- 1School of Chemical Engineering and Light Industry, Guangdong University of Technology, Guangzhou, China
- 2PCFM Lab, School of Materials Science and Engineering, Sun Yat-sen University, Guangzhou, China
- 3Guangdong Provincial Key Laboratory of Chemical Measurement and Emergency Test Technology, Institute of Analysis, Guangdong Academy of Sciences (China National Analytical Center, Guangzhou), Guangzhou, China
- 4Guangdong Provincial Key Laboratory of Plant Resources Biorefinery, Guangzhou, China
- 5Guangdong Provincial Key Laboratory of Optical Chemicals, XinHuaYue Group, Maoming, China
To understand the unprecedented difference between 6-endo and 5-exo selectivity in hypervalent iodine (III) promoted fluorocyclization of unsaturated carboxylic acids or alcohols by difluoroiodotoluene, density functional theory (DFT) studies have been performed to systematically compare both the previous proposed “fluorination first and cyclization later” mechanism and the alternative “cyclization first and fluorination later” mechanism. Our results revealed that the selectivity is mechanism-dependent. The unsaturated alcohol prefers the fluorination first and the 6-endo-tet cyclization later pathway, leading to the experimentally observed 6-endo ether product. In contrast, the unsaturated carboxylic acid plausibly undergoes the 5-exo-trig cyclization first and the fluorination later to the experimentally observed 5-exo lactone product. The pKa property of the functional group of the substrate is found to play a key role in determining the reaction mechanism. The provided insights into the mechanism-dependent selectivity should help advance the development of fluorocyclization reactions with hypervalent iodine reagents.
Introduction
Owing to their unique physical and chemical properties, organofluorine compounds are widely applied in pharmaceuticals (Ma and Cahard, 2008), agrochemicals (Theodoridis, 2006), functional materials (Preshlock et al., 2016), and many other areas (O’Hagan and Deng, 2015; Yang et al., 2015; Zhou Y. et al., 2016). For example, approximately 30% of all agrochemicals and 20% of all pharmaceuticals contain fluorine atom(s) in their structures (Berger et al., 2011). Incorporating fluorine atom(s) into new compounds can improve molecular efficiency, permeability, lipophilicity, and biological activity (Zhu et al., 2018). However, there are few organofluorine compounds known in nature; therefore, developing new methods of synthesizing new organofluorine compounds and understanding their reaction mechanism would be critically important (Yoneda, 2004; Zhou et al., 2016a; Kohlhepp and Gulder, 2016; Fustero et al., 2018; Sreenithya and Sunoj, 2019; Han and Zhang, 2020; Saito, 2020; Wang et al., 2020; Zheng and Xue, 2020; Jalil et al., 2021).
In recently developed methods of synthesizing organofluorine compounds, hypervalent iodine reagents gained increasing attention (Yoshimura and Zhdankin, 2016). For example, Charpentier et al. (2015) have applied Togni reagents to introduce trifluoromethyl into alkenyls. Ilchenko et al. (2016) reported introducing fluorine atom(s) into alkenyls using fluoro-iodoxole (Mai et al., 2018). Sawaguchi et al. (2000) utilized difluoroiodotoluene to introduce fluorine atom(s) into unsaturated alcohols and unsaturated carboxylic acids, as shown in Scheme 1A. This interesting transformation resulted in unprecedented different selectivity (6-endo vs. 5-exo, Scheme 1A) between unsaturated alcohol and unsaturated carboxylic acid substrates. It was proposed that the hypervalent iodine reagent promotes the fluorination of the C=C double bond, and then the nucleophilic substitution furnishes the cyclization products in 6-endo or 5-exo selectivity, as shown in Scheme 1B (the “fluorination first and cyclization later” mechanism). It is desired to understand what is the origin behind this unprecedented diverse selectivity. Although it was suggested that the 6-endo product could be formed from the 5-exo intermediate via a cyclo-oxonium species, this kind of highly constrained oxonium may be suspected to be thermodynamically plausible. Interestingly, an alternative mechanism was also proposed, where the intramolecular cyclization occurs first by the nucleophilic attack of the functional group to the activated alkene, followed by fluorination later, as shown in Scheme 1C (the “cyclization first and fluorination later” mechanism) (Cui et al., 2014; Yang et al., 2015; Han et al., 2017; Kitamura et al., 2017; Farshadfar et al., 2020). In other similar reactions, the 6-endo vs. 5-exo phenomenon also attracted many interests (Liu et al., 2014; Kong et al., 2015; Zhao et al., 2017; Zhao et al., 2018; Riley et al., 2021). However, the origin of this selectivity is still ambiguous. Further insights are desired to clarify the understanding of the reaction mechanism, which should be system-dependent on not only the substrates but also the hypervalent iodine reagent itself or even the reaction conditions. Specifically, in the works by Sawaguchi et al. (2000) and Yoneda (2004), the same difluoro-iodoarenes reagents promoted diverse selective reactions in similar conditions, which should be an ideal model to study the difference in selectivity and understand its mechanistic origin. In our previous study (Shu et al., 2019), we have uncovered the mechanism that the hypervalent iodine reagent promoted fluorocyclization of unsaturated alcohols via double acid activation. Herein, we utilized DFT studies to clarify the mechanism-dependent selectivity of Hara and Yoneda’s system. We also investigated the role of the acid dissociation constant (pKa) of the functional group on the reaction mechanism.
Calculation Details
All calculations were carried out with the Gaussian 09 program (Frisch et al., 2013). Geometry optimizations and frequency calculations were carried out with B97D functional (Grimme, 2006; Lu et al., 2012), LANL2DZ containing the corresponding basis set augmented with d polarization and p diffuse functions for I atom (Couty and Hall, 1996), and the 6-31G (d, p) for the other atoms. Higher Level single-point energy calculations were carried out in the solvent of dichloromethane with the SMD model (Marenich et al., 2009), M06-2X functional was employed (Zhao and Truhlar, 2008; Walker et al., 2013), SDD basis (Andrae et al., 1990) for iodine atom, and 6-311++G (d, p) for other atoms. The NBO analysis was carried out with M06-2X functional, the SDD model for iodine, and 6-311++G (d,p) for other atoms; the solvent is dichloromethane and with the SMD calculated model. Considering the entropic contribution is overestimated due to the ignorance of the suppression effect of solvent on the translational and rotational freedoms of the reactants, the MHP scheme proposed by Martin et al. (1998) is adopted. All the pictures of structures were generated by CYLview (Legault, 2009).
