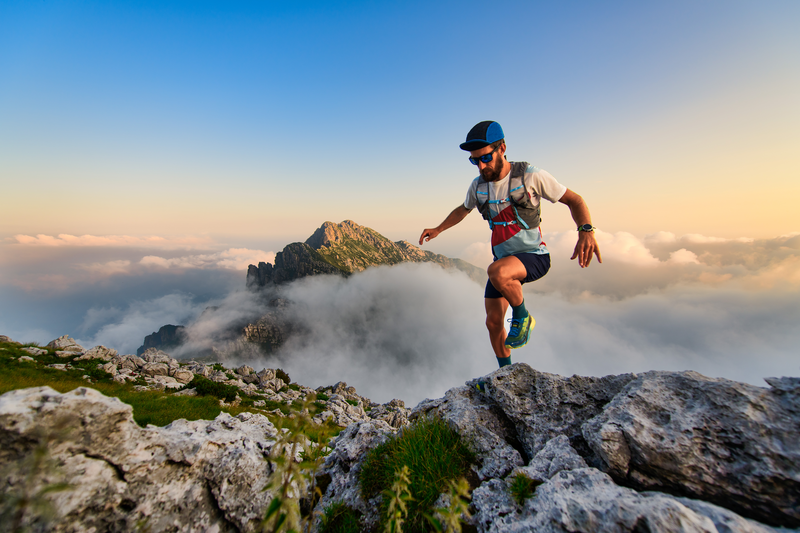
94% of researchers rate our articles as excellent or good
Learn more about the work of our research integrity team to safeguard the quality of each article we publish.
Find out more
REVIEW article
Front. Chem. , 01 June 2022
Sec. Medicinal and Pharmaceutical Chemistry
Volume 10 - 2022 | https://doi.org/10.3389/fchem.2022.890696
This article is part of the Research Topic Phosphonate Chemistry in Drug Design and Development, Volume II View all 5 articles
Phosphonates and bisphosphonates are stable analogs of phosphates and pyrophosphates that are characterized by one and two carbon–phosphorus bonds, respectively. Among the various phosphonates and bisphosphonates, hydroxy and amino substitutes are of interest as effective in medicinal and industrial chemistry. For example, hydroxy bisphosphonates have proven to be effective for the prevention of bone loss, especially in osteoporotic disease. On the other hand, different substitutions on the carbon atom connected to phosphorus have led to the synthesis of many different hydroxy- and amino-phosphonates and -bisphosphonates, each with its distinct physical, chemical, biological, therapeutic, and toxicological characteristics. Dialkyl or aryl esters of phosphonate and bisphosphonate compounds undergo the hydrolysis process readily and gave valuable materials with wide applications in pharmaceutical and agriculture. This review aims to demonstrate the ongoing preparation of various classes of hydroxy- and amino-phosphonates and -bisphosphonates. Furthermore, the current review summarizes and comprehensively describes articles on the biological applications of hydroxyl- and amino-phosphonates and -bisphosphonates from 2015 until today.
In living organisms, the phosphorus atom is one of the main elements that have an important role in biochemical reactions (Krazewski et al., 2020). Among the wide range of phosphorus compounds, phosphates and pyrophosphates have important roles in living organisms (Elliott et al., 2012). Phosphonates and bisphosphonates are stable analogs of phosphates and pyrophosphates that represent an important class of bioisosteres for chemical biologists and medicinal chemists (Park et al., 2021) (Horsman and Zechel 2017). The replacement of the P-O bond with the P-C bond increases their chemical and enzymatic stability. One of the highlighted applications of phosphonates has been reported in the COVID-19 pandemic (Jockusch et al., 2020). Among the various phosphonates and bisphosphonates, hydroxy- and amino-phosphonates and -bisphosphonates are of interest as effective in medicinal and industrial chemistry (Ebetino et al., 2022) (Santos et al., 2020). For example, hydroxy bisphosphonates are well-known bone disease drug candidates, and there are eight clinical hydroxy bisphosphonate drugs that have been introduced for bone diseases due to their high tendency to bind hydroxyapatite, the bone mineral compounds.
Hydroxy- and amino-phosphonates and -bisphosphonates have been prepared by various methods. First in this review, a short description will be presented ongoing the preparation methods of hydroxy- and amino-phosphonates and -bisphosphonates. Furthermore, the biological application of hydroxyl- and amino-phosphonates and -bisphosphonates from 2015 until today will be summarized and comprehensively described.
Hydroxyphosphonates and phosphonic acids inhibit enzymes such as renin, EPSP synthase HIV protease, and PTPases. Other biologically significant α-substituted phosphonates and phosphonic acids are also readily obtainable from hydroxyphosphonates (1). The base-catalyzed hydrophosphonylation of aldehydes (the Pudovik reaction) is one of the most important methods for the synthesis of hydroxyphosphonates (Sardarian and Kaboudin, 1997) (Supplementary Scheme S1 in SI).
In another method, the synthesis of hydroxyphosphonates has been reported from the reaction of carbonyl compounds with the addition of nucleophilic trialkyl phosphate (Vasikaran, 2001). In general, both reactions are performed in the presence of a catalyst and in some cases in the presence of the base (Radai 2019) (Radai and Keglevich 2018b). In recent years, enantiomerically pure hydroxyphosphonates have been synthesized by chiral-resolving agents or asymmetric chiral synthesis (Rádai et al., 2018a; Kaboudin et al., 2019a).
Aminophosphonates 2 is the most common category of phosphonate esters. A number of synthetic methods for the synthesis of aminophosphonates have been developed during the past two decades (Amira et al., 2021). Of these methods, the Kabachnik–Fields (Kaboudin and Moradi, 2005) (Bhagat and Chakraborti, 2007) synthesis of 1-aminophosphonates, catalyzed by a base or an acid, is the most convenient method (Varga and Keglevich 2021) (Sravya et al., 2021) (Supplementary Scheme S2 in SI).
The Kabachnik–Fields synthesis of aminoalkyl phosphonates is the nucleophilic addition of an amine to a carbonyl compound followed by the addition of a dialkyl phosphite to the resulting imine. Lewis acids such as InCl3, SnCl4, BF3, Et2O, ZnCl2, and MgBr2, have been used as catalysts. The aminophosphonates have also been synthesized by various other methods such as 1)) addition of P-H function to nitriles (Gancarz and Wieczorek, 1978), 2)) Arbuzov and Michaelis–Becker reactions (Seyferth et al., 1971), 3)) reaction of hydroxyphosphonate with amines (Kaboudin, 2003), 4)) condensation of X-NH2 with acyl phosphorus species (Worms et al., 1976), 5)) Curtius and Hofmann rearrangement of substituted phosphonoacetic esters (Barycki et al., 1970), and 6)) alkylation of nucleophilic precursors such as Schiff bases (Chandrasekhar et al., 2001). It seems that the Kabachnik–Fields reaction is the most efficient one among these routes. This reaction can be catalyzed by other catalysts such as BiCl3 (Zhan and Li, 2005), SnCl2 (Gallardo-Macias and Nakayama, 2010), CaCl2 (Kaboudin and Zahedi, 2008), or/and PPh3 (Tian et al., 2009). The reaction also can be promoted by heating or microwave irradiation (Kaboudin and Nazari, 2001; Ranu and Hajra, 2002; Macarie et al., 2019). In recent years, asymmetric synthesis of aminophosphonates has also been reported (Maestro et al., 2018, 2019; Maestro et al., 2020; Maestro et al., 2021).
The hydroxybisphosphonic acid derivatives 3 are important groups within organophosphorus pharmaceutics. Due to the high complexation ability of these compounds with calcium ions, the resorption of these ions is prevented. These compounds are used in the treatment of Paget’s disease, osteoporosis, and hypercalcemia. One of the most widely used methods for the synthesis of hydroxybisphosphonates is the reaction of carboxylic acids with phosphorus trichloride and phosphoric acids and followed by hydrolysis with water (Kieczykowski et al., 1995) (Supplementary Scheme S3 in SI).
The reaction proceeded via the formation of an ketophosphonate intermediate by the cross-linking of phosphorus nucleophile with the formed acyl chloride. In another process, the 1-hydroxy-1,1-bisphosphonates were prepared in high yield by the phosphite addition to ketophosphonates prepared from the Arbuzov reaction (Lecouvey and Leroux, 2000).
