- Centro NanoMat & Área Física, Departamento de Experimentación y Teoría de la Estructura de la Materia y Sus Aplicaciones (DETEMA), Facultad de Química, Universidad de la República, Montevideo, Uruguay
The main goal of this mini-review is to provide an updated state-of-the-art of the hybrid organic-inorganic materials focusing mainly on interface phenomena involving ionic and electronic transport properties. First, we review the most relevant preparation techniques and the structural features of hybrid organic-inorganic materials prepared by solution-phase reaction of inorganic/organic precursor into organic/inorganic hosts and vapor-phase infiltration of the inorganic precursor into organic hosts and molecular layer deposition of organic precursor onto the inorganic surface. Particular emphasis is given to the advances in joint experimental and theoretical studies discussing diverse types of computational simulations for hybrid-organic materials and interfaces. We make a specific revision on the separately ionic, and electronic transport properties of these hybrid organic-inorganic materials focusing mostly on interface phenomena. Finally, we deepen into mixed ionic-electronic transport properties and provide our concluding remarks and give some perspectives about this growing field of research.
Taxonomy and Definitions
Hybrid materials were previously defined by the IUPAC (International Union of Pure and Applied Chemistry) as materials composed of an intimate mixture of inorganic components, organic components, or both types, which usually interpenetrate on scales of less than 1 μm (Alemán et al., 2007). This definition excludes large-sized components for which the term composite is usually used in the literature, but does not exclude those named as nano-composites (with at least one component exhibiting at least one of its dimensions in the nanoscale) which, in practice, is also considered in the previous hybrid material definition. Then, the term hybrid organic-inorganic (hOI) materials refer to multi-component compounds having at least one of their organic or inorganic component in the sub-micrometric and, more usually, in the nano-metric size domain (Judeinstein and Sanchez, 1996). hOI materials can be classified into two classes named Class I (when organic and inorganic phases interact weakly via Van der Waals, hydrogen bonding, or electrostatic interactions) and Class II (when organic and inorganic phases interact strongly via chemical bonding interactions), without excluding those interacting simultaneously via both types of interactions (Class I and II) (Judeinstein and Sanchez, 1996). Although the previous definitions are more general, in the vast literature hOI materials refer mostly to polymers as organic material and metal oxide compounds as inorganic materials. The term hybrid organic-inorganic polymers has also been used in the literature mostly referring to organic polymers and inorganic materials blended by mutual dispersion at molecular dimensions (Schmidt, 1992; Saegusa, 1995). This terminology can be useful as it excludes the inorganic materials in the form of inorganic particles or nanoparticles and refers mostly to inorganic clusters on the molecular or macromolecular scale. However, other authors simply refer to the laters by using the hOI materials terminology as it still fits perfectly to its definition which does not specify a lower limit for inorganic components size (Sanchez and In, 1992; Sanchez and Ribot, 1993; Judeinstein and Sanchez, 1996). Our major focus in this mini-review will be given to organic-inorganic interface phenomena for hOI materials belonging to both Class I and Class II but only those involving organic polymers and metal oxide nanomaterials.
Preparation, Characterization and Computational Simulation of hOI Materials and Interfaces
Solution-Phase Reaction of Inorganic/Organic Precursor Using Organic/Inorganic Hosts
The solution-phase reaction of inorganic precursors into organic hosts such as organic polymers was first reported in the early nineties (Sanchez and In, 1992; Schmidt, 1992; Sanchez and Ribot, 1993; Saegusa, 1995). The mechanism for metal alkoxides hydrolysis-condensation towards metal oxide clusters or particles (widely known as the sol-gel method) has been thoroughly studied since the late sixties and it is quite well-understood (Livage et al., 1988; Brinker and Scherrer, 1989). Furthermore, the solution-phase reaction of inorganic precursors (metal alkyl or alkoxide) in the presence of organic polymers essentially follows the ordinary hydrolysis-condensation mechanism. However, the presence of certain functional groups in the polymer structure can interact via non-chemical (Class I) and chemical (Class II) bonding interactions with the metal alkoxide hydrolysis-condensation products at different stages such as the case depicted in Figure 1A—left panel.
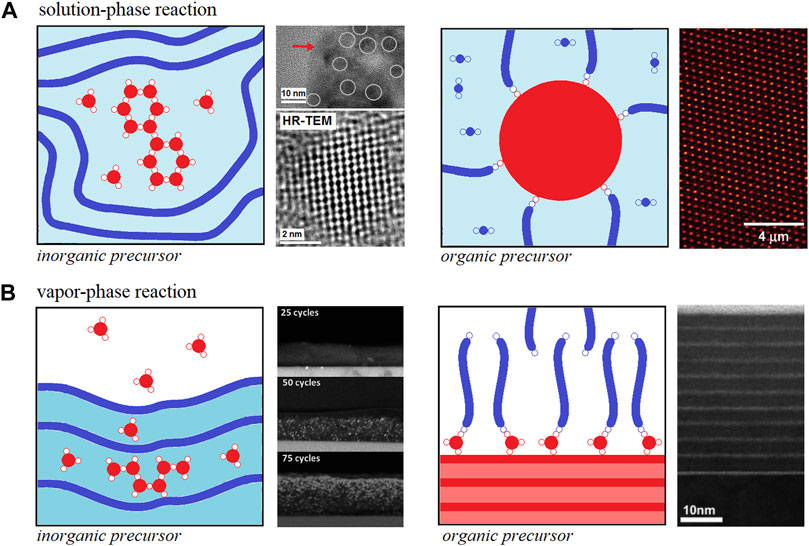
FIGURE 1. Schematic representation for (A) solution-phase and (B) vapor phase reactions to obtain hOI materials. Both cases include the preparation of hOI materials at expenses of inorganic precursors growth in the presence of organic polymer host (left panels) and using organic precursors polymerization in the presence of inorganic clusters/nanoparticles or surfaces (right panels). Each panel also includes: HRTEM images for PANI-TiO2 prepared via solution-phase reaction of titanium propoxide and polyaniline with further exposure to water vapor (A, left panel) [Portions of figures adapted/altered minimally with permission from Elsevier] (Mombrú et al., 2017a), confocal laser scanning microscopic images for PMMA-SiO2 prepared via surface-initiated atom transfer radical polymerization of MMA (A, right panel) [Portion of figure adapted/altered minimally with permission from American Chemical Society] (Ohno et al., 2006), Cross-section HRSEM images for P3HT-ZnO films obtained after 25, 50, 75 diethylzinc/water ALD cycles (B, left panel) [Portions of figure adapted/altered minimally with permission from Royal Society of Chemistry] (Obuchovsky et al., 2014). Cross-section HRTEM images of self-assembled organic multilayer/TiO2 nanolaminate films made by using sequential ALD/MLD cycles of titanium isopropoxide and 7-octenyltrichlorosilane vapors, respectively (B, right panel) [Portion of figure adapted/altered minimally with permission from American Chemical Society] (Lee et al., 2007).
On the other hand, the solution-phase reaction of organic precursor (organic monomer or oligomer) in the presence of inorganic hosts is a quite different process as the organic polymerization is the main reaction taking place. In this case, the inorganic hosts are usually nanoparticles or well-defined clusters interacting via non-chemical interactions during the organic polymerization (Class I) but these nanoparticles or well-defined clusters can be also pre- or post-functionalized with the organic monomer or oligomer to act as an initiator for organic polymerization (Class II) such as the case depicted in Figure 1A—right panel (Ohno et al., 2006). The mechanism behind hOI materials preparation is much more complex and will not be discussed further in this mini-review but the reader can refer to some outstanding reviews on this topic in the literature (Saegusa, 1995; Judeinstein and Sanchez, 1996; Sanchez et al., 2001; Krasia-Christoforou, 2015; Zhang et al., 2016a; Gon et al., 2017).
Vapor-Phase Infiltration of the Inorganic Precursor Into Organic Hosts and Molecular Layer Deposition of Organic Precursor Onto the Inorganic Surface
Over the past decade, a new approach to obtain hOI materials has emerged in which organic polymers are exposed to metalorganic vapors using different approaches such as multiple pulsed infiltration, (Lee et al., 2009; Lee et al., 2010), sequential infiltration synthesis (Peng et al., 2011; Tseng et al., 2011; Segal-Peretz et al., 2015) and sequential vapor infiltration (Gong et al., 2011; Dandley et al., 2014; Akyildiz et al., 2014; Obuchovsky et al., 2014). These approaches essentially respond to the same general mechanism involving the diffusion of inorganic precursor (metal alkyl or alkoxide) molecules into a “dried” organic polymer yielding to Class I and Class II hOI materials and, thus, the unifying term vapor-phase infiltration (VPI) processes and its corresponding reaction mechanisms have been recently proposed to describe all of them (Leng and Losego, 2017). VPI into different kinds of organic polymers [e.g. silk, collagen, polymethyl methacrylate (PMMA), polystyrene (PS), polybutylene terephthalate (PBT), polyethylene terephthalate (PET), polylactic acid (PLA) and polyethylene naphthalate (PEN)] using trimethylaluminum, diethylzinc and titanium isopropoxide precursors have proven to be successful routes toward hybrid polymers with Al2O3, TiO2 and ZnO interesting nanostructures such as the case depicted in Figure 1B—left panel (Lee et al., 2009; Lee et al., 2010; Gong et al., 2011; Peng et al., 2011; Tseng et al., 2011; Akyildiz et al., 2014; Dandley et al., 2014; Obuchovsky et al., 2014; Segal-Peretz et al., 2015; Leng and Losego, 2017).
On the other hand, the vapor-phase infiltration of organic precursors (organic monomer or oligomer) into “dried” inorganic hosts is not referred to as such in the literature as it is mostly limited to surface phenomena and the term molecular layer deposition (MLD) of organic precursor onto the inorganic surface is defined instead (Gregorczyk and Knez, 2016; Meng, 2017). MLD of organic precursors onto inorganic surfaces is a sister of the atomic layer deposition (ALD) technique but still much less explored due to the complexity of its mechanism (Gregorczyk and Knez, 2016; Meng, 2017). However, the successful advances using MLD for obtaining nanoscale films of organic polymers (Yoshimura et al., 1991; Yoshimura et al., 1992; Kim et al., 2005; Zhou et al., 2013; Atanasov et al., 2014; Kim et al., 2015) have also encouraged the preparation of hOI materials such as metal-based hybrid polymers named as alucones, zincones, titanicones among others (Dameron et al., 2008; Lee et al., 2007; Peng et al., 2009; Abdulagatov et al., 2013; Yoon et al., 2012; Park et al., 2016; Van de Kerckhove et al., 2016; Nisula and Karppinen, 2016). In the latter case, the host inorganic surface reacts with the metal-organic precursor vapor and once it is grafted to the surface it allows further organic oligomerization processes and, that is probably why most cases refer to Class II hOI materials such as the case depicted in Figure 1B—right panel (Yoshimura et al., 1991; Yoshimura et al., 1992; Kim et al., 2005; Lee et al., 2007; Dameron et al., 2008; Peng et al., 2009; Yoon et al., 2012; Abdulagatov et al., 2013; Zhou et al., 2013; Atanasov et al., 2014; Kim et al., 2015; Nisula and Karppinen, 2016; Park et al., 2016; Van de Kerckhove et al., 2016; Meng, 2017).
