- 1Education Ministry Key Laboratory of Renewable Energy Advanced Materials and Manufacturing Technology, Yunnan Normal University, Kunming, China
- 2School of Literature and Art Media, Anhui International Studies University, Hefei, China
Electrochemical water splitting to produce molecular hydrogen and oxygen provides a promising strategy engineering for scalable hydrogen production with high purity. Unfortunately, the sluggish kinetics of oxygen evolution reactions (OER) due to the interdependence multiple steps procedure require high overpotential to achieve appreciable catalytic current density, resulting in relatively low energy conversion efficiencies. Therefore, development of high-performance OER electrocatalysts is vital to drive the commercial application of water splitting. This review highlights current progress of representative catalyst electrocatalysts in the past decade. Active site regulation for excellent OER performance of precious metal single atoms catalyst, high-entropy alloy, transition metals oxides, transition metal chalcogenide are emphasized. And a more in-depth exploration of OER reaction mechanism by in situ technique and DFT results will be conducted. This review can provide the basis for the development and modification of OER electrocatalysts.
Introduction
Hydrogen energy is regarded as a renewable energy with application prospect because of its advantages such as wide source, high combustion heat value, high efficiency and zero pollution (Cai et al., 2020; Zhao et al., 2020; Bai et al., 2021; Tang et al., 2021). Although hydrogen is abundant in earth, it mainly exists in the natural world in the forms of a compound in water, fossil fuels and other media, and rarely exists in the form of H2 (Yan et al., 2021; Yu et al., 2022a). Therefore, the realization of efficient hydrogen production is the basis for the application of hydrogen energy (Yao et al., 2019; Wang et al., 2020; Zhao et al., 2021; Yu et al., 2022b). Electrolysis of water can directly convert water into hydrogen and oxygen to obtain high purity hydrogen. At the same time, the source of water is abundant, so the preparation of hydrogen by electrolysis of water is regarded as an ideal method for hydrogen production (Shah et al., 2022). Electrocatalytic water splitting includes hydrogen evolution reaction (HER) on cathode and oxygen evolution reaction (OER) on anode. However, OER is a four-electron coupled reaction, generating a high kinetic barrier and suffering from high overpotential and low efficiency (Hu et al., 2021). At present, the precious metals Ir, Ru, and their oxides show excellent OER electrocatalytic performance (Lee et al., 2012; Danilovic D. N. et al., 2014; Osgood et al., 2016). However, due to the scarcity of earth content, high price, and poor stability, precious metal catalysts cannot meet the large-scale industrial application of hydrogen production by electrolysis of water. Therefore, to exploit low cost, non-noble metal electrocatalyst and replace noble metal catalyst have become an important research topic, and design and modification of the active site have been recognized as the main approach to promote the intrinsic activity.
In general, the OER is a more complex process in comparison with the HER system, the mechanism of OER is complicated and still debatable on the anode catalysts. For conventional OER mechanism, a four-step proton/electron transfer step was proposed as described in Eqs 1–4.
where ∗ and ∗OH, ∗O, ∗OOH represent the active site and the adsorbed intermediate on the active site, respectively. In detail, OH− groups first adsorb on catalyst surface with release of an electron. Then the adsorbed ∗OH undergoes deprotonation to form O∗. The following O∗to react with another OH− to form the HOO∗intermediate subsequently decomposes to O2(g) and closing the whole OER cycle. The Gibbs free-energy change (ΔGi) for these four consecutive electrochemical reactions (Eqs 1–4) can be described in Eqs 5–8. The proton/electron reaction step with the maximum ΔG is regarded as the determining step, and the overpotential can be represented by ΔGmax/e-1.23. An ideal OER requires all the four elemental steps with reaction free energies 1.23 eV to realize no overpotential (ΔG1 = ΔG2 = ΔG3 = ΔG4 = 1.23 eV). Therefore, modulating reasonably the adsorption energy of intermediates ∗OH, ∗O, and ∗OOH) by optimized electronic structure has been considered as one of the promising strategy for enhancing OER.
