- 1School of Chemical Sciences and Engineering, Yachay Tech University, Urcuquí, Ecuador
- 2Laboratorio de Síntesis Orgánica, Facultad de Química, Universidad de la Habana, La Habana, Cuba
- 3School of Biological Sciences and Engineering, Yachay Tech University, Urcuquí, Ecuador
- 4Department of Surfactants and Nanobiotechnology, Institute for Advanced Chemistry of Catalonia (IQAC-CSIC), Barcelona, Spain
- 5CIBER-BBN, Networking Centre of Bioengineering, Biomaterials, and Nanomedicine and Department of Organic Chemistry, University of Barcelona, Barcelona, Spain
- 6School of Chemistry and Physics, University of KwaZulu-Natal, Durban, South Africa
Cancer is one of the diseases with the highest mortality rate. Treatments to mitigate cancer are usually so intense and invasive that they weaken the patient to cure as dangerous as the own disease. From some time ago until today, to reduce resistance generated by the constant administration of the drug and improve its pharmacokinetics, scientists have been developing drug delivery system (DDS) technology. DDS platforms aim to maximize the drugs’ effectiveness by directing them to reach the affected area by the disease and, therefore, reduce the potential side effects. Erythrocytes, antibodies, and nanoparticles have been used as carriers. Eleven antibody–drug conjugates (ADCs) involving covalent linkage has been commercialized as a promising cancer treatment in the last years. This review describes the general features and applications of DDS focused on the covalent conjugation system that binds the antibody carrier to the cytotoxic drug.
1 Introduction
The drug delivery system (DDS) is a strategy used to improve the target delivery or to control the release of pharmaceutical products in patients (Vega-Vásquez et al., 2020; Jeong et al., 2021a), which has been broadly accepted in the pharmaceutical industry. During the design and development of DDS, two main features are needed: drug targeting and controlled release (Volpi et al., 2021). The first is related to the area where the expected drug arrives, and the second feature is related to two variables: rate and time of drug release. In other words, a DDS is defined by the rate and the time it takes to release the drug in a specific area (Ojediran and Raji, 2010; Jeong et al., 2021b).
Regarding DDS development, the oral or intravenous route has been the most traditional drug delivery administration (Ojediran and Raji, 2010; Briolay et al., 2021), although others, including intranasal administration have also been developed in the last few years (Zhang et al., 2021c).
The main goal of DDS is to reduce the side effects usually caused by treatments and, therefore, allow the use of even more toxic drugs and improve the chemical stability of the drugs. Although cancer has traditionally been the target for DDS, the advent of the new era of oligonucleotide-based drugs is rapidly extending the use of DDS for treating other medical targets. Current cancer treatments are usually not very specific, affecting organs and tissues damage and the healthy others that weaken the patient so that cure can sometimes be as dangerous as the disease. DDS tries to maximize the drugs’ effectiveness by aiming them to a specific area affected by the disease (Xue et al., 2021).
In this context, novel techniques based on chemical conjugations have been carried out to find selective and effective DDS. Recently, a broad range of hybrid covalent entities based on biomolecules and nanoparticles covalently linked to the drug have been developed as efficient drug carriers (Figure 1). Some of them, precisely eleven ADC’s have been commercialized. The present review focuses on those systems designed and prepared through the chemical conjugation of drugs with specific carriers. The fundamental approach to DDS obtained through chemical conjugation using erythrocytes, antibodies, and nanoparticles in the last years are summarized. The main features and their efficiency as therapeutic agents are discussed, focusing on commercialized ADC entities.
2 Drugs Delivery Systems Based on Chemical Conjugation
DDS has been developed to find effective and selective entities to counteract several human diseases and avoid or minimize side effects. Bioentities such as erythrocytes or antibodies have been used as biocarriers. On the other hand, nanoparticles as a conveyor in DDS are also summarized (Figure 1).
2.1 Erythrocytes (Red Blood Cell) as a Carrier in Drug Delivery Systems
Blood is one of the most critical components of the circulatory system. It is responsible for irrigating the entire human body, and erythrocytes, also called red blood cell (RBC), are the most numerous cells present in the blood. RBC transport and exchanges oxygen and carbon dioxide between the lungs and tissues of other organs (Weisel and Litvinov, 2019). Erythrocytes are biocompatible, biodegradable, and nonimmunogenic, making them enjoyable to use in DDS development (Glassman et al., 2020; Zhang X. et al., 2021). The first report of DDS based on erythrocytes described the Daunorubicin linked through a chemical bond to nanoerythrocytes using glutaraldehyde as a homodifunctionalized linking arm. It was observed that the cytotoxicity of the conjugated drug was higher than the free drug when assessed on the P388D1 cancer cell line (Lejeune et al., 1994).
Erythrocytes are being successfully used in some pharmacological therapies due to their potential to transport molecular cargos in three different ways, inside it (embedded), physically adsorb nanocarriers onto its surface (RBC hitchhiking), or chemically linked to drugs (Villa et al., 2017; Alapan et al., 2018; Luo et al., 2021). We highlighted the third way in which chemical conjugation is present.
Ganguly et al. reported erythrocytes and tissue plasminogen activator (tPA or rPA) conjugation through a streptavidin-biotin technique in thromboprophylaxis. Before conjugation between erythrocytes and tPA/rPA, biotinylation of both biomolecules was carried out (Ganguly et al., 2005; Ganguly et al., 2007). Biotinylation is most commonly performed through chemical means, although enzymatic methods are also used. Nowadays, many biotinylation reagents are available, so chemical methods provide greater flexibility in the type of biotinylation needed. All biotinylation reagents have similar features, where the biotin group is present, but a reactive moiety gives biotinylation reagents distinct characteristics that are ideal for different types of experiments (Figure 2A) (Halfon-Domenech et al., 2011; Samavarchi-Tehrani et al., 2020). Because biotin has an extremely high affinity to the protein streptavidin, biotinylated erythrocytes (b-RBC) join streptavidin (SA) noncovalently, and the hybrid obtained entity is then conjugated to biotinylated alteplase (b-tPA) or alteplase (b-rPA) (Figure 2B). The final conjugate made it possible to improve the resistance of tPA to PAI-1 inhibitors, and hence these have a longer intravascular life (Ganguly et al., 2007). The conjugation of urokinase plasminogen activator (scuPA) to RBC as a carrier has also been reported using the same avidin-biotin technique (Murciano et al., 2009). The purpose of the conjugation of both receptors (b-RBC and b-tPA/b-rPA) is to prevent blood coagulation called thromboprophylaxis.
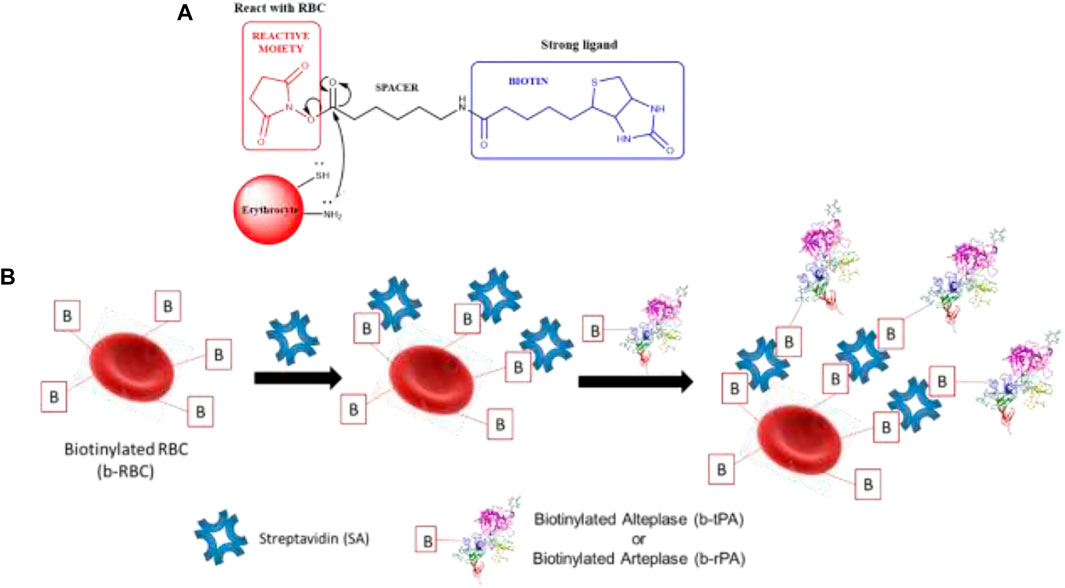
FIGURE 2. (A) Biotinylation reagents general structure. The reagent shown is NHS-LC-biotin, (B) a streptavidin-biotin technique using RBC as a carrier.
In the same way, a multifunctional DDS based on RBCs for magnetic field-enhanced drug delivery and imaging-guided combination therapy of cancer has been described (Wang et al., 2014). Biotin was covalently linked to RBC, and iron oxide nanoparticles (IONPs) coated with chlorine e6 (Ce6), a clinically used photodynamic agent, were attached to the membrane of the RBCs through avidin-biotin binding. Then, doxorubicin (DOX), a known anticancer drug, was loaded inside the RBCs, obtaining a DOX@RBC-IONP-Ce6-PEG structure with a long blood-circulation time and robust responses to the external magnetic field (Wang et al., 2014).
Another example of erythrocyte use in DDS was reported by Lorentz et al. (2015), which described the conjugation of Escherichia coli L-asparaginase-II (ASNase) with some copies of glycophorin A-binding peptide (ERY1). The procedure started with the chemical conjugation between Lys residues of ASNase and peptide ERY1 using succinimidyl 4-(N-maleimidomethyl)cyclohexane-1-carboxylate (SMCC) as a linker (Figure 3A). SMCC is one of the most useful bifunctional linkers used in bioconjugation, allowing the first reaction via amide formation (free amines from biomolecule or cargo and succinimidyl ester from SMCC). Then, the maleimide moiety would react with sulfhydryl groups of another entity (biomolecule or payload). After that, high-affinity binding of ASNase to erythrocytes was achieved through chemical conjugation of several copies of glycophorin A–binding peptide (ERY1). This peptide (ERY1) was conjugated to ASNase through its cysteine residue (Lorentz et al., 2015) (Figure 3B).
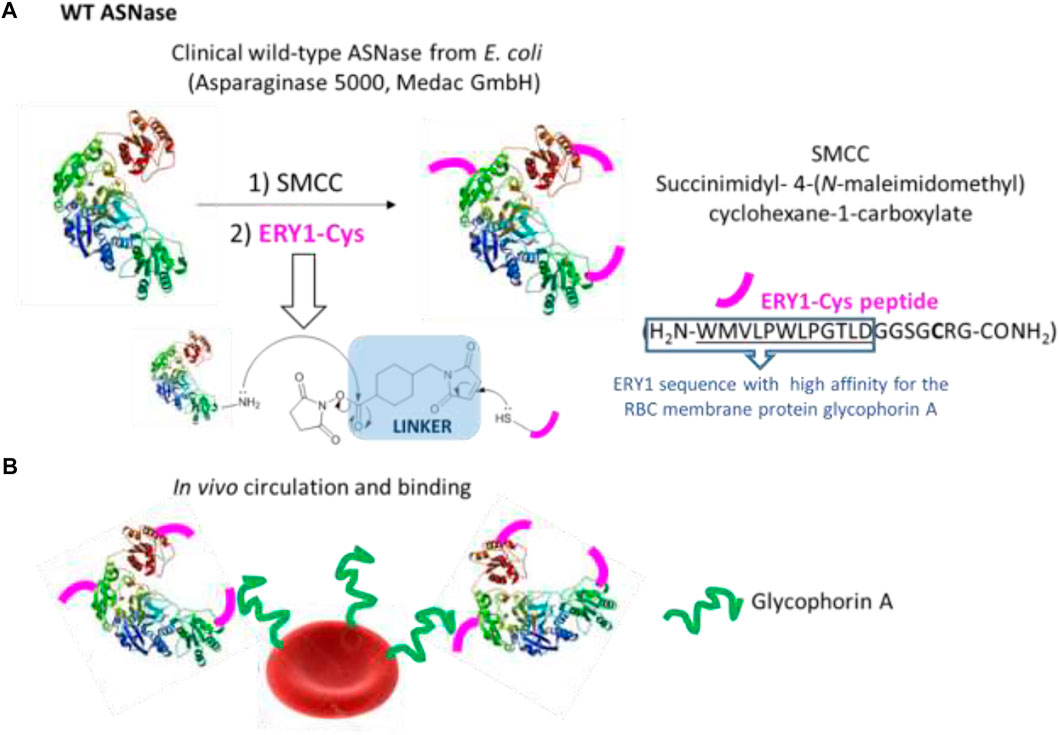
FIGURE 3. Conjugation of Escherichia coli L-asparaginase-II (ASNase) with copies of glycophorin A-binding peptide (ERY1). (A) Chemical conjugation between Lys residues of ASNase and peptide ERY1 using SMCC as a linker, (B) high-affinity binding of ASNase to erythrocytes through chemical conjugation of several copies of glycophorin A–binding peptide (ERY1).
The conjugation described earlier reduces the development of antibodies because it induces antigen-specific tolerance. Erythrocytes conjugated with ASNase have a drug pharmacodynamics effect more significant than ASNase administrated alone. Grimm et al. (2015b) used the same ERY1 peptide chemically conjugated the erythrocyte to ovalbumin through sulfosuccinimidyl-4-(N-maleimidomethyl)cyclohexane-1-carboxylate (Sulfo-SMCC) as a linker. This conjugation improved the response of CD4+ and CD8+ T cells to an antigen because these induced an antigen tolerance (Grimm et al., 2015a; Glassman et al., 2020).
2.1.1 Erythrocytes Based Drug Delivery Systems and the Market
Nowadays, there are no FDA-approved conjugate erythrocytes, but there are studies related to drugs encapsulated in erythrocytes in phases I, II, and III, such as those related to the dexamethasone-21-phosphate in ataxia-telangiectasia, asparaginase in pancreatic cancer, lymphoblastic leukemia, thymidine phosphorylase in mitochondrial neurogastrointestinal encephalomyopathy and RTX-134 in phenylketonuria (Rossi et al., 2020; Javed et al., 2021). In clinical trials, none of the conjugates include covalent bond formation in their preparation. The studies bet on the encapsulation of the drug or the mixture of drugs, using the erythrocytes as a carrier (NCT03267030, 2021; NCT02195180, 2014; Hammel et al., 2020; Rossi et al., 2020).
The DDS based on erythrocytes is complicated to bring to market because it needs to fulfill a pharmaceutical product’s parameters. Among specifications that make complex their market development are: 1) cell material source, which refers to the erythrocytes that come from analogous or homologous donated blood; 2) manufacturing process, which refers to the sterility of the cell suspension and the reduction of leukocytes; 3) product storage, regarding the solution where the erythrocytes are reserved to their prompt administration because they tend to degrade, as well as the problems associated with prolonged release of the cargo, and the potential interactions between cargo and erythrocytes (Bourgeaux et al., 2016; Rossi et al., 2020). Probably, erythrocytes-based DDS will be approved by the Center for Biologics Evaluation and Research (CBER) as the blood and other similar derivatives.
2.2 Nanoparticles as the Carrier in Drug Delivery Systems
Nanoparticles (NPs) are entities with an ultrasmall size; generally, with dimensions less than 200 nm, these dimensions confer different physical, chemical, or/and biological properties compared to a macro or microsample for the same material (Lungu et al., 2015; the United States Environmental Protection Agency, 2021). Nanoparticles could be classified as inorganic and organic NPs, where the first one involves gold and silica NPs, and the second are related to liposomes, micelles, and polymeric NPs (De Matteis and Rinaldi, 2018).
Some valuable features of NPs related to DDS are 1) suitable to be used in intravenous delivery, 2) a good behavior as site-specific drug targeting to treat several diseases, 3) a higher surface to volume ratio as compared with bulk material would provide a diminution in the dose of the drug and therefore a reduction in the toxicity; and 4) a prolonged circulation in the blood among others, which make them suitable for its use as DDS and promising results had been reported (Werengowska-Ciećwierz et al., 2015; Prasher et al., 2020; Cevaal et al., 2021).
Drugs can generally be aggregated on the external or internal nanocarrier surface or linked through chemical bioconjugation. Focused on DDS based on nanoparticles, emphasizing chemical conjugation, different kinds of NPs as polymeric (i.e., PLGA, PLA, PEG, hydrogel, chitosan analog), magnetic, and structures based on carbon have been extensively described (Friedman et al., 2013; Saw et al., 2021; Zielińska et al., 2021).