Aminobisphosphonates are important bisphosphonates with strong inhibitors of bone resorption that several typical structures of these compounds have been commercialized as drugs for the treatment of osteoporosis, Paget’s disease, and fibrous dysplasia (Chmielewska and Kafarski, 2016a) (Chmielewska and Kafarski, 2016b). Furthermore, due to their ability to complex metal ions, aminobisphosphonates have found important industrial applications, mainly, as corrosion inhibitors. Due to increasing interests in the biological activity of aminobisphosphonates, the development of methods for their synthesis is growing. Although several general procedures were previously elaborated to reach this goal, aminobisphosphonate chemistry is still developing quite substantially. Additionally, selected examples of aminobisphosphonate derivatization illustrate their usefulness for obtaining new diagnostic and therapeutic agents. A number of synthetic methods for the synthesis of 1-aminobisphosphonates have been developed using various starting materials (Kaboudin et al., 2019b) (Supplementary Scheme S4 in SI). One of the most important methods in the synthesis of 1-aminobisphosphonates is the use of amides or nitriles as substrates. Amides and nitriles are available in a wide variety of compounds and are easy to prepare.
However, there are many other procedures reported for the synthesis of aminobisphosphonates using isonitriles, oxophosphonates, and vinylidene bisphosphonates (Plöger et al., 1972; Fukuda et al., 1975; Bandurina et al., 1978; Wu et al., 2004; Szajnman et al., 2005; Yu et al., 2008; Roth et al., 2009; Midrier et al., 2011; Goldeman et al., 2012; Rodriguez, 2014). A wide range of aminomethylene bisphosphonic acids can be obtained from the simple three-component of amines, diethyl phosphite, and triethyl orthoformate (Kaboudin and Alipour, 2009).
Hydroxy- and amino-phosphonates and -bisphosphonates are an important class of compounds that are currently receiving significant attention. Various hydroxy- and amino-phosphonates and bisphosphonates structures have been synthesized and described in the literature with interesting applications. The growing interest in the biological activity of these compounds has stimulated the development of their applications.
Treatment of tuberculosis (TB) is problematic due to the emergence of Mycobacterium tuberculosis (Mt), so a new drug is needed. One of these targets is hypoxanthine-guanine phosphoribosyltransferase (HGPRT), which synthesizes 6-oxopurine nucleoside monophosphates, which are essential for DNA/RNA production. Combination of [3R, 4R]-4-hypoxanthin-9-yl-3-((S)-2-hydroxy-2-phosphonoethyl) oxy-1-N-(phosphonopropionyl)pyrrolidine and [3R, 4R]-4-guanin- 9-yl-3-((S)-2-hydroxy-2-phosphonoethyl)oxy-1-N(phosphonopropionyl)pyrrolidine is the most potent inhibitor of MtHGPRT (compound 5). This drug has low toxicity in mammalian cells (CC50 of 132 ± 20 μM). Therefore, it is a good inhibitor for anti-tuberculosis chemotherapy (Supplementary Scheme S5 in SI) (Eng et al., 2018).
Cheviet et al. (2020) reported the synthesis and biological activities of 24 novel hydroxyphosphonic acid derivatives, including 22 new non-cyclic nucleoside phosphonates. The compounds were studied as inhibitors of P. falciparum. Biological assays of phosphonic acid compounds (as sodium salts) on cell cultures revealed that the compounds’ effectiveness completely depends on the hydroxyl group, the chain length, and the nature of the base. Of all the compounds, derivative (R)-(4-(2-amino-6-oxo-9H-purin-9-yl)-2-hydroxybutyl)-phosphonic acid 6 appears to have the most suitable characteristics (i.e., guanine as nucleobase, a butyl chain, and a hydroxy group in the β-position and with R stereochemistry). It showed remarkable in vitro activity against P. falciparum-infected red blood cells (IC50 = 74 nM), and it has a high selectivity index (SI > 1,350). The compound has no toxicity on human cell lines. This was the first report on the antiplasmodial activity (in vitro and in vivo) of an acyclonucleoside phosphonate derivative (Supplementary Scheme S6 in SI).
The compounds 7 synthesized by the Kabachnik–Fields reaction. The in vitro cytotoxicity of the compounds was evaluated against HepG2 (human liver cancer cell line), SK-OV-3 (human ovarian cancer cell line), NCI-H460 (human large cell lung cancer cell line), and HL-7702 (human liver normal cell line) cell lines. The results show that, in HepG2 assay, compounds 7c exhibit more cytotoxicity activity than 5-FU drug. In SK-OV-3 assay, 7a and 7d exhibit more cytotoxicity activity than 5-FU. In NCI-H460 assay, 7c has more anticancer properties than 5-FU (Figure 1) (Yu et al., 2017).
Recently, a novel series of α-aminophosphonates 8 were synthesized through the Ugi three-component reaction (Supplementary Scheme S7 in SI). The compound 8 derivatives with R1: Bn, R2: Ph, and R3: Cy, and R1: Me, R2: p-SCCl3Ph, and R3: Cy showed good cytotoxicity against the A549 cell line (carcinomic human alveolar basal epithelial cell). They presented an IC50 Value of 16.14 ± 1.14 µM and 14.56 ± 2.53 µM, respectively (López-Francés et al., 2021). The same group also reported antiproliferative effect on A549 cells of some novel a-aminophosphonic acid derivatives 9–11 synthesized through the addition of O- and S- nucleophiles to 2H-azirines (Supplementary Scheme S8) (Carrraminana et al., 2020). All the compounds 9–11 showed selectivity on cancer cells (A549) over non-malignant cells (MCR-5).
Zhang et al. (2020) reported the synthesis and biological activities of novel sulfonamide-containing aminophosphonates 42 derivatives (Supplementary Scheme S8 in SI). Among the synthesized compounds 42, a derivative with R1 = 3-methoxy and R2 = methyl exhibited applicative COX-2 inhibitory (IC50 = 0.28 ± 0.05 µM). This compound also showed anticancer properties even more than cisplatin commercial drug. IC50 (µM) values of this aminophosphonate is 9.71 ± 0.47 for HeLa (human cervical cancer cells), 16.43 ± 0.62 for MCF-7 (human breast cancer cells), 2.34 ± 0.27 for HCT116 (human colon cancer cells), 12.51 ± 1.18 for HepG2 (human liver cancer cells), and 205.95 ± 2.36 for 293T (epithelial cell line).
Aita et al. (2021) reported the synthesis and biological activities of aminophosphonate derivatives of imatinib. According to their results in vitro cytotoxicity assay against human leukemia cells (K-562, U-973, and HL-60), human breast cancer cell (MCF-7), and human prostate cancer cell (DU-145) for the compounds 13a–d showed more anticancer activity than imatinib and doxorubicin drug (Figure 2).
One of the causes of Alzheimer’s disease is a lack of acetylcholine in the brain, so aminophosphonates as acetylcholine esterase (AChE) inhibitors can be used to prevent and treat Alzheimer’s. Shaikh et al. (2020) reported the synthesis and anticholine esterase activities of a series of novel N-substituted pyrazole-derived α-aminophosphonates 14. All the synthesized aminophosphonates were tested for their inhabitation of acetylcholine esterase by Ellman’s method. Among them, compounds 14a and 14b showed better activities than standard drugs (tacrine, rivastigmine, and galantamine). The antioxidant activities of the synthesized aminophosphonates 14 were assayed (Table 1).
Awad et al. (2018) reported the synthesis of compounds 15 through the one-pot Kabachnik–Fields reaction. They have examined their anticancer activities against HePG-2, MCF-7, HCT-116, and PC-3. The compounds 15a and 15b have good potential as anticancer agents. The results illustrate that compounds 15a and 15b had great antioxidant activities (Figure 3).
The compound 16 was synthesized by a three-component reaction of salicylaldehyde, malononitrile, and diethyl phosphite in the presence of 5 mol% of pentamethyldiethylenetriamine (PMDTA), under solvent-free conditions at 60°C. The in vitro cytotoxicity against human lung adenocarcinoma (A549), mouse fibroblasts (NIH/3T3) as a healthy cell line, and human promyelocytic leukemia (HL-60) has been studied. Among the synthesized aminophosphonates of 16, compounds 16a and 16b exhibited the highest anticancer activities, but none of them worked better than doxorubicin and bortezomib (Figure 4) (Tajti et al., 2021).