Regulation of Active Site for Promoting OER
Precious Metals Single Atom Catalyst
Precious metals as OER catalysts were reported as early as the 1860s, and the catalytic activity of different precious metals from low to high is Ru, Ir, Pd, Rh, and Pt in acidic electrolyte (Damjanovic et al., 1966; Chen Z. et al., 2021). Under the electric water driving voltage, the surface of precious metals will be transformed into corresponding oxides due to the strong oxidation atmosphere, and the surface will generate an oxide layer. The higher the electrocatalytic activity is, the easier it is to adsorb the intermediate state of reaction and form oxide layer. So, Ru has the highest catalytic activity but poor stability, while Pt requires a high overvoltage to occur OER but has good stability (Danilovic N. et al., 2014). At present, noble metal oxides such as rutile structure RuO2 and IrO2 exhibit excellent electrocatalytic activity under both acidic and alkaline conditions, and have been regarded as the benchmark of OER electrocatalysts. However, the employment of precious metal significantly aggravates the industrial hydrogen production costs via electrochemical water splitting. Single-atom catalysts (SACs) can minimize the utilization of precious metals by maximizing the atom efficiency; meanwhile, the controllable coordination environment and metal support interactions endow SACs as a new paradigm toward designing high-performance OER catalysts. The individual atomic structure of SACs and the interfacial effect between single atom and substrate support can effectively control the electron structure, which endows SACs with suitable and adjustable adsorption energy for reaction species, accelerating the OER kinetic process. Cao et al. (Lei et al., 2022) anchored high distribution density Ir single atom on the nickel-iron sulfide substrate support (Ir/NFS) by a simple two-step electrodeposition (Figure 1A). The obtained Ir/NFS catalyst possesses an excellent OER catalytic performance with a low overpotential of ∼170 mV at 10 mA cm−2 and a high turnover frequency of 9.85 s−1 at an overpotential of 300 mV in 1 M KOH electrolyte, which is superior to most previously reported OER catalysts, and even commercial IrO2 catalysts (Figure 1B). Recently, Ir single atoms were also anchored on Ni2P catalyst (IrSA-Ni2P) (Wang et al., 2021), the substrate change results in the significant improvement of OER performance, and IrSA-Ni2P obtains a record-high OER performance with an overpotential of 149 mV at 10 mA·cm−2. Meanwhile, the IrSA-Ni2P has a large current density of 154 mA·cm−2 at an overpotential of 300 mV, which is almost 28-fold that of the most widely used IrO2 catalyst. Gu et al. think that the isolated Ir-O-P/Ni-O-P bonding can optimize the adsorption energy of OER intermediate species to accelerate the adsorption/desorption process (Figure 1C). Furthermore, Cui et al. employ in situ cryogenic-photochemical reduction synthesis and DFT method to trace the origin of enhanced water oxidation activity of Ir single atom on NiFe oxyhydroxide substrate (Zheng et al., 2021). The X-ray absorption near edge structure (XANES) spectra results show that the valence of +5.3 for Ir0.1/Ni9Fe SAC, and the high-oxidation state of Ir possesses excellent OER performance with an overpotential of 183 mV at 10 mA·cm−2. The DFT results reveal that the high-oxidation state NiFeIr SAC (+4.8 and +5.6) models can weaker *OH binding and improved *OH and *OOH scaling to obtain a very low overpotential of 0.184 V.
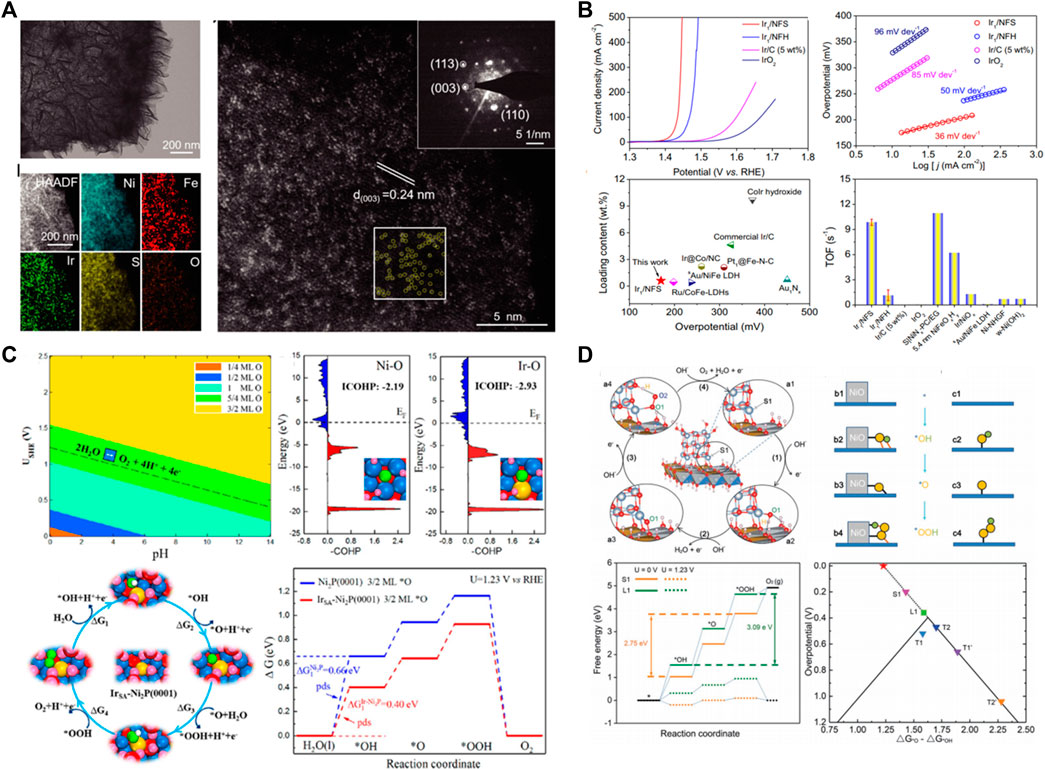
FIGURE 1. (A) Electron microscope analysis of Ir1/NFS. (B) Electrochemical OER performance of Ir1/NFS, Ir1/NFH, Ir/C (5 wt%), and IrO2 in 1.0 M KOH. (C) DFT calculation indicated that the isolated Ir-O-P/Ni-O-P bonding can optimize the adsorption energy of OER intermediate species to accelerate the adsorption/desorption process. (D) Structure model and DFT + U calculation results of NiO/NiFe LDH interface.