Chemical conjugation usually takes place on the surface of NPs. Different strategies are used to conjugate NPs with drug-like reactions. Amine-carboxyl, thiol-maleimide, thiol-thiol, hydrazide-aldehyde, and gold-thiol are the most priceless reactions used in the conjugation with NPs, allowed to generate amide, thioether, disulfide, hydrazine, gold-thiol, and triazole ring as covalent linkage, respectively (Friedman et al., 2013; Nikezić et al., 2020). In this regard, the drug or ligand structure determines the type of reaction (Figure 4).
2.2.1 Nanoparticles Based on Liposomes as the Carrier in Drug Delivery Systems
NPs based on liposomes can be considered one of the most hopeful carriers when incorporated into a controlled drug release system (DDS).
Liposomes are spherical vesicles that can be made from a suspension of phospholipids in a hydrophobic medium; this solvent is slowly removed or replaced by another hydrophilic, usually an aqueous solution, using dilution, evaporation, or dialysis processes. This change of solvent causes the phospholipids to self-assemble spontaneously. In this way, they form spherical bilayers and trap a large amount of the aqueous medium in which they are found. They vary in size but are generally smaller than 400 nm. When working with drugs or hydrophilic entities, they are trapped inside the bilayer; and the hydrophobic remains in the phospholipid layer (Figure 5A) (Akbarzadeh et al., 2013; Craciunescu et al., 2021; Kanojia et al., 2022).
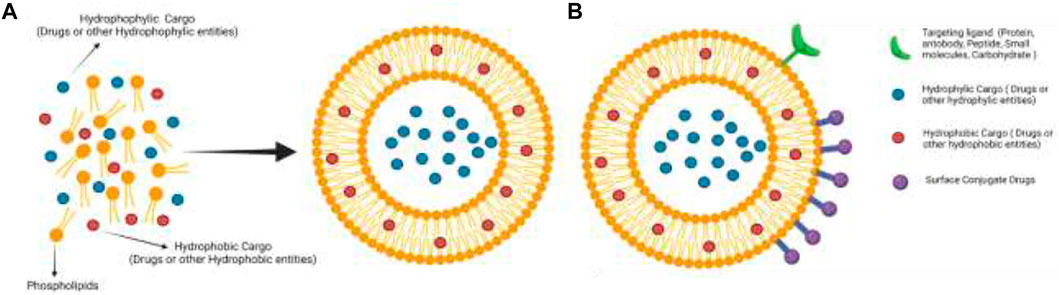
FIGURE 5. (A) General liposome formation. (B) The general structure of DDS is based on functionalized liposomes.
This carrier is one of the most studied to be used in DDS due to liposomes’ positive features. For example, they have a high loading level and a long time in circulation, changing drug pharmacokinetics compared to the drug administered alone. Liposomes as carriers are safe, biodegradable, and biocompatible, making them beneficial to theragnostic (Sonali et al., 2016) (Alwattar et al., 2021) even with imaging purposes when these are conjugated with quantum dots or gold nanoclusters (Liao et al., 2021; Yao et al., 2021).
In general, liposomes can transport drugs in different ways: trapped inside the bilayer (hydrophilic drug), remain in the phospholipid layer for hydrophobic drugs, and covalently link to the liposome surface (Figure 5B). Regarding chemical conjugation, ester and amide bonds have been extensively used in two ways: 1) to join phospholipid to the drug and then carry out the liposome formation; and 2) to decorate the liposome surface.
Liposomes have been used as biocarriers, and their conjugation with microbubbles (Chandan and Banerjee, 2018), some proteins (Sonali et al., 2016; Wang et al., 2021), or antibodies (Burande et al., 2020) allowed to obtain even DDS with anticancer activity (Zhang Y. et al., 2021). Different chemical approaches with different functional groups are used to carry out the conjugation (Aguilar-Pérez et al., 2020), but the more used are ester bonds that are formed between a carboxylic acid group and a hydroxyl group that could have the drug and the lipid, respectively. A linker or spacer such as succinic acid is used to facilitate this conjugation (Irby et al., 2017; Palko-Łabuz et al., 2021). Another helpful linker to lead the conjugation is a succinic anhydride moiety due to being functionalized (Muthu et al., 2015; Spanedda and Bourel-Bonnet, 2021).
Amide bonds are generated between a terminal carboxylic acid of the lipid and an amine group present in the drug (Lanigan et al., 2016; Papadopoulos and Kokotos, 2016; Sharma et al., 2021).
NPs have been conjugated to improve the pharmacokinetic and pharmacodynamic properties of these cargos, overcoming inconveniences like multidrug resistance (Nikezić et al., 2020).
2.2.2 Drug Delivery Systems Based on Nanoparticles as Marketed Drugs
Nowadays, in nanomedicine, PEGylated polymers nanoparticles are the most used and the most available in the market. For instance, Oncaspar® was one of the first nanotherapeutic approved by the FDA in 1994 (U.S. Food and Drug Admins, 1994), formed by L-asparaginase aminohydrolase covalently conjugated to monomethoxypolyethylene glycol (mPEG) through nonspecific random PEGylation of ε-amino groups on the lysine residues of the enzyme (Figure 6), which is used in chemotherapy for the treatment of acute lymphoblastic leukemia (ALL) (European Medicines Agency). Also using PEGylation procedures, Krystexxa® was developed in 2010 (U.S. Food and Drug Admins, 2010). The NPs consisted of a pegloticase and mPEG covalent conjugate and were designed to treat severe debilitating chronic tophaceous gout (European Medicines Agency, 2013). Four years later, Plegridy® was introduced in the pharmaceutical market (U.S. Food and Drug Admins, 2015) to treat relapsing-remitting multiple sclerosis (European Medicines Agency). PEGylation of interferon beta-1a using mPEG and O-2-methylpropionaldehyde linker was reported as its formation reaction of NPs (U.S. Food and Drug Admins, 2015) (European Medicines Agency). The last polymer-drug conjugated approved was ADYNOVATE (Amin, 2008) in 2015, which is a covalently conjugated ADVATE molecule with PEG. This conjugate is used to treat and control hemophilia A (RxList).
Several of these pharmaceutical products with novel technologies attract the research community’s attention. Invega Trinza® (Daghistani and Rey, 2016), with nanocrystal technology based on Paliperidone Palmitate structure, releases paliperidone slowly over a long period for the treatment of schizophrenia and schizoaffective disorder. Sublocade® (buprenorphine extended-release) (Daghistani and Rey, 2016) with the Atrigel technology in situ gel-forming system polymer (biodegradable 50:50 poly(DL-lactide-co-glycolide), and a biocompatible solvent, N-methyl-2-pyrrolidone (NMP) is thought to be a revolutionary product administrated to treat moderate to severe opioid use disorder. The most critical Sublocade® disadvantage is that it can only be administered via subcutaneous. In fact, serious harm or even death would result if this drug is administered intravenously.
DDS based on liposomes approved by the FDA are scarce, and most of them are PEGylated liposomes in which there is no covalent conjugation. For instance, the antibiotic doxorubicin used in chemotherapy was combined with liposome conjugated with PEG (Doxil®) to improve the circulation time of the drug and reduce toxicity (Gabizon, 2001). It was the first DDS based on liposomes approved by the FDA in 1995 (U.S. Food and Drug Admins, 1995). Twelve years later, Liposomal doxorubicin, in combination with another anticancer drug, bortezomib (Lipodox®), was developed for relapsed or refractory multiple myeloma (U.S. Food and Drug Admins, 1995; Ning et al., 2007). Lipodox® has not been approved yet by the FDA, but given the shortage of Doxil, it was accepted for the importation of Lipodox® because it has the same active ingredient, dosage, strength, and route of administration as the aforementioned FDA-approved drug Doxil (U.S. Food and Drug Admins, 2012a).
Additionally, some DDS based on PEGylated liposomes are still in clinical trials, and some good examples are thermosensitive doxorubicin in phase III, which is a promising treatment for liver cancer (Andriyanov et al., 2014; Yang et al., 2019). Liposomal Irinotecan in phase I could be used in advanced refractory solid tumors, advanced esophageal squamous cell carcinoma, and metastatic pancreatic adenocarcinoma (Chang et al., 2015; Bang et al., 2021; Liu et al., 2021).
The nanomedicines Patisiran/ONPATTRO, VYXEOS, and NBTXR3/Hensify have also been approved in the last few years (Anselmo and Mitragotri, 2019). Patisiran/ONPATTRO could be described as RNA-delivering lipid-based nanoparticles, which specifically inhibit hepatic synthesis of transthyretin. It was developed and marketed by Adams et al. (2018). On the other hand, VYXEOS is a liposomal formulation of daunorubicin and cytarabine in a fixed combination for the treatment of adults with newly diagnosed therapy-related acute myeloid leukemia (t-AML) or acute myeloid leukemia (AML) with myelodysplasia-related changes (AML-MRC) (Krauss et al., 2019). Finally, NBTXR3/Hensify is a new class of radio enhancer hafnium oxide nanoparticles sold by Nanobiotix (Bonvalot et al., 2017; Bonvalot et al., 2019).
In the last years, research related to the controlled release of drugs has moved toward the nanoscale. In this sense, several investigations aim to develop robust nanocarriers (nanoliposomes, polymeric, micelles, albumin-bound nanoparticles) with various biomedical, safe, and fast-acting applications (Anselmo and Mitragotri, 2019; Aguilar-Pérez et al., 2020; Tang et al., 2021). Although the most marketed liposome and NPs based DDS does not involve chemical conjugation, the broad range of DDS applying covalent conjugation and included in clinical trials, showed the near future of this field, promoting DDS with good stability, low toxicity, high control of particle size, and modulating the drug release.
2.3 Proteins as a Carrier in Drug Delivery Systems
Proteins are high molecular weight biomolecules commonly used to make DDS. Animal-originated proteins such as silk fibroin, collagen, gelatin, and mainly albumin and antibodies are proteic entities widely used for DDS (Jacob et al., 2018).
2.3.1 Albumin as the Carrier in Drug Delivery Systems
Albumin can be considered an excellent drug delivery platform because of its biochemical and biophysical properties. Anti-cancer drugs bound to albumin often benefit from significant advantages, including longer circulatory half-lives, tumor-targeted delivery, and easier administration relative to the drug alone (Sleep, 2015; Xu et al., 2022; Xu et al., 2022). Based on the albumin binding strategy, covalent conjugations are one of the most used methods of drug control release. Standard methods for direct, covalent conjugation involve binding the drug to either lysines, tyrosines, or the free SH-group on the cysteine-34 amino acid residue of albumin. In this regard, Etelcalcetide, a D-amino acid peptide, undergoes reversible disulfide exchange with serum albumin to form a serum albumin peptide conjugate (SAPC) that is too large (67 kDa) to be dialyzed. This disulfide bond between albumin and peptide allowed for a controlled release of Etelcalcetide, improving its pharmacokinetics (Wu et al., 2018).
Stenzel et al. have developed several procedures to apply the conjugation via the covalent bond of albumins with different cargos and promote the nanocarriers formation (Jiang et al., 2014; Dag et al., 2015; Dag et al., 2015; Taguchi et al., 2018). In this sense, albumin was conjugated via thiol-maleimide with maleimide functionalized poly[oligo(ethylene glycol) methyl ether methacrylate] to generate a polymer–albumin conjugate, which was able to condense positively charged proteins (Taguchi et al., 2018). In addition, poly(2-dimethylaminoethyl methacrylate was conjugated to albumin as a promising gene carrier (Taguchi et al., 2018).
(6-Maleimidocaproyl)hydrazone derivative of doxorubicin (ALDOX) has been conjugated to albumin through an acid hydrolyzable linker, demonstrating in vitro activity comparable to free doxorubicin (DOX) in breast and leukemia cell lines. The acid cleavable linker promotes the putative drug release in the acidic lysosome environment (Taguchi et al., 2018). The pirarubicin (4′-O-tetrahydropyranyldoxorubicin, THP), a semi-synthetic derivative of DOX has also been covalently conjugated to albumin to develop pH-sensitive conjugates. Using a thiol-maleimide coupling reaction, maleimide hydrazone derivatives of THP were conjugated with poly-thiolated albumin using 2-iminothiolane, via a thiol-maleimide coupling reaction (Tsukigawa et al., 2020).
Recently, a synthetic method to access covalently bound Bis-N-heterocyclic carbene (bis-NHC) gold(I) (Au(I) bis-NHC) with serum albumin adducts have been developed without affecting the metal center active site. The procedure can be described as a post-synthetic amide conjugation that relies on the use of the new Au(I) bisNHC complex bearing a carboxylic acid functional group (Sen et al., 2021).
2.3.2 Antibody as a Carrier in Drug Delivery Systems (Antibody-Drug Conjugates)
An antibody is a Y-shaped protein generated by the immune system in response to antigens such as infectious agents or foreign substances. Their main characteristic is its ability to bind to a specific region or epitope of the corresponding antigen, which allows the antibody to be specific and therefore has demonstrated applications in the treatment of some diseases, i.e., cancer (Whalley et al., 2018). In general, monoclonal antibodies (mAb) are generated through the hybridoma technique (Köhler and Milstein, 1992), which uses murine B cells and murine myeloma cells to produce murine mAbs with a unique specificity. The mAbs have been used to develop bioconjugation techniques, using chimeric and humanized antibodies with therapeutic purposes and hence in bioconjugation (Chiu et al., 2021; Ferraro et al., 2021).
Undoubtedly, one of the most successful DDS therapeutic alternatives is the Antibody-Drug conjugates (ADCs) due to the high specificity of these biomolecules and the structural facility (several functional groups available) to load the drug. For this reason, the amount and concentration of the cytotoxic agent used for the treatment are lower than the treatment of naked antibody or drug only (Diamantis and Banerji, 2016; Ferraro et al., 2021; Khattak et al., 2021). Since the objective is to focus cytotoxicity on tumor cells, patients tend to tolerate ADC treatment for more time. Another advantage of ADCs is that it takes more time to develop resistance than their constituent mAb (Thery et al., 2014; Dhritlahre and Saneja, 2021), which increases the therapeutic window for a more aggressive fight against cancer. In general, an ADC is formed by the mAb and the drug. Both entities are joined through crosslinking achieved with various reactive groups such as amines, thiols, alcohols, or even carboxylic acids present in either mAb or drug. Another successful way to achieve bioconjugation is the use of a linker. The linker can be a homo- or hetero-biofunctionalized molecule, whether the reaction occurs through the same functional group or two different kinds. In typical homodifunctionalized crosslinkers, conjugation occurs through the same functional group (e.g., conjugation between two amines or two sulphydryl groups). On the other hand, conjugation occurs through two different functional groups (e.g., conjugation between an amine and a thiol group (Kostova et al., 2021).
2.3.2.1 Linker Properties
As mentioned before, the drugs could be conjugated directly to the antibody. However, the linkers have helped prepare ADCs to facilitate bioconjugation reactions. Among the advantages of using a linker are preventing premature drug release, improving the liberation of the active drug at the target, and facilitating stability in the production process. The most important aspect of the linker is its physicochemical properties, which allows defining two main different kinds of linker:
Cleavable linker: It is characteristic of breaking one of its chemical bonds that binds the two molecular entities. This kind of linker has been successful in elaborating ADCs due to its stability in the synthesis process and the blood circulation of the conjugate for a long time. It allows the releasing of the drug under some conditions at the surrounding of the target cell or tissue-like overexpression of enzyme or acidic environment (Lu et al., 2016; Ramos Tomillero, 2016; Kostova et al., 2021).
Non-cleavable linker: Its principal function is to maintain links between biomolecule and drug. This feature represents a more significant advantage over cleavable linkers because it has more excellent stability in the plasma, thus increasing the specificity. The drug will not be released outside the target cells because the mAb degradation is carried out within the lysosome (McCombs and Owen, 2015; Ramos Tomillero, 2016; Kostova et al., 2021).
2.3.2.2 Chemical Conjugation
Chemical conjugation in the ADC uses covalent bond formation. This delivery system uses a linker between the cytotoxic agent and antibody (Parslow et al., 2016; Kostova et al., 2021). Preferred active sites on antibodies are the amine group of a lateral chain of exposed lysines or the sulfhydryl group of the exposed cysteines. The covalent coupling can be made mainly through two methods: lysine to amide or cysteine to nucleophilic attack.
Lys amide coupling: this method binds payload and Lys residues on the antibody using linkers containing activated carboxylic acid active esters. However, this conjugation can give multiple ADC species different drug-to-antibody ratios (DAR). With a DAR around of 3-4 because a specific antibody has an average of 10 residues of Lys chemically available of around 80 Lys that it would have. Therefore, this way of chemical conjugation generates a heterogeneous mixture with some species challenging to characterize and purify, which could provoke effects on the binding to the antigen and even on the safety profile of the ADC (Parslow et al., 2016; Tsuchikama and An, 2018).
