- 1School of Chemical Engineering of Guizhou Minzu University, Guizhou, China
- 2National Engineering Research Center for Compounding and Modification of Polymer Materials, Guizhou, China
- 3Polymer Composites Engineering Research Center of Guizhou Minzu University, Guiyang, China
The DOPO derivative-conjugated flame retardant 4, 4'-{1'', 4'' - phenylene - bis [amino - (10‴ - oxy -10‴-hydro-9‴-hydrogen-10‴ λ5 -phosphaphenanthrene-10''-yl)-methyl]}-diphenol (P-PPD-Ph) with two hydroxyl groups was synthesized. Polylactic acid conjugated flame-retardant composites with P-PPD-Ph were papered by using a twin-screw extruder. The flame-retardant properties of polylactic acid-conjugated flame-retardant composites were investigated. The flame-retardant properties of PLA-conjugated flame-retardant composites were characterized by the limiting oxygen index (LOI) and the vertical burning test (UL94). The results showed that the PLA-conjugated flame-retardant composites achieved a V-0 rating (UL-94, 3.2 mm) when the conjugated flame retardant was added at 5 wt%, and increase in LOI value from 22.5% to 31.4% relative to composites without added conjugated flame retardant. The flame-retardant mechanism of PLA-conjugated flame-retardant composites were further studied by TG-FTIR, the results showed that the P-PPD-Ph promoted the PLA-conjugated flame-retardant composites to decompose and also released fragments with quenching and dilution, which suggests that P-PPD-Ph for PLA-conjugated flame-retardant composites mainly play a role of the gas-phase flame retardant.
Introduction
In recent years, due to increasing depletion of petroleum resources and the pollution of petroleum-based polymers to the environment, environmentally friendly biodegradable materials have been rapidly developed (Bandehali et al., 2021). Among them, polylactic acid (PLA) receives more attention due to its excellent mechanical properties (Mirkhalaf and Fagerström, 2021), and it has been widely used in electrical and automotive applications as a replacement for some general-purpose plastics (Arroyo and Ryan, 2018; Li et al., 2018; Yang et al., 2022). However, PLA is an extremely flammable substance that will drip during the combustion process, thus limiting its application in the electrical industry (Sun et al., 2018; Ma et al., 2022). Therefore, the research on flame-retardant PLA is necessary. The addition of flame retardants is a common method for improving the flame retardancy of PLA, and there are many types of flame retardants suitable for PLA (Tawiah et al., 2018). Among them, organophosphorus compounds and their derivatives have received extensive attention because of their excellent flame retardancy and non-toxicity (Hirsch et al., 2017; Salmeia et al., 2018; Nazir and Gaan, 2020), and their applications are very broad (Wu et al., 2018; Bascucci et al., 2022; Wang et al., 2022).
In this series, 9,10-dihydro-9-oxa-10- phosphaphenanthrene-10-oxide (DOPO) and its derivatives have been hot in recent years. Schartel et al (2016) explored the flame retardant modes of action of DOPO derivatives, and DOPO derivatives have higher flame-retardant efficiency. Generally, there are two kinds of DOPO derivatives used to improve the flame-retardant properties of PLA. First, the additive DOPO derivatives are mixed into the polymer material, second, the reactive DOPO derivatives are directly reacted into the polymer chain. For instance, Long et al (2017) synthesized three kinds of Di-DOPO structures, namely, ethyl-DOPO, phenethyl-DOPO, and naphthalene-DOPO, and compounded them into PLA materials. Three kinds of PLA flame-retardant composites have good flame retardant performance, and at 10 wt% addition of the derivatives, all PLA flame-retardant composites achieved a V-0 rating (UL-94, 3.2 mm). As additive flame retardants, DOPO derivatives have shown good flame-retardant ability for polymers. However, additive DOPO derivatives exhibit practical drawbacks during application, such as migration problems and the deterioration of polymer performance (Chu et al., 2022). Therefore, introducing in active groups that react with the matrix in DOPO derivatives is a current essential work. Researchers have tried to introduce a variety of DOPO derivatives with different reactive groups. For instance, Tao et al. (Yu et al., 2017) synthesized of the 9,10-dihydro-9-oxa-10-phosphaphenanthrene-10-oxide and 1,6-hexane diisocyanate (DOPO-ICN) and applied it to jute/PLA flame-retardant composites. DOPO-ICN reacts with PLA, and this makes DOPO-ICN to not only improve the flame retardant properties of PLA flame-retardant composites but also create less impact on other properties of composites.
