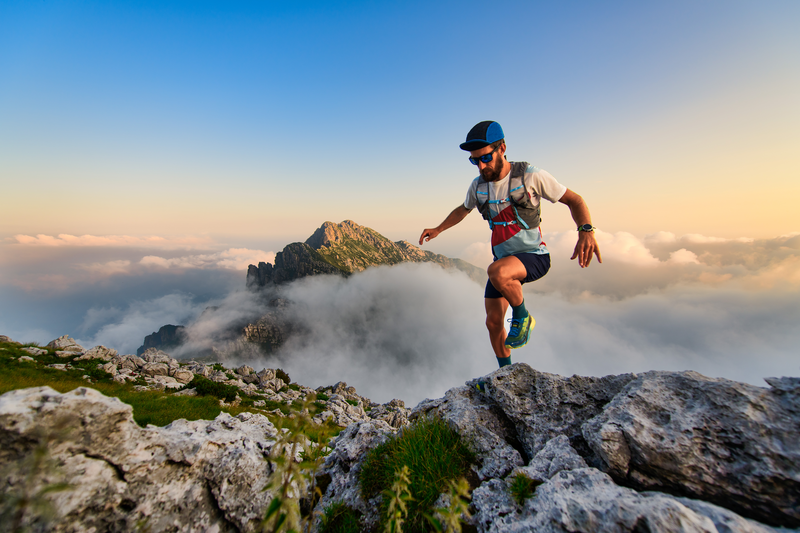
94% of researchers rate our articles as excellent or good
Learn more about the work of our research integrity team to safeguard the quality of each article we publish.
Find out more
ORIGINAL RESEARCH article
Front. Chem. , 14 June 2022
Sec. Organic Chemistry
Volume 10 - 2022 | https://doi.org/10.3389/fchem.2022.894603
This article is part of the Research Topic DNA-encoded Library and Chemistry View all 4 articles
An efficient method for the C-C bond formation via water soluble Na2PdCl4/sSPhos mediated Suzuki-Miyaura cross-coupling reaction of DNA-conjugated aryl iodide with (het)aryl boronic acids has been developed. This reaction proceeds at 37°C in water and acetonitrile (4:1) system. We also demonstrated that numerous aromatic and heteroaromatic boronic acids of different electronic natures, and harboring various functional groups, were highly compatible providing the desired coupling products in good to excellent yields. This DNA-compatible Suzuki-Miyaura cross-coupling reaction has strong potential to construct DNA-Encoded Libraries (DELs) in the context of drug discovery.
DNA-Encoded Library (DEL) technology is based on the concept from Brenner and Lerner (Brenner and Lerner 1992) and it is commonly used in the pharmaceutical industry to identify novel chemical matter that binds and modulates specific protein targets (Melkko et al., 2004; Melkko et al., 2007; Clark et al., 2009; Kleiner et al., 2011; Franzini et al., 2014; Salamon et al., 2016; Goodnow et al., 2017; Favalli et al., 2018; Neri and Lerner 2018; Ottl et al., 2019; Yuen et al., 2019; Zhao et al., 2019; Kunig et al., 2021; Shi et al., 2021). During the past decade, the use of DEL technology provided a great opportunity to identify drug-like compounds that can bind selectively to a variety of target proteins (Deng et al., 2012; Gentile et al., 2012; Disch et al., 2013; Samain et al., 2015; Seigal et al., 2015; Harris et al., 2016; Belyanskaya et al., 2017; Dawadi et al., 2020; Chamakuri et al., 2021). More recently, a number of different powerful applications leveraging the DEL technology have been proposed (Huang et al., 2022; Sunkari et al., 2022; Zhao et al., 2022). To expand the chemical space of these DNA-Encoded Libraries, a greater variety of DNA-Compatible reactions is required. Although much progress has been made in this direction (Potowski et al., 2021; Nie et al., 2022; Shen et al., 2022; Yang et al., 2022), the availability of efficient synthetic methods for the synthesis of DELs remains an important challenge.