Cys nucleophilic attack: this method binds payload previously modified with the thiol-reactive group and Cys residues of the antibody, generally using maleimide-type linkers (Parslow et al., 2016; Tsuchikama and An, 2018). Cys are less frequent in Abs than Lys, and there are forming disulfide bridges that are key for the structure of the Abs. Thus, the first step in using Cys as a reactive moiety of the Abs is to reduce the disulfide bridges partially. This step is critical because overreduction will lead to the Abs’ denaturalization. For example, in the antibody IgG type (commonly used in ADC), 16 Cys are present: twelve intrachain and four interchain disulfide bonds. These four interchain disulfide bonds are the target for conjugation because these are not important for structural stability (Zakharov et al., 2000), allowing limited conjugation sites (2-8 free thiols) due to the reactivity of the thiol group. There is still the possibility to end up with a heterogeneous mixture of ADC, although to a less extent than Lys conjugation. Cys coupling has been mostly used in clinical trials because it has a higher DAR (approximately between 0 and 8) than Lys amide coupling. The conjugates obtained with this technique are easier to characterize (McCombs and Owen, 2015; Tsuchikama and An, 2018).
Regarding cancer disease, the significant advantage of these systems is the delivery of the drug, which could have high toxicity, directly into the tumor (Goss et al., 2018; Dhritlahre and Saneja, 2021; Ferraro et al., 2021). The possibility to combine the favorable binding properties of mAbs with the activity of potent cytotoxic agents promises to increase the therapeutic index of therapeutic payloads.
Borek et al. (2018) reported a chemical conjugation of scFvF7-Fc antibody fragment through its cysteine residues to cytotoxic agent monomethyl auristatin E (MMAE) using a valine-citrulline linker and a maleimidocaproyl spacer (Doronina et al., 2003). In this case, enzymatic and selective cleave occurs in the bond between the peptide with the termini valine-citrulline and the corresponding drug (Figure 7A). This ADC can be internalized into cancer cells due to its composition, i.e., the antibody and linker. This study was carried out in search of an alternative for treating gastrointestinal cancer, with a high incidence of mortality and relatively short survival rates.
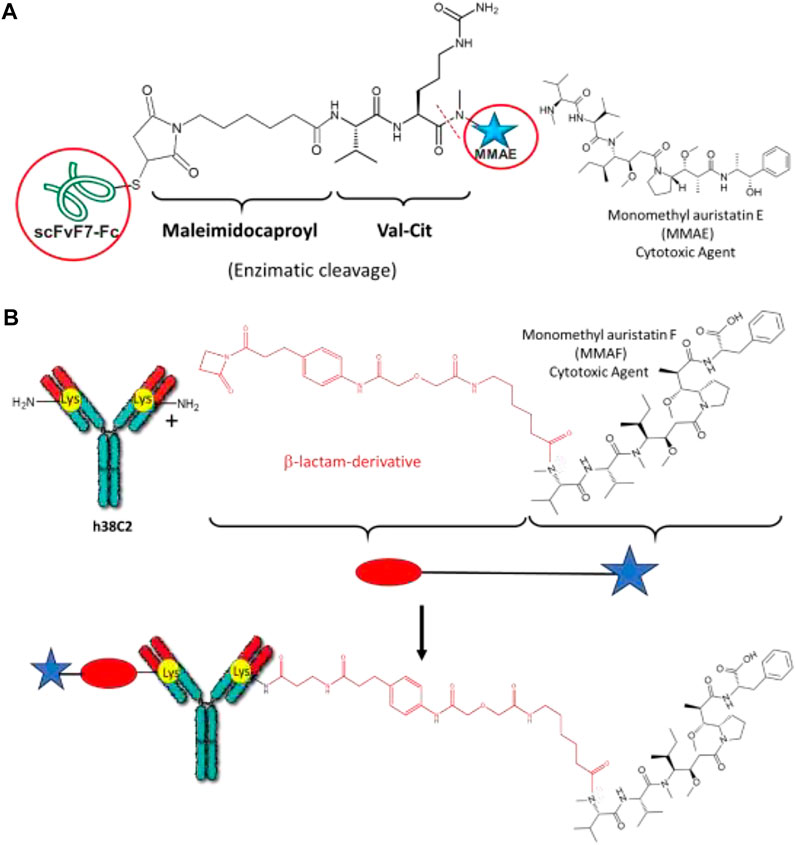
FIGURE 7. (A) Structures of the drug (MMAE) and mAb-drug conjugate. (B) Conjugation of the humanized anti-hapten monoclonal antibody (mAb) h38C2 through nucleophilic lysine with β-lactam derivatives (i.e., β-lactam functionalized monomethyl auristatin F (MMAF).
Recently, biophysical characterization of a DAR 8 ADC fully conjugated at all interchain Cys residues was reported to keep the structure and stability of the fully conjugated as an optimized and clinically relevant second-generation monomethyl auristatin-E (MMAE) drug-linker (Chiu et al., 2021).
On the other hand, numerous studies using Lys residues of ADCs have been carried out. In general, Lys-mediated chemical bioconjugation allows to obtain heterogeneous conjugates mixtures with 0–8 DAR, and consequently, each of them has different toxicity, pharmacokinetics, and efficacy. Although both Cys and Lys-assisted conjugation is still used, some alternative strategies have also been implemented to generate more homogeneous ADCs. For example, Nanna et al. (2017) reported the conjugation of the humanized antihapten monoclonal antibody (mAb) h38C2 through its most exceptionally nucleophilic Lys present at an 11-Å deep hydrophobic pocket in their structure. The mentioned Lys is capable of catalyzing aldol and retroaldol reactions. Thus, it can be selectively conjugated with β-lactam derivatives (i.e., β-lactam functionalized monomethyl auristatin F (MMAF)), generating a noncleavable and stable amide bond until its enzymatic degradation inside the target cells takes place (Figure 7B). The described conjugate could avoid the premature release of the cytotoxic agent. The other achievement of this study is generated homogeneous ADC’s (Nanna et al., 2017).
Valine-citrulline dipeptide has been used as a linker for ADCs such as DLYE5953A, a humanized IgG1 monoclonal antibody that targets the lymphocyte antigen 6 complex (LY6E) conjugated to Monomethylauristatin (MMAE) under the auspices of Genentech and showed antitumor activity in patients with solid metastatic tumors (Tolaney et al., 2021).
In the last years, various reports related to multiloading linkers have proposed a novel methodology incorporating two payload molecules into single monoclonal antibodies (mAbs). The main idea is to generate bi or tri-functionalized linkers, in which orthogonal reactions allow the incorporation of different payloads using covalent bonds (Levengood et al., 2017; Kumar et al., 2018; Zhang L. et al., 2021; Yamazaki et al., 2021). Levengood et al. (2017) prepared a linker with sequential Cys residues with orthogonal protection to enable site-specific conjugation of each drug and conjugated to IgG1 through the reduced interchain disulfides of the antibody and stable maleimide linkage. In 2018, a novel heterotrifunctional linker was developed by Kumar et al. (2018), providing a flexible platform that allows the coupling of two different drugs via copper-catalyzed azide-alkyne cycloaddition (CuAAC) and via oxime linkage using ketone as starting functional group, but also the conjugation to the antibody through cysteine—maleimide reaction. Recently, a one-pot, successive reaction method to produce dual payload conjugates with the site-specifically engineered cysteine and p-acetyl-phenylalanine using Herceptin (trastuzumab), an anti-HER2 antibodydrug widely used for breast cancer treatment, as a tool molecule as another approach to the specific conjugation of two different drugs, improving not only the potential to overcome drug resistance but enhance the therapeutic efficacy (Zhang L. et al., 2021).
2.3.2.3 Drug Delivery Systems Based on Antibody-Drug Conjugates as Marketed Drugs
At present, eleven ADC’s involving chemical conjugation hold market authorization for therapy of certain types of cancers (Figure 8) (do Pazo et al., 2021). Adcetris® (Brentuximab Vedotin, 2013; U.S. Food and Drug Administration (FDA), 2012b) is an antineoplastic agent used in the treatment of Hodgkin lymphoma and systemic anaplastic large cell lymphoma. B.V. is obtained through the covalent conjugation of the mouse-human chimeric monoclonal antibody IgG1 antiCD30 (cAC 10), with monomethyl auristatin E (MMAE). This ADC uses a maleimidocaproyl-valine-citruline-p-aminobenzyloxycarbonyl linker to promote the proteolytic cleavage by Cathepsin B. Through cysteine-maleimide reaction, the corresponding mAb is joined to the potent anti-mitotic agent (monomethyl auristatin E [MMAE]) (Figure 8A). This toxin is a synthetic antitubulin analog, using an enzymatically cleavable dipeptide linker. On average, each antibody molecule was conjugated into four groups of MMAE.
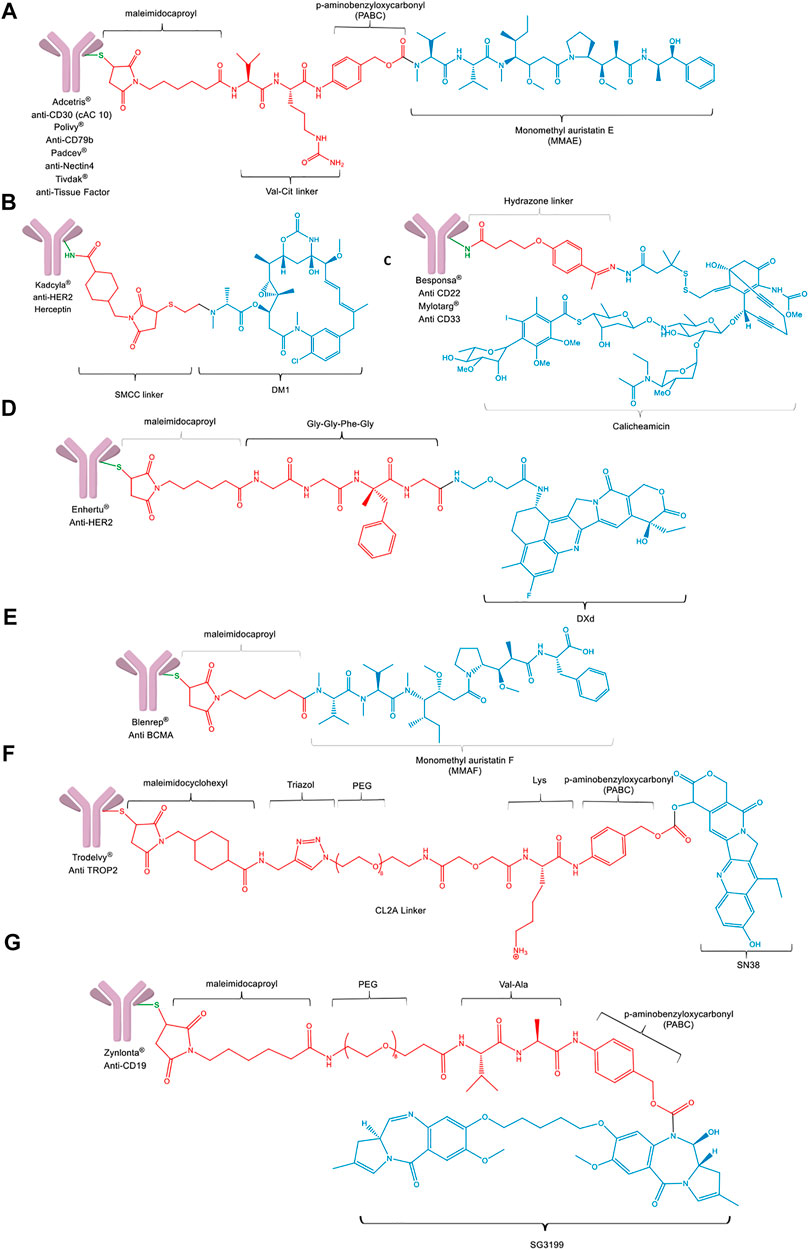
FIGURE 8. General structures of ADC’ are approved by the FDA. (A) Adcetris®, Polivy®, Padcev®, and Tivdak®. (B) Kadcyla®. (C) Besponsa® and Mylotarg®. (D) Enhertu®. (E) Blenrep®. (F) Trodelvy®. (G) Zynlonta®. All linkers are highlighted in red.
On the other hand, Kadcyla® (Trastuzumab emtansine) (U.S. Food and Drug Administration (FDA), 2013) is an innovative, unique, and selective antineoplastic drug used in patients with advanced breast cancer (HER2+). This ADC is composed of the antiHER2 antibody trastuzumab (Herceptin®) and the cytotoxic microtubules agent, DM1, bound through a bifunctional succinimidyl 4-(N-maleimidomethyl)cyclohexane-1-carboxylate (SMCC) as a non-cleavable linker (Figure 8B). This drug acts selectively on HER2-positive tumors cells, exercising, on the one hand, the mechanisms of action of the trastuzumab, and on the other hand, the powerful cytotoxic effect of DM1. The main advantage of this ADC is that it has a selective release, allowing it to minimize its side effects compared to other agents for the same pathology (Baron et al., 2015; U.S. Food and Drug Administration (FDA), 2013).
Besponsa® (Inotuzumab ozogamicina) (U.S. Food and Drug Adminstration (FDA), 2017a) is used in adults with acute lymphoblastic leukemia (ALL) whose disease has stopped responding to conventional chemotherapy (recurrence), or never responded to it (refractory). The carrier of Inotuzumab ozogamicina is a monoclonal antibody that attacks the CD22 protein, which is produced in excess on the surface of lymphoblastic leukemia cells. The antibody binds to a calicheamicin compound that kills cancer cells using a pH-sensitive hydrazone linker (Figure 8C). Once the inotuzumab antibody binds to CD22 in the cancer cells, the calicheamicin is released into the cell, where it damages cellular DNA and causes its death (Hamann et al., 2002; U.S. Food and Drug Adminstration (FDA), 2017a).
Mylotarg® (Gemtuzumab ozogamicin) (U.S. Food and Drug Administration (FDA), 2017b) is used for the treatment of acute myeloid leukemia (AML), a bone marrow cancer. Conjugation is similar to Besponsa; however, the different antibody is used. In this case, Inotuzumab ozogamicin consists of a recombinant humanized IgG4 kappa CD22-targeting monoclonal antibody covalently attached to calicheamicin derivative, which is a potent DNA-binding cytotoxic agent (Figure 8C) (Hamann et al., 2002; U.S. Food and Drug Administration (FDA), 2017b). Mylotarg was removed from the market in 2010 due to the high incidence of death in the patients, but it was restituted in 2017 combined with induction chemotherapy.
In 2019, three ADCs were approved by the FDA, Enhertu®, Padcev®, and Polivy®. The first mentioned conjugate is indicated for the treatment of breast cancer that is metastatic (that has spread to other parts of the body), or that cannot be removed by surgery when cancer overexpresses HER2. The humanized mAb Herceptin (antiHER2) is covalently linked to the topoisomerase I inhibitor deruxtecanan (DXd) using maleimidocaproyl and Gly-Gly-Phe-Gly tetrapeptide as a spacer and protease cleavable linker between the mAb and the cargo (Figure 8D) (Brentuximab Vedotin (SGN35) Drug Description). Padcev® and Polivy® are similar to Adcetris® (Figure 8A) (Process, 2019). Padcev (enfortumab vedotin-ejfv) is an ADC with a synthetic analog of the marine natural peptide dolastatin to treat refractory bladder cancer. In contrast, Polivy® (polatuzumab vedotin-piiq) is indicated to treat adult patients with relapsed or refractory diffuse large B-cell lymphoma.
In 2020, Blenrep® from GlaxoSmithKline and Trodelvy® from Gilead Sciences, Inc. was the ADCs approved by the FDA to treat multiple myeloma and Metastatic triple-negative breast cancer, respectively. Blenrep® includes an antiCD38 monoclonal antibody, a proteasome inhibitor, and an immunomodulatory agent joined by a noncleavable maleimidocaproyl linker (Figure 8E), while Trodelvy® is composed of a humanized monoclonal antibody, hRS7 IgG1κ (sacituzumab), the drug SN-38, a topoisomerase inhibitor maleimidocyclohexyl, and a hydrolyzable linker (called CL2A), which links the humanized monoclonal antibody to SN-38 (Figure 8F) (U.S. Food and Drug Administration (FDA), 2020). The synthetic platform for Blenrep® is similar to those previously described for PADCEV® and Polivy®. Nevertheless, the linker of Trodelvy® involves a triazole moiety, PEG units as spacer, and lysine in its structure chemically synthesized (The FDA-Approved Sacituzumab Govitecan for Triple-Negative Breast Cancer | Biopharma PEG).