High-Entropy Alloy Electrocatalyst
High-entropy alloy has been considered as the remarkable nonprecious metal-based OER electrocatalysts due to their adjustable composition, complex surface, enhanced wear resistance, etc. (Li et al., 2021; Park et al., 2022; Han et al., 2022). More importantly, OER is a four-electron/four-proton reaction process and involves the adsorption/desorption of several intermediates (HO*, O*, and HOO*) on the catalyst surface. Numerous studies revealed that the adsorption energy of intermediates has scale relation, such as ΔG(*OOH) = ΔG(*OH)+3.2, the ΔG*OOH can be restricted by ΔG*OH, which limit a minimum theoretical overpotential of about 0.37 V, and the adsorption energy of intermediates cannot be optimized independently on a catalyst composed of a single type of site. So the synergistic effect of multi-site was put forward to break or overcome the adsorption-energy scaling relations (Song et al., 2021). Du et al. design the NiO/NiFe LDH interface to introduce additional active sites, and the intermediates can be adsorbed by the interfacial dual-site, so the adsorption energy of each intermediate can be adjusted independently to decrease the overpotential into 0.2 V (Figure 1D) (Gao et al., 2019). As expected, NiO/NiFe LDH possesses a rather high TOF (0.71 s−1) and ultralow overpotential (0.2 V at 30 mA cm−2). High-entropy alloy has multiple attributes sites to synergistically reduce the multistep process of OER instead of the simple superposition of the performance of each metal ion, so multicomponent alloy generally possesses reinforced electrocatalytic performance compared with that of single-element or bimetallic alloy materials.
Jiang et al. synthesize CoNiCuMnAl)/C nanoparticles using a facile pyrolysis, and the optimized catalyst has low overpotential (215 mV at 10 mA cm−2) and Tafel slope (35.6 mV dec−1) (Wang et al., 2022). The DFT calculations show that Ni/Co can reduce the barrier potential of rate-determining step (O*→OOH*), and Al sites can promote the electrical conductivity (Feng et al., 2020). Yu et al. introduce Cr into representative NiCoFe system to synthesize (CrFeCoNi)97O3, and Cr ion can be etched during OER to promote the interfacial reconstruction and amorphization, resulting in an improved OER performance with a low overpotential of 196 mV at 10 mA cm−2 and Tafel slope of 29 mV dec−1 (Chen Z. J. et al., 2021). Generally, amorphous structure can offer more active sites and thus exhibit better OER performance compared with their crystalline counterparts. Liang et al. employed Mn doping to promote self-construction of active β-NiOOH intermediates for NiFeCoMnAl oxide, the β-NiOOH with optimized adsorption of *OH and *O intermediates can as active catalytic sites. The prepared NiFeCoMnAl oxide possesses improved OER performance compared with that of the reference sample NiFeCo and NiFeCoAl oxide (Han et al., 2022). Although high-entropy alloys have potential applications in electrocatalytic OER fields, they suffer from various challenges, such as complex synthesis method and the coordination mechanism of multisites.
Transition Metal Oxide Electrocatalyst
Since the earliest catalysts for electrocatalytic oxygen evolution, nickel, cobalt, iron-based oxides, hydroxides materials have lower catalytic activity than the precious metal iridium ruthenium compound, but their advantages such as lower price and excellent stability are bound to become the focus of research (Zhang et al., 2018; Gao et al., 2021; Wu et al., 2021). Cobalt oxide is at the top of the OER volcano diagram. Meanwhile, Co3O4 did not change significantly in the process of high potential and OER test by comparing the absorption spectra of Co3O4 under different voltages, which means that Co3O4 can maintain a very stable structure under the condition of OER reaction; thus, considerable efforts have been devoted to exploring efficient Co3O4 electrocatalysts. Co3O4 belongs to the spinel family, in which Co +2 and Co +3 play different roles in OER process (García-Mota et al., 2012; Bergmann et al., 2015; Lee et al., 2022) Wang et al. (Bo et al., 2005) studied the role of Co +2 and CO +3 ions in the OER process by replacing Co +2 with Zn and Co +3 with Al. The results show that the OER activity of CoAl2O4 (in the presence of Co +2) is similar to that of Co3O4 (in the presence of Co+2), but superior to that of ZnCo2O4 (in the presence of Co +3), which confirms that the bivalent Co+2 plays a dominant role in OER activity.