Computational Simulation Insights on Structural Features of hOI Materials and Interfaces
Computational simulation is quite relevant to predict or explain structural features of hOI materials and interfaces. For instance, classical Montecarlo (MC) and molecular dynamics (MD) are one of the best (non-atomistic and atomistic, respectively) approaches to model up to several nanometer large crystalline or amorphous organic and inorganic materials allowing obtaining rich information about molecular and nuclear position-related properties (Zeng et al., 2003; Heinz and Ramezani-Dakhel, 2016; Ramakrishnan et al., 2017; Eckert et al., 2020). To compute electronic-related properties, we must perform first-principles calculations such as Density Functional Theory (DFT) and ab-initio MD (AIMD) to access static and dynamic information, respectively (Zeng et al., 2003; Heinz and Ramezani-Dakhel, 2016; Ramakrishnan et al., 2017; Eckert et al., 2020). When performing first-principles calculations, due to the complexity of the hOI interface, one of the organic or inorganic phases is usually oversimplified to systems comprising organic monomer/inorganic surface (Alexandre et al., 2010; Semoto et al., 2011; Hofmann et al., 2013; Motta et al., 2015; Wang et al., 2017a; Pourrahimi et al., 2017; Liao et al., 2019) or organic oligomers/inorganic small clusters (Mombrú et al., 2017a; Ullah et al., 2017; Wang et al., 2020a). The rational simplification of the hOI interface model is a key to computing these calculations using an appropriate level of theory at a reasonable computational cost (Hofmann et al., 2021).
There are a lot of experimental characterization techniques to study hOI materials and interfaces such as nuclear magnetic resonance, X-ray photoemission, vibrational and optical spectroscopies to access chemical features and high-resolution electron, atomic force and tunneling microscopies, in addition to small/wide-angle X-ray scattering, to access structural features of hOI interfaces. Probably because of their popularity, infrared and Raman spectroscopies are the most commonly observed in the literature to study the organic-inorganic interactions mainly for Class II hOI materials (Atanasov et al., 2014; Yoon et al., 2012; Mombrú et al., 2017a; Wang et al., 2020a; Schöttner et al., 2020). Moreover, surface-enhanced Raman scattering (SERS) effect without using noble metals but inorganic (e.g. TiO2, MoO3, WO3, NiO, ZnO and Cu2O) (Sun et al., 2007; Alessandri, 2013; Bontempi et al., 2014; Qi et al., 2014; Shin et al., 2014; Wang et al., 2014; Cong et al., 2015; Bontempi et al., 2016; Wang et al., 2017b; Lin et al., 2017; Wu et al., 2017; Zhang et al., 2017; Demirel et al., 2018) or organic (e.g. graphene, thiophene oligomers) (Ling and Zhang, 2010; Yu et al., 2011; Ling et al., 2012; Ling et al., 2013; Huang et al., 2015; Kang et al., 2015; Zhang et al., 2016b; Lombardi, 2017; Yilmaz et al., 2017; Liu et al., 2018) semiconductors is rising and appears to be a very promising technique to study hOI interfaces. Not only there is a vast experimental database that can be useful for vibrational modes assignment but also infrared and, to a lesser extent, Raman spectra can be easily computed using first-principles calculations (Mombrú et al., 2017a; Wang et al., 2020a; Schöttner et al., 2020; Fernández-Werner et al., 2017; Pignanelli et al., 2017). Undoubtedly, nuclear magnetic resonance and X-ray photoelectron spectroscopies are other powerful tools to obtain chemical information about hOI interfaces but are less accessible than vibrational spectroscopies and computational calculations demand a high computational cost. High-resolution electron, atomic force and tunneling microscopies can give direct local information about hOI materials and interfaces but it is almost impossible to access a large amount of sample as in the case of using X-ray scattering techniques, thus both techniques should be complemented. Furthermore, the modeling of X-ray scattering data by using Rietveld (for crystalline) or Debye (for both crystalline and amorphous) methodologies are quite simple to perform and can be also assisted by classical molecular dynamics calculations (Scardi and Gelisio, 2016; Fernández-Werner et al., 2017; Pignanelli et al., 2017; Bokuniaeva and Vorokh, 2019; Bertolotti et al., 2020).
Mixed Ionic-Electronic Transport of hOI Materials and Interfaces
Ionic Transport
Since the early nineties, it has been evidenced that the presence of inorganic nanomaterials into polymer matrices increases the ionic conductivity and improves the mechanical properties of solid polymer electrolytes (Croce et al., 1998; Appetecchi et al., 1998; Croce et al., 2000; Serra Moreno et al., 2014). Although most of these cases involve no chemical bonding between inorganic nanomaterials and polymers, there is a vast variety of inorganic nanomaterials morphologies such as nanoparticles, nanorods and nanotubes acting as both passive and active fillers that have yielded an enhancement on the ionic conductivities (Romero et al., 2015; Liu et al., 2015; Pignanelli et al., 2018a; Pignanelli et al., 2018b). The enhancement of ionic conduction due to the presence of inorganic nanostructures is mostly related to the ionic-pair dissociation of ordinary salts mediated by the interaction with the inorganic surface, thus favoring the fixation of anionic species and favoring the cationic conduction as evidenced by Raman microscopy and schematically depicted in Figure 2A (Romero et al., 2015; Pignanelli et al., 2018a; Pignanelli et al., 2018b). There are other hOI materials involving chemical bonding between organic and inorganic oligomers that have shown an enhancement in the ionic conductivity with respect to their organic counterparts (Saikia et al., 2012; Vélez et al., 2013; Saikia et al., 2014). Other approaches using borate and carbonate salts as precursors where the “undesired” mobile anionic species are eliminated or chemically bonded to the polymer have been also reported favoring single ionic conduction of the “desired” mobile cation (Zhu et al., 2013; Zhang et al., 2014; Pignanelli et al., 2019). However, the advances on single lithium-ion conductors based on Class II hOI materials comprising well-defined inorganic clusters chemically bonded to organic polymers represent a more rational and promising approach (Onishi et al., 1996; Fujinami et al., 1997; Onishi et al., 1998; Fujinami et al., 2000; Villaluenga et al., 2013; Meyer et al., 2014; Zhao et al., 2015a; Zhao et al., 2015b; Lago et al., 2015).
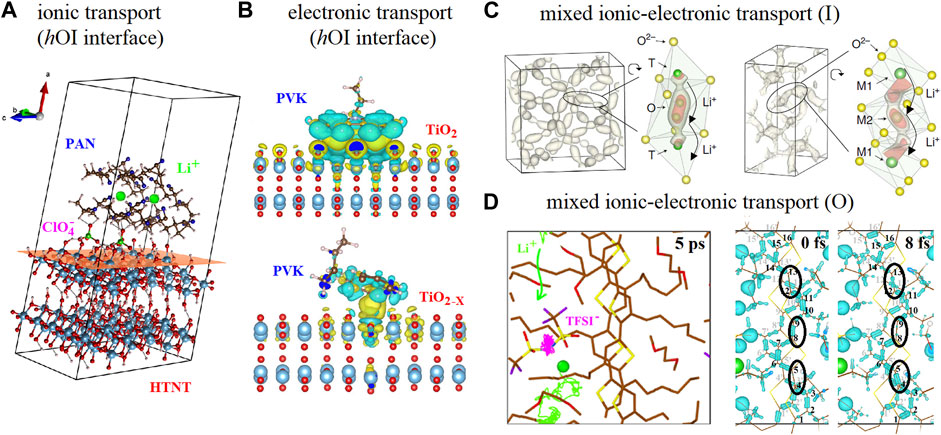
FIGURE 2. (A) AIMD calculations showing the Li+ - ClO4− ionic-pair dissociation for an hOI interface based on polyacrylonitrile (PAN) and titanate surface (HTNT) [Portion of figure adapted/altered minimally with permission from American Chemical Society] (Pignanelli et al., 2018a). (B) DFT calculations showing the electronic charge transfer interactions between vinyl carbazole monomer (PVK) and TiO2 surface including oxygen vacancies (TiO2-x) [Portion of figure adapted/altered minimally with permission from Elsevier] (Mombrú et al., 2019b). (C) AIMD calculations showing the probability density of Li+ spatial occupancy and the elongation feature of probability density along the migration channel with the corresponding isosurfaces for LLZO and LATP [Portion of figure adapted/altered minimally with permission from Nature Publishing Group] (He et al., 2017). (D) AIMD calculations showing Li+ and TFSI− transport at the P3HT side chain region mediated by glycol molecules (t < 5 ps) and the electron-phonon mediated electronic transport at the P3HT backbone (t < 20 fs) [Portion of figure adapted/altered minimally with permission from American Chemical Society] (Mombrú et al., 2022).
Regarding computational simulation studies, there is a relatively easy and low-cost DFT approach that provides insights on the interactions between ions and organic molecules/inorganic surfaces that can be used to explain dissociation processes but only represents a static picture without giving direct information about transport (Romero et al., 2016; Kwon et al., 2018; Pignanelli et al., 2019; Xie et al., 2019). To have direct insight on ionic transport for hOI materials and interfaces, larger-scale approaches using non-atomistic or atomistic calculations including organic oligomers and inorganic clusters or surfaces are necessary. MD can provide rich information about ion diffusion and transport directly from the trajectories but these calculations need to be conducted over sufficiently long time scales (Webb et al., 2015; Deng et al., 2016; Sun et al., 2016; Mogurampelly et al., 2017; Pignanelli et al., 2017; Xue et al., 2017; Patra et al., 2019; Pignanelli et al., 2021). However, such long-time scales are highly demanding for AIMD and this first-principle approach is rare in large systems (such as amorphous solids) and it is more usually performed for relatively small crystalline systems (Jalem et al., 2013; Meier et al., 2014; Mo et al., 2014). Furthermore, other first-principles calculations based on the nudged elastic band (NEB) method are also commonly used to determine the barrier of the ionic migration but it is also particularly useful for inorganic crystalline systems (Ong et al., 2011; Shi et al., 2012; Mo et al., 2014; Bachman et al., 2015; Moriwake et al., 2015; Zhang et al., 2018). Nonetheless, the vast majority of lithium-ion transport calculations are solely within the bulk of organic (Webb et al., 2015; Sun et al., 2016; Mogurampelly et al., 2017; Pignanelli et al., 2017; Xue et al., 2017; Patra et al., 2019; Pignanelli et al., 2021) or inorganic (Ong et al., 2011; Shi et al., 2012; Mo et al., 2014; Bachman et al., 2015; Moriwake et al., 2015; Deng et al., 2016; Zhang et al., 2018) phases, but still rarely for hOI materials and interfaces (Li et al., 2016; Pignanelli et al., 2018a; Li et al., 20202020). Recently, there have been interesting approaches based on AIMD and NEB calculations studying the solid electrolyte interface (SEI) comprising organic oligomers and metallic lithium electrodes (Yildirim et al., 2017; Merinov et al., 2019; Ramasubramanian et al., 2019; Merinov et al., 2020).