The FDPS (farnesyl diphosphate synthase), an enzyme in the sterol biosynthetic pathway, catalyzes synthesis of farnesyl diphosphate from the reaction of geranyl diphosphate with isopentenyl diphosphate. The intracellular location of this enzyme is an important target for bisphophonate drugs (zoledronic acid and Alendronate). Four complexes [Pt(en)]2ZL, [Pt(en)]2IPrBP, [Pt(en)]2MIBP, and [Pt(en)]2EIBP dinuclear platinum was designed and synthesized based on imidazolyl-containing bisphosphonates 17–20 with high affinity for hydroxyapatite (Supplementary Scheme S9 in SI). However, these complexes showed a little inhibitory effect on bone cancer cells with less anticancer activity. They have better selectivity in inhibiting hepatocarcinoma cells than normal liver cells, especially complex 17 at high concentrations (100 μM) (Qiu et al., 2015).
Some of the bisphosphonates were used clinically, and their phosphorus esters were studied to evaluate how the structure of bisphosphonates affects bone attachment. Bisphosphonates like clodronate lost the ability to bind to hydroxyapatite by adding an ester group to them, unlike medronate. But hydroxy-bisphosphonates still retained their ability to bind even by binding to the two ester groups (Supplementary Scheme S10 in SI). Regarding the binding of bisphosphonates, the results were as follows (Supplementary Scheme S14): 1) a hydroxyl group in the geminal carbon participates in the bonding process and increases the ability of bisphosphonates to bind to the bone. 2) The ability of binding of bisphosphonates decreases with an increasing number of ester groups. 3) The location of ester groups has a significant effect on their ability to bind to bisphosphonates. (Puljula et al., 2015).
Hsiao and Wiemer (2018) investigated phospho antigens, including diphosphates, bisphosphonates, and precursors (Supplementary Scheme S11 in SI), for their ability to induce leukemia cells to stimulate the secretion of Vγ9Vδ2 T-cell interferon-γ. Most of them showed their activity between 15 and 240 min. Potency (EC50 values) ranged between 8.4 nM and >100 μM. These findings showed better performance of prodrugs than other cases.
A potent EP4 receptor agonist was attached to the biologically inactive, bisphosphonate-based portion of the target bone. These single and doubly radiolabeled conjugates were made and showed to be stable in the blood, easily removed from the bloodstream, and effectively absorbed into the bone after in vivo dosing. It was found that doubly radiolabeled conjugate 29 splits widely between bone and liver, leaving the liver intact, and bone examination showed that the free EP4 agonist (compound 30) was released from bone-bound 29 with a half-life of 7 days. The compound 29 binds rapidly and completely to powdered bone minerals or to various forms of calcium phosphate to form a stable matrix suitable for implantation. It can also be converted to powder or solid forms and sterilized without decomposing or releasing 29 (Figure 5) (Thévenin et al., 2021).
A new three building blocks including doxorubicin bound by the linker to the bone-targeted hydroxybisphosphonate vector through imine bonds (compound 33) have been reported by David et al. (2019). Doxorubicin residue acts as an anticancer drug and the hydroxyl bisphosphonic acid group acts as a drug carrier to the target bone tissue. Due to the imine bond between doxorubicin and the vector to the linker, the doxorubicin drug was released in the target tissue with various acidic pH associated with the environment of the bone tumor. On the other hand, toxicity studies of compound 33 showed much less toxicity than doxorubicin. Furthermore, compound 33 had good effects on the osteosarcoma (Figure 6).
The covalent conjugation of the amino-bisphosphonate alendronate (ale) with the antimetabolite 5-fluoro 2′-deoxyuridine (5-FdU) is a new and effective drug to fight bone cancer. N4-(butyl-(4-hydroxy-4-phosphono) phosphate)-5-fluoro-2′deoxyuridine (5-FdU-alendronate, 5-FdU-ale) 34 has less toxicity than its two constituents in vitro and in vivo and is a promising candidate for the treatment of bone metastasis (Supplementary Scheme S12 in SI) (Schott et al., 2015).
Peng et al. (2017) showed that a novel zoledronic acid derivative 34 has antiproliferative and cytotoxic activity by inducing G1 cycle stopping and apoptosis and autophagy in the human colorectal cancer cell line HCT116. Induction of PTEN expression followed by inhibition of PI3K/Akt/mTOR is likely involved in these effects. These results provide a better understanding of the antitumor effects and underlying mechanisms of BPs in CRC treatment. Compound 35 had better antiproliferative effects on human CRC HCT116 cells than ZOL and may be used for CRC cancer therapy in the future (Supplementary Scheme S13 in SI).
A dual-action bone-targeting product 36 has been designed, synthesized, and evaluated for in vitro and in vivo metabolic stability by Xie et al. (2017). The compound was prepared from a combination of a highly potent anabolic selective agonist of the prostaglandin EP4 receptor and alendronic acid, a potent inhibitor of bone resorption optimally linked through a differentially hydrolyzable linker unit, N-4 carboxymethyl phenylmethyl oxycarbonyl-leucinyl-argininylpara aminolhen (Leu-Arg-PABA). It is designed to release the anabolic selective agonist activity of the prostaglandin EP4 receptor, and cathepsin K cleavage of the Leu-Arg-PABA element will release alendronic acid (Figure 7).
FIGURE 7. Conceptual differential enzymatic release of active EP4 agonist and bound bisphosphonate from the conjugate after binding to the bone surface.
Aoun et al. (2019) reported the synthesis and bone-targeting properties of a combination of terminal hydroxy-bisphosphonic function with a linear and convergent strategy (compounds 37 and 38). Under neutral conditions using the Arbuzov reaction with tris (trimethylsilyl) phosphite and a carboxylic acid precursor activated in situ with catecholborane, free hydroxy-bisphosphonic was introduced in a linear approach (Figure 8).
By masking the negative charge of the P-C-P structure in bisphosphonates (BP) with pivoxil esters, their capacity to inhibit tumor cell growth can be increased (Supplementary Scheme S4 in SI, compounds 39–45). BP pivoxil esters 39–45 are the most active structures for inhibiting the growth of hematopoietic cells with an IC50 value between 20 and 200 nM. In comparison, this value for zoledronic acid (Zol) is generally more than 20,000 nm. The compound tetrakispivaloyloxymethyl 2-(thiazole-2-ylamino)ethylidene-1,1-bisphosphonate (45) prevents the in vitro growth of tumor cells, especially hematopoietic cells at nm concentrations. Clinical studies have shown that it reduces the growth of human bladder cancer cells in a mouse model (Matsumoto et al., 2016).
Massarenti et al. (2017) reported the synthesis of novel aminobisphosphonates 46–48 as good candidates for antiresorption bone drugs (Supplementary Scheme S15 in SI).
Isoprenoid biosynthetic pathway (IBP) inhibitors include statins and nitrogenous bisphosphonates, which are used to treat bone disease and hypercholesterolemia. Reilly et al. (2015) reported the synthesis of the compound of disodium [(6z,11e,15e)-9-[bis(sodiooxy)phosphoryl]-17-hydroxy-2,6,12,16-tetramethylheptadeca-2,6,11,15-tetraen-9-yl] phosphonate 49 that selectively targets geranylgeranyl diphosphate synthase. Reduction of geranylgeranylation by the compound 49 inhibitor significantly reduces adrenal gland tumor metastasis in vivo (Supplementary Scheme S16 in SI).
A major therapeutic target for multiple myeloma is the geranylgeranyl diphosphate synthase enzyme. Inhibition of this enzyme disrupts protein geranylgeranylation, which disrupts intracellular protein trafficking. Some isoprenoid triazole bisphosphonates are potent and selective inhibitors of GGDPS. Bhuiyan et al. (2019) reported the synthesis and biological activities of some new analogs of triazole bisphosphonates to study their cellular and enzymatic activity. The compound 52 disrupts GGDPS with a minimal amount of inhibitory activity (Supplementary Scheme S17 in SI).
Malwal et al. (2019) reported the synthesis and biological activities of some novel bisphosphonates. After screening a library of inhibitors for biological activity against the long-chain prenyltransferase octaprenyl diphosphate synthase (OPPS), compound 51 showed IC50 values of ∼100 nM against heptaprenyl diphosphate synthase and 200 nM against a farnesyl diphosphate synthase (Supplementary Scheme S18 in SI).