Results and Discussion
On the basis of the aforementioned mechanistic understanding, the hypervalent iodine promoted fluorocyclization generally includes three stages: the activation of alkene, fluorination, and cyclization. DFT calculations have been performed to provide insights to understand the selectivity and the origin of the reaction by comprehensively comparing the mechanistic difference in “fluorination first and cyclization later” and the “cyclization first and fluorination later” (Scheme 1). The free energy profiles for the fluorocyclization of unsaturated carboxylic acid via the “fluorination first and cyclization later” mechanism or the “cyclization first and fluorination later” mechanism are depicted in Figures 1, 2, respectively. The results of the fluorocyclization of unsaturated alcohol through the “fluorination first and cyclization later” mechanism or the “cyclization first and fluorination later” mechanism are shown in Figures 3, 4, respectively.
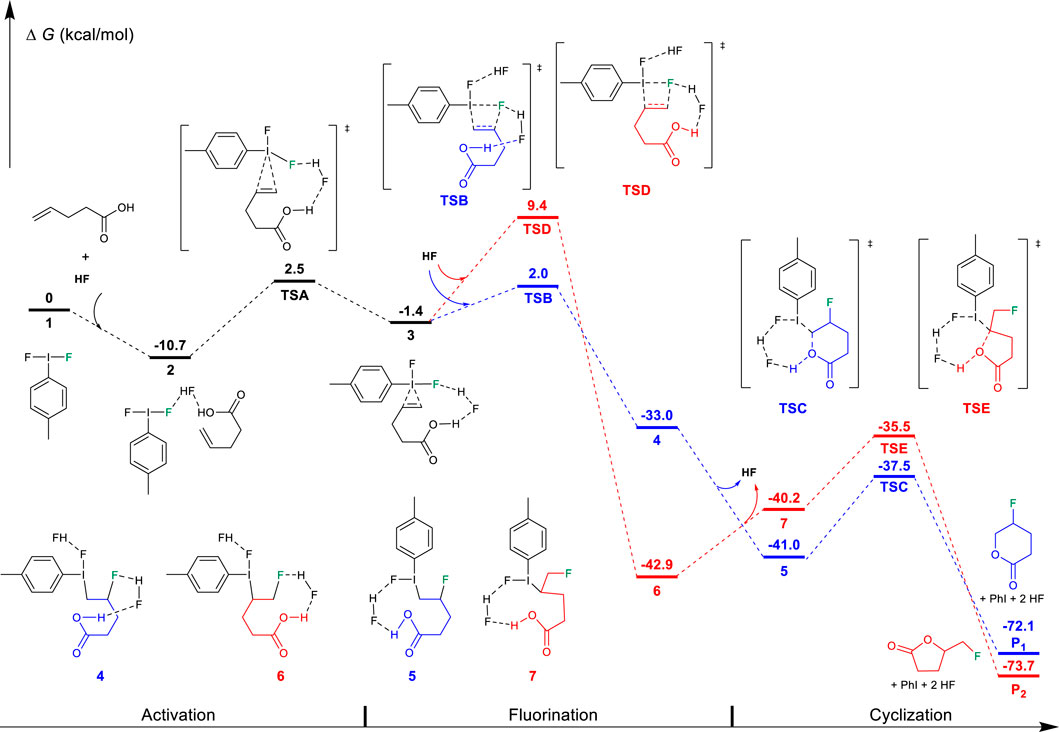
FIGURE 1. Free-energy profiles for the fluorocyclization of unsaturated carboxylic acid via the “fluorination first and cyclization later” mechanism. The preferred pathway is shown in blue.
“Fluorination First and Cyclization Later” Mechanism
According to previous studies (Shu et al., 2019), this hypervalent iodine-promoted fluorocyclization reaction preferred a metathesis fluorination mechanism via activated iodine (III)-π intermediate in which the Bronsted acid double-activation mode plays an important role due to the use of excess amine·HF. The formed iodine (III) iranium intermediate was predicted to be much higher in free energy (Shu et al., 2019). Therefore, in the “fluorination first and cyclization later” mechanism, the unsaturated carboxylic alcohol is first activated by the difluoroiodotoluene via TSA, producing an iodine (III)-π intermediate 3, as shown in Figure 1. In intermediate 3, the I-C bond lengths between iodine and alkene are calculated to be 2.699/2.895 Å, indicating a typical iodine (III)-π activation instead of an iodine (III) iranium activation (Zhou et al., 2016b; Zhang et al., 2017; Shu et al., 2019). The free energy barrier of the activation step is 13.2 kcal/mol.
After the activation, with the assistance of another HF as the Brønsted acid, the fluorination of the C=C double undergoes the metathesis mechanism via transition state TSB or TSD. TSB leads to the Markovnikov product 4, while TSD results in the anti-Markovnikov product 6. TSB (2.0 kcal/mol) is preferred over TSD (9.4 kcal/mol), which is in good agreement with Markovnikov’s rule. Both transition states have similar four-membered ring structures, where the I-C, I-F, C=C, and C-F bond lengths are calculated to be 2.514/2.575/1.400/2.342 Å in TSB and 2.611/2.536/1.397/2.227 Å in TSD, respectively. It can be found that the interaction between hypervalent iodine and alkene is stronger in TSB with longer I-F and C=C bond lengths, leading to a stronger I-C interaction (2.514 Å). However, the free energy preferred transition state TSB leads to the final 6-endo-tet annulation product P1 via the transition state TSC (−37.5 kcal/mol). This is a kinetically and thermodynamically facile step (ΔG⧧ = 3.5 kcal/mol); however, the predicted selectivity of the product is in sharp contrast to the experimental observation of a 5-exo product for the unsaturated carboxylic acid substrates. TSE has a relatively high ring constraint as compared to TSC due to the role of lactone in the five-membered ring transition state. Out of expectation, the annulation (6→7→TSE→P2) leads to the experimentally observed 5-exo product P2, which is predicted by the DFT results to be unfavored along the “fluorination first and cyclization later” mechanism (TSD, 9.4 kcal/mol). This strongly indicates that the “fluorination first and cyclization later” mechanism cannot explain the selectivity of the unsaturated carboxylic acid in difluoroiodotoluene-promoted fluorocyclization.