In this study, a reactive DOPO derivative (P-PPD-Ph conjugated flame retardant) was synthesized (as Figure 1A shows) and added the P-PPD-Ph into the PLA matrix. The flame retardant properties of PLA-conjugated flame retardant composites were characterized by limiting oxygen index (LOI) and vertical combustion test (UL94).
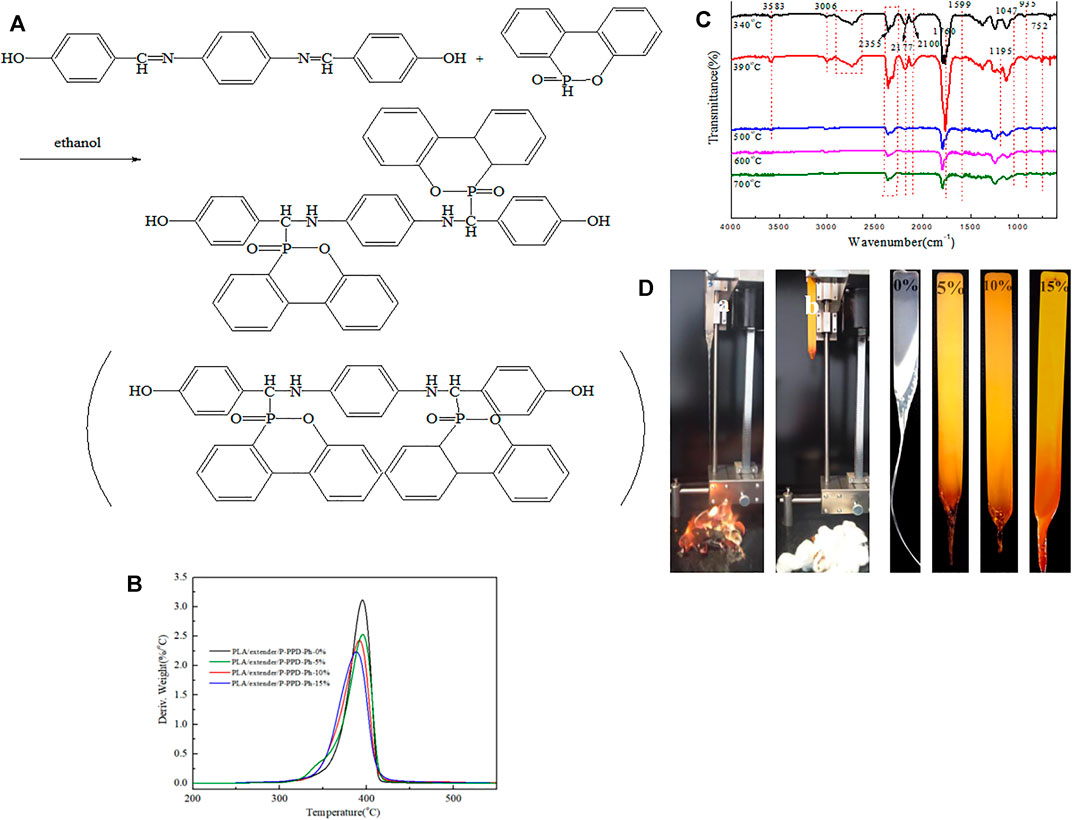
FIGURE 1. (A) Schematic diagram of synthetic P-PPD-Ph. (B) Thermal analysis of PLA and PLA-conjugated flame-retardant composites under nitrogen (N2) atmosphere. (C) FTIR spectra of the PLA-conjugated flame-retardant composites at different temperatures of TG under N2 atmosphere. (D) Photos of samples during the UL-94 test (a is PLA/P-PPD-Ph-0%, and b is PLA/P-PPD-Ph-5%).
Materials and Methods
Materials
9,10-Dihydro-9-oxa-10-phosphaphenanthrene10-oxide (DOPO) was purchased from Huawei Ruike Chemical Co., Ltd. (Beijing, China). PLA was purchased from Wuxi Diaisheng Epoxy Co., Ltd. (Wuxi, China). Epoxy chain extender (ADR-5481) was purchased from Sinopharm Group Chemical Reagent Co., Ltd. (Shanghai, China).