Electronic Transport
The electronic interactions in hOI materials have been extensively studied particularly for sensing, energy storage and energy conversion applications (Kumar et al., 2018; Singh et al., 2019; Duan et al., 2020; Niederhausen et al., 2021; Rathnayake et al., 2021; Zhang et al., 2021). The electronic interactions in these hOI materials and interfaces are mostly via proximity of the π-cloud of the organic phase with the inorganic surface, and thus Class I hOI materials are the most studied for these purposes. However, there have recent advances in Class I and II hOI materials comprising well-defined inorganic clusters with promising properties in a broad range of applications (Horn et al., 2021). One of the major challenges of hOI materials for polymer solar cells applications is the substitution of the fullerene electronic acceptors for inorganic metal oxides nanostructures counterparts in the active layer and also the enhancement of electronic transport at the hOI interface in the electrodes (Moet et al., 2007; Bouclé et al., 2008; Dridi et al., 2008; Lin et al., 2009; Chandrasekaran et al., 2011; Aashish et al., 2016; Ikram et al., 2016). The later reports comprise hOI materials based on different electronic conducting polymers with ZnO, TiO2 and In-doped SnO2 (ITO) inorganic nanostructures or surfaces (Moet et al., 2007; Bouclé et al., 2008; Dridi et al., 2008; Lin et al., 2009; Chandrasekaran et al., 2011; Aashish et al., 2016; Ikram et al., 2016). However, only a few studies have provided more fundamental insights on the electronic interactions and correlations with structural features for hOI materials and interfaces (Schlesinger et al., 2013; Whittaker-Brooks et al., 2014; Mombrú et al., 2017b; Mombrú et al., 2018; Singh et al., 2019).
As we mentioned earlier, it is quite challenging to perform computational simulation of hOI interfaces using first-principles level of theory and most calculations usually refer to organic molecules or oligomers interacting with inorganic surfaces. For instance, DFT studies on the electronic interactions of tetrafluoro-tetracyanoquinodimethane (F4-TCNQ)/ZnO surfaces (Xu et al., 2013), hexafluoro-tetracyano-naphthoquinodimethane (F6-TCNNQ)/ZnO surfaces (Schöttner et al., 2020), oligothiophenes and ZnO (and In-doped SnO2) surfaces (Timpel et al., 2020) has shed some light on the work function and other relevant electronic properties of these hOI interfaces. Other DFT studies on the electronic interactions between larger and more complex systems such as polyaniline oligomer/graphene oxide quantum dots (GQD) including edge functionalization (Mombrú et al., 2017c) and vinyl carbazole monomer/TiO2 surfaces including oxygen and titanium vacancy defects (Mombrú et al., 2019a; Mombrú et al., 2019b) has shown interesting features on the electronic properties evidencing the relevance of using more realistic models as depicted in Figure 2B. For instance, the difference between the LUMO level of vinyl carbazole and the conduction band of TiO2 surface with oxygen vacancies decreases thus favoring the energy barrier associated with the charge injection at this hOI interface (Mombrú et al., 2019b).
Mixed Ionic-Electronic Transport
There are a lot of recent examples of hOI materials with promising mixed ionic-electronic transport properties in different applications such as lithium and sodium-ion battery electrode (Sengodu and Deshmukh, 2015) and bio-electronic materials (Kousseff et al., 2022; Kukhta et al., 2022). There are also recent reviews on the most relevant and popular techniques that can be useful to characterize experimentally the mixed ionic-electronic transport in hOI materials (Romero et al., 2020; Wu et al., 2022). However, there are still very few reports dealing with computational simulation approaches of mixed ionic-electronic transport of hOI materials and interfaces mainly due to their extremely demanding computational cost. In the last years, there have been some advances but only for isolated inorganic (Nakayama et al., 2012; Suthirakun et al., 2014; He et al., 2017; Taylor et al., 2017; Kim et al., 20182019; Parras et al., 2018; Wind et al., 2018; Griffith et al., 2019; Heifets et al., 2019; Kang et al., 20192019; Wang et al., 2020b) and organic (Dong et al., 2019; Matta et al., 2020; Mombrú et al., 2020; Dong et al., 2021; Onorato et al., 2021; Zozoulenko et al., 2021; Mombrú et al., 2022) phases revealing quite interesting features.
For inorganic materials, the mixed ionic-electronic transport has been thoroughly studied for solid oxide combustible cells electrode materials (Sunarso et al., 2008) and DFT calculations have recently evidenced the simultaneous positional rearrangement of localized electrons during the oxygen vacancy ionic jump process following a concerted mechanism (Nakayama et al., 2012; Taylor et al., 2017). However, experimental and computational simulations on the mixed ionic-electronic transport in inorganic materials based on protons, lithium, sodium and potassium ions have been explored just recently (He et al., 2017; Wind et al., 2018; Griffith et al., 2019; Kang et al., 20192019; Wang et al., 2020b). Analyzing Li + dynamics from AIMD simulations, He et al have evidenced that most Li ions migrate in a highly concerted fashion which means that multiple ions hop simultaneously into their nearest sites within a few picoseconds as depicted in Figure 2C (He et al., 2017). Griffith et al have just evidenced by DFT calculations that, Li+ in TiNb2O7 with high states of lithiation exhibits a transition from interstitial-mediated to a vacancy-mediated diffusion mechanism, and the vacancy formation energetics may become rate-determining (Griffith et al., 2019). Within the same framework, the diffusion of Na+, K+, and Mg2+ was also examined in these inorganic structures and these cations exhibit very high diffusion barriers suggesting minimal ionic conduction at room temperature (Griffith et al., 2019). However, in the later report authors remark that they have used standard DFT to capture the mechanisms associated with ionic diffusion without the presence of simultaneous additional electron transfer processes and that it would be also important to further consider the full compositional range, not only the host structure and/or end point (Griffith et al., 2019).
For organic materials, due to their typical amorphous and/or crystalline nature, larger systems including the evaluation of these structural features are usually studied by MD calculations, at least to evaluate their effects on the isolated ionic or (indirectly) electronic transport (Dong et al., 2019; Mombrú et al., 2020; Dong et al., 2021; Onorato et al., 2021). Furthermore, MD simulations have been useful to evidence that, upon swelling cations interact with the polymer side chains while upon doping the excess anions penetrating the polymer microstructure are expected to be more closely interacting with the polymer backbone, stabilizing polarons (Matta et al., 2020; Cendra et al., 2019; Flagg et al., 2018; Inal et al., 2016; Savva et al., 2019). A quite challenging simultaneous access to ionic and electronic transport by AIMD calculations has been reported just recently for a system comprising crystalline P3HT polymer using explicit LiTFSI dopant and glycol molecules depicted in Figure 2D (Mombrú et al., 2022). In the later report, both ionic and electronic transport simultaneous calculations showed a good correlation with the experimental reports of similar mixed ionic−electronic conductors. Furthermore, these AIMD calculations have also allowed introducing the role of the explicit dopant in the interchain, intrachain, “effective” doping, and charge-transfer complex bonding distances, and their associated static and dynamic disorder effects on electronic transport (Mombrú et al., 2022).
Final Remarks and Perspectives
To make some final comments and define some perspectives, we conclude that, although quite computationally expensive, AIMD seems to be the best choice for modeling hOI interfaces as the mixed ionic-electronic transport of both organic and inorganic phases can be adequately modeled simultaneously and be compared with the available literature on their isolated counterparts. However, only a few of the computational simulation from those described above comprises mixed ionic-electronic transport using AIMD calculations (He et al., 2017; Taylor et al., 2017; Wind et al., 2018; Mombrú et al., 2022) so more efforts are definitively still needed in this field. It is also important to mention that the mixed ionic-electronic transport of hOI materials is much more complex as it includes crystalline and amorphous regions well above the nm scale that be no longer accessed through atomistic models. Furthermore, relevant processes in experimental devices occurring on the μm scale are out of reach for quantum chemistry and molecular dynamics, and thus continuum models also play an important role here to provide understanding at this level (Zozoulenko et al., 2021).
Author Contributions
MR, RF, and AM contributed to the conception and design of the study. DM and FP selected, compiled, and organized the literature references database. MR created the schematizations, adaptation of figure artwork, and wrote the first draft of the manuscript. DM, FP, RF, and AM wrote sections of the manuscript. All authors contributed to manuscript revision, read, and approved the submitted version.
Conflict of Interest
The authors declare that the research was conducted in the absence of any commercial or financial relationships that could be construed as a potential conflict of interest.
Publisher’s Note
All claims expressed in this article are solely those of the authors and do not necessarily represent those of their affiliated organizations, or those of the publisher, the editors and the reviewers. Any product that may be evaluated in this article, or claim that may be made by its manufacturer, is not guaranteed or endorsed by the publisher.
Acknowledgments
The authors wish to thank the support of Uruguayan CSIC, ANII, and PEDECIBA institutions.