On the contrary, for unsaturated alcohols, this “fluorination first and cyclization later” mechanism can explain the experimental phenomenon well. As shown in Figure 2, the activation of the unsaturated alcohol also leads to an iodine (III)-π intermediate 3′ (Zhou et al., 2016a; Zhang et al., 2017; Shu et al., 2019). The located 3′ structure has a very similar I···alkene interaction (2.685/2.871 Å) to that in structure 3. After the activation, the fluorination of the C=C bond of the unsaturated alcohol also prefers the Markovnikov transition state TSB′ (12.0 kcal/mol) in comparison to the higher anti-Markovnikov transition state TSD′ (24.1 kcal/mol). The Markovnikov product 4′ results in the 6-endo-tet annulation P1′ as the final product, which agrees with the experimental observation of the 6-endo product well, indicating that the difluoroiodotoluene-promoted fluorocyclization of unsaturated alcohol plausibly operates the “fluorination first and cyclization later” mechanism.
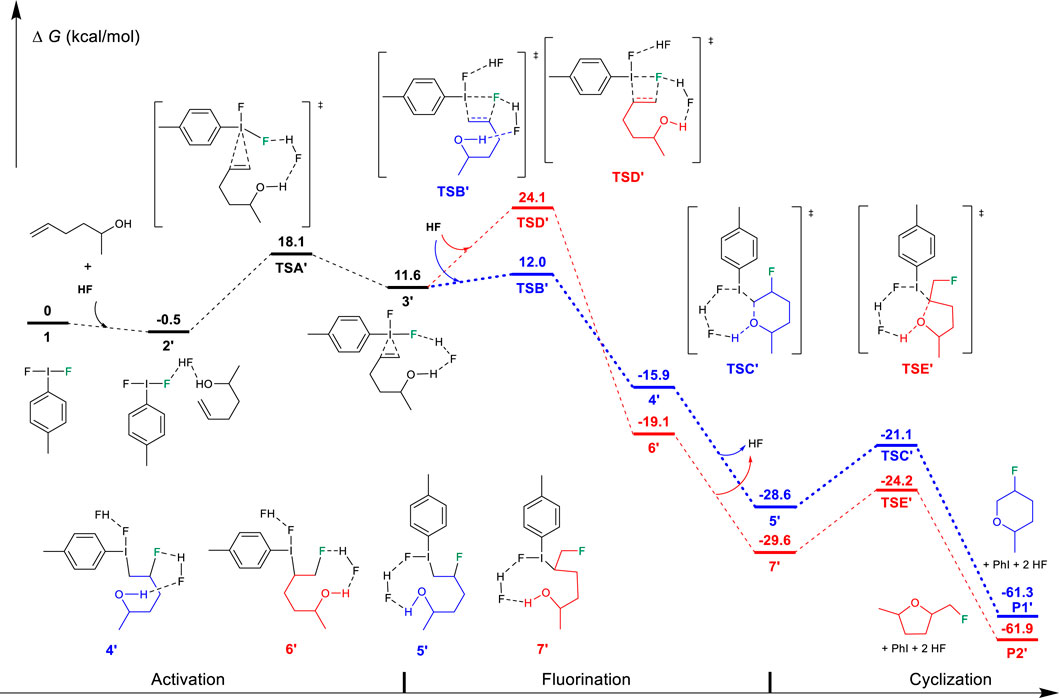
FIGURE 2. Free-energy profiles for the fluorocyclization of unsaturated alcohol via the “fluorination first and cyclization later” mechanism. The preferred pathway is shown in blue.
“Cyclization First and Fluorination Later” Mechanism
Alternative to the “fluorination first and cyclization later” mechanism, we have considered the possibility of nucleophilic addition of the oxygen atom of the functional group to the hypervalent iodine activated C=C double bond, followed by fluorination. The calculation results are shown in Figure 3 and Figure 4. The first step is also the activation process by the hypervalent iodine reagent to form the iodine (III)-π unsaturated carboxylic acid intermediate 3. After the activation, with the assistance of another HF molecule as the Brønsted acid, the carboxylate group nucleophilically attacks the C=C double bond to furnish the cyclization, accompanied by the dissociation of the proton, as shown in Figure 3. This cyclization step may also proceed via the Markovnikov transition state TSF (−0.4 kcal/mol) to the 5-exo-trig product 8 (−33.7 kcal/mol) or via the anti-Markovnikov transition state TSH (18.2 kcal/mol) to the 6-endo-trig product 10 (−27.3 kcal/mol). In addition to the Markovnikov rule, the high constraint of the [6.3.1] bicyclic structure of the transition state TSH in comparison to the 5,8-fused bicyclic structure of transition state TSF should mainly contribute to the higher free energy of TSH. Specifically, the cyclization transition state TSF (−0.4 kcal/mol) is lower in free energy than in the corresponding alkene fluorination transition state TSB (2.0 kcal/mol, Figure 1), indicating that the carboxylate oxygenation of the alkene is preferred over fluorination in Hara and Yoneda’s system using excess HF. After cyclization, the fluorination process occurs with the assistance of two bridging HF molecules. The fluorination transition state TSG (−42.1 kcal/mol) leads to the 5-exo-trig product P2, while the transition state TSI (−45.2 kcal/mol) produces the 6-endo product P1. Although TSG is slightly higher than TSI because a five-membered ring lactone causes more steric hindrance than the five-membered ring lactone, the reaction much preferred the Markovnikov transition state TSF (−0.4 kcal/mol) to the 5-exo product, which can explain the experimental results well. In sharp contrast to the results of the “fluorination and cyclization later” mechanism, these results strongly suggested that the difluoroiodotoluene-promoted fluorocyclization of unsaturated carboxylic acid should plausibly undergo the “cyclization first and fluorination later” mechanism to the 5-exo product. Notably, DFT results predict a lower cyclization transition state TSF (−0.4 kcal/mol, Figure 3) than that of the alkene fluorination transition state TSB (2.0 kcal/mol, Figure 1), further supporting the preference of the “cyclization first and fluorination later” mechanism for unsaturated carboxylic acid substrates.