Preparation of PLA-Conjugated Flame-Retardant Composites
Imine-containing compounds and P-PPD-Ph were synthesized according to literature procedures (Sun and Yao, 2011). PLA, chain extender, and P-PPD-Ph were dried for 4 h under vacuum at 80°C before use. PLA, epoxy chain extender (4 wt‰), and P-PPD-Ph (0, 5, 10, and 15 wt%) were mixed uniformly. Then, the mixture was extruded by using a twin-screw extruder (CTE 35, Coperion Keya Machinery Manufacturing Co., Ltd., China) at a temperature of 180–200°C and a screw speed of 300 rpm. The extruded pellets were then molded into samples for testing using an injection molding machine (CJ80MZ 2N CII, Zhende Plastic Machinery Factory, China) at 180–200°C.
Characterization
Thermal analysis (TG) was conducted in a Q50 thermal gravimetric analyzer (TA, United States) at a heating rate of 10°C/min and under nitrogen conditions. Approximately, 5 mg of the sample was weighed and placed in an aluminum ceramic with a gas flow of 60 ml/min.
The UL-94 test (SH5300, Guangzhou Xinhe Electronic Equipment Co., Ltd., China) was conducted with sample sizes of 130*10*3.2 mm3 in accordance with the ASTM UL 94-2006.
The LOI measurements were performed on an oxygen index flammability gauge (HC-2C) according to ASTM D 2863-97 standard with a sample dimension of 100 *6.5 *3.2 mm3.
Results and Discussion
Thermal Degradation Behavior of PLA-Conjugated Flame-Retardant Composites
The TG curves under N2 of PLA and PLA-conjugated flame-retardant composites containing P-PPD-Ph are presented in Figure 1B, and the corresponding data are listed in Table 1A. Relative to PLA/materials without the addition of flame retardant, the addition of flame-retardant P-PPD-Ph reduced the initial decomposition temperature (T−5%) of PLA-conjugated flame-retardant composites. This may be due to the decomposition temperature of P-PPD-Ph before PLA, and the decomposition of P-PPD-Ph changed the decomposition behavior and induced the PLA-conjugated flame-retardant composites to decompose in advance. Additionally, the residual carbon of PLA-conjugated flame- retardant composites at 700°C increased with the addition of the P-PPD-Ph conjugated flame retardant. This is due to the degradation of phosphorus-containing groups generating heat-resistant residues, which lead to inhibit the decomposition of PLA-conjugated flame-retardant composites (Battig et al., 20121), reduce the maximum thermal decomposition rate, and promote the formation of carbon layers, thereby increasing the char yield of PLA-conjugated flame-retardant composites. Also, when the addition of P-PPD-Ph conjugated flame retardant was 15 wt%, the char residue of the PLA-conjugated flame-retardant composites increased by 4.2% under 700°C. This shows that P-PPD-Ph conjugated flame retardant creates a condensed-phase flame-retardant effect on PLA-conjugated flame-retardant composites.

TABLE 1A. (A) TG and DTG data of PLA and PLA-conjugated flame-retardant composites derived from TG analysis.
The TG-FTIR of PLA-Conjugated Flame-Retardant Composites
Figure 1C shows the FTIR spectra of composites at different temperatures in the TG test under N2 conditions. In the PLA/P-PPD-Ph-15% initial decomposition stage (around 340°C), the absorption peak of P-O near 935 cm−1 appeared in the FTIR spectrum, and 1,599 cm−1 was the absorption peak of P-Ph, and the absorption peaks of CO2 (2,355 cm−1) and CO (2,177 cm−1 and 2,100 cm−1) appeared. The stretching vibration peak of carbonyl was near 1760 cm−1, and the absorption peak of C–H on the aliphatic chain was at 3,006 cm−1. Also, the absorption peak of hydrocarbons and carbon–oxygen species was 2,638–2,908 cm−1, and the absorption peak of -OH was 3,583 cm−1. With the increase of temperature to the maximum decomposition temperature (about 390°C), the thermal decomposition products of PLA/P-PPD-Ph-conjugated flame-retardant composites were detected by FTIR, and the absorption peak intensity was the strongest and new absorption peaks were detected. 752cm−1 may be the absorption peak of P-C, 1195cm−1 may be the absorption peak of P=O, and 935, 1,047, and 1195cm−1 were the absorption peaks of aromatic phosphorus-containing complexes, which was consistent with the related literature (Yu et al., 2020; Qin et al., 2021). It showed that the thermal decomposition of the PLA conjugated flame-retardant composites may release aromatic esters and aromatic phosphorus-containing compounds. These substances were beneficial to improve the flame retardancy of the conjugated flame-retardant composites and played a gas-phase flame-retardant effect. However, as the thermal decomposition temperature increased to 500°C, the peak intensity of FTIR significantly decreased, and the absorption peaks at 3,006 cm−1 (C–H) and 2,638–2,908 cm−1 (hydrocarbon and carbon–oxygen species, respectively) disappeared. When the temperature reached 600°C (final decomposition stage), the absorption peaks of P=O at 1195cm−1 and 3583cm−1 (-OH) disappeared, which corroborated that the thermal decomposition products of the conjugated flame-retardant composites may have PO2· and PO·, which can capture H· and OH·, so P-PPD-Ph played a gas-phase flame-retardant role in PLA-conjugated flame-retardant composites.