Lacbay et al. (2018) reported the synthesis of a new category of thienopyrimidine-based bisphosphonate (ThP-BP) inhibitors 52–58 of the human geranylgeranyl pyrophosphate synthase (hGGPPS) that block protein prenylation in multiple myeloma (MM) cells leading to cellular apoptosis. These inhibitors are also effective in preventing the proliferation of other types of cancer cells. Administering a dose of ThP-BP inhibitor to a MM mouse model confirmed in vivo downregulation of Rap1A geranylgeranylation and reduction of monoclonal immunoglobulins (M-protein, a biomarker of disease burden) in the serum. According to the results, hGGPPS is a valuable therapeutic target in oncology and specifically for treating multiple myeloma (Supplementary Scheme S19 in SI).
The insecticide O,O′-dimethyl (2,2,2-trichloro-1-hydroxyethyl) phosphonate 59, commonly known as trichlorfon or metrifonate, is a well-known hydroxyphosphonate discovered in 1950. The compound 59 is the precursor for the synthesis of 2,2-dichlorovinyl dimethyl phosphate (DDVP) 60 that acts on flies, ticks, fleas, and cockroaches by inhibiting acetylcholinesterase enzyme. Rádai et al. (2018a) reported the synthesis and herbicidal activity of a new family of hydroxyphosphonates containing pyrimidine and quinoline scaffolds (61 and 62). Quinoline-based hydroxyphosphonate 62 was found to be an efficient antibiotic agent against Gram-positive (Staphylococci and Bacillus megaterium-I) as well as Gram-negative (E. coli, Salmonella typhi, and Proteus vulgaris) bacteria. The compound 63 showed a good antibacterial and antifungal activity against Gram-positive Bacillus subtilis and Gram-negative E. coli as well. A family of hydroxyphosphonates 64 containing the hydroxy group in the aromatic ring was reported as potential anticancer agents (Supplementary Scheme S20 in SI).
Zhang et al. (2019) reported the synthesis and herbicidal activities of some new structures of hydroxyphosphonate 65. Studies showed that most of the prepared compounds with different substitutions have good herbicidal activity against Amaranthus retroflexus. Among them, the compounds (1-(5,5-dimethyl-2-oxido-1,3,2-dioxaphosphinan-2-yl)propyl-2-((4,6-dimethoxypyrimidin-2-yl)oxy)benzoate) 65a and ((5,5-dimethyl-2-oxido-1,3,2-dioxaphosphinan-2-yl) (phenyl)methyl-2-((4,6-dimethoxypyrimidin-2-yl)oxy)benzoate) 65b showed much higher post-emergency herbicide activity against monocotyledonous weeds (Supplementary Scheme S21 in SI).
Sun et al. (2018) reported the synthesis of novel aminophosphonates via the aza-formal [4 + 2] cycloaddition reaction between the β-methyl-α,β-unsaturated aldehyde and the cyclic ketiminophosphonate in the presence of 5 mol% of NHC (N-heterocyclic carbene) as the chiral catalyst and 120 mol% of dibenzoquinone as the external oxidant. The antibacterial properties of synthesized products were assayed against X. oryzae pv. oryzae. This bacterium causes a serious disease named bacterial blight (BB) in rice plants. Among the synthesized aminophosphonates, compounds 66a and 66b had the most antibacterial activities (Figure 9).
Four compounds of aminophosphonates synthesized by the Kabachnik–Fields reaction in the presence of magnesium perchlorate as Lewis acid. The compounds were tested for their herbicidal and insecticidal activities. The compound 67 showed herbicidal activities against Arabidopsis thaliana and Poa annua the in vitro and against Amaranthus retroflexus, Stellaria media, Lolium perenne, and Digitaria sanguinalis the in vivo method. The results showed that some compounds such as 67 (Ar = Ph, 4-MeOC6H4-, 4-BrC6H4-, 2-ClC6H4-, 4-FC6H4-, and 2-BrC6H4-) displayed 100% inhibition against Amaranthus retroflexus at the dose of 1,000 g/ha in postemergence treatment. Compounds 67–70 did not exhibit any herbicidal activities. Some derivatives of the compound 70 displayed selective insecticidal activities against Aphis species or Plutella xylostella (Supplementary Scheme S22 in SI) (Chen et al., 2015).
Asymmetric synthesis of dufulin-based aminophosphonates 71 was carried out using chiral thiourea organocatalysts (Q1 and Q2) with excellent enantioselectivity. The (R)-enantiomeric formed Q1, used as a catalyst, and the (S)-enantiomeric formed Q2. Antiviral activities of all prepared aminophosphonates were studied against cucumber mosaic virus (CMV). The results showed that (R)-enantiomers exhibited more antivirus activities than (S)-enantiomers. The outcomes of biological activity examinations illustrate that most of the synthesized compounds had antiviral potential. The compounds (R)-71a, (R)-71b, and (R)-71c even act better than dufulin and ningnanmycin (Figure 10) (Zhang et al., 2016).
N-Pyridylpyrazole derivatives of aminophosphonates 72 and 73 were synthesized and examined for their insecticidal and fungicidal activities. The results show that synthesized aminophosphonates displayed weak insecticidal activities toward Mythimna separata (Walker). Some of the compounds exhibited apparent fungicidal activity against six plant fungi such as Fusarium oxysporum, Cercospora arachidicola, and Physalospora piricola (Supplementary Scheme S23 in SI) (Wang et al., 2019).
The herbicidal activities of C-substituted derivatives of glyphosate 74 compared with pure glyphosate have been reported by Rogacz et al. (2020). All the tested aminophosphonic derivations were found to be safe for cultivated oat plants. Among the synthesized glyphosate derivatives, compound 74a displayed the highest herbicidal activity against gallant soldier and common sorrel. It has herbicidal activities even more than pure glyphosate (Supplementary Scheme S24 in SI).
1-Hydroxy-2-(3-pyridyl)ethylidene bisphosphonic acid monosodium was synthesized with 71% yield and labeled with technetium-99m. The 99mTc complex had a radiochemical purity of 99.2 ± 0.6% and was stable up to 6 h. The bio-distribution study showed a high absorption and long shelf life of the complex 75 in bone from 15 min (29 ± 2.5% ID/organ) to 4 h (35.1 ± 3.2 ID/organ) after injection. This provided a good tracker for bone imaging (Supplementary Scheme S25 in SI) (Motaleb et al., 2016).
Positron emission tomography (PET) imaging with bisphosphonate-labeled 68Ga is a powerful tool for cancer diagnosis and monitoring treatment aimed at bone metastasis. N,N′-bis [2-Hydroxy-5-(carboxyethyl)benzyl]ethylenediamine-N,N′-diacetic acid ligand 76, which contains a bisphosphonate group with a 68Ga label, was used as a valuable tool in bone imaging. Biodistribution and autoradiography studies of this combination showed that the tracer is taken up almost exclusively by the skeletal system with the least amount of accumulation of activity in other organs. This imaging agent can be used as a clinical tool to diagnose bone disorders and bone metastases in patients (Figure 11, complex 77) (Zha et al., 2020).
Calcium minerals such as hydroxyapatite can be detected in vivo using nuclear imaging agents. Keeling et al. (2020) reported synthesis and application of novel hydroxyl bisphosphonic acid 78 in imaging. Radiolabeling of the ligand with 68Ga gave a high radiochemical yield and purity (>95%). The experiments results showed that all 68Ga-BPs have a high affinity for a broad range of calcium minerals implicated in vascular calcification disease. Furthermore, 68Ga-ligand showed high potential for clinical translation as a cyclotron-independent calcium mineral PET radiotracer (Supplementary Scheme S26 in SI).
The di-zinc complex 79 binds to bisphosphonates with high affinity and good selectivity. The synthesized di-zinc(II) complex showed high affinity for bisphosphonates such as alendronate and etidronate. The prepared complex 80 was studied to treat a number of skeletal disorders and possessed anticancer properties. A modification with gold nanoparticles was used as a drug-loaded receptor with highly potential drug-delivery application. (Figure 12) (Torres-Huerta et al., 2020).
The development of appropriate drug delivery to tissues is one of the most important research areas in recent decades. Osteomyelitis pathogens are biofilms attached to bone tissue that disrupts antibiotic delivery and is now known as the bone marrow problem. Sedghizadeh et al. (2017) reported the synthesis and drug-delivery properties of novel bisphosphonate-ciprofloxacin 81. The “target and release” chemical strategy was used, and the results showed a significant therapeutic index against ciprofloxacin for the treatment of osteomyelitis in vivo. The stable release of the primary antibiotic occurred over time and increased the antibacterial effect of the leading drug in the target bone tissue (Figure 13).