References
Aashish, A., Ramakrishnan, R., Sudha, J. D., Sankaran, M., and Krishnapriya, G. (2016). Self-assembled Hybrid Polyvinylcarbazole-Titania Nanotubes as an Efficient Photoanode for Solar Energy Harvesting. Solar Energ. Mater. Solar Cell 151, 169–178. doi:10.1016/j.solmat.2016.03.007
Abdulagatov, A. I., Terauds, K. E., Travis, J. J., Cavanagh, A. S., Raj, R., and George, S. M. (2013). Pyrolysis of Titanicone Molecular Layer Deposition Films as Precursors for Conducting TiO2/Carbon Composite Films. J. Phys. Chem. C 117 (34), 17442–17450. doi:10.1021/jp4051947
Akyildiz, H. I., Lo, M., Dillon, E., Roberts, A. T., Everitt, H. O., and Jur, J. S. (2014). Formation of Novel Photoluminescent Hybrid Materials by Sequential Vapor Infiltration into Polyethylene Terephthalate Fibers. J. Mater. Res. 29, 2817–2826. doi:10.1557/jmr.2014.333
Alemán, J., Chadwick, A. V., He, J., Hess, M., Horie, K., Jones, R. G., et al. (2007). Definitions of Terms Relating to the Structure and Processing of Sols, Gels, Networks, and Inorganic–Organic Hybrid Materials (IUPAC Recommendations 2007). Pure Appl. Chem. 79, 1801–1829. doi:10.1515/ci.2008.30.1.27b
Alessandri, I. (2013). Enhancing Raman Scattering without Plasmons: Unprecedented Sensitivity Achieved by TiO2 Shell-Based Resonators. J. Am. Chem. Soc. 135, 5541–5544. doi:10.1021/ja401666p
Alexandre, Tkatchenko., Romaner, Lorenz., Hofmann, Oliver. T., Zojer, Egbert., Ambrosch-Draxl, Claudia., and Scheffler, Matthias. (2010). Van der Waals Interactions Between Organic Adsorbates and at Organic/Inorganic Interfaces. MRS Bull. 35, 435–442. doi:10.1557/mrs2010.581
Appetecchi, G. B., Croce, F., Dautzenberg, G., Mastragostino, M., Ronci, F., Scrosati, B., et al. (1998). Composite Polymer Electrolytes with Improved Lithium Metal Electrode Interfacial Properties: I. Elechtrochemical Properties of Dry PEO‐LiX Systems. J. Electrochem. Soc. 145, 4126–4132. doi:10.1149/1.1838925
Atanasov, S. E., Losego, M. D., Gong, B., Sachet, E., Maria, J.-P., Williams, P. S., et al. (2014). Highly Conductive and Conformal Poly(3,4-Ethylenedioxythiophene) (PEDOT) Thin Films via Oxidative Molecular Layer Deposition. Chem. Mater. 26 (11), 3471–3478. doi:10.1021/cm500825b
Bachman, J. C., Muy, S., Grimaud, A., Chang, H.-H., Pour, N., Lux, S. F., et al. (2015). Inorganic Solid-State Electrolytes for Lithium Batteries: Mechanisms and Properties Governing Ion Conduction. Chem. Rev. 116 (1), 140–162. doi:10.1021/acs.chemrev.5b00563
Bertolotti, F., Vivani, A., Moscheni, D., Ferri, F., Cervellino, A., Masciocchi, N., et al. (2020). Structure, Morphology, and Faceting of TiO2 Photocatalysts by the Debye Scattering Equation Method. The P25 and P90 Cases of Study. Nanomaterials 10 (4), 743. doi:10.3390/nano10040743
Bokuniaeva, A. O., and Vorokh, A. S. (2019). Estimation of Particle Size Using the Debye Equation and the Scherrer Formula for Polyphasic TiO2 Powder. J. Phys. Conf. Ser. 1410, 012057. doi:10.1088/1742-6596/1410/1/012057
Bontempi, N., Carletti, L., De Angelis, C., and Alessandri, I. (2016). Plasmon-free SERS Detection of Environmental CO2on TiO2surfaces. Nanoscale 8, 3226–3231. doi:10.1039/c5nr08380j
Bontempi, N., Salmistraro, M., Ferroni, M., Depero, L. E., and Alessandri, I. (2014). Probing the Spatial Extension of Light Trapping-Induced Enhanced Raman Scattering in High-Density Si Nanowire Arrays. Nanotechnology 25, 465705. doi:10.1088/0957-4484/25/46/465705
Bouclé, J., Chyla, S., Shaffer, M. S. P., Durrant, J. R., Bradley, D. D. C., and Nelson, J. (2008). Hybrid Solar Cells from a Blend of Poly(3-Hexylthiophene) and Ligand-Capped Tio2 Nanorods. Adv. Funct. Mater. 18, 622–633. doi:10.1002/adfm.200700280
Brinker, C. J., and Scherrer, G. (1989). Sol-Gel Science, the Physics and Chemistry of Sol-Gel Processing. San-Diego: Academic Press.
Cendra, C., Giovannitti, A., Savva, A., Venkatraman, V., McCulloch, I., Salleo, A., et al. (2019). Role of the Anion on the Transport and Structure of Organic Mixed Conductors. Adv. Funct. Mater. 29, 1807034. doi:10.1002/adfm.201807034
Chandrasekaran, J., Nithyaprakash, D., Ajjan, K. B., Maruthamuthu, S., Manoharan, D., and Kumar, S. (2011). Hybrid Solar Cell Based on Blending of Organic and Inorganic Materials-An Overview. Renew. Sust. Energ. Rev. 15 (2), 1228–1238. doi:10.1016/j.rser.2010.09.017
Cong, S., Yuan, Y., Chen, Z., Hou, J., Yang, M., Su, Y., et al. (2015). Noble Metal-Comparable SERS Enhancement from Semiconducting Metal Oxides by Making Oxygen Vacancies. Nat. Commun. 6, 7800. doi:10.1038/ncomms8800
Croce, F., Appetecchi, G. B., Persi, L., and Scrosati, B. (1998). Nanocomposite Polymer Electrolytes for Lithium Batteries. Nature 394, 456–458. doi:10.1038/28818
Croce, F., Persi, L., Ronci, F., and Scrosati, B. (2000). Nanocomposite Polymer Electrolytes and Their Impact on the Lithium Battery Technology. Solid State Ionics 135, 47–52. doi:10.1016/s0167-2738(00)00329-5
Dameron, A. A., Seghete, D., Burton, B. B., Davidson, S. D., Cavanagh, A. S., Bertrand, J. A., et al. (2008). Molecular Layer Deposition of Alucone Polymer Films Using Trimethylaluminum and Ethylene Glycol. Chem. Mater. 20 (10), 3315–3326. doi:10.1021/cm7032977
Dandley, E. C., Needham, C. D., Williams, P. S., Brozena, A. H., Oldham, C. J., and Parsons, G. N. (2014). Temperature-dependent Reaction between Trimethylaluminum and Poly(methyl Methacrylate) during Sequential Vapor Infiltration: Experimental and Ab Initio Analysis. J. Mater. Chem. C 2, 9416–9424. doi:10.1039/c4tc01293c
Demirel, G., Usta, H., Yilmaz, M., Celik, M., Alidagi, H. A., and Buyukserin, F. (2018). Surface-enhanced Raman Spectroscopy (SERS): an Adventure from Plasmonic Metals to Organic Semiconductors as SERS Platforms. J. Mater. Chem. C 6, 5314–5335. doi:10.1039/c8tc01168k
Deng, Z., Mo, Y., and Ong, S. P. (2016). Computational Studies of Solid-State Alkali Conduction in Rechargeable Alkali-Ion Batteries. NPG Asia Mater. 8, e254. doi:10.1038/am.2016.7
Dong, B. X., Nowak, C., Onorato, J. W., Ma, T., Niklas, J., Poluektov, O. G., et al. (2021). Complex Relationship between Side-Chain Polarity, Conductivity, and Thermal Stability in Molecularly Doped Conjugated Polymers. Chem. Mater. 33, 741–753. doi:10.1021/acs.chemmater.0c04153
Dong, B. X., Nowak, C., Onorato, J. W., Strzalka, J., Escobedo, F. A., Luscombe, C. K., et al. (2019). Influence of Side-Chain Chemistry on Structure and Ionic Conduction Characteristics of Polythiophene Derivatives: A Computational and Experimental Study. Chem. Mater. 31, 1418–1429. doi:10.1021/acs.chemmater.8b05257
Dridi, C., Barlier, V., Chaabane, H., Davenas, J., and Ben Ouada, H. (2008). Investigation of Exciton Photodissociation, Charge Transport and Photovoltaic Response of poly(N-Vinyl carbazole):TiO2nanocomposites for Solar Cell Applications. Nanotechnology 19, 375201. doi:10.1088/0957-4484/19/37/375201
Duan, Bin., Huo, Wangchen., Yuan, Yingbo., Huang, Jianhang., Liu, Yao., Zhang, Yuxin., et al. (2020). Organic-Inorganic-Induced Polymer Intercalation into Layered Composites for Aqueous Zinc-Ion Battery. Chem 6, 968–984.
Eckert, H., Montagna, M., Dianat, A., Gutierrez, R., Bobeth, M., and Cuniberti, G. (2020). Exploring the Organic-Inorganic Interface in Biosilica: Atomistic Modeling of Polyamine and Silica Precursors Aggregation Behavior. BMC Mat 2, 6. doi:10.1186/s42833-020-00012-z
Fernández-Werner, L., Pignanelli, F., Montenegro, B., Romero, M., Pardo, H., Faccio, R., et al. (2017). Characterization of Titanate Nanotubes for Energy Applications. J. Energ. Storage 12, 66–77. doi:10.1016/j.est.2017.04.002
Flagg, L. Q., Giridharagopal, R., Guo, J., and Ginger, D. S. (2018). Anion-Dependent Doping and Charge Transport in Organic Electrochemical Transistors. Chem. Mater. 30, 5380–5389. doi:10.1021/acs.chemmater.8b02220
Fujinami, T., Mehta, M. A., Sugie, K., and Mori, K. (2000). Molecular Design of Inorganic-Organic Hybrid Polyelectrolytes to Enhance Lithium Ion Conductivity. Electrochimica Acta 45, 1181–1186. doi:10.1016/s0013-4686(99)00379-5
Fujinami, T., Tokimune, A., Mehta, M. A., Shriver, D. F., and Rawsky, G. C. (1997). Siloxyaluminate Polymers with High Li+ Ion Conductivity. Chem. Mater. 9, 2236–2239. doi:10.1021/cm970399z
Gon, M., Tanaka, K., and Chujo, Y. (2017). Creative Synthesis of Organic-Inorganic Molecular Hybrid Materials. Bcsj 90, 463–474. doi:10.1246/bcsj.20170005
Gong, B., Peng, Q., Jur, J. S., Devine, C. K., Lee, K., and Parsons, G. N. (2011). Sequential Vapor Infiltration of Metal Oxides into Sacrificial Polyester Fibers: Shape Replication and Controlled Porosity of Microporous/Mesoporous Oxide Monoliths. Chem. Mater. 23, 3476–3485. doi:10.1021/cm200694w
Gregorczyk, K., and Knez, M. (2016). Hybrid Nanomaterials through Molecular and Atomic Layer Deposition: Top Down, Bottom up, and In-Between Approaches to New Materials. Prog. Mater. Sci. 75, 1–37. doi:10.1016/j.pmatsci.2015.06.004
Griffith, K. J., Seymour, I. D., Hope, M. A., Butala, M. M., Lamontagne, L. K., Preefer, M. B., et al. (2019). Ionic and Electronic Conduction in TiNb2O7. J. Am. Chem. Soc. 141 (42), 16706–16725. doi:10.1021/jacs.9b06669
He, X., Zhu, Y., and Mo, Y. (2017). Origin of Fast Ion Diffusion in Super-ionic Conductors. Nat. Commun. 8, 15893. doi:10.1038/ncomms15893
Heifets, E., Kotomin, E. A., Bagaturyants, A. A., and Maier, J. (2019). Thermodynamic Stability of Non-stoichiometric SrFeO3−δ: a Hybrid DFT Study. Phys. Chem. Chem. Phys. 21, 3918–3931. doi:10.1039/c8cp07117a
Heinz, H., and Ramezani-Dakhel, H. (2016). Simulations of Inorganic-Bioorganic Interfaces to Discover New Materials: Insights, Comparisons to experiment, Challenges, and Opportunities. Chem. Soc. Rev. 45, 412–448. doi:10.1039/c5cs00890e
Hofmann, O. T., Atalla, V., Moll, N., Rinke, P., and Scheffler, M. (2013). Interface Dipoles of Organic Molecules on Ag(111) in Hybrid Density-Functional Theory. New J. Phys. 15, 123028. doi:10.1088/1367-2630/15/12/123028
Hofmann, O. T., Zojer, E., Hörmann, L., Jeindl, A., and Maurer, R. J. (2021). First-principles Calculations of Hybrid Inorganic-Organic Interfaces: from State-Of-The-Art to Best Practice. Phys. Chem. Chem. Phys. 23, 8132–8180. doi:10.1039/d0cp06605b