Effects of the P-PPD-Ph on the Flame-Retardant Properties of PLA-Conjugated Flame-Retardant Composites
The effects of the P-PPD-Ph conjugated flame retardant on the flame retardant performance of PLA-conjugated flame-retardant composites were investigated by the vertical burning test (UL-94) and limiting oxygen index test (LOI), and the results are shown in Table 1B. As shown in Table 1B, when the content of P-PPD-Ph conjugated flame retardant is 5 wt%, the PLA-conjugated flame-retardant composites can pass the UL-94 V-0 level. As shown in Figure 1D, the melting dripping occurred in PLA and also in the PLA-conjugated flame-retardant composites. In PLA materials without the addition of flame retardant, splines were dropped in the form of wire-drawing after combustion, while after the P-PPD-Ph conjugated flame retardant was added, the molten droplets after combustion were dropped in the form of water drops. Moreover, PLA without the addition had droplets with fire, and the absorbent cotton was easily ignited, whereas the PLA conjugated flame retardant composites with 5% P-PPD-Ph added had droplets more rapidly without fire, and the absorbent cotton was well reserved. The results show that P-PPD-Ph can decompose the matrix in advance, thus producing molten droplets that take away the heat of the burning matrix (Xi et al., 2016), which is the same as the explanation on TG-FTIR of composites; the quenching and dilution effects of P-PPD-Ph can extinguish the fire on droplets during dripping, thereby the cotton will not be ignited. Also, the LOI value of PLA conjugated flame retardant composites increased from 22.5 to 34.0%, it achieved a good flame-retardant effect, and it can be seen that the prepared P-PPD-Ph conjugated flame retardant had higher flame-retardant efficiency.
Conclusion
In this study, P-PPD-Ph conjugated flame retardant with a double hydroxyl DOPO derivative was synthesized and reacted with the PLA matrix to prepare PLA-conjugated flame-retardant composites. The effects of P-PPD-Ph conjugated flame retardant content on the flame retardant performance of PLA composites were investigated. When the content of P-PPD-Ph conjugated flame retardant was 5 wt%, the PLA conjugated flame retardant composites passed the V0 grade (UL-94). Therefore, the P-PPD-Ph conjugated flame retardant has an effective flame-retardant effect on PLA. TG-FTIR tests showed that the compound has the inhibitory flame mechanism of the gas phase and condensed phase in PLA and has a good effect on promoting the formation of PLA-conjugated flame-retardant composites into carbon. When the V0 level is reached, the PLA-conjugated flame-retardant composites still have good mechanical properties.
Data Availability Statement
The raw data supporting the conclusion of this article will be made available by the authors, without undue reservation.
Author Contributions
DZ and KW prepared materials and carried out in experiments. MP, KW, and FT helped to analyze experimental data. CG, DB, SQ, and FT helped to characterize materials. DZ supervised the work. All authors contributed to revise the manuscript, approved the final version and agreed to be accountable for all aspects of this work.
Conflict of Interest
The authors declare that the research was conducted in the absence of any commercial or financial relationships that could be construed as a potential conflict of interest.
Publisher’s Note
All claims expressed in this article are solely those of the authors and do not necessarily represent those of their affiliated organizations, or those of the publisher, the editors, and the reviewers. Any product that may be evaluated in this article, or claim that may be made by its manufacturer, is not guaranteed or endorsed by the publisher.