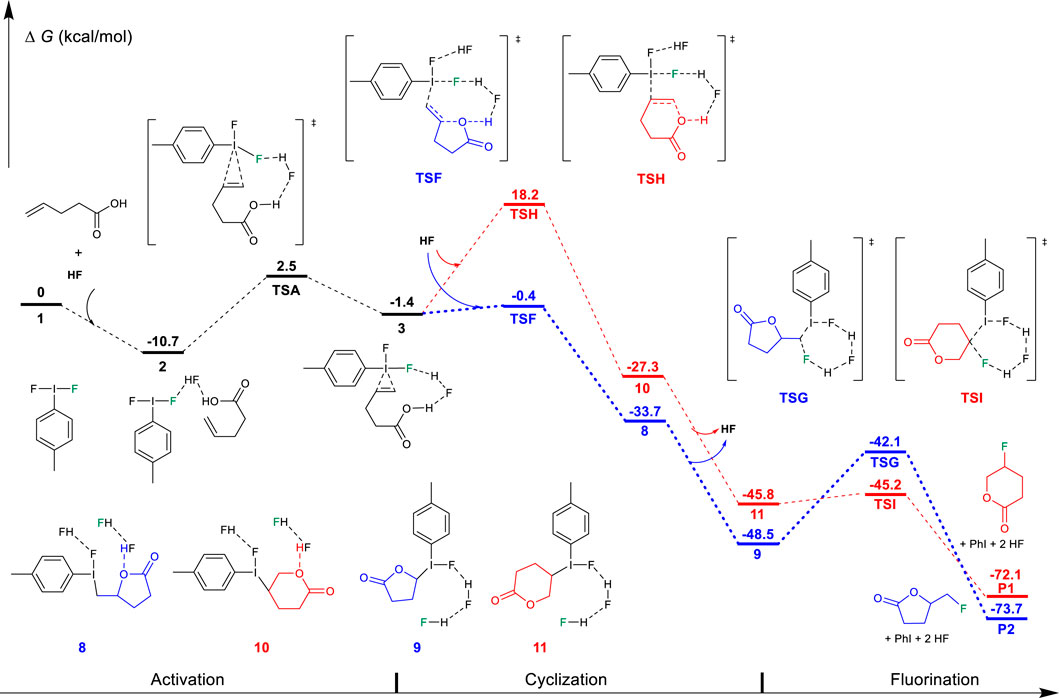
FIGURE 3. Free-energy profiles for the fluorocyclization of unsaturated carboxylic acid via the “cyclization first and fluorination later” mechanism. The preferred pathway is shown in blue.
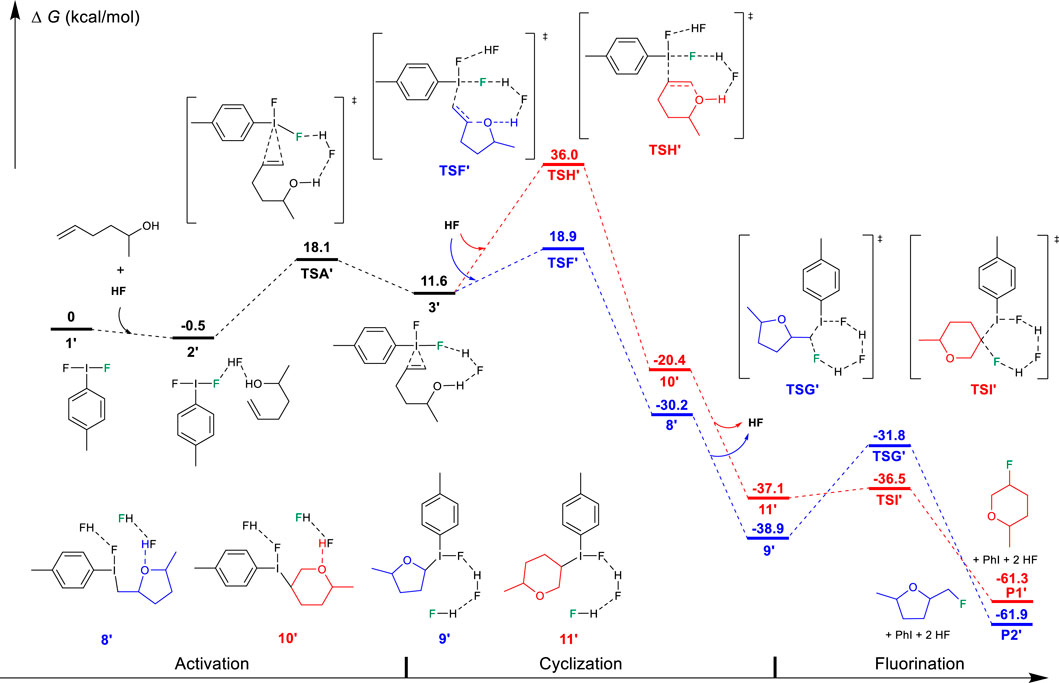
FIGURE 4. Free-energy profiles for the fluorocyclization of unsaturated alcohol via the “cyclization first and fluorination later” mechanism. The preferred pathway is shown in blue.
On the other hand, DFT results suggest that after the activation of the unsaturated alcohol (3′ in Figure 4), the hydroxyl-initiated cyclization has to overcome a much higher free energy barrier, either via a Markovnikov transition state TSF′ (18.9 kcal/mol) or via an anti-Markovnikov transition state TSH′ (36.0 kcal/mol). In comparison to the relatively lower transition states (TSB′, 12.0 kcal/mol; TSD′, 24.1 kcal/mol) for the fluorination of the C=C bond in the “cyclization first and fluorination later” mechanism (Figure 2), the unsaturated alcohol is essentially different in the reaction mechanism from the unsaturated carboxylic acid in the difluoroiodotoluene-promoted fluorocyclization and the selectivity of the substrates is actually mechanism-dependent, as summarized in Scheme 2.