Electronic structure modulation is widely used to regulate the active site so as to optimize the macroscopic catalytic performance of catalysts. Wang’s group (Xu L. et al., 2016) modified the surface of Co3O4 catalyst by plasma technology. Plasma etching produces more oxygen vacancies on the surface of Co3O4, which is beneficial to increase the specific surface area of the material and ensure that the Co3O4 surface has more OER catalytic active sites. In addition, oxygen vacancies introduce impurity energy into the Co3O4 band gap, which improves the electrical conductivity of the material. Compared with the original Co3O4, the plasma-sculpted Co3O4 has a faster OER kinetic process and a lower initial potential. At a voltage of 1.6 V, the current density of the plasmonized Co3O4 nanosheets was 10 times that of the untreated Co3O4. Xiao et al. (He et al., 2020) also adopt a novel highly controlled ion irradiation technology to regulate the electronic properties of Co3O4, and the active electron density can be precisely regulated by the concentration of oxygen vacancy contained Co3O4 (Co3O4-Ov) (Figure 2A).The optimized Co3O4-based catalysts exhibit an excellent overpotential of 260 mV at 10 mA cm−2(Figure 2B). Jiang et al. also report that oxygen vacancy not only enhance the adsorption of intermediates, and can also affect the water oxidation path (Figure 2B) (Wang et al., 2017).
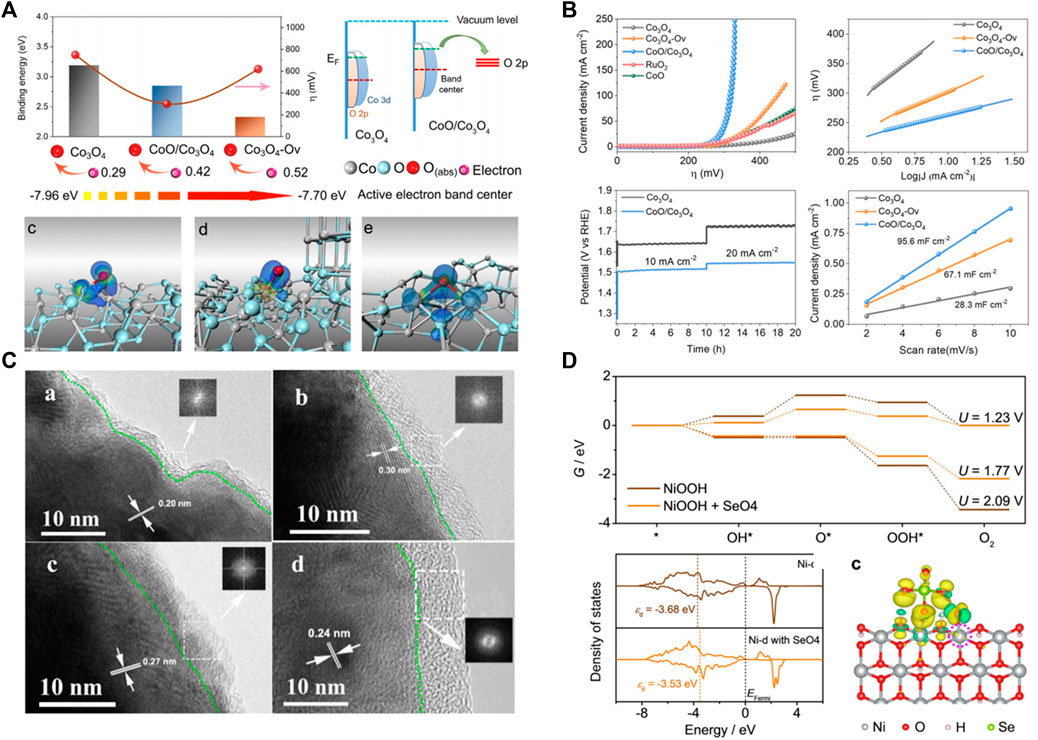
FIGURE 2. (A) DFT calculations of Co3O4, CoO/Co3O4, and Co3O4-Ov. (B) Electrochemical catalytic measurement of OER on Co3O4, Co3O4-Ov, CoO/Co3O4, CoO, and RuO2. (C) HRTEM images of postcatalysis samples of Ni, Ni3Se2, NiSe, and NiO. (D) DFT calculations of NiOOH and NiOOH + SeO4 indicate that the adsorption of SeO4 on NiOOH can shift up the d band center, resulting in a stronger bonding with the OER intermediates.