Horn, M. R., Singh, A., Alomari, S., Goberna-Ferron, S., Benages-Vilau, R., Chodankar, N., et al. (2021). Polyoxometalates (POMs): from Electroactive Clusters to Energy Materials. Energy Environ. Sci. 14, 1652–1700. doi:10.1039/d0ee03407j
Huang, S., Ling, X., Liang, L., Song, Y., Fang, W., Zhang, J., et al. (2015). Molecular Selectivity of Graphene-Enhanced Raman Scattering. Nano Lett. 15, 2892–2901. doi:10.1021/nl5045988
Ikram, M., Murray, R., Imran, M., Ali, S., and Shah, S. I. (2016). Enhanced Performance of P3HT/(PCBM:ZnO:TiO 2 ) Blend Based Hybrid Organic Solar Cells. Mater. Res. Bull. 75, 35–40. doi:10.1016/j.materresbull.2015.11.031
Inal, S., Malliaras, G. G., and Rivnay, J. (2016). Optical Study of Electrochromic Moving Fronts for the Investigation of Ion Transport in Conducting Polymers. J. Mater. Chem. C 4, 3942–3947. doi:10.1039/c5tc04354a
Jalem, R., Yamamoto, Y., Shiiba, H., Nakayama, M., Munakata, H., Kasuga, T., et al. (2013). Concerted Migration Mechanism in the Li Ion Dynamics of Garnet-type Li7La3Zr2O12. Chem. Mater. 25 (3), 425–430. doi:10.1021/cm303542x
Judeinstein, P., and Sanchez, C. (1996). Hybrid Organic-Inorganic Materials: a Land of Multidisciplinarity. J. Mater. Chem. 6 (4), 511–525. doi:10.1039/jm9960600511
Kang, H., Lee, J., Rodgers, T., Shim, J.-H., and Lee, S. (20192019). Electrical Conductivity of Delithiated Lithium Cobalt Oxides: Conductive Atomic Force Microscopy and Density Functional Theory Study. J. Phys. Chem. C 123, 17703–17710. doi:10.1021/acs.jpcc.9b03232
Kang, L., Chu, J., Zhao, H., Xu, P., and Sun, M. (2015). Recent Progress in the Applications of Graphene in Surface-Enhanced Raman Scattering and Plasmon-Induced Catalytic Reactions. J. Mater. Chem. C 3, 9024–9037. doi:10.1039/c5tc01759a
Kim, A., Filler, M. A., Kim, S., and Bent, S. F. (2005). Layer-by-Layer Growth on Ge(100) via Spontaneous Urea Coupling Reactions. J. Am. Chem. Soc. 127 (16), 6123–6132. doi:10.1021/ja042751x
Kim, D. H., Atanasov, S. E., Lemaire, P., Lee, K., and Parsons, G. N. (2015). Platinum-free Cathode for Dye-Sensitized Solar Cells Using Poly(3,4-Ethylenedioxythiophene) (PEDOT) Formed via Oxidative Molecular Layer Deposition. ACS Appl. Mater. Inter. 7 (7), 3866–3870. doi:10.1021/am5084418
Kim, N., Perry, N. H., and Ertekin, E. (20182019). Atomic Modeling and Electronic Structure of Mixed Ionic-Electronic Conductor SrTi1-xFexO3-X/2+δ Considered as a Mixture of SrTiO3 and Sr2Fe2O5. Chem. Mater. 31 (1), 233–243. doi:10.1021/acs.chemmater.8b04284
Kousseff, C. J., Halaksa, R., Parr, Z. S., and Nielsen, C. B. (2022). Mixed Ionic and Electronic Conduction in Small-Molecule Semiconductors. Chem. Rev. 122 (4), 4397–4419. doi:10.1021/acs.chemrev.1c00314
Krasia-Christoforou, T. (2015). Organic-Inorganic Polymer Hybrids: Synthetic Strategies and Applications. Hybrid Hierarchical Compos. Mater., 11–63. doi:10.1007/978-3-319-12868-9_2
Kukhta, N. A., Marks, A., and Luscombe, C. K. (2022). Molecular Design Strategies toward Improvement of Charge Injection and Ionic Conduction in Organic Mixed Ionic-Electronic Conductors for Organic Electrochemical Transistors. Chem. Rev. 122 (4), 4325–4355. doi:10.1021/acs.chemrev.1c00266
Kumar, P., Zaia, E. W., Yildirim, E., Repaka, D. V. M., Yang, S.-W., Urban, J. J., et al. (2018). Polymer Morphology and Interfacial Charge Transfer Dominate over Energy-dependent Scattering in Organic-Inorganic Thermoelectrics. Nat. Commun. 9, 5347. doi:10.1038/s41467-018-07435-z
Kwon, S. J., Jung, B. M., Kim, T., Byun, J., Lee, J., Lee, S. B., et al. (2018). Influence of Al2O3 Nanowires on Ion Transport in Nanocomposite Solid Polymer Electrolytes. Macromolecules 51 (24), 10194–10201. doi:10.1021/acs.macromol.8b01603
Lago, N., Garcia-Calvo, O., Lopez del del, J. M., Rojo, T., and Armand, M. (2015). All-Solid-State Lithium-Ion Batteries with Grafted Ceramic Nanoparticles Dispersed in Solid Polymer Electrolytes. ChemSusChem 8, 3039–3043. doi:10.1002/cssc.201500783
Lee, B. H., Ryu, M. K., Choi, S.-Y., Lee, K.-H., and Sung, M. M. (2007). Rapid Vapor-phase Fabrication of Organic−Inorganic Hybrid Superlattices with Monolayer Precision. J. Am. Chem. Soc. 129, 16034–16041. doi:10.1021/ja075664o
Lee, S.-M., Pippel, E., Gösele, U., Dresbach, C., Qin, Y., Chandran, C. V., et al. (2009). Greatly Increased Toughness of Infiltrated Spider Silk. Science 324, 488–492. doi:10.1126/science.1168162
Lee, S.-M., Pippel, E., Moutanabbir, O., Gunkel, I., Thurn-Albrecht, T., and Knez, M. (2010). Improved Mechanical Stability of Dried Collagen Membrane after Metal Infiltration. ACS Appl. Mater. Inter. 2, 2436–2441. doi:10.1021/am100438b
Leng, C. Z., and Losego, M. D. (2017). Vapor Phase Infiltration (VPI) for Transforming Polymers into Organic-Inorganic Hybrid Materials: a Critical Review of Current Progress and Future Challenges. Mater. Horiz. 4, 747–771. doi:10.1039/c7mh00196g
Li, Y., Leung, K., and Qi, Y. (2016). Computational Exploration of the Li-Electrode|Electrolyte Interface in the Presence of a Nanometer Thick Solid-Electrolyte Interphase Layer. Acc. Chem. Res. 49 (10), 2363–2370. doi:10.1021/acs.accounts.6b00363
Li, Y., Sun, Z., Liu, D., Gao, Y., Wang, Y., Bu, H., et al. (20202020). A Composite Solid Polymer Electrolyte Incorporating MnO2 Nanosheets with Reinforced Mechanical Properties and Electrochemical Stability for Lithium Metal Batteries. J. Mater. Chem. A. 8, 2021–2032. doi:10.1039/c9ta11542k
Liao, C., Yang, B., Zhang, N., Liu, M., Chen, G., Jiang, X., et al. (2019). Constructing Conductive Interfaces between Nickel Oxide Nanocrystals and Polymer Carbon Nitride for Efficient Electrocatalytic Oxygen Evolution Reaction. Adv. Funct. Mater. 29, 1904020. doi:10.1002/adfm.201904020
Lin, J., Shang, Y., Li, X., Yu, J., Wang, X., and Guo, L. (2017). Ultrasensitive SERS Detection by Defect Engineering on Single Cu2O Superstructure Particle. Adv. Mater. 29, 1604797. doi:10.1002/adma.201604797
Lin, Y.-Y., Chu, T.-H., Li, S.-S., Chuang, C.-H., Chang, C.-H., Su, W.-F., et al. (2009). Interfacial Nanostructuring on the Performance of Polymer/Tio2 Nanorod Bulk Heterojunction Solar Cells. J. Am. Chem. Soc. 131, 3644–3649. doi:10.1021/ja8079143
Ling, X., Wu, J., Xie, L., and Zhang, J. (2013). Graphene-Thickness-Dependent Graphene-Enhanced Raman Scattering. J. Phys. Chem. C 117, 2369–2376. doi:10.1021/jp310564d
Ling, X., Wu, J., Xu, W., and Zhang, J. (2012). Probing the Effect of Molecular Orientation on the Intensity of Chemical Enhancement Using Graphene-Enhanced Raman Spectroscopy. Small 8, 1365–1372. doi:10.1002/smll.201102223
Ling, X., and Zhang, J. (2010). First-Layer Effect in Graphene-Enhanced Raman Scattering. Small 6, 2020–2025. doi:10.1002/smll.201000918
Liu, D., Chen, X., Hu, Y., Sun, T., Song, Z., Zheng, Y., et al. (2018). Raman Enhancement on Ultra-clean Graphene Quantum Dots Produced by Quasi-Equilibrium Plasma-Enhanced Chemical Vapor Deposition. Nat. Commun. 9, 193. doi:10.1038/s41467-017-02627-5
Liu, W., Liu, N., Sun, J., Hsu, P.-C., Li, Y., Lee, H.-W., et al. (2015). Ionic Conductivity Enhancement of Polymer Electrolytes with Ceramic Nanowire Fillers. Nano Lett. 15, 2740–2745. doi:10.1021/acs.nanolett.5b00600
Livage, J., Henry, M., and Sanchez, C. (1988). Sol-gel Chemistry of Transition Metal Oxides. Prog. Solid State. Chem. 18, 259–341. doi:10.1016/0079-6786(88)90005-2
Matta, M., Wu, R., Paulsen, B. D., Petty, A. J., Sheelamanthula, , McCulloch, I., et al. (2020). Ion Coordination and Chelation in a Glycolated Polymer Semiconductor: Molecular Dynamics and X-ray Fluorescence Study. Chem. Mater. 32, 7301–7308. doi:10.1021/acs.chemmater.0c01984
Meier, K., Laino, T., and Curioni, A. (2014). Solid-State Electrolytes: Revealing the Mechanisms of Li-Ion Conduction in Tetragonal and Cubic LLZO by First-Principles Calculations. J. Phys. Chem. C 118 (13), 6668–6679. doi:10.1021/jp5002463
Meng, X. (2017). An Overview of Molecular Layer Deposition for Organic and Organic-Inorganic Hybrid Materials: Mechanisms, Growth Characteristics, and Promising Applications. J. Mater. Chem. A. 5, 18326–18378. doi:10.1039/c7ta04449f
Merinov, B. V., Naserifar, S., Zybin, S. V., Morozov, S., Goddard, W. A., Lee, , et al. (2020). Li-diffusion at the Interface between Li-Metal and [Pyr14][TFSI]-ionic Liquid: Ab Initio Molecular Dynamics Simulations. J. Chem. Phys. 152 (3), 031101. doi:10.1063/1.5132566
Merinov, B. V., Zybin, S. V., Naserifar, S., Morozov, S., Oppenheim, J., Goddard, W. A., et al. (2019). Interface Structure in Li-Metal/[Pyr14][TFSI]-Ionic Liquid System from Ab Initio Molecular Dynamics Simulations. J. Phys. Chem. Lett. 10, 4577–4586. doi:10.1021/acs.jpclett.9b01515
Meyer, M., Vechambre, C., Viau, L., Mehdi, A., Fontaine, O., Mourad, E., et al. (2014). Single-ion Conductor Nanocomposite Organic-Inorganic Hybrid Membranes for Lithium Batteries. J. Mater. Chem. A. 2, 12162–12165. doi:10.1039/c4ta02132k
Mo, Y., Ong, S. P., and Ceder, G. (2014). Insights into Diffusion Mechanisms in P2 Layered Oxide Materials by First-Principles Calculations. Chem. Mater. 26, 5208–5214. doi:10.1021/cm501563f
Moet, D. J. D., Koster, L. J. A., de Boer, B., and Blom, P. W. M. (2007). Hybrid Polymer Solar Cells from Highly Reactive Diethylzinc: MDMO-PPV versus P3HT. Chem. Mater. 19 (24), 5856–5861. doi:10.1021/cm070555u
Mogurampelly, S., Keith, J. R., and Ganesan, V. (2017). Mechanisms Underlying Ion Transport in Polymerized Ionic Liquids. J. Am. Chem. Soc. 139 (28), 9511–9514. doi:10.1021/jacs.7b05579
Mombrú, D., Romero, M., Faccio, R., and Mombrú, Á. W. (2017). Electronic Structure of Edge-Modified Graphene Quantum Dots Interacting with Polyaniline: Vibrational and Optical Properties. J. Phys. Chem. C 121, 16576–16583. doi:10.1021/acs.jpcc.7b03604
Mombrú, D., Romero, M., Faccio, R., and Mombrú, Á. W. (2022). Ab Initio Molecular Dynamics Assessment on the Mixed Ionic−Electronic Transport for Crystalline Poly(3-Hexylthiophene) Using Full Explicit Lithium-Based Dopants and Additives. Macromolecules 55, 113–124.