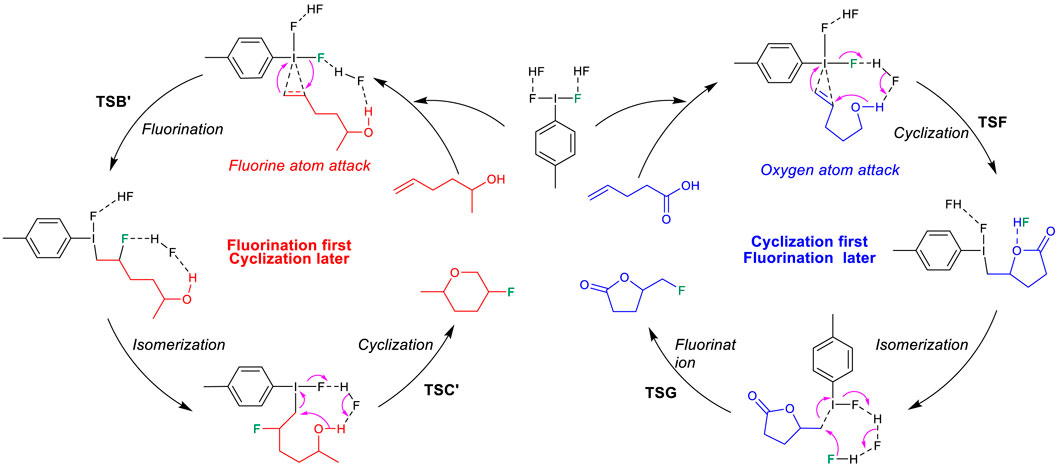
SCHEME 2. Mechanism-dependent selectivity in difluoroiodotoluene-promoted fluorocyclization of unsaturated alcohols and unsaturated carboxylic acids.
Origin of the Mechanism-Dependent Selectivity
To compare the mechanism-dependent selectivity in difluoroiodotoluene-promoted fluorocyclization of unsaturated alcohols and carboxylic acids, the key transition states involved in each mechanism are depicted in Figure 5. In the cyclization first mechanism, the cyclization transition state for unsaturated carboxylic acid (TSF, −0.4 kcal/mol) is much lower than that for unsaturated alcohol (TSF, −0.4 kcal/mol). NBO analysis indicates that the nucleophilic oxygen atom of carboxylic acid carries −0.856 NPA negative charge in TSF, while the nucleophilic oxygen atom of alcohol only has an NPA charge of −0.779 in TSF′. The stronger nucleophilic oxygen atom of carboxylic acid can also be well reflected by the degree of the deprotonation in TSF, in which the O…H distance is calculated to be 1.305 Å. In contrast, the alcohol is less deprotonated in TSF′ with the O-H bond lonely elongated to 1.014 Å. As a consequence, the I-F bond is highly dissociated (2.980 Å) in TSF, and the C=C bond has been highly activated by the hypervalent iodine during the cyclization (C-I bond, 2.489 Å; C=C bond, 1.403 Å). In the case of alcohol, the I-F bond is less dissociated (2.363 Å) in TSF′, and the C=C bond is less activated by the hypervalent iodine during the cyclization (C-I bond, 2.514 Å; C=C bond, 1.410 Å).
On the other hand, in the fluorination first mechanism, the NPA charge of the attacking fluorine atom is lower in TSB (−0.766, carboxylic acid) than in TSB′ (−0.765, alcohol), and the I-F bond is also more dissociated (2.575 Å, carboxylic acid) in TSB than in TSB′ (2.470 Å, alcohol). The difference in TSB and TSB′ can be attributed to the stronger Bronsted acid of carboxylic acid than alcohol in the double acid activation mode (Shu et al., 2019). Due to the more important role of the functional group difference in nucleophilicity for the cyclization first mechanism, the unsaturated carboxylic acid substrates prefer the “fluorination first and cyclization later” mechanism, whereas the unsaturated alcohol substrates would like to operate the “cyclization first and fluorination later” mechanism. The NPA charge and the deprotonation trend of the functional group strongly suggest that the pKa property of the substrate should play a crucial role in the preference of the reaction mechanism.
We have designed a series of substrates with different pKa values (Yang et al., 2020) to evaluate the free energy barriers of the 5-exo-trig cyclization. Figure 6 shows the structures of the studied substrates and the method by which the 5-exo-trig cyclization free energy barriers correlate with the pKa value of the reactants. In a general sense, the lower the pKa value of the reactants, the easier the deprotonation of the O-H bond. The carboxylic acid has the smallest pKa value corresponding to the most facile 5-exo-trig cyclization. On the other hand, unsaturated alcohols undergo the “fluorination first and cyclization later” mechanism with a larger pKa value. If we modified the alcohol to phenol with a lower pKa value, the tendency of the 5-exo-trig cyclization would become more favored. Specifically, phenol with an electronic-withdrawing substituent, such as nitro, will have an even lower free energy barrier for the 5-exo-trig cyclization. Although the studied reaction is not a stereoselective transformation, it can be expected that the property of the functional group would change the mechanism, highlighting its potential role in influencing the stereoselectivity.
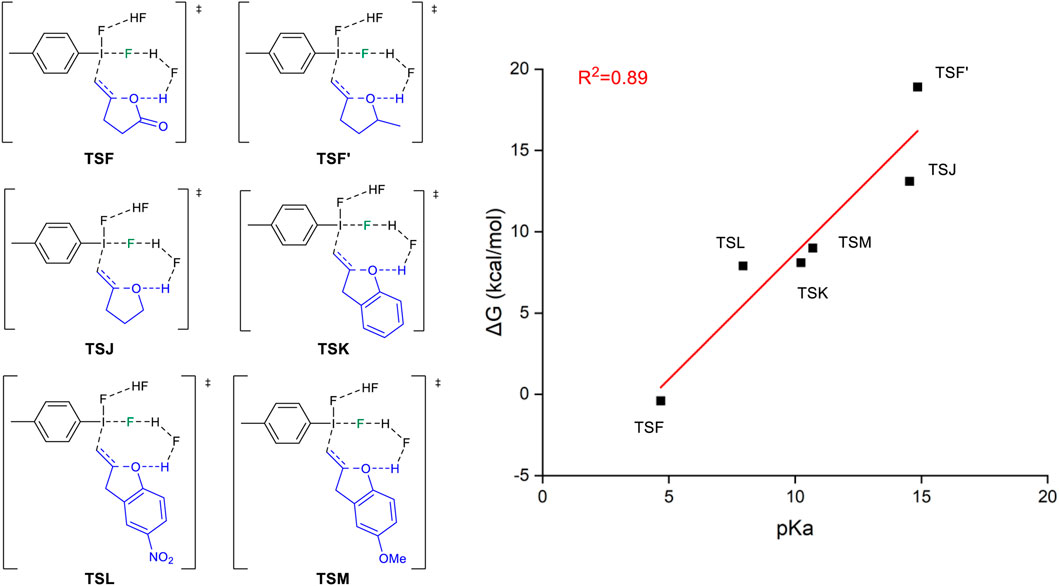
FIGURE 6. Relationship between 5-exo-tet cyclized energy barriers of selected reactants with different pKa values.