A DEL is a complex mixture composed of a large number of drug-like molecules in which each molecule is conjugated to a unique and specific DNA-oligomer that encodes its chemical structure. Due to the presence of DNA barcodes, and due to the process of generating a DEL that involves alternation of chemical and molecular steps (e.g., split-and-pool strategy), any chemical modification has to be performed in the presence of DNA. This implicates that chemical reaction conditions must be mild and compatible with aqueous conditions. Although progress has been made in this direction, the availability of efficient methods for the synthesis of DELs remains an important challenge.
During the last few years, the interest in DNA-compatible transition metal catalyzed cross-coupling reactions has increased, and especially for C-C bond formation using Pd-catalyzed Suzuki-Miyaura cross-coupling reaction (Miyaura and Suzuki 1995; Bellina et al., 2004; Martin and Buchwald 2008). Due to mild reaction conditions, commercial availability of coupling partners and high chemo-selectivity, the Suzuki–Miyaura cross-coupling reaction is now the second most utilized reaction in the field of medicinal chemistry (Brown and Bostrom 2016), after the amide bond formation reaction. While methods for the Suzuki-Miyaura cross-coupling reaction were reported in the context of DNA-Encoded Library synthesis (Scheme 1) (Omumi et al., 2011; Deng et al., 2015; Ding and Clark 2015; Litovchick et al., 2015; Ding et al., 2016a; Ding et al., 2016b; Li and Huang 2018; Nicholas et al., 2019; Xu et al., 2019; Qu et al., 2020; Favalli et al., 2021), there is a great need to further develop this reaction.
In 2011, Omumi et al. reported first Suzuki-Miyaura cross-coupling reaction in the presence of DNA (C8-Ar-G-modified oligonucleotides) using aryl boronic acids employing Pd(OAc)2 and a hydrophilic phosphine ligand, 3-tri (3-sulfonatophenyl)phosphine trisodium (TPPTS) (Omumi et al., 2011). In 2014, Ding et al. reported first Suzuki-Miyaura cross-coupling reaction using Pd(PPh3)4 under aqueous conditions in the context of DEL (Ding and Clark 2015). Later, the same group reported the reaction for less reactive DNA-conjugated aryl chlorides using a combination of phosphinous acid/Pd catalyst and the sSPhos ligand at 80°C (Ding et al., 2016a). Compared with Pd(PPh3)4, this catalyst system is better for the coupling of pyrimidinyl chloride and unreactive aryl chloride with challenging heteroaryl boronates. In 2015, two reports published the construction of 334 and 34.7 million-membered DELs synthesized in three cycles, in which they introduced boronic acid/ester building blocks by Suzuki cross-coupling in the second and the third cycle respectively (Deng et al., 2015; Litovchick et al., 2015). In 2016, Ding et al. reported the construction of 3.5 million-membered DEL in three cycles, in which they introduced Suzuki-Miyaura cross-coupling reaction in the second cycle (Ding et al., 2016b). Li et al. developed a robust Suzuki-Miyaura reaction protocol employing a water-soluble Pd-precatalyst for the coupling of DNA-linked aryl halides with a wide range of boronic acids/esters including heteroaryl boronates (Li and Huang 2018). Additionally, Nicholas et al. reported an alternative Pd(OAc)2 catalyzed DNA-compatible reaction with aromatic, heteroaromatic, and vinyl boronic acids at 60°C (Nicholas et al., 2019). Recently, Xu et al. also reported Suzuki-Miyaura cross-coupling reaction on-DNA with aryl fluorosulfonates as electrophiles at room temperature (Xu et al., 2019). Very recently Qu et al. developed a Pd-mediated Suzuki-Miyaura cross-coupling of DNA-conjugated aryl bromides with potassium Boc-protected aminomethyltrifluoroborate at 95°C (Qu et al., 2020). Most recently Favalli et al. reported the Suzuki-Miyaura cross-coupling reaction of DNA-conjugated aryl iodides with (het)aryl boronic acids at 70°C using Pd(OAc)2 and TPPTS (Favalli et al., 2021).
