namely four D-A shaped triphenylamine or biphenylamine endcapped indenone spirofluorene (SFD-TPA, SFD-OMeTPA, SFD-TAD, and SFD-OMeTAD), were designed and synthesized. With the introduction of electron-donating moieties and the extension of conjugation length, a series of changes in photophysical and electrochemical properties could be detected. Notably, in comparison with the optical gap (2.96 eV) of the reported spiro-OMeTAD, SFD-OMeTAD presents an optical gap as low as 1.87 eV. Moreover, density functional theory simulations were employed to further investigate their geometric and electronic structures. Finally, steady-state photoluminescence measurements proved the efficient charge separation and collection processes at the perovskite/HTM interface. It can be predicted that all four compounds with enhanced sunlight absorption capability and suitable frontier energy levels can be used as hole-transporting materials for perovskite solar cells.
Introduction
Perovskite solar cells (PSCs) have attracted considerable attention as next-generation energy sources because of their numerous advantages, such as facile processing, prominent power conversion efficiency (PCE), and relatively low fabrication cost (Li X. et al., 2016; Lee et al., 2016; Ge et al., 2018; Shang et al., 2018; Abuhelaiqa et al., 2019; Jiang A. et al., 2019; Bai et al., 2019). Hole-transporting materials (HTMs) are always required to construct high-efficiency PSCs (Stranks and Snaith, 2015; Gangala and Misra, 2018; Wang et al., 2018; Yu et al., 2019). The most commonly used HTM in record-breaking PSCs is 2, 2′, 7, 7′-tetrakis-(N,N-di-p-methoxyphenylamino)-9,9′-spirobifluorene (spiro-OMeTAD) (Li et al., 2015; Ma et al., 2015; Seo et al., 2016; Wang et al., 2016; Xu et al., 2017; Jiang Q. et al., 2019). However, doping lithium bis-(trifluoromethanesulfonyl)imide salt (LiTFSIA) and 4-tertbutylpyridine (TBP) into spiro-OMeTAD is essential to enhance carrier transporting mobilities (Zhou et al., 2018). As a consequence, the stability of PSCs is deceased due to the hydrophilic properties of these dopants.
Donor-acceptor (D-A) type small molecules can be regarded as a good candidate for HTMs because the D-A molecular backbone features intramolecular charge transfer (ICT) characteristics and a high dipole moment, which could induce self-doping and a built-in potential to boost charge extraction (Liu et al., 2015; Steck et al., 2015; Bi et al., 2016; Kim et al., 2016; Liu et al., 2016; Xu et al., 2018; Mai et al., 2021; Zhang et al., 2021). Li et al. reported a triphenylamine (donor) and tricyanovinylene (acceptor) substituted dipolar chromophore (BTPA-TCNE) to serve as an efficient dopant-free HTM for PSCs in 2016 (Li Z. et al., 2016), generating a promising PCE of ∼17.0%. This result outperforms the control devices using doped spiro-OMeTAD HTM. Recently, Guo et al. reported two novel D-A type HTMs with phenylamine groups as the donor units and imide-functionalized thiophene as the acceptor units (Wang et al., 2019). The dopant-free PSCs achieved a remarkable efficiency of 21.17% with negligible hysteresis and superior thermal stability and long-term stability under illumination, which breaks the long-time standing bottleneck in the development of dopant-free HTMs for highly efficient inverted PSCs.