Conclusion
DFT studies have been performed to understand the unprecedented different selectivity (6-endo vs. 5-exo) between unsaturated alcohol and unsaturated carboxylic acid substrates in hypervalent difluoroiodotoluene-promoted fluorocyclization reactions. Both the previously proposed “fluorination first and cyclization later” mechanism and the alternative “cyclization first and fluorination later” mechanism were compared. The unsaturated alcohol prefers the “fluorination first and cyclization later” mechanism, in which the alkene activated by hypervalent iodine will be fluorinated first with a calculated free energy of 12.0 kcal/mol for the transition state (TSB′), followed by the 6-endo-tet cyclization to furnish the experimentally observed six-membered ring ether product. In contrast, the “cyclization first and fluorination later” mechanism is less plausible for unsaturated alcohol because it has to overcome a higher 5-exo-trig cyclization transition state (TSF′, 18.9 kcal/mol), leading to the wrong product, five-membered ring ether, after fluorination in the later stage. In contrast, the unsaturated carboxylic acid operated the “cyclization first and fluorination later” mechanism instead of the previously proposed “fluorination first and cyclization later” mechanism. After activation by hypervalent iodine, unsaturated carboxylic acid undergoes a facile 5-exo-trig cyclization (TSF, −0.4 kcal/mol), followed by subsequent fluorination to produce the experimentally observed five-membered ring lactone product exactly. In contrast, in the “fluorination first and cyclization later” mechanism, the unsaturated carboxylic acid has to overcome a higher fluorination transition state (TSB, 2.0 kcal/mol) and then cyclizes to the wrong product, the 6-endo-tet lactone. The origin of the mechanism-dependent selectivity was analyzed, revealing that the pKa value of the functional group plays a crucial role in the origin of the mechanistic variation. The pKa property affects the NPA charge and the deprotonation trend of the functional group, thus influencing the trend of the first cyclization step. The presented mechanistic understanding could be helpful to the design and application of hypervalent iodine reagents in fluorination reactions.
Data Availability Statement
The Cartesian coordinates of computational structures and figures can be found in online Supplementary Material.
Author Contributions
All authors listed have made a substantial, direct, and intellectual contribution to the work and approved it for publication.
Funding
This work was supported by the NSFC (21977019, 21973113) and the Guangdong Natural Science Funds for Distinguished Young Scholar (No. 2015A030306027).
Conflict of Interest
The authors declare that the research was conducted in the absence of any commercial or financial relationships that could be construed as a potential conflict of interest.
Publisher’s Note
All claims expressed in this article are solely those of the authors and do not necessarily represent those of their affiliated organizations, or those of the publisher, the editors, and the reviewers. Any product that may be evaluated in this article, or claim that may be made by its manufacturer, is not guaranteed or endorsed by the publisher.
Supplementary Material
The Supplementary Material for this article can be found online at: https://www.frontiersin.org/articles/10.3389/fchem.2022.897828/full#supplementary-material
References
Andrae, D., HauBermann, U., Dolg, M., Stoll, H., and PreuB, H. (1990). Energy-adjustedab Initio Pseudopotentials for the Second and Third Row Transition Elements. Theoret. Chim. Acta 77 (2), 123–141. doi:10.1007/BF01114537
Berger, R., Resnati, G., Metrangolo, P., Weber, E., and Hulliger, J. (2011). Organic Fluorine Compounds: a Great Opportunity for Enhanced Materials Properties. Chem. Soc. Rev. 40 (7), 3496–3508. doi:10.1039/c0cs00221f
Charpentier, J., Früh, N., and Togni, A. (2015). Electrophilic Trifluoromethylation by Use of Hypervalent Iodine Reagents. Chem. Rev. 115 (2), 650–682. doi:10.1021/cr500223h
Couty, M., and Hall, M. B. (1996). Basis Sets for Transition Metals: Optimized Outerp Functions. J. Comput. Chem. 17 (11), 1359–1370. doi:10.1002/(SICI)1096-987X
Cui, J., Jia, Q., Feng, R.-Z., Liu, S.-S., He, T., and Zhang, C. (2014). Boron Trifluoride Etherate Functioning as a Fluorine Source in an Iodosobenzene-Mediated Intramolecular Aminofluorination of Homoallylic Amines. Org. Lett. 16 (5), 1442–1445. doi:10.1021/ol500238k
Farshadfar, K., Abdolalian, P., and Ariafard, A. (2020). Catalytic Role of Lewis Acids in ArIO‐Mediated Oxidative Fluorination Reactions Revealed by DFT Calculations. Eur. J. Org. Chem. 2020 (15), 2251–2259. doi:10.1002/ejoc.202000217
Frisch, M. J., Trucks, G. W., Schlegel, H. B., Scuseria, G. E., Robb, M. A., Cheeseman, J. R., et al. (2013). Gaussian 09". Revision D.01. Wallingford CT: Gaussian, Inc.