Last year, Zynlonta® and Tivdak® were incorporated into the list of conjugates FDA approved (Zynlonta FDA. Initial U.S. Approval: 2021, 2021; Tivdak FDA. Initial U.S. Approval: 2021, 2021). Zynlonta® comprises a humanized mAb Anti CD19 conjugated to SG3199, a cytotoxic pyrrolobenzodiazepine (PBD) dimer alkylating agent, through a protease-cleavable valinealanine linker, and using maleimido caproyl and eight PEG units (Figure 8G). This ADC is used for adult patients with relapsed or refractory large B-cell lymphoma after two or more lines of systemic therapy, including diffuse large B-cell lymphoma (DLBCL), not otherwise specified DLBCL arising from low-grade lymphoma, and high-grade B-cell lymphoma (Caimi et al., 2021). Tivdak® shares the synthetic platform as Adcetris®, Polivy®, and Padcev® (Figure 8A) but uses an antitissue factor as mAb, becoming the first approved ADC indicated for the treatment of adult patients with recurrent or metastatic cervical cancer with disease progression on or after chemotherapy (De Goeij et al., 2015; Coleman et al., 2021).
From the analysis of the recent literature (Tong et al., 2021), it is evident that, referring to controlled drug release systems involving chemical-assisted conjugation, the most successful to date have been the ADCs. Despite the broad range of chemical strategies developed to improve the payload and the ADC’s effectiveness, the most extended is those involving cysteine mediate maleimide conjugation with different linkers highlighting the valine—citrulline, which allows the protease cleavage.
2.4 Hybrid Conjugates as Carriers in Drug Delivery Systems
DDS generally uses only one biomolecule or nanoparticle as a carrier, which is sometimes ineffective. Scientists are conducting various experiments where a combination of biomolecules or biomolecules with nanoparticles has been made to find novel hybrid systems for therapeutic use in front of a particular disease, especially cancer. Considering that this disease has various types, it is impossible to have “general” DDS to treat it.
For example, in search of a conjugation brain delivery system able to cross the blood-brain barrier (BBB), Huang et al. (2013) studied lactoferrin (Lf) protein-like active principle conjugated to PEGylated liposomes (PL). The hybrid conjugate was prepared through the thiolization of Lf using Traut’s reagent, allowing to have a sulfhydryl group at the N-terminus. At the same time, PL was functionalized with maleimide to promote a subsequent thioether formation between the previously thiolated Lf and maleimide PL derivatives. Pharmacokinetics and pharmacodynamics properties of the conjugate Lf-PL were compared with PL, taking into account that PEGylation is helpful to improve the properties mentioned earlier (Milton Harris et al., 2001). The results showed a greater uptake of the conjugate system, mediated by the Lf receptor present on the surface of the glioblastomas (Arcella et al., 2015).
The research of Lin et al. (2017) presents an attractive DDS base on liposomes and antibodies. It was achieved by generating a half-antibody with free thiol groups available for the subsequent thioether formation. Selected maleimide-pegylated liposomes loaded with Triptolide (TPL), which has a cytotoxic effect of reducing tumor growth, were conjugated with the mAb and tested in lung tumor cells. Considering that carbonic anhydrase enzyme IX is present on the lung tumor cell’s surface, carbonic antianhydrase antibody IX conjugated to the surface of a liposome was used to target tumors in the lungs to improve the selectivity and precision in this type of DDS. Lung cancer is one of the most afflicted in the world population, and it has lower rates of long-term survival (World Health Organization, 2018).
2.4.1 Ligation/Bioconjugation Techniques Based on Multicomponent Reactions
Multicomponent reactions were also a powerful tool to generate covalent bonding, improving the conjugation between a broad range of biomolecules and cargos.
2.4.2 Lipid-Lipid and Lipid-Oligosaccharide Conjugation
A procedure based on MCR allows to conjugate two lipid tails to an oligosaccharide moiety by Ugi multicomponent reaction (U-MCR). Two variants were achieved in which a carbohydrate (oligosaccharides) is used as either the carboxylic acid or amino component. The oligosaccharides were functionalized with fatty acid and lipidic isocyanides, yielding glycolipids. Thus, this approach can multi-functionalize biomolecules in one spot synthesis, opening possibilities for accessing DDS analogs of anti-cancer glycolipids (Figure 9A) (Pérez-Labrada et al., 2012; Liu et al., 2019).
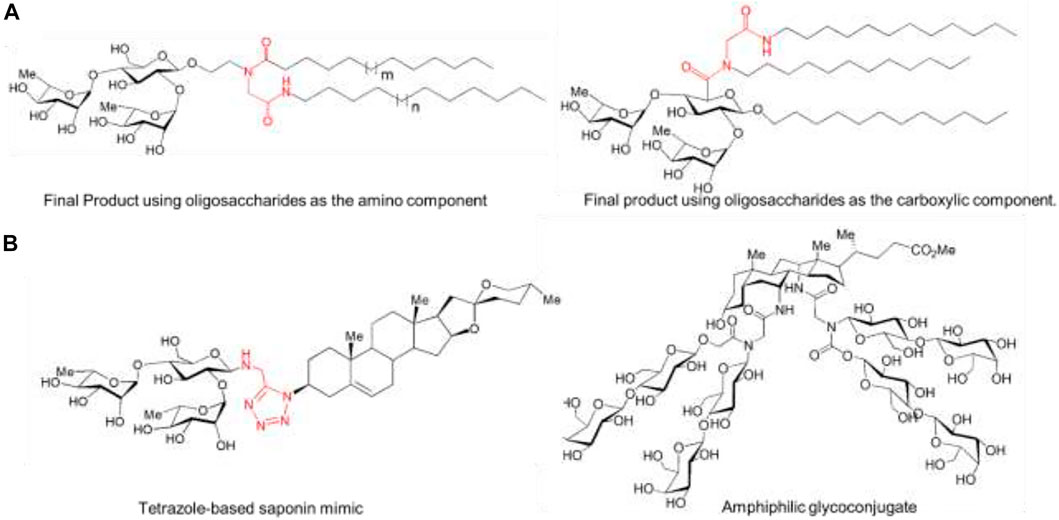
FIGURE 9. (A) Lipid-Lipid and lipid-oligosaccharide conjugates. (B) Oligosaccharide-steroid conjugates: Steroid-carbohydrate conjugates by multicomponent conjugation (MCC) of oligosaccharide to steroidal isocyanides. The most important covalent bond between entities is highlighted in red.
2.4.3 Oligosaccharide-Steroid Conjugation
The synthesis of steroid-carbohydrate derivatives by I-MCRs was carried out with spirostanic steroids and β-chacotrioside functionalized as either the amino or carboxylic acid component producing a library of cytotoxic analogs of diosgenyl-β-chacotrioside (tetrazole-based saponin mimic) for anti-cancer activity (Rivera et al., 2012) (Figure 9B). Even, to obtain more complex conjugation, it is ligated two oligosaccharide moieties to a bifunctional steroid, conjugation of 4 lactosyl fragments and acholic acid-derived di-isocyanide by means of a double Ugi reaction, rendering steroidal glycoconjugate (amphiphilic glycoconjugate), which the lactose units are located at the α face of concave cholanic skeleton (Rivera et al., 2013) (Figure 9B).
2.4.4 Peptide-Peptide and Carbohydrate-Peptide Ligation
The ligation of oligopeptides fragments by I-MCRs can be used as an approach for the Ugi multicomponent ligation (UML) of two pseudo peptides fragments to yield more stable and highly active peptides analogs using a highly diastereoselective UML procedure (Arabanian et al., 2009; Znabet et al., 2010; Pando et al., 2011). Moreover, the Ugi peptide ligation can be expanded by the development of the highly stereoselective organocatalytic MCR method for peptide-peptide or sugar-peptide conjugation, which is based on the use of enantiomerically enriched enol-hemiacetals as chiral inputs to conjugate isocyanopeptides to carbohydrates and amino peptides to form peptidic hybrids (Echemendía et al., 2015) (Figure 10A). Furthermore, the MCL of urea-peptide, a lipidic aldehyde, and a hybrid ribosyl-uridine isocyanide can produce a potent lipophilic antibacterial muraymicin analog (Figure 10B) (Tanino et al., 2010).
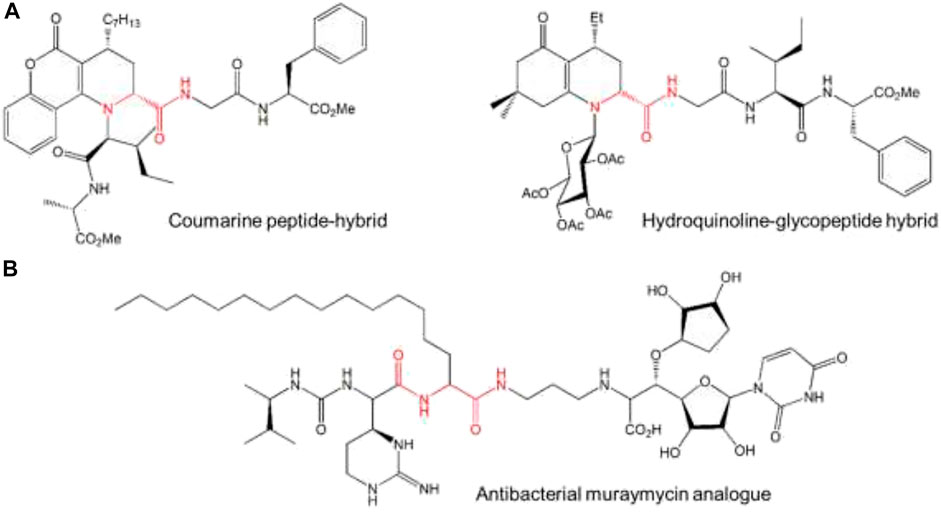
FIGURE 10. Peptide-peptide and carbohydrate peptide conjugation. (A) The final product of diastereoselective multicomponent ligation (MCL) of peptide and sugar residues to chiral bifunctional building blocks, (B) final product of MLC of urea-peptide, a lipid, and aminoribosyl-5-C-glycyludrine. The most important covalent bond between entities is highlighted in red.
2.4.5 Peptide-Steroids and Peptide-Lipid Conjugation
The synthesis of peptide-steroid conjugates can be achieved through multicomponent conjugation (MCC) of individual amino acids (A.A.s) (Rivera et al., 2006; Singla and Salunke, 2020). This concept can be expanded to creating a first-in-class family of peptide steroid conjugates (Unique class of N-steroidal peptide), highlighting the peptide backbone with the steroid skeleton as an N-substituent through an MCC of peptide carboxylic acid and isocyanopeptide or isocyanoacetate to a steroidal amine. These N-steroidal peptides can be further cyclized to yield cyclopeptide-steroid conjugate, in which the cyclopeptide residue can be found in different positions of either steroidal or the side chain (Figure 11) (Rivera et al., 2006; Singla and Salunke, 2020).
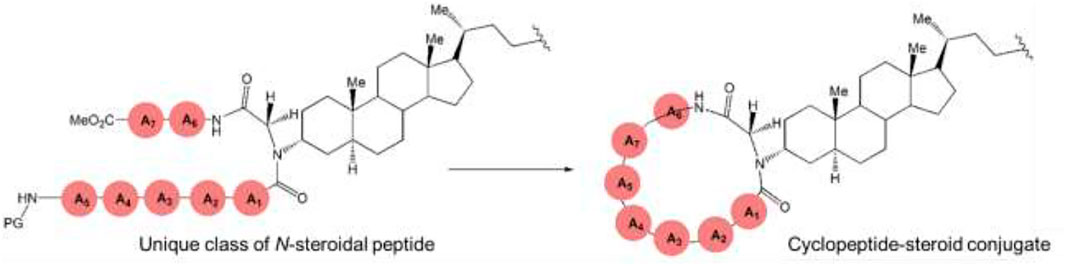
FIGURE 11. Peptide-steroid conjugation. Multicomponent conjugation (MCC) of two peptide fragments previously linked to the steroid to construct a unique N-steroidal cyclopeptide.
Moreover, the synthesis of antimicrobial lipopeptides can locate the lipidic tail either in the peptide termini as an internal amide or side-chain substituent (Morales et al., 2015; Wessjohann et al., 2016). The lipopeptides were created using a novel on-resin MCC of lipids and steroids to peptides based on solid-phase methodology, which enables the conjugation of either a lipid chain or a steroidal skeleton to the resin-bound peptide (RBP) (Figure 12A). Whereas subsequent A.A.s couplings allow the growth of the peptide, obtaining the desired lipopeptides and peptide-steroid conjugate. Similarly, a double ligation of an RBP at the N-terminus was carried out, allowing the N-terminal double lipidation and N-terminal lipidation and biotinylation (Figure 12B). Also, through a Ugi-azide reaction, it is obtained tetrazole lipopeptides and tetrazole peptidosteroid (Figure 12C) (Morales et al., 2015).
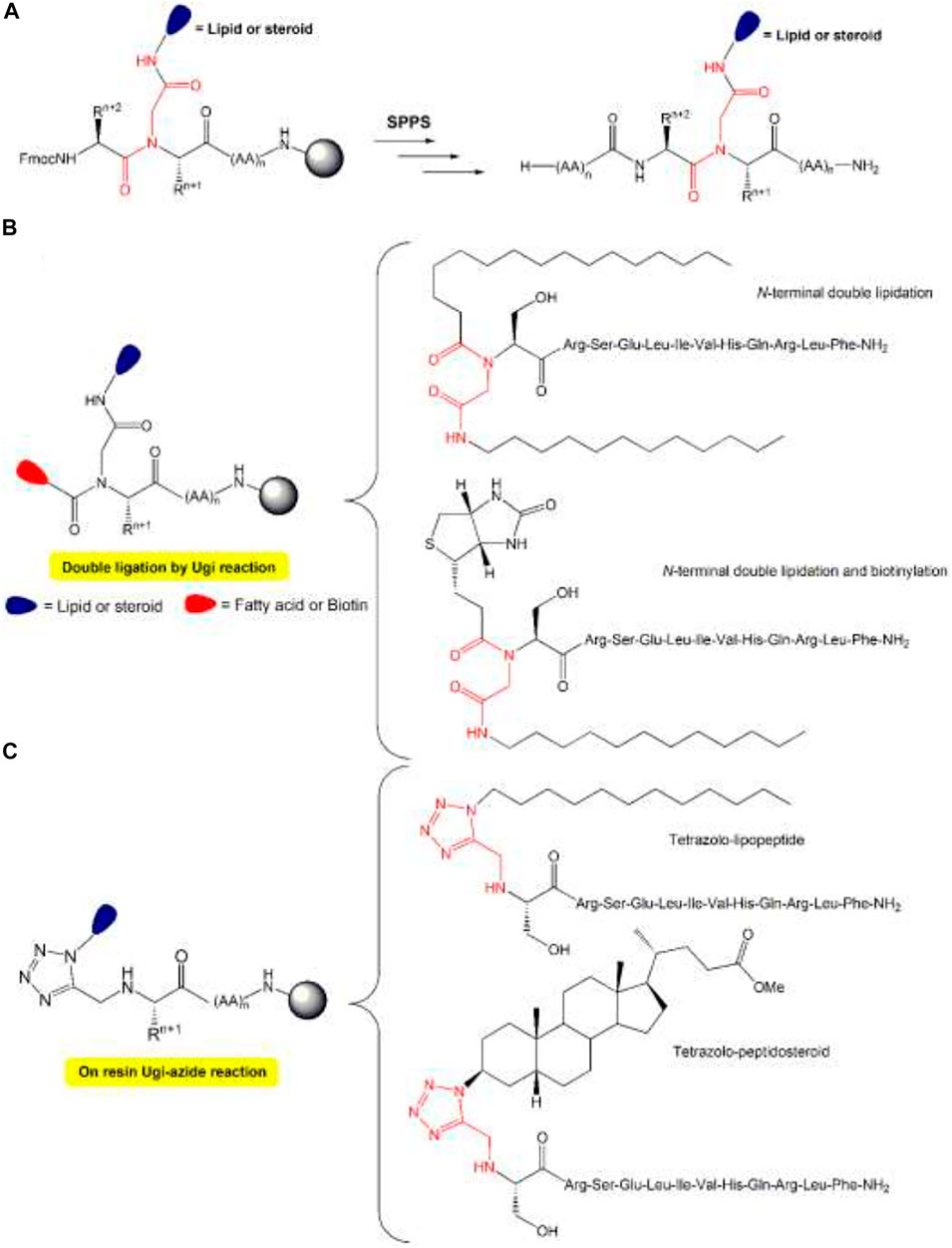
FIGURE 12. Peptide-steroid and peptide-lipid conjugates. (A) Solid phase ligation (SPL) of lipids and steroids by resin-bound peptide (RBP) using on-resin Ugi reaction, (B) double ligation by Ugi reaction, (C) SPL of lipids, biotinylated steroids by RBP using on-resin Ugi azide reaction. The most important covalent bond between entities is highlighted in red.