Inspired by these works, we report four novel D-A type HTMs as shown in Figure 1, using indenone spirofluorene as acceptor and arylamines as donors based on the following considerations: (1) the extension of conjugation area based on classic spiro core spiro-OMeTAD, which is better for property comparison; (2) nonplanar molecular configuration and large steric hindrance can effectively inhibit π−π aggregation and charge recombination between molecules, thereby promoting hole extraction from the perovskite layer (Pham et al., 2017; Zimmermann et al., 2017; Pham et al., 2018); (3) the rigid structural characteristics of the molecules have a relatively high glass transition temperature, favoring uniform thin film formation (Azmi et al., 2018; Jeon et al., 2018; Liu et al., 2018); (4) carbonyl group as a Lewis base can passivate Pb2+ defects on the surface of perovskite, reduce the activity, and improve its humidity stability (Zhang et al., 2018; Chen et al., 2019; Zou et al., 2019); (5) the N atom on the triarylamine has a strong electron donating ability, which is easily oxidized to generate a cationic radical, enabling a high hole mobility (Lin et al., 2003; Zhao et al., 2019). The novel HTMs were characterized by NMR, UV-Vis, and cyclic voltammetry spectroscopy. Steady-state photoluminescence (PL) was also employed to evaluate the hole extraction capability of the perovskite/HTMs interface.
Results and Discussion
The detailed synthetic routes for SFD-TPA, SFD-OMeTPA, SFD-TAD, and SFD-OMeTAD are shown in Scheme 1. 12H,12′H-10,10′-spirobi [indeno [2,1-b]fluorene] (2O-spiro) was used as starting material, which was synthesized according to our previous report (Xia et al., 2015). First, employing a bromine reagent, regioselective bromination at the α positions of 2O-spiro was attempted in C2H2Cl4. Tetrabrominated intermediate 2,2′,8,8′-tetrabromo-12H,12′H-10,10′-spirobi [indeno [2,1-b]fluorene] (3) could be obtained together with five-fold brominated by-products. The mixture was tough to be purified due to its poor solubility and similar polarity. An alternative synthesis strategy was developed. Using LiAlH4, 2O-spiro could be reduced to compound 1 in 72% yield. Thereafter, compounds 2 and 3 were prepared via bromination and oxidation reaction in yields of 15% and 47%, respectively. Finally, electron-donating group arylamines, as terminal moieties, were covalently bonded to 3 via four-fold Suzuki coupling reactions or Buchwald Hartwig cross-coupling reactions. SFD-TPA, SFD-OMeTPA, SFD-TAD, and SFD-OMeTAD were purified using silica gel column chromatography in yields of 40%, 42%, 38%, and 42%, respectively. The structures of the target molecules were fully characterized by 1H NMR, 13C NMR, and Maldi-TOF-Mass.
Optical properties. The spiro-OMeTAD dichloromethane solution is light yellow under sunlight, while the color of indenone spirofluorene cored products is deeper. UV-Vis absorption spectroscopy is used to study their optical properties in detail. As shown in Figure 2, these compounds display two bands in the range of 300–450 nm, which are assigned to n-π* transition and π−π* transition. Moreover, the bands in the range of 450–650 nm are observed obviously. This band is ascribed to the intramolecular charge transfer since there is no light absorption for spiro-OMeTAD and 2O-spiro (Xia et al., 2015). Furthermore, the UV-Vis absorption onset of diphenylamine moieties substituted indenone spirofluorene (SFD-TAD and SFD-OMeTAD) are in the longer wavelengths in comparison with triphenylamine substituted ones, indicating the direct linkage between 2O-spiro and N atoms favoring for effective charge transfer. The optical gaps of SFD-TPA, SFD-OMeTPA, SFD-TAD, and SFD-OMeTAD were calculated to be 2.22, 2.16, 1.97, and 1.87 eV, respectively, according to the formula, Eg = 1240/λonset.
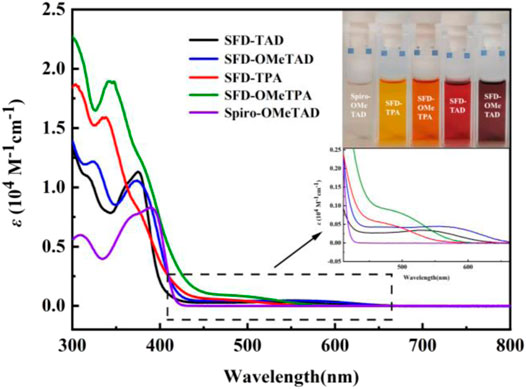
FIGURE 2. UV−vis absorption spectra and pictures of SFD-TPA, SFD-OMeTPA, SFD-TAD, and SFD-OMeTAD in CH2Cl2.