Despite these few reports that are mostly using high temperature conditions, there is a need to develop this reaction at relatively low temperature to avoid DNA degradation. It is well established that DNA is highly stable in physiological conditions (37°C or at lower temperature). Importantly, among those studies, only one study reported Suzuki-Miyaura cross-coupling reaction on-DNA, at room temperature, using a non-readily available coupling partner (aryl fluorosulfonate) (Xu et al., 2019). Here, we introduced a DNA friendly method performed at room temperature using water soluble Na2PdCl4/ sSPhos mediated Suzuki-Miyaura cross-coupling reaction. For that purpose, DNA-conjugated aryl iodide was used with over fifty boronic acids that are readily available and full DNA integrity was confirmed by mass spectrometry.
The Suzuki-Miyaura cross-coupling of DNA-conjugated aryl iodide 1a with phenyl boronic acid 2a in 4:1 water and acetonitrile system was first examined to optimize reaction conditions for synthesizing the DNA-conjugated biaryl product 3a. Previously, Pd(OAc)2/N-XantPhos catalysts that led to excellent yield in the cross-coupling of (hetero)aryl halides with boronic acids was examined (Wang et al., 2015). However, it did not result in the formation of 3a at 37°C after 24 h (Table 1, entry 1). Previously reported reaction conditions (Favalli et al., 2021, Li and Huang, 2018, Ding and Clark, 2015) were tested for this coupling reaction at 37°C (Table 1, entry 2-4), Pd(OAc)2 and sSPhos-Pd-G2 did not furnish the desired product, whereas Pd(PPh3)4 gave 41% yield at 37°C. Interestingly, when water soluble Pd catalyst Na2PdCl4 (20 equiv), N-XantPhos ligand (40 equiv) and K2CO3 were used, the product yield was 61% (Table 1, entry 5). On the other hand, when N-XantPhos was replaced with sSPhos, the desired product could be isolated with a 67% yield under the same conditions. (Table 1, entry 6). These results clearly indicate that the water soluble Pd catalyst might have a dramatic influence on this coupling reaction. This speculation was corroborated by the observation that Na2PdCl4 gave the good yield, while Pd(OAc)2and sSPhos-Pd-G2 gave no product formation. Noteworthy, when sSPhos was replaced with X-Phos and XantPhos, a decrease in yield was observed (Table 1, entries 7–8).
After identifying Na2PdCl4/sSPhos as the best catalyst, we examined different bases. This base screening revealed that Na2CO3, Cs2CO3, K3PO4, and CsOH gave poor yields, while KOH gave a comparable yield to K2CO3 (Table 1, entries 9–13). Next, using K2CO3 and by increasing the reaction time to 28 h, the yield increased to 81% (Table 1, entry 14). Doubling the amount of base (600 equiv) also increased the product yield and reached 94% (Table 1, entry 15). However, a further increase of the amount of base (1,500 equiv) led to a decrease in the product yield. Attempt to reduce the catalyst loading did not reach complete conversion anymore (Table 1, entries 16-17). When the percentage of solvent mixture was altered to be 1:1 (water and acetonitrile), a slight decrease of the yield was observed (Table 1, entry 18). Further solvent screening revealed that DMSO, DMF, DMA and THF gave lower yields, and 1,4-dioxane solvent gave a comparable yield (Table 1, entries 19–23).
Therefore, we concluded that the optimal condition is: 20 equiv of Pd catalyst, 40 equiv of ligand as the catalyst system and K2CO3 (600 equiv) as the base, 4:1 water and acetonitrile, at 37°C for 28 h.