Transition Metal Non-oxides
Although transition metals oxides have been regarded as a potential non-noble OER catalyst in alkaline conditions, their semiconductor property restricted industrial applications. Metal-phase transition metal sulfur (selenium, phosphorus, nitrogen) compounds, due to their good electrical conductivity, can effectively reduce the overpotential caused by impedance, superior to the corresponding semiconductor properties of transition metal oxides, and is also widely used in OER electrode materials. But OER often work in a larger potential, oxygen intermediates (*O, *OH, and * OOH) easily adsorbed on the catalyst surface, causing the transition metal (nitrogen, phosphorus, selenium) compound in the process of OER irreversible oxidation process of surface, the surface gradually phase transition metal oxide or hydroxide (Ji et al., 2020; Xu K. et al., 2016). Xie’s group (Xu K. et al., 2016) systematically compared and analyzed the structural stability of Ni, Ni3Se2, NiSe, and NiO as oxygen evolution electrodes before and after testing, and found that the surface of the catalyst would be oxidized to form an oxide layer of about 10 nm (Figure 2C). Mabayoje et al. (Mabayoje et al., 2016) also found a similar conclusion that the XRD of NiS after OER test failed to observe obvious diffraction peaks of nickel sulfide and nickel oxide. Furthermore, XPS results indicate that the surface of NiS is transformed into NiOx, which means that the surface phase of sulfide is transformed into amorphous oxide under OER operation. Cui et al. also report that CoS2, Co0.5Fe0.5S2, and Co0.37Ni0.26Fe0.37S2 were converted to CoO, Co0.5Fe0.5O2, Co0.37Ni0.26Fe0.37O2, respectively, during cyclic voltammetry by SEM and TEM results (Chen et al., 2015). Although the in-situ formed oxides are recognized as the real active species because t the catalyst reaction site mainly comes from the surface, the different transition metals (selenium, phosphorus, nitrogen) possesses distinct OER performance, and the role of nonmetallic elements on OER is still indistinct. Zhong et al. researched the influence of nonmetal S atom in OER of FeMOF, and the results showed that in situ formed -SO3 groups under OER condition can stabilize *OH or *OOH species by capturing their H+, which can break linear relationships between ΔGOH and ΔGOOH to reduce the overpotential. As a result, FeMOFs-SO3 shows a low overpotential of 218 mV at a current density of 10 mA cm−2. Zhang et al. also report that in-site formative selenate (SeO42-) during the OER process can promote the OER activity (Shi et al., 2020). DFT calculations indicate that the adsorption of SeO4 on NiOOH can shift up the d band center, resulting in a stronger bonding with the OER intermediates (Figure 2D).
Summary and Perspectives
The OER is a key process involved in energy and environment-related technologies, a great deal of research work has been focused on the development of efficient and stable OER electrocatalysts. Different catalytic systems, such as precious metals single-atom catalyst, high-entropy alloy, transition metals oxides, and transition metal chalcogenide, have unique features and advantages as OER electrocatalysts, and their performance records are constantly being improved by modified active center. On the one hand, by optimizing the electronic structure of the active site, the adsorption energy of the intermediate can reach the fixed point of the volcano diagram. On the other hand, the linear constraint between adsorption energy can be broken through the coordination of multiple sites, so as to obtain excellent catalytic performance.
However, the current OER catalysts suffer from various challenges. 1) The activity-stability of relationship. Stability is a another important parameter of OER for industrialization, most reported catalysts for OER undergo in situ electrochemical tuning to form the amorphous oxidation layer, which is the main active species for OER, the faster in situ electrochemical transformations can promote the OER activity. So the activity and stability of catalyst have some relationship, which requires the in situ characterization techniques and theoretical calculations for a deeper investigate. 2) The structure-activity of relationship. The adsorption energy of reaction intermediate depends on electron structure of catalyst, but the correlation between multiple reaction intermediate (*OH, *O, and *OOH) and electron structure is not identified. And the multisite synergistic effect between metal and metal sites or metal and nonmetal sites has not been deeply revealed. In general, a deeper understanding of OER reaction mechanism is urgently needed to support the design and exploitation of high-performance catalyst in the future.
Author Contributions
YT contributed to literature analysis and summary and manuscript writing. TZ is responsible for language modification. XW is responsible for the collecting and processing the figures in the article. SD acts as a supervisor to guide the writing and revision of the manuscript. All authors contributed to manuscript revision, read, and approved the submitted version.
Funding
This research was supported by the National Natural Science Foundation of China (Grant No. 61864012).
Conflict of Interest
The authors declare that the research was conducted in the absence of any commercial or financial relationships that could be construed as a potential conflict of interest.
Publisher’s Note
All claims expressed in this article are solely those of the authors and do not necessarily represent those of their affiliated organizations, or those of the publisher, the editors and the reviewers. Any product that may be evaluated in this article, or claim that may be made by its manufacturer, is not guaranteed or endorsed by the publisher.