Electrochemical properties. The electrochemical properties of SFD-TPA, SFD-OMeTPA, SFD-TAD, and SFD-OMeTAD were investigated by cyclic voltammetry (CV) in CH2Cl2 at a scan rate of 100 mV s−1. As shown in Figure 3, the CV of these four compounds displays reversible oxidation and reduction waves. Their reduction potentials are similar to that of the core 2O-spiro (Xia et al., 2015), and thus their lowest unoccupied molecular orbital (LUMO) energy levels are in the range of −3.24 to −3.35 eV. In comparison with the highest occupied molecular orbital (HOMO) energy level of spiro-OMeTPA, HOMO energy levels of SFD-TPA, SFD-OMeTPA, SFD-TAD, and SFD-OMeTAD decrease obviously, which is arising from the electron-withdrawing capability of carbonyl group from 2O-spiro. Moreover, with the onset voltage of the first oxidation potentials, the HOMO levels of SFD-TPA, SFD-OMeTPA, SFD-TAD, and SFD-OMeTAD were calculated to be −5.22, −4.96, −5.12, and −5.01 eV, respectively, according to the formula, EHOMO = -[EOx+4.80-E(Fc/Fc+)], all data are exhibited in Table 1. On the basis of these results, it is predictable that methoxy groups favor the HOMO level enhancement. Consequently, the HOMO values for SFD-TPA, SFD-OMeTPA, and spiro-OMeTAD properly match with the valence band edge of the perovskite, leading to an effective hole extraction from the HTM, but also an efficient electron-blocking due to the high LUMO level. To further obtain a greater understanding of the geometric structure, electron distribution and frontier orbital energy levels of SFD-TPA, SFD-OMeTPA, SFD-TAD, and SFD-OMeTAD, density functional theory (DFT) calculations are carried out at the B3LYP/6-31G level. As shown in Supporting Information (Supplementary Figure S1), the electron density of LUMO distribution is mainly on the central core, while HOMO energy levels are almost delocalized across the whole molecule skeleton, which is similar to spiro-OMeTAD. The DSC curves of four target compounds are provided in Supplementary Figure S24.
To investigate the photoinduced charge transfer and charge separation between novel HTMs and perovskite, the photoluminescence (PL) quenching experiments were carried out (Figure 4). Compared with the bare perovskite film, when HTMs were introduced, the PL response of the pristine perovskite film was significantly quenched. This result indicates effective hole extraction and transport from perovskite to HTMs, and the quenching extent for four novel HTMs is at the same level of spiro-OMeTPA. Therefore, indenone spirofluorene cored molecules are proved to be promising novel D-A HTMs for PSCs. The novel D-A type hole-transporting materials with low cost for PSCs commercialization will be designed and synthesized in our laboratory.
Conclusion
In summary, we have successfully constructed four novel D-A type indenone spirofluorene cored HTMs. The appropriate introduction of carbonyl groups into spiro-OMeTAD can not only lead intramolecular charge transfer effect but also modulate the frontier orbital energy levels. SFD-OMeTAD presents the optical gap as low as 1.87 eV, which significantly decreases in comparison with that (2.96 eV) of the reported spiro-OMeTAD. For the first time, we achieved the modification of classical spiro-OMeTAD into D-A type HTMs for perovskite solar cells.
Data Availability Statement
The datasets presented in this study can be found in online repositories. The names of the repository/repositories and accession number(s) can be found in the article/Supplementary Material.
Author Contributions
SL, XY, HW, TY, KW, and WC synthesized and characterized these novel compounds. JG, SH, and DX provided the idea and prepared the manuscript. RF and YY gave valuable advice on this work.