We next explored the substrate scope using the present optimized protocol and the results are summarized in Scheme 2. As expected, a number of aryl boronic acids bearing electron-rich, electron-deficient groups and functional groups at the para-position worked well, providing coupling products 3a-3p with 86–94% yields. Remarkably, some ortho- and meta-substituted aryl boronic acids were also applicable, leading to the formation of 3q-3w products with good yields. Sterically hindered 2,6-disubstituted phenyl boronic acids 3x and 3z afforded good to excellent conversion except 3y possibly due to bulky methoxy groups. Furthermore, 3,4-disubstituted, 3,5-disubstituted, 2,4-disubstituted and 2,5-disubstituted aryl boronic acids with electron-rich, electron-deficient groups also gave excellent yields (3aa-3aj). Additionally, coupling reaction of 2-naphthyl, 9-anthracenyl, fluorene-2-boronic acids proceeded smoothly to deliver the coupling products 3ak-3am respectively.
SCHEME 2. Suzuki-Miyaura cross-coupling reaction of aryl boronic acids with 1aa. aReaction Conditions: 1 equiv of 1a (1 mM in H2O), 200 equiv of aryl boronic acid (200 mM in ACN/H2O, 1:1), 20 equiv Na2PdCl4, 40 equiv sSPhos (10 mM in DMA), K2CO3, H2O:ACN (4:1), 37oC for 28 h; bH2O:1,4-dioxane (4:1).
Finally, after successful implementation of this protocol for the coupling of DNA-conjugated aryl iodide (1a) with aryl boronic acids, we next focused on the coupling of 1a with heteroaryl boronic acids. The results are summarized in Scheme 3. Thus, thiophene, furan, pyridyl and pyrimidyl boronic acids yielded the respective products (5a-5h) with good to excellent conversion (63–89%). The versatility of this methodology was further demonstrated by coupling 1a with fused heterocycles such as benzothiophene, indole, N-methyl indole, indazole, benzofuran and dibenzofuran boronic acids to give the respective coupling products (5i-5n) in moderate to excellent yields (51–95%). Furthermore, the coupling of DNA-conjugated aryl bromide with phenyl boronic acid is also compatible with the Suzuki-Miyaura reaction (41%). However, the coupling of DNA-conjugated aryl chloride with phenyl boronic acid yielded only 3%.
SCHEME 3. Suzuki-Miyaura cross-coupling reaction of heteroaryl boronic acids with 1aa. aReaction Conditions: 1 equiv of 1a (1 mM in H2O), 200 equiv of heteroaryl boronic acid (200 mM in ACN/H2O, 1:1), 20 equiv Na2PdCl4, 40 equiv sSPhos (10 mM in DMA), K2CO3, H2O:ACN (4:1), 37°C for 28 h; bH2O:1,4-dioxane.
In summary, we have developed an efficient method for the coupling of aryl iodide conjugated on double-stranded DNA with (het)aryl boronic acids via water soluble Na2PdCl4/sSPhos mediated Suzuki-Miyaura cross-coupling reaction. This reaction proceeds at 37°C in water and acetonitrile (4:1) system. These results demonstrate the scope of the Suzuki-Miyaura cross-coupling reaction for on-DNA substrates. The present protocol displays broad substrate scope and tolerates the functionality that would be very useful for construction of DNA-encoded libraries.
The original contributions presented in the study are included in the article/Supplementary Material, further inquiries can be directed to the corresponding authors.
VS and MF contributed to the conception of the study. The design of the experiments and the synthetic work was performed by VS. Data collection and analysis were carried out by VS and YS. VS and MF wrote the manuscript. VS, TN and MF contributed to the article revision.
This work was supported by the Fisher Center for Alzheimer’s Disease to MF (New York, United States) and by the JPB Foundation to MF (New York, United States).
The authors declare that the research was conducted in the absence of any commercial or financial relationships that could be construed as a potential conflict of interest.
All claims expressed in this article are solely those of the authors and do not necessarily represent those of their affiliated organizations, or those of the publisher, the editors and the reviewers. Any product that may be evaluated in this article, or claim that may be made by its manufacturer, is not guaranteed or endorsed by the publisher.