References
Bai, J., Mei, J., Liao, T., Sun, Q., Chen, Z. G., and Sun, Z. (2021). Molybdenum‐Promoted Surface Reconstruction in Polymorphic Cobalt for Initiating Rapid Oxygen Evolution. Adv. Energ. Mater. 12 (5), 2103247. doi:10.1002/aenm.202103247
Bergmann, A., Martinez-Moreno, E., Teschner, D., Chernev, P., Gliech, M., de Araújo, J. F., et al. (2015). Reversible Amorphization and the Catalytically Active State of Crystalline Co3O4 during Oxygen Evolution. Nat. Commun. 6, 8625. doi:10.1038/ncomms9625
Bo, C., Li, J., Yang, X., Hong, L., and Ning, W. (2005). Electrophoretic Deposition of ZnCo2O4 Spinel and its Electrocatalytic Properties for Oxygen Evolution Reaction. Electrochimica Acta 50 (10), 2059–2064. doi:10.1016/j.electacta.2004.09.014
Cai, W., Chen, R., Yang, H., Tao, H. B., Wang, H.-Y., Gao, J., et al. (2020). Amorphous versus Crystalline in Water Oxidation Catalysis: A Case Study of NiFe Alloy. Nano Lett. 20 (6), 4278–4285. doi:10.1021/acs.nanolett.0c00840
Chen, W., Wang, H., Li, Y., Liu, Y., Sun, J., Lee, S., et al. (2015). In Situ Electrochemical Oxidation Tuning of Transition Metal Disulfides to Oxides for Enhanced Water Oxidation. ACS Cent. Sci. 1 (5), 244–251. doi:10.1021/acscentsci.5b00227
Chen, Z. J., Zhang, T., Gao, X. Y., Huang, Y. J., Qin, X. H., Wang, Y. F., et al. (2021). Engineering Microdomains of Oxides in High-Entropy Alloy Electrodes toward Efficient Oxygen Evolution. Adv. Mater. 33 (33), e2101845. doi:10.1002/adma.202101845
Chen, Z., Liu, D., Gao, Y., Zhao, Y., Xiao, W., Xu, G., et al. (2021). Corrosive-coordinate Engineering to Construct 2D-3D Nanostructure with Trace Pt as Efficient Bifunctional Electrocatalyst for Overall Water Splitting. Sci. China Mater.. doi:10.1007/s40843-021-1943-5
Damjanovic, A., Dey, A., and Bockris, J. O. M. (1966). Electrode Kinetics of Oxygen Evolution and Dissolution on Rh, Ir, and Pt-Rh Alloy Electrodes. J. Electrochem. Soc. 113 (7), 739. doi:10.1149/1.2424104
Danilovic, D. N., Subbaraman, D. R., Chang, D., Chang, D., Kang, D. Y., Snyder, D. J., et al. (2014). Using Surface Segregation to Design Stable Ru-Ir Oxides for the Oxygen Evolution Reaction in Acidic Environments. Angew. Chem. Int. Ed. Engl. 53 (51), 14016–14021. doi:10.1002/anie.201406455
Danilovic, N., Subbaraman, R., Chang, K., Chang, S. H., Kang, Y., Snyder, J. D., et al. (2014). Activity-Stability Trends for the Oxygen Evolution Reaction on Monometallic Oxides in Acidic Environments. J. Phys. Chem. Lett. 5 (14), 2474–2478. doi:10.1021/jz501061
Feng, K., Zhang, D., Liu, F., Li, H., Xu, J., Xia, Y., et al. (2020). Highly Efficient Oxygen Evolution by a Thermocatalytic Process Cascaded Electrocatalysis over Sulfur‐Treated Fe‐Based Metal–Organic‐Frameworks. Adv. Energ. Mater. 10 (16), 2000184. doi:10.1002/aenm.202000184
Gao, X., Li, X., Yu, Y., Kou, Z., Wang, P., Liu, X., et al. (2021). Synergizing Aliovalent Doping and Interface in Heterostructured NiV Nitride@oxyhydroxide Core-Shell Nanosheet Arrays Enables Efficient Oxygen Evolution. Nano Energy 85. doi:10.1016/j.nanoen.2021.105961
Gao, Z. W., Liu, J. Y., Chen, X. M., Zheng, X. L., Mao, J., Liu, H., et al. (2019). Engineering NiO/NiFe LDH Intersection to Bypass Scaling Relationship for Oxygen Evolution Reaction via Dynamic Tridimensional Adsorption of Intermediates. Adv. Mater. 31 (11), e1804769. doi:10.1002/adma.201804769
García-Mota, M., Bajdich, M., Viswanathan, V., Vojvodic, A., Bell, A. T., and Nørskov, J. K. (2012). Importance of Correlation in Determining Electrocatalytic Oxygen Evolution Activity on Cobalt Oxides. J. Phys. Chem. C. 116 (39), 21077–21082. doi:10.1021/jp306303y
Han, M., Wang, C., Zhong, J., Han, J., Wang, N., Seifitokaldani, A., et al. (2022). Promoted Self-Construction of β-NiOOH in Amorphous High Entropy Electrocatalysts for the Oxygen Evolution Reaction. Appl. Catal. B: Environ. 301, 120764. doi:10.1016/j.apcatb.2021.120764