Funding
This work was supported by the Natural Science Foundation of Heilongjiang Youth Fund (YQ 2021B002), College of Pharmacy, Harbin Medical University Excellent Young Talents Funding (2019-YQ-05), Heilongjiang, Postdoctoral Scientific Research Developmental Fund (LBH-Q21125, LBH-Q20018), and State Key Laboratory of Urban Water Resource and Environment (Harbin Institute of Technology).
Conflict of Interest
The authors declare that the research was conducted in the absence of any commercial or financial relationships that could be construed as a potential conflict of interest.
Publisher’s Note
All claims expressed in this article are solely those of the authors and do not necessarily represent those of their affiliated organizations, or those of the publisher, the editors, and the reviewers. Any product that may be evaluated in this article, or claim that may be made by its manufacturer, is not guaranteed or endorsed by the publisher.
Supplementary Material
The Supplementary Material for this article can be found online at: https://www.frontiersin.org/articles/10.3389/fchem.2022.898320/full#supplementary-material
References
Abuhelaiqa, M., Paek, S., Lee, Y., Cho, K. T., Heo, S., Oveisi, E., et al. (2019). Stable Perovskite Solar Cells Using Tin Acetylacetonate Based Electron Transporting Layers. Energy Environ. Sci. 12, 1910–1917. doi:10.1039/c9ee00453j
Azmi, R., Nam, S. Y., Sinaga, S., Akbar, Z. A., Lee, C.-L., Yoon, S. C., et al. (2018). High-performance Dopant-free Conjugated Small Molecule-Based Hole-Transport Materials for Perovskite Solar Cells. Nano Energy 44, 191–198. doi:10.1016/j.nanoen.2017.12.002
Bai, S., Da, P., Li, C., Wang, Z., Yuan, Z., Fu, F., et al. (2019). Planar Perovskite Solar Cells with Long-Term Stability Using Ionic Liquid Additives. Nature 571, 245–250. doi:10.1038/s41586-019-1357-2
Bi, D., Mishra, A., Gao, P., Franckevičius, M., Steck, C., Zakeeruddin, S. M., et al. (2016). High‐Efficiency Perovskite Solar Cells Employing a S , N ‐Heteropentacene‐based D-A Hole‐Transport Material. Chemsuschem 9, 433–438. doi:10.1002/cssc.201501510
Chen, W., Wang, Y., Pang, G., Koh, C. W., Djurisic, A. B., Wu, Y., et al. (2019). Conjugated Polymer–Assisted Grain Boundary Passivation for Efficient Inverted Planar Perovskite Solar Cells. Adv. Funct. Mater. 29, 1808855. doi:10.1002/adfm.201808855
Gangala, S., and Misra, R. (2018). Spiro-linked Organic Small Molecules as Hole-Transport Materials for Perovskite Solar Cells. J. Mat. Chem. A 6, 18750–18765. doi:10.1039/c8ta08503j
Ge, Q.-Q., Shao, J.-Y., Ding, J., Deng, L.-Y., Zhou, W.-K., Chen, Y.-X., et al. (2018). A Two-Dimensional Hole-Transporting Material for High-Performance Perovskite Solar Cells with 20 % Average Efficiency. Angew. Chem. Int. Ed. 57, 10959–10965. doi:10.1002/anie.201806392
Jeon, N. J., Na, H., Jung, E. H., Yang, T.-Y., Lee, Y. G., Kim, G., et al. (2018). A Fluorene-Terminated Hole-Transporting Material for Highly Efficient and Stable Perovskite Solar Cells. Nat. Energy 3, 682–689. doi:10.1038/s41560-018-0200-6