Acknowledgments
The authors would like to acknowledge the financial support from the Natural Science Foundation of China (Grants: 52163001 and 51863004), Guizhou Province Science and Technology Plan Projects (Grants:CXTD[2021]005, [2020]1Y211, [2018]2812), Guiyang city experts workstation (Grant: ZJGZZ2021-07), Baiyun District Science and Technology Plan Projects (Grants: (2019)34, (2020)28, and (2019)21), and Polymer Composites Engineering Research Center of Guizhou Minzu University (Grant: GZMUGCZX(2021)01).
References
Arroyo, J., and Ryan, C. (2018). Incorporation of Carbon Nanofillers Tunes Mechanical And Electrical Percolation in PHBV: PLA Blends. Polymers 10, 1371. doi:10.3390/polym10121371
Bandehali, H., Sanaeepur, H., Amooghin, A. E., Shirazian, S., and Ramakrishna, S. (2021). Biodegradable Polymers For Membrane Separation. Sep. Purif. Technol. 269, 118731. doi:10.1016/j.seppur.2021.118731
Bascucci, C., Duretek, I., Lehner, S., Holzer, C., Gaan, S., Hufenus, R., et al. (2022). Investigating Thermomechanical Recycling of Poly (Ethylene Terephthalate) Containing Phosphorus Flame Retardants. Polym. Degrad. Stab. 195, 109783. doi:10.1016/j.polymdegradstab.2021.109783
Battig, A., Müller, P., Berlin, A., and Schartel, B. (2021). Hyperbranched Rigid Aromatic Phosphorus‐Containing Flame Retardants For Epoxy Resins. Macromol. Mater. Eng. 306, 2000731. doi:10.1002/mame.202000731
Chu, L., Qiu, S., Zhang, S., Xu, Z., Zhou, Y., Luo, X., et al. (2022). Exploration on Structural Rules of Highly Efficient Flame Retardant Unsaturated Polyester Resins. J. Colloid Interface Sci. 608, 142–157. doi:10.1016/j.jcis.2021.09.124
Hirsch, C., Striegl, B., Mathes, S., Adlhart, C., Edelmann, M., Bono, E., et al. (2017). Multiparameter Toxicity Assessment of Novel DOPO-Derived Organophosphorus Flame Retardants. Arch. Toxicol. 91, 407–425. doi:10.1007/s00204-016-1680-4
Li, Y., Gao, S., Dong, R., Ding, X., and Duan, X. (2018). Additive Manufacturing of PLA And CF/PLA Binding Layer Specimens Via Fused Deposition Modeling. J. Mater. Eng. Perform. 27, 492–500. doi:10.1007/s11665-017-3065-0
Long, L., Chang, Q., He, W., Xiang, Y., Qin, S., Yin, J., et al. (2017). Effects of Bridged DOPO Derivatives on the thermal Stability and Flame Retardant Properties of Poly(lactic Acid). Polym. Degrad. Stab. 139, 55–66. doi:10.1016/j.polymdegradstab.2017.03.016
Ma, X., Wu, N., Liu, P., and Cui, H. (2022). Fabrication of Highly Efficient Phenylphosphorylated Chitosan Bio-Based Flame Retardants For Flammable Pla Biomaterial. Carbohydr. Polym. 287, 119317. doi:10.1016/j.carbpol.2022.119317
Mirkhalaf, S., and Fagerström, M. (2021). The Mechanical Behavior of Polylactic Acid (Pla) Films: Fabrication, Experiments And Modelling. Mech. Time-Depend. Mater. 25, 119–131. doi:10.1007/s11043-019-09429-w
Nazir, R., and Gaan, S. (2020). Recent Developments in P (O/S)–N Containing Flame Retardants. J. Appl. Polym. Sci. 137, 47910. doi:10.1002/app.47910
Qin, S., Yang, Z., Zhang, S., Zhang, Z., and Yang, L. (2021). Comparison of Flame-Retardancy Property And Mechanism Between A Phosphate Ester And A Phosphoramine Flame-Retardants. J. Wuhan Univ. Technol.-Mat. Sci. Edit 36, 148–156. doi:10.1007/s11595-021-2388-8
Salmeia, K. A., Gooneie, A., Simonetti, P., Nazir, R., Kaiser, J.-P., Rippl, A., et al. (2018). Comprehensive Study on Flame Retardant Polyesters from Phosphorus Additives. Polym. Degrad. Stab. 155, 22–34. doi:10.1016/j.polymdegradstab.2018.07.006