Fustero, S., Sedgwick, D. M., Román, R., and Barrio, P. (2018). Recent Advances in the Synthesis of Functionalised Monofluorinated Compounds. Chem. Commun. 54 (70), 9706–9725. doi:10.1039/C8CC05181J
Grimme, S. (2006). Semiempirical GGA-type Density Functional Constructed with a Long-Range Dispersion Correction. J. Comput. Chem. 27 (15), 1787–1799. doi:10.1002/jcc.20495
Han, Y.-C., Zhang, Y.-D., Jia, Q., Cui, J., and Zhang, C. (2017). Hypervalent-Iodine-Mediated Ring-Contraction Monofluorination Affording Monofluorinated Five-Membered Ring-Fused Oxazolines. Org. Lett. 19 (19), 5300–5303. doi:10.1021/acs.orglett.7b02479
Han, Z. Z., and Zhang, C. P. (2020). Fluorination and Fluoroalkylation Reactions Mediated by Hypervalent Iodine Reagents. Adv. Synth. Catal. 362 (20), 4256–4292. doi:10.1002/adsc.202000750
Ilchenko, N. O., Cortés, M. A., and Szabó, K. J. (2016). Palladium-Catalyzed Iodofluorination of Alkenes Using Fluoro-Iodoxole Reagent. ACS Catal. 6 (1), 447–450. doi:10.1021/acscatal.5b02022
Jalil, A., Yang, Y. O., Chen, Z., Jia, R., Bi, T., Li, X., et al. (2021). Formation of Carbon-Oxygen Bond Mediated by Hypervalent Iodine Reagents under Metal-free Conditions. Mroc 18 (5), 540–605. doi:10.2174/1570193X17999200807140943
Kitamura, T., Miyake, A., Muta, K., and Oyamada, A. J. (2017). Hypervalent Iodine/HF Reagents for the Synthesis of 3-Fluoropyrrolidines. J. Org. Chem. 82 (22), 11721–11726. doi:10.1021/acs.joc.7b01266
Kohlhepp, S. V., and Gulder, T. (2016). Hypervalent Iodine(iii) Fluorinations of Alkenes and Diazo Compounds: New Opportunities in Fluorination Chemistry. Chem. Soc. Rev. 45 (22), 6270–6288. doi:10.1039/C6CS00361C
Kong, W., Guo, Q., Xu, Z., Wang, G., Jiang, X., and Wang, R. (2015). Iodine(III)-Mediated Oxy-Fluorination of Alkenyl Oximes: An Easy Path to Monofluoromethyl-Substituted Isoxazolines. Org. Lett. 17 (15), 3686–3689. doi:10.1021/acs.orglett.5b01646
Legault, C. Y. (2009). CYLview, 1.0b. Université de Sherbrooke. Available at: http://www.cylview.org (Accessed June 30, 2019).
Liu, Y.-Y., Yang, J., Song, R.-J., and Li, J.-H. (2014). Synthesis of 5-(Fluoromethyl)-4,5-Dihydroisoxazoles by Silver- Catalyzed Oxyfluorination of Unactivated Alkenes. Adv. Synth. Catal. 356 (14-15), 2913–2918. doi:10.1002/adsc.201400242
Lu, T., Porterfield, M. A., and Wheeler, S. E. (2012). Explaining the Disparate Stereoselectivities of N-Oxide Catalyzed Allylations and Propargylations of Aldehydes. Org. Lett. 14 (20), 5310–5313. doi:10.1021/ol302493d
Ma, J.-A., and Cahard, D. (2008). Update 1 of: Asymmetric Fluorination, Trifluoromethylation, and Perfluoroalkylation Reactions. Chem. Rev. 108 (9), PR1–PR43. doi:10.1021/cr800221v
Mai, B. K., Szabó, K. J., and Himo, F. (2018). Mechanisms of Rh-Catalyzed Oxyfluorination and Oxytrifluoromethylation of Diazocarbonyl Compounds with Hypervalent Fluoroiodine. ACS Catal. 8 (5), 4483–4492. doi:10.1021/acscatal.8b00667
Marenich, A. V., Cramer, C. J., and Truhlar, D. G. (2009). Universal Solvation Model Based on Solute Electron Density and on a Continuum Model of the Solvent Defined by the Bulk Dielectric Constant and Atomic Surface Tensions. J. Phys. Chem. B 113 (18), 6378–6396. doi:10.1021/jp810292n
Martin, R. L., Hay, P. J., and Pratt, L. R. (1998). Hydrolysis of Ferric Ion in Water and Conformational Equilibrium. J. Phys. Chem. A. 102 (20), 3565–3573. doi:10.1021/jp980229p
O’Hagan, D., and Deng, H. (2015). Enzymatic Fluorination and Biotechnological Developments of the Fluorinase. Chem. Rev. 115 (2), 634–649. doi:10.1021/cr500209t
Preshlock, S., Tredwell, M., and Gouverneur, V. (2016). 18F-Labeling of Arenes and Heteroarenes for Applications in Positron Emission TomographyF-Labeling of Arenes and Heteroarenes for Applications in Positron Emission Tomography. Chem. Rev. 116 (2), 719–766. doi:10.1021/acs.chemrev.5b00493
Riley, W., Jones, A. C., Singh, K., Browne, D. L., and Stuart, A. M. (2021). Accessing Novel Fluorinated Heterocycles with the Hypervalent Fluoroiodane Reagent by Solution and Mechanochemical Synthesis. Chem. Commun. 57 (60), 7406–7409. doi:10.1039/D1CC02587B
Saito, A. (2020). Hypervalent Iodine-mediated/Catalyzed Oxidative Cycloisomerization/Annulation of Alkynes for Metal-free Synthesis of Oxazoles. Coc 24 (18), 2048–2069. doi:10.2174/1385272824999200510232438
Sawaguchi, M., Hara, S., Fukuhara, T., and Yoneda, N. (2000). Fluorocyclization of Unsaturated Alcohols and Carboxylic Acids by Iodotoluene Difluoride and Amine-HF Complexes. J. Fluorine Chem. 104 (2), 277–280. doi:10.1016/S0022-1139(00)00241-4
Shu, S., Li, Y., Jiang, J., Ke, Z., and Liu, Y. (2019). Mechanism of Hypervalent Iodine Promoted Fluorocyclization of Unsaturated Alcohols: Metathesis via Double Acids Activation. J. Org. Chem. 84 (1), 458–462. doi:10.1021/acs.joc.8b02741
Sreenithya, A., and Sunoj, R. B. (2019). On the Activation of Hypercoordinate Iodine(iii) Compounds for Reactions of Current Interest. Dalton Trans. 48 (13), 4086–4093. doi:10.1039/C9DT00472F