Benyettou et al. (2015) reported a simple method for synthesis of a pH-sensitive drug-delivery system for doxorubicin (compound 82). The drug was ligated to a novel silver nanocomposite, and the results of the doxorubicin release studies showed that the process occurred in late endosomes/lysosomes and became evenly distributed throughout the cytosol (Supplementary Scheme S27 in SI).
More than 65% of the elderly people have hearing loss problems. One of the important drugs for hearing problems is dihydroxyflavone (DHF). This drug is selective and protects the neuron from apoptosis due to the potent agonist of tropomyosin receptor kinase B (TrkB). Delivery of DHF to the inner ear is the main challenge. Kempfle et al. (2018) reported the synthesis of new bisphosphonate−dihydroxyflavone 83 for a novel approach to the targeted delivery of drugs to treat sensorineural hearing loss. Bisphosphonates residue of the compound 83 provides an exciting compound for targeted delivery due to its affinity for bone minerals, including cochlear bone. The results showed that the compound 83 is a convenient targeted delivery of dihydroxyflavone to treat sensorineural hearing loss (Supplementary Scheme S28 in SI).
Aufaure et al. (2016) reported a novel NIR probe of Au nanoparticle-coated hydroxyl bisphohosphonate (compound 84). 1-Hydroxy-1,1-methylenebisphosphonate bearing an alkene functionality was used for the preparation of gold nanoparticles bearing an alkene functionality for the synthesis of compound 84 via a tetrazine click chemistry (Figure 14).
In summary, hydroxyl- and amino-phosphonates and -bisphosphonates are being developed due to their wide range of biological applications. Among them, hydroxybisphosphonates are gaining significant interest in pharmaceuticals due to their bone resorption inhibitors. These compounds are an important family of drugs from the clinical points in patients with metabolic bone disease. Aminobisphosphonates' properties are similar to hydroxybisphosphonates and therefore used as strong inhibitors of bone resorption for the treatment of Paget’s disease and osteoporosis. Hydroxy- and amino-phosphonates are being studied for enzyme inhibitors, anticancer properties, herbicides, and antibacterial properties. Different substitutions on the carbon atom connected to phosphorus have led to the synthesis of many phosphonates and bisphosphonates that have different physical, chemical, biological, therapeutic, and toxicological characteristics.
All authors listed have made a substantial, direct, and intellectual contribution to the work and approved it for publication.
The authors declare that the research was conducted in the absence of any commercial or financial relationships that could be construed as a potential conflict of interest.
All claims expressed in this article are solely those of the authors and do not necessarily represent those of their affiliated organizations, or those of the publisher, the editors, and the reviewers. Any product that may be evaluated in this article, or claim that may be made by its manufacturer, is not guaranteed or endorsed by the publisher.
The authors gratefully acknowledge support by the Institute for Advanced Studies in Basic Sciences (IASBS), Iran.
The Supplementary Material for this article can be found online at: https://www.frontiersin.org/articles/10.3389/fchem.2022.890696/full#supplementary-material
Aita, S., Badavath, V. N., Gundluru, M., Sudileti, M., Nemallapudi, B. R., Gundala, S., et al. (2021). Novel α-Aminophosphonates of Imatinib Intermediate: Synthesis, Anticancer Activity, Human Abl Tyrosine Kinase Inhibition, ADME and Toxicity Prediction. Bioorg. Chem. 109, 104718. doi:10.1016/j.bioorg.2021.104718
Amira, A., Aouf, Z., K'tir, H., Chemam, Y., Ghodbane, R., Zerrouki, R., et al. (2021). Recent Advances in the Synthesis of α‐Aminophosphonates: A Review. ChemistrySelect 6, 6137–6149. doi:10.1002/slct.202101360
Aoun, S., Sierocki, P., Lebreton, J., and Mathé-Allainmat, M. (2019). Linear and Convergent Syntheses of Bifunctional Hydroxy-Bisphosphonic Compounds as Potential Bone-Targeting Prodrugs. Synthesis 51 (18), 3556–3566. doi:10.1055/s-0037-1611540
Aufaure, R., Hardouin, J., Millot, N., Motte, L., Lalatonne, Y., and Guénin, E. (2016). Tetrazine Click Chemistry for the Modification of 1-Hydroxy-1,1-Methylenebisphosphonic Acids: Towards Bio-Orthogonal Functionalization of Gold Nanoparticles. Chem. Eur. J. 22 (45), 16022–16027. doi:10.1002/chem.201602899
Awad, M. K., Abdel-Aal, M. F., Atlam, F. M., and Hekal, H. A. (2018). Design, Synthesis, Molecular Modeling, and Biological Evaluation of Novel α-aminophosphonates Based Quinazolinone Moiety as Potential Anticancer Agents: DFT, NBO and Vibrational Studies. J. Mol. Struct. 1173, 128–141. doi:10.1016/j.molstruc.2018.06.094
Bandurina, T. A., Konyukhov, V. N., Ponomareva, O. A., Barybin, A. S., and Pushkareva, Z. V. (1978). Synthesis and Antitumor Activity of Aminophosphonic Acids. Pharm. Chem. J. 12 (11), 1428–1431. doi:10.1007/bf00772638
Barycki, J., Mastalerz, P., and Soroka, M. (1970). Simple Synthesis of 2-aminoethylphosphonic Acid and Related Compounds. Tetrahedron Lett. 11 (36), 3147–3150. doi:10.1016/s0040-4039(00)99713-2
Benyettou, F., Rezgui, R., Ravaux, F., Jaber, T., Blumer, K., Jouiad, M., et al. (2015). Synthesis of Silver Nanoparticles for the Dual Delivery of Doxorubicin and Alendronate to Cancer Cells. J. Mat. Chem. B 3 (36), 7237–7245. doi:10.1039/c5tb00994d
Bhagat, S., and Chakraborti, A. K. (2007). An Extremely Efficient Three-Component Reaction of Aldehydes/Ketones, Amines, and Phosphites (Kabachnik−Fields Reaction) for the Synthesis of α-Aminophosphonates Catalyzed by Magnesium Perchlorate. J. Org. Chem. 72 (4), 1263–1270. doi:10.1021/jo062140i
Bhuiyan, N. H., Varney, M. L., Bhattacharya, D. S., Payne, W. M., Mohs, A. M., Holstein, S. A., et al. (2019). ω-Hydroxy Isoprenoid Bisphosphonates as Linkable GGDPS Inhibitors. Bioorg. Med. Chem. Lett. 29 (19), 126633. doi:10.1016/j.bmcl.2019.126633
Branco Santos, J. C., de Melo, J. A., Maheshwari, S., de Medeiros, W. M. T. Q., de Freitas Oliveira, J. W., Moreno, C. J., et al. (2020). Bisphosphonate-Based Molecules as Potential New Antiparasitic Drugs. Molecules 25, 2602. doi:10.3390/molecules25112602
Carramiñana, V., Ochoa de Retana, A. M., Palacios, F., and de los Santos, J. M. (2020). Synthesis of α-Aminophosphonic Acid Derivatives through the Addition of O- and S-Nucleophiles to 2H-Azirines and Their Antiproliferative Effect on A549 Human Lung Adenocarcinoma Cells. Molecules 25 (15), 3332. doi:10.3390/molecules25153332