Schartel, B., Perret, B., Dittrich, B., Ciesielski, M., Krämer, J., Müller, P., et al. (2016). Flame Retardancy of Polymers: the Role of Specific Reactions in the Condensed Phase. Macromol. Mater. Eng. 301, 9–35. doi:10.1002/mame.201500250
Sun, C.-B., Mao, H.-D., Chen, F., and Fu, Q. (2018). Preparation of Polylactide Composite With Excellent Flame Retardance And Improved Mechanical Properties. Chin. J. Polym. Sci. 36, 1385–1393. doi:10.1007/s10118-018-2150-7
Sun, D., and Yao, Y. (2011). Synthesis of Three Novel Phosphorus-Containing Flame Retardants and Their Application in Epoxy Resins. Polym. Degrad. Stab. 96, 1720–1724. doi:10.1016/j.polymdegradstab.2011.08.004
Tawiah, B., Yu, B., and Fei, B. (2018). Advances in Flame Retardant Poly (Lactic Acid). Polymers 10, 876. doi:10.3390/polym10080876
Wang, J., Zhou, H., Pan, Z., Wu, H., and Wang, D. (2022). Synthesis of Low Phosphorus Flame Retardant Containing Benzimidazole And Hydroxyl And Its Application in Reducing Combustion Smoke For Epoxy Resin. Polym. Adv. Technol. 33, 1533–1543. doi:10.1002/pat.5619
Wu, H., Araby, S., Xu, J., Kuan, H.-C., Wang, C.-H., Mouritz, A., et al. (2018). Filling Natural Microtubules With Triphenyl Phosphate For Flame-Retarding Polymer Composites. Compos. Part A Appl. Sci. Manuf. 115, 247–254. doi:10.1016/j.compositesa.2018.09.030
Xi, W., Qian, L., Qiu, Y., and Chen, Y. (2016). Flame-retardant Behavior of Bi-group Molecule Derived from Phosphaphenanthrene and Triazine Groups on Polylactic Acid. Polym. Adv. Technol. 27, 781–788. doi:10.1002/pat.3714
Yang, R., Xu, G., Dong, B., Hou, H., and Wang, Q. (2022). A “Polymer To Polymer” Chemical Recycling of Pla Plastics By the “De–Re Polymerization” Strategy. Macromolecules 55, 1726–1735. doi:10.1021/acs.macromol.1c02085
Yu, H., Xu, X., Xia, Y., Pan, M., Zarshad, N., Pang, B., et al. (2020). Synthesis of A Novel Modified Chitosan As An Intumescent Flame Retardant For Epoxy Resin. E-Polymers 20, 303–316. doi:10.1515/epoly-2020-0036
Keywords: conjugated flame retardant, polylactic acid, flame retardant performance, gas phase flame retardant, composites
Citation: Zhang D, Pei M, Wei K, Tan F, Gao C, Bao D and Qin S (2022) Flame-Retardant Properties and Mechanism of Polylactic Acid-Conjugated Flame-Retardant Composites. Front. Chem. 10:894112. doi: 10.3389/fchem.2022.894112
Received: 11 March 2022; Accepted: 11 April 2022;
Published: 11 May 2022.
Edited by:
Haichang Zhang, Qingdao University of Science and Technology, ChinaReviewed by:
Xiaoli Hao, Shaanxi University of Technology, ChinaYiqun Wang, Chengdu University of Technology, China
Copyright © 2022 Zhang, Pei, Wei, Tan, Gao, Bao and Qin. This is an open-access article distributed under the terms of the Creative Commons Attribution License (CC BY). The use, distribution or reproduction in other forums is permitted, provided the original author(s) and the copyright owner(s) are credited and that the original publication in this journal is cited, in accordance with accepted academic practice. No use, distribution or reproduction is permitted which does not comply with these terms.
*Correspondence: Daohai Zhang, zhangdaohai6235@163.com; Chengtao Gao, chengtaogao@163.com; Dongmei Bao, dongtian1314521@163.com; Shuhao Qin, qinshuhao@126.com