Jiang, A., Wang, F., Xia, D., Li, M., Qiang, L., Zhu, Z., et al. (2019). Aluminum Nanoparticles Manufactured Using a Ball-Milling Method with Ammonium Chloride as a Grinding Aid: Achieving Energy Release at Low Temperature. New J. Chem. 43, 1851–1856. doi:10.1039/c8nj05356a
Jiang, Q., Zhao, Y., Zhang, X., Yang, X., Chen, Y., Chu, Z., et al. (2019). Surface Passivation of Perovskite Film for Efficient Solar Cells. Nat. Photonics 13, 460–466. doi:10.1038/s41566-019-0398-2
Kim, G.-W., Kang, G., Kim, J., Lee, G.-Y., Kim, H. I., Pyeon, L., et al. (2016). Dopant-free Polymeric Hole Transport Materials for Highly Efficient and Stable Perovskite Solar Cells. Energy Environ. Sci. 9, 2326–2333. doi:10.1039/c6ee00709k
Lee, J.-W., Kim, H.-S., and Park, N.-G. (2016). Lewis Acid-Base Adduct Approach for High Efficiency Perovskite Solar Cells. Acc. Chem. Res. 49, 311–319. doi:10.1021/acs.accounts.5b00440
Li, M.-H., Hsu, C.-W., Shen, P.-S., Cheng, H.-M., Chi, Y., Chen, P., et al. (2015). Novel Spiro-Based Hole Transporting Materials for Efficient Perovskite Solar Cells. Chem. Commun. 51, 15518–15521. doi:10.1039/c5cc04405g
Li, X., Bi, D., Yi, C., Décoppet, J.-D., Luo, J., Zakeeruddin, S. M., et al. (2016). A Vacuum Flash-Assisted Solution Process for High-Efficiency Large-Area Perovskite Solar Cells. Science 353, 58–62. doi:10.1126/science.aaf8060
Li, Z., Zhu, Z., Chueh, C.-C., Jo, S. B., Luo, J., Jang, S.-H., et al. (2016). Rational Design of Dipolar Chromophore as an Efficient Dopant-free Hole-Transporting Material for Perovskite Solar Cells. J. Am. Chem. Soc. 138, 11833–11839. doi:10.1021/jacs.6b06291
Lin, B. C., Cheng, C. P., and Lao, Z. P. M. (2003). Reorganization Energies in the Transports of Holes and Electrons in Organic Amines in Organic Electroluminescence Studied by Density Functional Theory. J. Phys. Chem. A 107, 5241–5251. doi:10.1021/jp0304529
Liu, X.-Y., Tang, X., Zhao, D., Song, B., Ding, L., Fan, J., et al. (2018). A Series of Spirofluorene-Based Host Materials for Efficient Phosphorescent Organic Light-Emitting Diodes. Org. Electron. 61, 70–77. doi:10.1016/j.orgel.2018.06.046
Liu, Y., Hong, Z., Chen, Q., Chang, W., Zhou, H., Song, T.-B., et al. (2015). Integrated Perovskite/Bulk-Heterojunction toward Efficient Solar Cells. Nano Lett. 15, 662–668. doi:10.1021/nl504168q
Liu, Y., Hong, Z., Chen, Q., Chen, H., Chang, W.-H., Yang, Y. M., et al. (2016). Perovskite Solar Cells Employing Dopant-free Organic Hole Transport Materials with Tunable Energy Levels. Adv. Mat. 28, 440–446. doi:10.1002/adma.201504293
Ma, S., Zhang, H., Zhao, N., Cheng, Y., Wang, M., Shen, Y., et al. (2015). Spiro-thiophene Derivatives as Hole-Transport Materials for Perovskite Solar Cells. J. Mat. Chem. A 3, 12139–12144. doi:10.1039/c5ta01155h
Mai, C. L., Zhou, Q., Xiong, Q., Chen, C. C., Xu, J., Zhang, Z., et al. (2021). Donor-π-Acceptor Type Porphyrin Derivatives Assisted Defect Passivation for Efficient Hybrid Perovskite Solar Cells. Adv. Funct. Mat. 31, 2007762. doi:10.1002/adfm.202007762