Mombrú, D., Romero, M., Faccio, R., and Mombrú, Á. W. (2020). Unraveling the Lithium Bis(trifluoromethanesulfonyl)imide (LiTFSI) Doping Mechanism of Regioregular Poly(3-Hexylthiophene): Experimental and Theoretical Study. J. Phys. Chem. C 124 (13), 7061–7070. doi:10.1021/acs.jpcc.0c00407
Mombrú, D., Romero, M., Faccio, R., Tumelero, M. A., and Mombrú, A. W. (2019a). Extremely Large Magnetic-Field-Effects on the Impedance Response of TiO2 Quantum Dots. Scientific Rep. 9, 5322. doi:10.1038/s41598-019-41792-z
Mombrú, D., Romero, M., Sandoval, M. G., Faccio, R., and Mombrú, Á. W. (2019b). Role of Surface Defects on the Adsorption of Poly(9-Vinylcarbazole) on TiO2 Using the Monomer as a Donor:acceptor Model. Appl. Surf. Sci. 487, 1104–1110. doi:10.1016/j.apsusc.2019.05.213
Mombrú, D., Romero, M., Faccio, R., Castiglioni, J., and Mombrú, A. W. (2017). In Situ growth of Ceramic Quantum Dots in Polyaniline Host via Water Vapor Flow Diffusion as Potential Electrode Materials for Energy Applications. J. Solid State. Chem. 250, 60–67. doi:10.1016/j.jssc.2017.03.016
Mombrú, D., Romero, M., Faccio, R., and Mombrú, A. W. (2017). Raman and Impedance Spectroscopy under Applied Dc Bias Insights on the Electrical Transport for Donor:Acceptor Nanocomposites Based on Poly(vinyl Carbazole) and TiO2 Quantum Dots. J. Phys. Chem. C 121, 23383–23391. doi:10.1021/acs.jpcc.7b08400
Mombrú, D., Romero, M., Faccio, R., and Mombrú, A. W. (2018). Raman Microscopy Insights on the Out-Of-Plane Electrical Transport of Carbon Nanotube-Doped PEDOT:PSS Electrodes for Solar Cell Applications. J. Phys. Chem. B 122, 2694–2701. doi:10.1021/acs.jpcb.8b00317
Moriwake, H., Gao, X., Kuwabara, A., Fisher, C. A. J., Kimura, T., Ikuhara, Y. H., et al. (2015). Domain Boundaries and Their Influence on Li Migration in Solid-State Electrolyte (La,Li)TiO3. J. Power Sourc. 276, 203–207. doi:10.1016/j.jpowsour.2014.11.139
Motta, A., Cannelli, O., Boccia, A., Zanoni, R., Raimondo, M., Caldarelli, A., et al. (2015). A Mechanistic Explanation of the Peculiar Amphiphobic Properties of Hybrid Organic-Inorganic Coatings by Combining XPS Characterization and DFT Modeling. ACS Appl. Mater. Inter. 7 (36), 19941–19947. doi:10.1021/acsami.5b04376
Nakayama, M., Ohshima, H., Nogami, M., and Martin, M. (2012). A Concerted Migration Mechanism of Mixed Oxide Ion and Electron Conduction in Reduced Ceria Studied by First-Principles Density Functional Theory. Phys. Chem. Chem. Phys. 14 (17), 6079. doi:10.1039/c2cp00020b
Niederhausen, J., Mazzio, K. A., and MacQueen, R. W. (2021). Inorganic-organic Interfaces in Hybrid Solar Cells. Electron. Struct. 3, 033002. doi:10.1088/2516-1075/ac23a3
Nisula, M., and Karppinen, M. (2016). Atomic/Molecular Layer Deposition of Lithium Terephthalate Thin Films as High Rate Capability Li-Ion Battery Anodes. Nano Lett. 16 (2), 1276–1281. doi:10.1021/acs.nanolett.5b04604
Obuchovsky, S., Deckman, I., Moshonov, M., Segal Peretz, T., Ankonina, G., Savenije, T. J., et al. (2014). Atomic Layer Deposition of Zinc Oxide onto and into P3HT for Hybrid Photovoltaics. J. Mater. Chem. C 2, 8903–8910. doi:10.1039/c4tc01629g
Ohno, K., Morinaga, T., Takeno, S., Tsujii, Y., and Fukuda, T. (2006). Suspensions of Silica Particles Grafted with Concentrated Polymer Brush: A New Family of Colloidal Crystals. Macromolecules 39, 1245–1249. doi:10.1021/ma0521708
Ong, S. P., Chevrier, V. L., Hautier, G., Jain, A., Moore, C., Kim, S., et al. (2011). Voltage, Stability and Diffusion Barrier Differences between Sodium-Ion and Lithium-Ion Intercalation Materials. Energ. Environ. Sci. 4, 3680–3688. doi:10.1039/c1ee01782a
Onishi, K., Matsumoto, M., Nakacho, Y., and Shigehara, K. (1996). Synthesis of Aluminate Polymer Complexes as Single-Ionic Solid Electrolytes. Chem. Mater. 8, 469–472. doi:10.1021/cm950388a
Onishi, K., Matsumoto, M., and Shigehara, K. (1998). Thioaluminate Polymer Complexes as Single-Ionic Solid Electrolytes. Chem. Mater. 10, 927–931. doi:10.1021/cm970717u
Onorato, J. W., Wang, Z., Sun, Y., Nowak, C., Flagg, L. Q., Li, R., et al. (2021). Side Chain Engineering Control of Mixed Conduction in Oligoethylene Glycol-Substituted Polythiophenes. J. Mater. Chem. A. 9, 21410–21423. doi:10.1039/d1ta05379e
Park, Y.-S., Kim, H., Cho, B., Lee, C., Choi, S.-E., Sung, M. M., et al. (2016). Intramolecular and Intermolecular Interactions in Hybrid Organic-Inorganic Alucone Films Grown by Molecular Layer Deposition. ACS Appl. Mater. Inter. 8 (27), 17489–17498. doi:10.1021/acsami.6b01856
Parras, J. P., Genreith-Schriever, A. R., Zhang, H., Elm, M. T., Norby, T., and De Souza, R. A. (2018). Is ReO3 a Mixed Ionic-Electronic Conductor? A DFT Study of Defect Formation and Migration in a BVIO3 Perovskite-type Oxide. Phys. Chem. Chem. Phys. 20 (12), 8008–8015. doi:10.1039/c7cp08214b
Patra, S., Thakur, P., Soman, B., Puthirath, A. B., Ajayan, P. M., Mogurampelly, S., et al. (2019). Mechanistic Insight into the Improved Li Ion Conductivity of Solid Polymer Electrolytes. RSC Adv. 9, 38646–38657. doi:10.1039/c9ra08003a
Peng, Q., Gong, B., VanGundy, R. M., and Parsons, G. N. (2009). "Zincone" Zinc Oxide−Organic Hybrid Polymer Thin Films Formed by Molecular Layer Deposition. Chem. Mater. 21 (5), 820–830. doi:10.1021/cm8020403
Peng, Q., Tseng, Y.-C., Darling, S. B., and Elam, J. W. (2011). A Route to Nanoscopic Materials via Sequential Infiltration Synthesis on Block Copolymer Templates. ACS Nano 5 (6), 4600–4606. doi:10.1021/nn2003234
Pignanelli, F., Romero, M., Castiglioni, J., Faccio, R., and Mombrú, A. W. (2019). Novel Synergistic In Situ Synthesis of Lithium-Ion Poly(ethylene Citrate)-TiO2 Nanocomposites as Promising Fluorine-free Solid Polymer Electrolytes for Lithium Batteries. J. Phys. Chem. Sol. 135, 109082. doi:10.1016/j.jpcs.2019.109082
Pignanelli, F., Romero, M., Esteves, M., Fernández-Werner, L., Faccio, R., and Mombrú, A. W. (2018). Lithium Titanate Nanotubes as Active Fillers for Lithium-Ion Polyacrylonitrile Solid Polymer Electrolytes. Ionics 25, 2607–2614. doi:10.1007/s11581-018-2768-z
Pignanelli, F., Romero, M., Faccio, R., Fernández-Werner, L., and Mombrú, A. W. (2018). Enhancement of Lithium-Ion Transport in Poly(acrylonitrile) with Hydrogen Titanate Nanotube Fillers as Solid Polymer Electrolytes for Lithium-Ion Battery Applications. J. Phys. Chem. C 122, 1492–1499. doi:10.1021/acs.jpcc.7b10725
Pignanelli, F., Romero, M., Faccio, R., and Mombrú, Á. W. (2017). Experimental and Theoretical Study of Ionic Pair Dissociation in a Lithium Ion-Linear Polyethylenimine-Polyacrylonitrile Blend for Solid Polymer Electrolytes. J. Phys. Chem. B 121, 6759–6765. doi:10.1021/acs.jpcb.7b04634
Pignanelli, F., Romero, M., Faccio, R., and Mombrú, Á. W. (2021). Short- and Long-Range Structure Correlations with Ionic Transport Near the Glass Transition for Lithium-Ion Polyacrylonitrile-Based Electrolytes Using DMSO Plasticizer. J. Non-Crystalline Sol. 561, 120744. doi:10.1016/j.jnoncrysol.2021.120744
Pourrahimi, A. M., Olsson, R. T., and Hedenqvist, M. S. (2017). The Role of Interfaces in Polyethylene/Metal-Oxide Nanocomposites for Ultrahigh-Voltage Insulating Materials. Adv. Mater. 30, 1703624. doi:10.1002/adma.201703624
Qi, D., Lu, L., Wang, L., and Zhang, J. (2014). Improved SERS Sensitivity on Plasmon-free TiO2 Photonic Microarray by Enhancing Light-Matter Coupling. J. Am. Chem. Soc. 136, 9886–9889. doi:10.1021/ja5052632
Ramakrishnan, S. K., Zhu, J., and Gergely, C. (2017). Organic-inorganic Interface Simulation for New Material Discoveries. Wires Comput. Mol. Sci. 7, e1277. doi:10.1002/wcms.1277