He, D., Song, X., Li, W., Tang, C., Liu, J., Ke, Z., et al. (2020). Active Electron Density Modulation of Co 3 O 4 ‐Based Catalysts Enhances Their Oxygen Evolution Performance. Angew. Chem. Int. Ed. 59 (17), 6929–6935. doi:10.1002/anie.202001681
Hu, X., Luo, G., Guo, X., Zhao, Q., Wang, R., Huang, G., et al. (2021). Origin of the Electrocatalytic Oxygen Evolution Activity of Nickel Phosphides: In-Situ Electrochemical Oxidation and Cr Doping to Achieve High Performance. Sci. Bull. 66 (7), 708–719. doi:10.1016/j.scib.2020.11.009
Ji, P., Luo, X., Chen, D., Jin, H., Pu, Z., Zeng, W., et al. (2020). Significantly Improved Water Oxidation of CoP Catalysts by Electrochemical Activation. ACS Sustain. Chem. Eng. 8 (48), 17851–17859. doi:10.1021/acssuschemeng.0c07169
Lee, W. H., Han, M. H., Ko, Y.-J., Min, B. K., Chae, K. H., and Oh, H.-S. (2022). Electrode Reconstruction Strategy for Oxygen Evolution Reaction: Maintaining Fe-CoOOH Phase with Intermediate-Spin State during Electrolysis. Nat. Commun. 13 (1), 605. doi:10.1038/s41467-022-28260-5
Lee, Y., Suntivich, J., May, K. J., Perry, E. E., and Shao-Horn, Y. (2012). Synthesis and Activities of Rutile IrO2 and RuO2 Nanoparticles for Oxygen Evolution in Acid and Alkaline Solutions. J. Phys. Chem. Lett. 3 (3), 399–404. doi:10.1021/jz2016507
Lei, Z., Cai, W., Rao, Y., Wang, K., Jiang, Y., Liu, Y., et al. (2022). Coordination Modulation of Iridium Single-Atom Catalyst Maximizing Water Oxidation Activity. Nat. Commun. 13 (1), 24. doi:10.1038/s41467-021-27664-z
Li, H., Lai, J., Li, Z., and Wang, L. (2021). Multi‐Sites Electrocatalysis in High‐Entropy Alloys. Adv. Funct. Mater. 31 (47). doi:10.1002/adfm.202106715
Mabayoje, O., Shoola, A., Wygant, B. R., and Mullins, C. B. (2016). The Role of Anions in Metal Chalcogenide Oxygen Evolution Catalysis: Electrodeposited Thin Films of Nickel Sulfide as "Pre-catalysts". ACS Energ. Lett. 1, 195–201. doi:10.1021/acsenergylett.6b00084
Osgood, H., Devaguptapu, S. V., Xu, H., Cho, J., and Wu, G. (2016). Transition Metal (Fe, Co, Ni, and Mn) Oxides for Oxygen Reduction and Evolution Bifunctional Catalysts in Alkaline media. Nano Today 11, 601–625. doi:10.1016/j.nantod.2016.09.001
Park, H., Bae, J. W., Lee, T. H., Park, I. J., Kim, C., Lee, M. G., et al. (2022). Surface-Tailored Medium Entropy Alloys as Radically Low Overpotential Oxygen Evolution Electrocatalysts. Small 18, e2105611. doi:10.1002/smll.202105611
Shah, K., Dai, R., Mateen, M., Hassan, Z., Zhuang, Z., Liu, C., et al. (2022). Cobalt Single Atom Incorporated in Ruthenium Oxide Sphere: A Robust Bifunctional Electrocatalyst for HER and OER. Angew. Chem. Int. Ed. Engl. 61 (4), e202114951. doi:10.1002/anie.202114951
Shi, Y., Du, W., Zhou, W., Wang, C., Lu, S., Lu, S., et al. (2020). Unveiling the Promotion of Surface‐Adsorbed Chalcogenate on the Electrocatalytic Oxygen Evolution Reaction. Angew. Chem. Int. Ed. 59 (50), 22470–22474. doi:10.1002/anie.202011097
Song, H., Wang, J., Zhang, Z., Shai, X., and Guo, Y. (2021). Synergistic Balancing Hydrogen and Hydroxyl Adsorption/desorption of Nickel Sulfide via Cation and Anion Dual-Doping for Boosting Alkaline Hydrogen Evolution. Chem. Eng. J. 420, 129842. doi:10.1016/j.cej.2021.129842
Tang, Y., Shen, K., Zheng, J., He, B., Chen, J., Lu, J., et al. (2021). D-Band Center Modulating of CoOx/Co9S8 by Oxygen Vacancies for Fast-Kinetics Pathway of Water Oxidation - ScienceDirect. Chem. Eng. J. 427, 130915. doi:10.1016/j.cej.2021.130915
Wang, J., Liu, J., Zhang, B., Wan, H., Li, Z., Ji, X., et al. (2017). Synergistic Effect of Two Actions Sites on Cobalt Oxides towards Electrochemical Water-Oxidation. Nano Energy 42, 98–105. doi:10.1016/j.nanoen.2017.10.044
Wang, J., Zhang, Z., Song, H., Zhang, B., Liu, J., Shai, X., et al. (2020). Water Dissociation Kinetic‐Oriented Design of Nickel Sulfides via Tailored Dual Sites for Efficient Alkaline Hydrogen Evolution. Adv. Funct. Mater. 31 (9), 2008578. doi:10.1002/adfm.202008578