Furthermore, it can produce microbial natural products analogs (cyclic lipopeptides analogs) efficiently by Ugi and Passerini reactions to obtain a simultaneous cyclization and peptide lipidation. It let conjugate either one or two exocyclic lipid tails and the macrocyclic ring closure (Figure 13A) (Morejón et al., 2017). Likewise, the same procedure was proved by an on-resin Ugi-Smiles reaction creating a multicomponent cyclo-ligation strategy that enables the simultaneous cyclization of peptide skeleton and its ligation to either lipidic moieties or fluorescent labels (El Kaïm et al., 2005; Morejón et al., 2016) (Figure 13B).
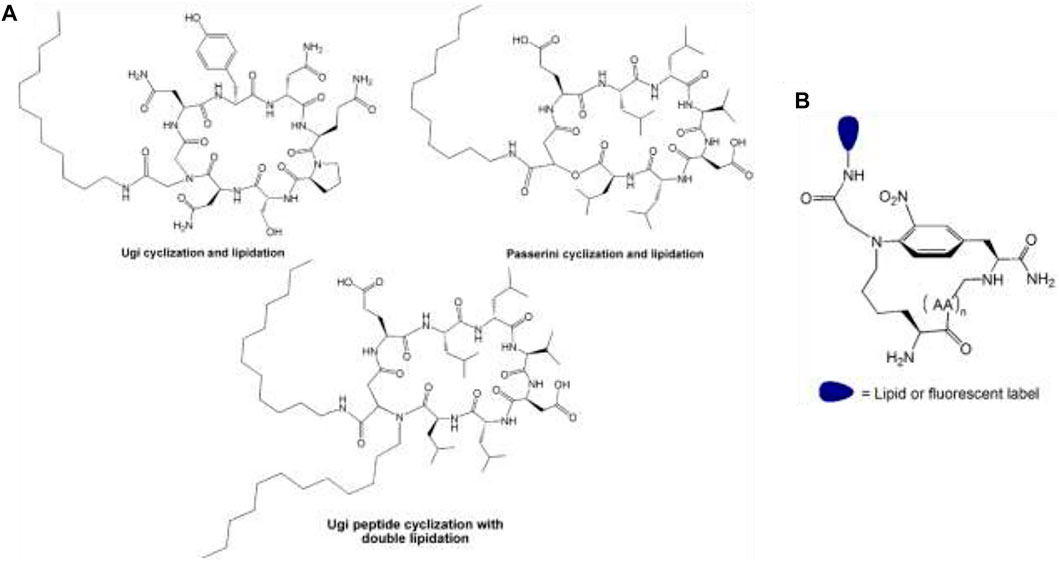
FIGURE 13. (A) Peptide-steroid and peptide-lipid conjugates. Products of simultaneous cyclization and lipidation of peptides by Ugi and Passerini reactions. (B) The pattern of the product was obtained using resin Ugi-smiles macrocyclization and ligation.
Related to lipopeptides, a monocyte-targeting delivery platform based on cationic liposomes is based on functionalizing the liposomal membrane with a cholesterol-anchored tri-arginine peptide (TriArg) has been developed, guiding the design of future DDS utilized for immunotherapy (Münter et al., 2022). In the same way, a class of dendritic lipopeptide (DLP) liposomal delivery platform, consisting of a guanidinium-based dendritic peptide moiety, has been developed. The DDS is pH-sensitive and charge-reversible functions to enhance tumor accumulation and cell penetration (Münter et al., 2022).
2.4.6 Protein Immobilization, Labeling, and Glycoconjugate
Protein immobilization can be achieved through efficient Ugi reaction procedures, immobilizing the enzyme glucose oxidase on a polymeric carrier. The glycoenzime undergoes periodate oxidation to obtain the aldehyde group in the glycosidic part. Then it is linked to “amino-functionalized glycidyl methacrylate polymer using an excess of acetic acid and cyclohexyl isocyanide” to produce the polymer-supported enzyme (Figure 14A) (Marek et al., 1983). Also, it was prepared bovine serum albumin (BSA) and horseradish peroxidase (HRP) conjugates by Ugi reaction using either the carboxylic acid or amino groups at the biomolecules surface, monosaccharides, and isocyano components to render several Ugi-derived glycoconjugates (Figure 14B). Although, this procedure has a problem due to the long reaction times that cause the protein denaturation as a result of protein crosslinking which can be solved by incorporating in Ugi reaction to increase protein reactivity of amino group and decrease reaction time (Ziegler et al., 2000; Méndez et al., 2018). Moreover, polysaccharide conjugation can be obtained by I-MCRs (Passerinin and Ugi reaction) to produce “biocompatible synthetic hydrogels” to immobilize enzymes in polysaccharide networks, which serve as carriers in DDS (De Nooy et al., 1999). In addition, an amperometric enzyme biosensor was developed for hydrogen peroxide immobilization of HRP on a gold electrode coated with sodium alginate by Ugi reaction (Figure 14C). This method consists of functionalizing polysaccharides with thiol groups by periodate oxidation and subsequent reductive amination. Once the polymer is attached to a gold electrode, it achieved electrode-immobilized enzyme using the Ugi bioconjugation procedure (Camacho et al., 2007). Similarly, the Ugi bioconjugation strategy is suitable for preparing thermostable neoglyconenzymes conjugating trypsin with sodium alginate and carboxymethyl cellulose yielding trypsin-polysaccharide glycoconjugates (García et al., 2009). In addition, protein labeling can be obtained through a Mannich-type multicomponent process conjugation at Tyr residues which consists of the mild reaction between the Tyr phenol ring and imines derived from aldehydes and electron-rich anilines carrying rhodamine tag to yield labeled proteins (Figure 14D) (Joshi et al., 2004) Another procedure of site-selective protein labeling based on MCRs is using Cu-catalyzed A3 couplings (Figure 14E) (Chilamari et al., 2017).
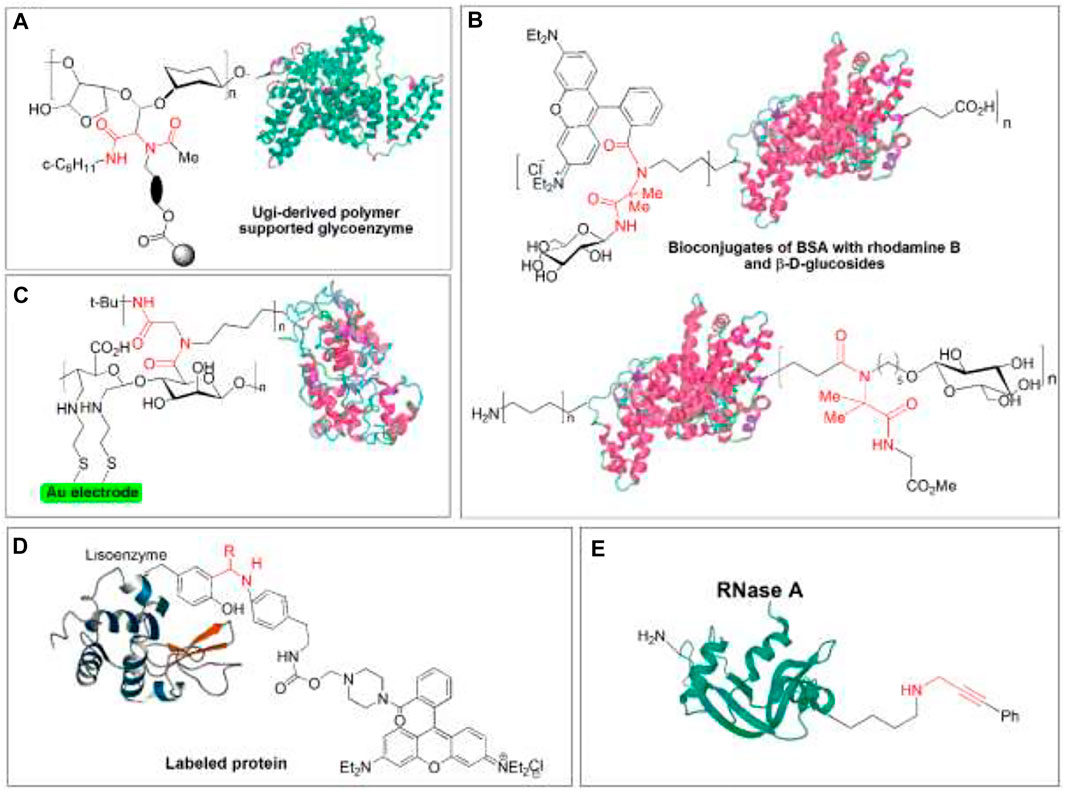
FIGURE 14. Protein immobilization, labeling, and glycoconjugate (A) Ugi-derived polymer-supported glycoenzyme; (B) final products from multicomponent conjugation (MCC) to polymeric support (see Panel (A)) and fluorescent label (rhodamine B) and carbohydrate (β-D-glucosides) using Ugi reaction; (C) multicomponent immobilization (MCI) of horseradish peroxidase (HRP) on a polysaccharide-coated gold electrode; (D) site-selective protein labeling at Tyr residue by Mannich type MCC; (E). Site-selective protein labeling at only one Lys residue by Cu-catalyzed A3 coupling MCC. The most important covalent bond between entities is highlighted in red.
Finally, the development of the multicomponent protein-polysaccharide conjugation method, which consists of UML of functionalized capsular polysaccharide (CP) of Streptococcus and Salmonella to carrier proteins like diphtheria and tetanus toxoids (DT and TT), is activated by reaction of glutamic and aspartic acid side chains with hydrazine (DTa and TTa). The products of this procedure showed significant antigenicity and elicited goods titer of functional specific antibodies (Figures 15A, B). Similarly, two different polysaccharides were conjugated to a protein using TEMPO-oxidized CPs14 (carboxylic component) and periodate oxidized CPs7F (oxo component) TTa (amino component), yielding a glycoconjugate with two different polysaccharide antigens conjugated to a carrier protein, which has dual polysaccharide antigenicity. This proved that this multicomponent bioconjugation method is efficient for developing multivalent vaccine candidates (Méndez et al., 2018) (Figure 15C).
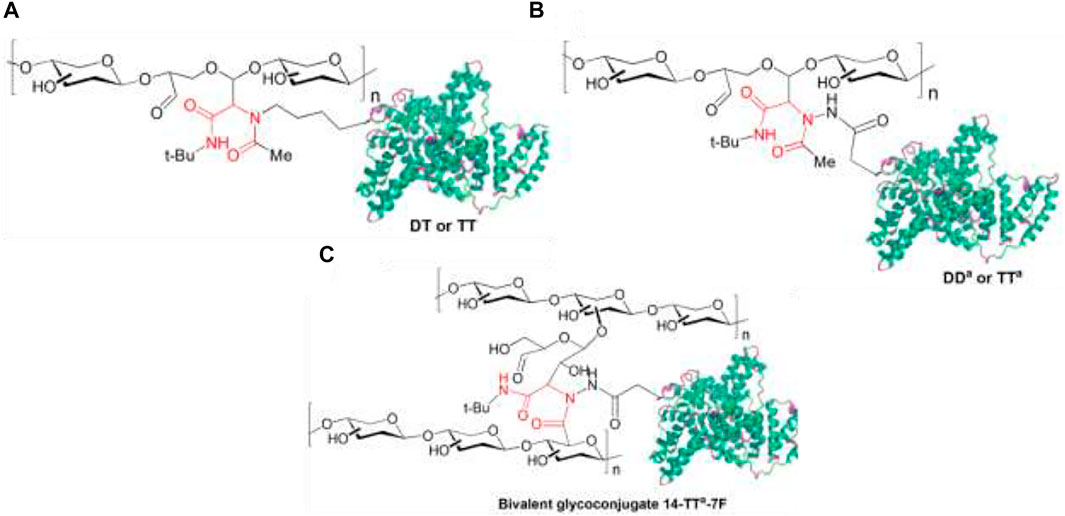
FIGURE 15. Final products obtained from protein immobilization, labeling, and glycoconjugate through (A) multicomponent conjugation (MCC) of oxo-functionalized capsular polysaccharide (CP) to nonactivated diphtheria (DT) and tetanus toxoids (TT); (B) hydrazide-activated DT and TT (DDa or TTa), and (C) MCC of two CPs to hydrazide-activated TT for the obtention of multivalent glycoconjugate vaccines. The most important covalent bond between entities is highlighted in red.
Polysaccharide-peptide and polysaccharide-protein conjugates are essential for targeted drug delivery into cells and tissue, and comprehensive reviews on its properties and applications as DDS have been published (Torres-Pérez et al., 2020; Walther and Zelikin, 2021; Kurbangalieva et al., 2022; Mohan et al., 2022).
3 Conclusion
DDS is an emergent field in Medicinal Chemistry. DDS based on Erythrocytes, Liposomes, Nanoparticles, and mainly mAb has been successfully developed, yielding entities able to avoid or minimize drug side effects or circumvent the resistance of the disease to certain drugs. Many of these conjugates are already on the market, and many others are already in clinical trials. Therefore, the search for more efficient DDS is continuously under development.
DDS has emerged as the potential solution to well-known drawbacks of drug administration, focusing on diseases like cancer and maximizing the effectiveness of the administered drugs by aiming them at the specific area affected by the disease. In this regard, erythrocytes, liposomes, nanoparticles, and antibodies have been covalently linked to a broad range of cargos, i.e., drugs, markers, and biomolecules, with promising bioapplications. Amide or disulfide formation was the chemical way most used to generate hybrid conjugates. On the other hand, multicomponent reactions (MCR) have been incorporated as a powerful tool in preparing conjugates, taking advantage of its proven efficiency and allowing the combination between lipids, oligosaccharides, steroids, and peptides. Despite the rapid development of DDS, nowadays, only a few DDSs based on liposomes nanoparticles or antibodies are successfully commercialized, which is expected considering the times associated with this kind of process (i.e., design, optimization, clinical trials, and final drug registration). The great variety of chemical reactions that can be adapted to different carriers gives this topic great importance that will be reflected in an increase in related research and the number of conjugates in the market and those that will be approved by the FDA shortly.
After much time, appreciable technological advances, a better understanding of controlled drug release systems, and resources invested in the research and development of these systems, there are tangible results. The approval of several ADCs focused on cancer therapy and extensive research focused on the discovery of novel systems that use erythrocytes, liposomes, nanoparticles, or proteins, indicated a promising future. We believe the true potential of using covalent conjugation applied in DDS as a pharmacological platform is only just being realized, understood, and exploited.
Author Contributions
AE and DC: Investigation and visualization. MS: Conceptualization, investigation, and reviewing. FA, NV, and HR: Conceptualization, methodology, supervision, writing-reviewing, and editing.
Funding
This work was funding by the Yachay Tech Internal Project (REG-INV-18-03244) “New linkers for bioconjugations and its therapeutics uses.”
Conflict of Interest
The authors declare that the research was conducted in the absence of any commercial or financial relationships that could be construed as a potential conflict of interest.
Publisher’s Note
All claims expressed in this article are solely those of the authors and do not necessarily represent those of their affiliated organizations, or those of the publisher, the editors, and the reviewers. Any product that may be evaluated in this article, or claim that may be made by its manufacturer, is not guaranteed or endorsed by the publisher.
Acknowledgments
The authors AE and DC are grateful for the support to the undergraduate research thesis, which is part of this document, by Yachay Tech. FA, HR, and NV appreciate the support through the Yachay Tech Internal Project “New linkers for bioconjugations and its therapeutics uses”.
References
Adams, D., Gonzalez-Duarte, A., O’Riordan, W. D., Yang, C.-C., Ueda, M., and Kristen, A. V. (2018). Patisiran, an RNAi Therapeutic, for Hereditary Transthyretin Amyloidosis. N. Engl. J. Med. 379, 11–21. doi:10.1056/nejmoa1716153
Aguilar-Pérez, K. M., Avilés-Castrillo, J. I., Medina, D. I., Parra-Saldivar, R., and Iqbal, H. M. N. (2020). Insight into Nanoliposomes as Smart Nanocarriers for Greening the Twenty-First Century Biomedical Settings. Front. Bioeng. Biotechnol. 8, 1441. doi:10.3389/fbioe.2020.579536
Akbarzadeh, A., Rezaei-sadabady, R., Davaran, S., Joo, S. W., and Zarghami, N. (2013). Liposome : Classification , Preparation , and Applications. Nanoscale Res. Lett. 1, 9. doi:10.1186/1556-276X-8-102
Alapan, Y., Yasa, O., Schauer, O., Giltinan, J., Tabak, A. F., Sourjik, V., et al. (2018). Soft Erythrocyte-Based Bacterial Microswimmers for Cargo Delivery. Sci. Robot. 3, eaar4423. doi:10.1126/scirobotics.aar4423
Alwattar, J. K., Mneimneh, A. T., Abla, K. K., Mehanna, M. M., and Allam, A. N. (2021). Smart Stimuli-Responsive Liposomal Nanohybrid Systems: A Critical Review of Theranostic Behavior in Cancer. Pharmaceutics 13, 355. doi:10.3390/pharmaceutics13030355
Amin, Y. (2008). Highlights of Prescribing Information. Of, H., Of, H., Information, P., and Information, P. White Oak, Maryland: Lulu.com. Available at: www.fda.gov/medwatch (Accessed February 24, 2022).