Chandrasekhar, S., Prakash, S. J., Jagadeshwar, V., and Narsihmulu, C. (2001). Three Component Coupling Catalyzed by TaCl5-SiO2: Synthesis of α-amino Phosphonates. Tetrahedron Lett. 42 (32), 5561–5563. doi:10.1016/s0040-4039(01)01053-x
Chen, J.-L., Tang, W., Che, J.-Y., Chen, K., Yan, G., Gu, Y.-C., et al. (2015). Synthesis and Biological Activity Evaluation of Novel α-Amino Phosphonate Derivatives Containing a Pyrimidinyl Moiety as Potential Herbicidal Agents. J. Agric. Food Chem. 63 (32), 7219–7229. doi:10.1021/acs.jafc.5b02335
Cheviet, T., Wein, S., Bourchenin, G., Lagacherie, M., Périgaud, C., Cerdan, R., et al. (2020). β-Hydroxy- and β-Aminophosphonate Acyclonucleosides as Potent Inhibitors of Plasmodium Falciparum Growth. J. Med. Chem. 63 (15), 8069–8087. doi:10.1021/acs.jmedchem.0c00131
Chmielewska, E., and Kafarski, P. (2016b). Physiologic Activity of Bisphosphonates - Recent Advances. Pharmsci 3, 56–78. doi:10.2174/1874844901603010056
Chmielewska, E., and Kafarski, P. (2016a). Synthetic Procedures Leading towards Aminobisphosphonates. Molecules 21 (11), 1474. doi:10.3390/molecules21111474
David, E., Cagnol, S., Goujon, J.-Y., Egorov, M., Taurelle, J., Benesteau, C., et al. (2019). 12b80 - Hydroxybisphosphonate Linked Doxorubicin: Bone Targeted Strategy for Treatment of Osteosarcoma. Bioconjugate Chem. 30 (6), 1665–1676. doi:10.1021/acs.bioconjchem.9b00210
Ebetino, F. H., Sun, S., Cherian, P., Roshandel, S., Neighbors, J. D., Hu, E., et al. (2022). Bisphosphonates: The Role of Chemistry in Understanding Their Biological Actions and Structure-Activity Relationships, and New Directions for Their Therapeutic Use. Bone 156, 116289. doi:10.1016/j.bone.2021.116289
Elliott, T. S., Slowey, A., Ye, Y., and Conway, S. J. (2012). The Use of Phosphate Bioisosteres in Medicinal Chemistry and Chemical Biology. Med. Chem. Commun. 3 (7), 735–751. doi:10.1039/c2md20079a
Eng, W. S., Rejman, D., Pohl, R., West, N. P., Woods, K., Naesens, L. M. J., et al. (2018). Pyrrolidine Nucleoside Bisphosphonates as Antituberculosis Agents Targeting Hypoxanthine-Guanine Phosphoribosyltransferase. Eur. J. Med. Chem. 159, 10–22. doi:10.1016/j.ejmech.2018.09.039
Fukuda, M., Okamoto, Y., and Sakurai, H. (1975). Synthesis of Dialkylaminomethylenediphosphonic Acids. Bcsj 48 (3), 1030. doi:10.1246/bcsj.48.1030
Gallardo-Macias, R., and Nakayama, K. (2010). Tin (II) Compounds as Catalysts for the Kabachnik-Fields Reaction under Solvent-free Conditions: Facile Synthesis of α-aminophosphonates. Synthesis 2010 (01), 57–62.
Goldeman, W., Kluczyński, A., and Soroka, M. (2012). The Preparation of N-Substituted Aminomethylidenebisphosphonates and Their Tetraalkyl Esters via Reaction of Isonitriles with Trialkyl Phosphites and Hydrogen Chloride. Part 1. Tetrahedron Lett. 53 (39), 5290–5292. doi:10.1016/j.tetlet.2012.07.085
Horsman, G. P., and Zechel, D. L. (2017). Phosphonate Biochemistry. Chem. Rev. 117, 5704–5783. doi:10.1021/acs.chemrev.6b00536
Hsiao, C.-H. C., and Wiemer, A. J. (2018). A Power Law Function Describes the Time- and Dose-Dependency of Vγ9Vδ2 T Cell Activation by Phosphoantigens. Biochem. Pharmacol. 158, 298–304. doi:10.1016/j.bcp.2018.10.035
Jockusch, S., Tao, C., Li, X., Anderson, T. K., Chien, M., Kumar, S., et al. (2020). A Library of Nucleotide Analogues Terminate RNA Synthesis Catalyzed by Polymerases of Coronaviruses that Cause SARS and COVID-19. Antivir. Res. 180, 104857. doi:10.1016/j.antiviral.2020.104857
Kaboudin, B. (2003). A Convenient Synthesis of 1-aminophosphonates from 1-hydroxyphosphonates. Tetrahedron Lett. 44 (5), 1051–1053. doi:10.1016/s0040-4039(02)02727-2
Kaboudin, B., Alavi, S., Kazemi, F., Aoyama, H., and Yokomatsu, T. (2019a). Resolution of Racemic α-Hydroxyphosphonates: Bi(OTf)3-Catalyzed Stereoselective Esterification of α-Hydroxyphosphonates with (+)-Dibenzoyl-L-Tartaric Anhydride. ACS Omega 4 (13), 15471–15478. doi:10.1021/acsomega.9b01722
Kaboudin, B., and Alipour, S. (2009). A Microwave-Assisted Solvent- and Catalyst-free Synthesis of Aminomethylene Bisphosphonates. Tetrahedron Lett. 50 (29), 4243–4245. doi:10.1016/j.tetlet.2009.05.016
Kaboudin, B., Esfandiari, H., Moradi, A., Kazemi, F., and Aoyama, H. (2019b). ZnCl2-Mediated Double Addition of Dialkylphosphite to Nitriles for the Synthesis of 1-aminobisphosphonates. J. Org. Chem. 84 (22), 14943–14948. doi:10.1021/acs.joc.9b02298
Kaboudin, B., and Moradi, K. (2005). A Simple and Convenient Procedure for the Synthesis of 1-aminophosphonates from Aromatic Aldehydes. Tetrahedron Lett. 46 (17), 2989–2991. doi:10.1016/j.tetlet.2005.03.037
Kaboudin, B., and Nazari, R. (2001). Microwave-assisted Synthesis of 1-aminoalkyl Phosphonates under Solvent-free Conditions. Tetrahedron Lett. 42 (46), 8211–8213. doi:10.1016/s0040-4039(01)01627-6
Kaboudin, B., and Zahedi, H. (2008). Calcium Chloride as an Efficient Lewis Base Catalyst for the One-Pot Synthesis of α-Aminophosphonic Esters. Chem. Lett. 37 (5), 540–541. doi:10.1246/cl.2008.540
Keeling, G. P., Sherin, B., Kim, J., San Juan, B., Grus, T., Eykyn, T. R., et al. (2020). [68Ga]Ga-THP-Pam: A Bisphosphonate PET Tracer with Facile Radiolabeling and Broad Calcium Mineral Affinity. Bioconjugate Chem. 32 (7), 1276–1289. doi:10.1021/acs.bioconjchem.0c00401
Kempfle, J. S., Nguyen, K., Hamadani, C., Koen, N., Edge, A. S., Kashemirov, B. A., et al. (2018). Bisphosphonate-linked TrkB Agonist: Cochlea-Targeted Delivery of a Neurotrophic Agent as a Strategy for the Treatment of Hearing Loss. Bioconjugate Chem. 29 (4), 1240–1250. doi:10.1021/acs.bioconjchem.8b00022
Kraszewski, A., Sobkowski, M., and Stawinski, J. (2020). H-phosphonate Chemistry in the Synthesis of Electrically Neutral and Charged Antiviral and Anticancer Pronucleotides. Front. Chem. 8, 595738. doi:10.3389/fchem.2020.595738
Lacbay, C. M., Waller, D. D., Park, J., Gómez Palou, M., Vincent, F., Huang, X. F., et al. (2018). Unraveling the Prenylation-Cancer Paradox in Multiple Myeloma with Novel Geranylgeranyl Pyrophosphate Synthase (GGPPS) Inhibitors. J. Med. Chem. 61 (15), 6904–6917. doi:10.1021/acs.jmedchem.8b00886
Lecouvey, M., and Leroux, Y. (2000). Synthesis of 1-Hydroxy-1,1-Bisphosphonates. Heteroat. Chem. 11 (7), 556–561. doi:10.1002/1098-1071(2000)11:7<556::aid-hc15>3.0.co;2-n
López-Francés, A., Del Corte, X., Martínez de Marigorta, E., Palacios, F., and Vicario, J. (2021). Ugi Reaction on α-Phosphorated Ketimines for the Synthesis of Tetrasubstituted α-Aminophosphonates and Their Applications as Antiproliferative Agents. Molecules 26 (6), 1654.