Theodoridis, G. (2006). “Chapter 4 Fluorine-Containing Agrochemicals: An Overview of Recent Developments,” in Advances in Fluorine Science. Editor A. Tressaud (Amsterdam: Elsevier), 121–175. doi:10.1016/s1872-0358(06)02004-5
Walker, M., Harvey, A. J. A., Sen, A., and Dessent, C. E. H. (2013). Performance of M06, M06-2X, and M06-HF Density Functionals for Conformationally Flexible Anionic Clusters: M06 Functionals Perform Better Than B3LYP for a Model System with Dispersion and Ionic Hydrogen-Bonding Interactions. J. Phys. Chem. A. 117 (47), 12590–12600. doi:10.1021/jp408166m
Wang, Q., Lübcke, M., Biosca, M., Hedberg, M., Eriksson, L., Himo, F., et al. (2020). Enantioselective Construction of Tertiary Fluoride Stereocenters by Organocatalytic Fluorocyclization. J. Am. Chem. Soc. 142 (47), 20048–20057. doi:10.1021/jacs.0c09323
Yang, Q., Li, Y., Yang, J. D., Liu, Y., Zhang, L., Luo, S., et al. (2020). Holistic Prediction of the P K a in Diverse Solvents Based on a Machine‐Learning Approach. Angew. Chem. Int. Ed. 59 (43), 19282–19291. doi:10.1002/anie.202008528
Yang, X., Wu, T., Phipps, R. J., and Toste, F. D. (2015). Advances in Catalytic Enantioselective Fluorination, Mono-, Di-, and Trifluoromethylation, and Trifluoromethylthiolation Reactions. Chem. Rev. 115 (2), 826–870. doi:10.1021/cr500277b
Yoneda, N. (2004). Advances in the Preparation of Organofluorine Compounds Involving Iodine And/or Iodo-Compounds. J. Fluorine Chem. 125 (1), 7–17. doi:10.1016/S0022-1139(03)00159-3
Yoshimura, A., and Zhdankin, V. V. (2016). Advances in Synthetic Applications of Hypervalent Iodine Compounds. Chem. Rev. 116 (5), 3328–3435. doi:10.1021/acs.chemrev.5b00547
Zhang, J., Szabó, K. J., and Himo, F. (2017). Metathesis Mechanism of Zinc-Catalyzed Fluorination of Alkenes with Hypervalent Fluoroiodine. ACS Catal. 7 (2), 1093–1100. doi:10.1021/acscatal.6b02731
Zhao, J., Jiang, M., and Liu, J.-T. (2017). Synthesis of Fluoromethyl-Substituted Isoxazolines via Transition Metal-free Oxyfluorination of Alkenyl Oximes. Adv. Synth. Catal. 359 (10), 1626–1630. doi:10.1002/adsc.201601405
Zhao, J., Jiang, M., and Liu, J.-T. (2018). Transition Metal-free Aminofluorination of β,γ-unsaturated Hydrazones: Base-Controlled Regioselective Synthesis of Fluorinated Dihydropyrazole and Tetrahydropyridazine Derivatives. Org. Chem. Front. 5 (7), 1155–1159. doi:10.1039/C7QO01105A
Zhao, Y., and Truhlar, D. G. (2008). The M06 Suite of Density Functionals for Main Group Thermochemistry, Thermochemical Kinetics, Noncovalent Interactions, Excited States, and Transition Elements: Two New Functionals and Systematic Testing of Four M06-Class Functionals and 12 Other Functionals. Theor. Chem. Account. 120 (1), 215–241. doi:10.1007/s00214-007-0310-x
Zheng, H., and Xue, X.-S. (2020). Recent Computational Studies on Mechanisms of Hypervalent Iodine(III)-Promoted Dearomatization of Phenols. Coc 24 (18), 2106–2117. doi:10.2174/1385272824999200620223218
Zhou, B., Haj, M. K., Jacobsen, E. N., Houk, K. N., and Xue, X.-S. (2018a). Mechanism and Origins of Chemo- and Stereoselectivities of Aryl Iodide-Catalyzed Asymmetric Difluorinations of β-Substituted Styrenes. J. Am. Chem. Soc. 140 (45), 15206–15218. doi:10.1021/jacs.8b05935
Zhou, B., Yan, T., Xue, X.-S., and Cheng, J.-P. (2016b). Mechanism of Silver-Mediated Geminal Difluorination of Styrenes with a Fluoroiodane Reagent: Insights into Lewis-Acid-Activation Model. Org. Lett. 18 (23), 6128–6131. doi:10.1021/acs.orglett.6b03134
Zhou, Y., Wang, J., Gu, Z., Wang, S., Zhu, W., Aceña, J. L., et al. (2016). Next Generation of Fluorine-Containing Pharmaceuticals, Compounds Currently in Phase II-III Clinical Trials of Major Pharmaceutical Companies: New Structural Trends and Therapeutic Areas. Chem. Rev. 116 (2), 422–518. doi:10.1021/acs.chemrev.5b00392
Keywords: fluorination, cyclization, 6-endo, 5-oxo, hypervalent iodine, mechanism, DFT
Citation: Su J, Shu S, Li Y, Chen Y, Dong J, Liu Y, Fang Y and Ke Z (2022) Mechanism-Dependent Selectivity: Fluorocyclization of Unsaturated Carboxylic Acids or Alcohols by Hypervalent Iodine. Front. Chem. 10:897828. doi: 10.3389/fchem.2022.897828
Received: 16 March 2022; Accepted: 05 April 2022;
Published: 10 May 2022.
Edited by:
Jian-Wei Han, East China University of Science and Technology, ChinaReviewed by:
Ran Fang, Shaanxi University of Science and Technology, ChinaXiaosong Xue, Shanghai Institute of Organic Chemistry (CAS), China
Donghui Wei, Zhengzhou University, China
Copyright © 2022 Su, Shu, Li, Chen, Dong, Liu, Fang and Ke. This is an open-access article distributed under the terms of the Creative Commons Attribution License (CC BY). The use, distribution or reproduction in other forums is permitted, provided the original author(s) and the copyright owner(s) are credited and that the original publication in this journal is cited, in accordance with accepted academic practice. No use, distribution or reproduction is permitted which does not comply with these terms.
*Correspondence: Yan Liu, yanliu@gdut.edu.cn; Yanxiong Fang, fangyx@gdut.edu.cn; Zhuofeng Ke, kezhf3@mail.sysu.edu.cn
†These authors have contributed equally to this work