Andriyanov, A. V., Koren, E., Barenholz, Y., and Goldberg, S. N. (2014). Therapeutic Efficacy of Combining PEGylated Liposomal Doxorubicin and Radiofrequency (RF) Ablation: Comparison between Slow-Drug-Releasing, Non-thermosensitive and Fast-Drug-Releasing, Thermosensitive Nano-Liposomes. PLoS One 9. doi:10.1371/journal.pone.0092555
Anselmo, A. C., and Mitragotri, S. (2019). Nanoparticles in the Clinic: An Update. Bioeng. Transl. Med. 4. doi:10.1002/btm2.10143
Arabanian, A., Mohammadnejad, M., Balalaie, S., and Gross, J. H. (2009). Synthesis of Novel Gn-RH Analogues Using Ugi-4MCR. Bioorg. Med. Chem. Lett. doi:10.1016/j.bmcl.2008.11.111
Arcella, A., Oliva, M. A., Staffieri, S., Aalberti, S., Grillea, G., Madonna, M., et al. (2015). In Vitro and In Vivo Effect of Human Lactoferrin on Glioblastoma Growth. J. Neurosurg. 123, 1026–1035. doi:10.3171/2014.12.JNS14512
Bang, K., Cheon, J., Jeong, J. H., Im, H. S., Kim, K. P., Ryoo, B. Y., et al. (2021). Clinical Outcomes of Liposomal Irinotecan Plus Fluorouracil/leucovorin for Metastatic Pancreatic Adenocarcinoma in Patients Previously Treated with Conventional Irinotecan-Containing Chemotherapy. Ther. Adv. Med. Oncol. 13. doi:10.1177/17588359211003053
Baron, J. M., Boster, B. L., and Barnett, C. M. (2015). Ado-Trastuzumab Emtansine (T-DM1): A Novel Antibody-Drug Conjugate for the Treatment of HER2-Positive Metastatic Breast Cancer J. Oncol. Pharm. Pract. 21, 132–142. doi:10.1177/1078155214527144
Bonvalot, S., Le Pechoux, C., De Baere, T., Kantor, G., Buy, X., Stoeckle, E., et al. (2017). First-in-human Study Testing a New Radioenhancer Using Nanoparticles (NBTXR3) Activated by Radiation Therapy in Patients with Locally Advanced Soft Tissue Sarcomas. Clin. Cancer Res. 23, 908–917. doi:10.1158/1078-0432.CCR-16-1297
Bonvalot, S., Rutkowski, P. L., Thariat, J., Carrère, S., Ducassou, A., Sunyach, M. P., et al. (2019). NBTXR3, a First-In-Class Radioenhancer Hafnium Oxide Nanoparticle, Plus Radiotherapy versus Radiotherapy Alone in Patients with Locally Advanced Soft-Tissue Sarcoma (Act.In.Sarc): a Multicentre, Phase 2–3, Randomised, Controlled Trial. Lancet Oncol. 20, 1148–1159. doi:10.1016/S1470-2045(19)30326-2
Borek, A., Sokolowska-Wedzina, A., Chodaczek, G., and Otlewski, J. (2018). Generation of High-Affinity, Internalizing Anti-fgfr2 Single-Chain Variable Antibody Fragment Fused with Fc for Targeting Gastrointestinal Cancers. PLoS One 13, 1–16. doi:10.1371/journal.pone.0192194
Bourgeaux, V., Lanao, J., Bax, B., and Yann, G. (2016). Drug-loaded Erythrocytes: On the Road toward Marketing Approval. Drug Des. Devel. Ther. 10, 665–676. doi:10.2147/DDDT.S96470.LK
Brentuximab Vedotin (SGN35) (2013). Drug Description. J. Antib. Drug Conjug. Available at: https://www.adcreview.com/brentuximab-vedotin-sgn35/.
Briolay, T., Petithomme, T., Fouet, M., Nguyen-Pham, N., Blanquart, C., and Boisgerault, N. (2021). Delivery of Cancer Therapies by Synthetic and Bio-Inspired Nanovectors. Mol. Cancer, 1–24. 2021 201 20. doi:10.1186/S12943-021-01346-2
Burande, A. S., Viswanadh, M. K., Jha, A., Mehata, A. K., Shaik, A., Agrawal, N., et al. (2020). EGFR Targeted Paclitaxel and Piperine Co-loaded Liposomes for the Treatment of Triple Negative Breast Cancer. AAPS PharmSciTech 21, 1–12. doi:10.1208/s12249-020-01671-7
Caimi, P. F., Ai, W., Alderuccio, J. P., Ardeshna, K. M., Hamadani, M., Hess, B., et al. (2021). Loncastuximab Tesirine in Relapsed or Refractory Diffuse Large B-Cell Lymphoma (LOTIS-2): a Multicentre, Open-Label, Single-Arm, Phase 2 Trial. Lancet Oncol. 22, 790–800. doi:10.1016/S1470-2045(21)00139-X
Camacho, C., Matías, J. C., García, D., Simpsom, B. K., and Villalonga, R. (2007). Amperometric Enzyme Biosensor for Hydrogen Peroxide via Ugi Multicomponent Reaction. Electrochem. Commun. 9, 1655–1660. doi:10.1016/j.elecom.2007.03.013
Cevaal, P. M., Ali, A., Czuba-Wojnilowicz, E., Symons, J., Lewin, S. R., Cortez-Jugo, C., et al. (2021). In Vivo T Cell-Targeting Nanoparticle Drug Delivery Systems: Considerations for Rational Design. ACS Nano 15, 3736–3753. doi:10.1021/acsnano.0c09514
Chandan, R., and Banerjee, R. (2018). Pro-apoptotic Liposomes-Nanobubble Conjugate Synergistic with Paclitaxel: A Platform for Ultrasound Responsive Image-Guided Drug Delivery. Sci. Rep. 8, 1–15. doi:10.1038/s41598-018-21084-8
Chang, T. C., Shiah, H. S., Yang, C. H., Yeh, K. H., Cheng, A. L., Shen, B. N., et al. (2015). Phase I Study of Nanoliposomal Irinotecan (PEP02) in Advanced Solid Tumor Patients. Cancer Chemother. Pharmacol. 75, 579–586. doi:10.1007/s00280-014-2671-x
Chilamari, M., Purushottam, L., and Rai, V. (2017). Site-Selective Labeling of Native Proteins by a Multicomponent Approach. Chem. - A. Eur. J. doi:10.1002/chem.201605938
Chiu, D., Pan, L., Fay, L., Eakin, C., and Valliere-Douglass, J. (2021). Structural Characterization of a Monomethylauristatin-E Based ADC that Contains 8 Drugs Conjugated at Interchain Cysteine Residues. J. Pharm. Biomed. Anal. 205, 114309. doi:10.1016/j.jpba.2021.114309
Coleman, R. L., Lorusso, D., Gennigens, C., González-Martín, A., Randall, L., Cibula, D., et al. (2021). Efficacy and Safety of Tisotumab Vedotin in Previously Treated Recurrent or Metastatic Cervical Cancer (innovaTV 204/GOG-3023/engot-Cx6): a Multicentre, Open-Label, Single-Arm, Phase 2 Study. Lancet Oncol. 22, 609–619. doi:10.1016/S1470-2045(21)00056-5
Craciunescu, O., Icriverzi, M., Florian, P. E., Roseanu, A., and Trif, M. (2021). Mechanisms and Pharmaceutical Action of Lipid Nanoformulation of Natural Bioactive Compounds as Efficient Delivery Systems in the Therapy of Osteoarthritis. Pharmaceutics 13, 1108. doi:10.3390/pharmaceutics13081108
Dag, A., Jiang, Y., Karim, K. J. A., Hart-Smith, G., Scarano, W., and Stenzel, M. H. (2015). Polymer-albumin Conjugate for the Facilitated Delivery of Macromolecular Platinum Drugs. Macromol. Rapid Commun. 36, 890–897. doi:10.1002/marc.201400576
Daghistani, N., and Rey, J. A. (2016). Invega Trinza: The First Four-Times-A-Year, Long-Acting Injectable Antipsychotic Agent. Pharm. Ther. 41, 222.
De Goeij, B. E. C. G., Satijn, D., Freitag, C. M., Wubbolts, R., Bleeker, W. K., Khasanov, A., et al. (2015). High Turnover of Tissue Factor Enables Efficient Intracellular Delivery of Antibody-Drug Conjugates. Mol. Cancer Ther. 14, 1130–1140. doi:10.1158/1535-7163.MCT-14-0798
De Matteis, V., and Rinaldi, R. (2018). “Toxicity Assessment in the Nanoparticle Era,” in Advances in Experimental Medicine and Biology (Cham: Springer), 1–19. doi:10.1007/978-3-319-72041-8_1
De Nooy, A. E. J., Masci, G., and Crescenzi, V. (1999). Versatile Synthesis of Polysaccharide Hydrogels Using the Passerini and Ugi Multicomponent Condensations. Macromolecules 32 (4), 1318–1320. doi:10.1021/ma9815455
Dhritlahre, R. K., and Saneja, A. (2021). Recent Advances in HER2-Targeted Delivery for Cancer Therapy. Drug DiscovToday 26, 1319–1329. doi:10.1016/j.drudis.2020.12.014
Diamantis, N., and Banerji, U. (2016). Antibody-drug Conjugates - an Emerging Class of Cancer Treatment. Br. J. Cancer 114, 362–367. doi:10.1038/bjc.2015.435
do Pazo, C., Nawaz, K., and Webster, R. M. (2021). The Oncology Market for Antibody-Drug Conjugates. Nat. Rev. Drug Discov. 20, 583–584. doi:10.1038/d41573-021-00054-2
Doronina, S. O., Toki, B. E., Torgov, M. Y., Mendelsohn, B. A., Cerveny, C. G., Chace, D. F., et al. (2003). Development of Potent Monoclonal Antibody Auristatin Conjugates for Cancer Therapy. Nat. Biotechnol. 21, 778–784. doi:10.1038/nbt832
Echemendía, R., De La Torre, A. F., Monteiro, J. L., Pila, M., Corrêa, A. G., Westermann, B., et al. (2015). Highly Stereoselective Synthesis of Natural-product-like Hybrids by an Organocatalytic/multicomponent Reaction Sequence. Angew. Chem. Int. Ed. 54 (26), 7621–7625. doi:10.1002/anie.201412074
El Kaïm, L., Grimaud, L., and Oble, J. (2005). Phenol Ugi-Smiles Systems: Strategies for the Multicomponent N-Arylation of Primary Amines with Isocyanides, Aldehydes, and Phenols. Angew. Chem. - Int. Ed. 44 (48), 7961–7964. doi:10.1002/anie.200502636
European Medicines Agency (2013). Krystexxa European Medicines Agency. Available at: https://www.ema.europa.eu/en/medicines/human/EPAR/krystexxa (Accessed May 2, 2022).
Ferraro, E., Drago, J. Z., and Modi, S. (2021). Implementing Antibody-Drug Conjugates (ADCs) in HER2-Positive Breast Cancer: State of the Art and Future Directions. Breast Cancer Res. 23, 1–11. doi:10.1186/s13058-021-01459-y
Friedman, A., Claypool, S., and Liu, R. (2013). The Smart Targeting of Nanoparticles. Curr. Pharm. Des. 19, 6315–6329. doi:10.2174/13816128113199990375
Gabizon, A. A. (2001). Pegylated Liposomal Doxorubicin: Metamorphosis of an Old Drug into a New Form of Chemotherapy. Cancer Invest. 19, 424–436. doi:10.1081/cnv-100103136
Ganguly, K., Krasik, T., Medinilla, S., Bdeir, K., Cines, D. B., Muzykantov, V. R., et al. (2005). Blood Clearance and Activity of Erythrocyte-Coupled Fibrinolytics. J. Pharmacol. Exp. Ther. 312, 1106–1113. doi:10.1124/jpet.104.075770.Wooster
Ganguly, K., Murciano, J., Westrick, R., Leferovich, J., Cines, D. B., and Muzykantov, V. R. (2007). The Glycocalyx Protects Erythrocyte-Bound Tissue-type Plasminogen Activator from Enzymatic Inhibition. J. Pharmacol. Exp. Ther. 321, 158–164. doi:10.1124/jpet.106.114405.plasma
García, A., Hernández, K., Chico, B., García, D., Villalonga, M. L., and Villalonga, R. (2009). Preparation of Thermostable Trypsin-Polysaccharide Neoglycoenzymes through Ugi Multicomponent Reaction. J. Mol. Catal. B Enzym. doi:10.1016/j.molcatb.2009.02.001
Glassman, P. M., Villa, C. H., Ukidve, A., Zhao, Z., Smith, P., Mitragotri, S., et al. (20202020). Vascular Drug Delivery Using Carrier Red Blood Cells: Focus on RBC Surface Loading and Pharmacokinetics. Pharm 12 (440 12), 440. doi:10.3390/PHARMACEUTICS12050440
Goss, G. D., Vokes, E. E., Gordon, M. S., Gandhi, L., Papadopoulos, K. P., Rasco, D. W., et al. (2018). Efficacy and Safety Results of Depatuxizumab Mafodotin (ABT-414) in Patients with Advanced Solid Tumors Likely to Overexpress Epidermal Growth Factor Receptor. Cancer 124, 2174–2183. doi:10.1002/cncr.31304
Grimm, A. J., Kontos, S., Diaceri, G., Quaglia-Thermes, X., and Hubbell, J. A. (2015b). Memory of Tolerance and Induction of Regulatory T Cells by Erythrocyte-Targeted Antigens. Sci. Rep. 5, 1–11. doi:10.1038/srep15907
Grimm, A. J., Stephan Kontos Giacomo Diaceri, X. Q.-T., and Hubbell, J. A. (2015a). Memory of Tolerance and Induction of Regulatory T Cells by Erythrocyte-Targeted Antigens. Sci. Rep. 5, 1–11. doi:10.1038/srep15907
Halfon-Domenech, C., Thomas, X., Chabaud, S., Baruchel, A., Gueyffier, F., Mazingue, F., et al. (2011). l-Asparaginase Loaded Red Blood Cells in Refractory or Relapsing Acute Lymphoblastic Leukaemia in Children and Adults: Results of the GRASPALL 2005-01 Randomized Trial. Br. J. Haematol. 153, 58–65. doi:10.1111/j.1365-2141.2011.08588.x
Hamann, P. R., Hinman, L. M., Hollander, I., Beyer, C. F., Lindh, D., Holcomb, R., et al. (2002). Gemtuzumab Ozogamicin, a Potent and Selective Anti-CD33 Antibody - Calicheamicin Conjugate for Treatment of Acute Myeloid Leukemia. Bioconjug. Chem. 13, 47–58. doi:10.1021/bc010021y
Hammel, P., Fabienne, P., Mineur, L., Metges, J. P., Andre, T., De La Fouchardiere, C., et al. (2020). Erythrocyte-encapsulated Asparaginase (Eryaspase) Combined with Chemotherapy in Second-Line Treatment of Advanced Pancreatic Cancer: An Open-Label, Randomized Phase IIb Trial. Eur. J. Cancer 124, 91–101. doi:10.1016/j.ejca.2019.10.020
Huang, F. Y. J., Chen, W. J., Lee, W. Y., Lo, S. T., Lee, T. W., and Lo, J. M. (2013). In Vitro and In Vivo Evaluation of Lactoferrin-Conjugated Liposomes as a Novel Carrier to Improve the Brain Delivery. Int. J. Mol. Sci. 14, 2862–2874. doi:10.3390/ijms14022862
Irby, D., Du, C., and Li, F. (2017). Lipid-Drug Conjugate for Enhancing Drug Delivery. Mol. Pharm. 14, 1325–1338. doi:10.1021/acs.molpharmaceut.6b01027
Jacob, J., Haponiuk, J. T., Thomas, S., and Gopi, S. (2018). Biopolymer Based Nanomaterials in Drug Delivery Systems: A Review. Mater. Today Chem. 9, 43–55. doi:10.1016/j.mtchem.2018.05.002
Javed, S., Alshehri, S., Shoaib, A., Ahsan, W., Sultan, M. H., Alqahtani, S. S., et al. (20212021). Chronicles of Nanoerythrosomes: An Erythrocyte-Based Biomimetic Smart Drug Delivery System as a Therapeutic and Diagnostic Tool in Cancer Therapy. Pharm 13, 368. 368 13. doi:10.3390/PHARMACEUTICS13030368
Jeong, W. Y., Kwon, M., Choi, H. E., and Kim, K. S. (2021a). Recent Advances in Transdermal Drug Delivery Systems: a Review. Biomater. Res. 25, 1–15. doi:10.1186/s40824-021-00226-6
Jeong, W. Y., Kwon, M., Choi, H. E., and Kim, K. S. (2021b2021). Recent Advances in Transdermal Drug Delivery Systems: a Review. Biomater. Res. 251 25, 1–15. doi:10.1186/S40824-021-00226-6
Jiang, Y., Liang, M., Svejkar, D., Hart-Smith, G., Lu, H., Scarano, W., et al. (2014). Albumin-micelles via a One-Pot Technology Platform for the Delivery of Drugs. Chem. Commun. 50, 6394–6397. doi:10.1039/c4cc00616j
Joshi, N. S., Whitaker, L. R., and Francis, M. B. (2004). A Three-Component Mannich-type Reaction for Selective Tyrosine Bioconjugation. J. Am. Chem. Soc. 126, 15942–15943. doi:10.1021/ja0439017
Kanojia, N., Sharma, N., Gupta, N., and Singh, S. (2022). Applications of Nanostructured Lipid Carriers: Recent Advancements and Patent Review. Biointerface Res. Appl. Chem. 12, 638–652. doi:10.33263/BRIAC121.638652
Khattak, Z. E., Hashmi, H., Khan, S. I., Aamir, S., Arif, U., Khan, A. I., et al. (2021). Dawn of a new era of Antibody-Drug Conjugates and Bispecific T-Cell Engagers for Treatment of Multiple Myeloma: a Systematic Review of Literature. Ann. Hematol. 100, 2155–2172. doi:10.1007/s00277-021-04599-5
Köhler, G., and Milstein, C. (19921975). Continuous Cultures of Fused Cells Secreting Antibody of Predefined Specificity. Biotechnology 24, 524–526. doi:10.1038/256495a0
Kostova, V., Désos, P., Starck, J. B., and Kotschy, A. (2021). The Chemistry behind Adcs. Pharmaceuticals 14, 442. doi:10.3390/ph14050442
Krauss, A. C., Gao, X., Li, L., Manning, M. L., Patel, P., Fu, W., et al. (2019). FDA Approval Summary: (Daunorubicin and Cytarabine) Liposome for Injection for the Treatment of Adults with High-Risk Acute Myeloid Leukemia. Clin. Cancer Res. 25, 2685–2690. doi:10.1158/1078-0432.CCR-18-2990
Kumar, A., Kinneer, K., Masterson, L., Ezeadi, E., Howard, P., Wu, H., et al. (2018). Synthesis of a Heterotrifunctional Linker for the Site-specific Preparation of Antibody-Drug Conjugates with Two Distinct Warheads. Bioorg. Med. Chem. Lett. 28, 3617–3621. doi:10.1016/j.bmcl.2018.10.043
Kurbangalieva, A., Zamalieva, R., Nasibullin, I., Yamada, K., and Tanaka, K. (2022). Homo- and Heterogeneous Glycoconjugates on the Basis of N-Glycans and Human Serum Albumin: Synthesis and Biological Evaluation. Mol 202227, 1285. Page 1285 27. doi:10.3390/MOLECULES27041285
Lanigan, R. M., Karaluka, V., Sabatini, M. T., Starkov, P., Badland, M., Boulton, L., et al. (2016). Direct Amidation of Unprotected Amino Acids Using B(OCH 2 CF 3 ) 3. Chem. Commun. 52, 8846–8849. doi:10.1039/C6CC05147B
Lejeune, A., Moorjani, M., Gicquaud, C., Lacroix, J., Poyet, P., and Gaudreault, C. R. (1994). Nanoerythrosome, a New Derivative of Erythrocyte Ghost: Preparation and Antineoplastic Potential as Drug Carrier for Daunorubicin. Anticancer Res. 14, 915–919. Available at: https://pubmed.ncbi.nlm.nih.gov/8074493/.