Macarie, L., Simulescu, V., and Ilia, G. (2019). Ultrasonic Irradiation Used in Synthesis of Aminophosphonates. Monatsh Chem. 150 (2), 163–171. doi:10.1007/s00706-018-2327-3
Maestro, A., Del Corte, X., López-Francés, A., Martínez de Marigorta, E., Palacios, F., and Vicario, J. (2021). Asymmetric Synthesis of Tetrasubstituted α-Aminophosphonic Acid Derivatives. Molecules 26 (11), 3202. doi:10.3390/molecules26113202
Maestro, A., Marigorta, E. M., Palacios, F., and Vicario, J. (2020). α‐Iminophosphonates: Useful Intermediates for Enantioselective Synthesis of α‐Aminophosphonates. Asian J. Org. Chem. 9 (4), 538–548. doi:10.1002/ajoc.202000039
Maestro, A., Martinez de Marigorta, E., Palacios, F., and Vicario, J. (2019). Enantioselective Aza-Reformatsky Reaction with Ketimines. Org. Lett. 21 (23), 9473–9477. doi:10.1021/acs.orglett.9b03669
Maestro, A., Martinez de Marigorta, E., Palacios, F., and Vicario, J. (2018). Enantioselective α-Aminophosphonate Functionalization of Indole Ring through an Organocatalyzed Friedel-Crafts Reaction. J. Org. Chem. 84 (2), 1094–1102. doi:10.1021/acs.joc.8b02843
Malwal, S. R., Chen, L., Hicks, H., Qu, F., Liu, W., Shillo, A., et al. (2019). Discovery of Lipophilic Bisphosphonates that Target Bacterial Cell Wall and Quinone Biosynthesis. J. Med. Chem. 62 (5), 2564–2581. doi:10.1021/acs.jmedchem.8b01878
Massarenti, C., Bortolini, O., Fantin, G., Cristofaro, D., Ragno, D., Perrone, D., et al. (2017). Fluorous-tag Assisted Synthesis of Bile Acid-Bisphosphonate Conjugates via Orthogonal Click Reactions: an Access to Potential Anti-resorption Bone Drugs. Org. Biomol. Chem. 15 (22), 4907–4920. doi:10.1039/c7ob00774d
Matsumoto, K., Hayashi, K., Murata-Hirai, K., Iwasaki, M., Okamura, H., Minato, N., et al. (2016). Targeting Cancer Cells with a Bisphosphonate Prodrug. ChemMedChem 11 (24), 2656–2663. doi:10.1002/cmdc.201600465
Midrier, C., Lantsoght, M., Volle, J.-N., Pirat, J.-L., Virieux, D., and Stevens, C. V. (2011). Hydrophosphonylation of Alkenes or Nitriles by Double Radical Transfer Mediated by Titanocene/propylene Oxide. Tetrahedron Lett. 52 (50), 6693–6696. doi:10.1016/j.tetlet.2011.09.096
Motaleb, M. A., Adli, A. S. A., El-Tawoosy, M., Sanad, M. H., and AbdAllah, M. (2016). An Easy and Effective Method for Synthesis and Radiolabelling of Risedronate as a Model for Bone Imaging. J. Label. Compd. Radiopharm. 59 (4), 157–163. doi:10.1002/jlcr.3384
Park, J., Pandya, V. R., Ezekiel, S. J., and Berghuis, A. M. J. (2021). Phosphonate and Bisphosphonate Inhibitors of Farnesyl Pyrophosphate Synthases: A Structure-Guided Perspective. Front. Chem. 8, 1233. doi:10.3389/fchem.2020.612728
Peng, Y., Qiu, L., Xu, D., Zhang, L., Yu, H., Ding, Y., et al. (2017). M 4 IDP, a Zoledronic Acid Derivative, Induces G1 Arrest, Apoptosis and Autophagy in HCT116 Colon Carcinoma Cells via Blocking PI3K/Akt/mTOR Pathway. Life Sci. 185, 63–72. doi:10.1016/j.lfs.2017.07.024
Plöger, W., Schindler, N., Wollmann, K., and Worms, K. (1972). Herstellung von 1‐Aminoalkan‐1, 1‐diphosphonsäuren. Z. für Anorg. Allg. Chem. 389 (2), 119–128.
Puljula, E., Turhanen, P., Vepsäläinen, J., Monteil, M., Lecouvey, M., and Weisell, J. (2015). Structural Requirements for Bisphosphonate Binding on Hydroxyapatite: NMR Study of Bisphosphonate Partial Esters. ACS Med. Chem. Lett. 6 (4), 397–401. doi:10.1021/ml5004603
Qiu, L., Lv, G., Cao, Y., Chen, L., Yang, H., Luo, S., et al. (2015). Synthesis and Biological Evaluation of Novel Platinum Complexes of Imidazolyl-Containing Bisphosphonates as Potential Anticancer Agents. J. Biol. Inorg. Chem. 20 (8), 1263–1275. doi:10.1007/s00775-015-1305-z
Rádai, Z. J. (2019). α-Hydroxyphosphonates as Versatile Starting Materials. Phosphorus, Sulfur, Silicon Relat. Elem. 194 (4-6), 425–437.
Rádai, Z., and Keglevich, G. (2018b). Synthesis and Reactions of α-Hydroxyphosphonates. Molecules 23, 1493.
Rádai, Z., Kiss, N. Z., Keglevich, G., Keglevich, G., Walter de Gruyter GmbH, , and Berlin, G. (2018a). Synthesis of α-hydroxyphosphonates, an Important Class of Bioactive Compounds. Organophosphorus Chem., 91–107. doi:10.1515/9783110535839-005
Ranu, B. C., and Hajra, A. (2002). A Simple and Green Procedure for the Synthesis of α-aminophosphonate by a One-Pot Three-Component Condensation of Carbonyl Compound, Amine and Diethyl Phosphite without Solvent and Catalyst. Green Chem. 4 (6), 551–554. doi:10.1039/b205747f
Reilly, J. E., Neighbors, J. D., Tong, H., Henry, M. D., and Hohl, R. J. (2015). Targeting Geranylgeranylation Reduces Adrenal Gland Tumor Burden in a Murine Model of Prostate Cancer Metastasis. Clin. Exp. Metastasis 32 (6), 555–566. doi:10.1007/s10585-015-9727-0
Rodriguez, J. (2014). Tetraethyl Vinylidenebisphosphonate: A Versatile Synthon for the Preparation of Bisphosphonates. Synthesis 46 (09), 1129–1142. doi:10.1055/s-0033-1340952
Rogacz, D., Lewkowski, J., Cal, D., and Rychter, P. (2020). Ecotoxicological Effects of New C-Substituted Derivatives of N-Phosphonomethylglycine (Glyphosate) and Their Preliminary Evaluation towards Herbicidal Application in Agriculture. Ecotoxicol. Environ. Saf. 194, 110331. doi:10.1016/j.ecoenv.2020.110331
Roth, A. G., Drescher, D., Yang, Y., Redmer, S., Uhlig, S., and Arenz, C. (2009). Potent and Selective Inhibition of Acid Sphingomyelinase by Bisphosphonates. Angew. Chem. Int. Ed. 48 (41), 7560–7563. doi:10.1002/anie.200903288
Sardarian, A. R., and Kaboudin, B. (1997). Surface-mediated Solid Phase Reactions: Preparation of Diethyl 1-hydroxyarylmethylphosphonates on the Surface of Magnesia. Synth. Commun. 27 (4), 543–551. doi:10.1080/00397919708003324
Schott, S., Vallet, S., Tower, R. J., Noor, S., Tiwari, S., Schem, C., et al. (2015). In Vitro and In Vivo Toxicity of 5-FdU-Alendronate, a Novel Cytotoxic Bone-Seeking Duplex Drug against Bone Metastasis. Invest. New Drugs 33 (4), 816–826. doi:10.1007/s10637-015-0253-3
Sedghizadeh, P. P., Sun, S., Junka, A. F., Richard, E., Sadrerafi, K., Mahabady, S., et al. (2017). Design, Synthesis, and Antimicrobial Evaluation of a Novel Bone-Targeting Bisphosphonate-Ciprofloxacin Conjugate for the Treatment of Osteomyelitis Biofilms. J. Med. Chem. 60 (6), 2326–2343. doi:10.1021/acs.jmedchem.6b01615
Seyferth, D., Marmor, R. S., and Hilbert, P. (1971). Reactions of Dimethylphosphono-Substituted Diazoalkanes. (MeO)2P(O)CR Transfer to Olefins and 1,3-dipolar Additions of (MeO)2P(O)C(N2)R. J. Org. Chem. 36 (10), 1379–1386. doi:10.1021/jo00809a014
Shaikh, S., Dhavan, P., Pavale, G., Ramana, M. M. V., and Jadhav, B. L. (2020). Design, Synthesis and Evaluation of Pyrazole Bearing α-aminophosphonate Derivatives as Potential Acetylcholinesterase Inhibitors against Alzheimer's Disease. Bioorg. Chem. 96, 103589. doi:10.1016/j.bioorg.2020.103589
Sravya, G., Balakrishna, A., Zyryanov, G. V., Mohan, G., Reddy, C. S., and Bakthavatchala Reddy, N. (2021). Synthesis of α-aminophosphonates by the Kabachnik-Fields Reaction. Phosphorus, Sulfur, Silicon Relat. Elem. 196 (4), 353–381. doi:10.1080/10426507.2020.1854258
Sun, J., Mou, C., Liu, C., Huang, R., Zhang, S., Zheng, P., et al. (2018). Enantioselective Access to Multi-Cyclic α-amino Phosphonates via Carbene-Catalyzed Cycloaddition Reactions between Enals and Six-Membered Cyclic Imines. Org. Chem. Front. 5 (20), 2992–2996. doi:10.1039/c8qo00877a
Szajnman, S. H., Ravaschino, E. L., Docampo, R., and Rodriguez, J. B. (2005). Synthesis and Biological Evaluation of 1-Amino-1,1-Bisphosphonates Derived from Fatty Acids against Trypanosoma Cruzi Targeting Farnesyl Pyrophosphate Synthase. Bioorg. Med. Chem. Lett. 15 (21), 4685–4690. doi:10.1016/j.bmcl.2005.07.060
Tajti, Á., Szabó, K. E., Popovics-Tóth, N., Iskanderov, J., Perdih, F., Hackler, L., et al. (2021). PMDTA-catalyzed Multicomponent Synthesis and Biological Activity of 2-Amino-4h-Chromenes Containing a Phosphonate or Phosphine Oxide Moiety. Org. Biomol. Chem. 19 (31), 6883–6891. doi:10.1039/d1ob01204e
Thévenin, M., Chen, G., Kantham, S., Sun, C., Glogauer, M., and Young, R. N. (2021). Design, Synthesis, Pharmacokinetics, and Biodistribution of a Series of Bone-Targeting EP4 Receptor Agonist Prodrugs for Treatment of Osteoporosis and Other Bone Conditions. ACS Pharmacol. Transl. Sci. 4 (2), 908–925.