The Supplementary Material for this article can be found online at: https://www.frontiersin.org/articles/10.3389/fchem.2022.894603/full#supplementary-material
Belyanskaya, S. L., Ding, Y., Callahan, J. F., Lazaar, A. L., and Israel, D. I. (2017). Discovering Drugs with DNA-Encoded Library Technology: From Concept to Clinic with an Inhibitor of Soluble Epoxide Hydrolase. Chembiochem 18, 837–842. doi:10.1002/cbic.201700014
Brenner, S., and Lerner, R. A. (1992). Encoded Combinatorial Chemistry. Proc. Natl. Acad. Sci. U.S.A. 89, 5381–5383. doi:10.1073/pnas.89.12.5381
Brown, D. G., and Boström, J. (2016). Analysis of Past and Present Synthetic Methodologies on Medicinal Chemistry: Where Have All the New Reactions Gone? J. Med. Chem. 59, 4443–4458. doi:10.1021/acs.jmedchem.5b01409
Chamakuri, S., Lu, S., Ucisik, M. N., Bohren, K. M., Chen, Y.-C., Du, H.-C., et al. (2021). DNA-encoded Chemistry Technology Yields Expedient Access to SARS-CoV-2 M Pro Inhibitors. Proc. Natl. Acad. Sci. U.S.A. 118. doi:10.1073/pnas.2111172118
Clark, M. A., Acharya, R. A., Arico-Muendel, C. C., Belyanskaya, S. L., Benjamin, D. R., Carlson, N. R., et al. (2009). Erratum: Design, Synthesis and Selection of DNA-Encoded Small-Molecule Libraries. Nat. Chem. Biol. 5, 772. doi:10.1038/nchembio1009-772
Dawadi, S., Simmons, N., Miklossy, G., Bohren, K. M., Faver, J. C., Ucisik, M. N., et al. (2020). Discovery of Potent Thrombin Inhibitors from a Protease-Focused DNA-Encoded Chemical Library. Proc. Natl. Acad. Sci. U.S.A. 117, 16782–16789. doi:10.1073/pnas.2005447117
Deng, H., O’Keefe, H., Davie, C. P., Lind, K. E., Acharya, R. A., Franklin, G. J., et al. (2012). Discovery of Highly Potent and Selective Small Molecule ADAMTS-5 Inhibitors that Inhibit Human Cartilage Degradation via Encoded Library Technology (ELT). J. Med. Chem. 55, 7061–7079. doi:10.1021/jm300449x
Deng, H., Zhou, J., Sundersingh, F. S., Summerfield, J., Somers, D., Messer, J. A., et al. (2015). Discovery, SAR, and X-Ray Binding Mode Study of BCATm Inhibitors from a Novel DNA-Encoded Library. ACS Med. Chem. Lett. 6, 919–924. doi:10.1021/acsmedchemlett.5b00179
Ding, Y., and Clark, M. A. (2015). Robust Suzuki-Miyaura Cross-Coupling on DNA-Linked Substrates. ACS Comb. Sci. 17, 1–4. doi:10.1021/co5001037
Ding, Y., DeLorey, J. L., and Clark, M. A. (2016a). Novel Catalyst System for Suzuki-Miyaura Coupling of Challenging DNA-Linked Aryl Chlorides. Bioconjugate Chem. 27, 2597–2600. doi:10.1021/acs.bioconjchem.6b00541
Ding, Y., Franklin, G. J., DeLorey, J. L., Centrella, P. A., Mataruse, S., Clark, M. A., et al. (2016b). Design and Synthesis of Biaryl DNA-Encoded Libraries. ACS Comb. Sci. 18, 625–629. doi:10.1021/acscombsci.6b00078
Disch, J. S., Evindar, G., Chiu, C. H., Blum, C. A., Dai, H., Jin, L., et al. (2013). Discovery of Thieno[3,2-D]pyrimidine-6-Carboxamides as Potent Inhibitors of SIRT1, SIRT2, and SIRT3. J. Med. Chem. 56, 3666–3679. doi:10.1021/jm400204k