Levengood, M. R., Zhang, X., Hunter, J. H., Emmerton, K. K., Miyamoto, J. B., Lewis, T. S., et al. (2017). Orthogonal Cysteine Protection Enables Homogeneous Multi-Drug Antibody–Drug Conjugates. Angew. Chem. Int. Ed. 56, 733–737. doi:10.1002/ANIE.201608292
Liao, C. C., Yu, H. P., Yang, S. C., Alalaiwe, A., Dai, Y. S., Liu, F. C., et al. (2021). Multifunctional Lipid-Based Nanocarriers with Antibacterial and Anti‐inflammatory Activities for Treating MRSA Bacteremia in Mice. J. Nanobiotechnology 19, 1–18. doi:10.1186/s12951-021-00789-5
Lin, C., Wong, B. C. K., Chen, H., Bian, Z., Zhang, G., Zhang, X., et al. (2017). Pulmonary Delivery of Triptolide-Loaded Liposomes Decorated with Anti-carbonic Anhydrase IX Antibody for Lung Cancer Therapy. Sci. Rep. 7, 1–12. doi:10.1038/s41598-017-00957-4
Liu, Q., Li, X., Bao, Y. S., Lu, J., Li, H., Huang, Z., et al. (2019). Chemical Synthesis and Functional Characterization of a New Class of Ceramide Analogues as Anti-cancer Agents. Bioorg. Med. Chem. 27, 1489–1496. doi:10.1016/j.bmc.2019.02.030
Liu, Y., Zhang, B., Xu, J., Wang, X., Tang, J., and Huang, J. (2021). Phase I Study of Liposomal Irinotecan (LY01610) in Patients with Advanced Esophageal Squamous Cell Carcinoma. Cancer Chemother. Pharmacol. 88, 403–414. doi:10.1007/s00280-021-04294-2
Lorentz, K. M., Kontos, S., Diaceri, G., Henry, H., and Hubbell, J. A. (2015). Engineered Binding to Erythrocytes Induces Immunological Tolerance to E. coli Asparaginase. Sci. Adv. 1, 1500112. doi:10.1126/sciadv.1500112
Lu, J., Jiang, F., Lu, A., and Zhang, G. (2016). Linkers Having a Crucial Role in Antibody–Drug Conjugates. Int. J. Mol. Sci. 17, 561. doi:10.3390/ijms17040561
Lungu, M., Neculae, A., Bunoiu, M., and Biris, C. (2015). Nanoparticles’ Promises and Risks: Characterization, Manipulation, and Potential Hazards to Humanity and the Environment. Nanoparticles’ Promises Risks Charact. Manip. Potential Hazards Humanit. Environ., 1–355. doi:10.1007/978-3-319-11728-7
Luo, G.-F., Chen, W.-H., Zeng, X., and Zhang, X.-Z. (2021). Cell Primitive-Based Biomimetic Functional Materials for Enhanced Cancer Therapy. Chem. Soc. Rev. 50, 945–985. doi:10.1039/D0CS00152J
Marek, M., Jarý, J., Valentová, O., and Vodrážka, Z. (1983). Immobilization of Glycoenzymes by Means of Their Glycosidic Components. Biotechnol. Lett. 5, 653–658. doi:10.1007/BF01386357
McCombs, J. R., and Owen, S. C. (2015). Antibody Drug Conjugates: Design and Selection of Linker, Payload and Conjugation Chemistry. AAPS J. 17, 339–351. doi:10.1208/s12248-014-9710-8
Méndez, Y., Chang, J., Humpierre, A. R., Zanuy, A., Garrido, R., Vasco, A. V., et al. (2018). Multicomponent Polysaccharide-Protein Bioconjugation in the Development of Antibacterial Glycoconjugate Vaccine Candidates. Chem. Sci. 9 (9), 2581–2588. doi:10.1039/c7sc05467j
Milton Harris, J., Martin, N. E., and Modi, M. (2001). Pegylation: A Novel Process for Modifying Pharmacokinetics. Clin. Pharmacokinet. 40, 539–551. doi:10.2165/00003088-200140070-00005
Mohan, T., Kleinschek, K. S., and Kargl, R. (2022). Polysaccharide Peptide Conjugates: Chemistry, Properties and Applications. CarbohydrPolym 280, 118875. doi:10.1016/J.CARBPOL.2021.118875
Morales, F. E., Garay, H. E., Muñoz, D. F., Augusto, Y. E., Otero-González, A. J., Reyes Acosta, O., et al. (2015). Aminocatalysis-mediated On-Resin Ugi Reactions: Application in the Solid-phase Synthesis of N -substituted and Tetrazolo Lipopeptides and Peptidosteroids. Org. Lett. doi:10.1021/acs.orglett.5b01147
Morejón, M. C., Laub, A., Kaluderović, G. N., Puentes, A. R., Hmedat, A. N., Otero-González, A. J., et al. (2017). A Multicomponent Macrocyclization Strategy to Natural Product-like Cyclic Lipopeptides: Synthesis and Anticancer Evaluation of Surfactin and Mycosubtilin Analogues. Org. Biomol. Chem. 15 (17), 3628–3637. doi:10.1039/c7ob00459a
Morejón, M. C., Laub, A., Westermann, B., Rivera, D. G., and Wessjohann, L. A. (2016). Solution- and Solid-phase Macrocyclization of Peptides by the Ugi-Smiles Multicomponent Reaction: Synthesis of N-Aryl-Bridged Cyclic Lipopeptides. Org. Lett. 18 (16), 4096–4099. doi:10.1021/acs.orglett.6b02001
Münter, R., Bak, M., Christensen, E., Kempen, P. J., Larsen, J. B., Kristensen, K., et al. (2022). Mechanisms of Selective Monocyte Targeting by Liposomes Functionalized with a Cationic, Arginine-Rich Lipopeptide. Acta Biomater. doi:10.1016/J.ACTBIO.2022.03.029
Murciano, J. C., Higazi, A. A. R., Cines, D. B., and Muzykantov, V. R. (2009). Soluble Urokinase Receptor Conjugated to Carrier Red Blood Cells Binds Latent Pro-urokinase and Alters its Functional Profile. J. Control Release 139, 190–196. doi:10.1016/j.jconrel.2009.07.003
Muthu, M. S., Kutty, R., Luo, Z., Xie, J., and Feng, S. S. (2015). Theranostic Vitamin E TPGS Micelles of Transferrin Conjugation for Targeted Co-delivery of Docetaxel and Ultra Bright Gold Nanoclusters. Biomaterials 39, 234–248. doi:10.1016/j.biomaterials.2014.11.008
Nanna, A. R., Li, X., Walseng, E., Pedzisa, L., Goydel, R. S., Hymel, D., et al. (2017). Harnessing a Catalytic Lysine Residue for the One-step Preparation of Homogeneous Antibody-Drug Conjugates. Nat. Commun. 8 (1), 1112. doi:10.1038/s41467-017-01257-1
NCT02195180 (2014). Efficacy and Safety of L-Asparaginase Encapsulated in RBC Combined with Gemcitabine or FOLFOX in 2nd Line for Progressive Metastatic Pancreatic Carcinoma. Available at: https://clinicaltrials.gov/show/nct02195180.
NCT03267030 (2021) Asparaginase Encapsulated in Erythrocytes for Patients with ALL and Hypersensitivity to PEG-Asparaginase - Full Text View - ClinicalTrials.Gov. Available at: https://clinicaltrials.gov/ct2/show/NCT03267030.
Nikezić, A. V. V., Bondžić, A. M., and Vasić, V. M. (2020). Drug Delivery Systems Based on Nanoparticles and Related Nanostructures. Eur. J. Pharm. Sci. 151, 105412. doi:10.1016/j.ejps.2020.105412
Ning, Y.-M., He, K., Dagher, R., Sridhara, R., Farrell, A. T., Justice, R., et al. (2007). Liposomal Doxorubicin in Combination with Bortezomib for Relapsed or Refractory Multiple Myeloma. Oncology (Williston Park) 21, 1503–1508. discussion 1511, 1513, 1516 passim.
Ojediran, J. O., and Raji, A. O. (2010). Chapter 2 Flow and Functional Models for Rheological Properties of Fluid Foods. Int. Food Res. J. 17, 1095–1106. doi:10.1007/978-1-4614-9230-6
Palko-Łabuz, A., Gliszczyńska, A., Skonieczna, M., Poła, A., Wesołowska, O., and Środa-Pomianek, K. (2021). Conjugation with Phospholipids as a Modification Increasing Anticancer Activity of Phenolic Acids in Metastatic Melanoma—In Vitro and In Silico Studies. Int. J. Mol. Sci. 22, 8397. doi:10.3390/ijms22168397
Pando, O., Stark, S., Denkert, A., Porzel, A., Preusentanz, R., and Wessjohann, L. A. (2011). The Multiple Multicomponent Approach to Natural Product Mimics: Tubugis, N-Substituted Anticancer Peptides with Picomolar Activity. J. Am. Chem. Soc. 133 (20), 7692–7695. doi:10.1021/ja2022027
Papadopoulos, G. N., and Kokotos, C. G. (2016). One-Pot Amide Bond Formation from Aldehydes and Amines via a Photoorganocatalytic Activation of Aldehydes. J. Org. Chem. 81, 7023–7028. doi:10.1021/acs.joc.6b00488
Parslow, A. C., Parakh, S., Lee, F. T., Gan, H. K., and Scott, A. M. (2016). Antibody-drug Conjugates for Cancer Therapy. Biomedicines 4, 17. doi:10.3390/biomedicines4030014
Pérez-Labrada, K., Brouard, I., Méndez, I., and Rivera, D. G. (2012). Multicomponent Synthesis of Ugi-type Ceramide Analogues and Neoglycolipids from Lipidic Isocyanides. J. Org. Chem. 77 (10), 4660–4670. doi:10.1021/jo300462m
Prasher, P., Sharma, M., Mudila, H., Gupta, G., Sharma, A. K., Kumar, D., et al. (2020). Emerging Trends in Clinical Implications of Bio-Conjugated Silver Nanoparticles in Drug Delivery. Colloids Interf. Sci. Commun. 35, 100244. doi:10.1016/j.colcom.2020.100244
Ramos Tomillero, I. (2016). Linkers for Bioconjugation PhD thesis. Available at: http://hdl.handle.net/10803/399915 (Accessed May 2, 2022).