Tian, Y.-P., Xu, F., Wang, Y., Wang, J.-J., and Li, H.-L. (2009). PPh3-catalysed One-Pot Three-Component Syntheses of α-aminophosphonates under Solvent-free Conditions. J. Chem. Res. 2009 (2), 78–80. doi:10.3184/030823409x401097
Torres-Huerta, A., Chan, T. G., White, A. J. P., and Vilar, R. (2020). Molecular Recognition of Bisphosphonate-Based Drugs by Di-zinc Receptors in Aqueous Solution and on Gold Nanoparticles. Dalton Trans. 49 (18), 5939–5948. doi:10.1039/d0dt00930j
Varga, P. R., and Keglevich, G. (2021). Synthesis of α-Aminophosphonates and Related Derivatives; the Last Decade of the Kabachnik-Fields Reaction. Molecules 26, 2511. doi:10.3390/molecules26092511
Vasikaran, S. D. (2001). Bisphosphonates: an Overview with Special Reference to Alendronate. Ann. Clin. Biochem. 38 (6), 608–623. doi:10.1258/0004563011901037
Wang, B. L., Zhu, H. W., Li, Z. M., Zhang, X., Yu, S. J., Ma, Y., et al. (2019). One‐pot Synthesis, Structure and Structure-Activity Relationship of Novel Bioactive Diphenyl/diethyl (3‐bromo‐1‐(3‐chloropyridin‐2‐yl)‐1 H ‐pyrazol‐5‐yl)(arylamino)methylphosphonates. Pest. Manag. Sci. 75 (12), 3273–3281. doi:10.1002/ps.5449
Worms, K., Schmidt-Dunker, M. J. b. G. K., and Maier, L. (1976). Organic Phosphorus Compounds. New York: Wiley, 7.
Wu, M., Chen, R., and Huang, Y. (2004). Convenient Synthesis of Analogs of Aminomethylene gem‐Diphosphonic Acid from Amines without Catalyst. Synth. Commun. 34 (8), 1393–1398. doi:10.1081/scc-120030688
Xie, H., Chen, G., and Young, R. N. (2017). Design, Synthesis, and Pharmacokinetics of a Bone-Targeting Dual-Action Prodrug for the Treatment of Osteoporosis. J. Med. Chem. 60 (16), 7012–7028. doi:10.1021/acs.jmedchem.6b00951
Yu, C.-M., Wang, B., and Chen, Z.-W. (2008). A Novel Synthesis of Alkylamino Substituted Methylenediphosphonates Using Bis(trichloromethyl) Carbonate and RCONR1R2. Chin. J. Chem. 26 (10), 1899–1901. doi:10.1002/cjoc.200890341
Yu, Y.-C., Kuang, W.-B., Huang, R.-Z., Fang, Y.-L., Zhang, Y., Chen, Z.-F., et al. (2017). Design, Synthesis and Pharmacological Evaluation of New 2-Oxo-Quinoline Derivatives Containing α-aminophosphonates as Potential Antitumor Agents. Med. Chem. Commun. 8 (6), 1158–1172. doi:10.1039/c7md00098g
Zha, Z., Wu, Z., Choi, S. R., Ploessl, K., Smith, M., Alexoff, D., et al. (2020). A New [68Ga]Ga-HBED-CC-Bisphosphonate as a Bone Imaging Agent. Mol. Pharm. 17 (5), 1674–1684. doi:10.1021/acs.molpharmaceut.0c00103
Zhan, Z. P., and Li, J. P. (2005). Bismuth(III) Chloride-Catalyzed Three‐Component Coupling: Synthesis of α‐Amino Phosphonates. Synth. Commun. 35 (19), 2501–2508. doi:10.1080/00397910500212692
Zhang, B., Hu, X.-T., Gu, J., Yang, Y.-S., Duan, Y.-T., and Zhu, H.-L. (2020). Discovery of Novel Sulfonamide-Containing Aminophosphonate Derivatives as Selective COX-2 Inhibitors and Anti-tumor Candidates. Bioorg. Chem. 105, 104390. doi:10.1016/j.bioorg.2020.104390
Zhang, G., Hao, G., Pan, J., Zhang, J., Hu, D., and Song, B. (2016). Asymmetric Synthesis and Bioselective Activities of α-Amino-phosphonates Based on the Dufulin Motif. J. Agric. Food Chem. 64 (21), 4207–4213. doi:10.1021/acs.jafc.6b01256
Keywords: phosphonates, bisphosphonates, inhibitors, medicine, insecticides, osteoporosis
Citation: Kaboudin B, Daliri P, Faghih S and Esfandiari H (2022) Hydroxy- and Amino-Phosphonates and -Bisphosphonates: Synthetic Methods and Their Biological Applications. Front. Chem. 10:890696. doi: 10.3389/fchem.2022.890696
Received: 06 March 2022; Accepted: 28 April 2022;
Published: 01 June 2022.
Edited by:
Pawel Kafarski, Wrocław University of Technology, PolandReviewed by:
Francisco Palacios, University of the Basque Country, SpainCopyright © 2022 Kaboudin, Daliri, Faghih and Esfandiari. This is an open-access article distributed under the terms of the Creative Commons Attribution License (CC BY). The use, distribution or reproduction in other forums is permitted, provided the original author(s) and the copyright owner(s) are credited and that the original publication in this journal is cited, in accordance with accepted academic practice. No use, distribution or reproduction is permitted which does not comply with these terms.
*Correspondence: Babak Kaboudin, a2Fib3VkaW5AaWFzYnMuYWMuaXI=, a2Fib3VkaW5AZ21haWwuY29t
†These authors have contributed equally to this work
Disclaimer: All claims expressed in this article are solely those of the authors and do not necessarily represent those of their affiliated organizations, or those of the publisher, the editors and the reviewers. Any product that may be evaluated in this article or claim that may be made by its manufacturer is not guaranteed or endorsed by the publisher.
Research integrity at Frontiers
Learn more about the work of our research integrity team to safeguard the quality of each article we publish.