Pham, H. D., Hu, H., Feron, K., Manzhos, S., Wang, H., Lam, Y. M., et al. (2017). Thienylvinylenethienyl and Naphthalene Core Substituted with Triphenylamines - Highly Efficient Hole Transporting Materials and Their Comparative Study for Inverted Perovskite Solar Cells. Sol. Rrl 1, 1700105. doi:10.1002/solr.201700105
Pham, H. D., Hu, H., Wong, F.-L., Lee, C.-S., Chen, W.-C., Feron, K., et al. (2018). Acene-based Organic Semiconductors for Organic Light-Emitting Diodes and Perovskite Solar Cells. J. Mater. Chem. C 6, 9017. doi:10.1039/C8TC01956H
Seo, J., Noh, J. H., and Seok, S. I. (2016). Rational Strategies for Efficient Perovskite Solar Cells. Acc. Chem. Res. 49, 562–572. doi:10.1021/acs.accounts.5b00444
Shang, R., Zhou, Z., Nishioka, H., Halim, H., Furukawa, S., Takei, I., et al. (2018). Disodium Benzodipyrrole Sulfonate as Neutral Hole-Transporting Materials for Perovskite Solar Cells. J. Am. Chem. Soc. 140, 5018–5022. doi:10.1021/jacs.8b01783
Steck, C., Franckevičius, M., Zakeeruddin, S. M., Mishra, A., Bäuerle, P., and Grätzel, M. (2015). A-D-A-type S,N-heteropentacene-based Hole Transport Materials for Dopant-free Perovskite Solar Cells. J. Mat. Chem. A 3, 17738–17746. doi:10.1039/c5ta03865k
Stranks, S. D., and Snaith, H. J. (2015). Metal-halide Perovskites for Photovoltaic and Light-Emitting Devices. Nat. Nanotech 10, 391–402. doi:10.1038/nnano.2015.90
Wang, X., Zhang, J., Yu, S., Yu, W., Fu, P., Liu, X., et al. (2018). Lowering Molecular Symmetry to Improve the Morphological Properties of the Hole-Transport Layer for Stable Perovskite Solar Cells. Angew. Chem. Int. Ed. 57, 12529–12533. doi:10.1002/anie.201807402
Wang, Y.-K., Yuan, Z.-C., Shi, G.-Z., Li, Y.-X., Li, Q., Hui, F., et al. (2016). Dopant-Free Spiro-Triphenylamine/Fluorene as Hole-Transporting Material for Perovskite Solar Cells with Enhanced Efficiency and Stability. Adv. Funct. Mat. 26, 1375–1381. doi:10.1002/adfm.201504245
Wang, Y., Chen, W., Wang, L., Tu, B., Chen, T., Liu, B., et al. (2019). Dopant-Free Small-Molecule Hole-Transporting Material for Inverted Perovskite Solar Cells with Efficiency Exceeding 21. Adv. Mater. 31, e1902781. doi:10.1002/adma.201902781
Xia, D., Gehrig, D., Guo, X., Baumgarten, M., Laquai, F., and Müllen, K. (2015). A Spiro-Bifluorene Based 3D Electron Acceptor with Dicyanovinylene Substitution for Solution-Processed Non-fullerene Organic Solar Cells. J. Mat. Chem. A 3, 11086–11092. doi:10.1039/c5ta00108k
Xu, B., Zhang, J., Hua, Y., Liu, P., Wang, L., Ruan, C., et al. (2017). Tailor-Making Low-Cost Spiro[fluorene-9,9′-xanthene]-Based 3D Oligomers for Perovskite Solar Cells. Chem 2, 676–687. doi:10.1016/j.chempr.2017.03.011
Xu, P., Liu, P., Li, Y., Xu, B., Kloo, L., Sun, L., et al. (2018). D-A-D-Typed Hole Transport Materials for Efficient Perovskite Solar Cells: Tuning Photovoltaic Properties via the Acceptor Group. ACS Appl. Mat. Interfaces 10, 19697–19703. doi:10.1021/acsami.8b04003