Favalli, N., Bassi, G., Bianchi, D., Scheuermann, J., and Neri, D. (2021). Large Screening of DNA-Compatible Reaction Conditions for Suzuki and Sonogashira Cross-Coupling Reactions and for Reverse Amide Bond Formation. Bioorg. Med. Chem. 41, 116206. doi:10.1016/j.bmc.2021.116206
Favalli, N., Bassi, G., Scheuermann, J., and Neri, D. (2018). DNA-encoded Chemical Libraries - Achievements and Remaining Challenges. FEBS Lett. 592, 2168–2180. doi:10.1002/1873-3468.13068
Favalli, N., Bassi, G., Zanetti, T., Scheuermann, J., and Neri, D. (2019). Screening of Three Transition Metal‐Mediated Reactions Compatible with DNA‐Encoded Chemical Libraries. Hca 102, e1900033. doi:10.1002/hlca.201900033
Franzini, R. M., Neri, D., and Scheuermann, J. (2014). DNA-encoded Chemical Libraries: Advancing beyond Conventional Small-Molecule Libraries. Acc. Chem. Res. 47, 1247–1255. doi:10.1021/ar400284t
Gentile, G., Merlo, G., Pozzan, A., Bernasconi, G., Bax, B., Bamborough, P., et al. (2012). 5-Aryl-4-carboxamide-1,3-oxazoles: Potent and Selective GSK-3 Inhibitors. Bioorg. Med. Chem. Lett. 22, 1989–1994. doi:10.1016/j.bmcl.2012.01.034
Goodnow, R. A., Dumelin, C. E., and Keefe, A. D. (2017). DNA-encoded Chemistry: Enabling the Deeper Sampling of Chemical Space. Nat. Rev. Drug Discov. 16, 131–147. doi:10.1038/nrd.2016.213
Harris, P. A., King, B. W., Bandyopadhyay, D., Berger, S. B., Campobasso, N., Capriotti, C. A., et al. (2016). DNA-encoded Library Screening Identifies Benzo[b][1,4]oxazepin-4-Ones as Highly Potent and Monoselective Receptor Interacting Protein 1 Kinase Inhibitors. J. Med. Chem. 59, 2163–2178. doi:10.1021/acs.jmedchem.5b01898
Huang, Y., Li, Y., and Li, X. (2022). Strategies for Developing DNA-Encoded Libraries beyond Binding Assays. Nat. Chem. 14, 129–140. doi:10.1038/s41557-021-00877-x
Kleiner, R. E., Dumelin, C. E., and Liu, D. R. (2011). Small-molecule Discovery from DNA-Encoded Chemical Libraries. Chem. Soc. Rev. 40, 5707–5717. doi:10.1039/c1cs15076f
Kunig, V. B. K., Potowski, M., Klika Škopić, M., and Brunschweiger, A. (2021). Scanning Protein Surfaces with DNA‐Encoded Libraries. Chemmedchem 16, 1048–1062. doi:10.1002/cmdc.202000869
Li, J.-Y., and Huang, H. (2018). Development of DNA-Compatible Suzuki-Miyaura Reaction in Aqueous Media. Bioconjugate Chem. 29, 3841–3846. doi:10.1021/acs.bioconjchem.8b00676
Litovchick, A., Dumelin, C. E., Habeshian, S., Gikunju, D., Guié, M.-A., Centrella, P., et al. (2015). Encoded Library Synthesis Using Chemical Ligation and the Discovery of sEH Inhibitors from a 334-Million Member Library. Sci. Rep. 5. doi:10.1038/srep10916
Martin, R., and Buchwald, S. L. (2008). Palladium-Catalyzed Suzuki−Miyaura Cross-Coupling Reactions Employing Dialkylbiaryl Phosphine Ligands. Acc. Chem. Res. 41, 1461–1473. doi:10.1021/ar800036s
Melkko, S., Dumelin, C., Scheuermann, J., and Neri, D. (2007). Lead Discovery by DNA-Encoded Chemical Libraries. Drug Discov. Today 12, 465–471. doi:10.1016/j.drudis.2007.04.007
Melkko, S., Scheuermann, J., Dumelin, C. E., and Neri, D. (2004). Encoded Self-Assembling Chemical Libraries. Nat. Biotechnol. 22, 568–574. doi:10.1038/nbt961