Ramasubramanian, A., Yurkiv, V., Foroozan, T., Ragone, M., Shahbazian-Yassar, R., and Mashayek, F. (2019). Lithium Diffusion Mechanism through Solid-Electrolyte Interphase in Rechargeable Lithium Batteries. J. Phys. Chem. C 123 (16), 10237–10245. doi:10.1021/acs.jpcc.9b00436
Rathnayake, H., White, J., and Dawood, S. (2021). Polysilsesquioxane-based Organic-Inorganic Hybrid Nanomaterials and Their Applications towards Organic Photovoltaics. Synth. Met. 273, 116705. doi:10.1016/j.synthmet.2021.116705
Romero, M., Faccio, R., and Mombrú, Á. W. (2016). Novel Fluorine-free 2,2′-Bis(4,5-Dimethylimidazole) Additive for Lithium-Ion Poly(methyl Methacrylate) Solid Polymer Electrolytes. RSC Adv. 6, 67150–67156. doi:10.1039/c6ra11838k
Romero, M., Faccio, R., Vázquez, S., and Mombrú, A. W. (2015). Enhancement of Lithium Conductivity and Evidence of Lithium Dissociation for LLTO-PMMA Nanocomposite Electrolyte. Mater. Lett. 172, 1–5. doi:10.1016/j.matlet.2016.02.128
Romero, M., Mombrú, D., Pignanelli, F., Faccio, R., Mombrú, A. W., and Mombrú, (2020). Mini-Review: Mixed Ionic-Electronic Charge Carrier Localization and Transport in Hybrid Organic-Inorganic Nanomaterials. Front. Chem. 8, 537. doi:10.3389/fchem.2020.00537
Saegusa, T. (1995). Organic-inorganic Polymers Hybrids. Pure Appl. Chem. 67 (No. 12), 1965–1970. doi:10.1351/pac199567121965
Saikia, D., Pan, Y.-C., Wu, C.-G., Fang, J., Tsai, L.-D., and Kao, H.-M. (2014). Synthesis and Characterization of a Highly Conductive Organic-Inorganic Hybrid Polymer Electrolyte Based on Amine Terminated Triblock Polyethers and its Application in Electrochromic Devices. J. Mater. Chem. C 2, 331–343. doi:10.1039/c3tc31555j
Saikia, D., Wu, H.-Y., Lin, C.-P., Pan, Y.-C., Fang, J., Tsai, L.-D., et al. (2012). New Highly Conductive Organic-Inorganic Hybrid Electrolytes Based on star-branched Silica Based Architectures. Polymer 53, 6008–6020. doi:10.1016/j.polymer.2012.11.012
Sanchez, C., and Ribot, F. (1993). Design of Hybrid Organic-Inorganic Materials Synthesized via Sol-Gel Chemistry. New J. Chem. 18 (10), 1007–1047.
Sanchez, C., and In, M. (1992). Molecular Design of Alkoxide Precursors for the Synthesis of Hybrid Organic-Inorganic Gels. J. Non-Crystalline Sol. 147-148, 1–12. doi:10.1016/s0022-3093(05)80584-8
Sanchez, C., Soler-Illia, G. J. d. A. A., Ribot, F., Lalot, T., Mayer, C. R., and Cabuil, V. (2001). Designed Hybrid Organic−Inorganic Nanocomposites from Functional Nanobuilding Blocks. Chem. Mater. 13, 3061–3083. doi:10.1021/cm011061e
Savva, A., Cendra, C., Giugni, A., Torre, B., Surgailis, J., Ohayon, D., et al. (2019). Influence of Water on the Performance of Organic Electrochemical Transistors. Chem. Mater. 31, 927–937. doi:10.1021/acs.chemmater.8b04335
Scardi, P., and Gelisio, L. (2016). Vibrational Properties of Nanocrystals from the Debye Scattering Equation. Sci. Rep. 6, 22221. doi:10.1038/srep22221
Schlesinger, R., Xu, Y., Hofmann, O. T., Winkler, S., Frisch, J., Niederhausen, J., et al. (2013). Controlling the Work Function of ZnO and the Energy-Level Alignment at the Interface to Organic Semiconductors with a Molecular Electron Acceptor. Phys. Rev. B 87, 155311. doi:10.1103/physrevb.87.155311
Schmidt, H. K. (1992). Organically Modified Silicates as Inorganic-Organic Polymers. Inorg. Organomet. Polym. Spec. Properties, 297–317. doi:10.1007/978-94-011-2612-0_20
Schöttner, L., Erker, S., Schlesinger, R., Koch, N., Nefedov, A., Hofmann, O. T., et al. (2020). Doping-Induced Electron Transfer at Organic/Oxide Interfaces: Direct Evidence from Infrared Spectroscopy. J. Phys. Chem. C 124, 4511–4516. doi:10.1021/acs.jpcc.9b08768
Segal-Peretz, T., Winterstein, J., Doxastakis, M., Ramírez-Hernández, A., Biswas, M., Ren, J., et al. (2015). Characterizing the Three-Dimensional Structure of Block Copolymers via Sequential Infiltration Synthesis and Scanning Transmission Electron Tomography. Acs Nano 9, 5333–5347. doi:10.1021/acsnano.5b01013
Semoto, T., Tsuji, Y., and Yoshizawa, K. (2011). Molecular Understanding of the Adhesive Force between a Metal Oxide Surface and an Epoxy Resin. J. Phys. Chem. C 115, 11701–11708. doi:10.1021/jp202785b
Sengodu, P., and Deshmukh, A. D. (2015). Conducting Polymers and Their Inorganic Composites for Advanced Li-Ion Batteries: a Review. RSC Adv. 5, 42109–42130. doi:10.1039/c4ra17254j
Serra Moreno, J., Armand, M., Berman, M. B., Greenbaum, S. G., Scrosati, B., and Panero, S. (2014). Composite PEOn:NaTFSI Polymer Electrolyte: Preparation, thermal and Electrochemical Characterization. J. Power Sourc. 248, 695–702. doi:10.1016/j.jpowsour.2013.09.137
Shi, S., Lu, P., Liu, Z., Qi, Y., Hector, L. G., Li, H., et al. (2012). Direct Calculation of Li-Ion Transport in the Solid Electrolyte Interphase. J. Am. Chem. Soc. 134 (37), 15476–15487. doi:10.1021/ja305366r
Shin, H.-Y., Shim, E.-L., Choi, Y.-J., Park, J.-H., and Yoon, S. (2014). Giant Enhancement of the Raman Response Due to One-Dimensional ZnO Nanostructures. Nanoscale 6, 14622–14626. doi:10.1039/c4nr04527k
Singh, D., Tao, R., and Lubineau, G. (2019). A Synergetic Layered Inorganic-Organic Hybrid Film for Conductive, Flexible, and Transparent Electrodes. Npj Flex Electron. 3, 10. doi:10.1038/s41528-019-0054-4
Sun, B., Mindemark, J., V. Morozov, E., Costa, L. T., Bergman, M., Johansson, P., et al. (2016). Ion Transport in Polycarbonate Based Solid Polymer Electrolytes: Experimental and Computational Investigations. Phys. Chem. Chem. Phys. 18, 9504–9513. doi:10.1039/c6cp00757k
Sun, Z., Zhao, B., and Lombardi, J. R. (2007). ZnO Nanoparticle Size-dependent Excitation of Surface Raman Signal from Adsorbed Molecules: Observation of a Charge-Transfer Resonance. Appl. Phys. Lett. 91, 221106. doi:10.1063/1.2817529
Sunarso, J., Baumann, S., Serra, J. M., Meulenberg, W. A., Liu, S., Lin, Y. S., et al. (2008). Mixed Ionic-Electronic Conducting (MIEC) Ceramic-Based Membranes for Oxygen Separation. J. Membr. Sci. 320, 13–41. doi:10.1016/j.memsci.2008.03.074
Suthirakun, S., Xiao, G., Ammal, S. C., Chen, F., zur Loye, H.-C., and Heyden, A. (2014). Rational Design of Mixed Ionic and Electronic Conducting Perovskite Oxides for Solid Oxide Fuel Cell Anode Materials: A Case Study for Doped SrTiO3. J. Power Sourc. 245, 875–885. doi:10.1016/j.jpowsour.2013.07.040
Taylor, F. H., Buckeridge, J., and Catlow, C. R. A. (2017). Screening Divalent Metals for A- and B-Site Dopants in LaFeO3. Chem. Mater. 29 (19), 8147–8157. doi:10.1021/acs.chemmater.7b01993
Timpel, M., Nardi, M. V., Wegner, B., Ligorio, G., Pasquali, L., Hildebrandt, J., et al. (2020). Oligothiophene‐Based Phosphonates for Surface Modification of Ultraflat Transparent Conductive Oxides. Adv. Mater. Inter. 7, 1902114. doi:10.1002/admi.201902114
Tseng, Y.-C., Peng, Q., Ocola, L. E., Czaplewski, D. A., Elam, J. W., and Darling, S. B. (2011). Enhanced Polymeric Lithography Resists via Sequential Infiltration Synthesis. J. Mater. Chem. 21, 11722–11725. doi:10.1039/c1jm12461g
Ullah, H., Tahir, A. A., and Mallick, T. K. (2017). Polypyrrole/TiO2 Composites for the Application of Photocatalysis. Sensors Actuators B: Chem. 241, 1161–1169. doi:10.1016/j.snb.2016.10.019
Van de Kerckhove, K., Mattelaer, F., Deduytsche, D., Vereecken, P. M., Dendooven, J., and Detavernier, C. (2016). Molecular Layer Deposition of "titanicone", a Titanium-Based Hybrid Material, as an Electrode for Lithium-Ion Batteries. Dalton Trans. 45 (3), 1176–1184. doi:10.1039/c5dt03840e
Vélez, J. F., Procaccini, R. A., Aparicio, M., and Mosa, J. (2013). Epoxy-silica Hybrid Organic–Inorganic Electrolytes with a High Li-Ion Conductivity. Electrochimica Acta 110, 200–207.