Wang, Q., Zhang, Z., Cai, C., Wang, M., Zhao, Z. L., Li, M., et al. (2021). Single Iridium Atom Doped Ni2P Catalyst for Optimal Oxygen Evolution. J. Am. Chem. Soc. 143 (34), 13605–13615. doi:10.1021/jacs.1c04682
Wang, S., Huo, W., Fang, F., Xie, Z., Shang, J. K., and Jiang, J. (2022). High Entropy alloy/C Nanoparticles Derived from Polymetallic MOF as Promising Electrocatalysts for Alkaline Oxygen Evolution Reaction. Chem. Eng. J. 429. doi:10.1016/j.cej.2021.132410
Wu, T., Ren, X., Sun, Y., Sun, S., Xian, G., Scherer, G. G., et al. (2021). Spin Pinning Effect to Reconstructed Oxyhydroxide Layer on Ferromagnetic Oxides for Enhanced Water Oxidation. Nat. Commun. 12 (1), 3634. doi:10.1038/s41467-021-23896-1
Xu, K., Ding, H., Lv, H., Tao, S., Chen, P., Wu, X., et al. (2016). Understanding Structure-dependent Catalytic Performance of Nickel Selenides for Electrochemical Water Oxidation. ACS Catal. 7 (1), 310–315. doi:10.1021/acscatal.6b02884
Xu, L., Jiang, Q., Xiao, Z., Li, X., Huo, J., Wang, S., et al. (2016). Plasma-Engraved Co3 O4 Nanosheets with Oxygen Vacancies and High Surface Area for the Oxygen Evolution Reaction. Angew. Chem. Int. Ed. Engl. 55 (17), 5277–5281. doi:10.1002/anie.201600687
Yan, M., Zhao, Z., Cui, P., Mao, K., Chen, C., Wang, X., et al. (2021). Construction of Hierarchical FeNi3@(Fe,Ni)S2 Core-Shell Heterojunctions for Advanced Oxygen Evolution. Nano Res. 14 (11), 4220–4226. doi:10.1007/s12274-021-3531-8
Yao, Y., Hu, S., Chen, W., Huang, Z.-Q., Wei, W., Yao, T., et al. (2019). Engineering the Electronic Structure of Single Atom Ru Sites via Compressive Strain Boosts Acidic Water Oxidation Electrocatalysis. Nat. Catal. 2 (4), 304–313. doi:10.1038/s41929-019-0246-2
Yu, W., Chen, Z., Xiao, W., Chai, Y., Dong, B., Wu, Z., et al. (2022a). Phosphorus Doped Two-Dimensional CoFe2O4 Nanobelts Decorated with Ru Nanoclusters and Co–fe Hydroxide as Efficient Electrocatalysts toward Hydrogen Generation. Inorg. Chem. Front.. doi:10.1039/D2QI00086E
Yu, W., Chen, Z., Zhao, Y., Gao, Y., Xiao, W., Dong, B., et al. (2022b). An In Situ Generated 3D Porous Nanostructure on 2D Nanosheets to Boost the Oxygen Evolution Reaction for Water-Splitting. Nanoscale 14, 4566–4572. doi:10.1039/D1NR08007E
Zhang, P., Li, L., Nordlund, D., Chen, H., Fan, L., Zhang, B., et al. (2018). Dendritic Core-Shell Nickel-Iron-Copper Metal/metal Oxide Electrode for Efficient Electrocatalytic Water Oxidation. Nat. Commun. 9 (1), 381. doi:10.1038/s41467-017-02429-9
Zhao, S., Tan, C., He, C.-T., An, P., Xie, F., Jiang, S., et al. (2020). Structural Transformation of Highly Active Metal-Organic Framework Electrocatalysts during the Oxygen Evolution Reaction. Nat. Energ. 5 (11), 881–890. doi:10.1038/s41560-020-00709-1
Zhao, Y., Gao, Y., Chen, Z., Li, Z., Ma, T., Wu, Z., et al. (2021). Trifle Pt Coupled with NiFe Hydroxide Synthesized via Corrosion Engineering to Boost the Cleavage of Water Molecule for Alkaline Water-Splitting. Appl. Catal. B: Environ. 297, 120395. doi:10.1016/j.apcatb.2021.120395
Keywords: oxygen evolution reaction, precious metals, transition metals, oxides, Sulfides
Citation: Tang Y, Zhang T, Wu X and Deng S (2022) Active Sites Regulation for High-Performance Oxygen Evolution Reaction Electrocatalysts. Front. Chem. 10:889470. doi: 10.3389/fchem.2022.889470
Received: 04 March 2022; Accepted: 28 March 2022;
Published: 27 April 2022.
Edited by:
Lin Lv, Hubei University, ChinaReviewed by:
Zexing Wu, Qingdao University of Science and Technology, ChinaYunjun Ruan, Guizhou University, China
Copyright © 2022 Tang, Zhang, Wu and Deng. This is an open-access article distributed under the terms of the Creative Commons Attribution License (CC BY). The use, distribution or reproduction in other forums is permitted, provided the original author(s) and the copyright owner(s) are credited and that the original publication in this journal is cited, in accordance with accepted academic practice. No use, distribution or reproduction is permitted which does not comply with these terms.
*Correspondence: Shukang Deng, skdeng@126.com