Miyaura, N., and Suzuki, A. (1995). Palladium-Catalyzed Cross-Coupling Reactions of Organoboron Compounds. Chem. Rev. 95, 2457–2483. doi:10.1021/cr00039a007
Neri, D., and Lerner, R. A. (2018). DNA-encoded Chemical Libraries: A Selection System Based on Endowing Organic Compounds with Amplifiable Information. Annu. Rev. Biochem. 87, 479–502. doi:10.1146/annurev-biochem-062917-012550
Nie, Q., Fang, X., Liu, C., Zhang, G., Fan, X., Li, Y., and Li, Y. (2022). DNA-Compatible ortho-Phthalaldehyde (OPA)-Mediated 2-Substituted Isoindole Core Formation and Applications. J. Org. Chem. 87, 2551–2558. doi:10.1021/acs.joc.1c02496
Omumi, A., Beach, D. G., Baker, M., Gabryelski, W., and Manderville, R. A. (2011). Postsynthetic Guanine Arylation of DNA by Suzuki−Miyaura Cross-Coupling. J. Am. Chem. Soc. 133, 42–50. doi:10.1021/ja106158b
Ottl, J., Leder, L., Schaefer, J. V., and Dumelin, C. E. (2019). Encoded Library Technologies as Integrated Lead Finding Platforms for Drug Discovery. Molecules 24, 1629. doi:10.3390/molecules24081629
Potowski, M., Luttig, R., Vakalopoulos, A., and Brunschweiger, A. (2021). Copper(I/II)-Promoted Diverse Imidazo[1,2-alpha]pyridine Synthesis on Solid-Phase Bound DNA Oligonucleotides for Encoded Library Design. Org. Lett. 23, 5480–5484. doi:10.1021/acs.orglett.1c01834
Qu, Y., Liu, S., Wen, H., Xu, Y., An, Y., Li, K., et al. (2020). Palladium-mediated Suzuki-Miyaura Cross-Coupling Reaction of Potassium Boc-Protected Aminomethyltrifluoroborate with DNA-Conjugated Aryl Bromides for DNA-Encoded Chemical Library Synthesis. Biochem. Biophysical Res. Commun. 533, 209–214. doi:10.1016/j.bbrc.2020.04.027
Rossi, R., Bellina, F., and Carpita, A. (2004). Palladium Catalysts for the Suzuki Cross-Coupling Reaction: An Overview of Recent Advances. Synthesis 2004, 2419–2440. doi:10.1055/s-2004-831223
Salamon, H., Klika Škopić, M., Jung, K., Bugain, O., and Brunschweiger, A. (2016). Chemical Biology Probes from Advanced DNA-Encoded Libraries. ACS Chem. Biol. 11, 296–307. doi:10.1021/acschembio.5b00981
Samain, F., Ekblad, T., Mikutis, G., Zhong, N., Zimmermann, M., Nauer, A., et al. (2015). Tankyrase 1 Inhibitors with Drug-like Properties Identified by Screening a DNA-Encoded Chemical Library. J. Med. Chem. 58, 5143–5149. doi:10.1021/acs.jmedchem.5b00432
Seigal, B. A., Connors, W. H., Fraley, A., Borzilleri, R. M., Carter, P. H., Emanuel, S. L., et al. (2015). The Discovery of Macrocyclic XIAP Antagonists from a DNA-Programmed Chemistry Library, and Their Optimization to Give Lead Compounds with In Vivo Antitumor Activity. J. Med. Chem. 58, 2855–2861. doi:10.1021/jm501892g
Shen, Y., Yang, G., Huang, W., Shaginian, A., Lin, Q., Wan, J., Li, J., Deng, Y., and Liu, G. (2022). Photoredox Deaminative Alkylation in DNA-Encoded Library Synthesis. Org. Lett. 24, 2650–2654. doi:10.1021/acs.orglett.2c00697
Shi, Y., Wu, Y.-r., Yu, J.-q., Zhang, W.-n., and Zhuang, C.-l. (2021). DNA-encoded Libraries (DELs): a Review of On-DNA Chemistries and Their Output. RSC Adv. 11, 2359–2376. doi:10.1039/d0ra09889b