Villaluenga, I., Bogle, X., Greenbaum, S., Gil de Muro, I., Rojo, T., and Armand, M. (2013). Cation Only Conduction in New Polymer-SiO2 Nanohybrids: Na+ Electrolytes. J. Mater. Chem. A. 1, 8348–8352. doi:10.1039/c3ta11290j
Wang, M. J., Wolfenstine, J. B., and Sakamoto, J. (2020). Mixed Electronic and Ionic Conduction Properties of Lithium Lanthanum Titanate. Adv. Funct. Mater. 30 (10), 1909140. doi:10.1002/adfm.201909140
Wang, M., Yu, Y., Cui, M., Cao, X., Liu, W., Wu, C., et al. (2020). Development of Polyoxometalate-Anchored 3D Hybrid Hydrogel for High-Performance Flexible Pseudo-solid-state Supercapacitor. Electrochimica Acta 329, 135181. doi:10.1016/j.electacta.2019.135181
Wang, X., Esfarjani, K., and Zebarjadi, M. (2017). First-Principles Calculation of Charge Transfer at the Silicon-Organic Interface. J. Phys. Chem. C 121, 15529–15537. doi:10.1021/acs.jpcc.7b03275
Wang, X., She, G., Xu, H., Mu, L., and Shi, W. (2014). The Surface-Enhanced Raman Scattering from ZnO Nanorod Arrays and its Application for Chemosensors. Sensors Actuators B: Chem. 193, 745–751. doi:10.1016/j.snb.2013.11.097
Wang, X., Shi, W., Jin, Z., Huang, W., Lin, J., Ma, G., et al. (2017). Remarkable SERS Activity Observed from Amorphous ZnO Nanocages. Angew. Chem. Int. Ed. 56, 9851–9855. doi:10.1002/anie.201705187
Webb, M. A., Jung, Y., Pesko, D. M., Savoie, B. M., Yamamoto, U., Coates, G. W., et al. (2015). Systematic Computational and Experimental Investigation of Lithium-Ion Transport Mechanisms in Polyester-Based Polymer Electrolytes. ACS Cent. Sci. 1, 198–205. doi:10.1021/acscentsci.5b00195
Whittaker-Brooks, L., McClain, W. E., Schwartz, J., and Loo, Y.-L. (2014). Donor-Acceptor Interfacial Interactions Dominate Device Performance in Hybrid P3HT-ZnO Nanowire-Array Solar Cells. Adv. Energ. Mater. 4 (16), 1400585. doi:10.1002/aenm.201400585
Wind, J., Mole, R. A., Yu, D., Avdeev, M., and Ling, C. D. (2018). Hydration Mechanisms and Proton Conduction in the Mixed Ionic-Electronic Conductors Ba4Nb2O9 and Ba4Ta2O9. Chem. Mater. 30 (15), 4949–4958. doi:10.1021/acs.chemmater.8b00950
Wu, H., Wang, H., and Li, G. (2017). Metal Oxide Semiconductor SERS-Active Substrates by Defect Engineering. Analyst 142, 326–335. doi:10.1039/c6an01959e
Wu, R., Matta, M., Paulsen, B. D., and Rivnay, J. (2022). Operando Characterization of Organic Mixed Ionic/Electronic Conducting Materials. Chem. Rev. 122 (4), 4493–4551. doi:10.1021/acs.chemrev.1c00597
Xie, Z., Wu, Z., An, X., Yoshida, A., Wang, Z., Hao, X., et al. (2019). Bifunctional Ionic Liquid and Conducting Ceramic Co-assisted Solid Polymer Electrolyte Membrane for Quasi-Solid-State Lithium Metal Batteries. J. Membr. Sci. 586, 122–129. doi:10.1016/j.memsci.2019.05.066
Xu, Y., Hofmann, O. T., Schlesinger, R., Winkler, S., Frisch, J., Niederhausen, J., et al. (2013). Space-Charge Transfer in Hybrid Inorganic-Organic Systems. Phys. Rev. Lett. 111, 226802. doi:10.1103/physrevlett.111.226802
Xue, S., Liu, Y., Li, Y., Teeters, D., Crunkleton, D. W., and Wang, S. (2017). Diffusion of Lithium Ions in Amorphous and Crystalline Poly(ethylene oxide)3:LiCF3SO3 Polymer Electrolytes. Electrochimica Acta 235, 122–128. doi:10.1016/j.electacta.2017.03.083
Yildirim, H., Haskins, J. B., Bauschlicher, C. W., and Lawson, J. W. (2017). Decomposition of Ionic Liquids at Lithium Interfaces. 1. Ab Initio Molecular Dynamics Simulations. J. Phys. Chem. C 121 (51), 28214–28234. doi:10.1021/acs.jpcc.7b09657
Yilmaz, M., Babur, E., Ozdemir, M., Gieseking, R. L., Dede, Y., Tamer, U., et al. (2017). Nanostructured Organic Semiconductor Films for Molecular Detection with Surface-Enhanced Raman Spectroscopy. Nat. Mater 16, 918–924. doi:10.1038/nmat4957
Yoon, B., Lee, B. H., and George, S. M. (2012). Highly Conductive and Transparent Hybrid Organic-Inorganic Zincone Thin Films Using Atomic and Molecular Layer Deposition. J. Phys. Chem. C 116 (46), 24784–24791. doi:10.1021/jp3057477
Yoshimura, T., Tatsuura, S., Sotoyama, W., Matsuura, A., and Hayano, T. (1992). Quantum Wire and Dot Formation by Chemical Vapor Deposition and Molecular Layer Deposition of One‐dimensional Conjugated Polymer. Appl. Phys. Lett. 60 (3), 268–270. doi:10.1063/1.106681
Yoshimura, T., Tatsuura, S., and Sotoyama, W. (1991). Polymer Films Formed with Monolayer Growth Steps by Molecular Layer Deposition. Appl. Phys. Lett. 59 (4), 482–484. doi:10.1063/1.105415
Yu, X., Cai, H., Zhang, W., Li, X., Pan, N., Luo, Y., et al. (2011). Tuning Chemical Enhancement of SERS by Controlling the Chemical Reduction of Graphene Oxide Nanosheets. ACS Nano 5, 952–958. doi:10.1021/nn102291j
Zeng, Q. H., Yu, A. B., Lu, G. Q., and Standish, R. K. (2003). Molecular Dynamics Simulation of Organic−Inorganic Nanocomposites: Layering Behavior and Interlayer Structure of Organoclays. Chem. Mater. 15 (25), 4732–4738. doi:10.1021/cm0342952
Zhang, B., Tan, R., Yang, L., Zheng, J., Zhang, K., Mo, S., et al. (2018). Mechanisms and Properties of Ion-Transport in Inorganic Solid Electrolytes. Energ. Storage Mater. 10, 139–159. doi:10.1016/j.ensm.2017.08.015
Zhang, N., Tong, L., and Zhang, J. (2016). Graphene-Based Enhanced Raman Scattering toward Analytical Applications. Chem. Mater. 28, 6426–6435. doi:10.1021/acs.chemmater.6b02925
Zhang, Q., Li, X., Ma, Q., Zhang, Q., Bai, H., Yi, W., et al. (2017). A Metallic Molybdenum Dioxide with High Stability for Surface Enhanced Raman Spectroscopy. Nat. Commun. 8, 14903. doi:10.1038/ncomms14903
Zhang, Y., Rohan, R., Sun, Y., Cai, W., Xu, G., Lin, A., et al. (2014). A Gel Single Ion Polymer Electrolyte Membrane for Lithium-Ion Batteries with Wide-Temperature Range Operability. RSC Adv. 4, 21163–21170. doi:10.1039/c4ra02729a
Zhang, Zhou., Han, Faming., Fang, Jie., Zhao, Chaowei., Li, Shuai., Wu, Yonggang., et al. (2021). An Organic–Inorganic Hybrid Material Based on Benzo[ghi]perylenetri-Imide and Cyclic Titanium-Oxo Cluster for Efficient Perovskite and Organic Solar Cells. CCS Chem. 3, 1217–1225. doi:10.31635/ccschem.021.202100825
Zhang, Z., Zhang, P., Wang, Y., and Zhang, W. (2016). Recent Advances in Organic-Inorganic Well-Defined Hybrid Polymers Using Controlled Living Radical Polymerization Techniques. Polym. Chem. 7, 3950–3976. doi:10.1039/c6py00675b
Zhao, H., Asfour, F., Fu, Y., Jia, Z., Yuan, W., Bai, Y., et al. (2015). Plasticized Polymer Composite Single-Ion Conductors for Lithium Batteries. ACS Appl. Mater. Inter. 7, 19494–19499. doi:10.1021/acsami.5b06096
Zhao, H., Jia, Z., Yuan, W., Hu, H., Fu, Y., Baker, G. L., et al. (2015). Fumed Silica-Based Single-Ion Nanocomposite Electrolyte for Lithium Batteries. ACS Appl. Mater. Inter. 7, 19335–19341. doi:10.1021/acsami.5b05419
Zhou, H., Toney, M. F., and Bent, S. F. (2013). Cross-Linked Ultrathin Polyurea Films via Molecular Layer Deposition. Macromolecules 46 (14), 5638–5643. doi:10.1021/ma400998m
Zhu, Y. S., Wang, X. J., Hou, Y. Y., Gao, X. W., Liu, L. L., Wu, Y. P., et al. (2013). A New Single-Ion Polymer Electrolyte Based on Polyvinyl Alcohol for Lithium Ion Batteries. Electrochimica Acta 87, 113–118. doi:10.1016/j.electacta.2012.08.114
Keywords: organic-inorganic hybrid, mixed ionic and electronic conducting, sol-gel—alkoxide route, computational modeling, polymer nano composite
Citation: Romero M, Mombrú D, Pignanelli F, Faccio R and Mombrú AW (2022) Hybrid Organic-Inorganic Materials and Interfaces With Mixed Ionic-Electronic Transport Properties: Advances in Experimental and Theoretical Approaches. Front. Chem. 10:892013. doi: 10.3389/fchem.2022.892013
Received: 08 March 2022; Accepted: 25 March 2022;
Published: 12 April 2022.
Edited by:
Gianfranco Pacchioni, University of Milano-Bicocca, ItalyReviewed by:
Michele Pavone, University of Naples Federico II, ItalyCopyright © 2022 Romero, Mombrú, Pignanelli, Faccio and Mombrú. This is an open-access article distributed under the terms of the Creative Commons Attribution License (CC BY). The use, distribution or reproduction in other forums is permitted, provided the original author(s) and the copyright owner(s) are credited and that the original publication in this journal is cited, in accordance with accepted academic practice. No use, distribution or reproduction is permitted which does not comply with these terms.
*Correspondence: Mariano Romero, bXJvbWVyb0BmcS5lZHUudXk=; Ricardo Faccio, cmZhY2Npb0BmcS5lZHUudXk=; Alvaro W. Mombrú, YW1vbWJydUBmcS5lZHUudXk=