Yu, W., Yang, Q., Zhang, J., Tu, D., Wang, X., Liu, X., et al. (2019). Simple Is Best: A P-Phenylene Bridging Methoxydiphenylamine-Substituted Carbazole Hole Transporter for High-Performance Perovskite Solar Cells. ACS Appl. Mat. Interfaces 11, 30065–30071. doi:10.1021/acsami.9b06933
Zhang, M., Dai, S., Chandrabose, S., Chen, K., Liu, K., Qin, M., et al. (2018). High-Performance Fused Ring Electron Acceptor-Perovskite Hybrid. J. Am. Chem. Soc. 140, 14938–14944. doi:10.1021/jacs.8b09300
Zhang, Z., Liang, L., Deng, L., Ren, L., Zhao, N., Huang, J., et al. (2021). Fused Dithienopicenocarbazole Enabling High Mobility Dopant-free Hole-Transporting Polymers for Efficient and Stable Perovskite Solar Cells. ACS Appl. Mat. Interfaces 13, 6688–6698. doi:10.1021/acsami.0c21729
Zhao, L., Qiu, L., Xia, D., Liu, S., Yi, X., Fan, J., et al. (2019). Cyclooctatetrathiophene-Cored Three-Dimensional Hole Transport Material Enabling over 19% Efficiency of Perovskite Solar Cells. ACS Appl. Energy Mat. 2, 8173–8180. doi:10.1021/acsaem.9b01658
Zhou, W., Wen, Z., and Gao, P. (2018). Less Is More: Dopant-free Hole Transporting Materials for High-Efficiency Perovskite Solar Cells. Adv. Energy Mat. 8, 1702512. doi:10.1002/aenm.201702512
Zimmermann, I., Urieta-Mora, J., Gratia, P., Arago, J., Grancini, G., Molina-Ontoria, A., et al. (2017). High-Efficiency Perovskite Solar Cells Using Molecularly Engineered, Thiophene-Rich, Hole-Transporting Materials: Influence of Alkyl Chain Length on Power Conversion Efficiency. Adv. Energy Mater. 7, 1601674. doi:10.1002/aenm.201601674
Keywords: spirofluorene, spiro-OMeTAD, indenone, organic semiconductor, polycyclic aromatic hydrocarbons
Citation: Liu S, Yi X, Wang H, Ye T, Wang K, Cao W, Guan J, Fan R, Yang Y, Hao S and Xia D (2022) The Evolution of Classical Spiro-OMeTAD: Synthesis of Arylamine Endcapped Indenone Spirofluorene. Front. Chem. 10:898320. doi: 10.3389/fchem.2022.898320
Received: 17 March 2022; Accepted: 21 April 2022;
Published: 31 May 2022.
Edited by:
Guigen Li, Texas Tech University, United StatesReviewed by:
Ming Cheng, Jiangsu University, ChinaGuanghui An, Heilongjiang University, China
Guanzhao Wu, Qilu Hospital of Shandong University (Qingdao), China
Copyright © 2022 Liu, Yi, Wang, Ye, Wang, Cao, Guan, Fan, Yang, Hao and Xia. This is an open-access article distributed under the terms of the Creative Commons Attribution License (CC BY). The use, distribution or reproduction in other forums is permitted, provided the original author(s) and the copyright owner(s) are credited and that the original publication in this journal is cited, in accordance with accepted academic practice. No use, distribution or reproduction is permitted which does not comply with these terms.
*Correspondence: Jing Guan, Z3VhbmppbmdAZW1zLmhyYm11LmVkdS5jbg==; Sue Hao, aGFvc3VlQGhpdC5lZHUuY24=; Debin Xia, eGlhQGhpdC5lZHUuY24=
†These authors have contributed equally to this work