Rivera, D. G., León, F., Concepción, O., Morales, F. E., and Wessjohann, L. A. (2013). A Multiple Multicomponent Approach to Chimeric Peptide-Peptoid Podands. Chem. - A. Eur. J. 19 (20), 6417–6428. doi:10.1002/chem.201201591
Rivera, D. G., Pando, O., and Coll, F. (2006). Synthesis of Peptidomimetic-Spirostane Hybrids via Ugi Reaction: a Versatile Approach for the Formation of Peptide-Steroid Conjugates. Tetrahedron 62 (35), 8327–8334. doi:10.1016/j.tet.2006.06.050
Rivera, D. G., Pérez-Labrada, K., Lambert, L., Dörner, S., Westermann, B., and Wessjohann, L. A. (2012). Carbohydrate-steroid Conjugation by Ugi Reaction: One-Pot Synthesis of Triple Sugar/pseudo-Peptide/spirostane Hybrids. Carbohydr. Res. 359, 102–110. doi:10.1016/j.carres.2012.05.003
Rossi, L., Pierigè, F., Aliano, M. P., and Magnani, M. (2020). Ongoing Developments and Clinical Progress in Drug-Loaded Red Blood Cell Technologies. BioDrugs 34, 265–272. doi:10.1007/s40259-020-00415-0
Samavarchi-Tehrani, P., Samson, R., and Gingras, A.-C. (2020). Proximity Dependent Biotinylation: Key Enzymes and Adaptation to Proteomics Approaches. Mol. Cel. Proteomics 19, 757–773. doi:10.1074/MCP.R120.001941
Saw, W. S., Anasamy, T., Foo, Y. Y., Kwa, Y. C., Kue, C. S., Yeong, C. H., et al. (2021). Delivery of Nanoconstructs in Cancer Therapy: Challenges and Therapeutic Opportunities. Adv. Ther. 4, 2000206. doi:10.1002/adtp.202000206
Sen, S., Perrin, M. W., Sedgwick, A. C., Lynch, V. M., Sessler, J. L., and Arambula, J. F. (2021). Covalent and Non-covalent Albumin Binding of Au(i) Bis-NHCsviapost-Synthetic Amide Modification. Chem. Sci. 12, 7547–7553. doi:10.1039/d1sc01055g
Sharma, S., Kaur, G., and Handa, S. (2021). Insights into Fast Amide Couplings in Aqueous Nanomicelles. Org. Process. Res. Dev. 25, 1960–1965. doi:10.1021/acs.oprd.1c00203
Singla, P., and Salunke, D. B. (2020). Recent Advances in Steroid Amino Acid Conjugates: Old Scaffolds with New Dimensions. Eur. J. Med. Chem. 187, 111909. doi:10.1016/j.ejmech.2019.111909
Sleep, D. (2015). Albumin and its Application in Drug Delivery. Expert Opin. Drug Deliv. 12, 793–812. doi:10.1517/17425247.2015.993313
Sonali, , Singh, R. P. S., Singh, N., Sharma, G., Vijayakumar, M. R., Koch, B., et al. (2016). Transferrin Liposomes of Docetaxel for Brain-Targeted Cancer Applications: Formulation and Brain Theranostics. Drug Deliv. 23, 1261–1271. doi:10.3109/10717544.2016.1162878
Spanedda, M. V., and Bourel-Bonnet, L. (2021). Cyclic Anhydrides as Powerful Tools for Bioconjugation and Smart Delivery. Bioconjug. Chem. 32, 482–496. doi:10.1021/acs.bioconjchem.1c00023
Taguchi, K., Lu, H., Jiang, Y., Hung, T. T., and Stenzel, M. H. (2018). Safety of Nanoparticles Based on Albumin-Polymer Conjugates as a Carrier of Nucleotides for Pancreatic Cancer Therapy. J. Mater. Chem. B 6, 6278–6287. doi:10.1039/C8TB01613E
Tang, L., Li, J., Zhao, Q., Pan, T., Zhong, H., and Wang, W. (2021). Advanced and Innovative Nano-Systems for Anticancer Targeted Drug Delivery. Pharmaceutics 13, 1151. doi:10.3390/pharmaceutics13081151
Tanino, T., Ichikawa, S., Al-Dabbagh, B., Bouhss, A., Oyama, H., and Matsuda, A. (2010). Synthesis and Biological Evaluation of Muraymycin Analogues Active against Anti-drug-resistant Bacteria. ACS Med. Chem. Lett. doi:10.1021/ml100057z
Thery, J. C., Spano, J. P., Azria, D., Raymond, E., and Penault Llorca, F. (2014). Resistance to Human Epidermal Growth Factor Receptor Type 2-targeted Therapies. Eur. J. Cancer 50, 892–901. doi:10.1016/j.ejca.2014.01.003
Tivdak Fda. Initial U.S. Approval: 2021 (2021). TIVDAK TM (Tisotumab Vedotin-Tftv) for Injection, for Intravenous Use Initial U.S. Approval: 2021. Ref. ID 4859741. Available at: https://www.accessdata.fda.gov/drugsatfda_docs/label/2021/761208s000lbl.pdf (Accessed February 25, 2022).
Tolaney, S. M., Do, K. T., Eder, J. P., LoRusso, P. M., Weekes, C. D., Chandarlapaty, S., et al. (2021). A Phase I Study of DLYE5953A, an Anti-ly6e Antibody Covalently Linked to Monomethyl Auristatin E, in Patients with Refractory Solid Tumors. Clin. Cancer Res. 26, 5588–5597. doi:10.1158/1078-0432.CCR-20-1067
Tong, J. T. W., Harris, P. W. R., Brimble, M. A., and Kavianinia, I. (2021). ZYNLONTATM (Loncastuximab Tesirine-Lpyl) for Injection, for Intravenous Use Initial U.S. Approval: 2021. Mol 26, 5847. Page 5847 26. doi:10.3390/MOLECULES26195847
Torres-Pérez, S. A., Torres-Pérez, C. E., Pedraza-Escalona, M., Pérez-Tapia, S. M., and Ramón-Gallegos, E. (2020). Glycosylated Nanoparticles for Cancer-Targeted Drug Delivery. Front. Oncol. 10, 2667. doi:10.3389/FONC.2020.605037/BIBTEX
Tsuchikama, K., and An, Z. (2018). Antibody-drug Conjugates: Recent Advances in Conjugation and Linker Chemistries. Protein Cell 9, 33–46. doi:10.1007/s13238-016-0323-0
Tsukigawa, K., Imoto, S., Yamasaki, K., Nishi, K., Tsutsumi, T., Yokoyama, S., et al. (2020). Synthesis and In Vitro Assessment of pH-Sensitive Human Serum Albumin Conjugates of Pirarubicin. Pharm 202114, 22. Page 22 14. doi:10.3390/PH14010022
United States Environmental Protection Agency (2021). Research on Nanomaterials | US EPA. US EPA. Available at: https://www.epa.gov/chemical-research/research-nanomaterials (Accessed May 2, 2022).
U.S. Food and Drug Administration (FDA) (2013). FDA Approves Kadcyla for Breast Cancer. Cancer Discov. 3, 366–2366. doi:10.1158/2159-8290.cd-nb2013-030
U.S. Food and Drug Administration (FDA) (2020). Novel Drug Approvals for 2020. U.S. Food Drug Adm. Available at: https://www.fda.gov/drugs/new-drugs-fda-cders-new-molecular-entities-and-new-therapeutic-biological-products/novel-drug-approvals-2020.
U.S. Food and Drug Admins (1995). DOXIL® (doxorubicin HCl liposome injection) for intravenous infusion. Available at: https://www.fda.gov/medwat (Accessed May 2, 2022).
U.S. Food and Drug Admins (2010). KRYSTEXXATM® (Pegloticase) Injection, for Intravenous Infusion. Available at: https://www.fda.gov/media/79472/download (Accessed May 2, 2022).
U.S. Food and Drug Admins (1994). ONCASPAR (Pegaspargase) Injection, for Intramuscular or Intravenous Use. Available at: https://www.accessdata.fda.gov/drugsatfda_docs/label/2021/103411s5201lbl.pdf (Accessed May 2, 2022).
U.S. Food and Drug Admins (2015). PLEGRIDY (Peginterferon Beta-1a) Injection, for Subcutaneous Injection. Available at: https://www.accessdata.fda.gov/drugsatfda_docs/label/2014/125499lbl.pdf (Accessed May 2, 2022).
U.S. Food and Drug Admins (2012a). Temporary Importation of Lipodox (Doxorubicin HCL Liposomal Injection). Available at: http://www.fda.gov/downloads/Drugs/DrugSafety/DrugShortages/UCM292634.pdf (Accessed May 2, 2022).
U.S. Food and Drug Adminstration (FDA) (2012b). ADCETRIS® (Brentuximab Vedotin) for injection, for intravenous use. Available at: https://www.accessdata.fda.gov/drugsatfda_docs/label/2014/125388_s056s078lbl.pdf (Accessed May 2, 2022).
U.S. Food and Drug Adminstration (FDA) (2017a). BESPONSA (Inotuzumab Ozogamicin). Available at: https://www.accessdata.fda.gov/drugsatfda_docs/label/2017/761040s000lbl.pdf (Accessed May 2, 2022).
U.S. Food and Drug Adminstration (FDA) (2017b). MYLOTARGT (Gemtuzumab Ozogamicin) for Injection. Available at: https://www.accessdata.fda.gov/drugsatfda_docs/label/2017/761060lbl.pdf (Accessed May 2, 2022).
Vega-Vásquez, P., Mosier, N. S., and Irudayaraj, J. (2020). Nanoscale Drug Delivery Systems: From Medicine to Agriculture. Front. Bioeng. Biotechnol. 8, 79. doi:10.3389/FBIOE.2020.00079/BIBTEX
Villa, C. H., Cines, D. B, Siegel, D. L., and Muzykantov, V. (2017). Erythrocytes as Carriers for Drug Delivery in Blood Transfusion and beyond. Transfus. Med. Rev. 31, 26–35. doi:10.1016/J.TMRV.2016.08.004
Volpi, S., Cancelli, U., Neri, M., and Corradini, R. (2021). Multifunctional Delivery Systems for Peptide Nucleic Acids. Pharmaceuticals 14, 1–31. doi:10.3390/ph14010014
Walther, R., and Zelikin, A. N. (2021). Chemical (Neo)glycosylation of Biological Drugs. Adv. Drug Deliv. Rev. 171, 62–76. doi:10.1016/J.ADDR.2021.01.021
Wang, C., Sun, X., Cheng, L., Yin, S., Yang, G., Li, Y., et al. (2014). Multifunctional Theranostic Red Blood Cells for Magnetic-Field-Enhanced In Vivo Combination Therapy of Cancer. Adv. Mater. 26, 4794–4802. doi:10.1002/ADMA.201400158
Wang, K., Shang, F., Chen, D., Cao, T., Wang, X., Jiao, J., et al. (2021). Protein Liposomes-Mediated Targeted Acetylcholinesterase Gene Delivery for Effective Liver Cancer Therapy. J. Nanobiotechnology 19, 1–15. doi:10.1186/s12951-021-00777-9
Weisel, J. W., and Litvinov, R. I. (2019). Red Blood Cells: The Forgotten Player in Hemostasis and Thrombosis J. Thromb. Haemost. 17, 271–282. doi:10.1111/JTH.14360
Werengowska-Ciećwierz, K., Wis̈niewski, M., Terzyk, A. P., and Furmaniak, S. (2015). The Chemistry of Bioconjugation in Nanoparticles-Based Drug Delivery System. Adv. Condens. Matter Phys. 2015, 1–27. doi:10.1155/2015/198175
Wessjohann, L. A., Morejón, M. C., Ojeda, G. M., Rhoden, C. R. B., and Rivera, D. G. (2016). Applications of Convertible Isonitriles in the Ligation and Macrocyclization of Multicomponent Reaction-Derived Peptides and Depsipeptides. J. Org. Chem. doi:10.1021/acs.joc.6b01150
Whalley, N. A., Walters, S., and Hammond, K. (2018). “Molecular Cell Biology,” in Molecular Medicine for Clinicians (W. H. & C. Freeman), 37–49. doi:10.18772/22008014655.9
Wu, B., Melhem, M., Subramanian, R., Chen, P., Jaramilla Sloey, B., Fouqueray, B., et al. (2018). Clinical Pharmacokinetics and Pharmacodynamics of Etelcalcetide, a Novel Calcimimetic for Treatment of Secondary Hyperparathyroidism in Patients with Chronic Kidney Disease on Hemodialysis. J. Clin. Pharmacol. 58, 717–726. doi:10.1002/jcph.1090
Xu, Y. D., Tian, L., Lai, R. Y., Li, Z., Procházková, E., Ho, J., et al. (2022). Development of an Albumin-Polymer Bioconjugate via Covalent Conjugation and Supramolecular Interactions. Bioconjug. Chem. 33, 321–332. doi:10.1021/ACS.BIOCONJCHEM.1C00536/SUPPL_FILE/BC1C00536_SI_001.PDF
Xue, Y., Bai, H., Peng, B., Fang, B., Baell, J., Li, L., et al. (2021). Stimulus-cleavable Chemistry in the Field of Controlled Drug Delivery. Chem. Soc. Rev. 50, 4872–4931. doi:10.1039/D0CS01061H
Yamazaki, C. M., Yamaguchi, A., Anami, Y., Xiong, W., Otani, Y., Lee, J., et al. (2021). Antibody-drug Conjugates with Dual Payloads for Combating Breast Tumor Heterogeneity and Drug Resistance. Nat. Commun. 12, 1–13. doi:10.1038/s41467-021-23793-7
Yang, W., Lee, J. C., Chen, M. H., Zhang, Z. Y., Bai, X. M., Yin, S. S., et al. (2019). Thermosensitive Liposomal Doxorubicin Plus Radiofrequency Ablation Increased Tumor Destruction and Improved Survival in Patients with Medium and Large Hepatocellular Carcinoma: A Randomized, Double-Blinded, Dummy-Controlled Clinical Trial in a Single Cent. J. Cancer Res. Ther. 15, 773–783. doi:10.4103/jcrt.JCRT_801_18
Yao, W., Liu, C., Wang, N., Zhou, H., Chen, H., and Qiao, W. (2021). An MRI-Guided Targeting Dual-Responsive Drug Delivery System for Liver Cancer Therapy. J. Colloid Interf. Sci. 603, 783–798. doi:10.1016/j.jcis.2021.06.151
Zakharov, Y. N., Raevski, I. P., Eknadiosians, E. I., Pinskaya, A. N., Pustovaya, L. E., and Borodin, V. Z. (2000). Pyroelectric Properties and Domain Structure of Modified lead Ferroniobate-Based Ceramics. Ferroelectrics 247, 47–52. doi:10.1080/00150190008214939
Zhang, L., Wang, Z., Wang, Z., Luo, F., Guan, M., Xu, M., et al. (2021a). A Simple and Efficient Method to Generate Dual Site-specific Conjugation ADCs with Cysteine Residue and an Unnatural Amino Acid. Bioconjug. Chem. 32, 1094–1104. doi:10.1021/acs.bioconjchem.1c00134
Zhang, X., Luo, M., Dastagir, S. R., Nixon, M., Khamhoung, A., Schmidt, A., et al. (2021b). Engineered Red Blood Cells as an Off-The-Shelf Allogeneic Anti-tumor Therapeutic. Nat. Commun. 12, 1–14. doi:10.1038/s41467-021-22898-3
Zhang, Y.-T., He, K.-J., Zhang, J.-B., Ma, Q.-H., Wang, F., and Liu, C.-F. (2021c2021). Advances in Intranasal Application of Stem Cells in the Treatment of central Nervous System Diseases. Stem Cel Res. Ther. 121 12, 1–10. doi:10.1186/S13287-021-02274-0
Zhang, Y., Xia, Q., Wu, T., He, Z., Li, Y., Li, Z., et al. (2021d). A Novel Multi-Functionalized Multicellular Nanodelivery System for Non-small Cell Lung Cancer Photochemotherapy. J. Nanobiotechnology 19, 1–23. doi:10.1186/s12951-021-00977-3
Ziegler, T., Gerling, S., and Lang, M. (2000). Preparation of Bioconjugates through an Ugi Reaction. Angew. Chem. - Int. Ed. doi:10.1002/1521-3773(20000616)39:12<2109::aid-anie2109>3.0.co;2-9
Zielińska, A., Szalata, M., Gorczyński, A., Karczewski, J., Eder, P., Severino, P., et al. (2021). Cancer Nanopharmaceuticals: Physicochemical Characterization and In Vitro/In Vivo Applications. Cancers (Basel) 13, 1896. doi:10.3390/cancers13081896
Znabet, A., Polak, M. M., Janssen, E., De Kanter, F. J. J., Turner, N. J., Orru, R. V. A., et al. (2010). A Highly Efficient Synthesis of Telaprevir by Strategic Use of Biocatalysis and Multicomponent Reactions. Chem. Commun. 46 (42), 7918. doi:10.1039/c0cc02823a
Zynlonta Fda. Initial U.S. Approval: 2021 (2021). ZYNLONTATM (Loncastuximab Tesirine-Lpyl) for Injection, for Intravenous Use. Ref. ID 4784313. Available at: https://www.accessdata.fda.gov/drugsatfda_docs/label/2021/761196s000lbl.pdf (Accessed February 25, 2022).
Keywords: drug delivery systems, biomolecules, carriers, covalent bioconjugation, linkers
Citation: Eras A, Castillo D, Suárez M, Vispo NS, Albericio F and Rodriguez H (2022) Chemical Conjugation in Drug Delivery Systems. Front. Chem. 10:889083. doi: 10.3389/fchem.2022.889083
Received: 03 March 2022; Accepted: 11 April 2022;
Published: 26 May 2022.
Edited by:
Guigen Li, Texas Tech University, United StatesReviewed by:
Kazuaki Taguchi, Keio University, JapanDong-Sheng Pei, Xuzhou Medical University, China
Copyright © 2022 Eras, Castillo, Suárez, Vispo, Albericio and Rodriguez. This is an open-access article distributed under the terms of the Creative Commons Attribution License (CC BY). The use, distribution or reproduction in other forums is permitted, provided the original author(s) and the copyright owner(s) are credited and that the original publication in this journal is cited, in accordance with accepted academic practice. No use, distribution or reproduction is permitted which does not comply with these terms.
*Correspondence: Nelson Santiago Vispo, bnZpc3BvQHlhY2hheXRlY2guZWR1LmVj; Fernando Albericio, QWxiZXJpY2lvQHVrem4uYWMuemE=; Hortensia Rodriguez, aG1yb2RyaWd1ZXpAeWFjaGF5dGVjaC5lZHUuZWM=
†These authors have contributed equally to this work and share the last authorship