Sunkari, Y. K., Siripuram, V. K., Nguyen, T.-L., and Flajolet, M. (2022). High-power Screening (HPS) Empowered by DNA-Encoded Libraries. Trends Pharmacol. Sci. 43, 4–15. doi:10.1016/j.tips.2021.10.008
Wang, D., Chen, H.-G., Tian, X.-C., Liang, X.-X., Chen, F.-Z., and Gao, F. (2015). Room-temperature Palladium-Catalysed Suzuki-Miyaura Coupling of Arylboric Acid with Aryl Chlorides. RSC Adv. 5, 107119–107122. doi:10.1039/c5ra19790b
Xu, H., Ma, F., Wang, N., Hou, W., Xiong, H., Lu, F., et al. (2019). DNA‐Encoded Libraries: Aryl Fluorosulfonates as Versatile Electrophiles Enabling Facile On‐DNA Suzuki, Sonogashira, and Buchwald Reactions. Adv. Sci. 6, 1901551. doi:10.1002/advs.201901551
Yang, S., Zhao, G., Gao, Y., Sun, Y., Zhang, G., Fan, X., Li, Y., and Li, Y. (2022). In-Solution Direct Oxidative Coupling for the Integration of Sulfur/Selenium Into DNA-Encoded Chemical Libraries. Chem. Sci. 13, 2604–2613. doi:10.1039/d1sc06268a
Yuen, L. H., Dana, S., Liu, Y., Bloom, S. I., Thorsell, A.-G., Neri, D., et al. (2019). A Focused DNA-Encoded Chemical Library for the Discovery of Inhibitors of NAD+-Dependent Enzymes. J. Am. Chem. Soc. 141, 5169–5181. doi:10.1021/jacs.8b08039
Zhao, G., Huang, Y., Zhou, Y., Li, Y., and Li, X. (2019). Future Challenges with DNA-Encoded Chemical Libraries in the Drug Discovery Domain. Expert Opin. Drug Discov. 14, 735–753. doi:10.1080/17460441.2019.1614559
Keywords: suzuki-miyaura cross-coupling reaction, palladium catalysis, C-C bond formation, DNA-encoded library (DEL), drug discovery
Citation: Siripuram VK, Sunkari YK, Nguyen T-L and Flajolet M (2022) DNA-Compatible Suzuki-Miyaura Cross-Coupling Reaction of Aryl Iodides With (Hetero)Aryl Boronic Acids for DNA-Encoded Libraries. Front. Chem. 10:894603. doi: 10.3389/fchem.2022.894603
Received: 11 March 2022; Accepted: 02 May 2022;
Published: 14 June 2022.
Edited by:
Xiaoyu Li, University of Hong Kong, Hong Kong SAR, ChinaReviewed by:
Yizhou Li, Chongqing University, ChinaCopyright © 2022 Siripuram, Sunkari, Nguyen and Flajolet. This is an open-access article distributed under the terms of the Creative Commons Attribution License (CC BY). The use, distribution or reproduction in other forums is permitted, provided the original author(s) and the copyright owner(s) are credited and that the original publication in this journal is cited, in accordance with accepted academic practice. No use, distribution or reproduction is permitted which does not comply with these terms.
*Correspondence: Vijay Kumar Siripuram, dmlqYXlrdW1hci5zaXJpcHVyYW1Acm9ja2VmZWxsZXIuZWR1; Marc Flajolet, bWFyYy5mbGFqb2xldEByb2NrZWZlbGxlci5lZHU=
Disclaimer: All claims expressed in this article are solely those of the authors and do not necessarily represent those of their affiliated organizations, or those of the publisher, the editors and the reviewers. Any product that may be evaluated in this article or claim that may be made by its manufacturer is not guaranteed or endorsed by the publisher.
Research integrity at Frontiers
Learn more about the work of our research integrity team to safeguard the quality of each article we publish.